- 1Department of Biology, University of Maryland, College Park, College Park, MD, United States
- 2Department of Biology, The State University of New York College at Geneseo, Geneseo, NY, United States
Exaggerated ornaments provide opportunities to understand how selection can operate at different levels to shape the evolution of a trait. While these features aid their bearer in attracting mates or fending off competitors, they can also be costly and influenced by the environment and genetic variation. The eyestalks of the stalk-eyed fly, Teleopsis dalmanni, are of interest because eyestalk length is the target of both intra- and intersexual selection and is also reduced by loci on a highly-divergent sex ratio X chromosome (XSR), a meiotic driver accounting for up to 30% of wild X chromosomes. Male stalk-eyed flies fight to control access to females and over food using a combination of low-intensity displays and high-intensity physical fights. We staged, filmed, and scored contests between pairs of eyespan-matched males to evaluate whether X chromosome type impacts the behavior and outcome of aggressive interactions. While our results broadly match expectations from previous studies, we found that XSR males used more high-intensity behaviors than males carrying a non-driving, standard X chromosome (XST), particularly when their eyestalks were of similar size or smaller than their opponents. Additionally, we found that when XSR males use high-intensity behaviors, they win more bouts than when they use low-intensity behaviors. Taken together, these results suggest that XSR impacts male aggressive behavior to compensate for the shorter eyestalks of XSR males and may help to explain how this selfish chromosome is maintained.
1 Introduction
Theoretical models explaining the evolution of sexually dimorphic traits by either inter- or intrasexual selection are legion (Maynard Smith, 1991; Andersson, 1994; Kokko et al., 2006), but often ignore the consequences of sex-linked inheritance of ornaments or weapons (but see Hastings, 1994; Reeve and Pfennig, 2003; Kirkpatrick and Hall, 2004). Sex linkage can influence how sexual selection operates, especially if there is a mechanism for the sex ratio to vary, such as meiotic drive. Meiotic drivers are alleles that are overrepresented in an individual’s gametes, causing deviations from Mendelian expectations. When a driver is on a sex chromosome and acts in the heterogametic sex, it distorts the sex ratio of the offspring and can either cause extinction or be maintained by balancing selection (reviewed in Jaenike, 2001; Lindholm et al., 2016). Models of sexual selection that include sex-linked inheritance and X chromosome meiotic drive suggest that female choice can evolve in response to the presence of drive (Lande and Wilkinson, 1999; Reinhold et al., 1999)—either females prefer not to mate with males carrying a drive element or prefer males carrying drive suppressors (Lyth et al., 2023).
Stalk-eyed flies (Teleopsis dalmanni) are a model for understanding how X-chromosome meiotic drive can influence both inter- and intrasexual selection. Male carriers of a drive X chromosome have a sex ratio phenotype, i.e., they produce broods that are 90%–100% female (Presgraves et al., 1997), but have reduced eyestalk length (Johns et al., 2005; Cotton et al., 2014) and are less competitive in both pre-and post-mating interactions (Wilkinson et al., 2006; Paczolt et al., 2023, but see Meade et al., 2020; Bates et al., 2023). Females are highly promiscuous (Wilkinson et al., 1998a, 2003) but prefer males with longer eyestalks (Wilkinson et al., 1998a; Hingle et al., 2001). Males also aggressively compete for control of aggregations of females (Wilkinson and Reillo, 1994), and fights are typically won by males with longer eyestalks (Panhuis and Wilkinson, 1999; Egge et al., 2011). Stalk-eyed fly fights are consistent with sequential assessment (Enquist and Leimar, 1983), a mutual assessment model in which individuals assess their opponents and themselves through a series of escalating behaviors until one individual retreats (Egge et al., 2011).
Recent genomic analyses (Paczolt et al., 2017; Reinhardt et al., 2023) have revealed that the T. dalmanni sex ratio X (XSR) diverged from the standard X (XST) hundreds of thousands of years ago. Due to a series of inversions, recombination was greatly reduced, which has led to extensive genetic differentiation between XST and XSR chromosomes, similar to many other drive systems (Lindholm et al., 2016). The X chromosome also represents 20% of the genome (Baker and Wilkinson, 2010; Reinhardt et al., 2023), providing ample material for the evolution of drive-associated differences in eyespan, sperm length (Johns and Wilkinson, 2007), and female sperm storage sizes (Wilkinson, 2005). Furthermore, the weak selection of male X-linked traits (Kirkpatrick and Hall, 2004) and reduced population size and recombination may have led to the accumulation of deleterious alleles on XSR relative to XST (Muller, 1964).
While previous work on sexual selection in T. dalmanni has focused on how XSR affects female mate choice (Wilkinson et al., 1998b; Lande and Wilkinson, 1999; Johns et al., 2005) or sperm competition (Wilkinson et al., 2006; Meade et al., 2020; Bates et al., 2023), the influence of XSR on behaviors involved in male–male competition prior to mating has not yet been examined. In this study, we report the results of two types of trials investigating if T. dalmanni males that carry XSR exhibit differences in aggressive behavior during trials against an opponent of similar size. One set of trials involved pairing outbred flies, which allowed direct comparisons of matches involving either or both XSR and XST individuals with comparable outbred genetic backgrounds. The other set of trials paired an outbred fly with a standardized, inbred opponent, thus allowing the comparison of individual XSR and XST focal males between trials. We hypothesized that XSR male aggressive behavior in size-matched contests may be more intense if they compensate for the disadvantage of their reduced ornament, or conversely, may be less intense if deleterious alleles on XSR negatively impact behaviors.
2 Methods
2.1 Aggression trials
Following previous studies of fighting in stalk-eyed flies (Panhuis and Wilkinson, 1999; Egge et al., 2011; Bubak et al., 2014), we quantified male aggressive behavior in two sets of trials conducted either at SUNY Geneseo (outbred pair trials) or at the University of Maryland, College Park (standardized opponent trials). We conducted trials with outbred and inbred flies as opponents to confirm that the results were robust to the opponents’ genetic background. At both sites, outbred male T. dalmanni were reared from stock populations descended from a collection made at Ulu Gombak, Malaysia, in 2012 (Paczolt et al., 2017) and maintained in population cages with approximately 100 individuals. The temperature was kept at 25°C on a 12-h light:dark cycle in a walk-in chamber, and humidity was held at 70%–90% by automated humidifiers or by twice-weekly manual watering. Flies were fed twice weekly with chopped and autoclaved whole-cob sweet corn treated with 0.1% methylparaben.
Sexually mature males at least 4 weeks post-eclosion were anesthetized using carbon dioxide; their eyespan and body length were measured from video-captured images to the nearest 0.01 mm using ImageJ; and a dot of paint was applied to the ventral thorax before each male was placed in a cage to recover. Because more aggression is displayed between males with similar eyespan (Panhuis and Wilkinson, 1999; Egge et al., 2011), males were paired with partners who differed in eyespan by no more than 5%. Each fly was used in only one trial.
All trials were conducted in transparent plastic arenas (10 cm × 3.5 cm × 6 cm) lined with moist blotting paper and initially divided into three sections by opaque plastic barriers. Each male was placed into an outer compartment of the arena and fasted for 20–24 h. At the beginning of the trial, a piece of agar gel made with corn juice was placed into the middle compartment as a food source and the barriers were removed and the barriers were removed (Supplementary Video 1). Trials were video recorded for 10 min. Scoring of each video was done blind to genotype. The same person scored all videos for each type of trial (outbred pairs—MP; standardized opponents—KP).
The occurrence of aggressive behaviors performed by each fly in each trial was scored from video recordings following a published ethogram (Egge et al., 2011), except for rear-up behavior, which was omitted because it always occurred with another behavior. Within a trial, we defined fighting “bouts” as beginning when either fly performed an aggressive behavior and ending when the flies were more than one body length apart or not facing each other for 3 s without exhibiting an aggressive behavior. A male was scored as losing a bout if he moved away from his opponent at the end of a bout either slowly (away) or quickly (retreat) and his opponent did not. A mutual move away or retreat was considered a tied bout. The majority of the trials involved multiple bouts.
Aggressive behaviors were categorized as either high-intensity (HI) behaviors that involved physical contact (tussle, attack/lunge, and jump attack) or low-intensity (LI) behaviors that involved males mutually displaying their eyestalks in close proximity (approach, flex and extend, and line-up eyestalks). Escalations were tallied for each individual as a transition from an LI to an HI behavior with no other behaviors or the start or end of a bout separating them. Similarly, de-escalations were defined as a transition from an HI to an LI behavior.
Males were collected immediately after each trial for DNA extraction and genotyping for X chromosome type (XSR or XST) using polymerase chain reaction (PCR) to amplify one of two markers (Supplementary Table S1) each of which predicts a drive phenotype in this population with a 95% accuracy (Paczolt et al., 2017; Paczolt et al., 2023).
2.2 Outbred pair trials
In a set of 36 trials, we used eyespan to match outbred males reared from a population in which XSR is segregating or from a family that shared an XSR grandfather from the same population. Mature males were chosen from mixed-sex cages of similar age containing 20–50 flies. We anesthetized 10–20 males, marked each with a colored dot on the thorax using one of five colors of paint pen, and then placed them into temporary holding cages. Each male was then paired with an opponent that had the least difference in eyespan and a different color. Videos were recorded on personal mobile phones, and behaviors were scored using JWatcher, v 0.9 (Blumstein and Daniel, 2007). DNA was extracted using Qiagen DNeasy or Puregene, Qiagen, Germany. PCR was performed using the comp162710 marker, and genotypes were distinguished by 1% agarose gel electrophoresis or, for some samples, by automated fragment analysis and the consensus of the comp162710 and ms-395 markers. Because flies were taken from a population polymorphic for a drive, three match types occurred: XST–XST (N = 19), XSR–XST (N = 13), and XSR–XSR (N = 4). Because XSR–XSR pairings were rare, we contrasted trials that included only XST males with those that included at least one XSR male.
2.3 Standardized opponent trials
In a separate set of 31 trials, focal outbred males competed against standardized inbred opponents. Focal males were lab-reared from the same source used above, while inbred males came from the T. dalmanni “2A” inbred line (Paczolt et al., 2017; Reinhardt et al., 2023), which includes only flies with XST chromosomes. The 2A inbred strain was derived from flies initially collected in 1989 and then used as a control line in a selection experiment (Wilkinson, 1993) prior to being inbred for 20 generations of brother–sister mating. Males were group-housed as virgins until at least 4 weeks post-eclosion. Outbred focal males and inbred opponents were then measured and housed individually in cages (13.5 cm × 12 cm × 13 cm) with food, to control for possible loser effects. Within each pairing, size matches were allowed to vary up to 5% of the focal male’s eyespan, and only the inbred flies were painted on the thorax. Males were kept for a median of 7 days (range: 5–8 days) in individual cages before being placed in an arena with each member of a pair waiting at the same time. Trials were recorded with a digital video camera, and behaviors were scored using Boris v. 8.25 (Friard and Gamba, 2016). DNA was extracted using a squish protocol (Gloor et al., 1993) followed by PCR and fragment analysis of amplicons from both the comp162710 and ms-395 markers (Applied BioSystems 3730xl). There were 11 focal males that carried the XSR chromosome. Differences in the methods used for the two trial types are summarized in Supplementary Table S2.
2.4 Data analysis
Statistical analyses were performed in JMP 17.0.0. Differences between trial type and matched pairs were assessed using Student’s t-tests for continuous variables, the Wilcoxon test for count data, and the Wilcoxon signed-rank test for paired analyses of counts. We used a linear model to evaluate whether the duration of time in which aggressive behavior was observed was influenced by the absolute value of the percent difference in eyespan, the trial type, or their interaction.
To analyze the outbred pair trials, we used generalized linear models (GLMs) assuming a Poisson distribution (log-link function) to determine whether the number of HI behaviors in a trial was influenced by the presence of an XSR male, the absolute value of the percent difference in eyespan, or their interaction. The same analysis was repeated for the number of LI behaviors and escalations. In the subset of XSR–XST trials, we used non-parametric paired tests to test the hypothesis that the XSR individuals performed different numbers of HI, LI, or escalations than their XST opponents.
For the standardized opponent trials, we similarly used GLMs (Poisson distribution, log-link function) to evaluate whether the number of HI or LI behaviors or escalations performed by the focal male in a trial was affected by his X type, the percent difference in eyespan between him and his opponent, or the interaction of these effects. To determine if aggressive behaviors or escalations occurred more often when the difference in eyespan was small, we included a quadratic term for the percent eyespan difference in the model.
In addition, we evaluated the importance of factors influencing the number of bouts won by the focal male using a binomial GLM. Factors in this model included the percent difference in eyespan, the focal male’s X type, the natural log ratio of the focal male’s HI to LI behaviors (focal HI:LI), and all two-way interactions between these effects. To interpret significant interactions, we repeated the analysis separately for XSR and XST males.
3 Results
3.1 Trial-type comparisons
Despite following similar methods, fly pairings were not identical in the two types of trials (Supplementary Table S2). Pairs in the outbred trials were more closely matched for absolute percent difference in eyespan than in the standardized opponent trials (Supplementary Table S3, t = −3.65, P = 0.0006). Body length did not differ between XSR and XST males in either trial type (Supplementary Table S3), but in the outbred trials, the eyespan of XSR males was smaller than that of XST males, whereas, in the standardized opponent trials, the eyespan of XSR and XST males did not differ (Supplementary Table S3). For both trial types, no aggressive behaviors were observed in three trials, so those trials were omitted from further analysis.
As expected from prior work, relative ornament size was an important determinant of fight duration across both types of trials (Figure 1A, L-R χ2 = 15.24, DF = 3, P = 0.0016). Total time spent fighting was greater when pairs of males were more closely matched, as measured by the absolute percent difference in eyespan (L-R χ2 = 7.33, P = 0.0068). Duration did not differ between trial types (L-R χ2 = 1.76, P = 0.18), and there was no detectable interaction between eyespan differences and trial types (L-R χ2 = 0.27, P = 0.6). Moreover, within trials of both types, escalations were more common than de-escalations (Figure 1B, Wilcoxon signed-rank test, Soutbred = −275.5, Poutbred < 0.0001; Sstandardized = −189.00, Pstandardized < 0.0001), and no trial had more de-escalations than escalations.
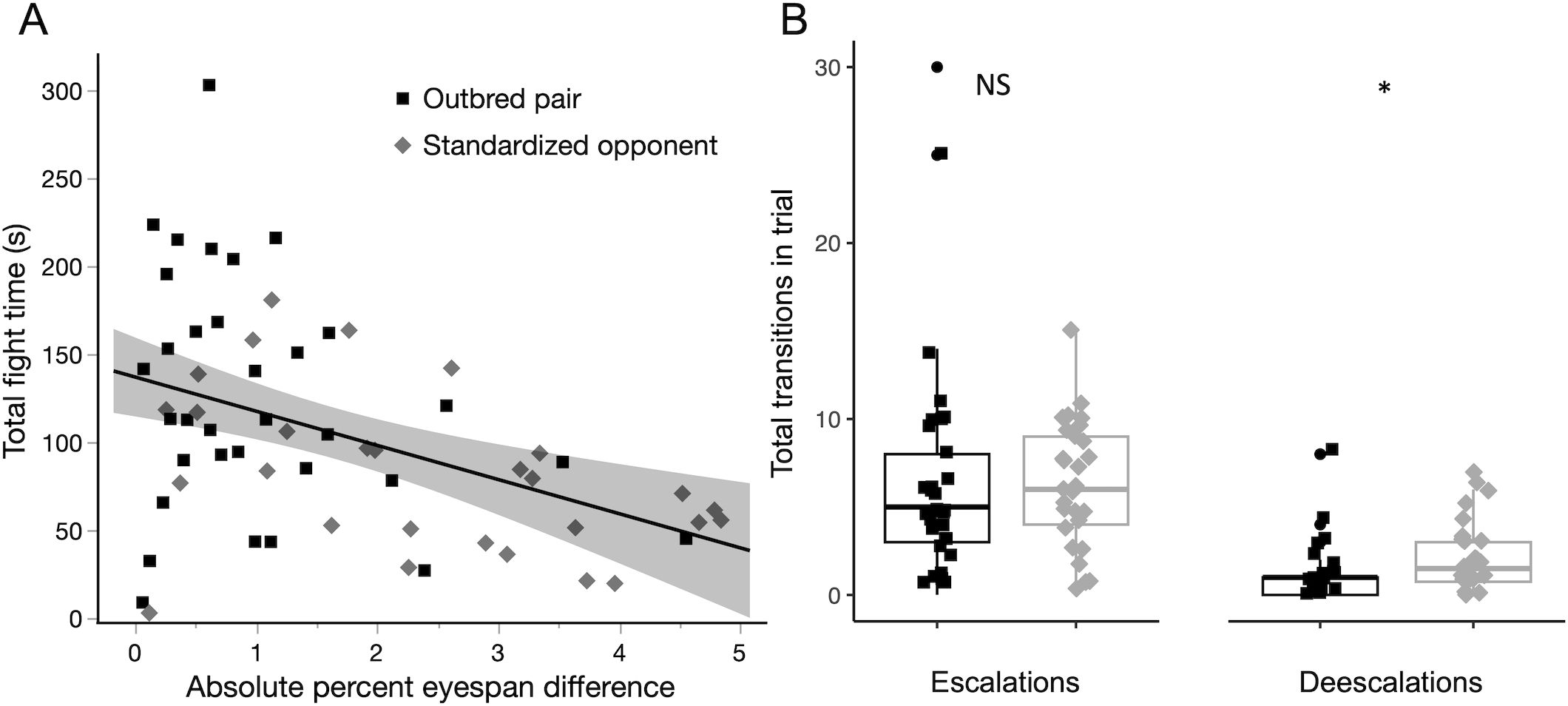
Figure 1. Analysis of both trial types show that, consistent with previous experiments in stalk-eyed flies, (A) flies fight significantly longer when they have a similarly-sized ornament and (B) within a bout of fighting, behaviors tend to escalate from low- to high-intensity behaviors significantly more often than they de-escalate from high- to low-intensity behaviors. There was no significant (NS) difference in the number of escalations between the trial types, but there were fewer de-escalations in the outbred pair trials than in the standardized opponent trails (* indicates P < 0.05).
All behaviors were observed in both types of trials, although not all behaviors were observed in all trials (Supplementary Tables S4, S5). The most common LI behavior was line-up, and the most common HI behavior was attack/lunge. Trial types did not differ in the total number of HI behaviors (Supplementary Figure S1A, Wilcoxon test, W = 371, P = 0.19), LI behaviors (Supplementary Figure S1B, W = 355, P = 0.12), bouts (Supplementary Figure S1C, W = 357.5, P = 0.13), or escalations (Figure 1B, W = 393, P = 0.32), although de-escalations were more common in the standardized opponent trials (Figure 1B, W = 298, P = 0.01).
3.2 Outbred pair trials
A GLM predicting HI behaviors revealed significant effects of the absolute percent difference in eyespan, the presence of an XSR male, and their interaction (Table 1). Specifically, trials including XSR males had more HI behavior overall, but especially when the difference in eyespan between competitors was small (Figure 2A). An identical GLM predicting LI behavior detected a significant effect only of the difference in eyespan, with closer matches having more LI behavior (Figure 2B, Table 1). The number of escalations from LI to HI behaviors exhibited similar patterns to those seen for HI behavior (Figure 2C, Table 1). Within 11 XSR–XST trials, we did not detect a difference in behaviors of paired XSR and XST males (Wilcoxon signed-rank test, Supplementary Table S6).
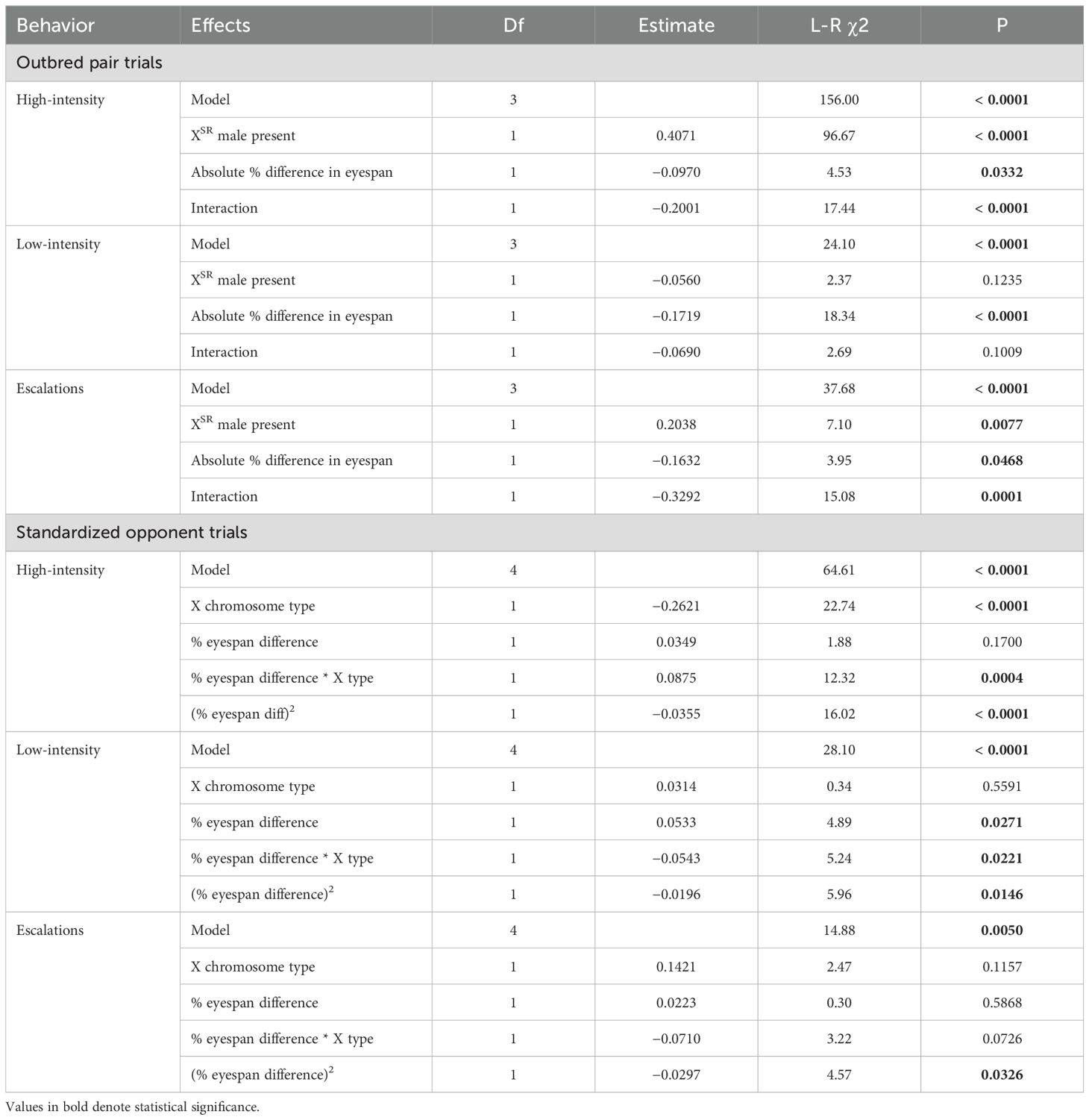
Table 1. Results of Poisson GLMs on the number of behaviors exhibited overall (outbred pair) or by the focal male (standardized opponent trials).
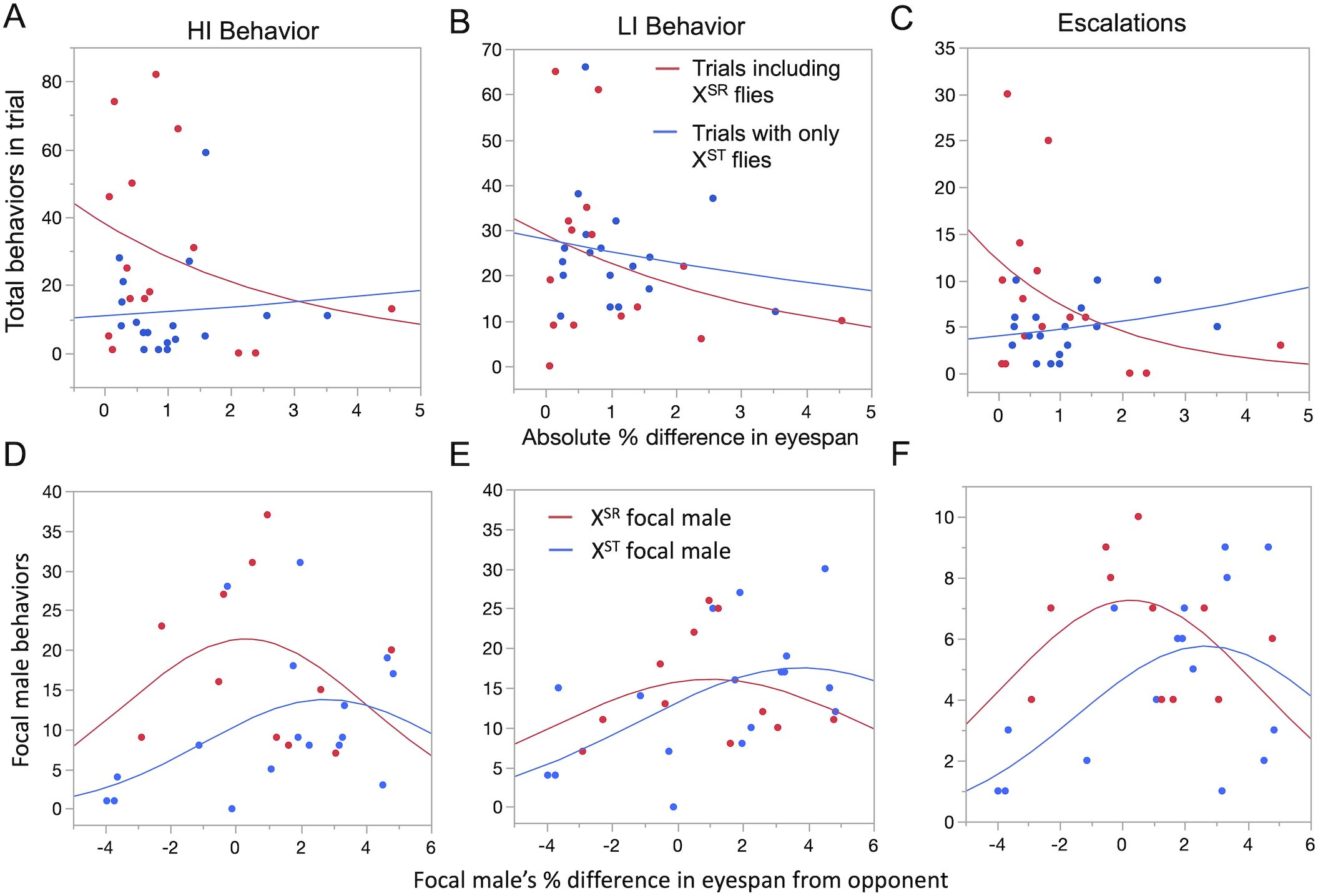
Figure 2. Effect of eyespan match and X chromosome type on high-intensity (HI), low-intensity (LI), and escalation behaviors for each type of trial. In outbred pair trials (A–C), total HI, LI, and escalation behaviors were more common in trials where pairs had similar eyespan, but only HI and escalations were affected by the presence of XSR males. In standardized opponent trials (D–F), HI, LI, and escalation behaviors were similarly highest when the eyespan was closely matched, as fit by a significant quadratic term. XSR focal males exhibited more HI behavior, especially in closely matched contests, but these effects were not observed for LI behaviors or escalations. The GLM results are summarized in Table 1.
3.3 Standardized opponent trials
In trials where outbred males competed against a standardized opponent from an inbred line, focal males exhibited more HI behaviors (Wilcoxon signed rank test, S = −137.0, P = 0.0007, Supplementary Figure S2) and more escalations (Wilcoxon signed rank test, S = −122.0, P = 0.0017) than their opponent, but there was no difference in the number of LI behaviors between focal males and their opponents (Wilcoxon signed rank test, S = 52.5, P = 0.24, Supplementary Figure S2).
GLMs that included the percent difference in eyespan as both a linear and quadratic effect (Table 1) revealed that focal male HI behaviors were more common when the focal male had an XSR chromosome (Figure 2D). A significant quadratic term indicates that HI behavior was also higher when the focal male had an eyespan similar to his opponent. A significant interaction between X type and percent difference in eyespan was also detected; males with the XST chromosome showed more HI behaviors when they had longer eyestalks than their opponent, whereas XSR males did not (Figure 2D). Focal male LI behaviors did not depend on X type but were less common when the focal male had a smaller eyespan than his opponent, as indicated by the significant terms for the effect of eyespan and the additional significant quadratic term (Table 1, Figure 2E). The significant interaction between X chromosome type and percent eyespan difference was due to XST males exhibiting more LI behaviors than XSR males when they were larger than their opponents. Focal males were also more likely to escalate when the eyespan difference was small (Table 1, Figure 2F).
A binomial GLM identified several predictors of focal male fight outcomes (Table 2). Focal males won more bouts if they used relatively more HI behaviors. Although a direct effect of X chromosome type was not detected, there were significant interactions between X type and percent difference in eyespan and between X type and focal HI:LI. We therefore fit separate GLMs for focal males of each type of X chromosome. The results of those models (Table 2) reveal that XST males won more bouts when they were larger than their opponents. The ratio of HI to LI behavior did not directly affect the outcome for XST males, but we did detect a weak effect of the interaction between the percent difference in eyespan and the ratio of HI to LI behaviors. In contrast, XSR males won more bouts when their eyespan was larger than their opponents’ and when they used more HI behaviors than LI behaviors (Table 2; Supplementary Figure S3).
4 Discussion
Head appendages, such as eye stalks, antlers, or bristles, have evolved in many different families of flies (Wilkinson and Dodson, 1997). These structures are typically exaggerated in males and are used in head-to-head confrontations when competing over access to mates or food. In the stalk-eyed fly, T. dalmanni, males with longer eyestalks win contests more often (Panhuis and Wilkinson, 1999; Egge et al., 2011), even when they are matched for body size. However, eyestalk length is influenced by the presence of a sex-ratio drive X chromosome (XSR), which reduces eyespan relative to body length (Wolfenbarger and Wilkinson, 2001; Johns et al., 2005; Cotton et al., 2014), and as a result, is expected to reduce the fighting success of XSR males. However, across two independent experiments, we found that XSR males exhibit more high-intensity behaviors than XST males when they have similar eyestalks as their opponents. Furthermore, we found that engaging in a higher ratio of high- to low-intensity aggressive behavior results in well-matched XSR males winning more bouts in contests against standardized opponents, a behavioral shift that may increase the mating success of XSR males despite their reduced ornament.
There are several plausible interpretations of these findings. One possibility is that the XSR chromosome carries alleles that increase high-intensity aggressive behavior. Previous studies have revealed that HI behaviors are impacted by serotonin (5-hydroxytryptamine or 5-HT), as flies with high 5-HT levels after ectopic administration exhibited more high-intensity behaviors and won more fights (Bubak et al., 2014), and RNAi of 5-HT receptors impacts aggressive behavior in males (Bubak et al., 2019). If a gene for a 5-HT receptor was on the X chromosome, XSR-associated differences in behavior might have evolved—however, all four 5-HT receptor genes (5-HT1B, 5-HT2A, 5-HT2B, and 5-HT7) in the T. dalmanni genome are autosomal (Reinhardt et al., 2023). RNA sequencing of the brains of males carrying each X-chromosome type could help identify unknown XSR-associated gene expressions that might influence aggressive behavior.
The difference could also be due to how XSR and XST males differently assess their own resource-holding potential (RHP) (Parker, 1974) and that of their opponents. RHP is expected to be related to ornament size due to the strong allometry between eyespan and body length (Wilkinson, 1993; Wilkinson and Dodson, 1997). It has been suggested that stalk-eyed flies use their own body size for self-assessment (Brandt and Swallow, 2009), and subsequent work (Egge et al., 2011) suggested that aggressive behavior is most consistent with mutual assessment due to the pattern of behavioral escalation with very little de-escalation, as seen here (Figure 1). Eyespan may be a better target than body length for assessment of the opponent as the allometry exceeds 1.0, so subtle differences in male eyespan can be observed more easily than subtle differences in body size (see Panhuis and Wilkinson, 1999). Eyespan may also be more visible than body size during the line-up behavior, which is often seen early in a bout prior to escalation. Because XSR males have reduced allometry of eyespan to body size, when matched for eyespan, XSR males should have marginally larger body sizes than their opponents, although we did not observe such a difference in these trials (Supplementary Table S3). Larger body size would both increase a male’s self-assessed RHP and allow them to better tolerate the period of fasting prior to fighting. Eyespan-matched XST opponents, meanwhile, would assess XSR males as having similar RHP and thus be willing to escalate to more HI behavior, explaining why both males are more aggressive in trials, including an XSR male (Figure 2). Female stalk-eyed flies also engage in aggressive interactions over food (Bubak et al., 2019), and the XSR chromosome also affects female eyespan, but the magnitude of the effect is approximately 25% that of the male effect (Johns et al., 2005). A test of the effect of XSR on female aggressive behavior would therefore be a useful additional test of these allometry-based hypotheses.
Previous studies have found that HI behavior correlates with the propensity of a fly to win an aggressive contest, but we found complex results (Table 2) in terms of whether the increased HI behavior associated with XSR impacted how likely a fly was to win a bout. Overall, we found no direct effect of X type on the number of bouts won (Supplementary Table S6, Table 2). However, in standardized opponent trials, XSR focal males that performed a higher proportion of high-intensity behaviors to low-intensity behaviors won more bouts. Meanwhile, among XST males, there was only a weak interaction effect between HI:LI behavior and percent difference in eyespan (Supplementary Figure S3).
These results have implications for the ecology and evolution of aggressive behavior in the stalk-eyed fly, as well as the prevalence of XSR in natural populations. Males typically compete for territories on root hairs underneath riverbanks and sometimes perch on the same hair as other males, whereas females approach and land near males with long eyestalks. If XSR males inaccurately self-assess, they may engage with XST males with larger eyestalks, providing proximity to females attracted to his ornament and providing more opportunities for mating. This may be balanced by the survival risks of engaging in more high-intensity behavior. One prediction of this finding would be that populations with a higher frequency of the XSR chromosome should exhibit more intensely aggressive behaviors than those with a lower frequency of XSR. These results may also help to explain how the XSR chromosome is maintained stably in the laboratory and the field (Wilkinson et al., 2003). Experimental evolutionary studies have revealed that in the absence of sexual selection, the XSR chromosome rapidly increases in frequency. In contrast, when females are allowed multiple mating opportunities, the XSR chromosome is maintained at frequencies similar to those found in the wild (Paczolt et al., 2023), indicating that some XSR males gain reproductive success, which may be aided by their willingness to engage in intense fighting behaviors.
Data availability statement
The original contributions presented in the study are publicly available. This data can be found here: https://doi.org/10.5061/dryad.79cnp5j51.
Ethics statement
The manuscript presents research on animals for which ethical approval is not required.
Author contributions
KP: Conceptualization, Data curation, Formal analysis, Funding acquisition, Investigation, Project administration, Supervision, Writing – original draft, Writing – review & editing. MP: Data curation, Formal analysis, Investigation, Methodology, Writing – review & editing. GTW: Data curation, Formal analysis, Writing – review & editing. GSW: Formal analysis, Funding acquisition, Project administration, Resources, Supervision, Visualization, Writing – review & editing. JR: Conceptualization, Data curation, Formal analysis, Funding acquisition, Investigation, Project administration, Supervision, Visualization, Writing – original draft, Writing – review & editing.
Funding
The author(s) declare financial support was received for the research, authorship, and/or publication of this article. Funding for this work was provided by the National Science Foundation (DEB-0952260 to GSW), the National Institute of Health (F32-GM106525 to KP), the Research Foundation for the State University of New York, and the Geneseo Foundation.
Acknowledgments
The authors would like to acknowledge the work of several talented undergraduate students: Ngan Nguyen and Nancy Nguyen conducted the trials for the standardized opponent trials at the University of Maryland. Austin Ainsworth and Mackenzie Hintze assisted in setting up trials and genotyping for the outbred pair trials at SUNY Geneseo.
Conflict of interest
The authors declare that the research was conducted in the absence of any commercial or financial relationship that could be construed as a potential conflict of interest.
Publisher’s note
All claims expressed in this article are solely those of the authors and do not necessarily represent those of their affiliated organizations, or those of the publisher, the editors and the reviewers. Any product that may be evaluated in this article, or claim that may be made by its manufacturer, is not guaranteed or endorsed by the publisher.
Supplementary material
The Supplementary Material for this article can be found online at: https://www.frontiersin.org/articles/10.3389/fetho.2024.1461681/full#supplementary-material
References
Baker R. H., Wilkinson G. S. (2010). Comparative genomic hybridization (CGH) reveals a neo-X chromosome and biased gene movement in stalk-eyed flies (Genus teleopsis). PloS Genet. 6, e1001121. doi: 10.1371/journal.pgen.1001121
Bates S., Meade L., Pomiankowski A. (2023). Meiotic drive does not impede success in sperm competition in the stalk-eyed fly, Teleopsis dalmanni. Evolution 77, 2326–2333. doi: 10.1093/evolut/qpad149
Blumstein D. T., Daniel J. C. (2007). Quantifying behavior the JWatcher way (Sunderland, Mass: Sinauer Associates).
Brandt Y., Swallow J. G. (2009). Do the elongated eye stalks of Diopsid flies facilitate rival assessment? Behav. Ecol. Sociobiol. 63, 1243–1246. doi: 10.1007/s00265-009-0774-x
Bubak A. N., Renner K. J., Swallow J. G. (2014). Heightened serotonin influences contest outcome and enhances expression of high-intensity aggressive behaviors. Behav. Brain Res. 259, 137–142. doi: 10.1016/j.bbr.2013.10.050
Bubak A. N., Watt M. J., Renner K. J., Luman A. A., Costabile J. D., Sanders E. J., et al. (2019). Sex differences in aggression: Differential roles of 5-HT2, neuropeptide F and tachykinin. PloS One 14, e0203980. doi: 10.1371/journal.pone.0203980
Cotton A., Foldvari M., Cotton S., Pomiankowski A. (2014). Male eyespan size is associated with meiotic drive in wild stalk-eyed flies (Teleopsis dalmanni). Heredity. 112 (4), 363-369. doi: 10.1038/hdy.2013.131
Egge A. R., Brandt Y., Swallow J. G. (2011). Sequential analysis of aggressive interactions in the stalk-eyed fly Teleopsis dalmanni. Behav. Ecol. Sociobiol. 65, 369–379. doi: 10.1007/s00265-010-1054-5
Enquist M., Leimar O. (1983). Evolution of fighting behaviour: Decision rules and assessment of relative strength. J. Theor. Biol. 102, 387–410. doi: 10.1016/0022-5193(83)90376-4
Friard O., Gamba M. (2016). BORIS : a free, versatile open-source event-logging software for video/audio coding and live observations. Methods Ecol. Evol. 7, 1325–1330. doi: 10.1111/2041-210X.12584
Gloor G. B., Preston C. R., Johnson-Schlitz D. M., Nassif N. A., Phillis R. W., Benz W. K., et al. (1993). Type I repressors of P element mobility. Genetics 135, 81–95. doi: 10.1093/genetics/135.1.81
Hastings I. M. (1994). Manifestations of sexual selection may depend on the genetic basis of sex determination. Proc. R. Soc Lond. B Biol. Sci. 258, 83–87. doi: 10.1098/rspb.1994.0146
Hingle A., Fowler K., Pomiankowski A. (2001). Size-dependent mate preference in the stalk-eyed fly Cyrtodiopsis dalmanni. Anim. Behav. 61, 589–595. doi: 10.1006/anbe.2000.1613
Jaenike J. (2001). Sex chromosome meiotic drive. Annu. Rev. Ecol. Syst. 32, 25–49. doi: 10.1146/annurev.ecolsys.32.081501.113958
Johns P. M., Wilkinson G. S. (2007). X chromosome influences sperm length in the stalk-eyed fly Cyrtodiopsis dalmanni. Heredity 99, 56–61. doi: 10.1038/sj.hdy.6800963
Johns P. M., Wolfenbarger L. L., Wilkinson G. S. (2005). Genetic linkage between a sexually selected trait and X chromosome meiotic drive. Proc. R. Soc B Biol. Sci. 272, 2097–2103. doi: 10.1098/rspb.2005.3183
Kirkpatrick M., Hall D. W. (2004). Sexual selection and sex linkage. Evolution 58, 683–691. doi: 10.1111/j.0014-3820.2004.tb00401.x
Kokko H., Jennions M. D., Brooks R. (2006). Unifying and testing models of sexual selection. Annu. Rev. Ecol. Evol. Syst. 37, 43–66. doi: 10.1146/annurev.ecolsys.37.091305.110259
Lande R., Wilkinson G. S. (1999). Models of sex-ratio meiotic drive and sexual selection in stalk-eyed flies. Genet. Res. 74, 245–253. doi: 10.1017/S0016672399004218
Lindholm A. K., Dyer K. A., Firman R. C., Fishman L., Forstmeier W., Holman L., et al. (2016). The ecology and evolutionary dynamics of meiotic drive. Trends Ecol. Evol. 31, 315–326. doi: 10.1016/j.tree.2016.02.001
Lyth S., Betancourt A. J., Price T. A. R., Verspoor R. L. (2023). The suppression of a selfish genetic element increases a male’s mating success in a fly. Ecol. Evol. 13, e10719. doi: 10.1002/ece3.10719
Maynard Smith J. (1991). Theories of sexual selection. Trends Ecol. Evol. 6, 146–151. doi: 10.1016/0169-5347(91)90055-3
Meade L., Finnegan S. R., Kad R., Fowler K., Pomiankowski A. (2020). Maintenance of fertility in the face of meiotic drive. Am. Nat. 195, 743–751. doi: 10.1086/707372
Muller H. J. (1964). The relation of recombination to mutational advance. Mutat. Res. 106, 2–9. doi: 10.1016/0027-5107(64)90047-8
Paczolt K. A., Reinhardt J. A., Wilkinson G. S. (2017). Contrasting patterns of X-chromosome divergence underlie multiple sex-ratio polymorphisms in stalk-eyed flies. J. Evol. Biol. 30, 1772–1784. doi: 10.1111/jeb.13140
Paczolt K. A., Welsh G. T., Wilkinson G. S. (2023). X chromosome drive is constrained by sexual selection and influences ornament evolution. Proc. R. Soc B Biol. Sci. 290, 20230929. doi: 10.1098/rspb.2023.0929
Panhuis T. M., Wilkinson G. S. (1999). Exaggerated male eye span influences contest outcome in stalk-eyed flies (Diopsidae). Behav. Ecol. Sociobiol. 46, 221–227. doi: 10.1007/s002650050613
Parker G. A. (1974). Assessment strategy and the evolution of fighting behaviour. J. Theor. Biol. 47, 223–243. doi: 10.1016/0022-5193(74)90111-8
Presgraves D. C., Severance E., Willrinson G. S. (1997). Sex chromosome meiotic drive in stalk-eyed flies. Genetics 147, 1169–1180. doi: 10.1093/genetics/147.3.1169
Reeve H. K., Pfennig D. W. (2003). Genetic biases for showy males: Are some genetic systems especially conducive to sexual selection? Proc. Natl. Acad. Sci. 100, 1089–1094. doi: 10.1073/pnas.0337427100
Reinhardt J. A., Baker R. H., Zimin A. V., Ladias C., Paczolt K. A., Werren J. H., et al. (2023). Impacts of sex ratio meiotic drive on genome structure and function in a stalk-eyed fly. Genome Biol. Evol., 15 (7), evad118. doi: 10.1093/gbe/evad118
Reinhold K., Engqvist L., Misof B., Kurtz J. (1999). Meiotic drive and evolution of female choice. Proc. R. Soc Lond. B Biol. Sci. 266, 1341–1345. doi: 10.1098/rspb.1999.0785
Wilkinson G. S. (1993). Artificial sexual selection alters allometry in the stalk-eyed fly Cyrtodiopsis dalmanni (Diptera: Diopsidae). Genet. Res. 62, 213–222. doi: 10.1017/S001667230003192X
Wilkinson G. S. (2005). Sex-linked correlated responses in female reproductive traits to selection on male eye span in stalk-eyed flies. Integr. Comp. Biol. 45, 500–510. doi: 10.1093/icb/45.3.500
Wilkinson G. S., Dodson G. N. (1997). “Function and evolution of antlers and eye stalks in flies,” in The Evolution of Mating Systems in Insects and Arachnids (Cambridge University Press, Cambridge, MA), 310–328.
Wilkinson G. S., Johns P. M., Kelleher E. S., Muscedere M. L., Lorsong A. (2006). Fitness effects of X chromosome drive in the stalk-eyed fly, Cyrtodiopsis dalmanni. J. Evol. Biol. 19, 1851–1860. doi: 10.1111/j.1420-9101.2006.01169.x
Wilkinson G. S., Kahler H., Baker R. H. (1998a). Evolution of female mating preferences in stalk-eyed flies. Behav. Ecol. 9, 525–533. doi: 10.1093/beheco/9.5.525
Wilkinson G. S., Presgraves D. C., Crymes L. (1998b). Male eye span in stalk-eyed flies indicates genetic quality by meiotic drive suppression. Nature 391, 276–279. doi: 10.1038/34640
Wilkinson G. S., Reillo P. R. (1994). Female choice response to artificial selection on an exaggerated male trait in a stalk-eyed fly. Proc. R. Soc B Biol. Sci. 255, 1–6. doi: 10.1098/rspb.1994.0001
Wilkinson G. S., Swallow J. G., Christensen S. J., Madden K. (2003). Phylogeography of sex ratio and multiple mating in stalk-eyed flies from Southeast Asia. Genetica 117, 37–46. doi: 10.1023/A:1022360531703
Keywords: meiotic drive, intrasexual selection, ornament, aggression, diopsid
Citation: Paczolt KA, Pritchard ME, Welsh GT, Wilkinson GS and Reinhardt JA (2024) Stalk-eyed flies carrying a driving X chromosome compensate by increasing fight intensity. Front. Ethol. 3:1461681. doi: 10.3389/fetho.2024.1461681
Received: 08 July 2024; Accepted: 22 August 2024;
Published: 21 October 2024.
Edited by:
Michael Moore, University of Colorado Denver, United StatesReviewed by:
Jason Vance, College of Charleston, United StatesAlycia Lackey, University of Louisville, United States
Copyright © 2024 Paczolt, Pritchard, Welsh, Wilkinson and Reinhardt. This is an open-access article distributed under the terms of the Creative Commons Attribution License (CC BY). The use, distribution or reproduction in other forums is permitted, provided the original author(s) and the copyright owner(s) are credited and that the original publication in this journal is cited, in accordance with accepted academic practice. No use, distribution or reproduction is permitted which does not comply with these terms.
*Correspondence: Kimberly A. Paczolt, a3BhY3pvbHRAdW1kLmVkdQ==; Josephine A. Reinhardt, cmVpbmhhcmR0QGdlbmVzZW8uZWR1