- 1Laboratorio de Biología de la Reproducción, Unidad de Morfología y Función (UMF), Facultad de Estudios Superiores (FES) Iztacala, Universidad Nacional Autónoma de México, Tlalnepantla, Edo. de México, Mexico
- 2Bioterio, Depto. de Biología Celular, Facultad de Ciencias, Universidad Nacional Autónoma de México, Ciudad de México, Mexico
- 3Colección Nacional de Mamíferos, Departamento de Zoología, Instituto de Biología, Universidad Nacional Autónoma de México, Ciudad de México, Mexico
The exhibition of paternal behaviour has been associated with high peripheral concentrations of oxytocin (OT) and levels of oxytocin receptors (OTRs) in the paraventricular and supraoptic nuclei of the hypothalamus. The presence of OTRs in the medial preoptic area (mPOA), bed nucleus of the stria terminalis (BNST), medial amygdala (MeA), and olfactory bulb (OB) has not been studied extensively in the paternal context, although these nuclei are essential in the regulation of paternal behaviour. The aim of this study was to determine whether there is an association between paternal response of sexually inexperienced males of the Mongolian gerbils (Meriones unguiculatus) and OT concentrations in plasma, as well as the presence of OTRs in the mPOA, BNST, MeA, and OB. We used sexually inexperienced gerbils: ten paternal (PAT) males and 10 males aggressive with pups (AGG). PAT males and AGG males were subjected to paternal behaviour tests. Then, blood samples were taken to quantify OT concentrations. The brains were dissected for OTR immunohistochemistry analyses. PAT males had significantly higher plasma levels of OT and numbers of cells with OTR immunoreactivity (ir) in the mPOA/BNST. These results indicated an association between paternal behaviour and peripheral concentrations of OT, as well as the presence of OTRs in the mPOA and BNST. This suggests that OT and OTRs could participate in the regulation of paternal behaviour in the Mongolian gerbil.
1 Introduction
In biparental rodents, the father participates significantly in the care of the pups. Depending on the species, the father displays crouching over the pups, grooming, pup retrieval and socialisation (Elwood, 1983; Clutton-Brock, 1991). Paternal care has a positive influence on the physical development and survival of the pups (Wuensch, 1985; Cantoni and Brown, 1997; Gubernick and Teferi, 2000). Furthermore, the father’s presence in the postpartum period impacts on brain development and social behaviours, such as paternal behaviour (Glasper et al., 2016; Cao et al., 2014; Fitzsimons and Villadsen, 2019). For example, father’s absence causes in male offspring a decrease in paternal care when they are fathers (Jia et al., 2011; Cao et al., 2014).
Neural bases of paternal behaviour have been little analysed (Lee and Brown, 2002, 2007; Romero-Morales et al., 2018). However, it has been proposed that subcortical neural circuits that regulate paternal behaviour are basically the same as those that regulate maternal behaviour (Numan, 2020). In the laboratory rat, sensory information from the pups, including olfactory stimulus is routed through medial amygdala (MeA) toward the medial preoptic area of the hypothalamus (mPOA) and bed nucleus of the stria terminalis (BNST). These nuclei facilitate parental behaviour, mainly mPOA that integrates all the inputs of the sensory stimuli from the pup, a process influenced by the hormonal milieu (Horrell et al., 2018).
Hormones, such as prolactin, testosterone, progesterone, and the neuropeptides vasopressin and oxytocin (OT) have been implicated in the regulation of paternal behaviour (Lonstein et al., 2015; Martínez et al., 2015; Bales and Saltzman, 2016; Horrell et al., 2018; Wang et al., 2018; Martínez et al., 2019; Numan, 2020). OT is synthesised in the magnocellular neurosecretory cells of the hypothalamic supraoptic nucleus (SON) and paraventricular nucleus (PVN). The magnocellular cells project to the posterior portion of the neurohypophysis, which releases OT into the bloodstream. OT is involved in the regulation of parturition, suckling, and other functions (Yoshihara et al., 2018). OT has also been associated with a variety of prosocial behaviours, such as establishment of a pair bond in monogamous species and social memory (Gimpl and Fahrenholz, 2001; Numan and Young, 2016; Scatliffe et al., 2019). OT and its receptor (OTR) participate in the regulation of sexual, aggressive, and parental behaviour (Gimpl and Fahrenholz, 2001; Dumais and Veenema, 2016; Yoshihara et al., 2018). In the mandarin vole (Microtus mandarinus), males exposed to the pups have significantly high OT-ir cell in the PVN than paired males (Song et al., 2010). In this rodent, fathers had a significantly higher serum concentration of oxytocin than virgin males (Yuan et al., 2019). In the California mouse, fathers and sexually inexperienced spontaneously paternal males had a similar level of OTR mRNA expression in the mPOA and MeA (Perea-Rodriguez et al., 2015). Levels of OT in plasma and the presence of OTRs in the mPOA and BNST have not been studied extensively in the paternal context although these nuclei have an essential function in the regulation of paternal behaviour (Lonstein et al., 2015; Horrell et al., 2018; Numan, 2020). The MeA and OB were also analysed because both nuclei are part of positive and negative mechanisms that regulate paternal behaviour. We put forward the hypothesis that the paternal response of sexually inexperienced gerbils is associated with high peripheral concentrations of OT and levels of OTRs in neural nuclei that are involved in the regulation of paternal behaviour. The aim of this study was to determine whether the paternal response of sexually inexperienced males of the Mongolian gerbil is associated with high OT concentrations in plasma, as well as OTR immunoreactivity (ir) in the mPOA, BNST, MeA, and OB. The Mongolian gerbil is a monogamous species, in this rodent the male displays paternal behaviour including crouching over pups, grooming, and sniffing of the pups and sporadically exhibits retrieval of the pups (Elwood, 1975).
2 Materials and methods
2.1 Animals
In this study, we used virgin male Mongolian gerbils between 180 and 210 days old. The animals were obtained from a colony established in our Laboratory. The gerbils used in this study were maintained under an inverted photoperiod of a 12 h:12 h light-dark cycle, with the onset of light at 18:00 h, at an ambient temperature between 17 and 21°C. The animals were fed with pellets (Lab Chow 5001, Nutrimentos Purina, México) and ad libitum water. Finely sliced carrot was also provided once per week. The gerbils were weaned at 25 to 28 days of age (Elwood, 1975). Two or three males were housed in a polycarbonate cage (37 × 27 × 15 cm) with sawdust bedding. They were kept in this condition from weaning until they were exposed to pups of the same species and screened to determine whether the males were paternal or aggressive with unfamiliar pups of the same species. The care that fathers provide to their pups is similar to that provided by sexually inexperienced males spontaneously paternal with the pups of their species, so much so that it has been proposed that these behaviours are regulated by the same neural circuit (Numan, 2020). The gerbils were considered paternal when they displayed crouching over pups and/or grooming and sniffing/contact of the pups (Elwood, 1975). Aggressive gerbils attack pups by biting and kill them if they are not removed quickly (Martínez et al., 2015).
2.2 Screening and paternal behaviour tests
Through the screening tests, 10 paternal (PAT) males and 10 males that were aggressive with the pups (AGG) were selected. The PAT males and AGG males were kept in groups of two or three individuals per cage until they were subjected to paternal behaviour tests (second exposure to pups) (Martínez et al., 2015). For the screening test, each male was placed in a cage with the same characteristics as the housing cage and clean sawdust bedding. After 10 min of habituation, two pups aged 1 to 3 days were introduced, and the male’s behaviour toward the pups was recorded. When the males exhibited paternal behaviour, the screening test lasted 5 minutes, but if they attacked the pups, the test was terminated immediately. Fifty-two sexually inexperienced gerbils were subjected to screen tests of paternal behaviour resulting in 36 (69%) aggressive males with the pups and 16 (31%) paternal males. Between 15 and 20 days after the screening tests, both PAT and AGG males were subjected to a paternal behaviour test (second exposure to pups) using the same method described for screening tests. When the males were paternal the tests lasted 15 minutes. We recorded the approach latency (the time that elapsed after the pups were introduced to the cage until the male contacted one of them). The time spent crouching over pups, grooming, and sniffing of the pups was also quantified. However, when they were aggressive with the pups, only the attack latency (time that elapsed after the pups were introduced to the cage until the male attacked one of them) was recorded. All males that displayed paternal or aggressive behaviour toward the pups in screen tests were persistently paternal or aggressive during paternal tests. The pups were used only once in behaviour tests. The behavioural tests were carried out between 10:00 and 13:00 h during the dark period under red light illumination. Sessions were videotaped with a high-definition infrared camera (IR Bullet camera, 2.1 megapixels). A single observer analysed the videos and was blind to the assigned group.
2.3 Hormone assay
Immediately after of completing the paternal behaviour test, five PAT and five AGG gerbils were chosen randomly and anesthetised with 5 mg/kg of xylazine and 60 mg/kg of ketamine through intramuscular injection (i.m.). Once the gerbils were anesthetised, blood samples (250 μL) were collected from the retro-orbital sinus using heparinised capillary tubes, each session took less than one minute. The other five PAT and five AGG gerbils were anesthetised with a heavy dose of 10 mg/kg xylazine and 90 mg/kg ketamine i.m., after they were perfused as described below. Blood samples were collected before perfusion using the same procedure described above. Blood samples were taken between 11:00 and 14:00 h. The plasma was separated by centrifugation and stored at −40°C. OT concentrations were quantified by a commercial ELISA kit (Abcam, UK, ab133050) with a sensitivity of 15 pg/ml, cross effect with AVP is < 0.02. This kit has already been used in several studies (Yuan et al., 2020; Maroun et al., 2020). The intra-assay and inter-assay coefficients of variation were 10% and 14%, respectively. The results showed a correlation between the serum dilutions and the standard curve. The recovery rate for OT was 100% (r = 1.0). The plate was read in a plate reader (model Multiskan Ascent V1.25 with a filter of 450-nm wavelength; Thermo Electron Corporation).
2.4 OTR immunohistochemistry analysis
Males were perfused through the heart with physiological saline, followed by 4% paraformaldehyde in sodium phosphate buffer (0.1 M, pH 7.6). The brain was removed and postfixed for 16 h in the same solution. The brain tissue was processed and cut into 7-μm-thick coronal sections with a microtome (Leica 820). The neural areas were located using the Stereotaxic Atlas of the Mongolian gerbil (Loskota et al., 1974). The locations of the neural areas were as follows: mPOA and BNST, −0.1 mm in image 520; MeA, −0.888 mm in image 590; and OB, +1.7 mm in image 300. After obtaining sections of the different neural areas, the samples were placed on gelatinised slides (Nutrient Gelatine, 70151-500G-F; Sigma−Aldrich, CA, USA). Each of the following steps was followed by rinsing in phosphate-buffered saline (PBS) for 5 min: (1) 10 min of incubation in 3% H2O2 in PBS, (2) 20 min of incubation in 5% normal goat serum (Vectastain ABC kit, PK-4000; Vector Laboratories) in PBS, and (3) 16 h of incubation at 25°C with a 1:500 dilution of OTR antibody (Bioss antibodies, bs-1314R) in PBS. After two 5-min rinses in PBS, the sections were incubated with biotinylated goat antirabbit antibody in PBS for 90 min and rinsed twice in PBS (Vectastain ABC kit, PK-6102; Vector Laboratories). The sections were then incubated by 30 min with an avidin-biotin complex (Vectastain ABC kit, PK-6100; Vector Laboratories), followed by two additional rinses with PBS. Finally, binding was visualised using 3,3′−diaminobenzidine as a chromogen (DAB Peroxidase Substrate, SK-4100; Vector Laboratories). The sections were dehydrated and then cover-slipped. For negative control sections, incubation with the primary antibody was omitted. All neural tissues were processed using the same assay.
2.5 Image analysis
The numbers of OTR-ir cells in the mPOA, BNST, MeA, and OB were quantified in microphotographs with an area of 180 μm2 (18 × 10 μm). Microphotographs were taken with a Motic camera (10 megapixels) attached to a Leica microscope. The quantification was performed bilaterally in the brains of five PAT males and five AGG males using four slides for each male. The number of OTR-ir cells in the mPOA, BNST, MeA, and OB was quantified by a single observer and was blind to the assigned group.
2.6 Western blotting of OTR antibody (Bioss antibodies, bs-1314R)
Gerbils with a similar age range to that already mentioned in this study were subjected to paternal behaviour tests, immediately after they were anesthetised and perfused intracardially with saline solution (0.9% NaCl) to wash out the nervous tissue. The brain tissue was removed and the hypothalamus was dissected and weighed. Hypothalamus tissues were lysed in ice-cold Pierce RIPA Buffer (Cat. 89900, Thermo Scientific, Rockford, Il, USA) containing a protease inhibitor cocktail (Cat. I3786, Sigma-Aldrich). According with Lowry et al., 1951, proteins were quantified, and the lysates were stored at −70°C until use. The protein samples were mixed with Laemmli buffer, subjected to 10% sodium dodecyl sulphate-polyacrylamide gel electrophoresis (SDS-PAGE), and transferred to polyvinylidene difluoride (PVDF) membranes (0.8 mA/cm2, 2 h) in 25 mmol/L Tris buffer, 192 mmol/L glycine and 20% methanol. After blocking nonspecific binding sites with Tris buffered-saline (TBS) supplemented with 5% BSA, the membranes were incubated overnight at 4°C with primary antibody for OTR (1:1000 dilution in PBS, bs-1314R, Bioss Antibodies). Antibody binding was detected using a secondary antibody labelled with horseradish peroxidase (HRP) in conjunction with a commercial chemiluminescence kit (Clarity Western ECL substrate, Bio-Rad, Hercules, CA, USA) following the supplier’s instructions. Blot image acquisition was performed using a C-Digit Blot Scanner (LI-COR Biotechnology, Lincoln, NE, USA). The protein expression of OTR in the hypothalamus of aggressive males with the pups and paternal males of Mongolian gerbils is shown in Figure 1. In this figure, it is observed that bands for OTR was detected at a molecular weight of about 47 kDa. The protein levels of OTR in the hypothalamus were more intense in paternal males compared to aggressive males toward pups.
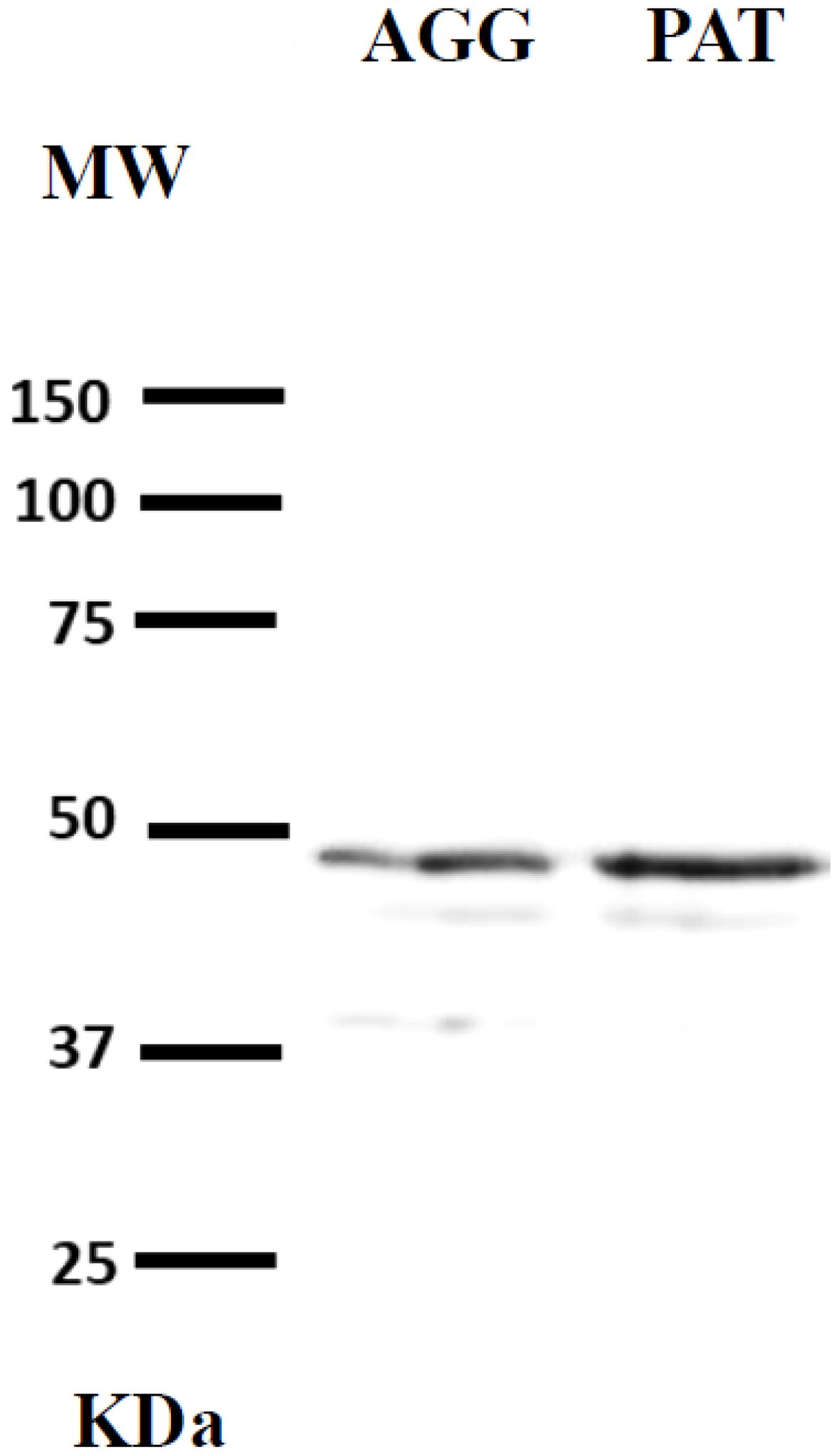
Figure 1. Protein expression of OTR in the hypothalamus of the aggressive males with the pups (AGG) and paternal males (PAT) of the Mongolian gerbil.
2.7 Statistical analysis
OT plasma levels were compared using a nonparametric Mann−Whitney U test since the data were not normally distributed (Anderson–Darling test, P > 0.05). Likewise, the numbers of OTR-ir cells in the mPOA, BNST, MeA, and OB were compared between PAT and AGG males using a nonparametric Mann−Whitney U test due to the nonnormality of the data (Anderson−Darling test, P > 0.05). Pearson correlations were used to examine the potential correlations between OT concentrations and the approach latency and the time spent crouching over, grooming, and sniffing the pups by PAT males. Likewise, the number of OTR-ir cells in the mPOA and BNST was correlated with the behavioural parameters already mentioned. The statistical analyses were performed using SPSS version 21.0 (IBM SPSS, Armonk, NY).
2.8 Ethical note
All experiments were performed in accordance with the ethical guidelines of the National Institutes of Health Guide for the Care and Use of Laboratory Animals (NIH Publication No. 8023) and the ethical guidelines and technical specifications of the Mexican Official Norm for the Production, Care and Use of Laboratory Animals (Sikes and Animal Care and Use Committee of the American Society of Mammalogists, 2016). The protocol of this investigation was approved by Comisión de Bioética de la Facultad de Estudios Superiores Iztacala de la Universidad Nacional Autónoma de México (Folio 704).
During the paternal behaviour tests four pups were slightly bitten, and their wounds were treated with gentian violet (1%), after which they were returned to their parents.
3 Results
3.1 Paternal behaviour, OT concentrations, and OTR immunoreactivity
All PAT males displayed paternal behaviour, which included crouching over, grooming, and sniffing of the pups. For AGG males, only the attack latency was recorded because the pups were removed as soon as they were attacked (Table 1). The concentrations of OT in plasma were significantly higher in PAT males than in AGG males (U = 105, P < 0.05, Figure 2). OT concentrations of PAT males are close to that reported for the male laboratory rat in which it is mentioned that this is 52.04 ± 5.63 pg/ml (Maroun et al., 2020).
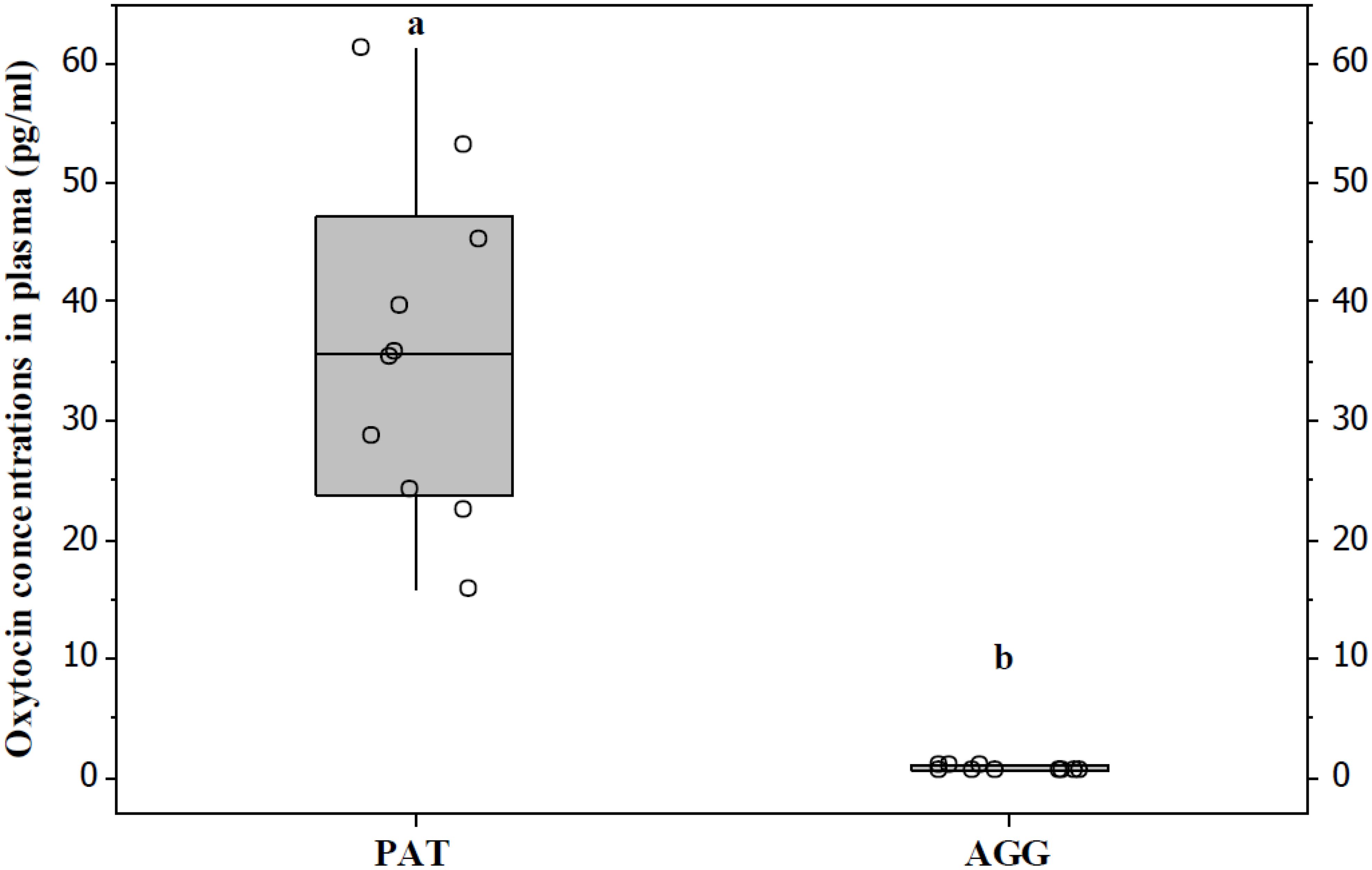
Figure 2. PAT males had significantly higher OXT levels than AGG males with the pups. Data are presented as medians and quartiles. Letters indicate significant differences.
The number of OTR-ir cells in the mPOA (U = 40, P < 0.05, Figures 3, 4) and BNST (U = 40, P < 0.05, Figures 3, 4) was significantly higher in PAT males than in AGG males. However, the number of OTR-ir cells in the MeA (U = 27.5, P > 0.05, Figures 3, 4) and OB (U = 27.5, P > 0.05, Figures 3, 4) was not significantly different between PAT males and AGG males.
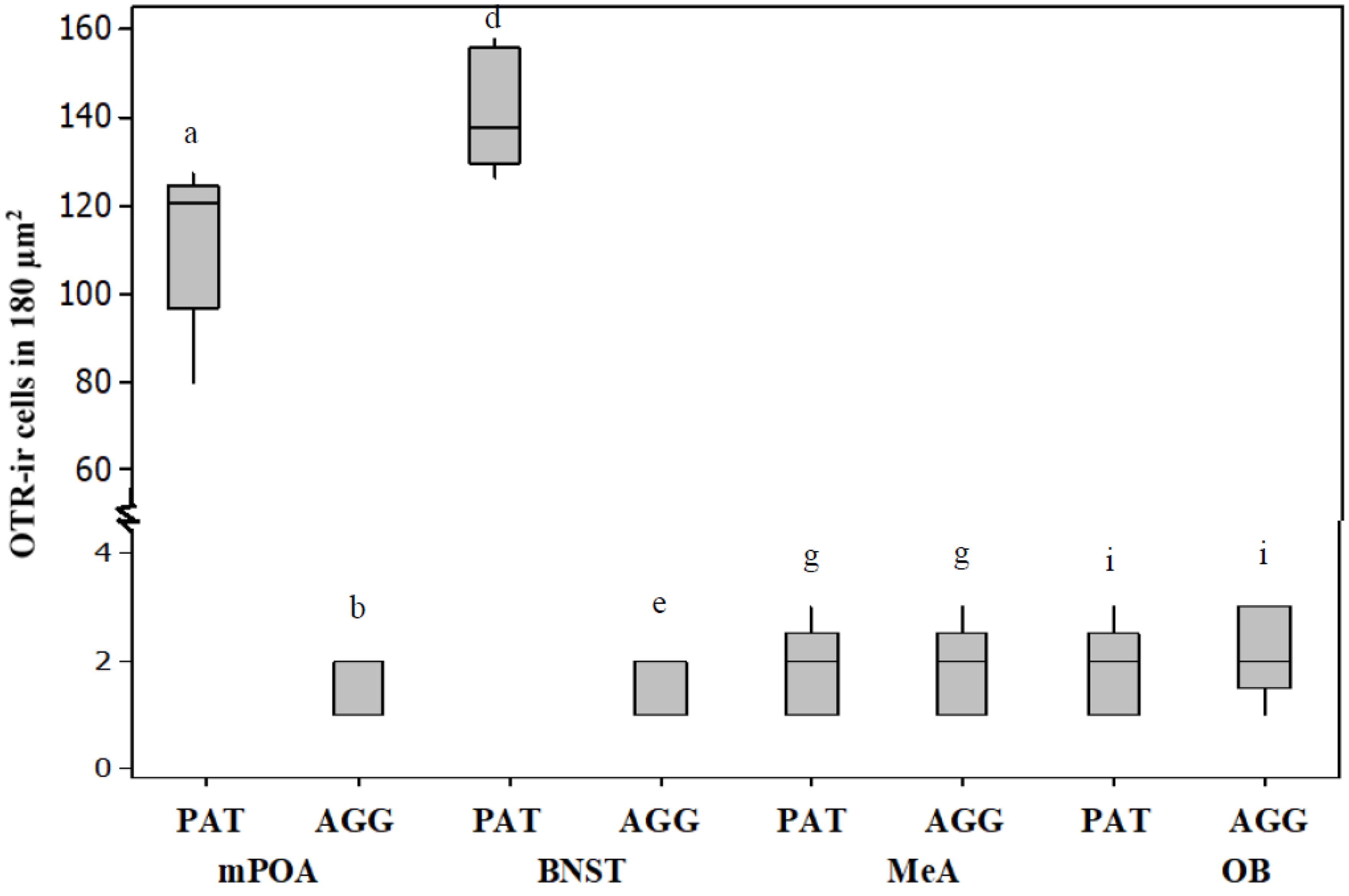
Figure 3. PAT males had significantly higher OXTR-ir in the mPOA and the BNST than AGG males with the pups. In MeA and OB there were no significant differences between PAT males and AGG males Data are presented as medians and quartiles (n = 5). Letters indicate significant differences.
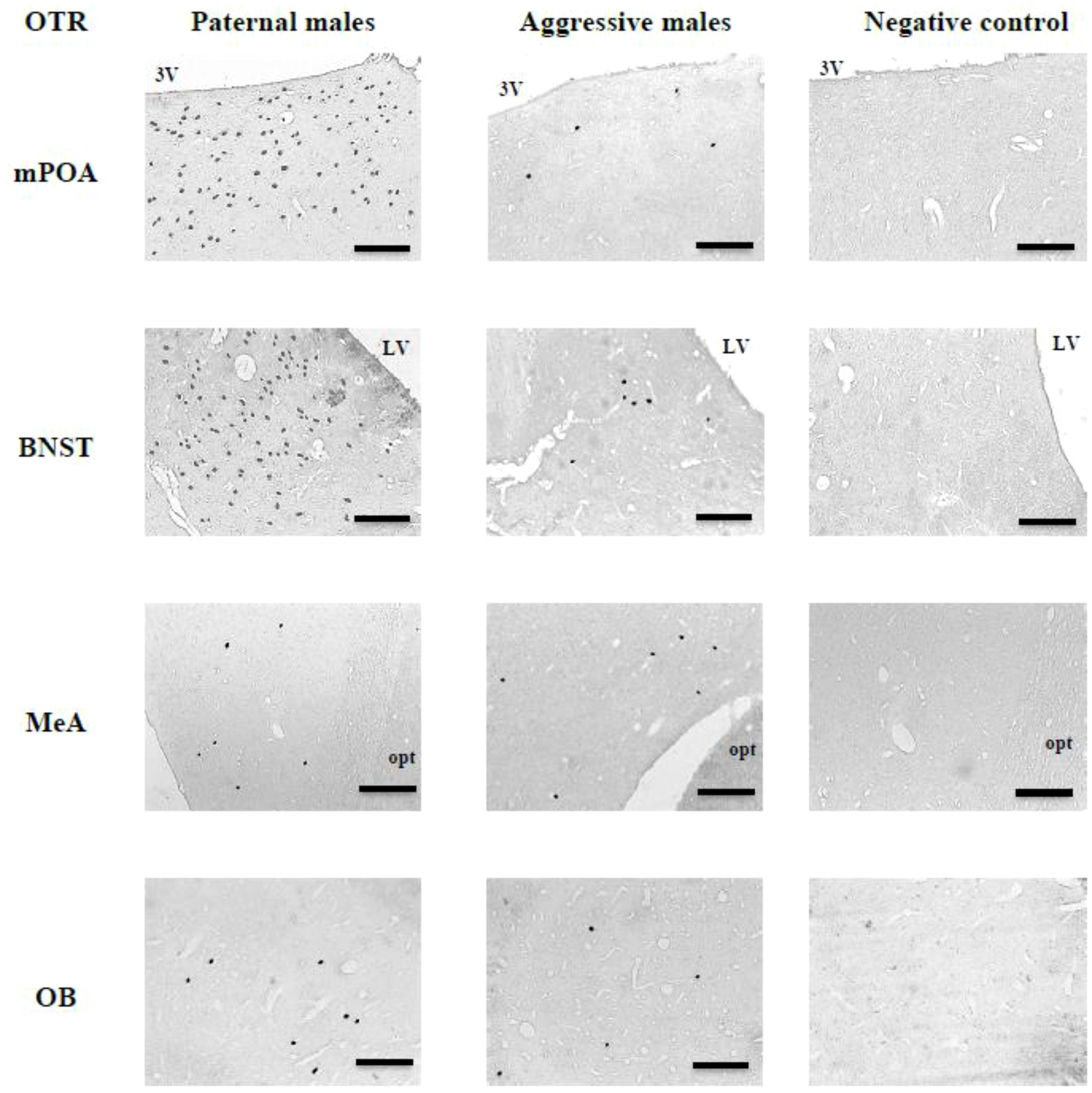
Figure 4. Representative photomicrographs showing OXTR-ir cells in the mPOA, BNST, MeA and OB of PAT males and AGG males with the pup, as well as negative control sections, in which the primary antibody was omitted. 3V, third ventricle; LVm lateral ventricle; opt, optic tract. Coronal sections, scale bars = 100 μm.
3.2 Correlations of OT concentrations and OTR-ir cells with paternal activities
OT concentrations were significantly and negatively correlated with the approach latency (r = -0.85, P < 0.01, n = 10, Figure 5), and positively correlated with the time spent crouching over pups (r = 0.97, P < 0.01, n = 10, Figure 5), grooming them (r = 0.95, P < 0.01, n = 10, Figure 5), and sniffing them (r = 0.93, P < 0.01, n = 10, Figure 5). The number of OTR-ir cells in the mPOA was significantly and negatively correlated with the approach latency (r = -0.83, P < 0.05, n = 5, Figure 6), and positively correlated with the time spent crouching over pups (r = 0.98, P < 0.01, n = 5, Figure 6), grooming them (r = 0.99, P < 0.01, n =5, Figure 6), and sniffing them (r = 0.97, P < 0.01, n = 5, Figure 6). The number of OTR-ir cells in the BNST was also negatively correlated with the approach latency (r = -0. 70, P < 0.05; n = 5, Figure 6), and positively correlated with the time that PAT gerbils spent crouching over pups (r = 0.93, P < 0.05, n = 5, Figure 6), grooming them (r = 0.90, P < 0.05, n = 5, Figure 6), and sniffing them (r = 0.98, P < 0.01, n = 5, Figure 6). Due to the low number of OTR-ir cells in MeA and OB, it was not possible to make correlations with paternal activities.
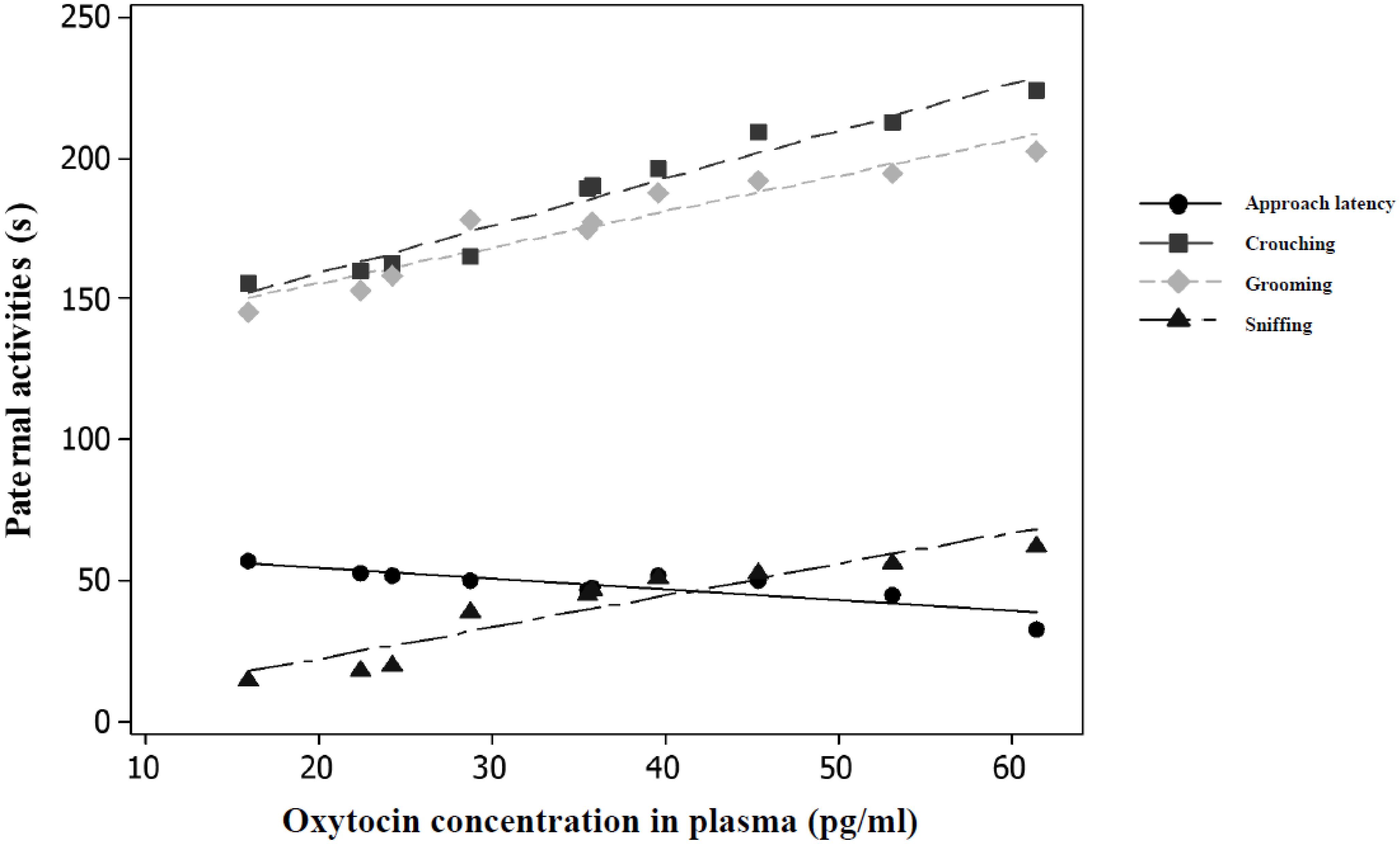
Figure 5. OXT concentrations were significantly correlated (P < 0.05, n = 10) with time spent in paternal activities in males PAT (n = 10).
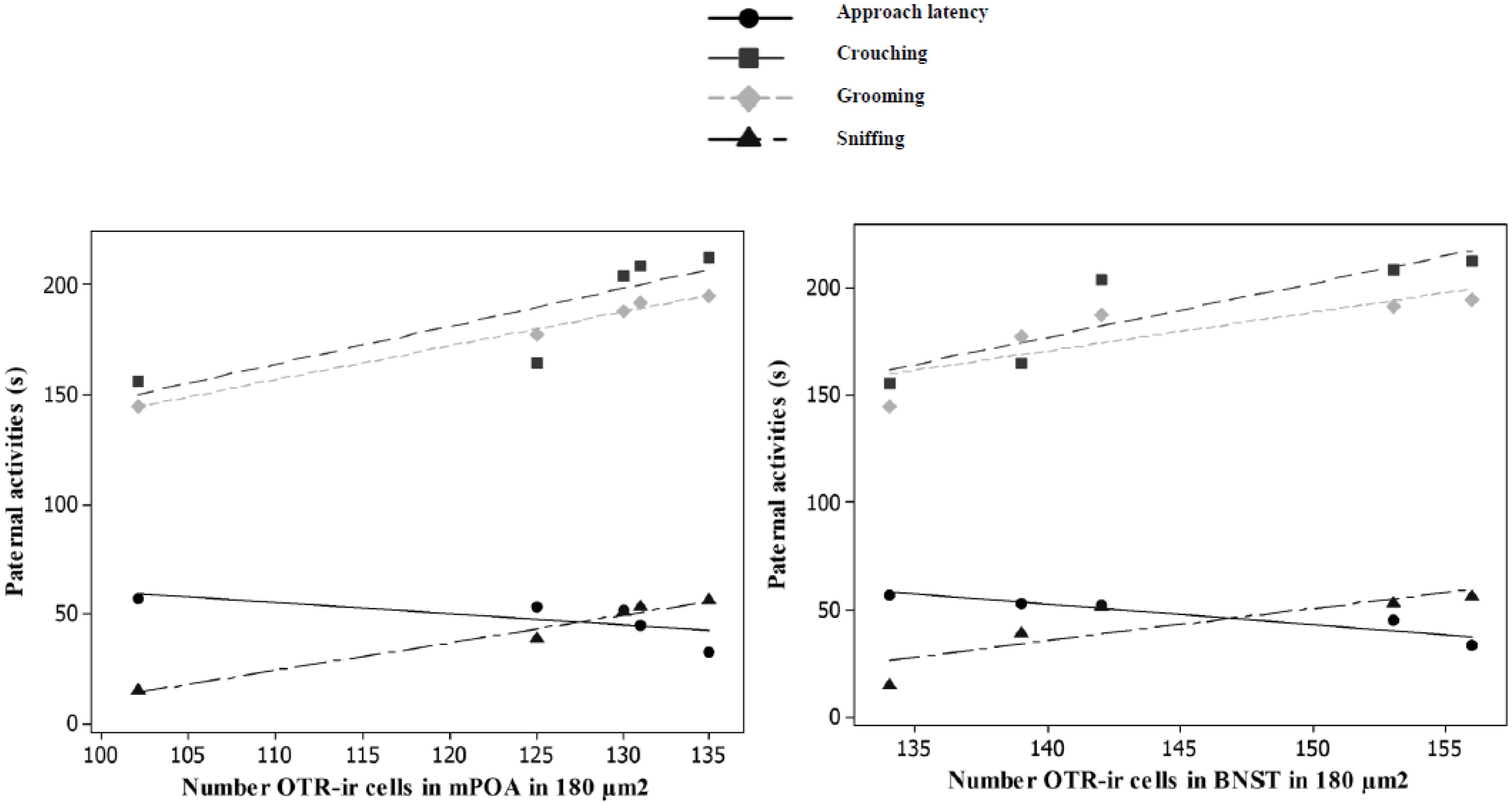
Figure 6. Significant correlations (P < 0.05, n = 5) were found between OXT concentrations and time spent in paternal activities.
4 Discussion
All the PAT male gerbils displayed grooming and sniffing of the pups. PAT males also had significantly higher levels of OT in plasma than AGG males. High concentrations of OT in PAT gerbils suggest that this neuropeptide could be involved in the regulation of paternal behaviour of this species. Furthermore, the association between the display of paternal behaviour and high OT concentrations can be attributed to paternal responses rather than to sexual or paternal experience, because sexually inexperienced gerbils were used in this study. We believe the gerbils that showed paternal behaviour have higher basal plasma OT concentrations than aggressive gerbils with the pups. However, this must be tested in a subsequent study. Regardless of baseline concentrations of OT, it is possible that interaction with the pups stimulates the central release of OT and that this causes an increase in plasma OT. Plasma OT concentration may be a biomarker of the activity of the central OT system (Neumann and Landgraf, 2012). In prairie voles, sexually inexperienced males that are exposed to the pups of same species have higher of OT concentrations in plasma compared with males that were not exposed to the pups (Kenkel et al., 2012). Furthermore, in prairie vole the treatment with an OT antagonist significantly reduces male parental behaviour and increases attack behaviour (Bales et al., 2004). In mandarin voles, new fathers have significantly higher levels of OT in serum than sexually inexperienced nonpaternal males (Yuan et al., 2019).
In this study, the number of OTR-ir cells in the mPOA and BNST in PAT males was significantly higher than that in AGG males. The significant presence of OTRs in males that interacted paternally with unfamiliar pups of their species suggests that this receptor is implicated in the regulation of paternal behaviour since the mPOA and BNST are involved in the regulation of parental behaviour (Lonstein et al., 2015; Horrell et al., 2018; Numan, 2020). In the Mongolian gerbil, mPOA and BNST have also been implicated in the regulation of paternal behaviour because these nuclei are significantly activated when sexually inexperienced paternal males interacted with pup’s same species compared with males that interacted candy, using as a marker of neural activation c-Fos (Romero-Morales et al., 2018). Coinciding with the results of this study, in mandarin voles has been shown that first-time fathers have higher levels of OTR mRNA expression in the mPOA than sexually inexperienced males (Yuan et al., 2019). In the laboratory rat, the exhibition of maternal behaviour is associated with an increase in the presence of OTRs or OTR mRNA expression in the mPOA/BNST (Pedersen et al., 1982; Meddle et al., 2007; Bosch and Neumann, 2012; Lonstein et al., 2015; Numan, 2020). In this rodent, studies of cause-effect relationships have widely demonstrated that OT and OTRs are part of the neuroendocrine mechanisms that regulate maternal behaviour (Pedersen et al., 1982; Meddle et al., 2007). Thus, intraventricular administration of OT to ovariectomised virgin females primed with estrogen-induced the onset of maternal behaviour (Pedersen and Prange, 1979; Pedersen et al., 1982). In addition, the intraventricular administration of an OTR antagonist to parturient rats removes the facilitating effect of OT on the onset of maternal behaviour (van Leengoed et al., 1987).
We did not find significant differences in the number of OTR-ir cells in the MeA and OB between PAT and AGG gerbils. This is possibly because both nuclei participate in positive and negative neuroendocrine mechanisms that regulate paternal behaviour (Kohl and Dulac, 2018; Horrell et al., 2018). Furthermore, very few OTR-ir cells were observed in the MeA and OB. This result differs from those found in California mice, in which the mRNA expression of OTR in the MeA was high in both fathers and sexually inexperienced males that interacted paternally with the pups (Perea-Rodriguez et al., 2015). This difference may be due to the species and/or the kit used to detect the presence of OTRs in neural areas. Perea-Rodriguez et al. (2015) reported that males with higher levels of OTR mRNA expression in the MeA displayed paternal behaviour more quickly than males with low mRNA expression. To the best of our knowledge, the association between the presence of OTRs in the OB and paternal behaviour has not been analysed. However, in laboratory rats, during parturition, OTR mRNA expression is increased in the OB (Meddle et al., 2007), and the direct administration of OT to the OB of virgin females facilitates the onset of maternal behaviour (Yu et al., 1996).
In this study, OT concentrations in plasma were significantly and positively correlated with paternal activities. Although it has been generally accepted that peripheral OT does not cross the blood−brain barrier (Yoshihara et al., 2018). As already mentioned, high concentrations of OT in plasma could be due to a high release of this neuropeptide at the central level (Neumann and Landgraf, 2012). In male rats, a positive correlation between the amount of paternal care and OT concentrations has also been indicated; intraventricular administration of 10 µg of OT increases grooming activity 9-fold compared to that of males injected with physiological saline solution (Drago et al., 1986).
We also found that the number of OTR-ir cells in the mPOA/BNST was significantly negatively correlated with the approach latency and significantly positively correlated with the time spent crouching over, grooming, and sniffing pups. This could suggest that OTR levels in the mPOA/BNST influence the amount of care given by paternal gerbils. This finding is interesting because in these nuclei, the receptors for the hormones that regulate parental behaviour, including estradiol, progesterone, prolactin, vasopressin, and OT, are mainly found in the mPOA (Lonstein et al., 2015; Horrell et al., 2018; Numan, 2020). In mandarin voles, sexually inexperienced males that display a high frequency of grooming have a greater number of OT-immunoreactive neurons in the PVN and SON of the hypothalamus compared with those that perform less grooming (Li et al., 2015). In this rodent, sexually inexperienced males that exhibit high levels of paternal behaviour also have a high number of OTRs in the nucleus accumbens, a brain region involved in the regulation of parental behaviour (Song et al., 2010; Horrell et al., 2018). In virgin juvenile female prairie voles, it was also reported that the time invested in contact with pups was correlated with the density of OTRs in the nucleus accumbens (Olazabal and Young, 2006). Likewise, virgin adult females of this rodent that display maternal behaviour have higher densities of OTRs in the nucleus accumbens than those that ignore or attack pups (Keebaugh and Young, 2011). Lactating rats that exhibit elevated levels of grooming have a higher OTR density in the mPOA and BNST than lactating mothers that perform less grooming (Champagne et al., 2003).
The significant correlation of OT concentrations in plasma and the number of OTR-ir cells in the mPOA/BNST with paternal activities suggests that OT and its receptor are involved in the neuroendocrine regulation of paternal behaviour in the Mongolian gerbil. However, the results of this study are correlational and therefore cannot provide evidence for a cause–effect relationship.
Future research should analyse the effect of intraventricular administration of OT and OTR antagonists on the paternal behaviour of the Mongolian gerbil.
Data availability statement
The raw data supporting the conclusions of this article will be made available by the authors, without undue reservation.
Ethics statement
The animal study was approved by Comisión de Bioética de la Facultad de Estudios Superiores Iztacala de la Universidad Nacional Autónoma de México (Folio 704). The study was conducted in accordance with the local legislation and institutional requirements.
Author contributions
LR: Conceptualization, Investigation, Methodology, Resources, Supervision, Writing – original draft, Writing – review & editing. BG: Methodology, Supervision, Writing – original draft. CA: Methodology, Supervision, Writing – original draft. FC: Methodology, Writing – original draft. JL: Conceptualization, Investigation, Project administration, Resources, Writing – original draft, Writing – review & editing.
Funding
The author(s) declare that financial support was received for the research, authorship, and/or publication of this article. This research was supported by Programa de Apoyo a Proyectos de Investigación e Innovación Tecnológica (IA205721) of Universidad Nacional Autónoma de México.
Conflict of interest
The authors declare that the research was conducted in the absence of any commercial or financial relationships that could be construed as a potential conflict of interest.
Publisher’s note
All claims expressed in this article are solely those of the authors and do not necessarily represent those of their affiliated organizations, or those of the publisher, the editors and the reviewers. Any product that may be evaluated in this article, or claim that may be made by its manufacturer, is not guaranteed or endorsed by the publisher.
References
Bales K. L., Kim A. J., Lewis-Reese A. D., Carter C. S. (2004). Both oxytocin and vasopressin may influence alloparental behavior in male prairie voles. Hormones Behav. 45, 354–361. doi: 10.1016/j.yhbeh.2004.01.004
Bales K. L., Saltzman W. (2016). Fathering in rodents: neurobiological substrates and consequences for offspring. Hormones Behav. 77, 249–259. doi: 10.1016/j.yhbeh.2015.05.021
Bosch O. J., Neumann I. D. (2012). Both oxytocin and vasopressin are mediators of maternal care and aggression in rodents: from central release to sites of action. Hormones Behav. 61, 293–303. doi: 10.1016/j.yhbeh.2011.11.002
Cantoni D., Brown R. E. (1997). Paternal investment and reproductive success in the California mouse, Peromyscus californicus. Anim. Behav. 54, 377–386. doi: 10.1006/anbe.1996.0583
Cao Y., Wu R., Tai F., Zhang X., Yu P., An X., et al. (2014). Neonatal paternal deprivation impairs social recognition and alters levels of oxytocin and estrogen receptor α mRNA expression in the MeA and NAcc, and serum oxytocin in mandarin voles. Hormones Behav. 65, 57–65. doi: 10.1016/j.yhbeh.2013.11.005
Champagne F. A., Francis D. D., Mar A., Meaney M. J. (2003). Variations in maternal care in the rat as a mediating influence for the effects of environment on development. Physiol. Behav. 79, 359–371. doi: 10.1016/s0031-9384(03)00149-5
Clutton-Brock T. H. (1991). The evolution of parental care Vol. 64 (New Jersey, USA: Princeton University Press). doi: 10.1093/acprof:oso/9780199692576.001.0001
Drago F., Pedersen C. A., Caldwell J. D., Prange J. A.J. (1986). Oxytocin potently enhances novelty-induced grooming behavior in the rat. Brain Res. 368, 287–295. doi: 10.1016/0006-8993(86)90573-1
Dumais K. M., Veenema A. H. (2016). Vasopressin and oxytocin receptor systems in the brain: sex differences and sex-specific regulation of social behavior. Front. Neuroendocrinol. 40, 1–23. doi: 10.1016/j.yfrne.2015.04.003
Elwood R. W. (1975). Paternal and maternal behaviour in the Mongolian gerbil. Anim. Behav. 23, 766–772. doi: 10.1016/0003-3472(75)90104-9
Elwood R. W. (1983). “Paternal care in rodents,” in Parental Behavior in Rodents. Ed. Elwood R. W. (Willey, New York), 235–257.
Fitzsimons E., Villadsen A. (2019). Father departure and children’s mental health: How does timing matter? Soc. Sci. Med. 222, 349–358. doi: 10.1016/j.socscimed.2018.11.008
Gimpl G., Fahrenholz F. (2001). The oxytocin receptor system: structure, function, and regulation. Physiol. Rev. 81, 629–683. doi: 10.1152/physrev.2001.81.2.629
Glasper E. R., Hyer M. M., Katakam J., Harper R., Ameri C., Wolz T. (2016). Fatherhood contributes to increased hippocampal spine density and anxiety regulation in California mice. Brain Behav. 6, e00416. doi: 10.1002/brb3.416
Gubernick D. J., Teferi T. (2000). Adaptive significance of male parental care in a monogamous mammal. Proc. R. Soc. London. Ser. B: Biol. Sci. 267, 147–150. doi: 10.1098/rspb.2000.0979
Horrell N. D., Hickmott P. W., Saltzman W. (2018). Neural regulation of paternal behavior in mammals: sensory, neuroendocrine, and experiential influences on the paternal brain. Neuroendocrine Regul. Behav. 43, 111–160. doi: 10.1007/7854
Jia R., Tai F., An S., Zhang X. (2011). Neonatal paternal deprivation or early deprivation reduces adult parental behavior and central estrogen receptor α expression in mandarin voles (Microtus mandarinus). Behav. Brain Res. 224, 279–289. doi: 10.1016/j.bbr.2011.05.042
Keebaugh A. C., Young L. J. (2011). Increasing oxytocin receptor expression in the nucleus accumbens of pre-pubertal female prairie voles enhances alloparental responsiveness and partner preference formation as adults. Hormones Behav. 60, 498–504. doi: 10.1016/j.yhbeh.2011.07.018
Kenkel W. M., Paredes J., Yee J. R., Pournajafi-Nazarloo H., Bales K. L., Carter C. S. (2012). Neuroendocrine and behavioural responses to exposure to an infant in male prairie voles. J. Neuroendocrinol. 24, 874–886. doi: 10.1111/j.1365-2826.2012.02301.x
Kohl J., Dulac C. (2018). Neural control of parental behaviors. Curr. Opin. Neurobiol. 49, 116–122. doi: 10.1016/j.conb.2018.02.002
Lee A. W., Brown R. E. (2002). Medial preoptic lesions disrupt parental behavior in both male and female California mice (Peromyscus californicus). Behav. Neurosci. 116, 968. doi: 10.1037/0735-7044.116.6.968
Lee A. W., Brown R. E. (2007). Comparison of medial preoptic, amygdala, and nucleus accumbens lesions on parental behavior in California mice (Peromyscus californicus). Physiol. Behav. 92, 617–628. doi: 10.1016/j.physbeh.2007.05.008
Li Y., Lian Z., Wang B., Tai F., Wu R., Hao P., et al. (2015). Natural variation in paternal behavior is associated with central estrogen receptor alpha and oxytocin levels. J. Comp. Physiol. A 201, 285–293. doi: 10.1007/s00359-015-0979-6
Lonstein J. S., Pereira M., Morrell J. I., Marler C. A. (2015). Parenting behavior. Physiol. Reproduction: New York, 2371–2437. doi: 10.1016/B978-0-12-397175-3.00051-X
Loskota W. J., Lomax P., Verity M. A. (1974). “A stereotaxic atlas of the Mongolian gerbil brain (Meriones unguiculatus),” in Ann Arbor Science (Publisher Inc, Michigan, USA).
Lowry O. H., Rosebrough N. J., Farr A. L., Randall R. J. (1951). Protein measurement with the Folin phenol reagent. J. Biol. Chem. 193, 265–275. doi: 10.1016/S0021-9258(19)52451-6
Maroun M., Sarussi-Elyahu A., Yaseen A., Hatoum O. A., Kritman M. (2020). Sex-dimorphic role of prefrontal oxytocin receptors in social-induced facilitation of extinction in juvenile rats. Trans. Psychiatry 10, 356. doi: 10.1038/s41398-020-01040-9
Martínez A., Arteaga-Silva M., Bonilla-Jaime H., Cárdenas M., Rojas-Castañeda J., Vigueras-Villaseñor R., et al. (2019). Paternal behavior in the Mongolian gerbil, and its regulation by social factors, T, ERα, and AR. Physiol. Behavior. 199, 351–358. doi: 10.1016/j.physbeh.2018.12.001
Martínez A., Ramos G., Martínez-Torres M., Nicolás L., Carmona A., Cárdenas M., et al. (2015). Paternal behavior in the Mongolian gerbil (Meriones unguiculatus): estrogenic and androgenic regulation. Hormones Behav. 71, 91–95. doi: 10.1016/j.yhbeh.2015.04.009
Meddle S. L., Bishop V. R., Gkoumassi E., van Leeuwen F. W., Douglas A. J. (2007). Dynamic changes in oxytocin receptor expression and activation at parturition in the rat brain. Endocrinology 148, 5095–5104. doi: 10.1210/en.2007-0615
Neumann I. D., Landgraf R. (2012). Balance of brain oxytocin and vasopressin: implications for anxiety, depression, and social behaviors. Trends Neurosci. 35, 649–659. doi: 10.1016/j.tins.2012.08.004
Numan M. (2020). The parental brain: mechanisms, development, and evolution (Oxford England: Oxford University Press).
Numan M., Young L. J. (2016). Neural mechanisms of mother–infant bonding and pair bonding: Similarities, differences, and broader implications. Hormones Behav. 77, 98–112. doi: 10.1016/j.yhbeh.2015.05.015
Olazabal D. E., Young L. J. (2006). Oxytocin receptors in the nucleus accumbens facilitate “spontaneous” maternal behavior in adult female prairie voles. Neuroscience 141, 559–568. doi: 10.1016/j.neuroscience.2006.04.017
Pedersen C. A., Ascher J. A., Monroe Y. L., Prange J. A.J. (1982). Oxytocin induces maternal behavior in virgin female rats. Science 216, 648–650. doi: 10.1126/science.7071605
Pedersen C. A., Prange J. A.J. (1979). Induction of maternal behavior in virgin rats after intracerebroventricular administration of oxytocin. Proc. Natl. Acad. Sci. 76, 6661–6665. doi: 10.1073/pnas.76.12.6661
Perea-Rodriguez J. P., Takahashi E. Y., Amador T. M., Hao R. C., Saltzman W., Trainor B. C. (2015). Effects of reproductive experience on central expression of progesterone, oestrogen α, oxytocin and vasopressin receptor mRNA in male California Mice (Peromyscus californicus). J. Neuroendocrinol. 27, 245–252. doi: 10.1111/jne.12264
Romero-Morales L., Cárdenas M., Martínez-Torres M., García-Saucedo B., Carmona A., Luis J. (2018). Neuronal activation associated with paternal and aversive interactions toward pups in the Mongolian gerbils (Meriones unguiculatus). Hormones Behav. 105, 47–57. doi: 10.1016/j.yhbeh.2018.07.010
Scatliffe N., Casavant S., Vittner D., Cong X. (2019). Oxytocin and early parent-infant interactions: A systematic review. Int. J. Nurs. Sci. 6, 445–453. doi: 10.1016/j.ijnss.2019.09.009
Sikes R. S., Animal Care and Use Committee of the American Society of Mammalogists (2016). 2016 Guidelines of the American Society of Mammalogists for the use of wild mammals in research and education. J. Mammalogy. 97, 663–688. doi: 10.1093/jmammal/gyw078
Song Z. Z., Tai F. D., Yu C. J., Wu R. Y., Zhang X., Broders H., et al. (2010). Sexual or paternal experiences alter alloparental behavior and the central expression of ERα and OT in male mandarin voles (Microtus mandarinus). Behav. Brain Res. 214, 290–300.2010. doi: 10.1016/j.bbr.2010.05.045
van Leengoed E., Kerker E., Swanson H. H. (1987). Inhibition of post-partum maternal behaviour in the rat by injecting an oxytocin antagonist into the cerebral ventricles. J. Endocrinol. 112, 275–282. doi: 10.1677/joe.0.1120275
Wang B., Wang L., Wang K., Tai F. (2018). The effects of fathering experience on paternal behaviors and levels of central expression of oxytocin and dopamine-2 type receptors in mandarin voles. Physiol. Behav. 193, 35–42. doi: 10.1016/j.physbeh.2018.02.043
Wuensch K. L. (1985). Effects of early paternal presence upon nonhuman offsprings’ development. Am. Zoologist 25, 911–923. doi: 10.1093/icb/25.3.911
Yoshihara C., Numan M., Kuroda K. O. (2018). Oxytocin and parental behaviors. Behav. Pharmacol. neuropeptides: oxytocin 35, 119–153. doi: 10.1007/978-3-319-63739-6
Yu G. Z., Kaba H., Okutani F., Takahashi S., Higuchi T. (1996). The olfactory bulb: a critical site of action for oxytocin in the induction of maternal behaviour in the rat. Neuroscience 72, 1083–1088. doi: 10.1016/0306-4522(95)00600-1
Yuan W., He Z., Hou W., Wang L., Li L., Zhang J. (2019). Role of oxytocin in the medial preoptic area (MPOA) in the modulation of paternal behavior in mandarin voles. Hormones Behav. 110, 46–55.019. doi: 10.1016/j.yhbeh.2019.02.014
Keywords: oxytocin, OTR, paternal behaviour, MPOA, BNST, MEA, OB
Citation: Romero-Morales L, García-Saucedo B, Carmona A, Cervantes FA and Luis J (2024) Paternal behaviour is associated with high levels of OT in plasma and the presence OTR in the mPOA/BNST in the Mongolian gerbil (Meriones unguiculatus). Front. Ethol. 3:1475119. doi: 10.3389/fetho.2024.1475119
Received: 02 August 2024; Accepted: 11 October 2024;
Published: 31 October 2024.
Edited by:
Aras Petrulis, Georgia State University, United StatesReviewed by:
Aubrey Kelly, Emory University, United StatesAllison Perkeybile, University of Virginia, United States
Copyright © 2024 Romero-Morales, García-Saucedo, Carmona, Cervantes and Luis. This is an open-access article distributed under the terms of the Creative Commons Attribution License (CC BY). The use, distribution or reproduction in other forums is permitted, provided the original author(s) and the copyright owner(s) are credited and that the original publication in this journal is cited, in accordance with accepted academic practice. No use, distribution or reproduction is permitted which does not comply with these terms.
*Correspondence: Juana Luis, bHVpc2RjQHVuYW0ubXg=