- Neurologische Universitätsklinik Freiburg, Freiburg, Germany
The current neurobiological consensus of a general dual loop system scaffolding human and primate brains gives evidence that the dorsal and ventral connections subserve similar functions, independent of the modality and species. However, most current commentators agree that although bees dance and chimpanzees grunt, these systems of communication differ qualitatively from human language. So why is language unique to humans? We discuss anatomical differences between humans and other animals, the meaning of lesion studies in patients, the role of inner speech, and compare functional imaging studies in language with other modalities in respect to the dual loop model. These aspects might be helpful for understanding what kind of biological system the language faculty is, and how it relates to other systems in our own species and others.
Introduction
Current cognitive, neuropsychological and neurobiological theories assume that a dual system scaffolds the organization of the brain. Primate models initially showed that two parallel pathways, an anterolateral or ventral and a caudolateral or dorsal, interact primary with non-primary visual (Mishkin et al., 1983; Kastner and Ungerleider, 2000) or acoustic cortex (Romanski et al., 1999a; Rauschecker and Tian, 2000; Tian et al., 2001). Neuropsychological (Clarke et al., 2000, 2002) and neuroimaging (Ahveninen et al., 2006; Bernal and Ardila, 2009) studies have at first discussed both these pathways also in human visual or acoustic systems and then the model has been extended to the motor system (Rizzolatti and Matelli, 2003) and to higher cognitive functions such as attention (Corbetta et al., 2005) and language (Paulesu et al., 2003; Demonet et al., 2005; Hickok and Poeppel, 2007). A recent review on connectivity of the prefrontal cortex in monkeys confirms the dorsal-ventral dichotomy of projections of long association connections to post-rolandic regions (Yeterian et al., 2012).
It is a novel method, diffusion-tensor-imaging (DTI) based fiber tracking, that finally makes it possible in vivo to identify long human association tracts for ventral and dorsal pathways, similar to animal data (Kreher et al., 2008). The extreme capsule (EmC) and uncinate fascicle (UF) are part of the ventral system, and the superior longitudinal fasciculi (SLF) (SLFI, II, III) and the arcuate fasciculus (AF) are all dorsal pathways (Makris et al., 1999; Wise, 2003; Parker et al., 2005; Anwander et al., 2007; Frey et al., 2008; Thiebaut de Schotten et al., 2011) (See Box 1). Using DTI, it is possible to correlate probabilistic tracking to functional imaging results, relating functionally defined ventral or dorsal pathways to specific tasks and modalities, thus identifying the possible functional role of the underlying pathways (Saur et al., 2010). Obviously, this has particular advantage for those higher faculties that are absent in animals.
Actual data seem to confirm only to some extent initial speculations based on anatomical or functional imaging data. For example, in the attention system, Umarova et al. showed that in addition to dorsal connections, an interaction between temporo-parietal cortex, anterior insula, and inferior frontal gyrus takes place along the ventral network (Umarova et al., 2010), refuting previous assumptions (Corbetta et al., 2005). In contrast to our textbook perception, a dual pathway model (as initially already discussed by Wernicke) is currently also accepted for language (Weiller et al., 2011), with few exceptions (Catani et al., 2005; Ross, 2010). Thus, current neurobiological consensus of a broad dual system scaffolding human and lower primate brains gives evidence that the dorsal and ventral connections subserve similar functions, independent of the modality (Weiller et al., 2011), even if the post- and pre-rolandic areas involved might be different and still modality-dependent.
However, a main difference between humans and lower primates is our unparalleled sophistication in communication capacity. We are not only able to describe objects concretely in words that can be understood by others, but we are also capable of connecting acoustic utterances (words) with very abstract connotations and emotions (“to be or not to be,” “yes we can”). But if language is based in principle on the same dual loop system used for communication in lower primates and this system is also found in other modalities (as perception or attention), why is language unique to humans? We will look at four aspects that are related to the dual loop system, and then ask the question again how unique language is.
What is Special in the Human Dual Loop Model?
To what extent does language share the same anatomical systems as lower primates and what are the differences? Several explanations are possible, and they need not be mutually exclusive. The first possibility (A) is that there is an evolutionary change in a specific pathway (ventral or dorsal). Another possibility (B) is the hypothesis of an anatomical-functional gradient along the dual system, meaning the additional development of pre- and post-rolandic modules located more anteriorly and posteriorly, functionally enabling a new level of interaction between dorsal and ventral pathways.
Anatomical innovations are generally discussed in an evolutionary framework of a serial evolution of humans from non-human primates. However, we will discuss that basic rules of brain organization, which humans still share not only with non-human primates, but also with cetaceans and songbirds, could also lead to a parallel and separate evolution (C).
The Neuroanatomical Differences of a Specific Pathway between Human and Non-Human Species may be Crucial for the Evolution of Language
The location of the EmC within the language zone, specifically connecting Broca's area in the frontal lobe and Wernicke's area in the temporal lobe and inferior parietal lobule, suggests that the EmC may have the prominent role for what Mesulam has called the language communication epicenter (Mesulam, 1998; Schmahmann and Pandya, 2006; Makris and Pandya, 2009; Weiller et al., 2009) and Meynert the “central language complex” (Meynert, 1866; Weiller et al., 2011). Indeed, a recent study of our lab showed that “mapping sound to meaning” crucially relates to the interaction between Broca's and Wernicke's area along connections running through the EmC (Saur et al., 2008, 2010) and electrical stimulation of the anterior floor of the EmC, corresponding to parts of the inferior fronto-occipital fascicle (IFOF), can generate semantic paraphasias (Duffau et al., 2005; Duffau, 2012).
Another, more actively discussed theory proposes that the dorsal pathway projecting from the posterior portion of Broca's area to the superior temporal region—the AF—seems to be of particular importance for language as it is involved in word repetition (Saur et al., 2008) and especially in phrase-structure grammar (Friederici, 2009, 2011). Non-human primates (as well newborns) may not be able to process hierarchically acoustic sequences as well as to repeat complex acoustic signals (Hauser et al., 2001). Fiber tracking studies in chimpanzees, macaques (Macaca mulatta) and humans reveal that particularly the AF within the dorsal pathways, even if undoubtedly present (Petrides and Pandya, 2009), became more prominent during the evolution (Rilling et al., 2008). In a recent comparative study of human and monkey association tracts of the frontal lobe, many similarities were present, but one major difference was found in the AF, with the majority of fibers from this tract projecting to the middle and inferior temporal gyri in human but not in monkey (Thiebaut de Schotten et al., 2012). Moreover, the white matter tracts in the cella media show an asymmetry favoring the left side in the degree of anatomical connectivity by microscopic examination of post-mortem specimens (Galuske et al., 2000), by structural T1 MRI (Paus et al., 1999) and by DTI (Buchel et al., 2004; Nucifora et al., 2005; Hagmann et al., 2006; Powell et al., 2006; Catani et al., 2007), even if further studies are clearly needed to establish the functional-anatomic relationship with respect to lateralization (Hagmann et al., 2006). Structural studies indicate that the dorsal pathway is weaker in children compared to adults (Zhang et al., 2007; Dubois et al., 2008; Lebel et al., 2008) and matures only after the age of seven (Brauer et al., 2011). Given the function of the dorsal system, i.e., the integration of forward and inverse models—from sensorimotor integration involved in language repetition (Saur et al., 2008), to overt or covert speech (Oppenheim and Dell, 2008) to integrative processing of long-time dependence (Friederici et al., 2006), it could be concluded that all these current tracking data indicate that the sophistication of this competence may be essential for the evolution of the language faculty and for human uniqueness in general.
However, whether the thickness of a pathway is the most determinate argument of human evolution is actually unclear. Thickness may be influenced by genetic selection and may depend on practice. In a genetically defined disease, decreased regional anisotropy of the left AF was found in children with Angelman syndrome, pointing to a possible relation between the AF, some aspect of language (i.e., production) and genetic (innate) constraints (Peters et al., 2011; Wilson et al., 2011). However, decreased fractional anisotropy in Angelman syndrome did not selectively involve the AF, but also the inferior fronto-occipital fasciculus, cingulum, anterior thalamic and brainstem radiation, as well as the uncinate fasciculus, suggesting that the loss of UBE3A gene expression may result in a widespread abnormal brain connectivity (Tiwari et al., 2012). Also, more is not always better: increased fractional anisotropy of the superior longitudinal fasciculus is associated with poor visuospatial abilities in Williams Syndrome (Hoeft et al., 2007), another neurodevelopmental disorder, and volumetric increase of arcuate projections are observed in autism (Casanova et al., 2010). Moreover, also practice can influence the size of a pathway. Professional musicians show an increased size of the right AF in relation to the degree of musical expertise (Oechslin et al., 2009), and melodic intonation therapy may induce an increase of fractional anisotropy values along the AF (Schlaug et al., 2009), suggesting a use dependency of the size of the pathway. Continuous speaking may determine the size of the AF, and humans are not only able to chatter but they do it incessantly. Thus, the higher volume of arcuate projections in adults in comparison to children and lower primates may not represent the main argument for human uniqueness of the language faculty.
The Hypothesis of an Anatomical-Functional Gradient along the Dual System
The ability to process syntactically complex rules for sure represents a core component of the human language faculty, as it allows its richness of expressivity that is lacking in the animal communication system, “where each sound is associated with a particular meaning but sounds are not recombined to form a new meaning” (Patel, 2008). However, till now, more detailed studies using combinations of fMRI and DTI are needed to explore this aspect in natural language. In Saur's experiment simple sentences were compared with reversed speech and, therefore, did not give any information about the ability of recursion in language (Saur et al., 2008). Even the capacity to classify items that have already been instantiated in a given pattern into simple phrase-structure sequences as in Friederici's artificial grammar task (Friederici et al., 2006) has to be differentiated from recursion. Indeed, the ability to recognize acoustic patterns defined by a self-embedding, context-free grammar, even if it seems not to be present in non-human primates, at least for the acoustic modality (Hauser et al., 2001), is not unique to humans, but also exists in songbirds (Gentner et al., 2006). Moreover, in Friederici's experiment, processing a more complex grammar involved not only dorsal but also ventral pathways, while more simple finite-state grammar relates only to the ventral one. Grammar complexity may be a factor to differentiate the ventral from the dorsal system (Friederici et al., 2006). Alternatively, processing of local as well as non-adjacent dependencies point to a time-independent analysis, and this aspect is processed along the ventral network (Belin and Zatorre, 2000; Rauschecker and Scott, 2009). Processing of long-distant dependencies could additionally necessitate a time-dependent analysis, which requires a continuous integration of feed-forward and inverse models and thus may be principally relate to the dorsal pathway (Belin and Zatorre, 2000; Rauschecker and Scott, 2009; Weiller et al., 2011). Such functional differentiation—i.e., between time-dependent and time-independent analysis (for further discussion, See section “Comparing The Dual Loop Model In Language With Other Modalities: What Are The Essential Characteristics Of The Ventral And Dorsal Pathways?”)—is also present in monkeys (Zuberbuhler, 2002). The pivotal element for evolution, therefore, as discussed in section “The Neuroanatomical Differences Of A Specific Pathway Between Human And Non-Human Species May Be Crucial For The Evolution Of Language,” may be not the volumetric increase of the projections of a specific pathway, but the higher cellular differentiation of the “terminal” brain regions connected by the “language dual system.” This enables enhanced connectivity of dorsal and ventral pathways and thus simultaneous processing between the two pathways, for which increasing evidence exists, not only in the language modality (Rosazza et al., 2009; Rolheiser et al., 2011), but also in the acoustic (Leavitt et al., 2011), visuospatial (Almeida et al., 2010) and visuomotor modality (Creem and Proffitt, 2001; Mahon et al., 2007).
In the course of the last 50 years or so, in addition to Wernicke's and Broca's area, other regions as supramarginal gyrus (SMG), inferior parietal lobe (IPL), angular gyrus, anterior temporal lobe have been identified to be fundamental for language processing. Although the brains of chimpanzee and macaque,—but also of bonobo, gorilla, and orangutan—show a human-like left-right-hemisphere asymmetry, cellular and functional heterogeneity and similar anatomical connectivity through dorsal and ventral systems (Cantalupo and Hopkins, 2001; Schenker et al., 2008), they possess a smaller (in term of “gyral” white matter) frontopolar cortex and smaller middle temporo-parietal regions (Schenker et al., 2005). At the microscopic level, in Broca's area (Schenker et al., 2008) and in the planum temporale (Anderson et al., 1999; Buxhoeveden et al., 2001a,b), a stronger left-right asymmetry (in terms of cellular density) and a greater horizontal spacing distance are observed in humans. The increased horizontal spacing in humans reflects an increased number of input and output connections and an increased microcircuitry. Indeed, comparative studies on structural connectivity found that in humans, tract terminations in middle and inferior temporal Gyrus, as well as in pars opercularis (Brodmann Area (BA) 44), pars triangularis (BA 45), pars orbitalis (BA 47) of the inferior frontal gyrus and surrounding regions are much stronger than in macaques or chimpanzees (Rilling et al., 2008; Petrides and Pandya, 2009). Ventral interactions between inferior parietal and inferior frontal lobe are reported in old anatomical (Berke, 1960) and DTI-based fiber tracing studies in humans (Saur et al., 2008; Makris and Pandya, 2009; Umarova et al., 2010; Vry et al., 2012). It is still an unsettled question, however, whether these reflect monosynaptic or polysynaptic pathways (Weiller et al., 2011).
All these data may suggest that in a system of two equivalent pathways, hierarchy is not determined by one specific pathway, although specific functions may primarily or crucially involve one of both, but rather by an extension of this system to regulatory, cytoarchitectonically more developed areas in prefrontal, temporal and parietal neocortex in humans (Weiller et al., 2011). The caudal extension along the temporal lobe is observed by processing tones and noise bursts into words and sentences (Rauschecker and Scott, 2009), while the additional involvement of even more caudal temporo-parietal areas (comprising Wernicke's area, angular and inferior parietal gyrus) is associated with increasing semantic complexity (Sharp et al., 2010). A gradient along the frontal and prefrontal cortex is thus linked to increasing abstraction of the underlying processes (Badre and D'Esposito, 2009). In a series of DTI studies from our lab, it seems that the terminations of the ventral system may be more anteriorly and posteriorly than the respective terminations of the dorsal system, in the sense that the ventral system seems to “embrace” the dorsal system (Figure 1). Additionally, an increase in connectivity within and between the cortical regions representing the terminal parts of the dual system could also induce a closer interaction between both dorsal and ventral systems and within each system. It is suggested that such an interaction could especially take place in the prefrontal cortex, with local connections permitting functional interactions of processes in dorsal and ventral pathways (Yeterian et al., 2012).
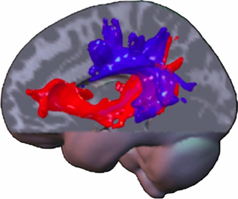
Figure 1. A composite display of tracking-related to dorsal and ventral connections from the different studies of our lab in various domains and modalities illustrates commonalities and differences. The ventral tracts have a wider radius, “embracing” the dorsal ones. Note that trackings between parietal and temporal lobe contain dorsal and ventral pathway-related fibers. The more laterally located dorsal pathway-related fibers may constitute the ascending limb of the arcuate fasciculus. The ventral pathway-related fibers may either use the MdlF for parieto-temporal exchange or a potential “parietal part” of the extreme capsule, which connects parietal cortex with prefrontal cortex (Makris and Pandya, 2009). This tract already displayed on the frozen sections of Ludwig and Klingler (Ludwig and Klinger, 1956). Fiber tracts within the temporal lobe may be related to the MdlF, before aligning with those from the parietal cortex and entering the extreme capsule for the prefrontal cortex, potentially being identical with the anterior part of what is called the IFOF. Note, anatomy is a vehicle for pathways but not identical and assumed fiber location derived from probabilistic tracking may be part of defined strong white matter tracts or not (like AF; SLF, IFOF). The latter ones run through anatomically defined regions, which contain mainly white matter and may be constituted of different long (and short) tracts as the cella media (containing the AF, SLF system) or the extreme capsule. Note: most association connections are reciprocal.
According to this view, the fronto-temporal interactions envelop the insula, the claustrum and the basal ganglia, anatomical structures with projections to almost all cortical regions (Edelstein and Denaro, 2004; Schmahmann and Pandya, 2006; Mathur et al., 2009). The exact role of these regions in language continues to be discussed, but at a very general level there is an agreement that they may carry out mainly integrative functions, enabling the information from one modality with information from other modalities: The basal ganglia seem to play a crucial “integration” role particularly along the dorsal system (Rauschecker and Scott, 2009). In the ventral system, the insula might be responsible for the integration of auditory information with other associative functions (Bamiou et al., 2003), or integration of basic information (features) for initial categorization or grouping (Bamiou et al., 2006). The claustrum seems to bind sensory inputs within and across sensory modalities to generate conscious percepts (Crick and Koch, 2005). Interestingly, Meynert already considered the region including the claustrum, insula and the ascending acoustic fibers in the external and EmC as the central language complex (Meynert, 1866).
Human Independent Evolution through Basic Universal Rules of Cortical Development for Brain Functional and Anatomical Segregation
Despite the deep evolutionary divergence between animals, adaptation to physically dissimilar environments, and very different neuroanatomical organization, accumulating evidence indicates that in all regions of the neocortex in humans and in all other mammalian species thus far evaluated, including dolphins (Mountcastle, 1957, 1978; Purves et al., 1992; Krubitzer, 1995; Manger et al., 1998; Buxhoeveden and Casanova, 2002; Butti and Hof, 2010; Casanova et al., 2010), but also songbirds [African gray parrots (Pepperberg and Shive, 2001) and starlings (Gentner et al., 2006)], the smallest level of vertical and horizontal organization in the cortex consists of cells assembled in minicolumns. Minicolumns represent the basic architectonic and physiological elements by which the neocortex organizes its myriad number of neurons (with various specializations), its pathways and intrinsic circuits into a coherent functional unit (Mountcastle, 1957, 1978; Szentagothai, 1983; Manger et al., 1998; Casanova et al., 2006). Evolution across species is generally related to an increase in the number of radial columnar units without significantly changing the number of neurons within each unit (Rakic, 1995). Indeed, despite the difference in cortex expansion, the size of modules in primates or cetaceans is similar to that described for small-brained mammals like the mouse, suggesting that module size is evolutionarily stable across species (Horton and Hedley-Whyte, 1984; Livingstone and Hubel, 1984; Tootell et al., 1996; Manger et al., 1998). “The ubiquity of modules and the apparent convergent evolution of module size” in primates, cetaceans, and carnivores, animals that are separated by as much as 130 million years of independent evolution, indicate that there may be underlying homologous rules of cortical development that cause “initial segregations” (Manger et al., 1998). One of the possible rules could be the “component placement optimization”: The length of cortico-cortical connections plays a key role in determining module size (Cherniak, 1994): the shorter the connections the more efficient the processing (Ringo, 1991; Szymanski et al., 1995). In humans, the number of modules increases with as a consequence longer association tracts connecting more distant modules in different areas. For the evolution of biological life, rapid reaction time to sensory input is crucial. Therefore, modules of a limited size are conserved also in an animal whose neocortex has undergone a huge expansion, where long cortico-cortical connections would require a large volume of metabolically active tissue and an increased conduction time (Horton and Hedley-Whyte, 1984; Tootell et al., 1996). The restricted range in size of modules having diverse cytoarchitectonic or histochemical features across highly divergent mammalian species may reflect “an independent evolution possibly due to selection for an optimal connection length” (Manger et al., 1998).
“Component placement optimization” and its balance with evolutionary constant increase of the minicolumns could explain why short connections, dorsal along the SLF as well ventral along EmC, are constant in primate evolution. The optimization of connective processes within minicolumns could be one of the fundamental criterions to adapt minicolumns in the various cortical areas according to their specific developmental and functional requirements. Species-specific differences of modular organization mainly regard the horizontal minicolumn spacing and the resulting numbers of the input and output pathways. In chimpanzees, high density modules localized in primary visual cortex (area 17) seem to be crucial to process color, form and motion; in dolphins, cell clusters in insula (Area 1) may subserve the processing of complex auditory stimuli associated with dolphin communication. So, a more extensive parcellation and the resulting increased hierarchical organization of modular cortical subdivisions of species-specific brain regions seems to led to functional optimization. Even if parcellation can theoretically be found throughout the cortex regardless of functional attributes, it selectively affects brain regions that are known to be functionally specialized.
Thus, it is maybe no coincidence that in humans, the presence of larger minicolumns spacing, as far as is known until now, is characteristic only of language-related cortical areas: in Broca's area (Amunts et al., 1999) and in the planum temporale (Anderson et al., 1999). No asymmetries were found in these two regions in chimpanzees and other primates (Sherwood et al., 2007). A recent tracking study showed not only a cytoarchitectonic, but also a tractographic parcellation within Broca's area (Anwander et al., 2007). Williams et al. made the step from micro- to macro-organization of the brain. They showed that compared to control subjects, autistic persons exhibit a reduced minicolumnar width and peripheral neuropil spacing, as well as an increased number of minicolumns (Williams and Casanova, 2010). This correlates with an increment of short connections (coming from each single minicolumn), which could be related to the highly efficient analytic processing sometimes observed in savants, and a decrease of longer connectivity (particularly of AF and cingulum bundle), which may relate to a deficit in the identification of relationships.
Both humans and non-human primates have commonalities in anatomy, and the capability of language in humans is often put in relation to these differences in anatomy, e.g., a larger AF in humans, as a “next step” in evolution, enabling e.g., complex grammar. However, humans did not evolve from the apes but we evolved with the apes from a common ancestor. As we discussed above, similarities of modular arrangements between homo sapiens and animals are not necessarily the product of the same development, but basic brain rules of brain organization can lead to new functions, even if emerging from similar structures (Northcutt and Kaas, 1995; Vates and Nottebohm, 1995). This could limit the significance of comparative studies on anatomical data in humans and non-human primates.
Can Human Anatomy of the Dual Loop Model be Reconciled with Cognitive Models of Speech?
The different perspectives of cognitive models and (anatomy-based) neurosciences seem to converge on a “two route model.” The famous “house model” of the early aphasiologists derived from patients studies [e.g., Lichtheim and Wernicke (Weiller et al., 2011)] has remained the basic framework for later models of single word processing, for example, Morton's Logogen model (Morton and Patterson, 1980). The latter remains the reference for cognitive studies of aphasic individuals. Cognitive models of speech processing and word production have, on the other hand, included evidence from psycholinguistic studies. All models contain representations both of phonological segments and of conceptual knowledge (Table 1). They differ, however, with regard to the number of levels, which mediate between phonological and conceptual information, i.e., whether processing is fully interactive or whether interaction is restricted. In the model of Levelt et al. two lexical levels mediate between semantics and phonology while the interaction between these levels is limited (Levelt et al., 1999). In contrast, the model of Dell et al. assumes a single lexical level while activation spreading is highly interactive (Dell et al., 1997). A similar debate between interactive accounts (MacDonald et al., 1994) and more modular models (Frazier, 1987; Friederici, 2002) has taken place in the area of sentence processing (see Friederici, 2002 for review).
In all models of word processing, repetition can be carried out along two independent routes. A “non-lexical route” maps perceived phonemes onto the response buffer and, subsequently, to articulation, while comprehension requires activation of semantic knowledge from auditory input. The conceptual representations may activate the word in a speaker's output lexicon, thus allowing for a second route for repetition.
A cognitive dual route model is also supported by the observations in the context of a recent case study from our laboratory (Bormann and Weiller, 2012). The subject in this study, BB, exhibited double dissociations between her ability to comprehend auditory words and to repeat these words. Occasionally, she would be able to repeat a word without comprehending its meaning (Examiner: “Please repeat the word ‘hedgehog.’ BB: ‘Hedgehog, hedgehog,’ I wonder what a hedgehog is.”). On other occasions, she would indicate comprehension of the word without being able to repeat it (Examiner: “Please repeat the word ‘hedge.’ BB: ‘I know this one!’ It's green, it may surround the garden, it may grow really high. But what was the word exactly?”). These examples provide evidence for a double dissociation between comprehension of a word's meaning and the ability to repeat words without comprehension. On the other hand, when available, both routes interact and contribute to word repetition (Jefferies et al., 2005).
The two routes assumed in cognitive models may be associated with the dorsal and ventral routes identified in our anatomic studies. The logogen model's non-lexical route may correspond to the dorsal route while comprehension of a word's or sentence's meaning may be associated with the ventral pathway. Our subject BB had severe deficits repeating non-words, so for repetition, she was mainly relying on the word's meaning. Semantic errors in repetition (Examiner: “Please repeat the word ‘raw’.” BB: “Was it ‘meat’. I should say it was ‘meat’”) further support this conclusion. Within a cognitive model, her lesions would affect phonology to articulatory mapping and a predominant reliance upon a word's meaning during repetition.
While it is generally accepted that language as other cognitive functions emerges from context dependent interaction in distributed, segregated and overlapping networks (Damasio, 1989; Mesulam, 1990), this knowledge has not really spread to patients studies. With the advent of DTI tracking techniques the network architecture of functions is reflected anatomically. If the dorsal tract would be needed for repetition and the ventral tract for comprehension (Saur et al., 2008), it seems attractive to attribute isolated repetition problems to lesions of the dorsal tract and relate transcortical sensory aphasia, i.e., mainly comprehension deficits, to lesions of the ventral tract. However, attributing functions to tracks rather than to regions would just shift the focus but not solve the general problem of phrenology. In a network approach, the different cortical regions interact closely, and thus are not independent. Interruption of the network has an impact on the remaining (“intact”) parts of the network. In other words, the functions of Broca's or Wernicke's area with an intact AF may not be the same as after that fascicle's destruction. This view does not contradict the assumption that a particular connection in a network on its own is necessary for e.g., repetition. However, the destruction of the interconnection may not result in a solitary repetition failure, but in a complete new phenomenological constellation, as the tract lesion affects the function of the regions it connects, and other regions in the remaining network, including in the other hemisphere, may become operational (Weiller et al., 1995). These assumptions make the complexity and fuzzy link of aphasic syndromes to focal lesions more understandable.
The difference may become clearer when comparing the method of symptom-lesion-mapping (e.g., Bates et al., 2003; Dronkers et al., 2004) with the “natural” occurrence of aphasic syndromes, i.e., compositions of symptoms. Across a series of 100 consecutive acute aphasics, comprehension problems (a score construed from tests examining: following verbal commands, word and sentence comprehension and results in the token test) using the Brunner–Munzel test map onto the EmC. Repetition problems (automated sequences as numbers or weekdays) map onto the AF (Kümmerer et al., 2010). Does this mean that comprehension is exclusively coded for or represented in the EmC? Rather not: in a network approach the observed comprehension deficit is produced by a lesion of the widespread “comprehension network.” Why then do the infarcts of patients with comprehension deficit map onto the EmC, rather than let's say, Wernicke's area? The reason may be that the EmC holds a strategic position within the comprehension network. Within the distributed “comprehension network” (Friederici et al., 1999; Vigneau et al., 2006) all cortical regions in the temporal lobe (anterior and posterior MTG, fusiform gyrus) are connected with regions in the inferior frontal gyrus (BA 45 and BA 47) via a relatively small tract through the EmC (Saur et al., 2010). The EmC represents a kind of bottleneck of the network or in other words is the site where a small lesion can affect the entire network. Simultaneously it is situated in the middle of the middle cerebral artery (MCA) territory. Therefore, chances are highest that infarcts of the anterior or posterior MCA territory overlap in this small region, causing an interruption of the comprehension network.
This kind of lesion-mapping approach assumes that comprehension problems are independent from other potential deficits. However, only looking at comprehension problems is an “unnatural” setting. In most cases single symptoms should not be seen in isolation. There are only very few patients with solely a comprehension (or a repetition) problem. Symptoms of patients with aphasia cluster in syndromes that can be defined and reliably classified. This is due to the irrigation territories of the MCA and due to the organization of language in the brain. Can the syndrome of Wernicke's aphasia be explained by a lesion of the posterior part of the temporal lobe (e.g., Wernicke's area)? Comprehension problems are a hallmark of Wernicke's aphasia and indeed most people would agree that the temporal lobe does play a role in semantics. But Wernicke aphasia is characterized by more features, e.g., fluent speech with prominent semantic or phonemic paraphasias, thus also a “defect” in speech production. Wernicke's area participates in the ventral as well as the dorsal pathway and a lesion there will have to affect the functioning of both pathways. While semantic jargon may be due to comprehension problems, referring to the ventral pathway, phonemic jargon may be related to a lesion of the dorsal pathway. The sylvian parieto-temporal region (Area SPT) participates in sound-to-articulation mapping in the dorsal pathway (Hickok and Poeppel, 2000). As Wernicke himself put it: “the word images do not take appropriate control over the motor images” (Wernicke, 1874). Thus, Wernicke's aphasia represents a new phenomenological constellation, a syndrome, more than just comprehension problems. The occurrence of this syndrome is explained by the irrigation of the posterior temporal lobe by a branch of the MCA and its presentation is due to the lesion of the temporal lobe and the lesion of both the ventral and the dorsal pathway. For this interpretation we do not have to assume any form of diaschisis or reorganization.
Conduction aphasia due to a lesion of the AF is another problem. Destruction of the AF affects the dorsal pathway, but the arcuate fascicle does not in itself contain the representation for repetition, instead the pathway's functionality is needed also for repetition, and vice versa repetition may relay on the ventral pathway as well. Repetition should be altered and comprehension may be intact (via the ventral pathway) in most cases with AF lesions. Repetition of pseudowords also activates the ventral system (Saur et al., 2008), but this may merely reflect lexical search when trying to identify pseudowords. However, there is patient evidence that both routes contribute to repetition. The rare syndrome of deep dysphasia where subjects make semantic errors in single word repetition (e.g., repeating “crown” as “king”) suggests a role of semantics in repetition. In addition, aphasic patients are better in repeating words they comprehend and are able to name in picture naming tasks in comparison to words which they do not understand (Jefferies et al., 2005). Semantic deficits may lead to mild repetition impairments because of the reduced support from meaning (Jefferies et al., 2005). Thus, repetition may be affected also outside conduction aphasia and lesions of the AF. Moreover, conduction aphasia is not restricted to repetition problems. What else would we have to expect with lesions of the AF, when referring to the dual loop system? Paraphasia through incorrect sensorimotor mapping in the dorsal pathway, “conduit d' approche” aiming to correct along the ventral pathway and also working memory deficits are often reported in patients with conduction aphasia. As in Wernicke's type aphasia, conduction aphasia is not to be reduced to repetition problems, and repetition not reduced to the AF.
The two pathways have different computational abilities, which are a prerequisite for various functions, depending on the modality. Lesions of a tract do affect the working of the entire network, resulting in a new phenomenological constellation, the syndrome is patient is presenting with. This syndrome is different from the loss of the supposed function, mediated by the pathway. Similarly, neglect and extinction can be differentiated by different effects of the lesions on the visuo spatial attention system (Umarova et al., 2011).
From Sound to Concept and Back: The Development of Inner Speech and the Dual Loop Model
So far, language in humans was discussed from an evolutionary view and in the perspective of cognitive models applied to patients with aphasia. However, the data do not yet explain how humans acquire the capacity for higher order thinking or abstraction. In this section, we want to argue that the development of “inner speech” in humans might be crucial for a simultaneous and close interaction between the two pathways, enabling the combination of phonological and abstract thought.
Jackendoff defined language as “essentially a mapping between sound and propositional or conceptual thought” (Jackendoff, 2009). Exactly how this mapping is achieved has been investigated in the context of psycholinguistic studies of language processing. Most cognitive models conceive of conceptual representations as independent from linguistic knowledge (Table 1) (Wernicke, 1906; Morton and Patterson, 1980; Freud, 1891; Dell et al., 1997; Gaskell and Marslen-Wilson, 1997; Levelt et al., 1999; Lambon Ralph et al., 2002). An aphasic speaker may be perfectly aware of the concept he or she is trying to name yet may be unable to access the word. Likewise, severely aphasic individuals may be unimpaired in tests of non-verbal reasoning, problem-solving and memory (Kertesz and Mccabe, 1975). There are several other examples of non-verbal thinking, such as face processing, mental rotation, spatial navigation, or tool use. Several contents of our mind do not map on lexical concepts and linguistic operations. In contrast, some aspects of language require less cognitive processing, e.g., the generation of automatic phonological sequences like counting or generating days of the week.
In most models, the process from sound to thought (and back) passes different levels of representation (Table 1). However, Vygotzky suggested that abstract thought processes and speech overlap, like two intersecting circles (Vygotzky, 1934). The overlapping part of thought and speech represents so-called “inner speech” or, as Vygotzky put it: “In their overlapping parts, thought and speech coincide to produce what is called verbal thought or inner speech, depending on the point of view.” In inner speech, several aspects from purely sensorimotor to more abstract can be discerned. Especially in the early French literature, inner speech (“notion du mot”) was seen purely as a means of (internal) sensorimotor mapping, or connecting phonological input and output properties, of course then related to working memory. Wernicke stated “The main task of the child that learns to speak is the imitation of the heard word” and this task, according to Wernicke, was performed by the direct (nowadays: dorsal) connection. “Only later the child is able to bind the word with a defined concept, long after the word has become a vast asset” (Wernicke, 1874). But later on words would be spoken via the semantic route: “Soon after we have learned to speak a word, we lose the intention only to reproduce sounds and plan to utter a meaning” (Wernicke, 1874). “We have to assume that (then) the majority of speech impulses reach the word concepts from the remaining cerebral cortex” (Wernicke, 1906). Recently, Oppenheim and Dell showed that inner speech is more abstract on a phonological level, because covert segmental errors produced with tongue twisters were less similar to the target phoneme than overt slips of the tongue (Oppenheim and Dell, 2008). Therefore, internalization of speech is more than internalizing the production and anticipation of sound. It is combined with a concomitant increasing understanding what these phonological internal representations mean.
Only few patient studies exist investigating this topic. In a rhyming paradigm, where orthography alone was not sufficient to determine the sound of the rhyme, Geva et al. showed that in aphasic subjects, deficits of inner (= covert) speech, over and above deficits in overt speech production and working memory, were found following lesions to the left pars opercularis of the inferior frontal gyrus (BA 44) and the SMG (Geva et al., 2011). The authors concluded that for this aspect of inner speech, it was mainly the dorsal pathway that was affected. A recent paper shows that at birth, anterior and posterior language zones can be activated specifically but are not yet fully functionally connected (Perani et al., 2011), and that the interaction between the two regions becomes significantly synchronized around 7 years of age (Friederici et al., 2011). This finding was put in relation to the fact that the dorsal pathway has not yet fully matured (Brauer et al., 2011) and that children up to the age of seven are rather poor at comprehending syntactically complex sentences (Hahne et al., 2004; Dittmar et al., 2008; Dubois et al., 2008).
Also the full development of inner speech appears to occur around this age. According to Vygotsky, we do have conceptual awareness at birth, but no inner speech (Vygotzky, 1934). Young children start by accompanying their actions with speech, which evolves into “egocentric” or “private” speech while thinking aloud around the age of 4. In the process of a few years till the age of around 7 years, egocentric speech is replaced by inner speech (Vygotzky, 1934; Ehrich, 2006; Wiley, 2006). Behavioral studies in normally developing children and those with attention deficit hyperactivity disorder (ADHD) and autism support the importance of this process. The amount of internal speech in children correlates with performance (Winsler et al., 2000, 2003; Winsler and Naglieri, 2003; Ostad and Sorensen, 2007). Children with ADHD seem to have a delayed development of internalization (Berk and Potts, 1991), while on the other hand, in high performing children with autism, private speech remains relevant for performance (Winsler et al., 2007).
All these different aspects suggest that it is only after the internalization of speech and complete interaction of both pathways within the dual loop model that it is possible to simultaneously combine phonological and abstract thought simultaneously (Figure 2). This might also be a reason why complex grammar, for which simultaneous analysis of both time-dependent and time-independent processing is required, can only be mastered after the age of seven. Only then can we use language as a tool to represent abstract concepts (Deutscher, 2005), and, as Jackendoff puts it, use language as a “scaffolding that makes possible certain varieties of reasoning more complex than are available to non-linguistic organisms” (Jackendoff, 1997), permitting self-description and reflection, self-questioning and problem-solving, (Diaz and Berk, 1992; Barkley, 2001).
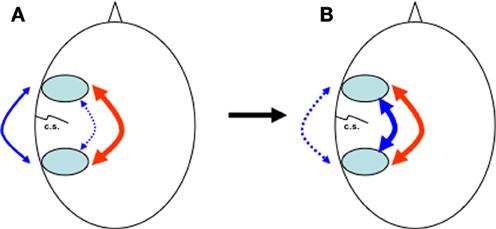
Figure 2. Heads seen from above with pre- and post-rolandic areas around the central sulcus (c.s.). (A) At birth, dorsal anatomical connections (blue, dotted line) between pre- and post-rolandic areas are present but immature. In the first years, influenced by the continuous percept and anticipation of the consequence of movement and speech via feedback (blue, continuous line), internal connections and representations synchronise and mature. (B) Over time, external behaviour (blue, dotted line) is increasingly replaced by internal representations (blue, continuous line), while the interaction between dorsal (blue, continuous line) and ventral (red) pathways increases. For novel tasks, the external pathway can still be used.
It is suggested that during this crucial age of 4–7 years in children, not only in language, but in all modalities the emergence of internal representations takes place (Vygotzky, 1978; Diaz and Berk, 1992), “following the same general sequence of stages as the internalization of speech” and outer-directed behavior becomes turned on the self as a means to control one's own behavior (Barkley, 2001).
Comparing the Dual Loop Model in Language with Other Modalities: What Are the Essential Characteristics of the Ventral and Dorsal Pathways?
There is no reason to suppose that the organization of the acoustic language system is different from other modalities. Current scientific evidence shows that a dual loop model, consisting of a dorsal and ventral pathway, can be found in different modalities, providing a scaffolding system for processing. In this dual loop model, hierarchy is not determined by one specific pathway, although specific functions may primarily or crucially involve one of both, but rather by an extension of this system in humans to regulatory, cytoarchitectonically more developed areas in prefrontal, temporal, and parietal neocortex (Weiller et al., 2011).
In Table 2 we summarize some of the main studies reporting the involvement of a dorsal or a ventral pathway by different tasks. Also listed are a series of (partly unpublished) studies from our lab, in which we used DTI-based fiber tracking (Kreher et al., 2008) to connect seed regions in post- and prerolandic brain regions active during fMRI. In all examined modalities (language, motor, attention), we found ventral and dorsal connections along the EmC and the AF/SLF systems, respectively (Figure 1). Thus, functions ascribed to the dorsal and ventral pathway in each modality may differ, but only in their modality-specific aspect.
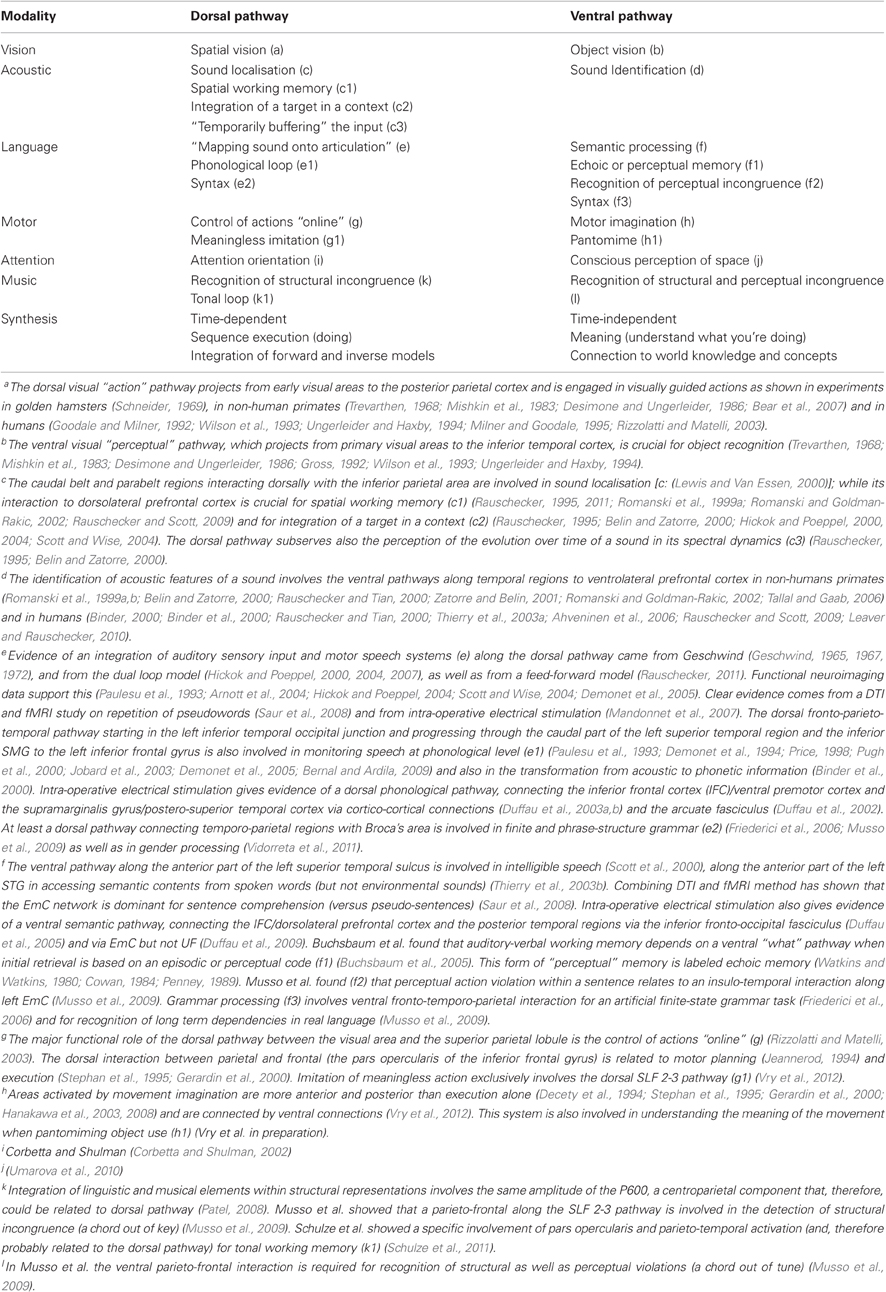
Table 2. Summary of possible functions per modality processed along the two pathways from different studies.
A Ventral Pathway for Time-Independent Processing
The ventral pathway was first described as the “what” pathway in the visual system of chimpanzees (Mishkin et al., 1983) and humans (Ungerleider and Haxby, 1994). Later, this function was assigned to the acoustic system as well. A ventral pathway, connecting the anterior belt and parabelt with the anterior temporal regions underlies auditory object identification (Romanski et al., 1999a,b; Rauschecker and Scott, 2009), with the anterior part of the left supratemporal gyrus (STG) involved in accessing semantic contents from spoken words vs. environmental sounds (Thierry et al., 2003b) as well from intelligible speech (Scott et al., 2000), while the inferior frontal gyrus (pars orbitalis) is necessary for sentence comprehension (as compared to pseudo-sentences) (Saur et al., 2008). Confirmation of the existence of a ventral semantic pathway, connecting the inferior frontal gyrus/dorsolateral prefrontal gyrus (IFG/DLPFG) and the posterior temporal regions via the inferior fronto-occipital fasciculus and EmC, but not the UF, comes from intra-operative electrical stimulation (Duffau et al., 2005, 2009). These data indicate that the antero-ventral speech processing pathway is crucial for mapping acoustic-phonemic cues onto lexical representations (Scott and Johnsrude, 2003).
Is thus “meaning” the more general function of this pathway? Several kinds of evidence show that the ventral pathway is involved in the identification of adjacent as well as non-adjacent syntactic relations of the perceived linguistic elements (Friederici et al., 2006; Musso et al., 2009) and of tonal dependencies (Musso et al., 2009). The ventral route is thus involved in the identification of structural relations independent of the modality and of the time of occurrence of each element.
A ventral pathway through the EmC seems to play an important and similar role also in other modalities. In the attentional system, a ventral tract, connecting the parietal and temporal lobe with the anterior insula and the ventrolateral prefrontal cortex, was interpreted as being critical for the integration of the perception of space for an intended action and for the correct estimation of the relevance of stimuli to the self (Umarova et al., 2010). In the motor system, areas in posterior parietal and prefrontal cortices involved in imagery of movements (Vry et al., 2012) as well in pantomiming object use (Vry et al., in preparation) are connected via the ventral route, putting the ventral tract in relation to symbolic acts and cognition.
We speculate that a more general function of the ventral system could be the extraction, relation and implementation of an invariant set of properties of the perceived elements (in a limited number of given possibilities, i.e., as “a priori” categories), which are related to semantic memory and meaning. Processing along the ventral pathway is, therefore not dependent on the temporal or spatial sequence of elements, rather it is optimized to test a limited number of possible combinations in order to extract meaning (Weiller et al., 2011).
A Dorsal Pathway for Time-Dependent Processing
The dorsal route was initially labeled the “where” pathway as it is found to be involved in processing spatial relations between visual or acoustic perceived objects as well as between oneself and external objects to identify visual motion and is used for the visual control of action (see Table 2, i–k). The term “how” pathway for the dorsal pathways was introduced later and relates to sensory-motor integration function (Kravitz et al., 2011). Individuals with brain damage of the dorsal visual pathway affecting the posterior and the superior parietal cortex suffer from optic ataxia. In this condition, a deficit of the visuomotor system, the size, shape, and color and even the location (the “where”) of the object remain intact, but the ability to identify object arrangements and to perform “goal-directed actions to visual targets” with any sort of precision and accuracy is disturbed (Perenin and Vighetto, 1988; Goodale et al., 1991; Milner et al., 2003). The auditory dorsal pathway is predominantly related to speech production (e) and was demonstrated to be involved in non-word repetition, thereby providing a phonology-to-articulation interface for correct speech (see Table 2, e1). Because the representation in semantic memory is lacking, the sensory percept of pseudowords has to be mapped on the motor representations for repetition.
However, the function of the acoustic dorsal pathway seems not to be limited to “mapping sound onto articulation” (Table 2), but rather serves to integrate linguistic or musical syntactic elements in a context (Patel, 2008; Musso et al., 2009), even to process phrase-structure grammar (Friederici et al., 2006), or to integrate and maintain the perceived auditory signals within context over temporal and spatial evolution (see Table 2, c2,3, e1).
Thus, the acoustic dorsal pathway would not be limited to “where” or “how” functions, rather its more general role, independent from the modality, is the capacity to analyze the sequence of segments, either in time or in space, as well as fast online integration between sensory event information and “internal models or emulators (Rauschecker and Scott, 2009). Spatial transformation as well as sensorimotor integration may be examples of adaptations used by forward models (predictors) and inverse models (controllers) (Rauschecker and Scott, 2009).
Through exercise and experience, moulds are developed, which can be called on quickly. By learning a movement like a signature, parameters for this movement are stored and can be accessed by another extremity on demand (Rijntjes et al., 1999). Thus, in the dorsal pathway, in contrast to the ventral pathway, stable connotations like blueprints can be developed in an infinite number of possibilities, the only constraint being the physical and computational limitations of movement themselves.
The mechanism for “online” analysis of sequences may be seen as a function of the dorsal system in other modalities as well. A dorsal network was recently described in the attentional network, where dorsal pathways along the superior longitudinal fascicle/AF system connected the parietal and temporal lobe with the premotor cortices (BA 6, 44, 8) and was interpreted to convey information needed for spatial stimulus orientation or processing of peri-personal space (Tables 1, 2) (Umarova et al., 2010). A similar pattern seems to exist in the motor system: during simple, repetitive, externally cued active or passive movements, a dorsal route connects the parietal cortex with premotor areas for sensorimotor mapping, motor control based on internal predictive models and sensory feedback (Wolpert et al., 1995; Wolpert and Miall, 1996; Grush, 2004; Shadmehr and Krakauer, 2008; Vry et al., 2012).
It is not clarified whether or not working memory predominantly uses the dorsal pathway. It is generally acknowledge that the frontal areas involved in working memory, as pars opercularis or precentral cortex, are mainly connected via the dorsal fiber system with parietal and temporal lobe (Paulesu et al., 1993; Wager and Smith, 2003). These tracts may be involved in short-term retention of the phonological input and lesions to the inferior parietal areas in the left hemisphere usually cause verbal short-term memory impairments (Vallar et al., 1997). In a recent fMRI study Buchsbaum et al. distinguish between perceptually based (“echoic”) memory, which relates to the ventral pathway, from phonological-articulatory memory, which was confirmed to be a predominant dorsal task (Buchsbaum et al., 2005).
Language: How Unique is It?
In summary, after internalization of speech and movement, a dual loop system, consisting of efficiently and flexibly interacting dorsal and ventral pathways, extending to a highly developed gradient along pre- and post-rolandic regions, seems to be basis of similar functions in all modalities, including language. We started with the assumption that language is unique in humans, and there are indeed anatomical reasons to support this notion. However, since there are so many anatomical and functional similarities with other modalities, it seems justified to ask the question: to what extent do also other modalities have unique properties in humans?
Should we expect that also human motor processing and attention is different from lower primates? Are humans better in understanding the meaning of movement than animals? Can a chimpanzee understand the meaning if a human experimenter pantomimes peeling a banana? Humans may have a thicker AF, which may allow them to speak easily and a lot. Are they therefore better in motor skills as well, can they therefore perform a perfect serve in tennis, slalom on a steep skiing slope or perform a complex piano play “by heart”? If language, through inner speech, is a prerequisite for the wealth of our inner world, is there an equivalent of inner speech in other modalities, and what is it like?
Again, the development of the dorsal and ventral pathway, in the frontal lobe converging on Broca's area, could be the crucial anatomical feature. Numerous studies have shown an involvement of area 45 when processing hierarchical structures not only in the language modality (Musso et al., 2003), but also in movement (Binkofski and Buccino, 2004; Fiebach and Schubotz, 2006; Tettamanti and Weniger, 2006), music (Tettamanti and Weniger, 2006; Musso et al., 2009), and in the homologue area of the right hemisphere, for attention (Umarova et al., 2010). If so, it is an unresolved question whether the differentiation in dorsal and ventral connections in humans along an anterior-posterior gradient, especially in Broca's area, evolved primarily for language and that other modalities were able to use these highly differentiated functional structures, or that a parallel development enabled all modalities, including language, to attain human specific attributes.
Conflict of Interest Statement
The authors declare that the research was conducted in the absence of any commercial or financial relationships that could be construed as a potential conflict of interest.
References
Ahveninen, J., Jaaskelainen, I. P., Raij, T., Bonmassar, G., Devore, S., Hamalainen, M., Levanen, S., Lin, F. H., Sams, M., Shinn-Cunningham, B. G., Witzel, T., and Belliveau, J. W. (2006). Task-modulated “what” and “where” pathways in human auditory cortex. Proc. Natl. Acad. Sci. U.S.A. 103, 14608–14613.
Almeida, J., Mahon, B. Z., and Caramazza, A. (2010). The role of the dorsal visual processing stream in tool identification. Psychol. Sci. 21, 772–778.
Amunts, K., Schleicher, A., Burgel, U., Mohlberg, H., Uylings, H. B., and Zilles, K. (1999). Broca's region revisited: cytoarchitecture and intersubject variability. J. Comp. Neurol. 412, 319–341.
Anderson, B., Southern, B. D., and Powers, R. E. (1999). Anatomic asymmetries of the posterior superior temporal lobes: a postmortem study. Neuropsychiatry Neuropsychol. Behav. Neurol. 12, 247–254.
Anwander, A., Tittgemeyer, M., Von Cramon, D. Y., Friederici, A. D., and Knosche, T. R. (2007). Connectivity-based parcellation of Broca's area. Cereb. Cortex 17, 816–825.
Arnott, S. R., Binns, M. A., Grady, C. L., and Alain, C. (2004). Assessing the auditory dual-pathway model in humans. Neuroimage 22, 401–408.
Badre, D., and D'Esposito, M. (2009). Is the rostro-caudal axis of the frontal lobe hierarchical? Nat. Rev. Neurosci. 10, 659–669.
Bamiou, D. E., Musiek, F. E., and Luxon, L. M. (2003). The insula (Island of Reil) and its role in auditory processing. Literature review. Brain Res. Brain Res. Rev. 42, 143–154.
Bamiou, D. E., Musiek, F. E., Stow, I., Stevens, J., Cipolotti, L., Brown, M. M., and Luxon, L. M. (2006). Auditory temporal processing deficits in patients with insular stroke. Neurology 67, 614–619.
Barkley, R. A. (2001). The executive functions and self-regulation: an evolutionary neuropsychological perspective. Neuropsychol. Rev. 11, 1–29.
Bates, E., Wilson, S. M., Saygin, A. P., Dick, F., Sereno, M. I., Knight, R. T., and Dronkers, N. F. (2003). Voxel-based lesion-symptom mapping. Nat. Neurosci. 6, 448–450.
Bear, M. F., Connors, B. W., and Paradiso, M. A. (2007). Neuroscience: Exploring the brain. Baltimore, MD: Lippincott Williams and Wilkins.
Belin, P., and Zatorre, R. J. (2000). ‘What’, ‘where’ and ‘how’ in auditory cortex. Nat. Neurosci. 3, 965–966.
Berk, L. E., and Potts, M. K. (1991). Development and functional significance of private speech among attention-deficit hyperactivity disordered and normal boys. J. Abnorm. Child Psychol. 19, 357–377.
Berke, J. J. (1960). The Claustrum, the external capsule and the extreme capsule of Macaca mulatta. J. Comp. Neurol. 115, 297–331.
Bernal, B., and Ardila, A. (2009). The role of the arcuate fasciculus in conduction aphasia. Brain 132, 2309–2316.
Binder, J. R., Frost, J. A., Hammeke, T. A., Bellgowan, P. S., Springer, J. A., Kaufman, J. N., and Possing, E. T. (2000). Human temporal lobe activation by speech and nonspeech sounds. Cereb. Cortex 10, 512–528.
Binkofski, F., and Buccino, G. (2004). Motor functions of the Broca's region. Brain Lang. 89, 362–369.
Bormann, T., and Weiller, C. (2012). “Are there lexicons?” A study of lexical and semantic processing in word-meaning deafness suggests “yes.” Cortex 48, 294–307.
Brauer, J., Anwander, A., and Friederici, A. D. (2011). Neuroanatomical prerequisites for language functions in the maturing brain. Cereb. Cortex 21, 459–466.
Buchel, C., Raedler, T., Sommer, M., Sach, M., Weiller, C., and Koch, M. A. (2004). White matter asymmetry in the human brain: a diffusion tensor MRI study. Cereb. Cortex 14, 945–951.
Buchsbaum, B. R., Olsen, R. K., Koch, P., and Berman, K. F. (2005). Human dorsal and ventral auditory streams subserve rehearsal-based and echoic processes during verbal working memory. Neuron 48, 687–697.
Bucy, P. C., and Kluver, H. (1955). An anatomical investigation of the temporal lobe in the monkey (Macaca mulatta). J. Comp. Neurol. 103, 151–251.
Butti, C., and Hof, P. R. (2010). The insular cortex: a comparative perspective. Brain Struct. Funct. 214, 477–493.
Buxhoeveden, D. P., and Casanova, M. F. (2002). The minicolumn and evolution of the brain. Brain Behav. Evol. 60, 125–151.
Buxhoeveden, D. P., Switala, A. E., Litaker, M., Roy, E., and Casanova, M. F. (2001a). Lateralization of minicolumns in human planum temporale is absent in nonhuman primate cortex. Brain Behav. Evol. 57, 349–358.
Buxhoeveden, D. P., Switala, A. E., Roy, E., Litaker, M., and Casanova, M. F. (2001b). Morphological differences between minicolumns in human and nonhuman primate cortex. Am. J. Phys. Anthropol. 115, 361–371.
Casanova, M. F., Trippe, J., Tilquist, C. R., and Switala, A. E. (2010). Dolphin insula reflects minicolumnar organization of mammalian isocortex. Transl. Neurosci. 1, 37–42.
Casanova, M. F., Van Kooten, I. A., Switala, A. E., Van Engeland, H., Heinsen, H., Steinbusch, H. W., Hof, P. R., Trippe, J., Stone, J., and Schmitz, C. (2006). Minicolumnar abnormalities in autism. Acta Neuropathol. 112, 287–303.
Caspers, S., Eickhoff, S. B., Rick, T., Von Kapri, A., Kuhlen, T., Huang, R., Shah, N. J., and Zilles, K. (2011). Probabilistic fibre tract analysis of cytoarchitectonically defined human inferior parietal lobule areas reveals similarities to macaques. Neuroimage 58, 362–380.
Catani, M., Allin, M. P., Husain, M., Pugliese, L., Mesulam, M. M., Murray, R. M., and Jones, D. K. (2007). Symmetries in human brain language pathways correlate with verbal recall. Proc. Natl. Acad. Sci. U.S.A. 104, 17163–17168.
Catani, M., Howard, R. J., Pajevic, S., and Jones, D. K. (2002). Virtual in vivo interactive dissection of white matter fasciculi in the human brain. Neuroimage 17, 77–94.
Catani, M., Jones, D. K., and Ffytche, D. H. (2005). Perisylvian language networks of the human brain. Ann. Neurol. 57, 8–16.
Clarke, S., Bellmann Thiran, A., Maeder, P., Adriani, M., Vernet, O., Regli, L., Cuisenaire, O., and Thiran, J. P. (2002). What and where in human audition: selective deficits following focal hemispheric lesions. Exp. Brain Res. 147, 8–15.
Clarke, S., Bellmann, A., Meuli, R. A., Assal, G., and Steck, A. J. (2000). Auditory agnosia and auditory spatial deficits following left hemispheric lesions: evidence for distinct processing pathways. Neuropsychologia 38, 797–807.
Corbetta, M., and Shulman, G. L. (2002). Control of goal-directed and stimulus-driven attention in the brain. Nat. Rev. Neurosci. 3, 201–215.
Corbetta, M., Kincade, M. J., Lewis, C., Snyder, A. Z., and Sapir, A. (2005). Neural basis and recovery of spatial attention deficits in spatial neglect. Nat. Neurosci. 8, 1603–1610.
Creem, S. H., and Proffitt, D. R. (2001). Grasping objects by their handles: a necessary interaction between cognition and action. J. Exp. Psychol. Hum. Percept. Perform. 27, 218–228.
Crick, F. C., and Koch, C. (2005). What is the function of the claustrum? Philos. Trans. R. Soc. Lond. B Biol. Sci. 360, 1271–1279.
Damasio, A. (1989). The brain binds entities and events by multiregional activation from convergence zones. Neural Comput. 1, 123–132.
Decety, J., Perani, D., Jeannerod, M., Bettinardi, V., Tadary, B., Woods, R., Mazziotta, J. C., and Fazio, F. (1994). Mapping motor representations with positron emission tomography. Nature 371, 600–602.
Dell, G. S., Schwartz, M. F., Martin, N., Saffran, E. M., and Gagnon, D. A. (1997). Lexical access in aphasic and nonaphasic speakers. Psychol. Rev. 104, 801–838.
Demonet, J. F., Price, C., Wise, R., and Frackowiak, R. S. (1994). A PET study of cognitive strategies in normal subjects during language tasks. Influence of phonetic ambiguity and sequence processing on phoneme monitoring. Brain 117(Pt 4), 671–682.
Demonet, J. F., Thierry, G., and Cardebat, D. (2005). Renewal of the neurophysiology of language: functional neuroimaging. Physiol. Rev. 85, 49–95.
Desimone, R., and Ungerleider, L. G. (1986). Multiple visual areas in the caudal superior temporal sulcus of the macaque. J. Comp. Neurol. 248, 164–189.
Deutscher, G. (2005). The Unfolding of Language: The Evolution of Mankind's Greatest Invention. London, UK: Random House.
Diaz, R. M., and Berk, L. E. (1992). Private Speech: From Social Interaction to Self-Regulation. Mahwah, NJ: Erlbaum.
Dittmar, M., Abbot-Smith, K., Lieven, E., and Tomasello, M. (2008). German children's comprehension of word order and case marking in causative sentences. Child Dev. 79, 1152–1167.
Dronkers, N. F., Wilkins, D. P., Van Valin, R. D. J. R., Redfern, B. B., and Jaeger, J. J. (2004). Lesion analysis of the brain areas involved in language comprehension. Cognition 92, 145–177.
Dubois, J., Dehaene-Lambertz, G., Perrin, M., Mangin, J. F., Cointepas, Y., Duchesnay, E., Le Bihan, D., and Hertz-Pannier, L. (2008). Asynchrony of the early maturation of white matter bundles in healthy infants: quantitative landmarks revealed noninvasively by diffusion tensor imaging. Hum. Brain Mapp. 29, 14–27.
Duffau, H. (2012). The “frontal syndrome” revisited: lessons from electrostimulation mapping studies. Cortex 48, 120–131.
Duffau, H., Capelle, L., Denvil, D., Gatignol, P., Sichez, N., Lopes, M., Sichez, J. P., and Van Effenterre, R. (2003a). The role of dominant premotor cortex in language: a study using intraoperative functional mapping in awake patients. Neuroimage 20, 1903–1914.
Duffau, H., Gatignol, P., Denvil, D., Lopes, M., and Capelle, L. (2003b). The articulatory loop: study of the subcortical connectivity by electrostimulation. Neuroreport 14, 2005–2008.
Duffau, H., Capelle, L., Sichez, N., Denvil, D., Lopes, M., Sichez, J. P., Bitar, A., and Fohanno, D. (2002). Intraoperative mapping of the subcortical language pathways using direct stimulations. An anatomo-functional study. Brain 125, 199–214.
Duffau, H., Gatignol, P., Mandonnet, E., Peruzzi, P., Tzourio-Mazoyer, N., and Capelle, L. (2005). New insights into the anatomo-functional connectivity of the semantic system: a study using cortico-subcortical electrostimulations. Brain 128, 797–810.
Duffau, H., Gatignol, P., Moritz-Gasser, S., and Mandonnet, E. (2009). Is the left uncinate fasciculus essential for language? A cerebral stimulation study. J. Neurol. 256, 382–389.
Edelstein, L. R., and Denaro, F. J. (2004). The claustrum: a historical review of its anatomy, physiology, cytochemistry and functional significance. Cell. Mol. Biol. (Noisy-le-grand) 50, 675–702.
Ehrich, J. F. (2006). Vygotskian inner speech and the reading process. Aust. J. Educ. Dev. Psychol. 6, 12–25.
Fiebach, C. J., and Schubotz, R. I. (2006). Dynamic anticipatory processing of hierarchical sequential events: a common role for Broca's area and ventral premotor cortex across domains? Cortex 42, 499–502.
Frazier, L. (1987). “Sentence processing: a tutorial review,” in Attention and Performance, XII. ed M. Coltheart (Hillsdale, NJ: Erlbaum).
Freud, S. (1891). Zur Auffassung der Aphasien. Eine kritische Studie, 2nd Edn. Leipzig, Wien: Franz Deuticke.
Frey, S., Campbell, J. S., Pike, G. B., and Petrides, M. (2008). Dissociating the human language pathways with high angular resolution diffusion fiber tractography. J. Neurosci. 28, 11435–11444.
Friederici, A. D. (2002). Towards a neural basis of auditory sentence processing. Trends Cogn. Sci. 6, 78–84.
Friederici, A. D. (2009). Pathways to language: fiber tracts in the human brain. Trends Cogn. Sci. 13, 175–181.
Friederici, A. D. (2011). The brain basis of language processing: from structure to function. Physiol. Rev. 91, 1357–1392.
Friederici, A. D., Bahlmann, J., Heim, S., Schubotz, R. I., and Anwander, A. (2006). The brain differentiates human and non-human grammars: functional localization and structural connectivity. Proc. Natl. Acad. Sci. U.S.A. 103, 2458–2463.
Friederici, A. D., Brauer, J., and Lohmann, G. (2011). Maturation of the language network: from inter—to intrahemispheric connectivities. PLoS ONE 6:e20726. doi: 10.1371/journal.pone.0020726
Friederici, A. D., Von Cramon, D. Y., and Kotz, S. A. (1999). Language related brain potentials in patients with cortical and subcortical left hemisphere lesions. Brain 122(Pt 6), 1033–1047.
Galuske, R. A., Schlote, W., Bratzke, H., and Singer, W. (2000). Interhemispheric asymmetries of the modular structure in human temporal cortex. Science 289, 1946–1949.
Gaskell, M. G., and Marslen-Wilson, W. D. (1997). Integrating form and meaning: a distributed model of speech perception. Lang. Cogn. Proc. 12, 613–656.
Gentner, T. Q., Fenn, K. M., Margoliash, D., and Nusbaum, H. C. (2006). Recursive syntactic pattern learning by songbirds. Nature 440, 1204–1207.
Gerardin, E., Sirigu, A., Lehericy, S., Poline, J. B., Gaymard, B., Marsault, C., Agid, Y., and Le Bihan, D. (2000). Partially overlapping neural networks for real and imagined hand movements. Cereb. Cortex 10, 1093–1104.
Geva, S., Jones, P. S., Crinion, J. T., Price, C. J., Baron, J. C., and Warburton, E. A. (2011). The neural correlates of inner speech defined by voxel-based lesion-symptom mapping. Brain 134, 3071–3082.
Goodale, M. A., and Milner, A. D. (1992). Separate visual pathways for perception and action. Trends Neurosci. 15, 20–25.
Goodale, M. A., Milner, A. D., Jakobson, L. S., and Carey, D. P. (1991). A neurological dissociation between perceiving objects and grasping them. Nature 349, 154–156.
Gross, C. G. (1992). Representation of visual stimuli in inferior temporal cortex. Philos. Trans. R. Soc. Lond. B Biol. Sci. 335, 3–10.
Grush, R. (2004). The emulation theory of representation: motor control, imagery, and perception. Behav. Brain Sci. 27, 377–96; discussion 396–442.
Hagmann, P., Cammoun, L., Martuzzi, R., Maeder, P., Clarke, S., Thiran, J. P., and Meuli, R. (2006). Hand preference and sex shape the architecture of language networks. Hum. Brain Mapp. 27, 828–835.
Hahne, A., Eckstein, K., and Friederici, A. D. (2004). Brain signatures of syntactic and semantic processes during children's language development. J. Cogn. Neurosci. 16, 1302–1318.
Hanakawa, T., Dimyan, M. A., and Hallett, M. (2008). Motor planning, imagery, and execution in the distributed motor network: a time-course study with functional MRI. Cereb. Cortex 18, 2775–2788.
Hanakawa, T., Immisch, I., Toma, K., Dimyan, M. A., Van Gelderen, P., and Hallett, M. (2003). Functional properties of brain areas associated with motor execution and imagery. J. Neurophysiol. 89, 989–1002.
Hauser, M. D., Newport, E. L., and Aslin, R. N. (2001). Segmentation of the speech stream in a non-human primate: statistical learning in cotton-top tamarins. Cognition 78, B53–B64.
Hickok, G., and Poeppel, D. (2000). Towards a functional neuroanatomy of speech perception. Trends Cogn. Sci. 4, 131–138.
Hickok, G., and Poeppel, D. (2004). Dorsal and ventral streams: a framework for understanding aspects of the functional anatomy of language. Cognition 92, 67–99.
Hickok, G., and Poeppel, D. (2007). The cortical organization of speech processing. Nat. Rev. Neurosci. 8, 393–402.
Hoeft, F., Barnea-Goraly, N., Haas, B. W., Golarai, G., Ng, D., Mills, D., Korenberg, J., Bellugi, U., Galaburda, A., and Reiss, A. L. (2007). More is not always better: increased fractional anisotropy of superior longitudinal fasciculus associated with poor visuospatial abilities in Williams syndrome. J. Neurosci. 27, 11960–11965.
Horton, J. C., and Hedley-Whyte, E. T. (1984). Mapping of cytochrome oxidase patches and ocular dominance columns in human visual cortex. Philos. Trans. R. Soc. Lond. B Biol. Sci. 304, 255–272.
Jackendoff, R. (2009). Parallels and nonparallels between language and music. Music Percept. 26, 195–204.
Jeannerod, M. (1994). The representing brain: neural correlates of motor intention and imagery. Behav. Brain Sci. 17, 187–245.
Jefferies, E., Jones, R. W., Bateman, D., and Ralph, M. A. (2005). A semantic contribution to nonword recall? Evidence for intact phonological processes in semantic dementia. Cogn. Neuropsychol. 22, 183–212.
Jobard, G., Crivello, F., and Tzourio-Mazoyer, N. (2003). Evaluation of the dual route theory of reading: a metanalysis of 35 neuroimaging studies. Neuroimage 20, 693–712.
Kastner, S., and Ungerleider, L. G. (2000). Mechanisms of visual attention in the human cortex. Annu. Rev. Neurosci. 23, 315–341.
Kertesz, A., and Mccabe, P. (1975). Intelligence and aphasia: performance of aphasics on Raven's coloured progressive matrices (RCPM). Brain Lang. 2, 387–395.
Kravitz, D. J., Saleem, K. S., Baker, C. I., and Mishkin, M. (2011). A new neural framework for visuospatial processing. Nat. Rev. Neurosci. 12, 217–230.
Kreher, B. W., Schnell, S., Mader, I., ll'yasov, K. A., Hennig, J., Kiselev, V. G., and Saur, D. (2008). Connecting and merging fibres: pathway extraction by combining probability maps. Neuroimage 43, 81–89.
Krubitzer, L. (1995). The organization of neocortex in mammals: are species differences really so different? Trends Neurosci. 18, 408–417.
Kümmerer, D., Kellmeyer, P., Glauche, V., Mader, I., Kloppel, S., Suchan, J., Karnath, H. O., Weiller, C., and Saur, D. (2010). “Damage of white matter fiber tracts in acute aphasic patients-a voxelwise lesionbehavior mapping study,” in 11th international Science of Aphasia Conference, (Potsdam, Germany). Available at: http://www.soa-online.com/programandlowbar;assets/final%20program%20abstracts.pdf
Lambon Ralph, M. A., Moriarty, L., and Sage, K. (2002). Anomia is simply a reflection of semantic and phonological impairments: evidence from a case-series study. Aphasiology 16, 56–82.
Leaver, A. M., and Rauschecker, J. P. (2010). Cortical representation of natural complex sounds: effects of acoustic features and auditory object category. J. Neurosci. 30, 7604–7612.
Leavitt, V. M., Molholm, S., Gomez-Ramirez, M., and Foxe, J. J. (2011). “What” and “where” in auditory sensory processing: a high-density electrical mapping study of distinct neural processes underlying sound object recognition and sound localization. Front. Integr. Neurosci. 5:23. doi: 10.3389/fnint.2011.00023
Lebel, C., Walker, L., Leemans, A., Phillips, L., and Beaulieu, C. (2008). Microstructural maturation of the human brain from childhood to adulthood. Neuroimage 40, 1044–1055.
Levelt, W. J., Roelofs, A., and Meyer, A. S. (1999). A theory of lexical access in speech production. Behav. Brain Sci. 22, 1–38; discussion 38–75.
Lewis, J. W., and Van Essen, D. C. (2000). Corticocortical connections of visual, sensorimotor, and multimodal processing areas in the parietal lobe of the macaque monkey. J. Comp. Neurol. 428, 112–137.
Livingstone, M. S., and Hubel, D. H. (1984). Anatomy and physiology of a color system in the primate visual cortex. J. Neurosci. 4, 309–356.
MacDonald, M. C., Pearlmutter, N. J., and Seidenberg, M. S. (1994). The lexical nature of syntactic ambiguity resolution [corrected]. Psychol. Rev. 101, 676–703.
Mahon, B. Z., Milleville, S. C., Negri, G. A., Rumiati, R. I., Caramazza, A., and Martin, A. (2007). Action-related properties shape object representations in the ventral stream. Neuron 55, 507–520.
Makris, N., and Pandya, D. N. (2009). The extreme capsule in humans and rethinking of the language circuitry. Brain Struct. Funct. 213, 343–358.
Makris, N., Meyer, J. W., Bates, J. F., Yeterian, E. H., Kennedy, D. N., and Caviness, V. S. (1999). MRI-Based topographic parcellation of human cerebral white matter and nuclei II. Rationale and applications with systematics of cerebral connectivity. Neuroimage 9, 18–45.
Mandonnet, E., Nouet, A., Gatignol, P., Capelle, L., and Duffau, H. (2007). Does the left inferior longitudinal fasciculus play a role in language? A brain stimulation study. Brain 130, 623–629.
Manger, P., Sum, M., Szymanski, M., Ridgway, S. H., and Krubitzer, L. (1998). Modular subdivisions of dolphin insular cortex: does evolutionary history repeat itself? J. Cogn. Neurosci. 10, 153–166.
Mathur, B. N., Caprioli, R. M., and Deutch, A. Y. (2009). Proteomic analysis illuminates a novel structural definition of the claustrum and insula. Cereb. Cortex 19, 2372–2379.
Mesulam, M. M. (1990). Large-scale neurocognitive networks and distributed processing for attention, language, and memory. Ann. Neurol. 28, 597–613.
Meynert, T. (1866). “Ein Fall von Sprachstörung, anatomisch begründet,” in XII. Band der Zeitschrift der, eds C. Braun, A. Duschek, and L. Schläger (Wien: K.u.K. Gesellschaft der Ärzte in Wien), 152–189.
Milner, A. D., and Goodale, M. A. (1995). The Visual Brain in Action. Oxford, Oxford University Press.
Milner, A. D., Dijkerman, H. C., Mcintosh, R. D., Rossetti, Y., and Pisella, L. (2003). Delayed reaching and grasping in patients with optic ataxia. Prog. Brain Res. 142, 225–242.
Mishkin, M., Ungerleider, L., and Macko, K. A. (1983). Object vision and spatial vision: two visual pathways. Trends Neurosci. 6, 414–417.
Morton, J., and Patterson, K. E. (1980). “A new attempt at an interpretation, or, an attempt at a new interpretation,” in Deep Dyslexia, eds M. Coltheart, K. E. P., and J. C. Marshall (London, UK: Routledge and Kegan Paul)
Mountcastle, V. B. (1957). Modality and topographic properties of single neurons of cat's somatic sensory cortex. J. Neurophysiol. 20, 408–434.
Mountcastle, V. B. (1978). “An organizing principle for cerebral function: the unit module and the distributed system,” in The Mindful Brain: Cortical Organization and the Group-Selective Theory, eds G. M. Edelman and V. B. Mountcastle (Cambridge, MA: MIT Press)
Musso, M., Glauche, V., Horn, A., and Weiller, C. (2009). The signature of human syntactic architecture. Neuroimage 47, S118.
Musso, M., Moro, A., Glauche, V., Rijntjes, M., Reichenbach, J., Buchel, C., and Weiller, C. (2003). Broca's area and the language instinct. Nat. Neurosci. 6, 774–781.
Northcutt, R. G., and Kaas, J. H. (1995). The emergence and evolution of mammalian neocortex. Trends Neurosci. 18, 373–379.
Nucifora, P. G., Verma, R., Melhem, E. R., and Gur, R. E. G. U. R. R. C. (2005). Leftward asymmetry in relative fiber density of the arcuate fasciculus. Neuroreport 16, 791–794.
Oechslin, M. S., Imfeld, A., Loenneker, T., Meyer, M., and Jancke, L. (2009). The plasticity of the superior longitudinal fasciculus as a function of musical expertise: a diffusion tensor imaging study. Front. Hum. Neurosci. 3:76. doi: 10.3389/neuro.09.076.2009
Oppenheim, G. M., and Dell, G. S. (2008). Inner speech slips exhibit lexical bias, but not the phonemic similarity effect. Cognition 106, 528–537.
Ostad, S. A., and Sorensen, P. M. (2007). Private speech and strategy-use patterns: bidirectional comparisons of children with and without mathematical difficulties in a developmental perspective. J. Learn. Disabil. 40, 2–14.
Parker, G. J., Luzzi, S., Alexander, D. C., Wheeler-Kingshott, C. A., Ciccarelli, O., and Lambon Ralph, M. A. (2005). Lateralization of ventral and dorsal auditory-language pathways in the human brain. Neuroimage 24, 656–666.
Paulesu, E., Frith, C. D., and Frackowiak, R. S. (1993). The neural correlates of the verbal component of working memory. Nature 362, 342–345.
Paulesu, E., Perani, D., Blasi, V., Silani, G., Borghese, N. A., De Giovanni, U., Sensolo, S., and Fazio, F. (2003). A functional-anatomical model for lipreading. J. Neurophysiol. 90, 2005–2013.
Paus, T., Zijdenbos, A., Worsley, K., Collins, D. L., Blumenthal, J., Giedd, J. N., Rapoport, J. L., and Evans, A. C. (1999). Structural maturation of neural pathways in children and adolescents: in vivo study. Science 283, 1908–1911.
Penney, C. G. (1989). Modality effects and the structure of short-term verbal memory. Mem. Cognit. 17, 398–422.
Pepperberg, I. M., and Shive, H. R. (2001). Simultaneous development of vocal and physical object combinations by a Grey parrot (Psittacus erithacus): bottle caps, lids, and labels. J. Comp. Psychol. 115, 376–384.
Perani, D., Saccuman, M. C., Scifo, P., Awander, A., Spada, D., Baldoli, C., Poloniato, A., Lohmann, G., and Friederici, A. D. (2011). Neural language networks at birth. Proc. Natl. Acad. Sci. U.S.A. 108, 16056–16061.
Perenin, M. T., and Vighetto, A. (1988). Optic ataxia: a specific disruption in visuomotor mechanisms. I. Different aspects of the deficit in reaching for objects. Brain 111(Pt 3), 643–674.
Peters, S. U., Kaufmann, W. E., Bacino, C. A., Anderson, A. W., Adapa, P., Chu, Z., Yallampalli, R., Traipe, E., Hunter, J. V., and Wilde, E. A. (2011). Alterations in white matter pathways in Angelman syndrome. Dev. Med. Child Neurol. 53, 361–367.
Petrides, M., and Pandya, D. N. (1988). Association fiber pathways to the frontal cortex from the superior temporal region in the rhesus monkey. J. Comp. Neurol. 273, 52–66.
Petrides, M., and Pandya, D. N. (2007). Efferent association pathways from the rostral prefrontal cortex in the macaque monkey. J. Neurosci. 27, 11573–11586.
Petrides, M., and Pandya, D. N. (2009). Distinct parietal and temporal pathways to the homologues of Broca's area in the monkey. PLoS Biol. 7:e1000170. doi: 10.1371/journal.pbio.1000170
Powell, H. W., Parker, G. J., Alexander, D. C., Symms, M. R., Boulby, P. A., Wheeler-Kingshott, C. A., Barker, G. J., Noppeney, U., Koepp, M. J., and Duncan, J. S. (2006). Hemispheric asymmetries in language-related pathways: a combined functional MRI and tractography study. Neuroimage 32, 388–399.
Price, C. J. (1998). The functional anatomy of word comprehension and production. Trends Cogn. Sci. 2, 281–288.
Pugh, K. R., Mencl, W. E., Jenner, A. R., Katz, L., Frost, S. J., Lee, J. R., Shaywitz, S. E., and Shaywitz, B. A. (2000). Functional neuroimaging studies of reading and reading disability (developmental dyslexia). Ment. Retard. Dev. Disabil. Res. Rev. 6, 207–213.
Purves, D., Riddle, D. R., and Lamantia, A. S. (1992). Iterated patterns of brain circuitry (or how the cortex gets its spots). Trends Neurosci. 15, 362–368.
Rakic, P. (1995). A small step for the cell, a giant leap for mankind: a hypothesis of neocortical expansion during evolution. Trends Neurosci. 18, 383–388.
Rauschecker, J. P., and Scott, S. K. (2009). Maps and streams in the auditory cortex: nonhuman primates illuminate human speech processing. Nat. Neurosci. 12, 718–724.
Rauschecker, J. P., and Tian, B. (2000). Mechanisms and streams for processing of “what” and “where” in auditory cortex. Proc. Natl. Acad. Sci. U.S.A. 97, 11800–11806.
Rauschecker, J. P. (1995). Compensatory plasticity and sensory substitution in the cerebral cortex. Trends Neurosci. 18, 36–43.
Rauschecker, J. P. (2011). An expanded role for the dorsal auditory pathway in sensorimotor control and integration. Hear. Res. 271, 16–25.
Rijntjes, M., Dettmers, C., Buchel, C., Kiebel, S., Frackowiak, R. S., and Weiller, C. (1999). A blueprint for movement: functional and anatomical representations in the human motor system. J. Neurosci. 19, 8043–8048.
Rilling, J. K., Glasser, M. F., Preuss, T. M., Ma, X., Zhao, T., Hu, X., and Behrens, T. E. (2008). The evolution of the arcuate fasciculus revealed with comparative DTI. Nat. Neurosci. 11, 426–428.
Ringo, J. L. (1991). Neuronal interconnection as a function of brain size. Brain Behav. Evol. 38, 1–6.
Rizzolatti, G., and Matelli, M. (2003). Two different streams form the dorsal visual system: anatomy and functions. Exp. Brain Res. 153, 146–157.
Rolheiser, T., Stamatakis, E. A., and Tyler, L. K. (2011). Dynamic processing in the human language system: synergy between the arcuate fascicle and extreme capsule. J. Neurosci. 31, 16949–16957.
Romanski, L. M., and Goldman-Rakic, P. S. (2002). An auditory domain in primate prefrontal cortex. Nat. Neurosci. 5, 15–16.
Romanski, L. M., Tian, B., Fritz, J., Mishkin, M., Goldman-Rakic, P. S., and Rauschecker, J. P. (1999a). Dual streams of auditory afferents target multiple domains in the primate prefrontal cortex. Nat. Neurosci. 2, 1131–1136.
Romanski, L. M., Bates, J. F., and Goldman-Rakic, P. S. (1999b). Auditory belt and parabelt projections to the prefrontal cortex in the rhesus monkey. J. Comp. Neurol. 403, 141–157.
Rosazza, C., Cai, Q., Minati, L., Paulignan, Y., and Nazir, T. A. (2009). Early involvement of dorsal and ventral pathways in visual word recognition: an ERP study. Brain Res. 1272, 32–44.
Ross, E. D. (2010). Cerebral localization of functions and the neurology of language: fact versus fiction or is it something else? Neuroscientist 16, 222–243.
Saur, D., Kreher, B. W., Schnell, S., Kummerer, D., Kellmeyer, P., Vry, M. S., Umarova, R., Musso, M., Glauche, V., Abel, S., Huber, W., Rijntjes, M., Hennig, J., and Weiller, C. (2008). Ventral and dorsal pathways for language. Proc. Natl. Acad. Sci. U.S.A. 105, 18035–18040.
Saur, D., Schelter, B., Schnell, S., Kratochvil, D., Kupper, H., Kellmeyer, P., Kummerer, D., Kloppel, S., Glauche, V., Lange, R., Mader, W., Feess, D., Timmer, J., and Weiller, C. (2010). Combining functional and anatomical connectivity reveals brain networks for auditory language comprehension. Neuroimage 49, 3187–3197.
Schenker, N. M., Buxhoeveden, D. P., Blackmon, W. L., Amunts, K., Zilles, K., and Semendeferi, K. (2008). A comparative quantitative analysis of cytoarchitecture and minicolumnar organization in Broca's area in humans and great apes. J. Comp. Neurol. 510, 117–128.
Schenker, N. M., Desgouttes, A. M., and Semendeferi, K. (2005). Neural connectivity and cortical substrates of cognition in hominoids. J. Hum. Evol. 49, 547–569.
Schlaug, G., Marchina, S., and Norton, A. (2009). Evidence for plasticity in white-matter tracts of patients with chronic Broca's aphasia undergoing intense intonation-based speech therapy. Ann. N.Y. Acad. Sci. 1169, 385–394.
Schmahmann, J. D., and Pandya, D. (2006). Fiber Pathways of the Brain. Oxford: Oxford University Press.
Schulze, K., Zysset, S., Mueller, K., Friederici, A. D., and Koelsch, S. (2011). Neuroarchitecture of verbal and tonal working memory in nonmusicians and musicians. Hum. Brain Mapp. 32, 771–783.
Scott, S. K., and Johnsrude, I. S. (2003). The neuroanatomical and functional organization of speech perception. Trends Neurosci. 26, 100–107.
Scott, S. K., and Wise, R. J. (2004). The functional neuroanatomy of prelexical processing in speech perception. Cognition 92, 13–45.
Scott, S. K., Blank, C. C., Rosen, S., and Wise, R. J. (2000). Identification of a pathway for intelligible speech in the left temporal lobe. Brain 123(Pt 12), 2400–2406.
Seltzer, B., and Pandya, D. N. (1984). Further observations on parieto-temporal connections in the rhesus monkey. Exp. Brain Res. 55, 301–312.
Shadmehr, R., and Krakauer, J. W. (2008). A computational neuroanatomy for motor control. Exp. Brain Res. 185, 359–381.
Sharp, D. J., Awad, M., Warren, J. E., Wise, R. J., Vigliocco, G., and Scott, S. K. (2010). The neural response to changing semantic and perceptual complexity during language processing. Hum. Brain Mapp. 31, 365–377.
Sherwood, C. C., Wahl, E., Erwin, J. M., Hof, P. R., and Hopkins, W. D. (2007). Histological asymmetries of primary motor cortex predict handedness in chimpanzees (Pan troglodytes). J. Comp. Neurol. 503, 525–537.
Stephan, K. M., Fink, G. R., Passingham, R. E., Silbersweig, D., Ceballos-Baumann, A. O., Frith, C. D., and Frackowiak, R. S. (1995). Functional anatomy of the mental representation of upper extremity movements in healthy subjects. J. Neurophysiol. 73, 373–386.
Szentagothai, J. (1983). The modular architectonic principle of neural centers. Rev. Physiol. Biochem. Pharmacol. 98, 11–61.
Szymanski, M. D., Bain, D. E., and Henry, K. R. (1995). “Auditory evoked potentials of a killer whale (Orcinus orca),” in Sensory systems of aquatic mammals, eds R. A. Kastelein, J. A. T., and P. E. Nachtigall (Woerden: De Spil), 1–10.
Tallal, P., and Gaab, N. (2006). Dynamic auditory processing, musical experience and language development. Trends Neurosci. 29, 382–390.
Tettamanti, M., and Weniger, D. (2006). Broca's area: a supramodal hierarchical processor? Cortex 42, 491–494.
Thiebaut de Schotten, M., Dell'Acqua, F., Forkel, S. J., Simmons, A., Vergani, F., Murphy, D. G., and Catani, M. (2011). A lateralized brain network for visuospatial attention. Nat. Neurosci. 14, 1245–1246.
Thiebaut de Schotten, M., Dell'Acqua, F., Valabregue, R., and Catani, M. (2012). Monkey to human comparative anatomy of the frontal lobe association tracts. Cortex 48, 82–96.
Thierry, G., Cardebat, D., and Demonet, J. F. (2003a). Electrophysiological comparison of grammatical processing and semantic processing of single spoken nouns. Brain Res. Cogn. Brain Res. 17, 535–547.
Thierry, G., Giraud, A. L., and Price, C. (2003b). Hemispheric dissociation in access to the human semantic system. Neuron 38, 499–506.
Tian, B., Reser, D., Durham, A., Kustov, A., and Rauschecker, J. P. (2001). Functional specialization in rhesus monkey auditory cortex. Science 292, 290–293.
Tiwari, V. N., Jeong, J. W., Wilson, B. J., Behen, M. E., Chugani, H. T., and Sundaram, S. K. (2012). Relationship between aberrant brain connectivity and clinical features in Angelman Syndrome: a new method using tract based spatial statistics of DTI color-coded orientation maps. Neuroimage 59, 349–355.
Tootell, R. B., Dale, A. M., Sereno, M. I., and Malach, R. (1996). New images from human visual cortex. Trends Neurosci. 19, 481–489.
Umarova, R. M., Saur, D., Kaller, C. P., Vry, M. S., Glauche, V., Mader, I., Hennig, J., and Weiller, C. (2011). Acute visual neglect and extinction: distinct functional state of the visuospatial attention system. Brain 134, 3310–3325.
Umarova, R. M., Saur, D., Schnell, S., Kaller, C. P., Vry, M. S., Glauche, V., Rijntjes, M., Hennig, J., Kiselev, V., and Weiller, C. (2010). Structural connectivity for visuospatial attention: significance of ventral pathways. Cereb. Cortex 20, 121–129.
Ungerleider, L. G., and Haxby, J. V. (1994). ‘What’ and ‘where’ in the human brain. Curr. Opin. Neurobiol. 4, 157–165.
Vallar, G., Di Betta, A. M., and Silveri, M. C. (1997). The phonological short-term store-rehearsal system: patterns of impairment and neural correlates. Neuropsychologia 35, 795–812.
Vates, G. E., and Nottebohm, F. (1995). Feedback circuitry within a song-learning pathway. Proc. Natl. Acad. Sci. U.S.A. 92, 5139–5143.
Vidorreta, J. G., Garcia, R., Moritz-Gasser, S., and Duffau, H. (2011). Double dissociation between syntactic gender and picture naming processing: a brain stimulation mapping study. Hum. Brain Mapp. 32, 331–340.
Vigneau, M., Beaucousin, V., Herve, P. Y., Duffau, H., Crivello, F., Houde, O., Mazoyer, B., and Tzourio-Mazoyer, N. (2006). Meta-analyzing left hemisphere language areas: phonology, semantics, and sentence processing. Neuroimage 30, 1414–1432.
Vry, M. S., Saur, D., Rijntjes, M., Umarova, R., Kellmeyer, P., Schnell, S., Glauche, V., Hamzei, F., and Weiller, C. (2012). Ventral and dorsal fiber systems for imagined and executed movement. Exp. Brain Res. 219, 203–216.
Wager, T. D., and Smith, E. E. (2003). Neuroimaging studies of working memory: a meta-analysis. Cogn. Affect. Behav. Neurosci. 3, 255–274.
Watkins, O. C., and Watkins, M. J. (1980). The modality effect and echoic persistence. J. Exp. Psychol. Gen. 109, 251–278.
Weiller, C., Bormann, T., Saur, D., Musso, M., and Rijntjes, M. (2011). How the ventral pathway got lost: and what its recovery might mean. Brain Lang. 118, 29–39.
Weiller, C., Isensee, C., Rijntjes, M., Huber, W., Muller, S., Bier, D., Dutschka, K., Woods, R. P., Noth, J., and Diener, H. C. (1995). Recovery from Wernicke's aphasia: a positron emission tomographic study. Ann. Neurol. 37, 723–732.
Weiller, C., Musso, M., Rijntjes, M., and Saur, D. (2009). Please don't underestimate the ventral pathway in language. Trends Cogn. Sci. 13, 369-70; 370–371.
Wernicke, C. (1874). Der aphasische Symptomenkomplex. Eine psychologische Studie auf Anatomischer Basis. Breslau: Cohn und Weigert.
Wernicke, C. (1906). “Der aphasische Symptomenkomplex,” in Deutsche Klinik am Eingang des zwanzigsten Jahrhunderts in akademischen Vorlesungen. ed E. V. Leyden (Berlin: Urban, Schwarzenberg), 487–556.
Wiley, N. (2006). Inner Speech as a Language: a Saussurean Inquiry. J. Theory Soc. Behav. 36, 319–341.
Williams, E. L., and Casanova, M. F. (2010). Autism and dyslexia: a spectrum of cognitive styles as defined by minicolumnar morphometry. Med. Hypotheses 74, 59–62.
Wilson, B. J., Sundaram, S. K., Huq, A. H., Jeong, J. W., Halverson, S. R., Behen, M. E., Bui, D. Q., and Chugani, H. T. (2011). Abnormal language pathway in children with Angelman syndrome. Pediatr. Neurol. 44, 350–356.
Wilson, F. A., Scalaidhe, S. P., and Goldman-Rakic, P. S. (1993). Dissociation of object and spatial processing domains in primate prefrontal cortex. Science 260, 1955–1958.
Winsler, A., and Naglieri, J. (2003). Overt and covert verbal problem-solving strategies: developmental trends in use, awareness, and relations with task performance in children aged 5 to 17. Child Dev. 74, 659–678.
Winsler, A., Abar, B., Feder, M. A., Schunn, C. D., and Rubio, D. A. (2007). Private Speech and executive functioning among high-functioning children with autistic spectrum disorders. J. Autism Dev. Disord. 37, 1617–1635.
Winsler, A., Carlton, M. P., and Barry, M. J. (2000). Age-related changes in preschool children's systematic use of private speech in a natural setting. J. Child Lang. 27, 665–687.
Winsler, A., De Leon, J. R., Wallace, B. A., Carlton, M. P., and Willson-Quayle, A. (2003). Private speech in preschool children: developmental stability and change, across-task consistency, and relations with classroom behaviour. J. Child Lang. 30, 583–608.
Wise, R. J. (2003). Language systems in normal and aphasic human subjects: functional imaging studies and inferences from animal studies. Br. Med. Bull. 65, 95–119.
Wolpert, D. M., and Miall, R. C. (1996). Forward models for physiological motor control. Neural Netw. 9, 1265–1279.
Wolpert, D. M., Ghahramani, Z., and Jordan, M. I. (1995). An internal model for sensorimotor integration. Science 269, 1880–1882.
Yeterian, E. H., Pandya, D. N., Tomaiuolo, F., and Petrides, M. (2012). The cortical connectivity of the prefrontal cortex in the monkey brain. Cortex 48, 58–81.
Zatorre, R. J., and Belin, P. (2001). Spectral and Temporal Processing in Human Auditory Cortex. Oxford, UK: Oxford University Press.
Keywords: language, DTI, dual loop model
Citation: Rijntjes M, Weiller C, Bormann T and Musso M (2012) The dual loop model: its relation to language and other modalities. Front. Evol. Neurosci. 4:9. doi: 10.3389/fnevo.2012.00009
Received: 16 December 2011; Accepted: 11 June 2012;
Published online: 03 July 2012.
Edited by:
Angela D. Friederici, Max Planck Institute for Human Cognitive and Brain Sciences, GermanyReviewed by:
Michel Thiebaut De Schotten, King's College London, UKAlfred Anwander, Max Planck Society, Germany
Copyright: © 2012 Rijntjes, Weiller, Bormann and Musso. This is an open-access article distributed under the terms of the Creative Commons Attribution Non Commercial License, which permits non-commercial use, distribution, and reproduction in other forums, provided the original authors and source are credited.
*Correspondence: Cornelius Weiller, Neurologische Universitätsklinik Freiburg, Breisacher Strasse 64, D-79106 Freiburg, Germany. e-mail: cornelius.weiller@uniklinik-freiburg.de