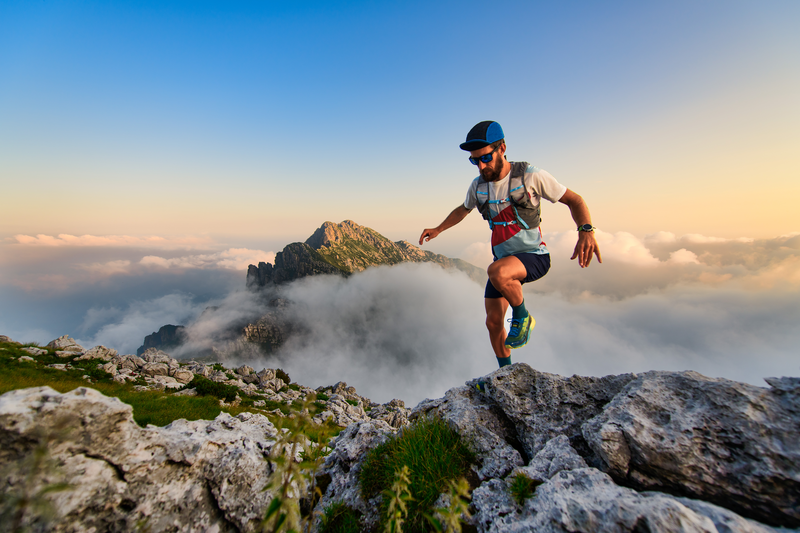
94% of researchers rate our articles as excellent or good
Learn more about the work of our research integrity team to safeguard the quality of each article we publish.
Find out more
ORIGINAL RESEARCH article
Front. Fish Sci. , 21 August 2024
Sec. Fish Ecology
Volume 2 - 2024 | https://doi.org/10.3389/frish.2024.1448809
Parrotfishes (family Scaridae) are important to coral reef ecosystems and highly prized by both commercial and recreational fishers in the Hawaiian Islands. Although parrotfishes are known to be strictly diurnal, our knowledge of their habitat use and movement patterns across daily timescales in tropical systems is still somewhat limited. Here, acoustic telemetry was used to determine parrotfish habitat use and movements within a coral reef seascape at Puakō, Hawai‘i. An array of acoustic receivers was deployed in a nearshore reef to track fine-scale movements of two common species: ember parrotfish (Scarus rubroviolaceus) and palenose parrotfish (Scarus psittacus). Transmitters were mounted externally on fish and tracked over a four-week period using an acoustic positioning system. Coral habitats (reef flat, fore reef) within the study area were mapped and used in conjunction with triangulated positions to characterize habitat use and movements. Findings indicated that both species commonly inhabited the fore reef habitat during the day (91.6% and 95.0% of total detections, respectively), spending limited time in the reef flat during the day and no time in this habitat at night. Activity spaces (based on 95% and 50% kernel utilization distributions) indicated that home ranges and core use of both species were significantly larger during the day than night, with daytime home ranges (mean ±1 SD) significantly greater for ember parrotfish (8,712 ± 1,991 m2) than palenose parrotfish (3,725 ± 1,254 m2). Hourly mean detections peaked during crepuscular periods for palenose parrotfish and to a lesser extent for ember parrotfish, which was linked to movements between shallower daytime (foraging) and nighttime (resting) areas. Kernel utilization distributions indicated that both species occupied similar core use areas of the seascape (overlap index > 0.5), with both intra- and interspecific overlapping distributions present. Finding from this study highlight the value of the fore reef habitat for both daytime foraging and nocturnal resting activities of ember parrotfish and palenose parrotfish. This study also affords valuable information on primary activity spaces of both species as well as overlap between these two common congeners, which is essential for developing management strategies to conserve parrotfish populations.
Coral reefs represent some of the most complex ecosystems on the planet, supporting high levels of productivity and biodiversity (1, 2). For the Hawaiian Islands, coral reefs play a key role in the marine ecosystem and local culture due to their biological significance and economic value. The Hawaiian Islands are one of the most remote coral reef systems in the world, and reefs are home to a high percentage of endemic species (3). The shore fishes of the main islands of Hawai‘i have an estimated 23% endemism (4). Coral reefs in Hawai‘i are estimated to be worth nearly 10 billion dollars, bringing in millions of dollars each year in marine tourism industry alone (5, 6). However, rapid population growth is causing a drastic increase in anthropogenic influences, resulting in negative impacts to the health of coral reef ecosystems in the Hawaiian Islands (3).
Parrotfishes are important components of tropical seascapes throughout the Hawaiian Islands and critical to the health and resilience for coral reef ecosystems throughout the world (7–9). As herbivores, parrotfishes graze heavily on algal turfs and macroalgae (10, 11) but also serve as primary agents of bioerosion on reefs by scraping away algae and associated matter from the calcium carbonate reef matrix (12–14). Parrotfishes are known to actively forage during the day in tropical seascapes but our understanding of habitat requirements and movements on coral reefs is lacking for many species. In addition, the scale of diurnal movements (i.e., home range) is unresolved for parrotfishes in many parts of their geographic range, and this information is critical for implementing conservation measures and establishing the spatial configuration of marine protected areas.
The aim of this study was to characterize the habitat use and movements of two common parrotfishes (ember or bicolor parrotfish Scarus rubroviolaceus [hereafter ember], palenose parrotfish Scarus psittacus) on the leeward coast of Hawai‘i using acoustic telemetry. Both species inhabit nearshore coral reefs and are targeted in commercial and non-commercial fisheries. Previous studies have shown that the relative abundance of parrotfishes and other herbivorous fishes in this region is high in comparison to other heavily fished reefs in the Pacific Ocean (15). This study was performed on a relatively pristine coral reef complex off Puakō, a dedicated Fisheries Management Area (FMA) that has elevated live coral cover compared to other reefs in the Hawaiian Islands (16, 17). Here we hypothesize that parrotfish will exhibit differences in habitat use among shallow reef flat, shallow fore reef, and deeper areas of the fore reef. Additionally, we expect to see differences in the home ranges and core areas between the two parrotfish species and therefore do not expect interspecific spatial overlap.
The study was conducted at Puakō in the district of South Kohala on the leeward side of the Island of Hawai‘i (19°58′ 05.37” N, 155° 51′ 14.13” W) (Figure 1). Various areas of Puakō's reef have been designated by the State of Hawai‘i as a FMA, which prohibits the use of any nets, except for throw nets, and engaging or attempting to engage in fish feeding (Department of Land and Natural Resources). Depths within the study area ranged from approximately < 1 to 30 m and two common reef zones or habitats were present in the study area (reef flat [ < 6 m], fore reef [6–30 m]) (Figure 1).
Figure 1. Study site in Puakō Bay, Island of Hawai‘i, highlighting the location of the high-density acoustic receiver array (orange circles). Shades of blue indicate depth intervals and reef zones: fore reef (6–30 m, darker shades) and reef flat (<6 m, lighter shades).
The two species of parrotfish, S. rubroviolaceus (ember parrotfish) and S. psittacus (palenose parrotfish) were selected as study species due to their relatively high abundance on coral reefs in Hawai‘i. A total of 16 individuals were captured at night with mesh nets: eight ember parrotfish and eight palenose parrotfish, ranging in size from 43 to 59 cm total length (TL). Each fish was brought back to shore and placed in holding tanks. While in holding, fish were closely monitored to ensure that no injuries had occurred during capture. Each fish was measured and tagged with cylindrical external coded acoustic transmitters (V8-4H) (Innovasea www.innovasea.com). Transmitters were mounted externally on the dorsal musculature immediately below the dorsal fin as described by Furey et al. (18) to maximize detectability (19). Each fish was released the following morning in the general location of the capture site.
An array of acoustic receivers (n = 14; Innovasea VR2W, 69 kHz) was placed on Puakō Reef to allow for acoustic positioning (Innovasea, Vemco Positioning System). Based on detection distances obtained from a preliminary trial in the study area, receivers spacing was conservative with locations approximately 40 m apart to maximize the potential for triangulation of tagged individuals within the array. Sync tags were also mounted on all receivers with two additional reference tags placed within the study area to ensure accurate position and time synchronization of the internal clocks among acoustic receivers (20, 21). The positioning system was in place for 35 days, and individual parrotfish were tracked from 6/12/2012 to 7/15/2012.
Time-indexed triangulated positions were filtered by horizontal position error (HPE) following (20). Triangulated positions with HPE < 12 were assumed to represent positioning errors of less than a few meters based on a comparable study in a backreef seascape in the Caribbean Sea (21). Thus, the analysis of position data was limited to triangulated positions with HPE values below this threshold.
Time spent by each fish within the monitored area was quantified by a residency index (RI) expressed as the number of days an individual was detected divided by the individual's potential detection period (i.e., period from the day an individual was released to the end of the study and removal of receivers) (22). RI was calculated for the entire array, and values range from 0 (absence) to 1 (permanent residency).
Triangulated positions were categorized into the distinct periods of the day (day, night, and twilight [dawn/dusk]) to investigate diel variability on fish activity. Duration of each phase was calculated daily using the civil twilight timetable (available at: https://www.timeanddate.com/sun/usa/honolulu?month=6&year=2012). The estimation of activity spaces for both species were restricted to the diurnal period due to the limited number of triangulated positions recorded during the night and twilight periods (Table 1). Diurnal activity spaces of both species were estimated with kernel density plots of triangulated positions using the R package adehabitatHR (23). Kernel utilization distributions (KUD) of 95% (home range) and 50% (core use) areas were estimated for individual parrotfish based on bivariate fixed kernels (24) using the ad hoc smoothing parameter to prevent under- and over-smoothing (25). KUD estimates were limited to fish with at least 150 diurnal triangulated positions and detected over a minimum of 14 days (ember parrotfish n = 4, and palenose parrotfish n = 4). An overlap index (OI) was calculated for both 95% and 50% KUDs for each pair of individuals to assess potential overlap of areas in the seascape within and between both species of parrotfish. Overlap was calculated as: OI = OV (KUDi, KUDi+1)÷ (KUDi + KUDi+1), where OV (KUDi, KUDi+1) is the overlap area between the home range or core use area of a pair of individuals, and (KUDi + KUDi+1) is the combination of home range or core use area of the two individuals (26, 27). OI values range from 0 (no overlap) to 1 (complete overlap). All statical measures are expressed as mean ± standard deviation (SD). Student's t-test was used to assess differences in both activity spaces (home range and core use areas) and OI between ember parrotfish and palenose parrotfish. Significance was tested at α = 0.05.
Table 1. Monitoring data for ember parrotfish (SR1–SR8) and palenose parrotfish (SP1–SP8) in Puakō Bay, Island of Hawai‘i.
Detections (> 114,000) of both parrotfish species resulted in over 11,000 triangulated positions: ember parrotfish (6,082) and palenose parrotfish (5,048) (Table 1). High quality estimates with HPE values < 12 accounted for 92% of triangulated positions, and all descriptions and analyses were limited to these data. Half of the tagged fish (four ember parrotfish and four palenose parrotfish) were detected for 14 or more days and showed moderate to high residency to the monitored area on the short term (RI = 0.48 to 0.91; Figure 2). The remaining fish were detected for 6 days or less, and one ember parrotfish was never detected. Detections and resulting triangulated positions were significantly higher during the day for both species, with daytime positions accounting for 98.7% and 95.7% of total positions for ember parrotfish and palenose parrotfish, respectively.
Figure 2. Abacus plot of ember parrotfish (SR1-SR8, blue) and palenose parrotfish (SP1-SP8, orange) monitored in Puakō Bay from 6/12/2012 to 7/15/2012. Asterisks indicate fish release dates.
Triangulated positions of tagged parrotfish were primarily observed on the fore reef at depths >6 m: ember parrotfish (91.6%) and palenose parrotfish (95.0%). Both species were observed at a wide range of depths during the day (1.2–30.3 m); however, limited use of the shallow water reef flat was observed for each species, accounting for < 7% of daytime detections. At night, triangulated positions for both species were observed exclusively in the deeper fore reef (range: 8.7–15.0 m), indicating the reef flat was not used as a nocturnal resting (i.e., sleeping) habitat. Triangulated positions for palenose parrotfish were at shallower depths during the midday (~1,000 to 1,600 h) and more consistent throughout the entire day for ember parrotfish (Figure 3).
Figure 3. Mean hourly detection (%) for (A) ember parrotfish and (B) palenose parrotfish. Background colors represent the different periods of the day: night (dark gray), twilight (light gray), and day (white).
Daytime home ranges (95% KUD) of individual ember parrotfish ranged from 6,392 to 10,914 m2 (mean: 8,712 m2 ± 1,991 m2 SD) and were centered on the fore reef with 78.7% to 95.2% of home ranges at depths >6 m (Figure 4). Similarly, home ranges of individual palenose parrotfish were concentrated on the fore reef (74.7 to 99.6 %), albeit 95% KUDs were significantly smaller in area than observed for ember parrotfish ranging from 2,218 to 5,067 m2 (mean: 3,725 m2 ± 1,254 m2 SD) (t = 4.2, p < 0.001). Daytime core use areas (50% KUD) for both species were entirely located on the fore reef, except for ember parrotfish SR5, which had a small percentage (2.6%) of triangulated positions on the reef flat. Core use areas of individual ember parrotfish were also significantly greater in area (mean:1,684 m2 ± 787 m2 SD) relative to palenose parrotfish (mean: 546 m2 ± 787 m2 SD) (t = 3.6, p = 0.010). The reduced number of nocturnal positions for both species prevented estimates of activity spaces at night.
Figure 4. Estimated home range (95% KUD, lighter shades) and core use area (50% KUD, darker shades) for ember parrotfish (SR1, SR5, SR7, SR8; shades of blue) and palenose parrotfish (SP2, SP4, SP5, SP7; shades of orange). Acoustic receiver locations (black circles) and nighttime triangulated positions (gray circles) are indicated on each map. Depth contours (3 m, 6 m, and 10 m) are also shown.
Low to moderate overlap was observed in daytime home ranges (OI range: 0.18 to 0.49; mean: 0.29 ± 0.09) and core use areas (range: 0 to 0.30; mean: 0.08 ± 0.11) between the two species (Figure 5). Intraspecific overlap in home range (95% KUD) was significantly greater among ember parrotfish individuals (OI = 0.52 ± 0.09) in comparison to palenose parrotfish individuals (OI = 0.22 ± 0.11) (t = 5.25, p < 0.001). The degree of intraspecific overlap in core use areas (50% KUD) was also greater among ember parrotfish (OI = 0.15 ± 0.20), while most palenose parrotfish individuals occupied non-overlapping core use areas (OI = 0.04 ± 0.10).
Figure 5. Intra- and interspecific overlap index (OI) calculated for each pair of ember parrotfish (SR1, SR5, SR7, SR8) and palenose parrotfish (SP2, SP4, SP5, SP7). OI was calculated between both home range (95% KUD, upper triangle) and core use area (50% KUD, lower triangle). Darker shades of blue indicate higher overlap and lighter shades indicate lower overlap. White blocks represent non-overlapping home ranges/core use areas.
Triangulated positions of both ember parrotfish and palenose parrotfish during the day were almost exclusively (>90%) in the deeper (6–30 m), more complex fore reef, with limited use of the shallow (< 6 m), less complex reef flat. Previous research has shown that adult parrotfish often select for areas of higher habitat complexity within coral reef seascapes (e.g., 27, 28), which may be responsible for the more limited use of the reef flat that is often dominated by lower-relief corals relative to the fore reef (16), including coral reefs in Hawai‘i proximal to Puakō (28). Many studies have demonstrated that habitat complexity (rugosity) is positively correlated with abundance and diversity of reef fishes, including parrotfish (29, 30). The greater structural complexity and coral diversity on the fore reef at Puakō appears responsible for the almost exclusive use of this habitat by both ember parrotfish and palenose parrotfish relative to the reef flat. While coral diversity in the fore reef enhances foraging opportunities for parrotfish (i.e., greater types of foraging substrates), the habitat complexity of the fore reef habitat also affords parrotfish with larger and more suitable refuge or shelter locations (i.e., crevices) within the coral seascape, likely lowering their predation risk (31, 32).
Diel shifts in movement and habitat use is relatively common for coral reef fishes (21, 33, 34), and prior research on parrotfish has demonstrated dichotomous day-night patterns with individuals foraging in shallower habitats of the seascapes during the day and moving into deeper areas of the reef at night (35, 36). Our findings are in accord with these patterns, with both ember parrotfish and palenose parrotfish moving to the shallow reef flat only during the day, with all detections at night in the deeper fore reef. Detection numbers and movements of both species were markedly greater during the day, with limited or no movement detected at night when parrotfish are known to rest/sleep in crevices or spaces within the reef matrix (29, 37). This was primarily due to the rugose nature of the structured reef habitat in the nighttime resting areas which could limit the acoustic detections unless the receiver was directly adjacent to the location of the resting fish. Additionally, individuals would have a reduced chance of being detected on multiple receivers due to their limited movement (and the structured habitat), therefore reducing the number of overall detections (37). Although limited in number, triangulated positions at night typically occurred in the same relative area across consecutive nights, indicative of fidelity to resting sites. Site fidelity by parrotfish to diurnal and/or nocturnal habitats has been reported previously in the Caribbean Sea (38), Red Sea (39), and equatorial Pacific Ocean (40). Nocturnal resting locations of both the ember parrotfish and palenose parrotfish were consistently within daytime home ranges for individuals with available nighttime position estimates.
Fidelity to activity spaces or territories by both ember parrotfish and palenose parrotfish supports other studies showing strong repetitive space-use dynamics of parrotfish to both diurnal and/or nocturnal areas on coral seascapes (35, 41). Previous research has demonstrated that daily space-use patterns of parrotfish may remain relatively fixed over time periods of months (27, 39), with more conspicuous changes in home ranges or core use areas occurring over longer time periods and often linked to ontogenetic shifts (42). In the present study, home range estimates for individuals of both species were ~6,000 to 10,000 m2 (<0.01 km2), which were noticeably lower than 95% KUD estimates for other parrotfish species tracked passively with acoustic telemetry (27, 40, 43). It is important to note that the spatial extent of the receiver array, tracking duration, type of tracking (active vs. passive), space use estimation methods, and age/stage/social status of the individual all influence home range estimates. Additionally, we did not have adequate sample sizes from both sexes across the two species to conduct an extensive analysis. Therefore, it is difficult to align the specific results of this study with results reported across different acoustic telemetry studies (23, 40, 44). As an example, Manning and McCoy (41) reported significantly smaller home ranges (< 500 m2) for five different species of parrotfish in the Caribbean Sea (Bonaire), which appears due to home ranges being based on averages of several short-term, active tracking events by divers for each species under investigation.
Low to moderate interspecific spatial overlap was observed in daytime home ranges and core use areas between ember parrotfish and palenose parrotfish at the Puakō study site, and this is suggestive of resource partitioning. Reduced resource overlap between these two congeners may serve to limit agonistic interactions and facilitate their coexistence in the Hawaiian Islands. Findings from this study are not surprising given that several studies have intimated that low spatial overlap of habitats, substrates, and reef zones by territorial species within the parrotfish complex minimizes competitive interactions (41, 45). Many parrotfish species exhibit haremic social systems wherein adult terminal phase males actively patrol and defend well-defined territories containing conspecific initial phase females against other conspecific terminal phase males (46). Therefore, territorial agonistic interactions and resulting establishment of low or non-overlapping space use areas in parrotfishes is expected to be more pronounced among intraspecific terminal phase males (41, 47). All palenose parrotfish monitored in Puakō reefs were adult males with low intraspecific overlap among their home ranges and completely non-overlapping core use areas in 5 of the 6 possible combinations within pairs of individuals. Conversely, ember parrotfish were all initial phase females, and thus may account for significantly higher overlap of activity spaces for this species. Higher overlap between core use areas of certain ember parrotfish (e.g., SR1-SR7 and SR5-SR8, with OI ~ 0.5) and low values between other pairs (OI = 0–0.05) may indicate that these fish were associated with two different harems (29).
Findings from this study indicate that Puakō reef serves as an important habitat for ember parrotfish and palenose parrotfish as both species showed strong site fidelity to daytime foraging and nocturnal resting areas on the reef. Although tagged adults of each species showed a strong preference for deeper and more complex areas of the fore reef, schools of juvenile parrotfishes were observed foraging on the shallow reef flat, highlighting the potential value of both the fore reef and reef flat as critical habitats of parrotfish conservation in Hawai‘i (5, 6, 17). The application of acoustic receiver arrays with the ability to triangulate fish position is increasingly used to describe fine-scale movement patterns and habitat use of reef fishes in coral seascapes, including parrotfish. In the present study, this approach was used to enhance our understanding the habitat requirements and movements of two exploited parrotfish, shining new light on their spatiotemporal activity spaces in coral seascapes. This information will serve to guide future management efforts (e.g., design of marine protected areas) for conserving both species throughout the Hawaiian Islands.
The raw data supporting the conclusions of this article will be made available by the authors, without undue reservation.
The animal study was approved by Institutional Animal Care and Use Committee (IACUC) Texas A&M. The study was conducted in accordance with the local legislation and institutional requirements.
SA: Data curation, Formal analysis, Investigation, Methodology, Project administration, Writing – original draft, Writing – review & editing. JT: Conceptualization, Funding acquisition, Investigation, Methodology, Project administration, Resources, Supervision, Writing – original draft, Writing – review & editing. DL: Formal analysis, Visualization, Writing – original draft, Writing – review & editing. MD: Data curation, Formal analysis, Investigation, Software, Validation, Visualization, Writing – original draft, Writing – review & editing. RW: Formal analysis, Investigation, Visualization, Writing – original draft, Writing – review & editing. JR: Conceptualization, Data curation, Formal analysis, Funding acquisition, Investigation, Methodology, Project administration, Resources, Software, Supervision, Validation, Visualization, Writing – original draft, Writing – review & editing.
The author(s) declare that financial support was received for the research, authorship, and/or publication of this article. This work was supported in part by funding from the McDaniel Charitable Foundation to JR.
Special thanks to K. Carlson, A. Daw, M. King, L. Kitchens, and A. Mickelson for their assistance with field work and data processing.
The authors declare that the research was conducted in the absence of any commercial or financial relationships that could be construed as a potential conflict of interest.
All claims expressed in this article are solely those of the authors and do not necessarily represent those of their affiliated organizations, or those of the publisher, the editors and the reviewers. Any product that may be evaluated in this article, or claim that may be made by its manufacturer, is not guaranteed or endorsed by the publisher.
1. Cote IM, Renyolds JD. Coral Reef Conservation. New York: Cambridge University Press. (2006) p. 606.
2. Brandl SJ, Rasher DB, Cote IM, Casey JM, Darling ES, Lefcheck JS, et al. Coral reef ecosystem functioning: eight core process and the role of biodiversity. Front Ecol Environ. (2019) 17:445–54. doi: 10.1002/fee.2088
3. Friedlander A, Aeby G, Brainard R, Brown E, Clark A, Coles S, et al. Status of coral reefs in the Hawaiian Archipelago. In: Status of Coral Reefs of the World. Volume 2, ed. C. Wilkinson. Townsville, QLD: Australian Institute of Marine Science (2004). p. 411–430.
4. Randall JE. Zoogeography of shore fishes of the Indo-Pacific region. Zool Stud. (1998) 37:227–68.
5. Cesar H, van Beukering P, Pintz S, Dierking J. Economic Value of the Coral Reefs of Hawai?i. Honolulu: Hawai?i Coral Reef Initiative, University of Hawai?i. (2002).
6. Cesar H, van Beukering P. Economic Valuation of the coral reefs of Hawai‘i. Pac Sci. (2004) 58:231–42. doi: 10.1353/psc.2004.0014
7. Mumby PJ. The impact of exploiting grazers (Scaridae) on the dynamics of Caribbean coral reefs. Ecol Appl. (2006) 16:747–69. doi: 10.1890/1051-0761(2006)016[0747:TIOEGS]2.0.CO;2
8. Hughes, T. P., Rodrigues, M. J., Bellwood, D. R., Ceccarelli, D., and Hoegh-Guldberg, O., McCook, L. J., et al. (2007). Phase shifts, herbivory, and the resilience of coral reefs to climate change. Curr. Biol. 17, 360–365. doi: 10.1016/j.cub.2006.12.049
9. Shantz AA, Ladd MC, Burkepile DE. Overfishing and the ecological impacts of extirpating large parrotfish from Caribbean coral reefs. Ecol Monogr. (2020) 90:e01403. doi: 10.1002/ecm.1403
10. Bellwood DR, Hughes TP, Folke C, Nystrom M. Confronting the coral reef crisis. Nature. (2004) 429:827–33. doi: 10.1038/nature02691
11. Cole AJ, Pratchett MS, Jones GP. Diversity and functional importance of coral-feeding fishes on tropical coral reefs. Fish Fish. (2008) 9:286–307. doi: 10.1111/j.1467-2979.2008.00290.x
12. Lellys NT, Moura RL, Bonaldo RM, Francini-Filho RB, Gibran FZ. Parrotfish functional morphology and bioerosion on SW Atlantic reefs. Mar Ecol Prog Ser. (2019) 629:149–63. doi: 10.3354/meps13102
13. Lange ID, Perry CT, Morgan KM, Roche R, Benkwitt CE, Graham NA, et al. Site-level variation in parrotfish grazing and bioerosion as a function of species-specific feeding metrics. Diversity. (2020) 2:379. doi: 10.3390/d12100379
14. Rice MM, Maher RL, Correa AM, Moeller HV, Lemoine NP, Shantz AA, et al. Macroborer presence on corals increases with nutrient input and promotes parrotfish bioerosion. Coral Reefs. (2020) 39:409–18. doi: 10.1007/s00338-020-01904-y
15. Smith TB, Nemeth RS, Blondeau J, Calnan JM, Kadison E, Herzlieb S, et al. Assessing coral reef health across onshore to offshore stress gradients in the US Virgin Islands. Mar Poll Bull. (2008) 56:1983–91. doi: 10.1016/j.marpolbul.2008.08.015
16. Ortiz DM, Tissot BN. Ontogenetic patterns of habitat use by reef-fish in a Marine Protected Area network: a multi-scaled remote sensing and in situ approach. Mar Ecol Prog Ser. (2008) 365:217–32. doi: 10.3354/meps07492
17. Tricas TC, Boyle KS. Parrotfish soundscapes: implications for coral reef management. Mar Ecol Prog Ser. (2021) 666:149–69. doi: 10.3354/meps13679
18. Furey NB, Dance MA, Rooker JR. Fine-scale movements and habitat use of juvenile southern flounder Paralichthys lethostigma in an estuarine seascape. J Fish Biol. (2013) 82:1469–83. doi: 10.1111/jfb.12074
19. Dance MA, Moulton DL, Furey NB, Rooker JR. Does transmitter placement or species affect detection efficiency of tagged animals in biotelemetry research? Fish. Res. (2016) 183:80–5. doi: 10.1016/j.fishres.2016.05.009
20. Dance MA, Rooker JR. Habitat- and bay-scale connectivity of sympatric fishes in an estuarine nursery. Est Coast Shelf Sci. (2015) 167:447–57. doi: 10.1016/j.ecss.2015.10.025
21. Rooker JR, Dance MA, Wells RJD, Quigg A, Hill R, Appledoorn RS, et al. Seascape connectivity and the influence of predation risk on the movement of fishes inhabiting a back-reef ecosystem. Ecosphere. (2018) 9:e02200. doi: 10.1002/ecs2.2200
22. Kraft S, Gandra M, Lennox RJ, Mourier J, Winkler AC, Abecasis D, et al. Residency and space use estimation methods based on passive acoustic telemetry data. Movem Ecol. (2023) 1:12. doi: 10.1186/s40462-022-00364-z
23. Calenge C. The package “adehabitat” for the R software: a tool for the analysis of space and habitat use by animals. Ecol Model. (2006) 197:516–9. doi: 10.1016/j.ecolmodel.2006.03.017
24. Worton BJ. Kernel methods for estimating the utilization distribution in home-range studies. Ecology. (1989) 70:164.
25. Kie JGA. rule-based ad hoc method for selecting a bandwidth in kernel home-range analyses. Anim Biotelemet. (2013) 1:1–2. doi: 10.1186/2050-3385-1-13
26. Alós J, March D, Palmer M, Grau A, Morales-Nin, B. Spatial and temporal patterns in Serranus cabrilla habitat use in the NW Mediterranean revealed by acoustic telemetry. Mar Ecol Prog Ser. (2011) 427:173–86. doi: 10.3354/meps09042
27. Lippi DL, Coxey MS, Rooker JR, Rezende SM, Dance MA, Gaspar AL, et al. Use of acoustic telemetry to evaluate fish movement, habitat use, and protection effectiveness of a coral reef no-take zone (NTZ) in Brazil. Mar Ecol Prog Ser. (2022) 688:113–31. doi: 10.3354/meps14020
28. Asner GP, Vaughn NR, Heckler J, Gove JM. Large-scale mapping of live coral to guide reef conservation. Proc Nat Acad Sci. (2020) 117:33711–8. doi: 10.1073/pnas.2017628117
29. Welsh JQ, Bellwood DR. Spatial ecology of the steephead parrotfish (Chlorurus microrhinos): an evaluation using acoustic telemetry. Coral Reefs. (2012) 31:55–65. doi: 10.1007/s00338-011-0813-8
30. Wilson SK, Dolman AM, Cheal AJ, Emslie MJ, Prachett MS, Sweatman HPA. Maintenance of fish diversity on disturbed coral reefs. Coral Reefs. (2009) 28:3–14. doi: 10.1007/s00338-008-0431-2
31. Catano LB, Gunn BK, Kelley MC, Burkepile DE. Predation risk, resource quality, and reef structural complexity shape territoriality in a coral reef herbivore. PLoS ONE. (2015) 10:e0118764. doi: 10.1371/journal.pone.0118764
32. Davis K, Carlson PM, Bradley D, Warner RR, Caselle JE. Predation risk influences feeding rates but competition structures space use for a common Pacific parrotfish. Oecologia. (2017) 184:139–49. doi: 10.1007/s00442-017-3857-9
33. Currey LM, Heupel MR, Simpfendorfer CA, Williams AJ. Assessing fine-scale diel movement patterns of an exploited coral reef fish. Anim Biotelemet. (2015) 3:41. doi: 10.1186/s40317-015-0072-5
34. Heidmann SL, Jossart J, Kimble M. Home range characteristics and diel patterns in space use of mutton snapper, Lutjanus analis, in St. Thomas, US Virgin Islands. Anim Biotelemet. (2021) 9:15. doi: 10.1186/s40317-021-00237-z
35. Gomi K, Nakamura Y, Kanda M, Honda K, Nakaoka M, Honma C, et al. Diel vertical movements and feeding behaviour of blue humphead parrotfish Scarus ovifrons in a temperate reef of Japan. J Fish Biol. (2021) 99:131–42. doi: 10.1111/jfb.14704
36. Pickholtz R, Kiflawi M, Buba Y, Chaikin S, Gavriel T, Lapid G, et al. Confronting the ‘nocturnal problem' in coral reefs: sleeping site selection and cocoon formation in parrotfishes. Coral Reefs. (2023) 42:811–25. doi: 10.1007/s00338-023-02389-1
37. Howard KG, Claisse JT, Clark TB, Boyle K, Parrish JD. Home range and movement patterns of the Redlip Parrotfish (Scarus rubroviolaceus) in Hawaii. Mar Biol. (2013) 160:1583–95. doi: 10.1007/s00227-013-2211-y
38. Lindholm J, Knight A, Kaufman L, Miller S. Site fidelity and movement of the parrotfishes Scarus coeruleus and Scarus taeniopterus at Conch Reef (northern Florida Keys). Carib J Sci. (2006) 42:138–44.
39. Pickholtz R, Kiflawi M, Crossin GT, Pickholtz E, Zamsky R, Kahan I, et al. Highly repetitive space-use dynamics in parrotfishes. Coral Reefs. (2022) 41:1059–73. doi: 10.1007/s00338-022-02258-3
40. Davis K, Carlson PM, Lowe CG, Warner RR, Caselle JE. Parrotfish movement patterns vary with spatiotemporal scale. Mar Ecol Prog Ser. (2017) 577:149–64. doi: 10.3354/meps12174
41. Manning JC, McCoy SJ. Territoriality drives patterns of fixed space use in Caribbean parrotfishes. Ecol Evol. (2023) 13:e9833. doi: 10.1002/ece3.9833
42. Welsh JQ, Goatley CHR, Bellwood DR. The ontogeny of home ranges: evidence from coral reef fishes. Proc Roy Soc B. (2013) 280:20132066. doi: 10.1098/rspb.2013.2066
43. La Mesa G, Consalvo I, Annuziatellis A, Canese S. Movement patterns of the parrotfish Sparisoma cretense in a Mediterranean marine protected area. Mar Environ Res. (2012) 82:59–68. doi: 10.1016/j.marenvres.2012.09.006
44. Brownscombe JW, Lédée EJ, Raby GD, Struthers DP, Gutowsky LFG, Nguyen VM, et al. Conducting and interpreting fish telemetry studies: considerations for researchers and resource managers. Rev Fish Biol Fish. (2019) 29:369–400. doi: 10.1007/s11160-019-09560-4
45. Adam TC, Kelley M, Ruttenberg BI, Burkepile DE. Resource partitioning along multiple niche axes drives functional diversity in parrotfishes on Caribbean coral reefs. Oecologia. (2015) 179:1173–85. doi: 10.1007/s00442-015-3406-3
46. Mumby PJ, Wabnitz CC. Spatial patterns of aggression, territory size, and harem size in five sympatric Caribbean parrotfish species. Environ Biol Fish. (2002) 63:265–79. doi: 10.1023/A:1014359403167
Keywords: parrotfish, acoustic telemetry, Hawai'i, diel activity, coral reef, home range, Scarus rubroviolaceus, Scarus psittacus
Citation: Annandale SF, Turner JP, Lippi DL, Dance M, Wells RJD and Rooker JR (2024) Habitat use and movements of parrotfishes in a Hawaiian coral reef seascape. Front. Fish Sci. 2:1448809. doi: 10.3389/frish.2024.1448809
Received: 13 June 2024; Accepted: 05 August 2024;
Published: 21 August 2024.
Edited by:
Kevin J. Hedges, Fisheries and Oceans Canada (DFO), CanadaReviewed by:
Ming-Yih Leu, National Dong Hwa University, TaiwanCopyright © 2024 Annandale, Turner, Lippi, Dance, Wells and Rooker. This is an open-access article distributed under the terms of the Creative Commons Attribution License (CC BY). The use, distribution or reproduction in other forums is permitted, provided the original author(s) and the copyright owner(s) are credited and that the original publication in this journal is cited, in accordance with accepted academic practice. No use, distribution or reproduction is permitted which does not comply with these terms.
*Correspondence: Jason P. Turner, anB0dXJuZXJAaGF3YWlpLmVkdQ==
Disclaimer: All claims expressed in this article are solely those of the authors and do not necessarily represent those of their affiliated organizations, or those of the publisher, the editors and the reviewers. Any product that may be evaluated in this article or claim that may be made by its manufacturer is not guaranteed or endorsed by the publisher.
Research integrity at Frontiers
Learn more about the work of our research integrity team to safeguard the quality of each article we publish.