- 1Department of Biological Sciences, Purdue University-Fort Wayne, Fort Wayne, IN, United States
- 2The Leatherback Trust, Edificio Los Yoses, San José, Costa Rica
- 3Coonamessett Farm Foundation, East Falmouth, MA, United States
- 4Centro Para el Estudio de Sistemas Marinos (CESIMAR) National Scientific and Technical Research Council (CONICET), Puerto Madryn, Argentina
- 5Department of Natural Resources, Cornell University, Ithaca, NY, United States
Pacific sailfish (Istiophorus platypterus) are the most abundant marlin in Central American waters and are a species of socioeconomic and ecological importance with sport fishing generating millions of dollars (USD) and thousands of jobs each year. Concurrently, sailfish are caught as bycatch in purse seine and longline fishing gear potentially threatening the stability of the population and sportfishing community. In this study, Wildlife Computers Mk10 satellite transmitters were deployed on sailfish (n = 6) which relayed real time Argos satellite locations and post-release light-derived geolocation positional estimates. The two location recording methods produced similar tracking intervals—deployment date until the final location date—(Argos: 33.0 ± 13.5 d; GPE: 32.0 ± 11.2 d), and detection days—number of days the transmitter recorded a location—(Argos: 7.8 ± 6.0 d; GPE: 12.3 ± 8.5 d). In total, displacement distances from initial tagging to final (Argos) location ranged from 339.92 to 985.59 km and crossed 6 different Exclusive Economic Zones. During migrating, sailfish exhibited alternating with-current and against-current movements, a pattern that was consistent in both the upwelling and non-upwelling seasons. Despite the known fluctuations associated with seasonal upwelling in the eastern Pacific, sailfish experienced relatively stable microenvironments with average temperature variability remaining within 2°C. Behavioral modification to achieve this consistency could be through depth use (48 ± 28 m vs. 37 ± 47 m), though this mechanism alone seems unlikely to fully explain their ability to mitigate environmental dynamics. Further research is needed to understand the mechanisms underlying these behavioral adaptations and the ecological factors that contribute to sailfish resilience. Additionally, strengthened protection measures are critical to ensure the conservation of sailfish in Costa Rica, including elimination of all commercial sale.
Introduction
In Pacific Costa Rica, the recreational fishing industry—an industry that generates over $520 million USD and attracts over 150,000 tourists annually pre COVID19— is, in part, supported by sailfish (Istiophorus platypterus) (1, 2). Despite the apparent health of this industry, these billfish were listed as vulnerable by IUCN in 2022 (1, 3, 23). Sport fishing has a long history in this area, but trophy size decreased by 35% between the 1960s and early 2000s (4). Subsequently Article 76 of Costa Rica's Fisheries and Aquaculture Law 8436 (2005) listed sailfish as a species of tourist-sport interest and prohibited commercial fisheries from targeting sailfish, requiring that they release any live sailfish they catch, and reduced incidental mortality from 15 to 10% (INCOPESCA and FECOP). Management techniques implemented over the last decade have had some success in reducing mortality rates in tunas and billfishes (5), however these efforts are not sufficient. While not a lucrative meat, Sailfish is still common in Central American and foreign markets (2, 4) and landing rates of sailfish are currently above estimated natural mortality rates (6, 7). To address this, dedicated organizations are pushing for a total ban on sailfish sale, notably Operation Sailfish [(2); FECOP]; however, advancing conservation requires a deeper understanding of sailfish biology and ecology, particularly their habitat use and response to environmental variability.
Sailfish are highly migratory, traveling 1000′s of kilometers from tagging locations (8–12), yet research suggests they may also exhibit habitat fidelity and possibly seasonal shuttling behavior (13). Their movements are influenced by oceanographic features, particularly water temperature and dissolved oxygen. Sailfish prefer warmer waters where the thermocline is deeper, providing access to oxygen-rich water (2, 14–16, 24). While they can make transient dives into deeper, colder, water (9), this ability appears to be limited by an 8°C change in water temperature (9, 12, 17). Billfish have some ability to maintain body temperature when diving, however 15°C is when other large pelagic predators like tunas reach a point at which they can no longer respond to the need for rapid movement such as catching prey or escaping a predator (17). The thermal range for Pacific sailfish, as compiled by Boyce et al. (18), has an average minimum of 20.5°C and an average maximum of 27.85°C, with a preferred temperature range of 25–27.8°C. Sailfish in the Pacific are not just confined to the surface by temperature, they are also limited by dissolved oxygen and the hypoxic environment that often accompanies this colder water (14). Therefore, we assume that sailfish will be sensitive to the annual fluctuations that accompany the seasonal upwelling along the coastline and in the Costa Rica Dome (2, 14, 19, 20). The Costa Rica Dome is a distinct biological habitat in the eastern Pacific with seasonally predictable variability in temperature, and the biomass of phytoplankton and zooplankton are higher than surrounding water (20). Understanding how sailfish use this dynamic habitat and respond to seasonal oceanographic changes is critical to informing effective conservation measures, particularly in the face of increasing human pressures and climate variability.
Here we report satellite telemetry data from Pacific sailfish, a vulnerable and regionally important population (2, 24, 25), tagged in 2008 and 2009 in the offshore waters near Costa Rica. We used Argos-linked tags to relay real time locations whenever the sailfish was basking at the surface, in addition to post-hoc geolocation positional estimates (GPE; a more common way to track non-air breathing animals) to track Pacific sailfish captured on sportfishing boats during the upwelling and non-upwelling seasons. We predict that sailfish will modify their behavior with season and ocean current direction. Given their localized abundance, economic importance, and vulnerable status, we aim to add to the currently limited understanding on the movement ecology of Pacific sailfish to improve conservation efforts.
Methods
From June 2008 through June 2009, we attached nine satellite transmitters to Pacific sailfish (Figure 1) captured from recreational fishing vessels off the coast of Costa Rica (Figure 2; Table 1). Fish were captured by sport-fishing teams using conventional tackle and were then held in the water near the boat for transmitter attachment. We attached the transmitter by inserting a nylon anchor into the muscle, posterior to the head and ventral to the dorsal sheath. The anchor system consisted of a hydroscopic needle being used to pass monofilament line medio-laterally through the fleshy ridge forming the dorsal fin sheath, with a silicon button acting as an anchor within the sheath. The placement ensures that the extension and retraction of the dorsal fin was not affected. The fish was then reinvigorated by slowly towing it beside the boat, which facilitated ram ventilation (26).
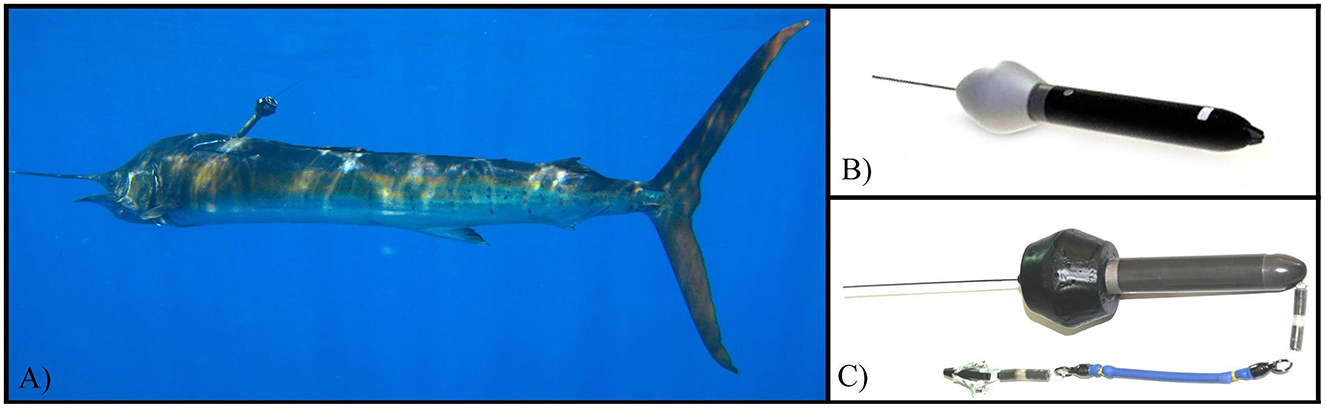
Figure 1. Views of modified pop-up archival tags. (A) Modified pop-up transmitter deployed on Pacific sailfish. Flotation collars provide buoyancy to allow real-time transmission as well as positioning the tag above the body reducing irritation as the fish swims. (B) A depiction of an unmodified or “stock” Mk10 pop-up archival transmitter (Wildlife Computers). (C) A modified Mk10 pop-up archival transmitter. Notice increased flotation compared to (B) and shock absorbing tether.
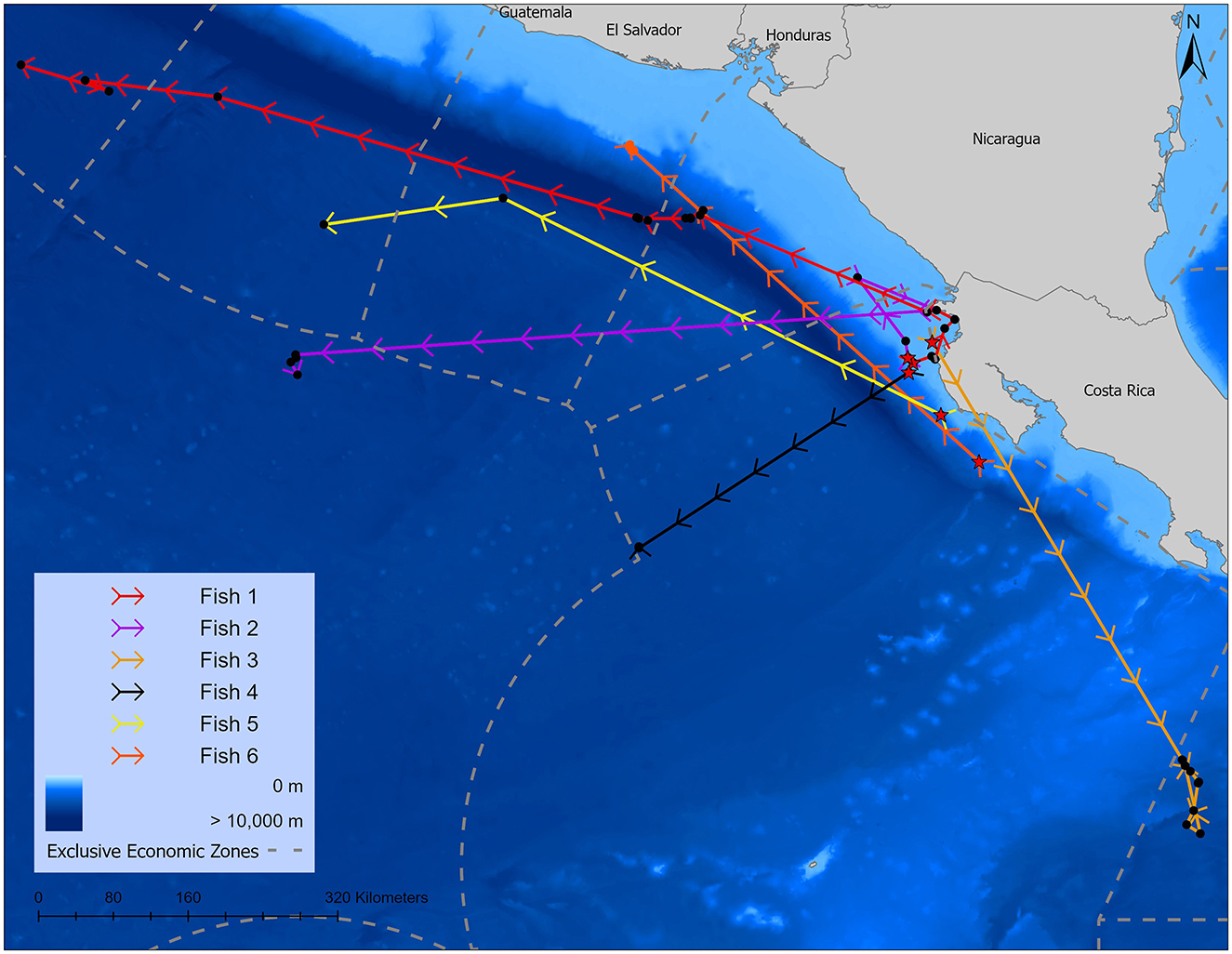
Figure 2. Tracks of Argos locations from sailfish tagged in 2008–2009 in Costa Rica, stars indicate capture location. Map was made in ArcGIS Pro using GADM shapefiles (https://gadm.org/index.htm), GEBCO bathymetry, and Exclusive Economic Boundaries shapefile (http://www.marineregions.org).
For this study we programmed nine pop-up archival satellite transmitters (Mk10, Wildlife Computers Inc. Redmond, WA) to provide real time data whenever the fish swam slowly near, or basked at the surface, in addition to archival data to transmit on a determined release date (day 250), max depth (±4 m), and temperature (every 10 s, ±0.1°C). Each transmitter was fitted with a flotation collar, providing approximately 70 grams of additional buoyancy, encouraging an upright posture and increasing the height of the antenna above the water line when at the surface (Figure 1).
For Argos locations, data are reported with various location qualities (LC) with accuracies ranging from 150 m to more than 1 km (LC = 3, 2, 1, 0; respectively), with other location qualities of unknown accuracies (LC = A, B; Argos, 20081). We used the best LC for each day of data to get one point per day. In addition to these, we estimated locations derived from contemporary light-based geolocation techniques using light data that was recorded by each tag during the deployment interval. Geolocation positional estimates (GPE locations) were created using the tag manufacturer's proprietary software, WC-GPE2 (Version: 2.00.0027 11-Apr-2014), which compares transmitter light to known dusk and dawn, then we averaged the dawn and dusk estimates such that one location per day remained.
Then, we calculated the distance between the two consecutive points and the east-west distance using the Haversine equation (used to calculate distance on a sphere):
where φ is the latitude and λ is the longitude, and R is earth's radius (mean radius = 6,371 km). Here, A and B signify the two consecutive location points. Together these segments allowed us to calculate track cumulative length, and the angle from due East.
We used ArcGIS Pro (Version 3.2.1, 2023, Esri, Redlands, CA, USA) to overlay location data with satellite imagery of bathymetry (GEBCO 2023, 15 arc-second interval grid, https://www.gebco.net), and exclusive economic zone boundaries (http://www.marineregions.org). We calculated ocean current direction by using data from Copernicus Marine Services (https://marine.copernicus.eu) and ArcGIS Pro to transform vector information into the angle from due East. Specifically, we used a 0.083° grid of the northward ocean velocity and the eastward ocean velocity, and within ArcGIS Raster Calculator, we calculated the direction of the ocean current in each cell. We then were able to average the ocean current direction experienced by the sailfish over the duration of its track. Next, we calculated the cosine similarity between the two angles using:
where θ1 and θ2 are angles, calculated from due East, for the sailfish track and ocean current movement at the track segment, respectively. An angle close to 0° or 360° indicates a direction of movement that is east, an angle of ~180° indicates a westward movement direction. A cosine similarity of −1 means the movement direction of the fish was directly against the direction of ocean current movement. A cosine similarity of 1 indicates that the fish was moving in the same direction as the ocean currents. We then weighted the SC by the line segment length.
Upwelling in Pacific Costa Rica usually begins between November and December and lasts through March or April. For the purposes of this study, fish tracked in January–March were tracked during the upwelling season, and fish tracked during June–August were tracked during the non-upwelling season. One fish was tracked from March into April, which is the transition from the upwelling to the non-upwelling season (Fish 6).
Results
Six of the nine transmitters relayed usable Argos satellite locations and light-loc geolocations (Table 1; Figure 2; Supplementary Figure 1). The mean (±SD) tracking interval—defined as the deployment date until the final location date—was 33.0 ± 13.5 days (Argos) and 32.0 ± 11.2 days (GPE), however, our detection days—defined as the number of days the transmitter recorded a location—were fewer at 7.8 ± 6.0 (Argos) and 12.3 ± 8.5 (GPE) days. In total, displacement distances from initial tagging to final (Argos) location ranged from 339.92 to 985.59 km with a mean displacement of 606.75 ± 217.26 km (Table 1). During the tracking interval, Argos locations placed the fish in waters belonging to six different countries (Exclusive Economic Zones) and in international waters (Figure 2). GPE locations, commonly used in non-air breathing animals, are included for reference, but we felt they did not add depth to this discussion; therefore, we have only included them as Supplementary material.
During both the upwelling season (n = 2; weight SC = 0.17) and the non-upwelling season (n = 4; weight SC = 0.055), the direction of sailfish movement, as derived from Argos data, was nearly perpendicular to the ocean current direction but showed a slight alignment in the same general direction (Figure 3). Taken together, the average weighted cosine similarity between Argos derived track direction and ocean current direction was 0.09 ± 0.55, meaning the with-current movements of the fish occurred as often as the against-current movements of the fish. Using temperature readers onboard the transmitters, thermal environments experienced in the non-upwelling seasons (June–August) were in the ~28°C range while fish experienced thermal environments up to 2°C colder on average in the upwelling season (January–March). Specifically, average monthly transmitter temperature recordings were 28.6 ± 0.3°C (Jun 2008), 28.1 ± 0.4°C (Jul 2008), 28.0 ± 0.3°C (Aug 2008), 27.4 ± 0.7°C (Jan 2009), 27.1 ± 0.8°C (Feb 2009), 27.3 ± 1.2°C (Mar 2009), and 29.0 ± 0.2°C (Apr 2009). While the maximum dive depth (256 m) was recorded during the non-upwelling season, on average, dive depth was deeper in the upwelling season (Jan–March 2009; 48 ± 28 m, n = 111), compared to the previous non-upwelling season (Jun–Aug 2008; 37 ± 47 m, n = 186). Fish 6 did not appear to change its dive pattern across the tracking period, despite the potential shift in upwelling strength.
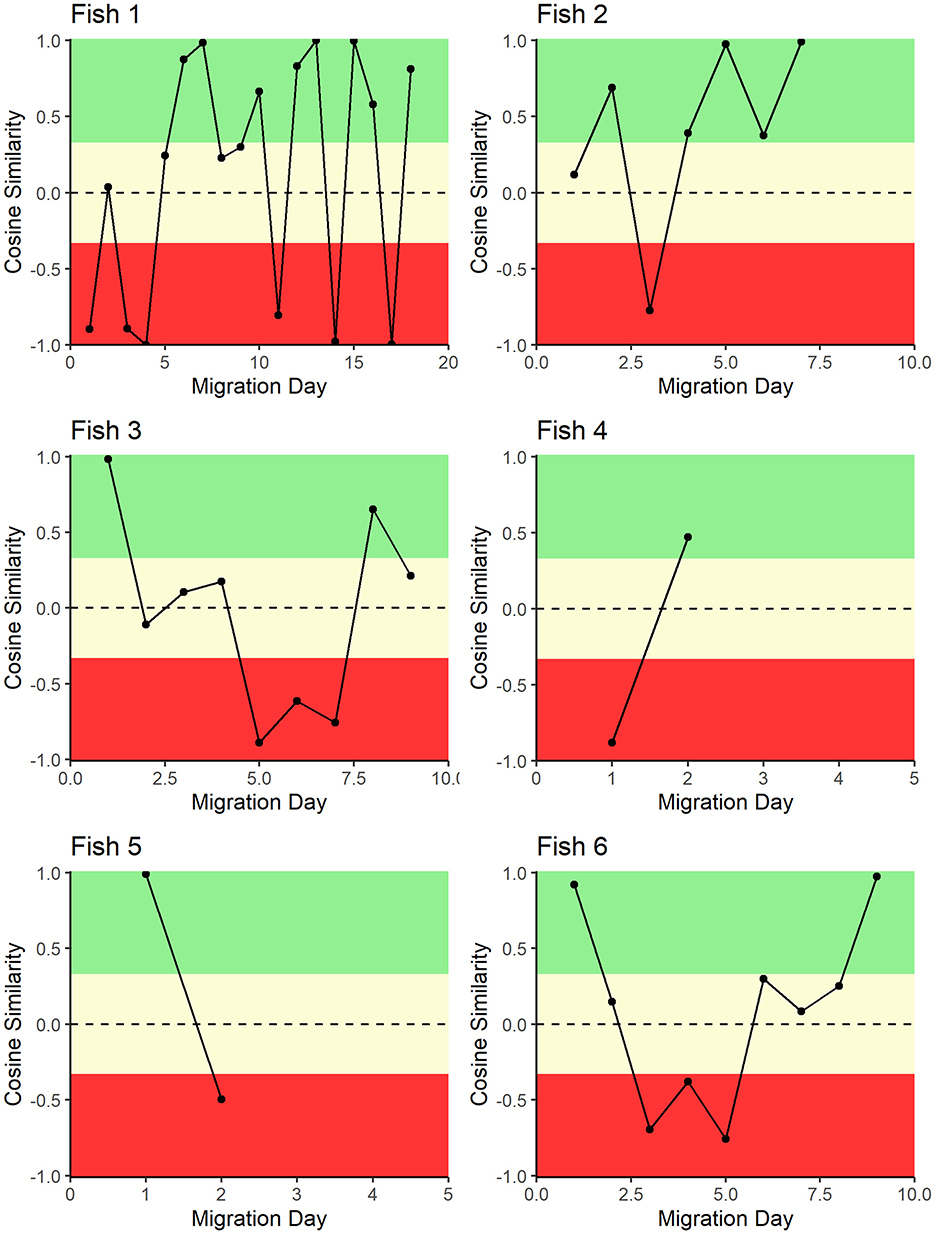
Figure 3. Daily cosine similarity between sailfish migration direction and ocean current direction. Cosine similarity close to +1 indicates that the direction of fish movement was with ocean currents, whereas a cosine similarity close to −1 indicates fish movement direction against ocean currents. In the figure, sections in green were designated as generally with the currents, sections in yellow were designated as generally across the currents, and sections in red were generally against ocean currents. Each panel represents the migration of a single fish.
Discussion
In 2008 and 2009, we used Wildlife Computers Mk10 satellite transmitters to relay real time Argos locations of Pacific sailfish across the Exclusive Economic Zones (EEZ) of six countries and international waters. Our findings highlight the critical need for multinational cooperation to protect the health of sailfish populations, particularly as countries like Costa Rica economically and socially depend on them (1). While neighboring countries—including Mexico, Guatemala, El Salvador, Nicaragua, and Panama—have taken steps to extend special protection to sailfish by either completely banning the sale of billfish or extending longline fishing bans to 75 miles offshore [for an overview of these fishing laws see (2)], Costa Rica lags behind in implementing similar protections (1, 8). This is particularly concerning given Costa Rica has a very large EEZ (574,725 km2), where 7% of Argos locations (one out of 14 transmissions) occurred outside the 30-mile protected zone, putting these fish at increased risk from commercial fishing operations during these times.
The observed transmission bias may be partly explained by fish behavior. While within the 30-mile protected area, sailfish spent more time near the surface (shallower max dive depths), allowing for increased transmission opportunities. In contrast, during eastward migration, increased max dive depths reduced transmission frequency, potentially underestimating the percentage of sailfish locations outside protected zones. Further, increased dive depth may indicate foraging behavior which would further increase the chance that fish may interact with fishing gear.
Our results align with those of Marrari et al. (2), indicating that sailfish adjust their movements to maintain a stable thermal environment despite seasonal oceanographic changes characteristic of the Eastern Pacific. In the Eastern Pacific, the non-upwelling season features well-formed coastal currents, warmer surface temperature (29°C) and reduced pelagic primary productivity (19). As a result, sailfish might adopt roaming behavior as prey distribution becomes less predictable and cold-water avoidance is unnecessary. In the upwelling season, the water movement direction is primarily offshore, driving upwelling of cold water from below the thermocline causing surface temperature to decrease (25°C) while primary productivity increases (20). Under these conditions we anticipated behavioral shifts to capitalize on the increased productivity, however, no seasonal differences in migration patterns were observed between fish tracked in the non-upwelling season (Fish 1–4) and those tracked in the upwelling season (Fish 5, 6). Interestingly, despite the background currents' potential to influence fish displacement and movement direction, individuals tagged within a short period and in similar locations (Fish 1–3) exhibited divergent migration pathways, ranging from northward to southward trajectories. This variability could reflect individual-level differences in behavior or habitat preference, potentially influenced by localized prey availability, internal physiological states, or micro-scale oceanographic features not captured in our tracking data. While sailfish generally exhibit energy-conserving behaviors, such as aligning movements with prevailing currents, this divergence highlights the complexity of their movement ecology and suggests that broad-scale ocean current patterns alone may not fully explain their displacement. Fine-scale environmental drivers, such as thermal gradients, eddies, or prey distribution, may play a more prominent role in guiding sailfish movement on short time scales. These patterns warrant further investigation, considering Marrari et al. (2) found intra-annual variability in capture abundance from sportfishing vessels with higher capture rates in the upwelling season and lower capture rates in the non-upwelling season over the last 10 years.
Sailfish movements during our tracking period appear to reflect an energy-conserving strategy in response to ocean currents. Insofar as the cosine similarity between the direction of fish movement and the direction of ocean current movement displays a switching behavior where fish altered their relative position to move with the prevailing currents while swimming. Again, we did not observe seasonal patterns in this behavior. It is important to note that these analyses relied on Argos data, which are limited to surface positions when fish were within approximately one meter of the ocean surface (close enough to allow transmission between the Mk10 and the satellite) and the resulting location had to be of high enough quality to use (more than four messages). As such, our data lacks high temporal resolution (e.g., hourly or daily), potentially missing fine-scale movements, such as within-eddy or short-term current-following behaviors. These behaviors may play a critical role in optimizing movement strategy while navigating oceanographic features like sea surface temperature, salinity, oxygen, and chlorophyll concentrations (16, 21). Despite these limitations, models suggest that sailfish maintain consistent behavioral patterns even under El Niño Southern Oscillation (ENSO) influences (22).
The continuity of these movement patterns over 15 years, despite increasing anthropogenic pressures such as fishing, boat traffic, tourism, and pollution, has important conservation implications. It suggests that sailfish in this region are resilient and continue to rely on critical habitats in the area. However, this resilience may not indicate that the region is free from stress, rather, the persistence of these behaviors could underscore the region's importance as a biodiversity hotspot, where conditions are suitable for multiple species despite ongoing pressure. The observed stability in sailfish movements, even during ENSO phases, raises questions about how future climate change impacts, such as shifts in ocean temperature, currents, and productivity might influence these patterns. While the region may currently function as a refuge, these findings highlight the need for proactive management to ensure long-term habitat integrity.
Since the collection of these data, commercial sailfish catch volumes have continued to increase (2). Regional fisheries management organizations, such as the Inter-American Tropical Tuna Commission in the Eastern Pacific, regulate sailfish stocks within their respective jurisdictions. While other countries in Central and North America have implemented additional protections for sailfish, Costa Rica remains behind in adopting similar measures. Our study shows that sailfish use coastal zones with fishing gear restrictions, however, they also occupy pelagic waters and international waters where such protections are largely absent. Sailfish behavior—including shallow diving behavior association with highly productive zones, and basking at the surface—make them especially vulnerable to fishing gear (1, 9, 24).
In conclusion, we observed that sailfish experienced <2°C variability in their thermal environment despite dynamic oceanic environments, and appear to optimize energy expenditure by aligning movements and behavior with ocean current direction. However, significant knowledge gaps remain, particularly regarding the fine-scale interactions between billfish behavior and oceanographic features. To address this, we recommend future research focus on integrating the traditional ecological knowledge of recreational and artisanal fishermen, who may have knowledge and insights into fish ecology, controlled physiological studies examining the response of sailfish to thermal environments and ocean currents, similar studies to ours with finer-scale fish location data, and predictive modeling that incorporates passive and active ocean current-fish relationships when predicting habitat use and movement. We believe these research directions will help improve the applicability of conservation strategies and ensure the long-term viability of sailfish populations in the Eastern Pacific. The persistence of sailfish movement patterns further emphasizes the ecological importance of this region and the need to address both localized and global threats, such as climate change, to safeguard these critical habitats.
Data availability statement
The raw data supporting the conclusions of this article will be made available by the authors, without undue reservation.
Ethics statement
The animal study was approved by Purdue University Animal Care and Use Committee (protocol number 1111000251). The study was conducted in accordance with the local legislation and institutional requirements.
Author contributions
CC-B: Data curation, Formal analysis, Methodology, Writing – original draft, Writing – review & editing. SP: Conceptualization, Data curation, Formal analysis, Investigation, Methodology, Supervision, Writing – original draft, Writing – review & editing. GB: Formal analysis, Methodology, Project administration, Supervision, Writing – original draft, Writing – review & editing. SF: Investigation, Methodology, Writing – review & editing. SM: Conceptualization, Funding acquisition, Investigation, Project administration, Resources, Supervision, Writing – original draft, Writing – review & editing. FP: Conceptualization, Data curation, Formal analysis, Funding acquisition, Investigation, Methodology, Project administration, Resources, Supervision, Writing – original draft, Writing – review & editing.
Funding
The author(s) declare financial support was received for the research, authorship, and/or publication of this article. This study was supported by Purdue University Fort Wayne, Cornell University, and The Leatherback Trust through the professors.
Acknowledgments
The authors thank the members of the sportfishing community who helped us deploy transmitters and educated us about the need for sailfish conservation. There is an endless list of crews and teams that allowed us on their charters and this project would not have been possible without their support. Their dedication to conservation is inspiring. We thank Dr. P. Santidrian Tomillo and R. Piedra for help with permitting and the Costa Rican government for permits to conduct conservation research. We thank C. Kent-Dotson for help with data analysis, and Dr. J. Spotila for advising. Some paragraphs were edited for grammar and clarity using open AI's Chat GPT 4.0 as a final edit assistance.
Conflict of interest
The authors declare that the research was conducted in the absence of any commercial or financial relationships that could be construed as a potential conflict of interest.
Publisher's note
All claims expressed in this article are solely those of the authors and do not necessarily represent those of their affiliated organizations, or those of the publisher, the editors and the reviewers. Any product that may be evaluated in this article, or claim that may be made by its manufacturer, is not guaranteed or endorsed by the publisher.
Supplementary material
The Supplementary Material for this article can be found online at: https://www.frontiersin.org/articles/10.3389/frish.2024.1476026/full#supplementary-material
Footnotes
References
1. Cascante A, Marín H. El Aporte macroeconómico y local de la pesca deportiva en Costa Rica. San José: FECOP (2019).
2. Marrari M, Chaves-Campos J, Villanueva MM, Martinez-Fernandez D, Sandoval HM, Meier TS. Trends and variability in local abundances of sailfish Istiophorus platypterus in Pacific waters of Costa Rica: controls and effects on recreational fisheries. Front Mar Sci. (2023) 10:88006. doi: 10.3389/fmars.2023.1088006
3. Collette BB, Di Natale A, Fox W, Graves J, Juan Jorda M, Pohlot B, et al. Istiophorus platypterus. The IUCN Red List of Threatened Species. (2022) e.T170338A46649664. doi: 10.2305/IUCN.UK.2022-1.RLTS.T170338A46649664.en
4. Erhardt NM, Fitchett MD. On the seasonal dynamic characteristics of the sailfish, Istiophorus platypterus, in the Eastern Pacific off Central America. Bull Mar Sci. (2006) 79:589–606.
5. Juan-Jordá MJ, Murua H, Arrizabalaga H, Merino G, Pacoureau N, Dulvy NK. Seventy years of tunas, billfishes, and sharks as sentinels of global ocean health. Science. (2022) 378:eabj0211. doi: 10.1126/science.abj0211
6. Myers RA, Worm B. Rapid worldwide depletion of predatory fish communities. Nature. (2003) 423:280–3. doi: 10.1038/nature01610
7. Pohlot BG, Ehrhardt N. An analysis of sailfish daily activity in the Eastern Pacific Ocean using satellite tagging and recreational fisheries data. ICES J Mar Sci. (2018) 75:871–9. doi: 10.1093/icesjms/fsx082
8. Prince ED, Holts DB, Snodgrass D, Orbesen ES, Luo J, Domeier ML, et al. Transboundary movement of sailfish, Istiophorus platypterus, off the Pacific coast of Central America. Bull Mar Sci. (2006) 79:827–38.
9. Chiang WC, Musyl MK, Sun CL, Chen SY, Chen WY, Liu DC, et al. Vertical and horizontal movements of sailfish (Istiophorus platypterus) near Taiwan determined using pop-up satellite tags. J Exp Mar Bio Ecol. (2011) 397:129–35. doi: 10.1016/j.jembe.2010.11.018
10. Reygondeau G, Maury P, Beaugrand G, Fromentin JM, Fonteneau A, Cury P. Biogeography of tuna and billfish communities. J Biogeogr. (2012) 39:114–29. doi: 10.1111/j.1365-2699.2011.02582.x
11. Filous A, Friedlander AM, Toribiong M, Lennox RJ, Mereb G, Golbuu Y. The movements of yellowfin tuna, blue marlin, and sailfish within the Palau National Marine Sanctuary and the western Pacific Ocean. ICES J Mar Sci. (2022) 79:445–56. doi: 10.1093/icesjms/fsac010
12. Thoya P, Kadagi NI, Wambiji N, Williams SM, Pepperell J, Möllmann C, et al. Environmental controls of billfish species in the Indian Ocean and implications for their management and conservation. Divers Distrib. (2022) 28:1554–67. doi: 10.1111/ddi.13525
13. Macías-Zamora R, Olivos-Ortiz A, Vidaurri-Sotelo AL, Carrasco-Águila MÁ, Torres-Orozco E. Spatial explicit model for seasonal migration of sailfish (Istiophorus platypterus) in the Mexican Pacific. Hidrobiológica. (2011) 21:126–34.
14. Prince ED, Goodyear CP. Hypoxia-based habitat compression of tropical pelagic fishes. Fish Oceanogr. (2006) 15:451–64. doi: 10.1111/j.1365-2419.2005.00393.x
15. Mourato BL, Carvalho F, Musyl M, Amorim A, Pacheco JC, Hazin H, et al. Short-term movements and habitat preferences of sailfish, Istiophorus platypterus (Istiophoridae), along the southeast coast of Brazil. Neotrop Ichthyol. (2014) 12:861–70. doi: 10.1590/1982-0224-20130102
16. Ferrette BLDS, Mourato B, Hazin FHV, Arocha F, Williams SM, Rodrigues Junior CE, et al. Global phylogeography of sailfish: deep evolutionary lineages with implications for fisheries management. Hydrobiologia. (2021) 848:3883–904. doi: 10.1007/s10750-021-04587-w
17. Brill RW, Lowe TE, Cousins KL. How water temperature really limits the vertical movements of tunas and billfishes–it's the heart stupid. In: International Congress on Biology of Fish. American Fisheries Society, Towson University. Towson, MD (1998). p. 4.
18. Boyce DG, Tittensor DP, Worm B. Effects of temperature on global patterns of tuna and billfish richness. Mar Ecol Prog Ser. (2008) 355:267–76. doi: 10.3354/meps07237
19. Fiedler PC, Philbrick V, Chavez FP. Oceanic upwelling and productivity in the eastern tropical Pacific. Limnol Oceanogr. (1991) 36:1834–50. doi: 10.4319/lo.1991.36.8.1834
20. Fiedler PC. The annual cycle and biological effects of the Costa Rica Dome. Deep Sea Res I. (2002) 49:321–38. doi: 10.1016/S0967-0637(01)00057-7
21. Crespo-Neto O, Díaz-Delgado E, Acosta-Pachón TA, Martínez-Rincón RO. Spatial segregation by size of billfishes bycaught by the tuna purse-seine fishery in the Eastern Pacific Ocean. Fish Res. (2021) 241:106001. doi: 10.1016/j.fishres.2021.106001
22. Martinez-Rincon RO, Ortega-Garcia S, Vaca-Rodriguez JG, Griffiths SP. Development of habitat prediction models to reduce by-catch of sailfish (Istiophorus platypterus) within the purse-seine fishery in the eastern Pacific Ocean. Mar Freshwater Res. (2015) 66:644–53. doi: 10.1071/MF14062
23. Holland SM, Ditton RB, Graefe AR. An ecotourism perspective on billfish fisheries. J Sustain Tourism. (1998) 6:97–116. doi: 10.1080/09669589808667305
24. Haulsee DE, Blondin HE, Logan RK, Crowder LB. Where do the billfish go? using recreational catch data to relate local and basin scale environmental conditions to billfish occurrence in the Eastern tropical pacific. Fish Oceanogr. (2022) 31:135–48. doi: 10.1111/fog.12567
25. Heberer L, Nasby-Lucas N. The SWFSC 2020 Billfish Newsletter. SWFSC Cooperative Billfish Tagging Program, California (2021).
Keywords: cosine similarity, fisheries, satellite telemetry, migration, geolocation positional estimates, Costa Rica
Citation: Clyde-Brockway CE, Patel SH, Blanco G, Friederichk SJ, Morreale S and Paladino FV (2025) Pacific sailfish (Istiophorus platypterus) in the Eastern Pacific Ocean, association with ocean currents and seasonal effects of upwelling using real-time Argos locations. Front. Fish Sci. 2:1476026. doi: 10.3389/frish.2024.1476026
Received: 05 August 2024; Accepted: 23 December 2024;
Published: 21 January 2025.
Edited by:
Kevin J. Hedges, Fisheries and Oceans Canada (DFO), CanadaReviewed by:
Eloisa Pinheiro Giareta, Federal University of Paraná, BrazilCharles Bangley, Dalhousie University, Canada
Copyright © 2025 Clyde-Brockway, Patel, Blanco, Friederichk, Morreale and Paladino. This is an open-access article distributed under the terms of the Creative Commons Attribution License (CC BY). The use, distribution or reproduction in other forums is permitted, provided the original author(s) and the copyright owner(s) are credited and that the original publication in this journal is cited, in accordance with accepted academic practice. No use, distribution or reproduction is permitted which does not comply with these terms.
*Correspondence: Chelsea E. Clyde-Brockway, Y2hlbHNlYUBsZWF0aGVyYmFjay5vcmc=
†ORCID: Chelsea E. Clyde-Brockway orcid.org/0000-0001-7713-8348
Samir Harshad Patel orcid.org/0000-0002-2177-5038
Gabriela Blanco orcid.org/0000-0001-6794-4207
Stephen Morreale orcid.org/0000-0003-3249-5346
Frank V. Paladino orcid.org/0000-0002-6452-0086