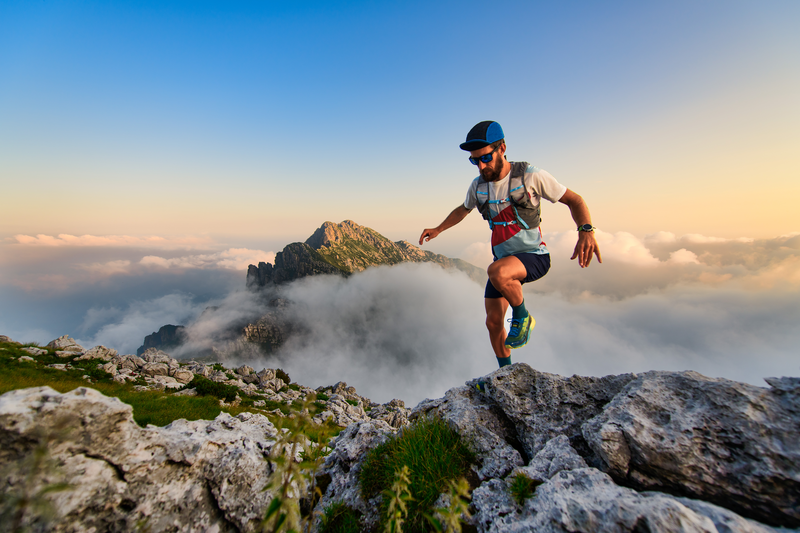
95% of researchers rate our articles as excellent or good
Learn more about the work of our research integrity team to safeguard the quality of each article we publish.
Find out more
PERSPECTIVE article
Front. Ethol. , 07 January 2025
Sec. Adaptation and Evolution
Volume 3 - 2024 | https://doi.org/10.3389/fetho.2024.1473358
This article is part of the Research Topic Sexual Selection and Evolutionary Fitness View all 6 articles
Conspicuous sexual signals come with costs and benefits. Such signals increase reproductive success but may also reduce survival or viability. It has recently been suggested that non-signal traits may alleviate some of those costs (termed “compensatory traits”). In this perspective piece, we argue that biological rhythms should be considered in the milieu of compensatory traits, as they can reduce the natural selection burden of signaling. This may be particularly true for the many sexual signals that are ephemeral (i.e., only periodically present like a courtship dance). Biological rhythms (e.g., circadian and circannual rhythms) are ubiquitous in nature and help organisms perform the right activity at the right time—this includes the timing of many sexual signals and reproductive traits. Timing itself may, in fact, reduce the costs of such sexual signals. Here, we review sexual signals that are governed by biological rhythms and discuss how signal modality and type (ornament, weapon, dominance trait) account for differences in how chronobiology may act as a compensatory trait. We then consider how biologists might examine the untested role of chronobiology as a compensatory trait and set forth compelling questions for future work.
Sexual selection arises when one sex competes for access to matings (or gametes) with the opposite sex (Andersson, 1994). This competition has resulted in a striking diversity of sexually selected signals—showy plumage, elaborate displays, and sophisticated acoustic advertisement that can showcase the location, identity, and quality of a signaler to an intended receiver (Andersson, 1994). Trade-offs are at the heart of signaling in the context of sexual selection because conspicuous sexual signals come with costs and benefits. The cost of signaling is reduced survival or viability, while the benefit is increased reproduction or fecundity (Getty, 2006; Figure 1). For instance, acoustic sexual signals (Table 1) produced by insects and anurans often attract mates but at the potential cost of attracting unintended receivers like deadly predators or parasites (Sakaluk and Belwood, 1984; Cade, 1975; Ryan et al., 1981; Zuk et al., 2006).
Figure 1. Conceptual figure demonstrating how biological rhythms can indirectly reduce the costs of ornaments (after Swallow and Husak, 2011). We use the Pacific field cricket in this example. The male (top left) produces a song (ornament) that female receivers (top right) use to make mating decisions. Singing requires high energetic expenditure and incurs trade-offs including the inability to forage while singing (a time allocation trade-off) and a trade-off between attracting mates and deadly parasitism (bottom right). The timing of singing (the “-” arrow of natural selection) may reduce the cost of deadly parasitism specifically. Males that coexist with eavesdropping parasitoids shift their singing time to avoid risk (Westwood et al., in prep).
Demonstrated costs of signaling may include reduced time that can be spent on other needs like foraging, production costs, reductions in immunity, locomotory costs, and increased risk of predation and parasitism (Kotiaho et al., 1998; Pope, 2000; Liker and Székely, 2005; Bonduriansky et al., 2008; Zuk and Kolluru, 1998; McCullough and Emlen, 2013). Indeed, selection from unintended receivers has even resulted in novel, protective sexual signals in crickets (Zuk et al., 2006; Heinen-Kay et al., 2020) and contributed to population divergence in guppies (Endler, 1980, 1982).
Importantly, sexual signals do not evolve in isolation. Rather, they are part of an integrated organismal phenotype (Ghalambor et al., 2003;. Ghalambor et al., 2004; Cornwallis and Uller, 2010) with underlying genetic and phenotypic correlations and represent ‘dynamic compromises’ (Husak et al., 2024) of a suite of selective pressures. Here, we are interested in whether biological timing may mediate trade-offs associated with sexual signaling that arise in this context. We argue that biologists should consider biological timing a part of the integrated organismal phenotype, and particularly so in the context of sexual selection. Recently, attention has been brought to the role of compensatory or cost-reducing traits, which are morphological, physiological, or behavioral traits that reduce the burden of (in this case) sexual signaling (Møller, 1996; Husak and Fox, 2006). If selection favors efficient and optimal investment and minimizes costs (Getty, 2006), over time, it should favor the evolution of compensation, and the more expensive a sexually selected signal is, the stronger should be the selection for compensation (Oufiero and Garland, 2007). Notably, such a process may make it difficult to detect signaling costs if they have already been compensated for (Husak et al., 2011). Note that we are not particularly concerned here with whether signals are honest (Grafen, 1990; Penn and Számadó, 2020) or not—rather, we are interested in whether (for signals that do bear some cost) biological timing appears to have evolved in ways that buffer some of those costs.
Seemingly, most research examining compensatory traits has focused on morphology (Swallow and Husak, 2011). For instance, increased ventricle mass (a compensatory trait) reduces the negative effect of sword length (a sexually selected signal) on swimming endurance in 57 species of swordtail fishes (genus Xiphophorus; Oufiero and Garland, 2007). In another example, increased wing size helps male birds compensate for the aerodynamic costs associated with long, ornamental tails (Møller et al., 1998; Evans and Hatchwell, 1992; Craig, 1989), and sexually dimorphic species of male stalk-eyed flies compensate for reduced flight performance due to increased eye spans with increased wing area (Husak et al., 2011). Despite their significance, uncovering compensatory traits mitigating sexually selected signals such as acoustic advertisement and behavioral displays has received considerably less attention. This lack of focus may be attributed to challenges in data collection since such traits are ephemeral and may only be present periodically (e.g., at certain times-of-day or times-of-year; see “Period,” Table 1).
We propose that the timing of signaling (biological rhythms) may be an important and overlooked mechanism that compensates for the costs of signals and perhaps particularly so for more ephemeral signals. Biological rhythms (Table 1) are behavioral, morphological, physiological, developmental, or molecular phenomena whose recurrence is synchronized with periodic, geophysical cycles (Dunlap et al., 2004). They are ubiquitous in nature and help organisms take advantage of the opportunities and cope with the difficulties presented by a rhythmic environment (Westwood et al., 2019). Indeed, organisms must perform a great variety of periodic behaviors and physiologies, and not all such activities can, or should, co-occur [i.e., biological rhythms provide both “intrinsic” and “extrinsic” adaptive value (Table 1); Sharma, 2003; Jabbur et al., 2024]. For example, yeast reduces mutation rates by temporally constraining cell division to the reductive phase of metabolism (“intrinsic” adaptive value; Chen et al., 2007), and numerous organisms (such as algae) have been found to coincide UV-sensitive activities to the nighttime, thus avoiding harmful UV radiation (“extrinsic” adaptive value; Nikaido and Johnson, 2000). As such, scheduling activities to their optimal timing is thought to be adaptive (Hutchinson et al., 1993; Sharma, 2003). Biological rhythms are typically characterized as being endogenously driven (i.e., they persist in the absence of external time cues, or, “Zeitgebers”; Table 1), and at least in the case of circadian rhythms (i.e., ~24 h rhythms; Table 1), they are seemingly so advantageous that nearly all eukaryotes have biological pacemakers in nearly every cell (Dunlap, 1999).
A timing phenotype may be both sexually selected as well as compensatory given that multiple vectors of selection could act on the same trait in different ways. The notion that biological rhythms can be targets of sexual selection is not new. Hau et al. (2017) provide a compelling framework and wealth of examples by which circadian (and circannual; Table 1) rhythms are directly acted on by sexual selection (e.g., experimental delay of singing increases the likelihood of being cuckolded in male blue tits; Greives et al., 2015). However, here, we draw the reader’s attention to cases where the signal is something other than timing itself (e.g., the song itself rather than the timing of singing) and biological timing reduces the burden of that signal (i.e., is compensatory). It is not hard to envision a situation where performing a costly sexual signal at the “wrong” time-of-day or year would be disadvantageous, if not deadly, to an organism. Indeed, if selection optimizes benefits while reducing costs, performing mating behaviors only at certain times should minimize the risk of predation and/or parasitism (Bulla et al., 2016), help organisms synchronize mate-seeking behaviors between the sexes (Roth et al., 2009; Davidson and Menaker, 2003), and reduce intraspecific competition for soundscapes (Ancillotto and Labadessa, 2023). Given that all sexually selected signals are necessarily being produced at a given time (i.e., time is always happening), we argue that the milieu of compensatory evolution can and should be expanded to consider biological rhythms.
Here, we explore examples of rhythmic, sexually selected signals. Due to the strict criteria for illustrating the endogenous nature of biological rhythms (see Box 1), not every sexually selected signal we discuss has been shown to be driven by a cellular pacemaker [e.g., they have not been examined under constant environmental conditions for months (circalunar) or years (circannual)]. These experimental perturbations are highly artificial, are logistically and sometimes ethically impossible, and serve only to show endogeneity and do not accurately reflect the environment. Moreover, it can be assumed that most observed biological rhythms indeed have a molecular basis given that it has consistently been shown to be the case (Vitaterna et al., 2001). Considering that their compensatory value is not necessarily dependent on their molecular basis, we have opted to include examples of biological rhythms even in cases for which the presence of a molecular pacemaker has not been confirmed.
Box 1. Clocks 101: schematic and short introduction to biological rhythms for novice readers.. A. Schematic depicting an entrained biological rhythm (left side) which is then released into free-running conditions (i.e., when the Zeitgeber is removed; right side). The behavior or physiology (y-axis) is shown as an oscillating, solid black line that peaks and troughs (i.e., reaches its maximum and minimum, respectively; highlighted in red) with ~24-h periodicity. The distance between the peak and trough is referred to as the amplitude (also highlighted in red). Solid gray bars during the entrained portion refer to the dark phase (i.e., lights-off), and white bars indicate the light phase (i.e., lights-on). Once released into free-running conditions, constant darkness is experienced (shown as an entirely gray background). Such visual identifiers are standard in chronobiological research.B. Schematic portraying the circannual breeding plumage (left) and circadian singing rhythms (to the right in pop-out) of the superb fairy wren (Dalziell and Cockburn, 2008). The duration of each period is highlighted in red (1 year and 24 h for circannual and circadian rhythms, respectively), and the solid gray bars on the right depict the dark phase of the circadian period (shown as 12 h).
Countless examples exist of sexual signals that have daily or circadian underpinnings, particularly behavioral signals such as acoustic advertisement and mating displays (Hau et al., 2017). Several proximate and ultimate hypotheses have been proposed to explain the timing of sexual displays, including circadian hormone cycling (Staicer et al., 2019), female fertility (Mace, 1987), foraging conditions (Kacelnik and Krebs, 1983), and predation risk (Staicer et al., 2019). Many of these hypotheses are compatible with the notion of chronobiological compensation that we propose. As stated above, the optimization of the timing of sexual signaling to reduce natural selection pressures should reduce the cost of the signal and, thus, play a compensatory role. Interestingly, there exists a behavioral and physiological connection between many circadian and circannual rhythms, as circadian acoustic advertisement and mating displays are often only performed at certain times-of-year (Box 1; Hau et al., 2017). First, let us explore some examples of sexually selected signals that are governed by circadian rhythms and how these rhythms may compensate for their costs.
The dawn chorus of birds is one of nature’s most charismatic and quintessential alarm clocks and perhaps the best-known example of a circadian signal. Various mutually inclusive environmental factors likely influence the timing of the dawn chorus. Due to lower light and temperature levels, costs associated with foraging at dawn are much greater than later in the day (Kacelnik, 1979), and so scheduling non-foraging behaviors (such as mating) at this time helps birds to get the best “bang for their buck” out of each 24-h cycle. While numerous other hypotheses exist (see Gil and Llusia, 2020), taken together, each of these factors contributes to the optimal timing of dawn chorus in many bird species. This timing adaptively reduces the natural selection costs (e.g., foraging and energetic costs) associated with sexual signaling, supporting our thesis that biological timing itself is an important compensatory trait that can be selected.
Consider the circadian calling behavior of male Pacific field crickets (Teleogryllus oceanicus; Westwood et al., 2024; Zuk et al., 1993). These crickets are native to Australasia but are introduced in the Hawaiian Islands where they coincide with the deadly, acoustically orienting parasitoid fly Ormia ochracea. While a male calling song is important for attracting female crickets to mate, it also poses the risk of attracting gravid female flies in search of suitable hosts for their larvae. Evidence suggests that the male T. oceanicus in Hawaii (but not in other parts of their range where flies are absent) has evolved altered circadian rhythms in its calling behavior in response to fly parasitism (Westwood et al., in prep; Zuk et al., 1993), presumably to align mating behaviors to times-of-day that the risk of parasitism is relatively low (Figure 1). As such, the timing of male calling behavior may, in part, compensate for its risk. Importantly, the timing of biological rhythms operates within a broad set of constraints (i.e., there is typically more than one reason that a certain behavior or physiology occurs at a given time-of-day; Westwood et al., 2019; Sharma, 2003). We do not intend to suggest that circadian rhythms (or other biological rhythms) evolved solely as a means to alleviate the cost of sexually selected signals; rather, it is likely one of the many benefits conferred by optimizing the timing of producing or displaying sexual signals. Indeed, the biological timing of sexual signals may adaptively evolve to help ease their burden (as is the case in T. oceanicus).
In addition to the chorus of crickets and birds, singing in frogs is another well-documented example of a daily, acoustic display. While certain species are nocturnally active (Murphy, 1999; Bridges et al., 2000), others predominantly sing during the daytime (Zigler et al., 2023). Considering the high energetic cost of calling [e.g., European tree frogs (Hyla arborea) experience a 30–40-fold increase in metabolic rate while calling; Grafe and Thein, 2001), only singing while females are receptive would reduce costs. Calling frogs are also subject to eavesdropping predatory bats (Tuttle and Ryan, 1981) and biting midges (McKeever, 1977), and it is possible that changed biological timing could reduce those natural selection costs; these study systems seem like natural contexts in which to experimentally address timing as compensation. Gibbons (Family: Hylobatidae) are also notable for their sex- and species-specific songs (Cheyne, 2008), and males are known to chorus at dawn (Chivers, 1976; Mitani, 1985). Male gibbons sing to advertise to females (Cowlishaw, 1992), signal condition (Barelli et al., 2013), assert territorial dominance (Henwood and Fabrick, 1979), and advertise pair-bond status (Wickler, 2010). As with birds, the timing of the dawn chorus in gibbons is likely due to a confluence of environmental factors, such as favorable conditions for sound transmission (Clink et al., 2020), optimizing the timing of foraging (Cheyne, 2008), and thermoregulation (Cowlishaw, 1996). It is easy to imagine a scenario in which spending precious daylight hours singing (which is often initiated before sunrise; Cheyne et al., 2008) in lieu of foraging could be costly and indeed may coincide an energetically demanding behavior with a time-of-day that females are unreceptive or environmental conditions are thermally and/or acoustically unfavorable. Thus, optimizing the timing of sexual signaling may reduce costs.
In contrast with the relatively fast circadian period, circannual rhythms are governed by the leisurely rotation of the Earth around the sun (Box 1). Sunlight acts as the primary Zeitgeber of circannual rhythms, with annual changes in daylength setting the pace of the molecular clock (Gwinner, 1981; Gwinner, 1986). However, for equatorial species (such as many birds), changes in the annual intensity of daytime light, which directly corresponds to rainy and dry seasons, may act as the primary Zeitgeber (Dawson et al., 2001). In an ultimate sense, circannual mating systems align reproduction with times-of-year when food availability is greatest and offspring survival is most ensured (Baker, 1938; Kreitzman and Foster, 2010). Thus, in species with short gestation periods, mating and parturition may occur within a single season, whereas species with long gestation periods mate and parturate in different seasons (Bronson, 1989, 1988). Whether short gestation periods facilitate a more phenotypically plastic breeding phenology, as compared to species with long gestation periods which must rely more greatly on the predictability of circannual timing mechanisms, is an interesting and timely area of research given the concerns surrounding climate change (see, e.g., Tyler et al., 2024; Froy et al., 2019; Bonnet et al., 2019; Renaud et al., 2019; Mougi, 2020; Aubry and Williams, 2022).
One key feature of endogenous, biological rhythms is that they help organisms to anticipate, rather than directly respond to, changes in the environment. While this is certainly necessary for primary sexual signals (gonadal and egg development; Gwinner, 2012), sexually selected signals too must develop in advance of mating. For example, male birds of many species develop showy breeding plumage (Peters et al., 2013), many fish species develop breeding coloration (Kodric-Brown, 2015), female mandrills (Mandrillus sphinx) show marked increases in sexual skin tumescence (Setchell and Dixson, 2001), and male deer grow antlers (Goss, 2012) in advance of the breeding season. In the case of breeding plumage, male birds can reduce the maintenance cost of such sexual traits by producing them only in anticipation of the mating season and removing them otherwise (Møller, 1996). In one example, rock ptarmigan develop brilliant white plumage during snowy winters, that females then trade in for their cryptic breeding plumage when the snow begins to melt. In contrast, males retain their white feathers approximately 3 weeks longer, making them attractive to females while simultaneously conspicuous to predators. Once females begin egg incubation and can no longer be fertilized, males soil their flashy attire with mud and dust prior to the onset of their molt (Montgomerie et al., 2001). As evidenced by this creative stopgap, the costs associated with conspicuous plumage are indeed quite high, and losing (or soiling) them quickly after the breeding season is advantageous. This is a clear example of how precise timing of sexual signaling can compensate for some of the cost (in this case predation) of a conspicuous signal.
Variation in the timing of producing sexually selected signals may help, or hinder, an individual’s reproductive success. On one hand, being one of the first individuals in a population to ready themselves for reproduction may result in more matings, but only if the opposite sex is receptive and potentially at the cost of increased conspicuousness to predators and/or parasites. Interestingly, musth in African elephants has been shown to be circannual, though asynchronous among males (i.e., each male goes into musth at the same time each successive year, but this varies among males). This discordant timing likely reduces intrasexual conflict among males and is enabled by the availability of females in estrous throughout the year (Poole, 1987). Critically, intrasexual conflict in elephants involves energetically expensive combat that may result in serious injury—the circannual yet asynchronous nature of musth helps to resolve such conflicts (Murphy et al., 2019; Keerthipriya et al., 2019). Individual variation in the timing of male display is also sometimes seen in bird species where young adult males are unable to compete with older, more experienced males. As such, these young male birds may reduce the costs of conspicuous plumage (e.g., predation) by delaying plumage maturation and aligning their mating efforts to times when intrasexual competition is less fierce (Hawkins et al., 2012). However, this strategy is facultative and such subadult males are likely to mate when and if the opportunity arises (Studd and Robertson, 1985). Delaying the development of breeding plumage to times when matings are most likely to be acquired underscores the cost of such traits and how compensation via biological timing may in fact ease those associated costs.
Compared to the wealth of circadian rhythm research, a relative paucity of studies exists concerning circatidal and circalunar rhythms [while a Google Scholar search (July 2024) for the key term “circadian clock” returns ~604,000 results, searches for “circatidal clock” and “circalunar clock” return 291 and 333 results, respectively]. As such, both their molecular machinery and underlying mechanisms are less well understood (Rock et al., 2022). Yet, there is something enchanting, and indeed primordial, about the power of the ocean tides and the moon to drive biological rhythms. Circatidal rhythms are dictated by the tidal cycle making them the shortest rhythm we discuss (~12.4 h), while circalunar rhythms correspond to the lunar cycle and last approximately 1 month (~29.5 days; Rock et al., 2022).
Most evidence for circatidal rhythms focuses on foraging (e.g., the mangrove cricket; Satoh, 2017) and movement (usually upward and downward migrations in sand or water; e.g., Gamble and Keeble, 1903; Barnwell, 1966). While we are unaware of sexual signals governed by circatidal rhythms, they most certainly exist; and given the incredible environmental fluctuations that occur over short periods of time in tidal regions, timing, which minimizes costs associated with sexual signaling, should certainly be selected for.
There are numerous examples of primary sexual traits that are governed by circalunar rhythms such as synchronous spawning in marine invertebrates like corals (e.g., Lin et al., 2021) and a myriad of examples in teleost fishes that include traits like egg-laying behavior, migration to spawning grounds, and gonadal maturation (e.g., Shima et al., 2020; Takemura et al., 2004; reviewed in Takemura et al., 2010; Takemura et al., 2004). In each of these cases, there are certainly mating behaviors (including sexual signaling) that are governed by the same lunar cycles. For instance, numerous species of damselfish exhibit peak reproductive activity near the full and/or new moon (Takemura et al., 2010; Robertson et al., 1990). It is hypothesized that circalunar rhythms in teleost fishes evolved to minimize predation risk (Takemura et al., 2010), suggesting that these rhythms are compensatory. There are some striking examples of circalunar sexual signaling in insects and birds as well. Around the full and new moon, midges of the species Clunio marinus emerge from the ocean to perform nuptial dances, mate, and oviposit (Kaiser et al., 2016; Figure 2). This timing corresponds with the most extreme low tides that expose larval habitat; offspring survival would undoubtedly be negatively impacted if individuals were to miss this crucial breeding window. Note that some of these examples blur the line between timing being compensatory versus timing being sexually selected itself. Interestingly, male African houbara bustard birds perform nocturnal courtship displays with visual and acoustic components, but only during a full moon (Figure 2). It is hypothesized that the bright moonlight helps displaying males detect predators and maximizes the visibility of the visual display (Alonso et al., 2021). If the bright moonlight helps displaying males detect and avoid predators, the timing of this behavior may compensate for the cost of predation.
Figure 2. Photos of organisms with periodic sexually selected signals. Circadian: (A) Crested lark singing during dawn chorus (Wikimedia Commons) and (B) tungara frog calling for mates (Wikimedia Commons); circannual: (C) rock ptarmigan male (front) and female (back; Wikimedia Commons) and (D) elk using antlers in male–male competition (photo credit: Christopher Welsh); circalunar: (E) Houbara bustard male with nocturnal, moonlight-triggered display (Wikimedia Commons) and (F) midge (Clunio marinus; photo credit: Kage and Neumann).
Organisms send sexual signals through a variety of sensory channels or signal modalities. The most common of these are visual, acoustic, chemical, and tactile (Breed and Moore, 2021). Here, we explore whether particular modalities that each have their own costs and benefits might affect whether and how timing might compensate for the costs of these signals. We acknowledge that much of this is speculative, as we are unaware of any research in this specific area.
As signals are not transmitted to receivers in a vacuum, but rather in complex environments containing physical features (vegetation, etc.), competing signalers, potential mates, and eavesdropping natural enemies, certain modalities are more effective and less costly in particular environments (Endler, 1993). For instance, visual signals are well known for being conspicuous, including to eavesdropping predators, and can be quickly transferred to receivers (at the speed of light); however, they require light and are impeded by objects like vegetation (reviewed in Breed and Moore, 2021). In contrast, chemical, acoustic, and tactile signals can function in the dark and are not impeded by objects, though they each have additional advantages and potential costs. Acoustic signals can be airborne or substrate-borne and have the major advantage of traveling long distances and providing detailed information about the location of the sender. Even animals that lack ears (like many insects) can still gather vibrational information (Hill, 2009). However, acoustic signals attract many predators and parasites, are energy-intensive (Gammon and Baker, 2004), and are easily masked by environmental noise (noise being background stimuli, natural or anthropogenic, in any modality that can compete with or mask a signal). Tactile and chemical signals are less susceptible to being masked by noise. Chemical signals are long-lasting even after the sender has moved locations, and often evolve to be quite specific, which limits the ability of other organisms to eavesdrop on them. Interestingly, tactile signals are only useful in very close proximity, which makes them rather private—one can only eavesdrop on a tactile signal if they are also touching the sender (Breed and Moore, 2021).
Visual signals often include exaggerated morphological structures like growths (e.g., horn, long feather) or striking coloration as well as behavioral components that show off these morphological elements. Thus, the investment in growing and maintaining these signals is likely to be high in terms of energy and may impede locomotion and increase conspicuousness to predators and parasites. Such costs may be reduced by exhibiting or growing visual signals only during a specific breeding season [e.g., in deer (Goss, 2012) and birds (Møller, 1996)] via circannual or even circalunar rhythms. There are also often circadian rhythms in the behavioral component (display) of the signal that may align the display with the timing of environmental conditions or receiver behavior that allow it to be most effectively received (e.g., Guevara-Fiore et al., 2010). In contrast, acoustic signals may require specialized structures to produce sounds like the syrinx, modified wings, or even modified body structures used for substrate-borne vibrations, which they maintain throughout their adult lives. The energy required to produce acoustic signals in real-time can be quite costly (Grafe and Thein, 2001). Additionally, conspicuous acoustic signals suffer danger from eavesdroppers and can be masked by environmental and anthropogenic noise and competing signals. We would thus predict that the timing of acoustic signals is optimized to minimize masking noise, risk of predation and parasitism, and lost foraging costs. Indeed, examples of compensation for each of these costs can be found in the literature, for instance shaping the circadian rhythms in bird song (Ancillotto and Labadessa, 2023; Bulla et al., 2016; Kacelnik, 1979).
Unlike acoustic and visual signals, there is a reduced risk of masking or eavesdropping on chemical signals since receivers require specific receptors to perceive signals. Similarly, tactile signals are the most private mode of communication and thus likely have a very low risk of masking or eavesdropping. Given all of that, we hypothesize that if natural selection costs of masking and risk of predation and parasitism are the highest for visual and acoustic signals, we would expect those modalities to experience stronger selection for timing as compensation, as compared to chemical and tactile communication, which are generally more private communication channels. Interestingly, depending on their molecular structure, chemical signals may be ephemeral or may remain in the environment for a long period of time, which could make studying their rhythmicity challenging.
In this section, we consider whether biological rhythms might compensate for different types of sexually selected signals in different manners. We are prompted to consider this possibility because sexually selected signals can be thought of as falling into one of four major categories: ornaments (Table 1), dominance traits, combat traits, and intrasexually selected weapons (Table 1; Rico-Guevara and Hurme, 2019). Ornaments are morphological, acoustic, behavioral, or chemical features, which evolve through intersexual selection (between the sexes; McCullough et al., 2016), while the other three types of traits evolve via intrasexual selection. For example, in gorillas, males have distinctive fur coloration that serves as a status indicator (dominance), musculoskeletal traits like back muscles and enlarged arms that aid in combat (combat), and canine teeth and fists that are used to harm other males during fights (intrasexually selected weapons; Rico-Guevara and Hurme, 2019; Caillaud et al., 2008). While fascinating chemical weapons exist (Nekaris et al., 2020), the term weapon is often defined narrowly to describe “projections used in combat”; using such a definition, these traits are always morphological/structural (Emlen, 2008). Examples of weapons include spicules in nematodes, pedipalps in spiders, hammers in mantis shrimp, mandibles in insects, jaws in fishes, fangs in frogs, horns in lizards, and canines and horns in mammals (Rico-Guevara and Hurme, 2019). One could imagine that ornaments, weapons, dominance traits, and combat traits may differ in their costs and benefits, which may affect how chronobiology mediates these trade-offs.
The onset of ornamentation may differ slightly in timing compared to intrasexually selected signals. To illustrate this idea, consider three-spined stickleback fish (reviewed in Tinbergen and van Iersel, 1947). Males arrive at breeding sites before females are present. They build nests and use dominance and combat traits to establish territories, while intersexual selection begins later after nests are established and females become gravid. There are many such examples where intrasexual selection precedes intersexual selection, and it begs the question of whether and how the same Zeitgebers are able to coordinate traits important for each. Since social behaviors may act as Zeitgebers [e.g., in bees (Bloch and Robinson, 2001) and in diverse vertebrates (Favreau et al., 2009)], the switch from intra- to intersexual behaviors may involve social cues (like the presence of mates). Furthermore, the switch itself from intrasexual behaviors like combat and dominance to intersexual signaling likely has trade-offs. In the stickleback example above, one can imagine the costs to switching early (not enough investment in territory and nest to be competitive) versus late (missed mating opportunities).
It is possible that intrasexually selected weapons are more costly than other types of signals. Weapons that are morphological structures must be grown by organisms, which requires time and energy. Weapons may also be costly to maintain and may hinder movement, sometimes leading to their adaptive loss when not in use (e.g., antlers, which are gained and lost circannually; Goss, 2012). Additionally, there is likely less plasticity associated with weapons as compared to, for instance, behavioral traits that are immediately flexible. One needs only to consider the development time and cost of maintaining antlers (weapons) compared to the short-term cost of engaging in combat. If weapons are indeed more costly, we might expect there to be stronger selection for compensation, including through biological rhythms, though more ephemeral traits may be less constrained evolutionarily. Additionally, there are multiple contexts where chronobiological compensation could occur, for example, the onset of development, the rate of development, and the timing of the loss of the weapon.
Conventional approaches for examining compensation for sexually selected signals involve quantifying ornament size (assuming larger ornaments are more costly) alongside the relative extent of the associated compensatory trait; finding a positive relationship between these variables indicates compensation (Husak et al., 2011). Here, we suggest five possible approaches (Table 2) that link variation in biological timing with variation in sexual signals and the traits expected to trade-off with signals (e.g., predation risk, immune function, and survival). The first two approaches parallel traditional methods described above, whereas the final three allow for a more experimental approach, with the last two capitalizing on tools available in chronobiology (e.g., the ability to experimentally shift or knockout rhythms). Note, of course, that there will be system-specific nuances that will certainly affect trade-offs associated with signaling (e.g., trade-offs can be condition-dependent; Tomkins et al., 2004; Hill, 2011), and biological timing is only one of the many types of compensatory traits that may evolve with signals in a correlated manner (Swallow and Husak, 2011).
Table 2. Five experimental approaches that could be used to investigate the role of biological rhythms as compensation for sexually selected signals [For each, we indicate the expected result that would support the idea of biological rhythms as compensatory traits (middle column) and discuss the relative tractability and important considerations for each approach (right column)].
Given the paucity, if not the absence, of studies examining chronobiology as compensation for sexually selected signals, there exists ample opportunity to investigate such questions. We propose the following areas of interest as a springboard for future studies. Indeed, we hope future work not only expands upon these areas but illuminates entirely new avenues of research.
First, as humans disrupt ecosystems at an unprecedented rate, anthropogenic change will undoubtedly alter canonical environmental clock cues (namely, light and temperature) and thus biological rhythms as a whole. A timely question then is, whether and how anthropogenic effects such as artificial light at night (ALAN) and anthropogenic noise alter Zeitgebers and thus clocks, and how this may alter the compensatory effect of clocks. For example, ALAN advances the onset of dawn calling in songbirds and increases male extra-pair paternity (Kempenaers et al., 2010). Frogs sing less and move around more when exposed to ALAN (Baker and Richardson, 2006). Regarding circannual rhythms, many animals breed once a year and optimize that timing based on resource availability. “False spring” or other human-induced climate change events will likely shift the timing of breeding seasons. For instance, caribou antler growth, courtship, and calving are aligned with the availability of high-quality forage, but altered plant phenology is leading to a mismatch between calving and food availability and is hypothesized to be contributing to the global decline of the species (Vors and Boyce, 2009).
As is unfortunately all too common in our field, we have so far ignored the important role of receivers. Receivers, often females, may have preferences that are rhythmic that may also be costly. For example, female scarab beetles release sex pheromone every 48 h, which aligns with male receivers’ receptor gene and response to the sex pheromone, which has the same unusual 48-h periodicity (Wang et al., 2024). It is possible that rhythmic female preferences dictate rhythmic sexual signals rather than vice versa. In other words, the timing of receiver preferences has been optimized to minimize trade-offs, and male signaling simply evolves in response. This example highlights the pernicious bias in our field to study male traits and the selection acting on them before investigating female traits (Ah-King, 2022). Relatedly, systems where males are the receiver should not be ignored; mosquitoes in tropical regions mate in highly synchronized and time-of-day-specific daily swarms where males are the phonotactic sex (Somers et al., 2022). Thus, a fundamental and largely ignored question is, what is the role of receiver preferences when sexual signals are chronobiological? An understanding of this basic feature of animal communication will then certainly impact how we think about whether and how rhythms compensate for the costs of sexual signals—for instance, if timing is optimized to reduce costs for receivers, the signaler may not also be able to optimize timing to reduce signaling costs (sexual conflict; Ferguson et al., 2015). Furthermore, if the timing of signals and preferences have coevolved to minimize costs to both signalers and receivers, a shift in the timing of either the signal or the preference would decouple them, and one party would lose the compensatory value of timing.
Finally, different life history strategies and mating systems lead to different costs of signals, and this may select for different rhythmic compensation. We can illustrate this idea using weapons. Regarding life history, animals that have evolved to have a very short lifespan may not benefit from developing and then losing a costly weapon, while animals with long lifespans and multiple breeding seasons may benefit from losing costly weapons when not in use (e.g., ungulates that lose and regrow antlers; large and elaborate duck penises that decrease their mass by five times during the non-breeding season each year; Höhn, 1960). As for mating systems, sexual selection differs among systems (e.g., similar selection on males and females in monogamous systems and much stronger selection on males in polygynous systems, where males have multiple female mates). This should translate to differences in how selection acts on weapons, leading to larger and more costly weapons in polygynous systems compared to monogamous or polygynandrous (both males and females mate multiply). Relatedly, polygynous animals across taxa have greater sexual size dimorphism than monogamous species (Clutton-Brock, 1985); with bigger costs, we would expect stronger selection for compensation, including through timing. The exciting question then is, whether and how do life history and mating systems shape the costs of sexual signals and the compensation of those costs through timing?
Understanding how and why sexual signals evolve has fascinated biologists since Darwin initially proposed that exaggerated male traits may evolve via female choice (Darwin, 1871). A central tenet of this theory is that such traits should impose trade-offs on the bearer (Andersson, 1994). We propose that the timing of the production, maintenance, and display of such traits may help to ease their cost. The idea that biological rhythms are crucial to survival and reproduction is not new (timing underlies nearly all behavior and physiology); however, the goal of chronobiology for the majority of its tenure has been to understand its molecular underpinnings. Given the advances in this area and its ubiquity, calls to incorporate biological timing into a diversity of research have been made (see Westwood et al., 2019; Nelson et al., 2024). Indeed, time is a neglected factor in many biological studies (Nelson et al., 2024), and considering when a sexual signal is developed, maintained, and/or displayed may demystify whether and how chronobiology compensates for costly sexual signaling. We hope this work inspires the much-needed integration between chronobiologists and evolutionary biologists.
The original contributions presented in the study are included in the article/supplementary material. Further inquiries can be directed to the corresponding author.
MW: Conceptualization, Funding acquisition, Investigation, Project administration, Writing – original draft, Writing – review & editing. EB: Conceptualization, Funding acquisition, Investigation, Project administration, Writing – original draft, Writing – review & editing. GW: Conceptualization, Investigation, Project administration, Visualization, Writing – original draft, Writing – review & editing. RT: Conceptualization, Funding acquisition, Investigation, Project administration, Writing – original draft, Writing – review & editing.
The author(s) declare that financial support was received for the research, authorship, and/or publication of this article. The personnel on this manuscript were supported by grants from the NSF including IOS 1846520 and DEB 2012041 to RT, DBI 2208928 to MW, and IOS 2240950 to EB and RT.
We thank the special issue editors for encouraging our submission and two reviewers and D.T. for their careful consideration of our manuscript. We acknowledge that the University of Denver where this work was conducted is located on unceded territories of Indigenous peoples.
The authors declare that the research was conducted in the absence of any commercial or financial relationships that could be construed as a potential conflict of interest.
All claims expressed in this article are solely those of the authors and do not necessarily represent those of their affiliated organizations, or those of the publisher, the editors and the reviewers. Any product that may be evaluated in this article, or claim that may be made by its manufacturer, is not guaranteed or endorsed by the publisher.
Ah-King M. (2022). “The history of sexual selection research provides insights as to why females are still understudied.” Nature Communications 13.1, 6976.
Alonso J. C., Abril-Colón I., Palacín C. (2021). Moonlight triggers nocturnal display in a diurnal bird. Anim. Behav. 171, 87–98. doi: 10.1016/j.anbehav.2020.11.011
Ancillotto L., Labadessa R. (2023). A noisy neighbour: acoustic competition drives changes in song traits and spatiotemporal behaviour of orthopterans. Anim. Behav. 201, 117–124. doi: 10.1016/j.anbehav.2023.05.007
Arroyo-Solís A., Castillo J. M., Figueroa E., López-Sánchez J., Slabbekoorn H. (2013). Experimental evidence for an impact of anthropogenic noise on dawn chorus timing in urban birds. J. Avian 44.3, 288–296. doi: 10.1111/j.1600-048X.2012.05796.x
Aschoff JüCheckt. a. e. (1951). Die 24-stunden-periodik der maus unter konstanten umgebungsbedingungen. Die Naturwissenschaften 38, 506–507. doi: 10.1007/BF00628863
Aubry L. M., Williams C. T. (2022). Vertebrate phenological plasticity: from molecular mechanisms to ecological and evolutionary implications. Integr. Comp. Biol. 62, 958–715. doi: 10.1093/icb/icac121
Baker B. J., Richardson J. M. L. (2006). The effect of artificial light on male breeding-season behaviour in green frogs, rana clamitans melanota. Can. J. Zool. 84, 1528–1532. doi: 10.1139/z06-142
Baker J. R. (1938). The evolution of breeding seasons. Evolution: Essays Aspects Evolutionary Biol., 161–177.
Barelli C., Mundry R., Heistermann M., Hammerschmidt K. (2013). Cues to androgens and quality in male gibbon songs. PloS One 8, e827485. doi: 10.1371/journal.pone.0082748
Barnwell F. H. (1966). Daily and tidal patterns of activity in individual fiddler crab (genus UCA) from the woods hole region. Biol. Bull. 130, 1–17. doi: 10.2307/1539948
Bloch G., Robinson G. E. (2001). Chronobiology. Reversal of honeybee behavioural rhythms. Nature 410, 1048. doi: 10.1038/35073661
Bonduriansky R., Maklakov A., Zajitschek F., Brooks R. (2008). Sexual selection, sexual conflict and the evolution of ageing and life span. Funct. Ecol. 22, 443–453. doi: 10.1111/j.1365-2435.2008.01417.x
Bonnet Timothée, Morrissey M. B., Morris A., Morris S., Clutton-Brock T. H., Pemberton J. M., et al. (2019). The role of selection and evolution in changing parturition date in a red deer population. PloS Biol. 17, e30004935. doi: 10.1371/journal.pbio.3000493
Bridges A. S., Dorcas M. E., Montgomery W. L. (2000). Temporal variation in anuran calling behavior: implications for surveys and monitoring programs. Copeia 2000, 587–592. doi: 10.1643/0045-8511(2000)000[0587:TVIACB]2.0.CO;2
Bronson F. H. (1988). Mammalian reproductive strategies: genes, photoperiod and latitude. Reproduction Nutrition Dev. 28, 335–347. doi: 10.1051/rnd:19880301
Bruce V. G., Pittendrigh C. S. (1957). Endogenous rhythms in insects and microorganisms. Am. Nat. 91, 179–955. doi: 10.1086/281977
Bulla M., Valcu M., Dokter A. M., Dondua A. G., Kosztolányi András, Rutten A. L., et al. (2016). Unexpected diversity in socially synchronized rhythms of shorebirds. Nature 540, 109–113. doi: 10.1038/nature20563
Cade W. (1975). Acoustically orienting parasitoids: fly phonotaxis to cricket song. Sci. (New York N.Y.) 190, 1312–1313. doi: 10.1126/science.190.4221.1312
Caillaud D., Levréro F., Gatti S., Ménard N., Raymond M. (2008). Influence of male morphology on male mating status and behavior during interunit encounters in western lowland gorillas. Am. J. Phys. Anthropol. 135, 379–885. doi: 10.1002/ajpa.v135:4
Chen Z., Odstrcil E. A., Tu B. P., McKnight S. L. (2007). Restriction of DNA replication to the reductive phase of the metabolic cycle protects genome integrity. Science 316, 1916–1919. doi: 10.1126/science.1140958
Cheyne S. M. (2008). Effects of meteorology, astronomical variables, location and human disturbance on the singing apes: hylobates albibarbis. Am. J. Primatol. 70, 386–392. doi: 10.1002/ajp.20502
Cheyne S. M., Thompson C. J.H., Phillips A. C., Hill R. M.C., Limin S. H. (2008). Density and population estimate of gibbons (Hylobates albibarbis) in the sabangau catchment, central Kalimantan, Indonesia. Primates; J. Primatol. 49, 50–565. doi: 10.1007/s10329-007-0063-0
Chivers D. J. (1976). Communication within and between family groups of siamang (Symphalangus syndactylus). Behaviour 57, 116–135. doi: 10.1163/156853976X00136
Clink D. J., Ahmad A. H., Klinck H. (2020). Gibbons aren’t singing in the rain: presence and amount of rainfall influences ape calling behavior in Sabah, Malaysia. Sci. Rep. 10, 12825. doi: 10.1038/s41598-020-57976-x
Clutton-Brock T. H. (1985). “Size, Sexual Dimorphism, and Polygyny in Primates,” in Size and Scaling in Primate Biology. Ed. Jungers W. L. (Springer US, Boston, MA), 51–60.
Cornwallis C. K., Uller T. (2010). Towards an evolutionary ecology of sexual traits. Trends Ecol. Evol. 25, 145–525. doi: 10.1016/j.tree.2009.09.008
Cowlishaw G. (1996). Sexual selection and information content in gibbon song bouts. Ethol.: Formerly Z. Fur Tierpsychologie 102, 272–284.
Craig A. (1989). Tail length and sexual selection in the polygynous longtailed widow(Euplectes progne): A cautionary tale. S. AFR. J. SCI./S.-AFR. TYDSKR. WET 85, 523–524.
Dalziell A. H., Cockburn A. (2008). Dawn song in superb fairy-wrens: A bird that seeks extrapair copulations during the dawn chorus. Anim. Behav. 75, 489–5005. doi: 10.1016/j.anbehav.2007.05.014
Davidson A. J., Menaker M. (2003). Birds of a feather clock together – sometimes: social synchronization of circadian rhythms. Curr. Opin. Neurobiol. 13, 765–695. doi: 10.1016/j.conb.2003.10.011
Dawson A., King V. M., Bentley G. E., Ball G. F. (2001). Photoperiodic control of seasonality in birds. J. Biol. Rhythms 16, 365–380. doi: 10.1177/074873001129002079
Dunlap J. C. (1999). Molecular bases for circadian clocks. Cell 96, 271–290. doi: 10.1016/S0092-8674(00)80566-8
Dunlap J. C., Loros J. J., DeCoursey P. J. (2004). Chronobiology: Biological Timekeeping (Oxford University Press, Incorporated).
Emlen D. J. (2008). The evolution of animal weapons. Annu. Rev. Ecol. Evolution Systematics 39, 387–413. doi: 10.1146/annurev.ecolsys.39.110707.173502
Endler J. A. (1980). NATURAL SELECTION ON COLOR PATTERNS IN POECILIA RETICULATA. Evolution; Int. J. Organic Evol. 34, 76–91. doi: 10.2307/2408316
Endler J. A. (1982). CONVERGENT AND DIVERGENT EFFECTS OF NATURAL SELECTION ON COLOR PATTERNS IN TWO FISH FAUNAS. Evolution; Int. J. Organic Evol. 36, 178–188. doi: 10.2307/2407979
Endler J. A. (1993). The color of light in forests and its implications. Ecol. Monogr. 63, 1–27. doi: 10.2307/2937121
Evans M., Hatchwell B. J. (1992). An experimental study of male adornment in the scarlet-tufted malachite sunbird: II. The role of the elongated tail in mate choice and experimental evidence for a handicap. Behav. Ecol. Sociobiol. 29, 421–427. doi: 10.1007/bf00170172
Favreau Angélique, Richard-Yris M.-A., Bertin A., Houdelier Cécilia, Lumineau S. (2009). Social influences on circadian behavioural rhythms in vertebrates. Anim. Behav. 77, 983–895. doi: 10.1016/j.anbehav.2009.01.004
Ferguson C. T.J., O’Neill T. L., Audsley N., Isaac R.E. (2015). The sexually dimorphic behaviour of adult drosophila suzukii: elevated female locomotor activity and loss of siesta is a post-mating response. J. Exp. Biol. 218, 3855–3861. doi: 10.1242/jeb.128951
Froy H., Martin J., Stopher K. V., Morris A., Morris S., Clutton-Brock T. H., et al. (2019). Consistent within-individual plasticity is sufficient to explain temperature responses in red deer reproductive traits. J. Evolutionary Biol. 32, 1194–12065. doi: 10.1111/jeb.v32.11
Gamble F. W., Keeble F. (1903). The bionomics of convoluta roscoffensis, with special reference to its green cells. Proc. R. Soc. London 72, 93–98. doi: 10.1242/jcs.s2-47.187.363
Gammon D. E., Baker M. C. (2004). Song repertoire evolution and acoustic divergence in a population of black-capped chickadees, poecile atricapillus. Anim. Behav. 68, 903–135. doi: 10.1016/j.anbehav.2003.10.030
Getty T. (2006). Sexually selected signals are not similar to sports handicaps. Trends Ecol. Evol. 21, 83–88. doi: 10.1016/j.tree.2005.10.016
Ghalambor C. K., Reznick D. N., Walker J. A. (2004). Constraints on adaptive evolution: the functional trade-off between reproduction and fast-start swimming performance in the trinidadian guppy (Poecilia reticulata). Am. Nat. 164, 38–505. doi: 10.1086/421412
Ghalambor C. K., Walker J. A., Reznick D. N. (2003). Multi-trait selection, adaptation, and constraints on the evolution of burst swimming performance. Integr. Comp. Biol. 43, 431–438. doi: 10.1093/icb/43.3.431
Gil D., Llusia D. (2020). “The Bird Dawn Chorus Revisited,” in Coding Strategies in Vertebrate Acoustic Communication, edited by Thierry Aubin and Nicolas Mathevon (Springer International Publishing, Cham), 45–90.
Grafe T. U., Thein J. (2001). Energetics of calling and metabolic substrate use during prolonged exercise in the European treefrog hyla arborea. J. Comp. Physiol. B Biochemical Systemic Environ. Physiol. 171, 69–76. doi: 10.1007/s003600100185
Grafen A. (1990). Biological signals as handicaps. J. Theor. Biol. 144, 517–546. doi: 10.1016/S0022-5193(05)80088-8
Greives T. J., Kingma S. A., Kranstauber B., Mortega K., Wikelski M., van Oers K., et al. (2015). Costs of sleeping in: circadian rhythms influence cuckoldry risk in a songbird. Funct. Ecol. 29, 1300–13075. doi: 10.1111/fec.2015.29.issue-10
Guevara-Fiore P., Stapley J., Watt P. J. (2010). Mating effort and female receptivity: how do male guppies decide when to invest in sex? Behav. Ecol. Sociobiol. 64, 1665–1725. doi: 10.1007/s00265-010-0980-6
Gwinner E. (1981). Circannual rhythms: their dependence on the circadian system. Biol. Clocks Seasonal Reprod. Cycles, 153–169.
Gwinner E. (1986). “Evidence for Circannual Rhythms,” in Circannual Rhythms: Endogenous Annual Clocks in the Organization of Seasonal Processes. Ed. Gwinner E. (Springer Berlin Heidelberg, Berlin, Heidelberg), 11–38.
Gwinner E. (2012). Circannual Rhythms: Endogenous Annual Clocks in the Organization of Seasonal Processes (Germany: Springer Science & Business Media).
Hau M., Dominoni D., Casagrande S., Buck C.L., Wagner G., Hazlerigg D., et al. (2017). Timing as a sexually selected trait: the right mate at the right moment. Philos. Trans. R. Soc. London Ser. B Biol. Sci. 372, 20160249. doi: 10.1098/rstb.2016.0249
Hawkins G. L., Hill G. E., Mercadante A. (2012). Delayed plumage maturation and delayed reproductive investment in birds. Biol. Rev. Cambridge Philos. Soc. 87, 257–745. doi: 10.1111/j.1469-185X.2011.00193.x
Heinen-Kay J. L., Nichols R. E., Zuk M. (2020). Sexual signal loss, pleiotropy, and maintenance of a male reproductive polymorphism in crickets. Evolution; Int. J. Organic Evol. 74, 1002–1095. doi: 10.1111/evo.13952
Henwood K., Fabrick A. (1979). A quantitative analysis of the dawn chorus: temporal selection for communicatory optimization. Am. Nat. 114, 260–745. doi: 10.1086/283473
Hill P. S.M. (2009). How do animals use substrate-borne vibrations as an information source? Die Naturwissenschaften 96, 1355–1371. doi: 10.1007/s00114-009-0588-8
Hill G. E. (2011). Condition-dependent traits as signals of the functionality of vital cellular processes: condition is more than nutrition. Ecol. Lett. 14, 625–634. doi: 10.1111/j.1461-0248.2011.01622.x
Höhn E. O. (1960). Seasonal changes in the mallard’s penis and their hormonal control. Proc. Zoological Soc. London 134, 547–555.
Husak J. F., Fox S. F. (2006). Field use of maximal sprint speed by collared lizards (Crotaphytus collaris): compensation and sexual selection. Evolution; Int. J. Organic Evol. 60, 1888–1955. doi: 10.1111/j.0014-3820.2006.tb01230.x
Husak J. F., Ribak G., Wilkinson G. S., Swallow J. G. (2011). Compensation for exaggerated eye stalks in stalk-eyed flies (Diopsidae). Funct. Ecol. 25, 608–165. doi: 10.1111/j.1365-2435.2010.01827.x
Husak J. F., Sorlin M. V., Lailvaux S. P. (2024). Counting the costs of expensive tissues: mating system, brain size, and IGF-1 affect the ecological costs of transport in mammals. Front. Ethol. 3. doi: 10.3389/fetho.2024.1464308
Hutchinson J. M.C., McNamara J. M., Cuthill I. C. (1993). Song, sexual selection, starvation and strategic handicaps. Anim. Behav. 45, 1153–1775. doi: 10.1006/anbe.1993.1139
Jabbur M. Luísa, Dani C., Spoelstra K., Dodd A. N., Johnson C. H. (2024). Evaluating the adaptive fitness of circadian clocks and their evolution. J. Biol. Rhythms 39, 115–345. doi: 10.1177/07487304231219206
Kacelnik A. (1979). The foraging efficiency of great tits (Parus major L.) in relation to light intensity. Anim. Behav. 27, 237–241. doi: 10.1016/0003-3472(79)90143-X
Kacelnik A., Krebs J. R. (1983). The dawn chorus in the great tit (Parus major): proximate and ultimate causes. Behaviour 83, 287–308. doi: 10.1163/156853983X00200
Kaiser T. S., Poehn B., Szkiba D., Preussner M., Sedlazeck F. J., Zrim A., et al. (2016). The genomic basis of circadian and circalunar timing adaptations in a midge. Nature 540, 69–73.
Keerthipriya P., Nandini S., Gautam H., Revathe T., Vidya T. N. C. (2019). Musth and its effects on male–male and male–female associations in Asian elephants. J. Mammal. 101, 259–705. doi: 10.1093/jmammal/gyz032
Kempenaers B., Borgström P., Loës P., Schlicht E., Valcu M. (2010). Artificial night lighting affects dawn song, extra-pair siring success, and lay date in songbirds. Curr. Biol.: CB 20, 1735–1395. doi: 10.1016/j.cub.2010.08.028
Kodric-Brown A. (2015). Sexual dichromatism and temporary color changes in the reproduction of fishes1. Integr. Comp. Biol. 38, 70–81.
Kotiaho J. S., Alatalo R. V., Mappes J., Nielsen M. G., Parri S., Rivero A. (1998). Energetic costs of size and sexual signalling in a wolf spider. Proc. R. Soc. London Ser. B: Biol. Sci. 265, 2203–2209. doi: 10.1098/rspb.1998.0560
Kreitzman L., Foster R. (2010). Seasons of Life: The Biological Rhythms That Enable Living Things to Thrive and Survive (Great Britain: Profile Books).
Liker András, Székely Tamás (2005). Mortality costs of sexual selection and parental care in natural populations of birds. Evolution; Int. J. Organic Evol. 59, 890–975. doi: 10.1111/j.0014-3820.2005.tb01750.x
Lin C.-H., Takahashi S., Mulla A. J., Nozawa Y. (2021). Moonrise timing is key for synchronized spawning in coral dipsastraea speciosa. Proc. Natl. Acad. Sci. United States America 118. doi: 10.1073/pnas.2101985118
Mace R. (1987). The dawn chorus in the great tit paras major is directly related to female fertility. Nature 330, 745–746. doi: 10.1038/330745a0
McCullough E. L., Emlen D. J. (2013). Evaluating the costs of a sexually selected weapon: big horns at a small price. Anim. Behav. 86, 977–855. doi: 10.1016/j.anbehav.2013.08.017
McCullough E. L., Miller C. W., Emlen D. J. (2016). Why sexually selected weapons are not ornaments. Trends Ecol. Evol. 31, 742–515. doi: 10.1016/j.tree.2016.07.004
McKeever S. (1977). Observations of corethrella feeding on tree frogs (Hyla). Mosquito News 37, 522–523. doi: 10.5555/19770549843
Mitani J. C. (1985). Location-specific responses of gibbons (Hylobates muelleri) to male songs. Z. Fur Tierpsychologie 70, 219–224. doi: 10.1111/j.1439-0310.1985.tb00513.x
Møller A. P. (1996). The cost of secondary sexual characters and the evolution of cost-reducing traits. Ibis 138, 112–119. doi: 10.1111/j.1474-919X.1996.tb04401.x
Møller A. P., Barbosa A., Cuervo J. J., de Lope F., Merino S., Saino N. (1998). Sexual selection and tail streamers in the barn swallow. Proc. R. Soc. London Ser. B: Biol. Sci. 265, 409–414. doi: 10.1098/rspb.1998.0309
Montgomerie R., Lyon B., Holder K. (2001). Dirty ptarmigan: behavioral modification of conspicuous male plumage. Behav. Ecol.: Off. J. Int. Soc. Behav. Ecol. 12, 429–385. doi: 10.1093/beheco/12.4.429
Mougi A. (2020). Diversity of Functional Traits and Interactions: Perspectives on Community Dynamics (Singapore: Springer Nature).
Murphy C. G. (1999). Nightly timing of chorusing by male barking treefrogs (Hyla gratiosa): the influence of female arrival and energy. Copeia 1999, 333–347. doi: 10.2307/1447479
Murphy D., Mumby H. S., Henley M. D. (2019). Age differences in the temporal stability of a male African elephant (Loxodonta africana) social network. Behav. Ecol.: Off. J. Int. Soc. Behav. Ecol. 31, 21–315. doi: 10.1093/beheco/arz152
Nekaris K. A. I., Campera M., Nijman V., Birot Hélène, Rode-Margono E. J., Fry B. G., et al. (2020). Slow lorises use venom as a weapon in intraspecific competition. Curr. Biol.: CB 30, R1252–R1253. doi: 10.1016/j.cub.2020.09.034
Nelson R. J., DeVries A.C., Prendergast B. J. (2024). Researchers need to better address time-of-day as a critical biological variable. Proc. Natl. Acad. Sci. United States America 121, e23169591215. doi: 10.1073/pnas.2316959121
Nikaido S. S., Johnson C. H. (2000). Daily and circadian variation in survival from ultraviolet radiation in chlamydomonas reinhardtii. Photochem. Photobiol. 71, 758–765. doi: 10.1562/0031-8655(2000)071<0758:DACVIS>2.0.CO;2
Numata H., Helm B. (2015). Annual, Lunar, and Tidal Clocks: Patterns and Mechanisms of Nature’s Enigmatic Rhythms (Japan: Springer).
Oufiero C. E., Garland T. (2007). Evaluating performance costs of sexually selected traits. Funct. Ecol. 21, 676–689. doi: 10.1111/j.1365-2435.2007.01259.x
Penn D. J., Számadó S. (2020). The handicap principle: how an erroneous hypothesis became a scientific principle. Biol. Rev. Cambridge Philos. Soc. 95, 267–905. doi: 10.1111/brv.12563
Peters A., Kingma S. A., Delhey K. (2013). Seasonal male plumage as a multi-component sexual signal: insights and opportunities. Emu - Austral Ornithol. 113, 232–475. doi: 10.1071/MU12083
Poole J. H. (1987). Rutting behavior in african elephants: the phenomenon of musth. Behaviour 102, 283–316. doi: 10.1163/156853986X00171
Pope D. S. (2000). Testing function of fiddler crab claw waving by manipulating social context. Behav. Ecol. Sociobiol. 47, 432–437. doi: 10.1007/s002650050687
Renaud L.-A., Pigeon G., Festa-Bianchet M., Pelletier F. (2019). Phenotypic plasticity in bighorn sheep reproductive phenology: from individual to population. Behav. Ecol. Sociobiol. 73. doi: 10.1007/s00265-019-2656-1
Rico-Guevara A., Hurme K. J. (2019). Intrasexually selected weapons. Biol. Rev. Cambridge Philos. Soc. 94, 60–1015. doi: 10.1111/brv.2019.94.issue-1
Robertson D.R., Petersen C. W., Brawn J. D. (1990). Lunar reproductive cycles of benthic-brooding reef fishes: reflections of larval biology or adult biology? Ecol. Monogr. 60, 311–295. doi: 10.2307/1943060
Rock A., Wilcockson D., Last K. S. (2022). Towards an understanding of circatidal clocks. Front. Physiol. 13, 830107. doi: 10.3389/fphys.2022.830107
Roth T., Sprau P., Schmidt R., Naguib M., Amrhein V. (2009). Sex-specific timing of mate searching and territory prospecting in the nightingale: nocturnal life of females. Proc. Biol. Sciences/The R. Soc. 276, 2045–2050. doi: 10.1098/rspb.2008.1726
Ryan M. J., Tuttle M. D., Taft L. K. (1981). The costs and benefits of frog chorusing behavior. Behav. Ecol. Sociobiol. 8, 273–785. doi: 10.1007/BF00299526
Sakaluk S. K., Belwood J. J. (1984). Gecko phonotaxis to cricket calling song: A case of satellite predation. Anim. Behav. 32, 659–625. doi: 10.1016/S0003-3472(84)80141-4
Satoh A. (2017). Constant light disrupts the circadian but not the circatidal rhythm in mangrove crickets. Biol. Rhythm. Res. 48, 459–463. doi: 10.1080/09291016.2016.1275392
Setchell J. M., Dixson A. F. (2001). Circannual changes in the secondary sexual adornments of semifree-ranging male and female mandrills (Mandrillus sphinx). Am. J. Primatol. 53, 109–121. doi: 10.1002/1098-2345(200103)53:3<109::AID-AJP2>3.0.CO;2-I
Sharma V. K. (2003). Adaptive significance of circadian clocks. Chronobiol. Int. 20, 901–919. doi: 10.1081/CBI-120026099
Shima J. S., Osenberg C. W., Alonzo S. H., Noonburg E. G., Mitterwallner P., Swearer S. E. (2020). Reproductive phenology across the lunar cycle: parental decisions, offspring responses, and consequences for reef fish. Ecology 101, e030865. doi: 10.1002/ecy.v101.8
Smith M. J., Harper D. G. C. (1995). Animal signals: models and terminology. J. Theor. Biol. 177, 305–115. doi: 10.1006/jtbi.1995.0248
Somers J., Georgiades M., Su M. P., Bagi J., Andrés M., Alampounti A., et al. (2022). Hitting the right note at the right time: circadian control of audibility in anopheles mosquito mating swarms is mediated by flight tones. Sci. Adv. 8, eabl4844. doi: 10.1126/sciadv.abl4844
Staicer C. A., Spector D. A., Horn A. G. (2019). “24. The Dawn Chorus and Other Diel Patterns in Acoustic Signaling,” in Ecology and Evolution of Acoustic Communication in Birds (USA: Cornell University Press), 426–453.
Studd M. V., Robertson R. J. (1985). Life span, competition, and delayed plumage maturation in male passerines: the breeding threshold hypothesis. Am. Nat. 126, 101–155. doi: 10.1086/284399
Swallow J., Husak J. (2011). Compensatory traits and the evolution of male ornaments. Behaviour 148, 1–295. doi: 10.1163/000579510X541265
Takemura A., Rahman Md S., Nakamura S., Park Y. Ju, Takano K. (2004). Lunar cycles and reproductive activity in reef fishes with particular attention to rabbitfishes. Fish Fisheries 5, 317–285. doi: 10.1111/j.1467-2679.2004.00164.x
Takemura A., Rahman M. S., Park Y. J. (2010). External and internal controls of lunar-related reproductive rhythms in fishes. J. Fish Biol. 76, 7–26. doi: 10.1111/j.1095-8649.2009.02481.x
Tinbergen N., van Iersel J. J. A. (1947). Displacement reactions’ in the three-spined stickleback. Behaviour 1, 56–63.
Tomkins J. L., Radwan J., Kotiaho J. S., Tregenza T. (2004). Genic capture and resolving the lek paradox. Trends Ecol. Evol. 19, 323–285. doi: 10.1016/j.tree.2004.03.029
Tuttle M. D., Ryan M. J. (1981). Bat predation and the evolution of frog vocalizations in the neotropics. Sci. (New York N.Y.) 214, 677–678. doi: 10.1126/science.214.4521.677
Tyler N. J. C., Post E., Hazlerigg D. G. (2024). Weak coupling between energetic status and the timing of reproduction in an arctic ungulate. Sci. Rep. 14, 6352. doi: 10.1038/s41598-024-56550-z
Vitaterna M. H., Takahashi J. S., Turek F. W. (2001). Overview of circadian rhythms. Alcohol Res. Health 25, 85–93. doi: 10.1016/S1530-0277(01)00036-7
Vors L. S., Boyce M. S. (2009). Global declines of caribou and reindeer. Global Change Biol. 15, 2626–2335. doi: 10.1111/j.1365-2486.2009.01974.x
Wang Y., Dong H., Qu Y., Zhou Y., Qin J., Li K., et al. (2024). Circabidian rhythm of sex pheromone reception in a scarab beetle. Curr. Biol.: CB 34, 568–78.e5. doi: 10.1016/j.cub.2023.12.057
Westwood M. L., Geissmann Q., O’Donnell A. J., Rayner J., Schneider W., Zuk M., et al. (2024). Machine learning reveals singing rhythms of male pacific field crickets are clock controlled. Behav. Ecol. 35, arad098. doi: 10.1093/beheco/arad098
Westwood M. L., O’Donnell A. J., de Bekker C., Lively C. M., Zuk M., Reece S. E. (2019). The evolutionary ecology of circadian rhythms in infection. Nat. Ecol. Evol. 3, 552–605. doi: 10.1038/s41559-019-0831-4
Wickler W. (2010). Vocal dueting and the pair bond. Z. Fur Tierpsychologie 52, 201–209. doi: 10.1111/j.1439-0310.1980.tb00711.x
Zigler A., Straw S., Tokuda I., Bronson E., Riede T. (2023). Critical calls: circadian and seasonal periodicity in vocal activity in a breeding colony of Panamanian golden frogs (Atelopus zeteki). PloS One 18, e02865825. doi: 10.1371/journal.pone.0286582
Zuk M., Kolluru G. R. (1998). Exploitation of sexual signals by predators and parasitoids. Q. Rev. Biol. 73, 415–385. doi: 10.1086/420412
Zuk M., Rotenberry J. T., Tinghitella R. M. (2006). Silent night: adaptive disappearance of a sexual signal in a parasitized population of field crickets. Biol. Lett. 2, 521 LP–524. doi: 10.1098/rsbl.2006.0539
Keywords: circadian rhythms, circannual rhythms, circalunar rhythms, circatidal rhythms, compensatory trait, signal modality, ornament, weapon
Citation: Westwood ML, Broder ED, Welsh GT and Tinghitella RM (2025) Chronobiology as compensation: can biological rhythms compensate for sexual signals? Front. Ethol. 3:1473358. doi: 10.3389/fetho.2024.1473358
Received: 30 July 2024; Accepted: 06 December 2024;
Published: 07 January 2025.
Edited by:
Simon Lailvaux, University of New Orleans, United StatesReviewed by:
Szabolcs Számadó, Budapest University of Technology and Economics, HungaryCopyright © 2025 Westwood, Broder, Welsh and Tinghitella. This is an open-access article distributed under the terms of the Creative Commons Attribution License (CC BY). The use, distribution or reproduction in other forums is permitted, provided the original author(s) and the copyright owner(s) are credited and that the original publication in this journal is cited, in accordance with accepted academic practice. No use, distribution or reproduction is permitted which does not comply with these terms.
*Correspondence: Mary L. Westwood, bWFyeXdlc3R3b29kMUBnbWFpbC5jb20=
Disclaimer: All claims expressed in this article are solely those of the authors and do not necessarily represent those of their affiliated organizations, or those of the publisher, the editors and the reviewers. Any product that may be evaluated in this article or claim that may be made by its manufacturer is not guaranteed or endorsed by the publisher.
Research integrity at Frontiers
Learn more about the work of our research integrity team to safeguard the quality of each article we publish.