- 1MARBEC, Univ Montpellier, CNRS, IFREMER, IRD, Sète, France
- 2EPHE-UPVD-CNRS, USR 3278 CRIOBE, Labex Corail, PSL Research University, Papetaoi, French Polynesia
- 3Institut Universitaire de France (IUF), Paris, France
Introduction: Overexploitation of sharks and the destruction of their habitat has led to severe population declines and the need for conservation and management actions. Effective conservation management requires knowledge of the size at which a shark matures and an understanding of their breeding season, fundamental information to maintain appropriate population levels.
Methods: Here we used reproductive endocrinology, estrogen and androgen steroids, in combination with rare direct observations of mating, visual monitoring of reproductive status such as gestation and mating scars, as well as parentage analysis, to assess reproductive biology in male and female Chondrichthyans from the wild.
Results and discussion: Lengths at sexual maturity of female and male blacktip reef sharks corresponded closely with plasma 17β-estradiol, testosterone and 11-ketotestosterone measures respectively, but we found considerable variation in androgen levels for mature males. Size at sexual maturity of male and female blacktip reef shark deduced from direct or indirect evidence (mating scars or parentage assignment respectively, corresponded closely with plasma 17β-estradiol, testosterone and 11-ketotestosterone measures respectively, but we found considerable variation in androgen levels for mature males. Females attained sexual maturity from around 121 to 123 cm and males from 104 to 111 cm. The mating season extends from September to February but female 17β-estradiol levels are elevated 1 month prior to mating. Endocrinology has brought additional important information to the reproductive biology and ecology of blacktip reef sharks.
Introduction
Over the last few decades, many shark species have experienced severe population declines due to overexploitation and habitat destruction (1–4), enhancing the need for conservation and management actions (1, 5). Although the magnitude of population declines varies between species and regions, the k-selected life history traits of most shark species: slow growth, long life-span, late sexual maturity, long gestation and low fecundity, render them highly vulnerable to exploitation and explain slow recovery rates observed. Effective conservation efforts rely on our knowledge of shark life history strategies, particularly reproductive biology and ecology, as ensuring that species have the capacity to reproduce is fundamental to maintain appropriate population levels (6, 7). Therefore, understanding reproductive parameters, including the size at which an animal matures and temporal patterns in reproduction (breeding season), are essential if species are to be managed appropriately.
Shark reproduction has been investigated using both dead animals (8, 9) and non-lethal methods (10–12). Lethal methods include dissections to infer the maturation status of reproductive organs, determine size at maturity and estimate the breeding season. The use of non-lethal methods to determine breeding season is increasing including either rare direct observations of mating (13–15), visual monitoring of reproductive status such as gestation stages or mating scars (16–19), or endoscopy and ultrasonography (20–22). In addition, endocrinology can determine both breeding season and size at maturity (23–28). Of all these non-lethal methods, reproductive endocrinology, which measures the concentrations of hormones that act as either triggers or regulators of all aspects of reproduction, may be the most accurate and reliable to assess the reproductive biology and ecology in male and female elasmobranchs (29). The use of male and female steroid hormones to study reproduction has been on the rise in elasmobranchs under captive or semi-captive conditions (30–32), in wild individuals but using reproductive organ dissections from dead animals to validate plasma-based results (33–36), or in the wild using completely non-lethal methods with the release of sharks at sea after blood sampling and using other complementary methods to validate plasma-based results of maturity (28, 37, 38).
In female elasmobranchs, the ovary produces three main gonadal steroids: 17β-estradiol (E2), testosterone (T) and progesterone (P4) (39). 17β-Estradiol is the major female reproductive hormone, primarily linked to both hepatic vitellogenin synthesis, leading to the growth and maturation of ovarian follicles, and reproductive tract development [e.g., (23, 26, 29, 40, 41)]. Concentrations of T closely track fluctuations in circulating E2 during ovulatory cycles in female elasmobranchs (23, 33), serving as a precursor for E2 synthesis to facilitate vitellogenesis (42). Plasma levels of P4 neither distinguish juvenile from adult female oviparous draftboard sharks, Cephaloscyllium laticeps (10), nor correlate with other reproductive parameters in oviparous winter skates, Leucoraja ocellata (34). While P4 plays a more significant role in the reproductive cycle of viviparous species being elevated for a short period at the beginning of pregnancy (24, 27, 43), P4 correlates with E2 in the viviparous Atlantic Sharpnose Shark Rhizoprionodon terraenovae (44) and E2 is still the more important hormone, at higher concentrations than P4 and for longer periods (27, 45). Therefore, in this study we concentrated solely on E2 in females in our viviparous model species.
In male elasmobranchs, testosterone (T) seems to be the primary androgen steroid (24, 46). Testosterone plays a major role in the regulation of testis development (47, 48), particularly in regulating the final stages of the development and maturation of spermatocysts and stimulation of the development of secondary sex characteristics (24, 33, 34, 36). Male elasmobranchs also produce E2 and P4, although their role in male reproduction is less clear and as T and P4 levels, as well as T and E2 levels, show positive correlations, likely due to P4 being a precursor for T (25, 36), we only concentrated on T. 11-ketotestosterone (11KT) is the main androgen in teleost fishes (49) and has been reported in the bonnethead shark, Sphyrna tiburo (24) and in the dogfish Scyliorhinus canicula (46), yet very few studies have studied the role of 11KT in elasmobranch sexual maturity and their breeding season. Our study aims to use steroid hormones collected from completely non-lethal methods to describe the size at sexual maturity and the breeding season for both female and male blacktip reef sharks. We will measure E2 in females and 11KT, in addition to T in males, to examine their roles in determining sexual maturity and the breeding season for blacktip reef sharks.
The blacktip reef shark Carcharhinus melanopterus is a widespread reef-associated shark species of the Indo-Pacific (50) classified as Vulnerable by the IUCN Red list (2020) and shows highly variable life history traits (i.e., growth rate, size at birth, and male size at maturity) and reproductive periods across its range (51). This species is viviparous, has an annual reproductive cycle (16) and females show reproductive philopatry, returning to the same nursery every year to give birth to a litter of 2–3 neonate sharks (52). Mating activity is difficult to observe and mainly consists of rare mating observations (15) as well as indirect evidence of recent mating attempts via observations of fresh mating scars (19). While size at sexual maturity of male blacktip reef sharks has been inferred from external claspers (51), size at sexual maturity of females has only been determined previously using dead animals (53). In French Polynesia, sharks have been protected since 2006 in what is currently the largest shark sanctuary in the world (54), and lethal methods for examining shark life histories are therefore prohibited which has encouraged the development of non-lethal approaches to study their biology and behavior (18, 52, 55, 56). To further our understanding of the population dynamics of blacktip reef sharks throughout French Polynesia, we used non-lethal methodologies to accurately determine their size at maturity, breeding season, gestation and parturition.
In this study, we aim to determine (i) annual reproductive biology i.e., the breeding season and parturition from visual identification (clasper calcification, mating scars, gestation, umbilical scars) as well as hormone levels; (ii) the abiotic parameters, photoperiod and sea surface water temperature, that influence both the breeding season and hormone levels; (iii) the total length at maturity of females based on mating scars, gestation, parentage analyses and plasma E2 measures and confirm the total length at maturity for males using plasma steroid levels; and (iv) the efficacy of steroid hormones as a non-lethal method to estimate sexual maturity and breeding season in blacktip reef sharks.
Materials and methods
Capture and non-lethal sampling methods
A total of 268 Carcharhinus melanopterus were caught around Moorea (17° 30′ S; 149° 50′ W) in French Polynesia between 2008 and 2011. Adults and subadult sharks were caught from a fishing line on a boat inside and outside the lagoon on a monthly basis (51). Newborn sharks were caught from the shore with gillnets (50 m long, 1.5 m high, 6 cm mesh) positioned perpendicular to the shoreline in nursery areas (52). The total length of the 268 blacktip reef sharks was measured and ranged from 48 to 157 cm. Individuals were sexed when possible (clasper calcification in males; see Size at sexual maturity below; (51)), photographed and fin-clipped for genetic parentage analysis and all individuals were released back to the sea within 5–10 min after capture with no mortality. Ethical approval for the study was granted from The Animal Ethics Committee, Center National de la Recherche Scientifique (Permit Number: 006725). This study was conducted under the authority of the Direction de environment de Polynésie française (DIREN) under the convention 5129/MCE/ENV.
The 268 individuals (146 males and 122 females) included all size ranges. Among these 268 individuals, blood samples were taken from 135 individuals (78 males and 57 females with total length ranging from 54 to 153 cm; see “Blood sampling and reproductive endocrinological parameters” below). In addition to these captures, other non-lethal sampling methods were carried out. In 2005 and 2007 on a sporadic basis and regularly between 2008 and 2010, we carried out underwater surveys (~200 dives) dedicated to monitoring the presence of sharks along the Northern reef of Moorea using photo-identification (55). During these surveys additional information on blacktip reef sharks was also collected including photographs of mating scars on females (19) and gestation monitoring by identifying individuals and following their abdomen growth. All morphological measures on captured sharks were taken to the nearest centimeter.
Abiotic measurements
Average monthly water temperature was determined from daily temperature monitoring of Moorea's reef using bottom-mounted thermistors over the same time period as shark captures between 2008 and 2010 by CRIOBE SNO (https://observatoire.criobe.pf). A continuous time series of water temperature data, measured using underwater temperature data loggers (Onset Optic StowAway) located on fixed stations in the backreef, the barrier reef and the outer slope at Tiahura at 1, 2, and 25 m depth, respectively. These loggers have recorded the temperature every hour since 1998 with an accuracy of 0.01°C. Daily temperatures were average across the three stations.
Photoperiod was calculated on a daily basis as the difference in time (hours) between sunrise and sunset from 2008 to 2010 data provided from the United States Naval Observatory Astronomical Applications Department (http://aa.usno.navy.mil/data/docs/MoonFraction.php).
Size at sexual maturity
Chondrichthyan males typically have external paired claspers (i.e., copulatory appendages), which are extensions of the posterior bases of the pelvic fins. Sexually immature individuals have short, soft and smooth claspers, which during maturation, exhibit accelerated growth and calcification (57). As such, sexual maturity status of males can be reliably and non-invasively determined externally using clasper elongation and degree of calcification (31, 51, 58, 59).
However, this is not possible in females as they lack any external organs representative of maturity. Therefore, we used two non-invasive methods to determine sexual maturity of captured female sharks: parentage analysis and steroid hormone levels. As blacktip reef sharks do not disperse far from their parental island (52), parentage analysis of juvenile blacktip reef sharks can be used to determine the smallest size at which adult blacktip reef sharks can be a parent i.e., were sexually mature (confirm for male and determine for females). We captured, measured and genotyped 250 blacktip reef sharks as part of a parallel study (52) between 2008 and 2010. Sharks were divided into three categories according to their size: juveniles (< 70 cm, the smallest ones being newborn sharks showing apparent umbilical scars (19), “subadults” (70 < TL < 110 cm) and “adults” (>110 cm) according to the average size at maturity known for this species (51, 60). Of the 250 captured sharks, 61 were juveniles captured in their nursery areas and 189 were subadult/adults. Juveniles were then assigned to a subadult or adult pair when possible and the smallest subadult or adult that was assigned as a parent was used to determine the minimum total length at sexual maturity. Steroid hormone levels were also used to corroborate our genetic findings and determine sexual maturity of females not identified as parents. From our global population survey, parentage analysis assigned 43 of the 61 genotyped juveniles to at least one parent or a pair of parents among the 189 genotyped subadult or adult sharks around the island. From these 43 juveniles, 19 (44.2%) were assigned only to a female, 18 (41.8%) only to a male and 6 (14.0%) to a pair of parents (52). Furthermore, using individuals captured in the same year as paternity/maternity assignment, as well as steroid hormone levels of reproductively active male and female sharks, we determined minimum total length at sexual maturity.
Blood sampling and reproductive endocrinological parameters
Blood was immediately sampled from 135 individuals (78 males and 57 females) within 3–5 min of the original disturbance (capture on fishing line or gillnet). A minimum of 250 μl of blood was drawn laterally from the caudal vein using a heparinized 15 gauge needle and 10 ml plastic disposable syringe. Syringes were kept on ice on the boat until processing (< 60 min). Individual blood samples were transferred to eppendorfs and centrifuged (Sigma Centrifuge 1–14; http://www.sigma-zentrifugen.de/) at 10,000 g for 5 min. The supernatant, a yellow plasma layer, was collected and stored at −20°C until analysis.
Plasma 11-ketotestosterone (11KT) and testosterone (T) were measured from 78 males and 17β estradiol (E2) from 57 females using EIA kits (11KT EIA Kit, No. 582751; T EIA Kit, No. 582701; E2 EIA Kit, No. 582251; Cayman Chemicals, SPI BIO; www.spibio.com) and a Beckman Coulter AD 340 Spectrophotometer at 405 nm as described in Mills et al. (61) after validation with parallel displacement of serially diluted plasma to the standard curve and determination of intra- and inter-assay variabilities (see Supplementary material). The 11KT kit has already been previously validated for this species (61), the kits for T and E2 were used after validation with parallel displacement of serially diluted plasma to the standard curve (see Supplementary Table 1, Supplementary Figures 1, 2).
Data analysis
To determine the abiotic parameters corresponding to annual reproductive activity, we carried out correlations between the number of mating observations and abiotic factors (temperature and day length). We also tested the correlations between steroid hormone concentration in adult sharks (males > 110 cm TL and females >120 cm TL as determined by the smallest mother assigned from parentage analysis, see below) and abiotic factors. We compared hormone concentrations between mature and immature and between the breeding and non-breeding seasons. To determine the role of steroid hormones in size at sexual maturity, we first plotted hormone concentrations against total length of sharks. We then generated maturity ogives from steroid hormone concentration values above which individuals were considered mature, and determined the length at which 50% of the individuals were mature (L50). Size at maturity based on hormone data (L50) was then compared to the size at maturity determined from clasper calcification for males (L50) and minimum size at which a female was assigned to a newborn shark from parentage analysis (i.e., minimum size at sexual maturity). The size at which a female was found to be a genetic parent might be some months after reaching sexual maturity and hence our measures might overestimate the minimum size at sexual maturity especially when compared with the L50 provided by E2 data, therefore we acknowledge that these estimates may not always be directly comparable. However, the range in TL between the minimum size of mature individuals and the maximum size of immature individuals is generally narrow and comparing these estimates can still be valuable to confirm an approximate size at maturity. To determine the role of steroid hormones in the reproductive biology in blacktip reef sharks we used a Kruskal-Wallis test to compare steroid hormone concentrations (11KT and T for males and E2 for females) of mature individuals between months. Pairwise comparison post-hoc tests were used to assess specific month to month variations in hormone concentrations.
Results
Of the 146 male sharks caught, sexual maturity was determined for 94 males. The 122 females required parentage analysis and steroid hormone levels to determine their sexual maturation status. From underwater surveys conducted around the island, 41 females were determined to be reproductively active based on mating scars and gestation status, of which 18 were caught and measured which provided data on length at sexual maturity.
Breeding season
Based on a rare direct observation of mating on the 27th December 2009 (Figure 1A) and indirect evidence of mating inferred from 33 cases of fresh mating scars reported in years 2005, 2007, 2008, 2009, 2010, and 2013 (Figure 1B), and the absence of mating scars between March and October, we determined that the mating period for C. melanopterus occurs between October and February, peaking from December to February (Figure 1C). Parturition was also inferred from gestation monitoring and date at capture of smallest newborn sharks with visible open umbilical scars and occurs between September and January (Figure 1C).
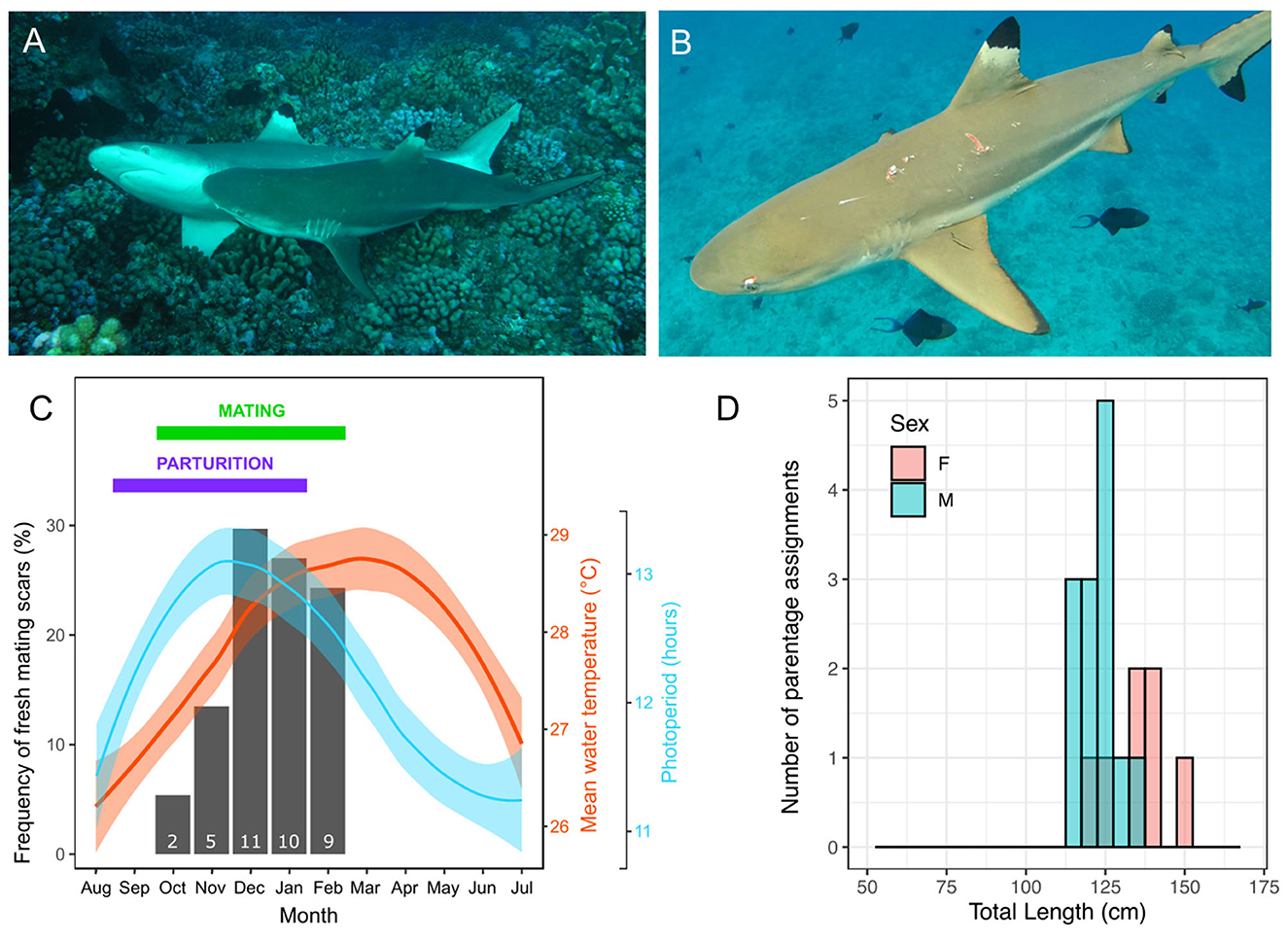
Figure 1. Reproductive season in blacktip reef sharks, Carcharhinus melanopterus. (A) A rare observation of C. melanopterus reproduction photographed on the fore reef of Tiputa pass in Rangiroa (French Polynesia) on the 27th December 2009; (B) An example of a female C. melanopterus with fresh mating scars photographed on the 10th November 2013 in Moorea (French Polynesia); (C) Monthly frequency of mating events inferred from fresh mating scars on females (black bars, with associated number of animals with mating scars indicated at the bottom of the bar) and mean monthly (95% CI) water temperature (red line and shading) and photoperiod (blue line and shading) in Moorea. The months corresponding to the breeding season and parturition are summarized with a green and purple horizontal, respectively. Parturition was inferred from gestation monitoring and date at capture of smallest newborn sharks with visible open umbilical scars (19, 52) and confirmed by Debaere et al. (62); (D) Distributions of total length of reproductively active male and female sharks based on parentage analyses (based on individuals assigned a newborn shark for which we had measurements during the previous mating season). Photo credits: (A) Yves Lefèbvre, (B) Johann Mourier.
Correlations between abiotic factors and both the breeding season and endocrinology
The initiation of the mating period corresponds with increasing day length and increasing temperature, although mating period shows a better fit with photoperiod (Figure 1C). The frequency of mating scars was positively correlated with photoperiod (Pearson correlation coefficient r = 0.755, df = 10, p < 0.005) and with temperature although it was not significant (Pearson correlation coefficient r = 0.454, df = 10, p = 0.138). All hormone levels showed positive correlations with photoperiod but varied correlations with sea temperature (graphs of correlations are available in Supplementary Figure 3), although these correlations need to be considered with caution due to relatively small sample size. There was a positive correlation between plasma 11KT concentrations and photoperiod (r = 0.621, df = 53, p < 0.001) and a negative correlation with temperature (r = −0.294, df = 53, p = 0.029). Similarly, there was a positive correlation between plasma T concentrations and photoperiod (r = 0.579, df = 51, p < 0.001), but no correlation with temperature (r = −0.130, df = 51, p = 0.351). There was a positive correlation between plasma E2 concentrations in females and photoperiod (r = 0.601, df = 37, p < 0.001) and a negative correlation with temperature (r = −0.524, df = 37, p < 0.001).
Size at sexual maturity
Based on parentage analysis we were able to assign 25 males as sires of the 61 juvenile blacktip reef sharks sampled. Thirteen of these males were captured and measured during the previous mating season and the smallest male to father a pup was 114 cm TL (mean ± SE = 123.23 ± 1.58 cm TL, for the 13 males; Figure 1D). Using parentage analysis we were also able to assign 19 females as mothers of the 61 juveniles. Seven of these females were captured and measured during the months preceding giving birth and the smallest reproductively mature female was 121 cm TL (mean ± SE = 134.71 ± 3.50 cm TL, for seven females; Figure 1D).
Breeding season, size at sexual maturity, and reproductive endocrinology
In order to accurately determine the role of hormones in estimating size at sexual maturity, we did not include hormone measures taken outside of the mating season (March to September inclusive) in analyses, as values of mature individuals were significantly lower outside compared to inside the breeding season (t-test: 11KT, t = 3.88, N = 59, p < 0.0026; T, t = 2.87, N = 59, p = 0.0177), but we show all values in the figures. Furthermore, although concentrations for 11KT were not significantly different between mature and immature males outside the mating season (t-test: t = 2.22, N = 56, p = 0.0541) concentrations were significantly different for T (t-test: t = 3.48, N = 57, p = 0.0015). For males during the mating season, there were significant differences in 11KT and T concentrations with sizes related to sexual maturity based on clasper calcification, i.e., between mature (>110 cm TL) and immature (< 110 cm TL) males (t-test: 11KT, t = 7.23, N = 39, p < 0.0001; T, t = 3.72, N = 35, p = 0.0047). However, the relationship between 11KT and T levels with TL showed considerable variation for similar-sized males around the size at which males reach sexual maturity, suggesting that not all males may be sexually active for a given TL. Levels of 11KT began to increase from a TL of 90 cm (Figure 2A) and T concentrations from a TL of 104 cm (Figure 2B), which corresponds to the beginning of clasper calcification.
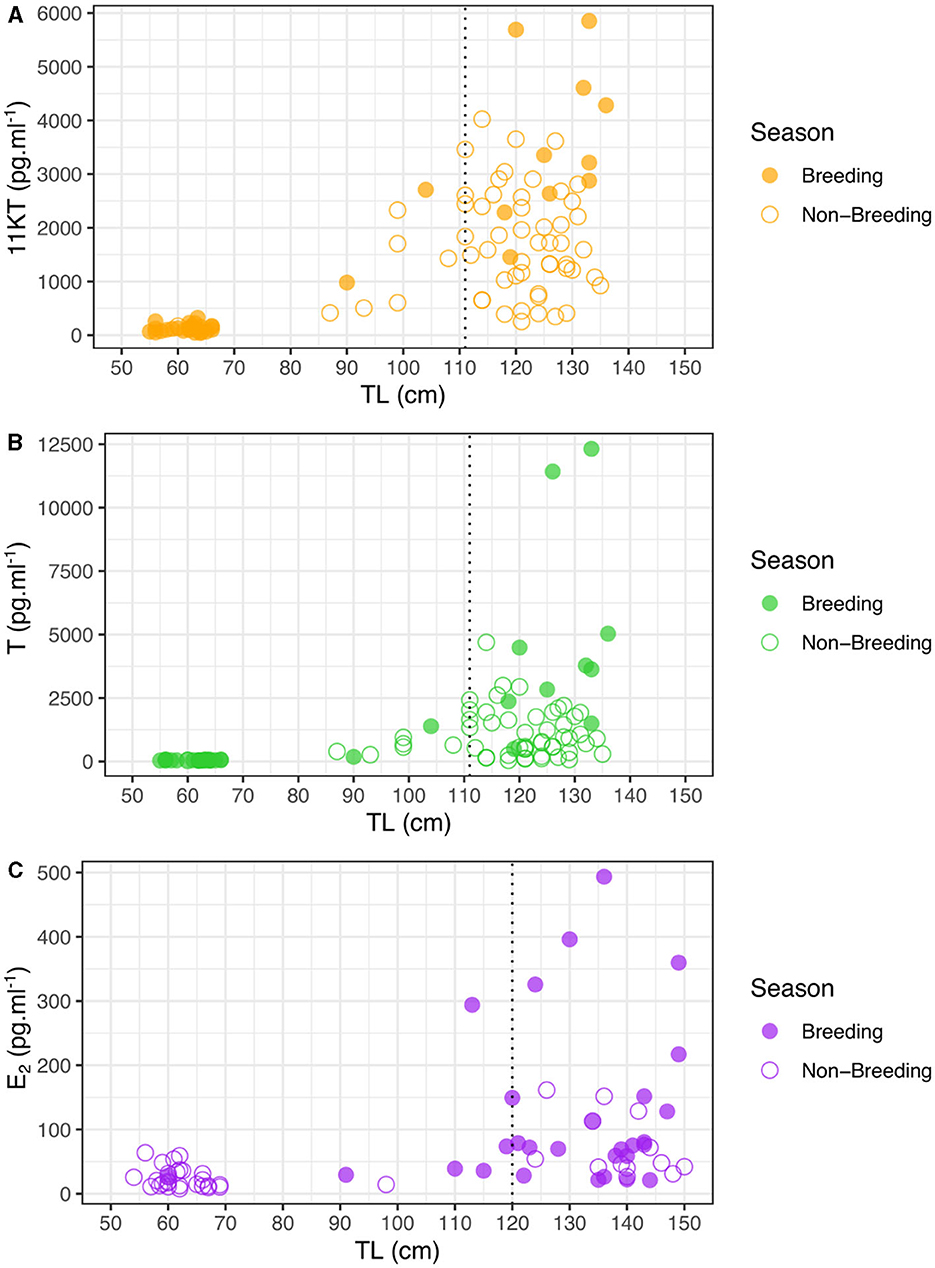
Figure 2. Steroid hormone concentrations of Carcharhinus melanopterus during the mating season (October-February) and outside as a function of total length (TL) for (A) 11KT in males, (B) T in males, and (C) E2 in females. Vertical dashed lines indicate size at sexual maturity inferred from calcified claspers (A, B) and parentage analyses (C).
We then created maturity ogives based on steroid hormones by inferring the proportion of individuals that had concentrations above a certain value as a function of TL. Maturity ogives generated from 11KT predicted that sexual maturity of 50% of males occurred at 95 cm, 99 cm and 104 cm TL for concentrations over 1,000, 1,500, and 2,000 pg.ml−1, respectively. Therefore, the 11KT concentration thresholds that returned a 50% maturity prediction closest to that generated using calcified claspers (i.e., 50% maturity at 111 cm TL) was 2,000 pg.ml−1 at 104 cm TL (Figure 3A). Maturity ogives generated from T predicted that sexual maturity of 50% of males occurred at 100, 115, and 114 cm TL for concentrations over 1,000, 1,500, and 2,000 pg.ml−1, respectively. Therefore, the T concentration thresholds that returned a 50% maturity prediction closest to that generated using calcified claspers (i.e., maturity at 111 cm TL) was 2,000 pg.ml−1 at 114 cm TL or 1,500 pg.ml−1 at 115 cm TL (Figure 3B). We also adopted the same approach using the data from inside and outside the breeding season, but maturity ogives were less reliable and tended to overestimate size at maturity compared to external maturity indicators (Supplementary Figure 4), supporting our choice to investigate size at maturity during the breeding season.
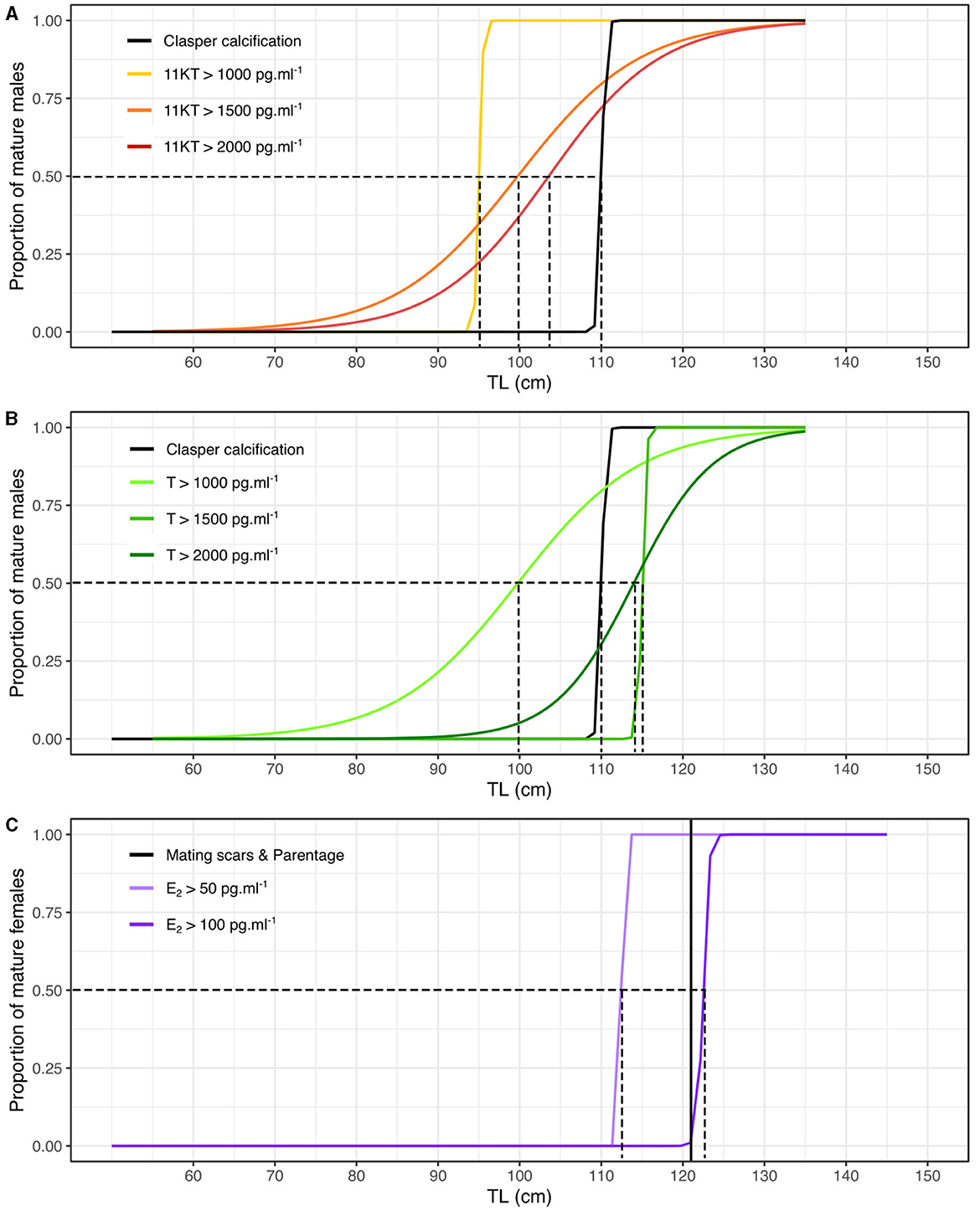
Figure 3. Maturity ogives based on morphological, parentage and steroid hormone analyses for male and female blacktip reef sharks during the mating season (October-February). (A) Maturity ogives of males based on 11KT concentrations (in black the maturity ogive based on proportion of calcified claspers). (B) Maturity ogives of males based on T concentrations (in black the maturity ogive based on proportion of calcified claspers). (C) Maturity ogives of females based on E2 concentrations (the black vertical line corresponds to the smallest TL of females assigned as parents using parentage analyses). The dotted lines correspond to the TL at which 50% of males and females are sexually mature.
There was a clear shift in female E2 levels with size, with hormone levels strongly increasing for female sharks larger than 120 cm, although there were only limited samples at larger sizes (Figure 2C). Size at sexual maturity based on E2 (~120 cm) corresponds well with size at sexual maturity based on parentage analyses in this study (121 cm) as well as with the appearance of mating scars. In a similar manner as for males, during the mating season there was a significant difference in E2 concentrations between mature and immature females (t-test: t = 9.73, N = 32, p = 0.009) but not outside of the mating season (t-test: t = −0.24, N = 42, p = 0.8186), corroborating our decision to only analyze samples taken during the breeding season. In addition, E2 concentrations were significantly higher for mature females during the mating season than outside this period (t-test: t = 8.06, N = 39, p = 0.0098).
Maturity ogives generated from E2 predicted that sexual maturity of 50% of females occurred at 113 cm and 123 cm TL for concentrations over 50 and 100 pg.ml−1, respectively. Therefore, the E2 concentration threshold that returned female 50% maturity prediction closest to that generated using the smallest female assigned as mother of a sampled offspring using parentage analysis (i.e., maturity at 121 cm TL) was 100 pg.ml−1 at 123 cm TL (Figure 3C). However, the smallest female assigned as a mother might have become sexually mature prior to mating and may overestimate the smallest size at maturity and E2 threshold. Therefore, female 50% maturity likely occurs between 113 and 123 cm TL at concentrations of E2 between 50 and 100 pg.ml−1. We also recognize that the sample size of mature females was small and our results need to be taken with caution. Similarly, maturity ogives were less reliable and tended to overestimate size at maturity compared to external maturity indicators using the data from inside and outside the breeding season (Supplementary Figure 4).
In order to accurately determine the role of hormones in the reproductive biology of blacktip reef sharks, only those measures taken from sexually mature males and females were subsequently analyzed over the year. Hormone concentrations showed significant seasonal variation in mature male sharks (Kruskal-Wallis test: 11KT: χ2 = 32.2, N = 59, p < 0.0001; T: χ2 = 26.8, N = 57, p = 0.0015; Figure 4). 11KT concentrations in males were highest in October compared to the rest of the year but the difference was only significant compared to April and May (Dunn test: p < 0.01 after Bonferroni correction; Figure 4A). Testosterone concentrations in mature males were also highest in October compared to the rest of the year but the difference was only significant compared to May (Dunn test: p < 0.01 after Bonferroni correction; Figure 4B).
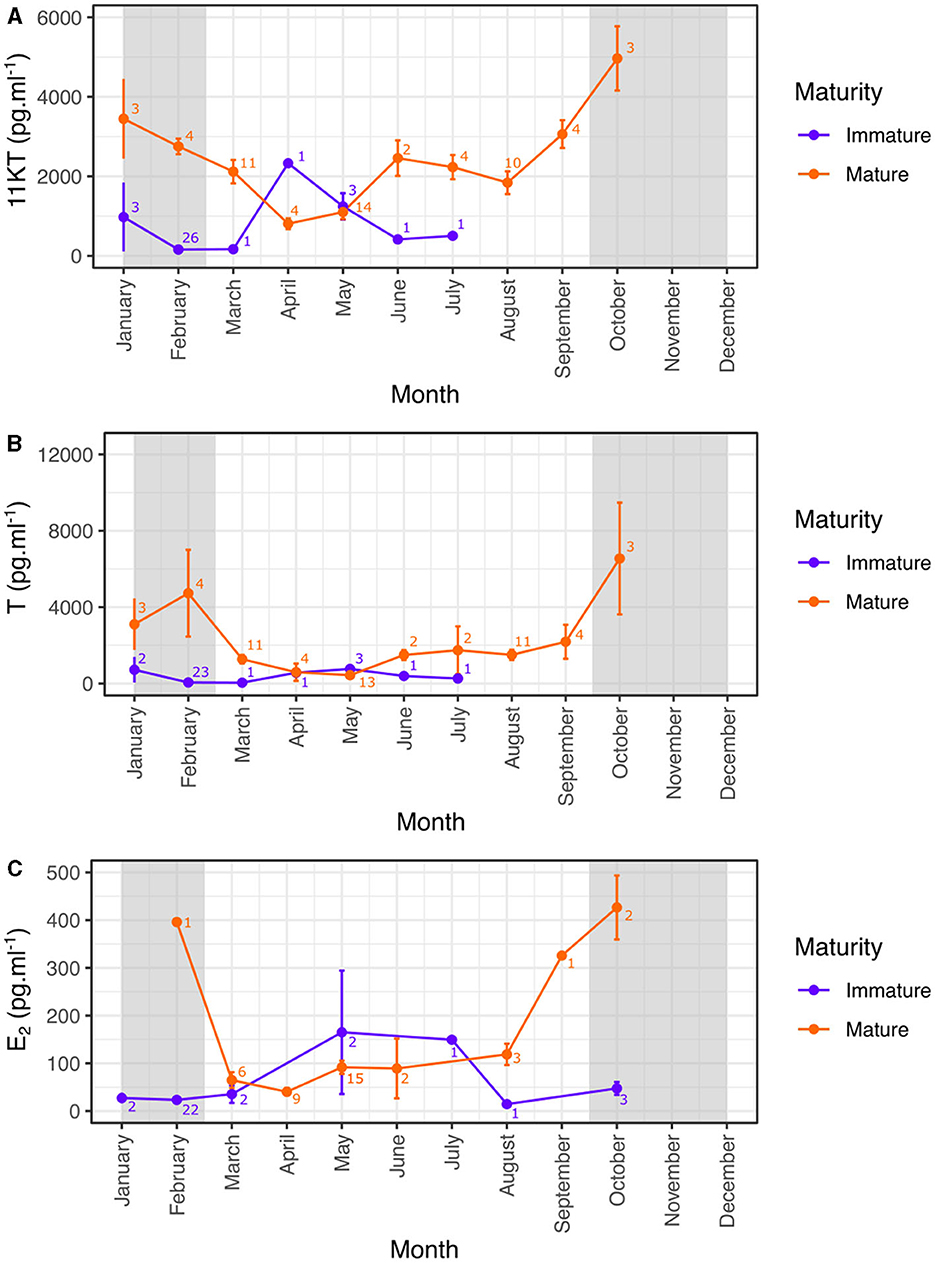
Figure 4. Monthly variation (mean ± SE) of reproductive hormones in mature and immature male (A, B) and female (C) blacktip reef sharks. Light gray areas indicate the mating period. 11KT, 11-ketotestosterone; T, testosterone; E2, 17β-estradiol.
Mature females also showed seasonal variation in E2 concentrations (Kruskal-Wallis test: E2: χ2 = 19.1, N = 39, p = 0.0079) with E2 concentrations in mature females highest in October compared to the rest of the year but the difference was only significant compared to April (Dunn test: p < 0.01 after Bonferroni correction; Figure 4C). Our results also highlight that levels of E2 began increasing before the start of the reproductive season from September, but remained high to the end of the mating season.
Discussion
This study tests the use of various non-lethal methodologies to obtain reproductive information, breeding season and sexual maturity, of a common reef shark, the blacktip reef shark, Carcharhinus melanopterus. Length at sexual maturity of female blacktip reef sharks was deduced from mating scars, gestation and parentage assignment and these corresponded closely with plasma E2 measures. Total length at maturity for males previously determined from external claspers, was also confirmed using plasma steroid levels, T and 11KT, but we found variation in androgen levels. The breeding season was deduced from both direct and indirect evidence of mating activity such as mating scars, gestation, umbilical scars or parentage assignment, and these corresponded well with concentrations of estrogen and androgen steroid hormones, in females and males, respectively. We also identified photoperiod as the abiotic parameter that regulates steroid hormone levels, driving the start of the mating period. We discuss our findings in light of size at maturity for male and female blacktip reef sharks and their reproductive cycle and breeding season.
The influence of abiotic factors
Abiotic factors expressed here as temperature and photoperiod seem to influence steroid hormone concentrations and mating activity in blacktip reef sharks in agreement with the endocrine regulation of spermatogenesis in the catshark, S. canicula (63). Day length had the most obvious influence as it was positively correlated with male and female steroid hormones and with the number of mating scars on females and the progressive switch of male blacktip reef sharks to an active breeding condition, although our findings are contrary to Mull et al. (64). As daylength increases, plasma concentrations of T and 11KT increase suggesting that photoperiod is the mechanistic driver of androgen hormones, that initiate the start of breeding. Thus, the onset of spermatogenic activity in male C. melanopterus after September is likely triggered by the environmental cue daylength, resulting in the production of androgens and other steroid hormones.
The present data indicate that plasma 11KT and E2 concentrations are negatively correlated with ambient water temperature. In epaulet sharks Hemiscyllium ocellatum that show a clear unimodal annual cycle, seasonal changes in plasma androgen concentrations were negatively correlated with water temperature (26) and a weak negative correlation with E2 was also found. Positive and negative correlations between temperature and E2 were reported in female Australian sharpnose sharks Rhizoprionodon taylori (65) and zebra shark Stegostoma fasciatum (66), respectively. Such differences between studies could be in part due to differences in data analyses, or by non-linear relationships between sex steroids and temperature when considering the whole range of seawater temperatures (67). While we still need to understand the mechanism by which temperature regulates steroid hormones, it is likely an important factor in elasmobranch reproductive biology and will need further investigation.
Size at sexual maturity
To infer female sexual maturity, several non-lethal approaches have been proposed and employed. The presence or absence of hymen has been used to assess maturity in females, although this method demonstrated some limitations and bias (68). Internal reproductive structures and sexual stages, especially pregnancy, can be examined using ultrasonography but requires specialized equipment and reproductive status is not always easy to determine (22, 28). Finally, reproductive hormones may be used as indicators of maturation status. All studies on elasmobranchs to date suggest that a single or a combination of plasma steroid hormones can provide accurate indicators of maturational status and size at maturity can be subsequently inferred (10, 27, 31, 41, 45, 69). In this study, we first used the results of a parentage analysis previously conducted on this population in Moorea (52) to determine that the smallest size of a female assigned as a mother was 121 cm TL, providing us with an indicator of size at maturity for females, although this TL might overestimate the smallest size at maturity. We then showed that the smallest mature females determined from the presence of mating scars and concentrations of plasma E2 above 100 pg.ml−1 were ~123 cm TL, corresponding closely, within 2 cm, to the smallest size determined from parentage analysis (Figures 1C, 3C). Differences in size at sexual maturity between morphological and hormonal maturity have previously been reported in elasmobranchs, where hormonal pathways associated with sexual maturity have been shown to lag behind morphological maturity in the bonnethead shark (70), the winter skate (71), and the thorny skate Amblyraja radiata (72). The size at maturity of female blacktip reef sharks also varies between locations, ranging from 110 cm TL in Aldabra in Indian Ocean (73) to 130–135 cm TL in northern-Australia (53) both determined using lethal methods and between 121 and 123 cm TL in French Polynesia using non-lethal methods (this study). Such disparities in size at maturity may be due to differences in latitude between sampled areas, which has previously been observed in bonnethead sharks (74) and may be adaptive responses to different environmental conditions (75).
The smallest male with calcified claspers previously measured was 111 cm TL and the largest immature male was 114 cm TL (51), and this estimation was confirmed in this study using both parentage analysis, as the size of the smallest male known to father a sampled pup was 114 cm (Figure 1D) and endocrine analyses, as concentrations of both 11KT and T levels increased from ~104 cm (Figures 1A, B, 4B). Our results also agree with the size at maturity of male blacktip reef sharks estimated at 105 cm TL using lethal methods in northern-Australia, but see caveats outlined above for regional differences (53). However, considerable variation in androgen levels were found in mature males, indicating that steroid hormone measures in addition to clasper calcification criteria are necessary to accurately determine size at maturity in male sharks.
Maturity ogives suggested sizes at maturity slightly lower than those indicated from calcified claspers for males and parentage analyses for females, which indicates that some males or females in the population could mature more precociously than most of the population as displayed by higher hormone concentrations at lower TLs. In addition, the smallest male with calcified claspers and the smallest female assigned as a mother with parentage analysis might reflect the average size at maturity for the population but might underestimate the smallest size at maturity.
The observed variation in androgen levels of mature male blacktip reef sharks is likely due to differences in male hierarchical status, abundance or competition between males. Elasmobranch reproductive behavior involves aggressive social interactions among males and females, and the formation of social groups is complex and involves courtship behaviors (13–15, 76–78). Androgen steroids initiate, promote, and sustain behavioral aspects of sexual conflicts including swimming speed in elasmobranchs, and androgen levels are dependent on hierarchical status in sand tiger sharks, Carcharias taurus (32). We therefore propose that a similar hierarchical status exists within blacktip reef shark male social groups, especially in fluctuating densities enhancing competition between males, and would explain the large variation in androgen levels for males of similar size (120–135 cm). High T and 11KT values may indicate dominant males, and relatively lower T and 11KT values indicating subordinate males which, despite being sexually mature, have suppressed androgens in a similar manner to that shown in teleost fish [e.g., (79, 80)]. While there is no direct evidence of dominance hierarchy in blacktip reef sharks, observations from captive males in public aquariums provide some evidence of a hierarchy between males with leaders and followers (Mourier, unpublished data). It is also possible that a proportion of males in the population could be reproductively inactive during one season, skipping a reproductive season as already shown in females from certain shark populations [e.g., (18)].
Breeding season
The concentrations of E2 in female blacktip reef sharks were highest from September to February corresponding to 1 month prior, and during, the mating season determined from the presence of mating scars. The patterns of E2 levels in female elasmobranchs in the current study parallel those found in other adult female viviparous elasmobranch species in which significantly higher values were found in pre-ovulatory and mating females, and lowest levels during early pregnancy. Elevated E2 levels during the pre-ovulatory period were found in the Atlantic sharpnose shark Rhizoprionodon terraenovae (44), the Atlantic stingray, Dasyatis sabina (40), the bonnethead shark, Sphyrna tiburo (23), and the little skate, Raja erinacea (30). Circulating E2 concentrations generally peak during the period of follicular development in viviparous elasmobranchs and have roles in regulating synthesis of the yolk protein precursor, vitellogenin Vtg (81), in stimulating the uptake of vitellogenin by the elasmobranch oocyte [(82); review in Callard et al. (83)] and in follicular growth leading to ovulation (29). Elevated E2 concentrations during ovulation in viviparous sharks have also been linked with the passage of the fertilized egg to the uterus (23, 33, 40, 84).
The elevated concentrations of E2 in female sharks in September, which corresponds to 1 month prior to the mating season, agree with post-ovulatory rises in E2 concentrations that have also been observed during mid to late pregnancy in females of other seasonally breeding elasmobranchs, such as the bonnethead shark and the Atlantic stingray (23, 33, 40). Since follicular development for the subsequent reproductive cycle does not begin until after parturition in these species, increased levels of E2 may reflect another possible role of this hormone in gestation and parturition. Indeed, elevated E2 during late pregnancy in several shark species has been found to prepare the uterus for parturition, by increasing levels of the hormone relaxin, that enlarges the cervix to allow embryo passage during parturition (85, 86). In agreement, in our study, the clear rise in E2 concentrations from September corresponds to the period of parturition. As the blacktip reef shark has a 1-year reproductive cycle, breeding every year, it is not surprising that E2 concentrations were high in all sexually mature females during the mating season, as opposed to other species that do not reproduce every year and have contrasting E2 concentrations (28).
Concentrations of T and 11KT were also elevated in male blacktip reef sharks from October and February corresponding to the mating season. In male elasmobranchs, testis development and seasonal spermatogenesis are both regulated by androgens (81). T levels are often elevated during the middle to late stages of spermatogenesis, which is coincident with the presence of mature spermatocysts in the testes (25, 26, 33–35, 40). The T levels measured in this study, agree with previous findings and suggest that T correlates with gonadal recrudescence, final sperm maturation, and the onset of copulatory activity in blacktip reef sharks (81). 11KT is thought to be the main androgen in teleost fishes but its function in male elasmobranchs is less clear. Circulating 11KT has been found to contribute to testicular development in some elasmobranchs (24, 46, 64) and elevated levels in blacktip reef sharks during the mating season would agree with these previous findings. In studies where both T and 11KT were examined, the patterns of both androgens were very similar (24, 46), which we can confirm in this study. However, although 11KT levels were lower outside of the mating season, they did not decrease to levels as low as T, whose levels closely followed the mating season and remained at relatively lower levels for the rest of the year. Our study shows that T levels alone would be an appropriate non-lethal method for determining the breeding season in male blacktip reef sharks.
A non-lethal method for breeding season or reproductive events and maturity assessment in sharks
In the current study, we report on the successful use of non-lethal methods to determine sexual maturity and breeding season in blacktip reef sharks. Measurement of plasma steroid hormones provided a helpful method to classify both male and female sharks as juveniles or adults and also contributed in determining the seasonality of reproduction, in particular the mating season, without killing any animal. Results from steroid hormone levels were confirmed by other external information such as contribution to birthing events assigned from parentage analyses or fresh mating scars in females or calcified claspers in males. However, female E2 concentrations were more informative to assess sexual maturity than 11KT and T concentrations in males, the latter showing high variation between mature individuals. In addition, our study also suggests that assessment of size at maturity may be more reliable using hormone concentrations measured during the breeding season (at least for the blacktip reef shark). Such an approach needs to be conducted in conjunction with other non-lethal acquired information such as clasper calcification or observations of mating scars.
Data availability statement
The raw data supporting the conclusions of this article will be made available by the authors, without undue reservation.
Ethics statement
The animal study was approved by the Animal Ethics Committee, Center National de la Recherche Scientifique (Permit Number: 006725) and was conducted under the authority of the Direction de environment de Polynésie française (DIREN) under the convention 5129/MCE/ENV. The study was conducted in accordance with the local legislation and institutional requirements.
Author contributions
JM: Conceptualization, Data curation, Formal analysis, Investigation, Methodology, Software, Validation, Visualization, Writing—original draft, Writing—review & editing. SP: Funding acquisition, Project administration, Resources, Supervision, Writing—review & editing. SM: Conceptualization, Formal analysis, Funding acquisition, Investigation, Methodology, Project administration, Resources, Validation, Writing—original draft, Writing—review & editing.
Funding
The author(s) declare financial support was received for the research, authorship, and/or publication of this article. Financial support was provided by the Agence Nationale de la Recherche (ANR-11-JSV7-012-01/Live and Let Die) to SM, the Direction à l'Environnement (DIREN) of French Polynesia, the Coordination Unit of the Coral Reef Initiatives for the Pacific (CRISP Program), Proscience in French Polynesia, and the Institut Universitaire de France to SM.
Acknowledgments
We are grateful to the Center de Recherche Insulaire et Observatoire de l'Environnement (CRIOBE) staff for their technical support, as well as the volunteers and students who assisted in field data collection.
Conflict of interest
The authors declare that the research was conducted in the absence of any commercial or financial relationships that could be construed as a potential conflict of interest.
The author(s) declared that they were an editorial board member of Frontiers, at the time of submission. This had no impact on the peer review process and the final decision.
Publisher's note
All claims expressed in this article are solely those of the authors and do not necessarily represent those of their affiliated organizations, or those of the publisher, the editors and the reviewers. Any product that may be evaluated in this article, or claim that may be made by its manufacturer, is not guaranteed or endorsed by the publisher.
Supplementary material
The Supplementary Material for this article can be found online at: https://www.frontiersin.org/articles/10.3389/frish.2023.1284949/full#supplementary-material
References
1. Dulvy NK, Fowler SL, Musick JA, Cavanagh RD, Kyne PM, Harrison LR, et al. Extinction risk and conservation of the world's sharks and rays. Elife. (2014) 3:e00590. doi: 10.7554/eLife.00590
2. Dulvy NK, Pacoureau N, Rigby CL, Pollom RA, Jabado RW, Ebert DA, et al. Overfishing drives over one-third of all sharks and rays toward a global extinction crisis. Curr Biol. (2021) 31:4773–87.e8. doi: 10.1016/j.cub.2021.08.062
3. Pacoureau N, Rigby CL, Kyne PM, Sherley RB, Winker H, Carlson JK, et al. Half a century of global decline in oceanic sharks and rays. Nature. (2021) 589:567–71. doi: 10.1038/s41586-020-03173-9
4. Sherman CS, Simpfendorfer CA, Pacoureau N, Matsushiba JH, Yan HF, Walls RHL, et al. Half a century of rising extinction risk of coral reef sharks and rays. Nat Commun. (2023) 14:15. doi: 10.1038/s41467-022-35091-x
5. Dulvy NK, Simpfendorfer CA, Davidson LNK, Fordham SV, Bräutigam A, Sant G, et al. Challenges and priorities in shark and ray conservation. Curr Biol. (2017) 27:R565–72. doi: 10.1016/j.cub.2017.04.038
6. Dulvy NK, Reynolds JD. Predicting extinction vulnerability in skates. Conserv Biol. (2002) 16:440–50. doi: 10.1046/j.1523-1739.2002.00416.x
7. Simpfendorfer CA, Heupel MR, White WT, Dulvy NK, Simpfendorfer CA, Heupel MR, et al. The importance of research and public opinion to conservation management of sharks and rays: a synthesis. Mar Freshw Res. (2011) 62:518–27. doi: 10.1071/MF11086
8. Whitney NM, Crow GL. Reproductive biology of the tiger shark (Galeocerdo cuvier) in Hawaii. Mar Biol. (2007) 151:63–70. doi: 10.1007/s00227-006-0476-0
9. Harry AV, Simpfendorfer CA, Tobin AJ. Improving age, growth, and maturity estimates for aseasonally reproducing chondrichthyans. Fish Res. (2010) 106:393–403. doi: 10.1016/j.fishres.2010.09.010
10. Awruch CA, Frusher SD, Pankhurst NW, Stevens JD. Non-lethal assessment of reproductive characteristics for management and conservation of sharks. Mar Ecol Prog Ser. (2008) 355:277–85. doi: 10.3354/meps07227
11. Hammerschlag N, Sulikowski J. Killing for conservation: the need for alternatives to lethal sampling of apex predatory sharks. Endanger Species Res. (2011) 14:135–40. doi: 10.3354/esr00354
12. Verkamp HJ, Hammerschlag N, Quinlan J, Langan JA, Sulikowski JA. Preliminary investigation of reproductive hormone profiles in the blacktip shark (Carcharhinus limbatus), a placental viviparous species, in southern Florida. Mar Freshw Res. (2022) 73:520–7. doi: 10.1071/MF21235
13. Carrier JC, Pratt HL, Martin LK. Group reproductive behaviors in free-living nurse sharks, Ginglymostoma cirratum. Copeia. (1994) 1994:646–56. doi: 10.2307/1447180
14. Whitney NM, Pratt HL, Carrier JC. Group courtship, mating behaviour and siphon sac function in the whitetip reef shark, Triaenodon obesus. Anim Behav. (2004) 68:1435–42. doi: 10.1016/j.anbehav.2004.02.018
15. McCauley DJ, Papastamatiou YP, Young HS. An observation of mating in free-ranging blacktip reef sharks, Carcharhinus melanopterus. Pac Sci. (2010) 64:349–52. doi: 10.2984/64.2.349
16. Porcher IF. On the gestation period of the blackfin reef shark, Carcharhinus melanopterus, in waters off Moorea, French Polynesia. Mar Biol. (2005) 146:1207–11. doi: 10.1007/s00227-004-1518-0
17. Bansemer CS, Bennett MB. Reproductive periodicity, localised movements and behavioural segregation of pregnant Carcharias taurus at Wolf Rock, southeast Queensland, Australia. Mar Ecol Prog Ser. (2009) 374:215–27. doi: 10.3354/meps07741
18. Mourier J, Buray N, Schultz JK, Clua E, Planes S. Genetic network and breeding patterns of a sicklefin lemon shark (Negaprion acutidens) population in the Society Islands, French Polynesia. PLoS ONE. (2013) 8:e73899. doi: 10.1371/journal.pone.0073899
19. Chin A, Mourier J, Rummer JL. Blacktip reef sharks (Carcharhinus melanopterus) show high capacity for wound healing and recovery following injury. Conserv Physiol. (2015) 3:cov062. doi: 10.1093/conphys/cov062
20. Carrier JC, Murru FL, Walsh MT, Pratt Jr HL. Assessing reproductive potential and gestation in nurse sharks (Ginglymostoma cirratum) using ultrasonography and endoscopy: an example of bridging the gap between field research and captive studies. Zoo Biol. (2003) 22:179–87. doi: 10.1002/zoo.10088
21. Daly J, Gunn I, Kirby N, Jones R, Galloway D. Ultrasound examination and behavior scoring of captive broadnose sevengill sharks, Notorynchus cepedianus (Peron, 1807). Zoo Biol. (2007) 26:383–95. doi: 10.1002/zoo.20155
22. Whittamore JM, Bloomer C, Hanna GM, McCarthy ID. Evaluating ultrasonography as a non-lethal method for the assessment of maturity in oviparous elasmobranchs. Mar Biol. (2010) 157:2613–24. doi: 10.1007/s00227-010-1523-4
23. Manire CA, Rasmussen LEL, Hess DL, Hueter RE. Serum steroid hormones and the reproductive cycle of the female bonnethead shark, Sphyrna tiburo. Gen Comp Endocrinol. (1995) 97:366–76. doi: 10.1006/gcen.1995.1036
24. Manire CA, Rasmussen L, Gross TS. Serum steroid hormones including 11-ketotestosterone, 11-ketoandrostenedione, and dihydroprogesterone in juvenile and adult bonnethead sharks, Sphyrna tiburo. J Exp Zool. (1999) 284:595–603. doi: 10.1002/(SICI)1097-010X(19991001)284:5<595::AID-JEZ15>3.0.CO;2-6
25. Manire CA, Rasmussen LEL. Serum concentrations of steroid hormones in the mature male bonnethead shark, Sphyrna tiburo. Gen Comp Endocrinol. (1997) 107:414–20. doi: 10.1006/gcen.1997.6937
26. Heupel MR, Whittier JM, Bennett MB. Plasma steroid hormone profiles and reproductive biology of the epaulette shark, Hemiscyllium ocellatum. J Exp Zool. (1999) 284:586–94.
27. Awruch CA, Jones SM, Asorey MG, Barnett A. Non-lethal assessment of the reproductive status of broadnose sevengill sharks (Notorynchus cepedianus) to determine the significance of habitat use in coastal areas. Conserv Physiol. (2014) 2:cou013. doi: 10.1093/conphys/cou013
28. Sulikowski JA, Wheeler CR, Gallagher AJ, Prohaska BK, Langan JA, Hammerschlag N. Seasonal and life-stage variation in the reproductive ecology of a marine apex predator, the tiger shark Galeocerdo cuvier, at a protected female-dominated site. Aquat Biol. (2016) 24:175–84. doi: 10.3354/ab00648
29. Awruch CA. Reproductive endocrinology in chondrichthyans: the present and the future. Gen Comp Endocrinol. (2013) 192:60–70. doi: 10.1016/j.ygcen.2013.05.021
30. Koob TJ, Tsang P, Callard IP. Plasma estradiol, testosterone, and progesterone levels during the ovulatory cycle of the Skate (Raja Erinacea)1. Biol Reprod. (1986) 35:267–75. doi: 10.1095/biolreprod35.2.267
31. Gelsleichter J, Rasmussen LEL, Manire CA, Tyminski J, Chang B, Lombardi-Carlson L. Serum steroid concentrations and development of reproductive organs during puberty in male bonnethead sharks, Sphyrna tiburo. Fish Physiol Biochem. (2002) 26:389–401. doi: 10.1023/B:FISH.0000009292.70958.65
32. Henningsen AD, Murru FL, Rasmussen LEL, Whitaker BR, Violetta GC. Serum levels of reproductive steroid hormones in captive sand tiger sharks, Carcharias taurus (Rafinesque), and comments on their relation to sexual conflicts. Fish Physiol Biochem. (2008) 34:437. doi: 10.1007/s10695-008-9202-9
33. Tricas TC, Maruska KP, Rasmussen LEL. Annual cycles of steroid hormone production, gonad development, and reproductive behavior in the Atlantic stingray. Gen Comp Endocrinol. (2000) 118:209–25. doi: 10.1006/gcen.2000.7466
34. Sulikowski JA, Tsang PCW, Howell WH. An annual cycle of steroid hormone concentrations and gonad development in the winter skate, Leucoraja ocellata, from the western Gulf of Maine. Mar Biol. (2004) 144:845–53. doi: 10.1007/s00227-003-1264-8
35. Awruch CA, Pankhurst NW, Frusher SD, Stevens JD. Endocrine and morphological correlates of reproduction in the draughtboard shark Cephaloscyllium laticeps (Elasmobranchii: Scyliorhinidae). J Exp Zool Part Ecol Integr Physiol. (2008) 309A:184–97. doi: 10.1002/jez.445
36. Hoffmayer ER, Sulikowski JA, Hendon JM, Parsons GR. Plasma steroid concentrations of adult male Atlantic sharpnose sharks, Rhizoprionodon terraenovae, in the northern Gulf of Mexico, with notes on potential long term shifts in reproductive timing. Environ Biol Fishes. (2010) 88:1–7. doi: 10.1007/s10641-010-9603-3
37. Hammerschlag N, Skubel RA, Sulikowski J, Irschick DJ, Gallagher AJ. A comparison of reproductive and energetic states in a marine apex predator (the tiger shark, Galeocerdo cuvier). Physiol Biochem Zool. (2018) 91:933–42. doi: 10.1086/698496
38. Talwar BS, Bond ME, Williams S, Brooks EJ, Chapman DD, Howey LA, et al. Reproductive timing and putative mating behavior of the oceanic whitetip shark Carcharhinus longimanus in the eastern Bahamas. Endanger Species Res. (2023) 50:181–94. doi: 10.3354/esr01231
39. Gelsleichter J. Hormonal regulation of elasmobranch physiology. In:Carrier JC, Musick JA, Heithaus MR, , editors. Biology of Sharks and Their Relatives. Boca Raton, FL: CRC Press (2004). p. 287–323.
40. Snelson FF, Rasmussen LEL, Johnson MR, Hess DL. Serum concentrations of steroid hormones during reproduction in the Atlantic stingray, Dasyatis sabina. Gen Comp Endocrinol. (1997) 108:67–79. doi: 10.1006/gcen.1997.6949
41. Sulikowski JA, Driggers WB, Ingram GW, Kneebone J, Ferguson DE, Tsang PCW. Profiling plasma steroid hormones: a non-lethal approach for the study of skate reproductive biology and its potential use in conservation management. Environ Biol Fishes. (2007) 80:285–92. doi: 10.1007/s10641-007-9257-y
42. Tsang PCW, Callard IP. Regulation of ovarian steroidogenesis in vitro in the viviparous shark, Squalus acanthias. J Exp Zool. (1992) 261:97–104. doi: 10.1002/jez.1402610111
43. Koob TJ, Callard IP. Reproductive endocrinology of female elasmobranchs: lessons from the little skate (Raja erinacea) and spiny dogfish (Squalus acanthias). J Exp Zool. (1999) 284:557–74. doi: 10.1002/(SICI)1097-010X(19991001)284:5<557::AID-JEZ12>3.0.CO;2-P
44. Prohaska BK, Tsang PCW, Driggers WB, Hoffmayer ER, Sulikowski JA. Development of a nonlethal and minimally invasive protocol to study elasmobranch reproduction. Mar Coast Fish. (2013) 5:181–8. doi: 10.1080/19425120.2013.788590
45. Rasmussen LEL, Murru FL. Long-term studies of serum concentrations of reproductively related steriod hormones in individual captive Carcharhinids. Mar Freshw Res. (1992) 43:273–81. doi: 10.1071/mf9920273
46. Garnier DH, Sourdaine P, Jégou B. Seasonal variations in sex steroids and male sexual characteristics in Scyliorhinus canicula. Gen Comp Endocrinol. (1999) 116:281–90. doi: 10.1006/gcen.1999.7369
47. Cuevas ME, Callard G. Androgen and progesterone receptors in shark (Squalus) testis: characteristics and stage-related distribution. Endocrinology. (1992) 130:2173–82. doi: 10.1210/endo.130.4.1547734
48. Sourdaine P, Garnier DH. Stage-dependent modulation of Sertoli cell steroid production in dogfish (Scyliorhinus canicula). Reproduction. (1993) 97:133–42. doi: 10.1530/jrf.0.0970133
49. Borg B. Androgens in teleost fishes. Comp Biochem Physiol C Pharmacol Toxicol Endocrinol. (1994) 109:219–45. doi: 10.1016/0742-8413(94)00063-G
50. Vignaud TM, Mourier J, Maynard JA, Leblois R, Spaet JLY, Clua E, et al. Blacktip reef sharks, Carcharhinus melanopterus, have high genetic structure and varying demographic histories in their Indo-Pacific range. Mol Ecol. (2014) 23:5193–207. doi: 10.1111/mec.12936
51. Mourier J, Mills SC, Planes S. Population structure, spatial distribution and life-history traits of blacktip reef sharks Carcharhinus melanopterus. J Fish Biol. (2013) 82:979–93. doi: 10.1111/jfb.12039
52. Mourier J, Planes S. Direct genetic evidence for reproductive philopatry and associated fine-scale migrations in female blacktip reef sharks (Carcharhinus melanopterus) in French Polynesia. Mol Ecol. (2013) 22:201–14. doi: 10.1111/mec.12103
53. Chin A, Simpfendorfer C, Tobin A, Heupel M. Validated age, growth and reproductive biology of Carcharhinus melanopterus, a widely distributed and exploited reef shark. Mar Freshw Res. (2013) 64:965–75. doi: 10.1071/MF13017
54. Séguigne C, Mourier J, Clua É, Buray N, Planes S. Citizen science provides valuable data to evaluate elasmobranch diversity and trends throughout the French Polynesia's shark sanctuary. PLoS ONE. (2023) 18:e0282837. doi: 10.1371/journal.pone.0282837
55. Mourier J, Vercelloni J, Planes S. Evidence of social communities in a spatially structured network of a free-ranging shark species. Anim Behav. (2012) 83:389–401. doi: 10.1016/j.anbehav.2011.11.008
56. Kiszka JJ, Mourier J, Gastrich K, Heithaus MR. Using unmanned aerial vehicles (UAVs) to investigate shark and ray densities in a shallow coral lagoon. Mar Ecol Prog Ser. (2016) 560:237–42. doi: 10.3354/meps11945
57. McLaughlin RH, O'Gower AK. Life history and underwater studies of a heterodont shark. Ecol Monogr. (1971) 41:271–89. doi: 10.2307/1948494
58. Joung S-J, Chen C-T. Reproduction in the sandbar shark, Carcharhinus plumbeus, in the waters off Northeastern Taiwan. Copeia. (1995) 1995:659–65. doi: 10.2307/1446762
59. Norman BM, Stevens JD. Size and maturity status of the whale shark (Rhincodon typus) at Ningaloo Reef in Western Australia. Fish Res. (2007) 84:81–6. doi: 10.1016/j.fishres.2006.11.015
60. Papastamatiou YP, Caselle JE, Friedlander AM, Lowe CG. Distribution, size frequency, and sex ratios of blacktip reef sharks Carcharhinus melanopterus at Palmyra Atoll: a predator dominated ecosystem. J Fish Biol. (2009) 75:647–54. doi: 10.1111/j.1095-8649.2009.02329.x
61. Mills SC, Mourier J, Galzin R. Plasma cortisol and 11-ketotestosterone enzyme immunoassay (EIA) kit validation for three fish species: the orange clownfish Amphiprion percula, the orangefin anemonefish Amphiprion chrysopterus and the blacktip reef shark Carcharhinus melanopterus. J Fish Biol. (2010) 77:769–77. doi: 10.1111/j.1095-8649.2010.02693.x
62. Debaere SF, Weideli OC, Bouyoucos IA, Eustache KB, Trujillo JE, De Boeck G, et al. Quantifying changes in umbilicus size to estimate the relative age of neonatal blacktip reef sharks (Carcharhinus melanopterus). Conserv Physiol. (2023) 11:coad028. doi: 10.1093/conphys/coad028
63. Dobson S, Dodd JM. The roles of temperature and photoperiod in the response of the testis of the dogfish, Scyliorhinus canicula L. to partial hypophysectomy (ventral lobectomy). Gen Comp Endocrinol. (1977) 32:114–5. doi: 10.1016/0016-6480(77)90088-0
64. Mull CG, Lowe CG, Young KA. Photoperiod and water temperature regulation of seasonal reproduction in male round stingrays (Urobatis halleri). Comp Biochem Physiol A Mol Integr Physiol. (2008) 151:717–25. doi: 10.1016/j.cbpa.2008.08.029
65. Waltrick D, Jones SM, Simpfendorfer CA, Awruch CA. Endocrine control of embryonic diapause in the Australian sharpnose shark Rhizoprionodon taylori. PLoS ONE. (2014) 9:e101234. doi: 10.1371/journal.pone.0101234
66. Nozu R, Murakumo K, Yano N, Furuyama R, Matsumoto R, Yanagisawa M, et al. Changes in sex steroid hormone levels reflect the reproductive status of captive female zebra sharks (Stegostoma fasciatum). Gen Comp Endocrinol. (2018) 265:174–9. doi: 10.1016/j.ygcen.2018.03.006
67. Elisio M, Awruch CA, Massa AM, Macchi GJ, Somoza GM. Effects of temperature on the reproductive physiology of female elasmobranchs: the case of the narrownose smooth-hound shark (Mustelus schmitti). Gen Comp Endocrinol. (2019) 284:113242. doi: 10.1016/j.ygcen.2019.113242
69. Rasmussen LEL, Gruber SH. Serum concentrations of reproductively-related circulating steroid hormones in the free-ranging lemon shark, Negaprion brevirostris. Environ Biol Fishes. (1993) 38:167–74. doi: 10.1007/BF00842913
70. Gelsleichter J, Manire CA, Szabo NJ, Cortés E, Carlson J, Lombardi-Carlson L. Organochlorine concentrations in bonnethead sharks (Sphyrna tiburo) from four Florida estuaries. Arch Environ Contam Toxicol. (2005) 48:474–83. doi: 10.1007/s00244-003-0275-2
71. Sulikowski JA, Kneebone J, Elzey S, Jurek J, Danley PD. The reproductive cycle of the thorny skate (Amblyraid radiata) in the western Gulf of Maine. Fish Bull. (2005) 536–43. Available online at: http://fishbull.noaa.gov/1033/sulihowski.pdf
72. Sulikowski JA, Kneebone J, Elzey S, Jurek J, Howell WH, Tsang PCW. Using the composite variables of reproductive morphology, histology and steroid hormones to determine age and size at sexual maturity for the thorny skate Amblyraja radiata in the western Gulf of Maine. J Fish Biol. (2006) 69:1449–65. doi: 10.1111/j.1095-8649.2006.01207.x
73. Stevens JD. Life-history and ecology of sharks at Aldabra Atoll, Indian Ocean. Proc R Soc Lond B Biol Sci. (1984) 222:79–106. doi: 10.1098/rspb.1984.0050
74. Lombardi-Carlson LA, Cortés E, Parsons GR, Manire CA. Latitudinal variation in life-history traits of bonnethead sharks, Sphyrna tiburo, (Carcharhiniformes : Sphyrnidae) from the eastern Gulf of Mexico. Mar Freshw Res. (2003) 54:875–83. doi: 10.1071/mf03023
75. Conover DO. The Relation between Capacity for growth and length of growing season: evidence for and implications of countergradient variation. Trans Am Fish Soc. (1990) 119:416–30. doi: 10.1577/1548-8659(1990)119<0416:TRBCFG>2.3.CO;2
76. Johnson RH, Nelson DR. Copulation and possible olfaction-mediated pair formation in two species of Carcharhinid sharks. Copeia. (1978) 1978:539–42. doi: 10.2307/1443626
77. Tricas TC, Le Feuvre EM. Mating in the reef white-tip shark Triaenodon obesus. Mar Biol. (1985) 84:233–7. doi: 10.1007/BF00392492
78. Pratt HL, Carrier JC. A review of elasmobranch reproductive behavior with a case study on the nurse shark, Ginglymostoma cirratum. Environ Biol Fishes. (2001) 60:157–88. doi: 10.1023/A:1007656126281
79. Mills SC, O'Donnell JL, Bernardi G, Beldade R. Natural endocrine profiles of the group-living skunk anemonefish Amphiprion akallopisos in relation to their size-based dominance hierarchy. J Fish Biol. (2018) 92:773–89. doi: 10.1111/jfb.13559
80. Scaia MF, Cavallino L, Pandolfi M. Social control of spermatogenesis and steroidogenesis in cichlid fish: a comparative approach. Reproduction. (2020) 159:R31–43. doi: 10.1530/REP-18-0650
81. Maruska KP, Gelsleichter J. Chapter 11—hormones and reproduction in Chondrichthyan fishes. In:Norris DO, Lopez KH, , editors. Hormones and Reproduction of Vertebrates. London: Academic Press (2011). p. 209–37.
82. Craik JCA. An annual cycle of vitellogenesis in the elasmobranch Scyliorhinus canicula. J Mar Biol Assoc U. K. (1978) 58:719–26. doi: 10.1017/S0025315400041369
83. Callard IP, Etheridge K, Giannoukos G, Lamb T, Perez L. The role of steroids in reproduction in female elasmobranchs and reptiles. J Steroid Biochem Mol Biol. (1991) 40:571–5. doi: 10.1016/0960-0760(91)90278-D
84. Tsang PCW, Callard IP. Morphological and endocrine correlates of the reproductive cycle of the aplacental viviparous dogfish, Squalus acanthias. Gen Comp Endocrinol. (1987) 66:182–9. doi: 10.1016/0016-6480(87)90266-8
85. Tsang P, Callard IP. In vitro steroid production by ovarian granulosa cells of Squalus acanthias. Biol Bull. (1983) 23:78–9.
Keywords: non-lethal assessment, shark reproduction, size at sexual maturity, breeding season, estrogen and androgen steroid hormones, mating, parentage analysis, life history traits
Citation: Mourier J, Planes S and Mills SC (2024) Steroid hormones as a non-lethal assessment of the reproductive biology in male and female blacktip reef sharks. Front. Fish Sci. 1:1284949. doi: 10.3389/frish.2023.1284949
Received: 29 August 2023; Accepted: 11 December 2023;
Published: 11 January 2024.
Edited by:
Ryan Daly, Oceanographic Research Institute, South AfricaReviewed by:
Cynthia Awruch, National Scientific and Technical Research Council (CONICET), ArgentinaJuan Carlos Perez Jimenez, The South Border College (ECOSUR), Mexico
Copyright © 2024 Mourier, Planes and Mills. This is an open-access article distributed under the terms of the Creative Commons Attribution License (CC BY). The use, distribution or reproduction in other forums is permitted, provided the original author(s) and the copyright owner(s) are credited and that the original publication in this journal is cited, in accordance with accepted academic practice. No use, distribution or reproduction is permitted which does not comply with these terms.
*Correspondence: Johann Mourier, johann.mourier@umontpellier.fr