- 1Emeritus Professor, The Fletcher School and Co-director Global Development and Environment Institute, Tufts University, Medford, MA, United States
- 2Vernon Roosa Professor of Applied Science, Trinity College, Hartford, CT, United States
- 3Charles Bullard Fellow in Forest Research, Harvard Forest, Petersham, MA, United States
- 4Senior Ecologist, Highstead Foundation, Redding, CT, United States
Climate change and loss of biodiversity are widely recognized as the foremost environmental challenges of our time. Forests annually sequester large quantities of atmospheric carbon dioxide (CO2), and store carbon above and below ground for long periods of time. Intact forests—largely free from human intervention except primarily for trails and hazard removals—are the most carbon-dense and biodiverse terrestrial ecosystems, with additional benefits to society and the economy. Internationally, focus has been on preventing loss of tropical forests, yet U.S. temperate and boreal forests remove sufficient atmospheric CO2 to reduce national annual net emissions by 11%. U.S. forests have the potential for much more rapid atmospheric CO2 removal rates and biological carbon sequestration by intact and/or older forests. The recent 1.5 Degree Warming Report by the Intergovernmental Panel on Climate Change identifies reforestation and afforestation as important strategies to increase negative emissions, but they face significant challenges: afforestation requires an enormous amount of additional land, and neither strategy can remove sufficient carbon by growing young trees during the critical next decade(s). In contrast, growing existing forests intact to their ecological potential—termed proforestation—is a more effective, immediate, and low-cost approach that could be mobilized across suitable forests of all types. Proforestation serves the greatest public good by maximizing co-benefits such as nature-based biological carbon sequestration and unparalleled ecosystem services such as biodiversity enhancement, water and air quality, flood and erosion control, public health benefits, low impact recreation, and scenic beauty.
Introduction
Life on Earth as we know it faces unprecedented, intensifying, and urgent imperatives. The two most urgent challenges are (1) mitigating and adapting to climate change (Intergovernmental Panel on Climate Change, 2013, 2014, 2018), and (2) preventing the loss of biodiversity (Wilson, 2016; IPBES, 2019). These are three of the Sustainable Development Goals, Climate, Life on Land and Life under Water (Division for Sustainable Development Goals, 2015), and significant international resources are being expended to address these crises and limit negative impacts on economies, societies and biodiverse natural communities. The recent 1.5 Degree Warming Report of the Intergovernmental Panel on Climate Change (2018) was dire and direct, stating the need for “rapid, far-reaching and unprecedented changes in all aspects of society.” We find that growing additional existing forests as intact ecosystems, termed proforestation, is a low-cost approach for immediately increasing atmospheric carbon sequestration to achieve a stable atmospheric carbon dioxide concentration that reduces climate risk. Proforestation also provides long-term benefits for biodiversity, scientific inquiry, climate resilience, and human benefits. This approach could be mobilized across all forest types.
Forests are essential for carbon dioxide removal (CDR), and the CDR rate needs to increase rapidly to remain within the 1.5 or 2.0°C range (Intergovernmental Panel on Climate Change, 2018) specified by the Paris Climate Agreement (2015). Growing existing forests to their biological carbon sequestration potential optimizes CDR while limiting climate change and protecting biodiversity, air, land, and water. Natural forests are by far the most effective (Lewis et al., 2019). Technologies for direct CDR from the atmosphere, and bioenergy with carbon capture and storage (BECCS), are far from being technologically ready or economically viable (Anderson and Peters, 2016). Furthermore, the land area required to supply BECCS power plants with tree plantations is 7.7 million km2, or approximately the size of Australia (Intergovernmental Panel on Climate Change, 2018). Managed plantations that are harvested periodically store far less carbon because trees are maintained at a young age and size (Harmon et al., 1990; Sterman et al., 2018). Furthermore, plantations are often monocultures, and sequester less carbon more slowly than intact forests with greater tree species diversity and higher rates of biological carbon sequestration (Liu et al., 2018). Recent research in the tropics shows that natural forests hold 40 times more carbon than plantations (Lewis et al., 2019).
Alternative forest-based CDR methods include afforestation (planting new forests) and reforestation (replacing forests on deforested or recently harvested lands). Afforestation and reforestation can contribute to CDR, but newly planted forests require many decades to a century before they sequester carbon dioxide in substantial quantities. A recent National Academy study titled Negative Emissions Technologies and Reliable Sequestration: A Research Agenda discusses afforestation and reforestation and finds their contribution to be modest (National Academies of Sciences, 2019). The study also examines changes in conventional forest management, but neglects proforestation as a strategy for increasing carbon sequestration. Furthermore, afforestation to meet climate goals requires an estimated 10 million km2–an area slightly larger than Canada (Intergovernmental Panel on Climate Change, 2018). The massive land areas required for afforestation and BECCS (noted above) compete with food production, urban space and other uses (Searchinger et al., 2009; Sterman et al., 2018). More importantly, neither of these two practices is as effective quantitatively as proforestation in the next several decades when it is needed most. For example, Law et al. (2018) reported that extending harvest cycles and reducing cutting on public lands had a larger effect than either afforestation or reforestation on increasing carbon stored in forests in the Northwest United States. In other regions such as New England (discussed below), longer harvest cycles and proforestation are likely to be even more effective. Our assessment on the climate and biodiversity value of natural forests and proforestation aligns directly with a recent report that pinpointed “stable forests” – those not already significantly disturbed or at significant risk – as playing an outsized role as a climate solution due to their carbon sequestration and storage capabilities (Funk et al., 2019).
Globally, terrestrial ecosystems currently remove an amount of atmospheric carbon equal to one-third of what humans emit from burning fossil fuels, which is about 9.4 GtC/y (109 metric tons carbon per year). Forests are responsible for the largest share of the removal. Land use changes, i.e., conversion of forest to agriculture, urban centers and transportation corridors, emit ~1.3 GtC/y (Le Quéré et al., 2018). However, forests' potential carbon sequestration and additional ecosystem services, such as high biodiversity unique to intact older forests, are also being degraded significantly by current management practices (Foley et al., 2005; Watson et al., 2018). Houghton and Nassikas (2018) estimated that the “current gross carbon sink in forests recovering from harvests and abandoned agriculture to be −4.4 GtC/y, globally.” This is approximately the current gap between anthropogenic emissions and biological carbon and ocean sequestration rates by natural systems. If deforestation were halted, and secondary forests were allowed to continue growing, they would sequester −120 GtC between 2016 and 2100 or ~12 years of current global fossil carbon emissions (Houghton and Nassikas, 2018). Northeast secondary forests have the potential to increase biological carbon sequestration between 2.3 and 4.2-fold (Keeton et al., 2011).
Existing proposals for “Natural Climate Solutions” do not consider explicitly the potential of proforestation (Griscom et al., 2017; Fargione et al., 2018). However, based on a growing body of scientific research, we conclude that protecting and stewarding intact diverse forests and practicing proforestation as a purposeful public policy on a large scale is a highly effective strategy for mitigating the dual crises in climate and biodiversity and ultimately serving the “greatest good” in the United States and the rest of the world. Table 1 summarizes some of the key literature supporting this point.
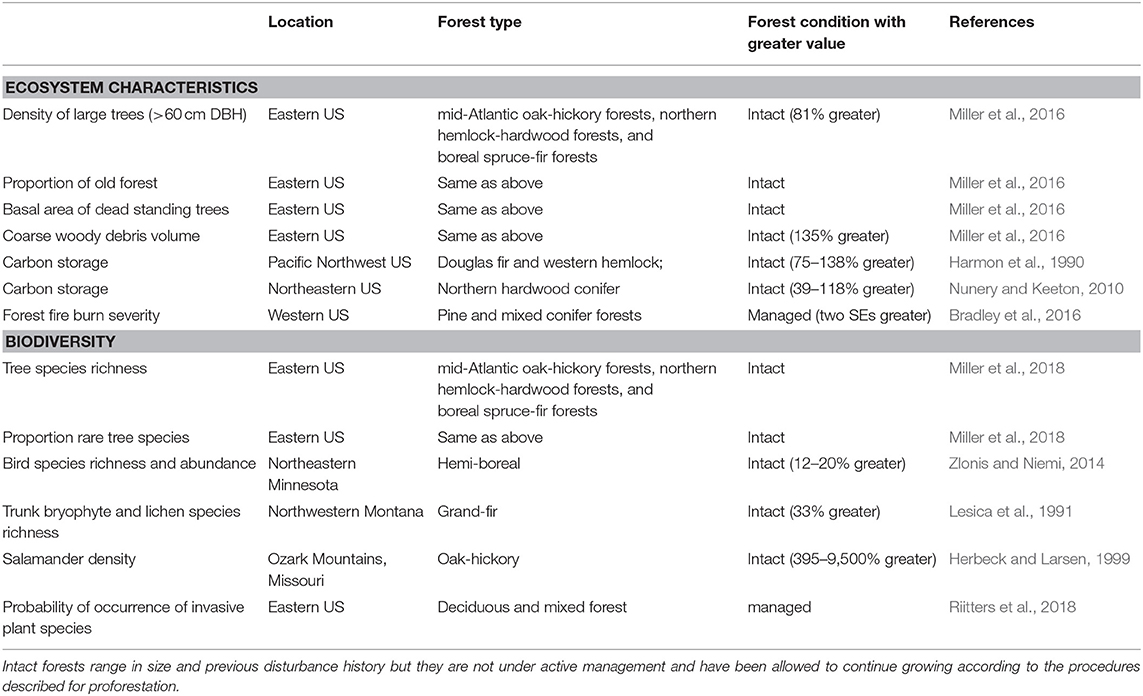
Table 1. Comparison of climate and biodiversity benefits of intact (either old-growth forest or younger forest managed as Gap 1 or Gap 2, and thus protected from logging and other resource extraction) and traditionally managed forests for multiple forest types in the United States.
A Small Fraction of U.S. Forests is Managed to Remain Intact
Today, <20% of the world's forests remain intact (i.e., largely free from logging and other forms of extraction and development). Intact forests are largely tropical forests or boreal forests in Canada and Russia (Watson et al., 2018). In the U.S.—a global pioneer in national parks and wildlife preserves—the percentage of intact forest in the contiguous 48 states is only an estimated 6–7% of total forest area (Oswalt et al., 2014), with a higher proportion in the West and a lower proportion in the East. Setting aside a large portion of U.S. forest in Inventoried Roadless Areas (IRAs) was groundbreaking yet only represents 7% of total forest area in the lower 48 states—and, ironically, management of some IRAs allows timber harvest and road building (Williams, 2000), a scenario happening currently in the Tongass National Forest in Alaska (Koberstein and Applegate, 2018). These scant percentages worldwide and particularly in the U.S. are insufficient to address pressing national and global issues such as rising CO2 levels, flooding, and biodiversity loss, as well as provide suitable locations for recreation and associated public health benefits (Cordell, 2012; Watson et al., 2018). In heavily populated and heavily forested sub-regions in the Eastern U.S., such as New England, the total area dedicated as intact (i.e., primary management is for trails and hazard removals) is even more scarce, comprising only ~3% of land area. Just 2% of the region is legally protected from logging and other resource extraction (Figure 1). A large portion of forest managed currently as intact or “reserved forest” – and thus functioning as “stable forest” (Funk et al., 2019) – is designated solely by administrative regulations that can be altered at any time.
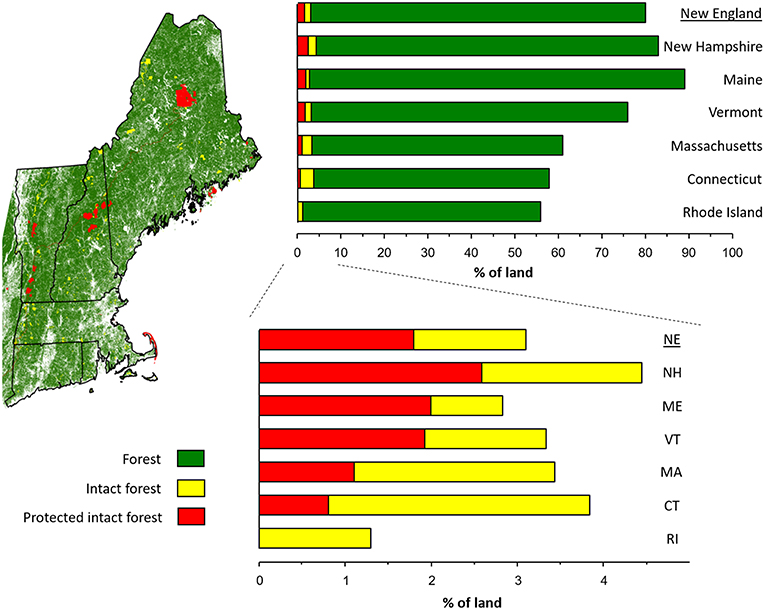
Figure 1. Distribution of forest cover and intact “wildland” forest across six New England states. At left, map of overall forest cover (green) vs. forest protected legally (red) or managed currently (yellow) as intact in New England. At right, regional and state specific % forest cover (green), % managed as intact Gap 1 (limited intervention other than trails and hazard removals) but not protected legally (yellow), and % legally protected as intact forest (red, designated U.S Geological Survey (USGS) Gap 1 or Gap 2 and primarily federal and state wilderness areas, and certain national parks). Adapted and compiled from National Conservation Easement Database (2014); United States Geological Survey (2019a,b), and the University of Montana (2019). USGS Gap level 1 or 2 lands receive the highest level of protection from logging and other resource extraction and generally correspond with IUCN protected categories 1a, 1b, and II (https://gapanalysis.usgs.gov/blog/iucn-definitions/).
Intact forests in the U.S. include federal wilderness areas and national parks, some state parks, and some privately-owned holdings and conservation trust lands. Recent studies reveal that intact forests in national parks tend to be older and have larger trees than nearby forests that are not protected from logging (Miller et al., 2016; Table 1). Scaling up protection of intact forests and designating and significantly expanding reserved forest areas are public policy imperatives that are compatible with public access and with the country's use of forest products. Identifying suitable forest as intact (for carbon sequestration, native biodiversity, ecosystem function, etc.) can spawn new jobs and industries in forest monitoring, tourism and recreation, as well as create more viable local economies based on wood reuse and recycling. Public lands with significant biodiversity and proforestation potential also provide wildlife corridors for climate migration and resilience for many species.
Proforestation Increases Biological Carbon Sequestration and Long-Term Storage in U.S. Forests
Net forest carbon reflects the dynamic between gains and losses. Carbon is lost from forests in several ways: damage from natural disturbances including insects and pathogens (“pests”), fire, drought and wind; forest conversion to development or other non-forest land; and forest harvest/management. Together, fires, drought, wind, and pests account for ~12% of the carbon lost in the U.S.; forest conversion accounts for ~3% of carbon loss; and forest harvesting accounts for 85% of the carbon lost from forests each year (Harris et al., 2016). Forests in the Southern US have the highest percentage of carbon lost to timber harvest (92%) whereas the Western US is notably lower (66%) because of the greater contribution of fires to carbon removal. The Northern U.S. is roughly equivalent to the national average at 86% (Harris et al., 2016).
Proforestation produces natural forests as maximal carbon sinks of diverse species (while supporting and accruing additional benefits of intact forests) and can reduce significantly and immediately the amount of forest carbon lost to non-essential management. Because existing trees are already growing, storing carbon, and sequestering more carbon more rapidly than newly planted and young trees (Harmon et al., 1990; Stephenson et al., 2014; Law et al., 2018; Leverett and Moomaw, in preparation), proforestation is a near-term approach to sequestering additional atmospheric carbon: a significant increase in “negative emissions” is urgently needed to meet temperature limitation goals.
The carbon significance of proforestation is demonstrated in multiple ways in larger trees and older forests. For example, a study of 48 undisturbed primary or mature secondary forest plots worldwide found, on average, that the largest 1% of trees [considering all stems ≥1 cm in diameter at breast height (DBH)] accounted for half of above ground living biomass (The largest 1% accounted for ~30% of the biomass in U.S. forests due to larger average size and fewer stems compared to the tropics) (Lutz et al., 2018). Each year a single tree that is 100 cm in diameter adds the equivalent biomass of an entire 10–20 cm diameter tree, further underscoring the role of large trees (Stephenson et al., 2014). Intact forests also may sequester half or more of their carbon as organic soil carbon or in standing and fallen trees that eventually decay and add to soil carbon (Keith et al., 2009). Some older forests continue to sequester additional soil organic carbon (Zhou et al., 2006) and older forests bind soil organic matter more tightly than younger ones (Lacroix et al., 2016).
If current management practices continue, the world's forests will only achieve half of their biological carbon sequestration potential (Erb et al., 2018); intensifying current management practices will only decrease living biomass carbon and increase soil carbon loss. Forests in temperate zones such as in the Eastern U.S. have a particularly high untapped capacity for carbon storage and sequestration because of high growth and low decay rates (Keith et al., 2009) and because of recent recovery from an extensive history of timber harvesting and land conversion for agriculture in the 18th, 19th, and early 20th centuries (Pan et al., 2011; Duveneck and Thompson, 2019). In New England, median forest age is about 75 years of age (United States Forest Service, 2019), which is only about 25–35% of the lifespan of many of the common tree species in these forests (Thompson et al., 2011). Much of Maine's forests have been harvested continuously for 200 years and have a carbon density less than one-third of the forests of Southern Vermont and New Hampshire, Northwestern Connecticut and Western Massachusetts—a region that has not been significantly harvested over the past 75–150 years (National Council for Air Stream Improvement, 2019). Western Massachusetts in particular has a significant portion classifed as Tier 1 matrix forest, defined as “large contiguous areas whose size and natural condition allow for the maintenance of ecological processes” (Databasin, 2019). However, forests managed as intact do not need to be large or old in absolute terms to have ecological value: disturbances create gaps and young habitats, and the official policy of the Commonwealth of Massachusetts Department of Environmental Management (now Department of Conservation and Recreation) considers an old-growth forest of at least 2 hectares ecologically significant (Department of Environmntal Management, 1999).
As shown in Table 1, ecosystem services accrue as forests age for centuries. Far from plateauing in terms of carbon sequestration (or added wood) at a relatively young age as was long believed, older forests (e.g., >200 years of age without intervention) contain a variety of habitats, typically continue to sequester additional carbon for many decades or even centuries, and sequester significantly more carbon than younger and managed stands (Luyssaert et al., 2008; Askins, 2014; McGarvey et al., 2015; Keeton, 2018). A recent paper affirmed that letting forests grow is an effective way to sequester carbon—but unlike previous studies it suggested that sequestration is highest in “young” forests (Pugh et al., 2019). This conclusion is problematic for several reasons. One confounding factor is that older forests in the tropics were compared to young forests in temperate and boreal areas; temperate forests in particular have the highest CO2 removal rates and overall biological carbon sequestration (Keith et al., 2009) but this high rate is not limited to young temperate and boreal forests. The age when sequestration rates decrease is not known, and Pugh et al. defined “young” as up to 140 years. As noted above, Keeton et al. (2011) estimate that secondary forests in the Northeast have the potential to increase their biological carbon sequestration several-fold. More field work is needed across age ranges, species and within biomes, but the inescapable conclusion is that growing forests is beneficial to the climate and maintaining intact forest has additional benefits (Table 1). We conclude that proforestation has the potential to provide rapid, additional carbon sequestration to reduce net emissions in the U.S. by much more than the 11% that forests provide currently (United States Environmental Protection Agency, 2019). A recent report on natural climate solutions determined that negative emissions could be increased from 11 to 21% even without including proforestation (Fargione et al., 2018). Quantified estimates of increased forest sequestration and ecosystem services were based on re-establishing forests where possible and lengthening rotation times on private land; they explicitly did not account for proforestation potential on public land.
Although biological carbon storage in managed stands, regardless of the silvicultural prescription, is generally lower than in unmanaged intact forests (Harmon et al., 1990; Ford and Keeton, 2017)—even after the carbon stored in wood products is included in the calculation—stands managed with reduced harvest frequency and increased structural retention sequester more carbon than more intensively managed stands (Nunery and Keeton, 2010; Law et al., 2018). Such an approach for production forests, or “working” forests—balancing resource extraction with biological carbon sequestration—is often termed “managing for net carbon” or “managing for climate change” and an approach that should be promoted alongside dedicating significant areas to intact ecosystems. Oliver et al. (2014) acknowledge a balance between intact and managed forest and suggest that long term storage in “efficient” wood products like wood building materials (with the potential for less carbon emissions compared to steel or concrete, termed the “avoidance pathway”) can offer a significant carbon benefit. To achieve this, some questionable assumptions are that 70% of the harvested wood is merchantable and stored in a lasting product, all unmerchantable wood is removed and used, harvesting occurs at optimum intervals (100 years) and carbon sequestration tapers off significantly after 100 years. Forestry models underestimate the carbon content of older, larger trees, and it is increasingly clear that trees can continue to remove atmospheric carbon at increasing rates for many decades beyond 100 years (Robert T. Leverett, pers. comm. Stephenson et al., 2014; Lutz et al., 2018; Leverett et al., under review). Because inefficient logging practices result in substantial instant carbon release to the atmosphere, and only a small fraction of wood becomes a lasting product, increasing market forces and investments toward wood buildings that have relatively short lifetimes could increase forest extraction rates significantly and become unsustainable (Oliver et al., 2014).
Habitat Protection, Biodiversity and Scientific Value of Proforestation
Large trees and intact, older forests are not only effective and cost-effective natural reservoirs of carbon storage, they also provide essential habitat that is often missing from younger, managed forests (Askins, 2014). For example, intact forests in Eastern U.S. national parks have greater tree diversity, live and dead standing basal area, and coarse woody debris, than forests that are managed for timber (Miller et al., 2016, 2018; Table 1). The density of cavities in older trees and the spatial and structural heterogeneity of the forest increases with stand age (Ranius et al., 2009; Larson et al., 2014), and large canopy gaps develop as a result of mortality of large trees, which result in dense patches of regeneration (Askins, 2014). These complex structures and habitat features support a greater diversity of lichens and bryophytes (Lesica et al., 1991), a greater density and diversity of salamanders (Petranka et al., 1993; Herbeck and Larsen, 1999), and a greater diversity and abundance of birds in old, intact forests than in nearby managed forests (Askins, 2014; Zlonis and Niemi, 2014; Table 1). Forest bird guilds also benefit from small intact forests in urban landscapes relative to unprotected matrix forests (Goodwin and Shriver, 2014). Several bird species in the U.S. that are globally threatened—including the wood thrush, cerulean warbler, marbled murrelet, and spotted owl are, in part, dependent on intact, older forests with large trees (International Union for Conservation of Nature, 2019). Two species that are extinct today—Bachman's warbler and Ivory-billed woodpecker—likely suffered from a loss of habitat features associated with old forests (Askins, 2014).
Today, forest managers often justify management to maintain heterogeneity of age structures to enhance wildlife habitat and maintain “forest health” (Alverson et al., 1994). However, early successional forest species (e.g., chestnut-sided warbler and New England cottontail) that are common targets for forest management may be less dependent on forest management than is commonly believed (cf. Zlonis and Niemi, 2014; Buffum et al., 2015). Management also results in undesirable consequences such as soil erosion, introduction of invasive and non-native species (McDonald et al., 2008; Riitters et al., 2018), loss of carbon—including soil carbon (Lacroix et al., 2016), increased densities of forest ungulates such as white-tailed deer (Whitney, 1990)—a species that can limit forest regeneration (Waller, 2014)—and a loss of a sense of wildness (e.g., Thoreau, 1862).
Forest health is a term often defined by a particular set of forestry values (e.g., tree regeneration levels, stocking, tree growth rates, commercial value of specific species) and a goal of eliminating forest pests. Although appropriate in a commercial forestry context, these values should not be conflated with the ability of intact natural forests to continue to function and even thrive indefinitely and provide a diversity of habitats on their own (e.g., Zlonis and Niemi, 2014). Natural forests, regardless of their initial state, naturally develop diverse structures as they age and require from us only the time and space to self-organize (e.g., Larson et al., 2014; Miller et al., 2016).
Intact forests provide irreplaceable scientific value. In addition to a biodiverse habitat an intact forest provides an area governed by natural ecological processes that serve as important scientific controls against which to compare the effects of human activities and management practices (Boyce, 1998). Areas without resource extraction (i.e., timber harvesting, hunting), pest removal, or fire suppression allow for a full range of natural ecological processes (fire, herbivory, natural forest development) to be expressed (Boyce, 1998). Only if we have sufficient natural areas can we hope to understand the effects of human activities on the rest of our forests. Additional research and monitoring projects that compare ecological attributes between intact and managed forests at a range of spatial scales will also help determine how effective protected intact forests can be at conserving a range of biota, and where additional protected areas may need to be established (e.g., Goodwin and Shriver, 2014; Jenkins et al., 2015).
Proforestation and Forest Fires
Given the increase in forest area burned in the United States over the past 30 years (National Interagency Fire Center, 2019), it is important to address the relationship between forest management and forest fires. There is a widely held perception that the severity and size of recent fires are directly related to the fuels that have accumulated in the understory due to a lack of forest management to reduce these fuels (i.e., pulping, masticating, thinning, raking, and prescribed burning; Reinhardt et al., 2008; Bradley et al., 2016). However, some evidence suggests that proforestation should actually reduce fire risk and there are at least three important factors to consider: first, fire is an integral part of forest dynamics in the Western U.S.; second, wildfire occurrence, size, and area burned are generally not preventable even with fuel removal treatments (Reinhardt et al., 2008); and third, the area burned is actually far less today than in the first half of the twentieth century when timber harvesting was more intensive and fires were not actively suppressed (Williams, 1989; National Interagency Fire Center, 2019). Interestingly, in the past 30 years, intact forests in the Western U.S. burned at significantly lower intensities than did managed forests (Thompson et al., 2007; Bradley et al., 2016; Table 1). Increased potential fuel in intact forests appear to be offset by drier conditions, increased windspeeds, smaller trees, and residual and more combustible fuels inherent in managed areas (Reinhardt et al., 2008; Bradley et al., 2016). Rather than fighting wildfires wherever they occur, the most effective strategy is limiting development in fire-prone areas, creating and defending zones around existing development (the wildland-urban interface), and establishing codes for fire-resistant construction (Cohen, 1999; Reinhardt et al., 2008).
Proforestation and Ecosystem Services: Serving the Greatest Good
In 1905 Gifford Pinchot, Chief of the U.S. Forest Service, summarized his approach to the nation's forests when he wrote “…where conflicting interests must be reconciled, the question will always be decided from the standpoint of the greatest good of the greatest number in the long run.” This ethos continues to define the management approach of the U.S. Forest Service from its inception to the present day. Remarkably, however, even in 2018 the five major priorities of the Forest Service do not mention biodiversity, carbon storage, or climate change as major aspects of its work (United States Forest Service, 2018).
Today, the needs of the nation have changed: emerging forest science and the carbon and biodiversity benefits of proforestation demand a focus on growing intact natural public and private forests, including local parks and forest reserves (Jenkins et al., 2015). There is also a growing need across the country, and particularly within reach of highly populated areas, for additional local parks and protected forest reserves that serve and provide the public with solitude, respite, and wild experiences (e.g., Thoreau, 1862). Detailed analysis of over one thousand public comments regarding management of Hoosier National Forest, a public forest near population centers in several states, revealed a strong belief that wilderness contributes to a sense of well-being. Responses with the highest frequency reflected an interest in preservation and protection of forests and wildlife, a recognition of the benefits to human physical and mental health, a sense of ethical responsibility, opposition to damage and destruction, monetary concerns, and a preponderance of sadness, fear and distress over forest loss (Vining and Tyler, 1999).
Quantifiable public health benefits of forests and green spaces continue to emerge, and benefits are highest in populations with chronic and difficult-to-treat conditions like anxiety, depression, pain and post-traumatic stress disorder (Karjalainen et al., 2010; Frumkin et al., 2017; Hansen et al., 2017; Oh et al., 2017). In the United Kingdom “growing forests for health” is the motto of the National Health Service Forest (2019) and there is a recognized need for evidence-based analysis of human health co-benefits alongside nature-based ecosystem services (Frumkin et al., 2017).
Policy Recommendations
To date, the simplicity of the idea of proforestation has perhaps been stymied by inaccurate or non-existent terminology to describe it. Despite a number of non-binding international forest agreements (United Nations Conference on Environment Development, 1992; United Nations Forum on Forests, 2008; Forest Declaration, 2014) and responsibilities by a major UN organization [Food and Agriculture Organization (FAO)], current climate policies lack science-based definitions that distinguish forest condition—including the major differences between young and old forests across a range of ecosystem services. Lewis et al. (2019) further note that broad definitions and confused terminology have an unfortunate result that policymakers and their advisers mislead the public (Lewis et al., 2019). Most discussions concerning forest loss and forest protection are in terms of percentage of land area that has tree canopy cover (Food and Agriculture Organization, 2019). This lack of specificity significantly hampers efforts to evaluate and protect intact forests, to quantify their value, and to dedicate existing forests as intact forests for the future. For example, the UN Framework Convention on Climate Change and the FAO consider and group tree plantations, production forests, and mature intact forests equally under the general term “forest” (Mackey et al., 2015). In addition, “forest conservation” simply means maintaining “forest cover” and does not address age, species richness or distribution—or the degree that a forest ecosystem is intact and functioning (Mackey et al., 2015). The erroneous assumption is that all forests are equivalently beneficial for a range of ecosystem services—a conclusion that is quantitatively inaccurate in terms of biological carbon sequestration and biodiversity as well as many other ecosystem services.
Practicing proforestation should be emphasized on suitable public lands as is now done in U.S. National Parks and Monuments. Private forest land owners might be compensated to practice proforestation, for sequestering carbon and providing associated co-benefits by letting their forests continue to grow. At this time, we lack national policies that quantify and truly maximize benefits across the landscape. At a regional scale, however, some conservation visions do explicitly recognize and promote the multiple values and services associated with forest reserves or wildlands (e.g., Foster et al., 2010) and climate offset programs can be used explicitly to support proforestation. For example, a recent project by the Nature Conservancy protected 2,185 hectares (5,400 acres) in Vermont as wildland and is expected to yield ~$2 M over 10 years for assuring long-term biological carbon storage (Nature Conservancy, 2019). Burnt Mountain is now protected by a “forever wild” easement and part of a 4,452 hectare (11,000 acre) preserve. More public education and similar incentives are needed.
Conclusions
To meet any proposed climate goals of the Paris Climate Agreement (1.5, 2.0° C, targets for reduced emissions) it is essential to simultaneously reduce greenhouse gas emissions from all sources including fossil fuels, bioenergy, and land use change, and increase CDR by forests, wetlands and soils. Concentrations of these gases are now so high that reducing emissions alone is insufficient to meet these goals. Speculation that untested technologies such as BECCS can achieve the goal while allowing us to continue to emit more carbon has been described as a “moral hazard” (Anderson and Peters, 2016). Furthermore, BECCS is not feasible within the needed timeframe and CDR is urgent. Globally, existing forests only store approximately half of their potential due to past and present management (Erb et al., 2018), and many existing forests are capable of immediate and even more extensive growth for many decades (Lutz et al., 2018). During the timeframe while seedlings planted for afforestation and reforestation are growing (yet will never achieve the carbon density of an intact forest), proforestation is a safe, highly effective, immediate natural solution that does not rely on uncertain discounted future benefits inherent in other options.
Taken together, proforestation is a rapid and essential strategy for achieving climate and biodiversity goals and for serving the greatest good. Stakeholders and policy makers need to recognize that the way to maximize carbon storage and sequestration is to grow intact forest ecosystems where possible. Certainly, all forests have beneficial attributes, and the management focus of some forests is providing wood products that we all use. But until we acknowledge and quantify differences in forest status (Foster et al., 2010), we will be unable to develop policies (and educate landowners, donors, and the public) to support urgent forest-based benefits in the most effective, locally appropriate and cost-effective manner. A differentiation between production forests and natural forest ecosystems would garner public support for a forest industry with higher value products and a renewed focus on reducing natural resource use—and for recycling paper and wood. It could also spur long-overdue local partnerships between farms and forests—responsible regional composting keeps jobs and resources within local communities while improving soil health and increasing soil carbon (Brown and Cotton, 2011). The forest industry as a whole can benefit from proforestation-based jobs that focus on scientific data collection, public education, public health and a full range of ecosystem services.
In sum, proforestation provides the most effective solution to dual global crises—climate change and biodiversity loss. It is the only practical, rapid, economical, and effective means for atmospheric CDR among the multiple options that have been proposed because it removes more atmospheric carbon dioxide in the immediate future and continues to sequester it long-term. Proforestation will increase the diversity of many groups of organisms and provide numerous additional and important ecosystem services (Lutz et al., 2018). While multiple strategies will be needed to address global environmental crises, proforestation is a very low-cost option for increasing carbon sequestration that does not require additional land beyond what is already forested and provides new forest related jobs and opportunities along with a wide array of quantifiable ecosystem services, including human health.
Author Contributions
WM, SM, and EF contributed equally to conceiving, writing and editing this manuscript and all agree to its publication.
Funding
Supported by Charles Bullard Fellowship in Forest Research, Harvard Forest (SM).
Conflict of Interest Statement
The authors declare that the research was conducted in the absence of any commercial or financial relationships that could be construed as a potential conflict of interest.
Acknowledgments
The authors thank the reviewers for improving the manuscript with substantive and thoughtful comments and thank David N. Ruskin, Ph.D. (Trinity College) for feedback and assistance throughout.
References
Alverson, W. S., Waller, D., and Kuhlmann, W. (1994). Wild Forests: Conservation Biology and Public Policy. Washington, DC: Island Press.
Anderson, K., and Peters, G. (2016). The trouble with negative emissions. Science 354, 182–183 doi: 10.1126/science.aah4567
Askins, R. A. (2014). Saving the World's Deciduous Forests: Ecological Perspectives From East Asia, North America, and Europe. New Haven, CT: Yale University Press.
Boyce, M. S. (1998). Ecological-process management and ungulates: Yellowstone's conservation paradigm. Wildlife Soc. Bull. 26, 391–398.
Bradley, C. M., Hanson, C. T., and DellaSala, D. A. (2016). Does increased forest protection correspond to higher fire severity in frequent-fire forests of the western United States? Ecosphere 7:e01492. doi: 10.1002/ecs2.1492
Brown, S., and Cotton, N. (2011). Changes in soil properties and carbon content following compost application: Results of on-farm sampling. Compost Sci. Util. 19, 87–96. doi: 10.1080/1065657X.2011.10736983
Buffum, B., McGreevy, T. J. Jr., Gottfried, A. E., Sullivan, M. E., and Husband, T. P. (2015). An analysis of overstory tree canopy cover in sites occupied by native and introduced cottontails in the Northeastern United States with recommendations for habitat management for new England Cottontail. PloS ONE 10:e0135067. doi: 10.1371/journal.pone.0135067
Cohen, J. D. (1999). Reducing the Wildland Fire Threat to Homes: Where and How Much? U.S.D.A Forest Service Gen.Tech. Rep., PSW-GTR-173, 189-195. Available online at: https://www.fs.fed.us/rm/pubs_other/rmrs_1999_cohen_j001.pdf (accessed April 15, 2019).
Cordell, H. K. (2012). Outdoor Recreation Trends and Futures: A Technical Document Supporting the Forest Service 2010 RPA Assessment. Asheville, NC: United States Department of Agriculture, Southern Research Station. Available online at: https://www.srs.fs.usda.gov/pubs/gtr/gtr_srs150.pdf
Databasin (2019). Available online at: https://databasin.org/datasets/68c240fb9dc14fda8ccd965064fb3321 (accessed April 15, 2019).
Department of Environmntal Management (1999). Old Growth Policy. State of Massachusetts, Division of Forest and Parks, Bureau of Forestry.
Division for Sustainable Development Goals (2015). Sustainable Development Goals. Knowledge Platform, United Nations. Available online at: https://sustainabledevelopment.un.org/?menu=1300 (accessed april 15, 2019).
Duveneck, M. J., and Thompson, J. R. (2019). Social and biophysical determinants of future forest conditions in New England: effects of a modern land-use regime. Global Environ. Change 55, 115–129. doi: 10.1016/j.gloenvcha.2019.01.009
Erb, K.-H., Kastner, T., Plutzar, C., Bais, A. L. S., Carvalhais, N., Fetzel, T., et al. (2018). Unexpectedly large impact of forest management and grazing on global vegetation biomass. Nature 553, 73–76. doi: 10.1038/nature25138
Fargione, J. E., Bassett, S., Boucher, T, Bridgham, S. D., Conant, R. T., Cook-Patton, S. C., et al. (2018). Natural climate solutions for the United States. Sci Adv. 4:eaat1869. doi: 10.1126/sciadv.aat1869
Foley, J. A., DeFries, R., Asner, G. P., Barford, C., Bonan, G., Carpenter, S. R., et al. (2005). Global consequences of land use. Science 309, 570–574. doi: 10.1126/science.1111772
Food Agriculture Organization (2019). Food Agriculture Organization of the United Nations. Available online at: http://www.fao.org/home/en/ (accessed April 15, 2019).
Ford, S. E., and Keeton, W. E. (2017). Enhanced carbon storage through management for old-growth characteristics in northern hardwoods. Ecosphere 8, 1–20. doi: 10.1002/ecs2.1721
Forest Declaration (2014). New York Declaration on Forests. Available online at: Available online at: http://forestdeclaration.org/about/ (accessed April 15, 2019).
Foster, D. R., Donahue, B. M., Kittredge, D. B., Lambert, K. F., Hunter, M. L., Hall, B. R., et al. (2010). Wildlands and Woodlands: A Vision for the New England Landscape. Cambridge, MA: Harvard University Press. Available online at: https://www.wildlandsandwoodlands.org/sites/default/files/Wildlands%20and%20Woodlands%20New%20England.pdf
Frumkin, H., Bratman, G. N., Breslow, S. J., Cochran, B., Kahn, P. H. Jr., Lawler, J. J., et al. (2017). Nature contact and human health: a research agenda. Environ. Health Perspect. 125:075001. doi: 10.1289/EHP1663
Funk, J. M., Aguilar-Amuchastegui, N., Baldwin-Cantello, W., Busch, J., Chuvasov, E., Evans, T., et al. (2019). Securing the climate benefits of stable forests. Clim. Policy. doi: 10.1080/14693062.2019.1598838
Goodwin, S. E., and Shriver, W. G. (2014). Using a bird community index to evaluate national parks in the urbanized national capital region. Urban Ecosyst. 17, 979–990. doi: 10.1007/s11252-014-0363-2
Griscom, B. W., Adams, J., Ellis, P. W., Houghton, R. A., Lomax, G., Miteva, D. A., et al. (2017). Natural climate solutions. Proc. Natl. Acad. Sci. U.S.A 114, 11645–11650. doi: 10.1073/pnas.1710465114
Hansen, M. M., Jones, R., and Tocchini, K. (2017). Shinrin-Yoku (forest bathing) and nature therapy: a state-of-the-art review. Int. J. Environ. Res. Public Health 14:851. doi: 10.3390/ijerph14080851
Harmon, M. E., Ferrell, W. K., and Franklin, J. F. (1990). Effects on carbon storage of conversion of old-growth forests to young forests. Science 247, 699–702. doi: 10.1126/science.247.4943.699
Harris, N. L., Hagen, S. C., Saatchi, S. S., Pearson, T. R. H., Woodall, C. W., Domke, G. M., et al. (2016). Attribution of net carbon change by disturbance type across forest lands of the conterminous United States. Carbon Balance Manag. 11:24. doi: 10.1186/s13021-016-0066-5
Herbeck, L. A., and Larsen, D. R. (1999). Plethodontid salamander response to silvicultural practices in Missouri Ozark forests. Conserv. Biol. 13, 623–632. doi: 10.1046/j.1523-1739.1999.98097.x
Houghton, R. A., and Nassikas, A. A. (2018). Negative emissions from stopping deforestation and forest degradation, globally. Glob. Change Biol. 24, 350–359. doi: 10.1111/gcb.13876
Intergovernmental Panel on Climate Change (2013). “Summary for policymakers,” in Climate Change 2013: The Physical Science Basis. Contribution of Working Group I to the Fifth Assessment Report of the Intergovernmental Panel on Climate Change, eds T. F. Stocker, D. Qin, G.-K. Plattner, M. Tignor, S. K. Allen, J. Boschung, A. Nauels, Y. Xia, V. Bex, and P. M. Midgley (New York, NY: Cambridge University Press). Available online at: https://www.ipcc.ch/report/ar5/wg1/.
Intergovernmental Panel on Climate Change (2014). “Summary for policymakers,” in Climate Change 2014: Impacts, Adaptation, and Vulnerability. Part A: Global and Sectoral Aspects. Contribution of Working Group II to the Fifth Assessment Report of the Intergovernmental Panel on Climate Change, eds C. B. Field, V. R. Barros, D. J. Dokken, K. J. Mach, M. D. Mastrandrea, T. E. Bilir, M. Chatterjee, K. L. Ebi, Y. O. Estrada, R. C. Genova, B. Girma, E. S. Kissel, A. N. Levy, S. Maccracken, P. R. Mastrandrea, and L. L. White (New York, NY: Cambridge University Press). Available online at: https://www.ipcc.ch/report/ar5/wg2/.
Intergovernmental Panel on Climate Change (2018). “Summary for Policymakers,” in Global warming of 1.5°C. An IPCC Special Report on the impacts of global warming of 1.5°C Above Pre-Industrial Levels and Related Global Greenhouse Gas Emission Pathways, in the Context of Strengthening the Global Response to the Threat of Climate Change, Sustainable Development, and Efforts to Eradicate Poverty, eds V. Masson-Delmotte, P. Zhai, H. O. Pörtner, D. Roberts, J. Skea, P. R. Shukla, A. Pirani, W. Moufouma-Okia, C. Péan, R. Pidcock, S. Connors, J. B. R. Matthews, Y. Chen, X. Zhou, M. I. Gomis, E. Lonnoy, T. Maycock, M. Tignor, and T. Waterfield. (Geneva: World Meteorological Society). Available online at: https://www.ipcc.ch/sr15/.
Intergovernmental Science-Policy Platform on Biodiversity Ecosystem Services (IPBES). (2019). Available online at: https://www.ipbes.net/
International Union for Conservation of Nature (2019). IUCN Red List of Threatened Species Available online at: https://www.iucnredlist.org/ (accessed April 15, 2019).
Jenkins, C. N., Van Houtan, K. S., Pimm, S. L., and Sexton, J. O. (2015). US protected lands mismatch biodiversity priorities. Proc. Natl. Acad. Sci.U.S.A. 112, 5081–5086. doi: 10.1073/pnas.1418034112
Karjalainen, E., Sarjala, T., and Raitio, H. (2010). Promoting human health through forests: overview and major challenges. Environ. Health Prev. Med. 15, 1–8. doi: 10.1007/s12199-008-0069-2
Keeton, W. S., Whitman, A. A., McGee, G. C., and Goodale, C. L. (2011). Late-successional biomass development in northern hardwood-conifer forests of the Northeastern United States. Forest Sci. 57, 489–505. doi: 10.1093/forestscience/57.6.489
Keeton, W. S. (2018). “Source or sink? Carbon dynamics in old-growth forests and their role in climate change mitigation,” in Ecology and Recovery of Eastern Old-growth Forests, eds A. Barton and W. S. Keeton (Washington, DC: Island Press), 340.
Keith, H., Mackey, B. G., and Lindenmayer, D. B. (2009). Re-evaluation of forest biomass carbon stocks and lessons from the world's most carbon-dense forests. Proc. Natl. Acad. Sci. U.S.A. 106, 11635–11640. doi: 10.1073/pnas.0901970106
Koberstein, P., and Applegate, J. (2018). Trump's Great American Forest Liquidation Sale. Cascadia Times. Available online at: https://www.times.org/forest-liquidation-sale (accessed April 15, 2019).
Lacroix, E. M., Petrenko, C. L., and Friedland, A. J. (2016). Evidence for losses from strongly bound SOM pools after clear cutting in a northern hardwood forest. Soil Sci. 181, 202–207. doi: 10.1097/SS.0000000000000147
Larson, A. J., Lutz, J. A., Donato, D. C., Freund, J. A., Swanson, M. E., Hillerislambers, J., et al. (2014). Spatial aspects of tree mortality strongly differ between young and old-growth forests. Ecology 96, 2855–2861. doi: 10.1890/15-0628.1
Law, B. E., Hudiburg, T. W., Berner, L. T., Kent, J. J., Buotte, P. C., and Harmon, M. E. (2018). Land use strategies to mitigate climate change in carbon dense temperate forests. Proc. Natl. Acad. Sci. U.S.A. 115, 3663–3668. doi: 10.1073/pnas.1720064115
Le Quéré, C., Andrew, R. M., Friedlingstein, P., Sitch, S., Pongratz, J., Manning, A. C., et al. (2018). Global carbon budget 2017. Earth Syst. Sci. Data 10, 405–448. doi: 10.5194/essd-10-405-2018
Lesica, P., Mccune, B., Cooper, S. V., and Hong, W. S. (1991). Differences in lichen and bryophyte communities between old-growth and managed second-growth forests in the Swan Valley, Montana Can. J. Botany 69, 1745–1755. doi: 10.1139/b91-222
Lewis, S. L., Wheeler, C. E., Mitchard, E. T. A., and Koch, A. (2019). Restoring natural forests is the best way to remove atmospheric carbon. Nature 568, 25–28. doi: 10.1038/d41586-019-01026-8
Liu, X., Stefan, T., Jin-Sheng, H., Pascal, A. N., Helge, B., Zhiyao, T., et al. (2018). Tree species richness increases ecosystem carbon storage in subtropical forests. Proc. R. Soc. B 285:20181240. doi: 10.1098/rspb.2018.1240
Lutz, J. A., Furniss, T. J., Johnson, D. J., Davies, S. J., Allen, D., Alonso, A., et al. (2018). Global importance of large-diameter trees. Glob. Ecol. Biogeogr. 27, 849–864. doi: 10.1111/geb.12747
Luyssaert, S., Schulze, E. D., Börner, A., Knohl, A., Hessenmöller, D., Law, B. E., et al. (2008). Old-growth forests as global carbon sinks. Nature 455, 213–215. doi: 10.1038/nature07276
Mackey, B., Dellasala, D. A., Kormos, C., Lindenmayer, D., Kumpel, N., Zimmerman, B., et al. (2015). Policy options for the world's primary forests in multilateral environmental agreements. Conserv. Lett. 8, 139–147. doi: 10.1111/conl.12120
McDonald, R. I., Motzkin, G., and Foster, D. R. (2008). Assessing the influence of historical factors, contemporary processes, and environmental conditions on the distribution of invasive species. J. Torrey Bot. Soc. 135, 260–271. doi: 10.3159/08-RA-012.1
McGarvey, J. C., Thompson, J. R., Epstein, H. E., and Shugart, H. H. Jr. (2015). Carbon storage in old-growth forests of the Mid-Atlantic: toward better understanding the eastern forest carbon sink. Ecology 96, 311–317. doi: 10.1890/14-1154.1
Miller, K. M., Dieffenbach, F. W., Campbell, J. P., Cass, W. B., Comiskey, J. A., Matthews, E. R., et al. (2016). National parks in the eastern United States harbor important older forest structure compared with matrix forests. Ecosphere 7:e01404. doi: 10.1002/ecs2.1404
Miller, K. M., Mcgill, B. J., Mitchell, B. R., Comiskey, J., Dieffenbach, F. W., Matthews, E. R., et al. (2018). Eastern national parks protect greater tree species diversity than unprotected matrix forests. Forest Ecol. Manag. 414, 74–84. doi: 10.1016/j.foreco.2018.02.018
National Academies of Sciences Engineering, and Medicine. (2019). Negative Emissions Technologies and Reliable Sequestration: A Research Agenda. Washington, DC: The National Academies Press.
National Conservation Easement Database (2014) Available online at: conservationeasement.us (accessed April 15, 2019).
National Council for Air Stream Improvement (2019). United States Department of Agriculture Forest Service. COLE: Carbon on Line Estimator. Available online at: https://www.fs.usda.gov/ccrc/index.php?q=tools/cole (accessed April 15, 2019).
National Health Service Forest (2019). National Health Service. Available online at: http://nhsforest.org/ (accessed April 15, 2019).
National Interagency Fire Center (2019). Total Wildland Fires and Acres (1926-2017). Available online at: https://www.nifc.gov/fireInfo/fireInfo_stats_totalFires.html (accessed April 15, 2019).
Nature Conservancy (2019). The. “Burnt Mountain Beauty: Explore Vermont's Newest Preserve and the State's Largest Carbon Project”. Available online at: https://www.nature.org/en-us/about-us/where-we-work/united-states/vermont/stories-in-vermont/burnt-mountain-beauty/ (accessed April 15, 2019).
Nunery, J. S., and Keeton, W. S. (2010). Forest carbon storage in the northeastern United States: net effects of harvesting frequency, post-harvest retention, and wood products. Forest Ecol. Manag. 259, 1363–1375. doi: 10.1016/j.foreco.2009.12.029
Oh, B., Lee, K. J., Zaslawski, C., Yeung, A., Rosenthal, D., Larkey, L., et al. (2017). Health and well-being benefits of spending time in forests: systematic review. Environ. Health Prev. Med. 22:71. doi: 10.1186/s12199-017-0677-9
Oliver, C. D., Nassar, N. T., Lippke, B. R., and McCarter, J. B. (2014). Carbon, fossil fuel, and biodiversity mitigation with wood and forests. J. Sustain. Forest. 33, 248–275. doi: 10.1080/10549811.2013.839386
Oswalt, S. N., Smith, W. B., Miles, P. D., and Pugh, S. A. (2014). Forest Resources of the United States, 2012: A Technical Document Supporting the Forest Service 2010 Update of the RPA Assessment. United States Department of Agriculture, Forest Service, Washington Office. (Washington, DC), Gen. Tech. Rep. WO-91. doi: 10.2737/WO-GTR-91
Pan, Y., Chen, J. M., Birdsey, R., McCullough, K., He, L., and Deng, F. (2011). Age structure and disturbance legacy of North American forests. Biogeosciences 8, 715–738. doi: 10.5194/bg-8-715-2011
Paris Climate Agreement (2015). Available online at: https://unfccc.int/sites/default/files/english_paris_agreement.pdf (accessed April 15, 2019).
Petranka, J. W., Eldridge, M. E., and Haley, K. E. (1993). Effects of timber harvesting on southern Appalachian salamanders. Conserv. Biol. 7, 363–370. doi: 10.1046/j.1523-1739.1993.07020363.x
Pugh, T. A. M., Lindeskog, M., Smith, B., Poulter, B., Arneth, A., Haverd, V., et al. (2019). Role of forest regrowth in global carbon sink dynamics. Proc. Natl. Acad. Sci. U.S.A. 116, 4382–4387. doi: 10.1073/pnas.1810512116
Ranius, T., Niklasson, M., and Berg, N. (2009). Development of tree hollows in pedunculate oak (Quercus robur). Forest Ecol. Manag. 257, 303–310. doi: 10.1016/j.foreco.2008.09.007
Reinhardt, E. D., Keane, R. E., Calkin, D. E., and Cohen, J. D. (2008). Objectives and considerations for wildland fuel treatment in forested ecosystems of the interior western United States. Forest Ecol. Manag. 256, 1997–2006. doi: 10.1016/j.foreco.2008.09.016
Riitters, K., Potter, K., Iannone, B., Oswalt, C., Guo, Q., and Fei, S. (2018). Exposure of protected and unprotected forest to plant invasions in the eastern United States. Forests 9:723. doi: 10.3390/f9110723
Searchinger, T. D., Hamburg, S. P., Melillo, J., Chameides, W., Havlik, P., Kammen, D. M., et al. (2009). Climate change. Fixing a critical climate accounting error. Science 326, 527–528. doi: 10.1126/science.1178797
Stephenson, N. L., Das, A. J., Condit, R., Russo, S. E., Baker, P. J., Beckman, N. G., et al. (2014). Rate of tree carbon accumulation increases continuously with tree size. Nature 507, 90–93. doi: 10.1038/nature12914
Sterman, J. D., Siegel, L., and Rooney-Varga, J. N. (2018). Reply to comment on 'Does replacing coal with wood lower CO2 emissions? Dynamic lifecycle analysis of wood bioenergy'. Environ. Res. Lett. 13:128003. doi: 10.1088/1748-9326/aaf354
Thompson, J. R., Foster, D. R., Scheller, R., and Kittredge, D. (2011). The influence of land use and climate change on forest biomass and composition in Massachusetts, USA. Ecol. Appl. 21, 2425–2444. doi: 10.1890/10-2383.1
Thompson, J. R., Spies, T. A., and Ganio, L. M. (2007). Reburn severity in managed and unmanaged vegetation in a large wildfire. Proc. Natl. Acad. Sci. U.S.A. 104, 10743–10748. doi: 10.1073/pnas.0700229104
Thoreau, H. D. (1862). Walking. The Atlantic Monthly, A Magazine of Literature, Art, and Politics. Available online at: https://www.theatlantic.com/magazine/archive/1862/06/walking/304674/.
United Nations Conference on Environment Development (1992). Non-Legally Binding Authoritative Statement of Principles for a Global Consensus on the Management, Conservation and Sustainable Development of all Types of Forests. Available online at: http://www.un-documents.net/for-prin.htm (accessed April 15, 2019).
United Nations Forum on Forests (2008). Non-Legally Binding Instrument on All Types of Forests. Available online at: http://www.undocs.org/A/res/62/98 (accessed April 15, 2019).
United States Environmental Protection Agency (2019). Sources of Greenhouse Gas Emissions Available online at: https://www.epa.gov/ghgemissions/sources-greenhouse-gas-emissions (accessed April 15, 2019).
United States Forest Service (2019). Forest Inventory and Analysis National Program, Forest Inventory EVALIDator web-application Version 1.8.0.00. U.S. Department of Agriculture, Forest Service, Northern Research Station. Available online at: https://apps.fs.usda.gov/Evalidator/evalidator.jsp (accessed April 15, 2019).
United States Forest Service. Leadership Corner. (2018). Available online at: https://www.fs.fed.us/blogs/five-national-priorities-build-legacy-guide-agency-forward-0 (accessed April 15, 2019).
United States Geological Survey (2019a). Gap Analysis Program. Protected Areas Database of the United States (PADUS), version 1.3 Combined Feature Class. (accessed April 15, 2019).
United States Geological Survey (2019b). National Land Cover Database Land Cover Collection Available online at: https://catalog.data.gov/dataset/national-land-cover-database-nlcd-land-cover-collection (accessed April 15, 2019).
University of Montana (2019). Wilderness Connect. Available online at: https://wilderness.net/ (accessed April 15, 2019).
Vining, J., and Tyler, D. E. (1999). Values, emotions and desired outcomes reflected in public responses to forest management lans. Hum. Ecol. Rev. 6, 21–34.
Waller, D. M. (2014). “Effects of deer on forest herb layers,” in The Herbaceous Layer in Forests of Eastern North America, Ch. 16, 2nd Edn, ed F. S. Gilliam (New York, NY: Oxford University Press), 369–399. doi: 10.1093/acprof:osobl/9780199837656.003.0016
Watson, J. E. M., Evans, T., Venter, O., Williams, B., Tulloch, A., Stewart, C., et al. (2018). The exceptional value of intact forest ecosystems. Nat. Ecol. Evol. 2, 599–610. doi: 10.1038/s41559-018-0490-x
Whitney, G. G. (1990). The history and status of the hemlock-hardwood forests of the Allegheny Plateau. J. Ecol. 1, 443–458. doi: 10.2307/2261123
Williams, M. (1989). Americans and Their Forests: A Historical Geography. New York, NY: Cambridge University Press.
Williams, M. (2000). Available online at: https://www.fs.usda.gov/Internet/FSE_DOCUMENTS/fsm8_035779.pdf (accessed April 15, 2019).
Wilson, E. O. (2016). Half-Earth: Our Planet's Fight for Life. New York, NY: Liveright Publishing Corp.
Zhou, G., Liu, S., Li, Z., Zhang, D., Tang, X., Zhou, C., et al. (2006). Old-growth forests can accumulate carbon in soils. Science. 314:1417. doi: 10.1126/science.1130168
Keywords: biodiversity crisis, Pinchot, afforestation, reforestation, forest ecosystem, biological carbon sequestration, old-growth forest, second-growth forest
Citation: Moomaw WR, Masino SA and Faison EK (2019) Intact Forests in the United States: Proforestation Mitigates Climate Change and Serves the Greatest Good. Front. For. Glob. Change 2:27. doi: 10.3389/ffgc.2019.00027
Received: 19 January 2019; Accepted: 20 May 2019;
Published: 11 June 2019.
Edited by:
Alexandra C. Morel, University of Oxford, United KingdomReviewed by:
Don Waller, University of Wisconsin System, United StatesDominick Anthony DellaSala, Geos Institute, United States
Copyright © 2019 Moomaw, Masino and Faison. This is an open-access article distributed under the terms of the Creative Commons Attribution License (CC BY). The use, distribution or reproduction in other forums is permitted, provided the original author(s) and the copyright owner(s) are credited and that the original publication in this journal is cited, in accordance with accepted academic practice. No use, distribution or reproduction is permitted which does not comply with these terms.
*Correspondence: William R. Moomaw, william.moomaw@tufts.edu