- 1NSW Department of Primary Industries and Regional Development, Sydney Institute of Marine Science, Mosman, NSW, Australia
- 2NSW Department of Primary Industries and Regional Development, Fisheries Conservation Technology Unit, Coffs Harbour, NSW, Australia
- 3School of the Environment, University of Queensland, Brisbane, QLD, Australia
- 4The Roslin Institute and Royal (Dick) School of Veterinary Studies, The University of Edinburgh, Easter Bush Campus, Edinburgh, United Kingdom
The most common method of estimating teleost ages is via sectioned otoliths. With diminishing funding and policies around cost-recovery from fishing industries, exploring cost-effective methods of estimating ages is warranted. The present study used 18 years of size-at-age data collected from monitoring of the commercial halfbeak (Hyporhamphus australis) fishery off New South Wales, Australia, to predict age classes from otolith weights, while considering other sources of variability such as sex, fish length, and year, month, and location of capture. We observed a significant linear relationship between age class and mean otolith weight. A generalized linear mixed model predicted 1-year olds with an 82% success rate; but was less successful for other ages. Year of sampling explained the greatest variability in the model and the distributions of otolith weights for each age class had considerable overlap. We conclude that substantial inter-annual variability in the age-class to otolith weight relationship, in addition to the relatively low precision when aging H. australis by counting annuli in sectioned otoliths, limits the predictive capacity of this model for future monitoring. Nevertheless, substantial cost savings could be made through recalibrating the model for new samples through direct aging of a subset of otoliths each year. The population of H. australis is continuing to rebuild from a previously overfished state, with an expectation that older fish will become more abundant in the fishery. Age estimation from counting annuli in sectioned otoliths is likely to be the most reliable method of identifying older individuals.
1 Introduction
Knowing teleost ages is a prerequisite to understanding fundamental biological characteristics, including growth and resilience to fishing (1). The most common method for accurately estimating individual teleost age is through the science of sclerochronology (2), using otoliths, which are calcified structures located in a right and left labyrinth behind the brain that accumulate material and grow throughout a fish's life (1, 3–5). Otolith function relates to hearing and the vestibular system; however, a permanent record of a fish's history is provided by the continuous deposition of calcium carbonate, usually the polymorph aragonite, within a gelatinous protein matrix (6). This material alternates in opaque and translucent increments which, following validation of periodicity, can be counted to estimate age at various temporal scales, but those with the most research, and the most use are daily and annual (3, 7). Because of their unique characteristic as natural data loggers, otoliths may be the most important biological structure for fisheries scientists (8).
By facilitating information on age compositions in exploited teleost populations, otolith reads are a vital tool for assessing stock health and sustainability (9). Age-based stock assessments are commonly used and comprise powerful analyses to support fisheries management (10). So important are age-based assessments globally that substantial resources are routinely committed to otolith-based monitoring, despite the expense associated with collection, processing and reading (11). As one example, following an informal survey of fisheries laboratories during 1999, Campana and Thorrold (1) estimated that at a minimum more than one million otoliths may have been aged at a cost of potentially CAN$10 million. During the subsequent two decades, in response to requirements for better assessments of global fish stocks, otolith aging efforts have substantially increased along with costs, which can exceed USD$60 per otolith (12). Intuitively, any efforts at minimizing expenses while still maintaining data quality are coherent strategies for all agencies.
A potential, ancillary cost-saving approach for analyzing otoliths involves quantifying and then extrapolating correlations between their weight and fish ages (5). However, while significant linear relationships have been noted for several species [e.g., (13–18)] models to predict individual fish ages from otolith weights have had various levels of success. Deficits are likely due to several factors including, but not limited to, variability in otolith weights between different age classes, spatial and temporal variations in the relationship, and/or reader errors from direct otolith interpretation (3, 5). In cases where there are no biases, or biases can be corrected, success has been achieved in modeling age compositions, rather than individual fish age (15, 19, 37).
Off New South Wales (NSW), Australia, one harvested teleost population that has been managed via monitoring of commercial catch and effort data and counting validated annuli in sectioned sagittal otoliths is the eastern sea garfish (Hyporhamphus australis) (20, 21). Schools of this surface-dwelling species are targeted by boat-based lampara nets—similar to those used for other species within the family Hemiramphidae (“halfbeaks”) around the world (22).
The NSW fishery for H. australis was assessed as being overfished for a decade during the early 2000s (20) but has since recovered and was transitioned to quota-management in 2017. Currently, the estimated sustainable harvest is supported by an annual age-based stock assessment using a combination of catch, effort and mortality derived through relative year-class strength (23, 24). Aging precision is relatively low in this species with the process of sectioning otoliths and reading them using microscopy costly (~AUD$30 an otolith).
In NSW, full cost-recovery from industry for monitoring and assessing jurisdictional fisheries is planned and as such it is appropriate that cost-effective alternatives to current practices are explored. Stewart et al. (25) reported a significant linear relationship between age in days and otolith weight for H. australis; however, no attempt has been made to predict age using this relationship. We therefore aimed to explore the feasibility of estimating age compositions without direct aging of otoliths through establishing models of H. australis age with otolith weights and other sampling variables.
2 Methods
2.1 Experimental design
Age-related commercial fishery monitoring data were available for 6,155 sampled H. australis for 18 years, spanning 2004 to 2021 inclusive [see Stewart and Hughes (21) for more details]. Sampled fish were measured as fork length (FL) which in H. australis is the distance from the upper jaw to the fork in the tail, to the nearest cm rounding down, weighed to the nearest gram and had their sagittal otoliths removed. Otoliths were accessed ventrally by removing the gills, scoring the thickened region of the vertebrae with a scalpel blade and snapping the head back. The otoliths were removed using forceps, cleaned and stored in small envelopes containing information on the sample. The sex of each fish was determined from a macroscopic examination of the gonads. Single otoliths were subsequently weighed to the nearest 1 mg, with the right otolith always weighed unless obviously deformed in which case the left otolith was used. The resulting data included the estimated age (years) of each fish estimated from counts of annuli, along with sex, FL, body weight and the predictor variable of interest: single sagittal otolith weight. Fish age was estimated to the nearest integer, so that 0+ hereafter represents 0–1 years, 1+ represents 1–2 years, etc. The experimental data also included sampling year, month, location (port of landing), and one degree latitude band.
2.2 Statistical models
We initially tested the hypothesis that mean otolith weight increased linearly with estimated fish age using the coefficient of determination (R2), calculated as the square of Pearson's correlation coefficient between the two terms. Otolith weight and fish age were initially coded as continuous covariates, ranging from 11.3 to 37.7 mg and 0 to 5 years, respectively (Supplementary Table 1).
We then attempted to predict fish age based on the complete experimental data, which included the response (age), fixed treatment variables (sex, FL, body weight, and otolith weight) and random blocking factors (year, month, and location). Age was now coded as an ordinal factor with six ordered levels (0+, 1+, …, 5+ years). The observational (and experimental) units for age were the 6,155 individuals, as characterized by the date and location of their catch. The fixed treatment variable of primary interest, otolith weight, was coded as a continuous covariate, ranging from 0.87 to 46.95 mg (Supplementary Figure 1). The remaining treatment variables were the factor “sex” and covariates “FL” (14.7–35.5 cm) and “body weight” (11.3–215.4 mg). However, FL and body weight were almost colinear, and so, only the former was considered hereafter. The random blocking factors were “year” (18 levels), “month” (12 levels), “location” (29 levels), and their relevant interactions.
A generalized linear mixed model (GLMM) was fitted to the ordinal response using ASReml-R (26), assuming a cumulative multinomial distribution with logit link function. The cumulative thresholds were set at the boundaries between age classes (0|1, 1|2, …, 4|5 years). ASReml-R calculates the cumulative probability of response (individual) i belonging to age class j as:
where θj is the threshold coefficient for age class j, β is the vector of fixed effects and regression coefficients with design matrix Xi for individual i, and u is the vector of random blocking effects with design matrix Zi. The probability of individual i belonging to a specific age class is then obtained by manipulating the cumulative probabilities as required. The prediction of age class for new individuals is considered below.
ASReml-R fits GLMMs using penalized quasi-likelihood (27), and then obtains residual maximum likelihood estimates of the variance parameters and empirical best linear unbiased estimates or predictions of the fixed and random effects, respectively. The significance of fixed terms was determined using approximate Wald chi-square tests, conducted using an incremental sum of squares with sex and FL followed by otolith weight.
2.3 Age prediction of new individuals
The cumulative probability of new individual i belonging to age class j is calculated as:
where is the design matrix for new individual i, which contains information on otolith weight, sex and FL. The cumulative probability calculated here is obtained by conditioning on the random effects at their average value of zero, but note that appropriate random effects can be included where required (e.g., date of catch). Also note that the GLMM can be updated with new data to estimate new year effects and their interaction with month and location.
3 Results
Ages ranged between 0+ and 5+ years, but were mostly (81%) 1+ or 2+ years (Figure 1, Supplementary Table 1). The coefficient of determination confirmed that mean otolith weight increased with age (significant positive linear relationship; R2 = 0.95, Supplementary Table 1).
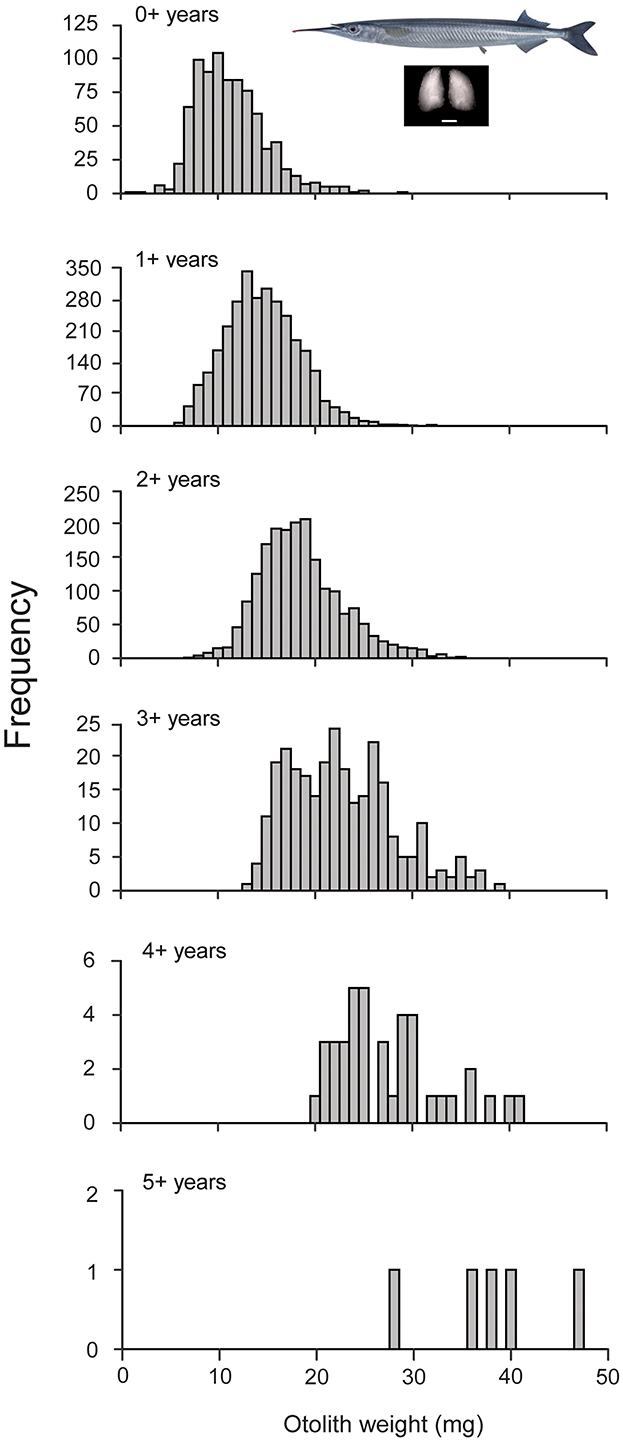
Figure 1. Histograms of otolith weights for 6,155 individuals in the Hyporhamphus australis dataset, by age class. The mean otolith weight across all age classes was 15.87 mg. The images are of Hyporhamphus australis and the sagittal otolith pair. Scale bar on the otolith image is 1 mm.
The GLMM indicated that year was the major source of variation, followed by interactions between year and month and between year, month, and location (Table 1). The model had a high classification success rate for fish aged 1+ years, with 82% having the same observed and predicted ages and most (85%) mis-classified fish predicted to be 2+ years (Table 2). The next highest successful classification rate was for fish aged 2+ years, with 59% having the same observed and predicted ages and most (94%) mis-classified fish predicted to be 1+ years (Table 2). Overall, the age compositions derived from observed and predicted data were similar with age 1+ being slightly over-estimated, and other age classes slightly under-estimated (Figure 2). The GLMM successfully predicted the age for 67% of all fish (Supplementary Figure 1).
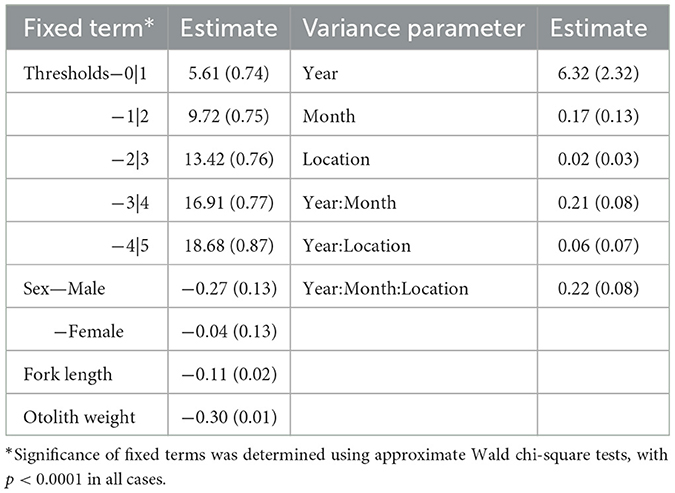
Table 1. Estimates of fixed terms and variance parameters from the GLMM fitted to the Hyporhamphus australis dataset, with standard errors in parentheses.
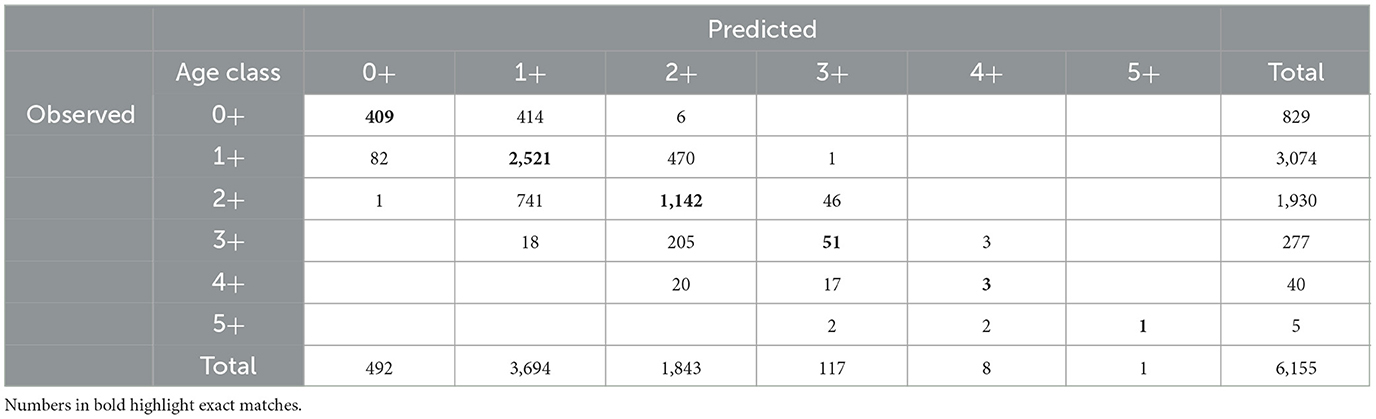
Table 2. Observed (rows) vs. predicted (columns) ages for 6,155 individuals in the Hyporhamphus australis dataset.
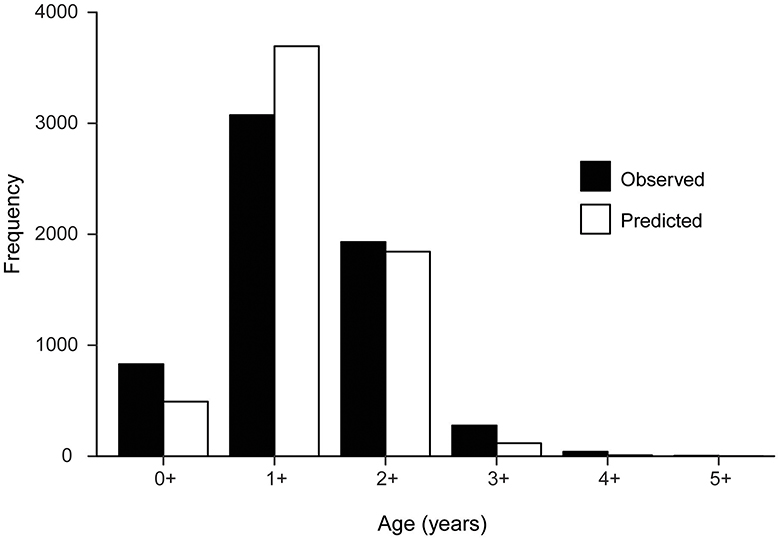
Figure 2. Histograms of observed and predicted ages for 6,155 individuals in the Hyporhamphus australis dataset.
Within age classes there was variability in the otolith weights likely to be associated with successful and unsuccessful prediction of age class (Supplementary Figure 1). Heavier otolith weights in 1+ fish were more likely to be mis-classified; whereas lighter otolith weights in the older (2+ to 5+) age classes were more likely to be mis-classified.
4 Discussion
This study was initiated under the hypothesis that H. australis was an ideal candidate to estimate fish age from otolith weights—a supposition based on the species being relatively fast growing (21) with only few (6) age classes present in samples from commercial landings. However, the model we developed was only partially successful in predicting fish age from otolith weight and covariates sex, fish length, year, month, and location. Age class 1+ had a more than 80% successful prediction rate from the GLMM, but with an upward bias of mis-classifying individuals toward age class 2+. Older age classes tended to exhibit downward bias toward age class 1+. This was likely driven by the predominance of age class 1+ fish in the dataset, representing 50% of all fish sampled. Nonetheless, to the authors' knowledge this is the first attempt at predicting age class in teleosts from otolith weights using a GLMM.
The relationship between fish age and otolith weight is influenced by physiological and environmental factors, including variation in fish growth rates and the deposition of aragonite and organic matter into otoliths, driven by water chemistry, temperature and salinity (5, 28, 29). Our modeling indicated considerable temporal and spatial variability for H. australis, with the random blocking factor year being the greatest source of variation, and month and location also influential. Hyporhamphus australis is a fast-growing species with relatively large otoliths (21) and exhibits considerable inter-annual variability in year-class strength and population size (23, 24). This species also inhabits temperate waters off eastern Australia, a region acknowledged as a global hotspot for ocean warming (30). These factors make it highly likely that H. australis have substantial inter-annual variation in growth rates, which in turn influences the relationship between age and otolith weight.
Our dataset spanned 18 years and provides an important insight into the large effect of inter-annual variability on the predictive capacity of age class from otolith weight. Such limitations could potentially be overcome if future monitoring calibrated the relationship between age class, otolith weight, and covariates (sex and length) for a representative sub-sample from each new sample of fish and was applied only to that year of sampling. Whether such an approach would be cost-effective would need to be considered in terms of how large a sub-set of fish would need to be aged to calibrate the predictive model when, on average, 435 fish have been sampled each year during the past decade.
As hypothesized, and similar to many other species (31), the otoliths of H. australis get heavier with fish age, albeit with considerable overlap in otolith weight distributions between age classes. In addition to temporal effects on the predictive success of the model, it is highly probable that the life-history and otolith appearance of H. australis contributed to the broad range of otolith weights within each year class for at least two reasons. First, H. australis have a protracted (~7 month) spawning season (32), meaning that in combination with variable growth rates (21) fish within the same age class can represent a wide-range of sizes and associated otolith weights. Second, the otoliths of H. australis are difficult to interpret due to the diffuse and inconsistent appearance of the annuli in sections (21). Consequently, aging precision is at the lower end generally reported for teleosts (3, 21), and it is possible that many of the individual samples with incorrect age predictions from the GLMM resulted from inaccurate age estimates during sectioned otolith reads, rather than a failure of the true age-class to otolith weight relationship.
Previous studies have highlighted that precise prediction of age class from otolith weights may not be possible in species that exhibit substantial overlap in the distributions of otolith weights between age classes (19, 33). We also found that predicting the ages of H. australis with high accuracy from otolith weight, including covariates such as sex and fish length, could not be achieved across the entire age-range of samples. Nevertheless, the GLMM produced estimates of expected levels and directional bias for each observed age class, meaning that a simple bias adjustment could be applied to future predictions of age compositions derived from new otolith samples. This approach would substantially reduce the costs associated with the routine age assessment of H. australis but comes with the risk of estimating biased age compositions if the relationship between fish age, otolith weight, sex, and fish length in future years differs to that observed in the 18-year dataset analyzed here. Given the substantial year effect observed in the GLMM, such risk may be considerable. A more precautionary approach may be to recalibrate the model based on a representative subset each year as described above, while continuing to directly age fish with heavier otoliths. This approach may be important as the population of H. australis is continuing to rebuild from a previously overfished state (20), with an expectation that older (>3 years) fish will become increasingly apparent in the landed catch. The species has been reported to attain at least 6 years of age (23), and if similar to its conspecific Hyporhamphus melanochir may attain 10 years (34, 35). Direct age estimation from counting annuli in sectioned otoliths is likely to be the most reliable method of identifying these older individuals.
Beyond the utility of the data presented for H. australis here, deciphering predictive relationships between age classes and otolith weights for other species is likely to benefit fisheries agencies more broadly. Identifying potential candidate species for augmenting direct age estimation from reading sectioned otoliths using otolith weights would substantially reduce processing costs and the financial burden for industry under cost-recovery policies. Species within the Hemiramphidae are typically challenging to age precisely due to the diffuse appearance of annuli within the family (35, 36) and our method of estimating age from otolith weights and other variables would be worth investigating for other species within the family globally.
Data availability statement
The raw data supporting the conclusions of this article will be made available by the authors, without undue reservation.
Ethics statement
Ethical approval was not required for the study involving animals in accordance with the local legislation and institutional requirements because the research was done on dead fish obtained from fish markets to be sold for human consumption.
Author contributions
JS: Conceptualization, Data curation, Formal analysis, Funding acquisition, Investigation, Methodology, Project administration, Resources, Supervision, Writing – original draft, Writing – review & editing. MB: Conceptualization, Formal analysis, Investigation, Methodology, Writing – original draft, Writing – review & editing. DT: Conceptualization, Data curation, Formal analysis, Investigation, Methodology, Writing – original draft, Writing – review & editing.
Funding
The author(s) declare financial support was received for the research, authorship, and/or publication of this article. This study was funded by the New South Wales Department of Primary Industries.
Conflict of interest
The authors declare that the research was conducted in the absence of any commercial or financial relationships that could be construed as a potential conflict of interest.
Publisher's note
All claims expressed in this article are solely those of the authors and do not necessarily represent those of their affiliated organizations, or those of the publisher, the editors and the reviewers. Any product that may be evaluated in this article, or claim that may be made by its manufacturer, is not guaranteed or endorsed by the publisher.
Supplementary material
The Supplementary Material for this article can be found online at: https://www.frontiersin.org/articles/10.3389/frish.2024.1518118/full#supplementary-material
References
1. Campana SE, Thorrold SR. Otoliths, increments, and elements: keys to a comprehensive understanding of fish populations? Can J Fish Aquat Sci. (2001) 58:30–8. doi: 10.1139/f00-177
2. Panfili J, de Pontual H, Troadec H, Wright PJ. Manual of Fish Sclerochronology. Plouzané: Ifremer-IRD coedition (2002). p. 464.
3. Campana SE. Accuracy, precision and quality control in age determination, including a review of the use and abuse of age validation methods. J Fish Biol. (2001) 59:197–242. doi: 10.1111/j.1095-8649.2001.tb00127.x
4. Campana SE. Photographic Atlas of Fish Otoliths of the Northwest Atlantic Ocean (No. 133). Ottawa, ON: NRC Research Press (2004).
5. Lou DC, Mapstone BD, Russ GR, Davies CR, Begg GA. Using otolith weight-age relationships to predict age-based metrics of coral reef fish populations at different spatial scales. Fish Res. (2005) 71:279–94. doi: 10.1016/j.fishres.2004.09.003
6. Carlstrom D. A crystallographic study of vertebrate otoliths. Bio Bull. (1963) 125:441–63. doi: 10.2307/1539358
7. Secor DH, Dean JM, Campana SE. Recent Developments in Fish Otolith Research. Columbia, SC: University of South Carolina Press (1995).
8. Begg GA, Campana SE, Fowler AJ, Suthers IM. Otolith research and application: current directions in innovation and implementation. Mar Freshw Res. (2005) 56:477–83. doi: 10.1071/MF05111
9. Hilborn R, Walters CJ. Quantitative Fisheries Stock Assessment: Choice, Dynamics & Uncertainty. London: Chapman & Hall, International Thomson Publishing (1992).
11. Lambert G, Helser TE, Berger A, Olsen E, Hastie J, O'Malley J. NOAA Technical Memorandum: Importance of Age Data Collection for Stock Assessments: a US National Perspective. Report from the Otolith Sampling Size Working Group (OSSWG) (2017).
12. Siskey MR, Punt AE, Hulson PJF, Bryan MD, Ianelli JN, Thorson JT. The estimated impact of changes to otolith field-sampling and ageing effort on stock assessment inputs, outputs, and catch advice. Can J Fish Aquat Sci. (2022) 80:115–31. doi: 10.1139/cjfas-2022-0050
13. Pawson MG. Using otolith weight to age fish. J Fish Biol. (1990) 36:521–31. doi: 10.1111/j.1095-8649.1990.tb03554.x
14. Wilson CA, Dean MJ, Prince ED, Lee DW. An examination of sexual dimorphism in Atlantic and Pacific blue marlin using body weight, sagittae weight, and age estimates. J Exp Mar Biol Ecol. (1991) 151:209–55. doi: 10.1016/0022-0981(91)90125-G
15. Worthington DG, Fowler AJ, Doherty PJ. Variation in the relationship between otolith weight and age: implications for the estimation of two tropical damselfish (Pomacentrus moluccesis and P. wardi). Can J Fish Aquat Sci. (1995) 52:233–42. doi: 10.1139/f95-023
16. Cardinale M, Arhenius B, Johnsson B. Potential use of otolith weight for the determination of age-structure of Baltic cod (Gadus morhua) and plaice (Pleuronectes platessa). Fish Res. (2000) 45:239–52. doi: 10.1016/S0165-7836(99)00122-8
17. Kwok, KY, Ni I-H. Age and growth of cutlassfishes, Trichiurus spp., from the South China Sea. Fish Bull. (2000) 98:748.
18. Nazir A, Khan MA. Using otolith weight to predict the age of different stocks of Sperata aor (Siluriformes: Bagridae) from the River Ganga. Rev Biol Trop. (2019) 67:534–40. doi: 10.15517/rbt.v67i3.33985
19. Hanson SD, Stafford CP. Modeling otolith weight using fish age and length: applications to age determination. Trans Am Fish Soc. (2017) 146:778–90. doi: 10.1080/00028487.2017.1310138
20. Stewart J. NSW Stock Status Summary 2018/19 - Eastern Sea Garfish (Hyporhamphus australis). NSW Department of Primary Industries Fisheries (2020). p. 9.
21. Stewart J, Hughes JM. Age validation and growth of three commercially important hemiramphids in south-eastern Australia. J Fish Biol. (2007) 70:65–82. doi: 10.1111/j.1095-8649.2006.01256.x
22. McBride RS, Styer JR. Species composition, catch rates, and size structure of fishes captured in the south Florida lampara net fishery. Mar Fish Rev. (2002) 64:21. Available at: https://hdl.handle.net/10535/6962
23. Broadhurst MK, Kienzle M, Stewart J. Natural and fishing mortalities affecting eastern sea garfish, Hyporhamphus australis inferred from age-frequency data using hazard functions. Fish Res. (2018) 198:43–9. doi: 10.1016/j.fishres.2017.10.016
24. Kienzle M, Broadhurst MK, Pletzer A, Stewart J. A Bayesian approach to estimating mortality rates using hazard functions: application to an Australian halfbeak, Hyporhamphus australis fishery. Fish Res. (2021) 243:106066. doi: 10.1016/j.fishres.2021.106066
25. Stewart J, Hughes JM, Gray CA, Walsh C. Life History, Reproductive Biology, Habitat Use and Fishery Status of Eastern Sea Garfish (Hyporhamphus australis) and River Garfish (H. regularis ardelio) in NSW Waters. Cronulla, NSW: NSW Department of Primary Industries (2005).
26. Butler DG, Cullis BR, Gilmour AR, Gogel BJ, Thompson R. ASReml-R Reference Manual Version 4.2 (2023). Available at: https://asreml.kb.vsni.co.uk/wp-content/uploads/sites/3/ASReml-R-Reference-Manual-4.2.pdf (accessed January 1, 2024).
27. Breslow NE, Clayton DG. Approximate inference in generalized linear mixed models. JASA. (1993) 88:9–25. doi: 10.1080/01621459.1993.10594284
28. Degens ET, Deuser WG, Haedrich RL. Molecular structure and composition of fish otoliths. Mar Biol. (1969) 2:105–13. doi: 10.1007/BF00347005
29. Fowler AJ. Annulus formation in otoliths of coral reef fish—a review. In:Secor DH, Dean JM, Campana SE, , editors. Recent Developments in Fish Otolith Research. Columbia, SC: University of South Carolina Press (1995). p. 319–29.
30. Hobday AJ, Pecl GT. Identification of global marine hotspots: sentinels for change and vanguards for adaptation action. Rev Fish Biol Fish. (2014) 24:415–25. doi: 10.1007/s11160-013-9326-6
31. Pacheco C, Bustamante C, Araya M. Mass-effect: understanding the relationship between age and otolith weight in fishes. Fish Fish. (2021) 22:623–33. doi: 10.1111/faf.12542
32. Hughes JM, Stewart J. Reproductive biology of three commercially important Hemiramphid species in south-eastern Australia. Environ Biol Fishes. (2006) 75:237–56. doi: 10.1007/s10641-006-0023-3
33. Fletcher WJ. A test of the relationship between otolith weight and age for the pilchard Sardinops neopilchardus. Can J Fish Aquat Sci. (1991) 48:35–8. doi: 10.1139/f91-005
34. Jones GK. Growth and mortality in a lightly fished population of garfish (Hyporhamphus melanchir), in Baird Bay, South Australia. Trans Roy Soc South Australia. (1990) 114:37–45.
35. Jones GK, Ye Q, Ayvazian S, Coutin P. Fisheries Biology and Habitat Ecology of Southern Sea Garfish (Hyporhamphus melanochir) in Southern Australian Waters. Final Report Project 93/133. Henley Beach, SA: Fisheries Research and Development Corporation (2002). p. 331.
36. McBride RS, Thurman PE. Reproductive biology of Hemiramphus brasiliensis and H. balao (Hemiramphidae): maturation, spawning frequency, and fecundity. Biol Bull. (2003) 204:57–67. doi: 10.2307/1543496
Keywords: otolith weight, teleost, aging, Hyporhamphus, generalized linear mixed model
Citation: Stewart J, Broadhurst MK and Tolhurst DJ (2024) Using otolith weights to estimate age for eastern sea garfish, Hyporhamphus australis. Front. Fish Sci. 2:1518118. doi: 10.3389/frish.2024.1518118
Received: 28 October 2024; Accepted: 18 November 2024;
Published: 06 December 2024.
Edited by:
Periklis Kleitou, Marine and Environmental Research Lab (MER), CyprusReviewed by:
Kelsey James, National Oceanic and Atmospheric Administration, United StatesSalman Khan, Aligarh Muslim University, India
Gary Stringer, University of Louisiana at Monroe, United States
Copyright © 2024 Stewart, Broadhurst and Tolhurst. This is an open-access article distributed under the terms of the Creative Commons Attribution License (CC BY). The use, distribution or reproduction in other forums is permitted, provided the original author(s) and the copyright owner(s) are credited and that the original publication in this journal is cited, in accordance with accepted academic practice. No use, distribution or reproduction is permitted which does not comply with these terms.
*Correspondence: John Stewart, am9obi5zdGV3YXJ0QGRwaS5uc3cuZ292LmF1