- Centre for Wildlife Ecology, Department of Biological Sciences, Simon Fraser University, Burnaby, BC, Canada
We describe a natural situation that supports predictions of theoretical scenarios in which predators tactically influence the food - safety trade-off faced by prey to increase their vulnerability. By using low-cost ‘false attacks’ or otherwise advertising their presence, predators force prey to spend time in refuges or in other forms of safety-enhancing behavior, during which foraging is impaired or impossible. Prey must compensate by taking extra risks at other times or places to meet their energy requirements, and as a consequence become easier to capture. We used data on the occurrence of over-ocean flocking (OOF) by Pacific dunlins (Calidris alpina pacifica), and on the timing and success of attacks by peregrines. OOF is a safe but energetically expensive alternative to traditional roosting, and largely replaced the latter in Boundary Bay of southwest British Columbia as the presence of wintering peregrines rose during the 1990s. Peregrines appear to use ‘false’ or ‘non-serious’ attacks to shift the occurrence of OOF to a tidal time frame earlier than is ideal for dunlins, thereby creating later hunting opportunities during which dunlins were vulnerable than otherwise would have been the case. The shift increased dunlin mortality substantially. Tactics used by predators such as prominent perching, salient signals and unpredictable appearances, could have evolved because this forces prey to increase their level of caution, rendering them more vulnerable at other times or places.
Introduction
It is rarely easy to catch prey, even for top predators considered to be highly effective. Based on a lifetime of watching wild peregrine falcons (Falco peregrinus) for example, Dekker (2009) estimated that the success rate of hunts is only ~14%. The ‘life – dinner’ principle attributes this to the idea that selection against unsuccessful escape is more stringent than against an unsuccessful hunt (but see Humphreys and Ruxton, 2020). Wolf and Mangel (2007) model a predator-prey behavioral game, novel in that it exposes a largely unexplored class of tactics that predators could theoretically use to heighten capture success.
Wolf & Mangel’s model is motivated by transient (also called Bigg’s) killer whales (Orcina orca) hunting Steller sea lions, Eumetopias jubatus (Baird and Dill, 1995). In the model both killer whales and sea lions must capture enough prey to avoid starvation, and sea lions must also avoid capture by killer whales. Sea lions are safer when foraging close to a haulout to which they can flee for refuge if killer whales appear - but prey are less abundant there (‘Ashmole’s halo’; Gaston et al., 2008). The model explores whether killer whales could influence this behavioral trade-off to their advantage.
When killer whales are absent, sea lions can forage without regard for the danger these top predators pose. As the presence of killer whales increases sea lions spend more time fleeing or on the refuge. If high enough, sea lions may have to forage at greater distances in order to meet their requirements. The accessibility of a haulout also affects the hunting tactics of killer whales. If sea lions are easily able to flee to a refuge, killer whales must increase their hunting activity to capture enough prey. In a similar vein, Beauchamp and Ruxton (2012) and Beauchamp (2016) asks whether and how predators could adjust the timing of attacks to influence hunting success. In this game prey are ‘responsive’ to predator stimuli, gradually relaxing their vigilance following an attack. A predator that waits longer between attacks thus faces less wary prey, but risks missing opportunities. Prey that remain wary for too long lose too much foraging time. In both these scenarios, the best tactic for each depends on that used by the other.
Analyzing the interactions of strategic agents such as predators and prey requires a game theoretical approach (Houston and McNamara, 1999). Wolf and Mangel (2007) show how an evolutionary stable strategy (ESS) pair can be specified (using the ‘best response’ procedure; McNamara et al., 1999) at which killer whales cannot improve the rate of prey capture by altering the attack rate, nor can sea lions improve their survival by altering the foraging distance. But predators have an additional tactic: they can make ‘false’ or ‘non-serious’ attacks that avoid the costs (e.g. risk of injury, stalking time) of a serious predation attempt. Such attacks may have low probability of success, but they force prey to take evasive actions or otherwise increase caution. Killer whales, for example, may cruise near haulouts prominently displaying their presence, forcing sea lions to spend more time out of the water. Under some circumstances this could force sea lions to compensate by foraging at greater distances, where they can later be more easily captured.
There are many descriptions in the literature of predators using tactics such as stealth, camouflage, lures or scaring techniques. Tactical deception of competitors for food (e.g. Munn, 1986) or mates (Jukema and Piersma, 2006) has also been described. For example, when scrambling against a competitor for a prey item, bluish-slate antshrikes (Thamnomanes schistogynus; a sentinel species in neotropical ant-following mixed-flocks of birds) sometimes give an alarm call - even if a predator is not present - gaining an advantage when the competitor pauses to scan for a predator. But do predatory tactics of the sort envisioned by Wolf and Mangel (2007) actually happen in nature? Here we describe a natural situation that appears to match their hypothetical scenario.
Pacific dunlins (Calidris alpina pacifica) are shorebirds that winter in large groups on temperate mudflats. Falcons (merlins Falco columbarius and especially peregrines Falco peregrinus) are their most important predators (Page and Whitacre, 1975; Dekker et al., 2012). Raptors are generally much more successful when able to surprise prey (Cresswell, 1996). At Boundary Bay in southwestern British Columbia, the majority of hunts made by peregrines are ‘open’ (i.e. pursuit far out over the mudflat). However most kills are made by ‘stealth hunting’ (62%, Dekker and Ydenberg, 2004), even though the tidally-driven availability of dunlins foraging close enough to shoreline cover to be ambushed supports only 12% of the hunts observed. Open hunts are usually prolonged, but stealth hunts are short, and abandoned if initially unsuccessful.
Dunlins and other shorebirds gather in large, dense groups to sleep and preen while the tide is high (‘roosting’). At Boundary Bay Pacific dunlins largely abandoned this traditional form of roosting during the 1990s, and instead took up ‘over ocean flocking’ (‘OOF’ or ‘high tide flight’; Dekker, 1998; Ydenberg et al., 2010). Diurnal high tide periods are now often spent circling in a loose flock high over the bay several hundred metres from shore. OOF has occasionally been described at other wintering locations, but appears uncommon. Roosting high on the intertidal zone along the edge of or even amongst salt marsh vegetation (Conklin and Colwell, 2007) or in nearby fields is dangerous because falcons can hunt by stealth at such locations. The steady rise after the mid-1970s in the number of peregrines present during winter on Boundary Bay (Ydenberg et al., 2017) made OOF the preferred behavioral mode for the high tide period.
Wolf and Mangel’s (2007) hypothesis is summarized in Figure 1. The underlying concept is that foragers facing a shortfall (such as of foraging time, or in body condition) reduce the effort devoted to anti-predator behavior in order to be able to compensate (McNamara and Houston, 1990). Reports from migratory stopover sites, for example, show that individual shorebirds with low body condition are over-represented among predator kills (Dierschke, 2003; Yosef et al., 2011), presumably because they take greater risks in foraging (see Lank and Ydenberg, 2003). The Wolf – Mangel hypothesis is that predators exploit this fact by forcing prey to spend extra time or energy in avoidance, so shifting their activity to times or places at which they are more vulnerable.
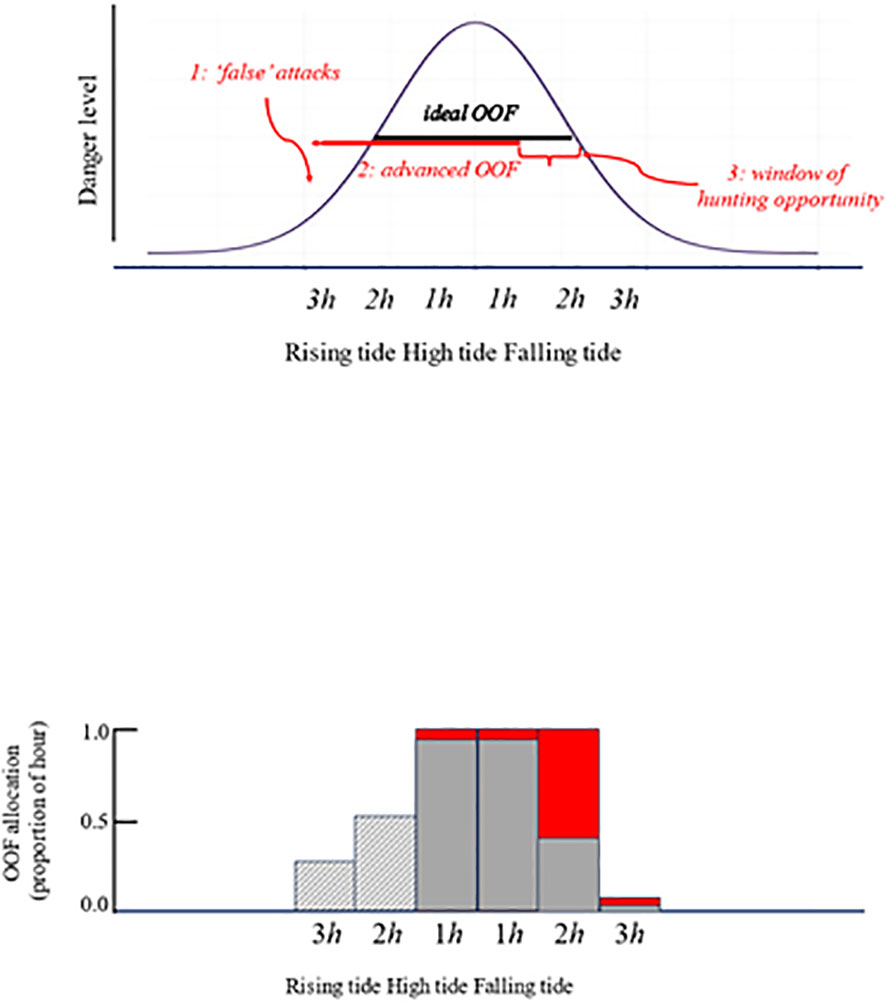
Figure 1 upper panel Schematic presentation of the Wolf – Mangel hypothesis applied to the peregrine – dunlin game. As the tide rises dunlins must forage closer to the shoreline, where peregrines are able to hunt by stealth; it becomes more dangerous as the tide level rises (solid curve). Over-ocean flocking (OOF) is much safer than foraging or roosting on the mudflat, but is energetically expensive. Dunlins ideally allocate the affordable duration of OOF (3.1h) to the highest tide levels (‘ideal OOF’ bar). Peregrines can induce an early start to OOF with ‘false’ or ‘non-serious’ attacks (1), which advance the onset of OOF (2), thereby creating a window of hunting opportunity (3) after OOF ceases and the tide is still high. The highest kill rate is observed during this window. lower panel The predicted (red) and observed (gray) allocation of OOF by dunlins at Boundary Bay, British Columbia, as a proportion of each of the hourly intervals before and after high tide. Red shading indicates a shortfall in OOF allocation, which creates hunting opportunity for peregrines. Hatched gray shading indicates over-allocation to OOF, superfluous because the danger level is low anyway.
Here we describe the temporal distribution of OOF by dunlins during the high tide period, the hunting activity of peregrines, measure the kill rate inflicted, estimate if and by how much OOF lowers mortality, and evaluate whether peregrines are able to affect the temporal distribution of OOF to their advantage, as predicted by the Wolf – Mangel hypothesis.
Methods
Peregrines and dunlins were observed during winter visits of one - three weeks to Boundary Bay on the Fraser River estuary in southwestern British Columbia (49°50’N, 123°0’W), made by Dick Dekker between1994 and 2012 (reported in Dekker, 1998; Dekker and Ydenberg, 2004; Dekker, 2013). Boundary Bay is 16 km in length with an intertidal zone several kilometres wide, bordered by 10 – 250m of salt marsh. The tidal rhythm is semi-diurnal, with amplitude up to 4.1m. Observations were made from the gravelled road on the 2m high dike backing the salt marsh, sometimes from a parked vehicle. Vantage points give unobstructed views (~2 km or more in each direction) of tidal flats and salt marsh. Some 20 - 40,000 dunlins are winter residents in the area, usually foraging in large flocks (Shepherd and Lank, 2004). Peregrines are present throughout the winter (Lank et al., 2003).
Over-ocean flocking by dunlins was recorded during dawn – dusk watches on 34 days in January 2006 and October 2008. Based on the majority of the flock, dunlins were classed at intervals of several minutes as engaged in OOF, or in foraging (spread over the mudflat surface, mostly along the tideline; see Jiménez et al., 2015). Other activities such as roosting were observed only rarely. The start and end of OOF were noted. OOF is easily distinguished from the other types of flight used by dunlins (foraging flights, transit flights, escape flights; see Reurink et al., 2016). It involves all or most of the dunlins present, and the large flock is usually easily visible as it circles aloft over the bay (Dekker, 1998). The flight mode is distinct, with much gliding, and low speed (Hentze, 2012). In very high winds, the flock may stream to and fro a few metres above the waves.
The time and success of peregrine attacks was systematically recorded on 151 days, using methods described by Dekker (2013); (Dekker and Ydenberg, 2004). An ‘attack’ or ‘hunt’ is defined as one completed attempt by a peregrine to seize a prey of which the outcome could be ascertained. An attack was considered successful if the falcon seized a dunlin or forced it into the water. Captured prey were often kleptoparasitized by bald eagles and occasionally by other raptors (Dekker et al., 2012). Bald eagles do not themselves catch dunlins, and dunlins largely ignore them. Kleptoparasitism increases the level of depredation on dunlins by peregrines, in order to replace the prey lost to kleptoparasites.
Our analyses are confined to the 6h period centered on the diurnal high tide. The observation time reported here is somewhat lower than the total reported in Dekker (2013) and Dekker and Ydenberg (2004), because their observation periods sometimes extended beyond the 6h interval. We divided the 6h high tide periods into one-hour blocks (3 – 2h, 2 – 1, and 1 – 0h before and after high tide) which for convenience are termed the ‘3h- pre-high tide interval’, the ‘2h- post-high tide interval’, etc. Poor weather or darkness sometimes interrupted observations, and combined with the second diurnal high tide that occurs on some days to spread observation hours unequally across the 6h tidal window.
The first objective of our analysis was to assess whether OOF reduces the mortality inflicted by peregrines. Dekker’s (1998); (see also Ydenberg et al., 2010) hypothesis that OOF is an effective anti-predator tactic is supported by the fact that almost all observed kills were of dunlins not engaged in OOF. To make quantitative estimates we compare ‘risk’ and ‘danger’, terms defined by Lank and Ydenberg (2003)); Ydenberg et al., 2022; see also Creel et al., 2019 who use the equivalent terms ‘realized risk’ and ‘inherent danger’). Risk is the actual mortality (measured as kills h-1, in each of the six hourly intervals). Danger is the mortality that would occur if prey undertook no antipredator behavior (here OOF). We estimate the level of danger by the mortality rate (kills h-1) while dunlins were not engaged in OOF in each of the six hourly intervals.
We tabulated for each of the six hourly intervals the observation time, the proportion dunlins spent in OOF (hence also the time not spent in OOF), and the number of kills observed. From these summary data we calculated the overall kill rate (total number of kills observed divided by total observation time). For each of the six hourly intervals we calculated the risk (kills per observation hour); the relative risk (relative to the average kill rate); and the level of danger (kill rate while dunlins were not engaged in OOF).
We took the average time spent in OOF on the days that it occurred as the estimate of the time that could be allocated to OOF. For greatest effectiveness as an anti-predator defense, the time available for OOF should be allocated in descending order to the most dangerous hourly intervals (i.e. when not engaging in OOF would lead to highest mortality). The hypothesis predicts that peregrines would benefit from shifting the allocation of OOF to reduce its occurrence in the most dangerous hourly intervals. We used simple correlations among the six hourly intervals to compare (i) risk and danger; (ii) the allocation (prop.) of time to OOF and risk; and (iii) the allocation (prop.) of time to OOF and danger.
Results
Over-ocean flocking
OOF was observed on 23 of the 34 days (68%), and dunlins spent 69.8 (57%) of the total of 123 observation hours on these days engaged in OOF. The 11 days without OOF included 7 days on which high tide occurred long before dawn, 2 days with continuous rain, one windless day with an early high tide, and one apparently ‘normal’ day. The mean daily duration of OOF over all 34 days was 2.05h, but it lasted on average 3.1h on the 23 days that it did occur. The temporal distribution of OOF observed over the 6h high tide period is summarized in Table 1. The 1h intervals immediately pre- and post-high tide are almost entirely devoted to OOF, with the allocation declining in both directions from the high tide point.
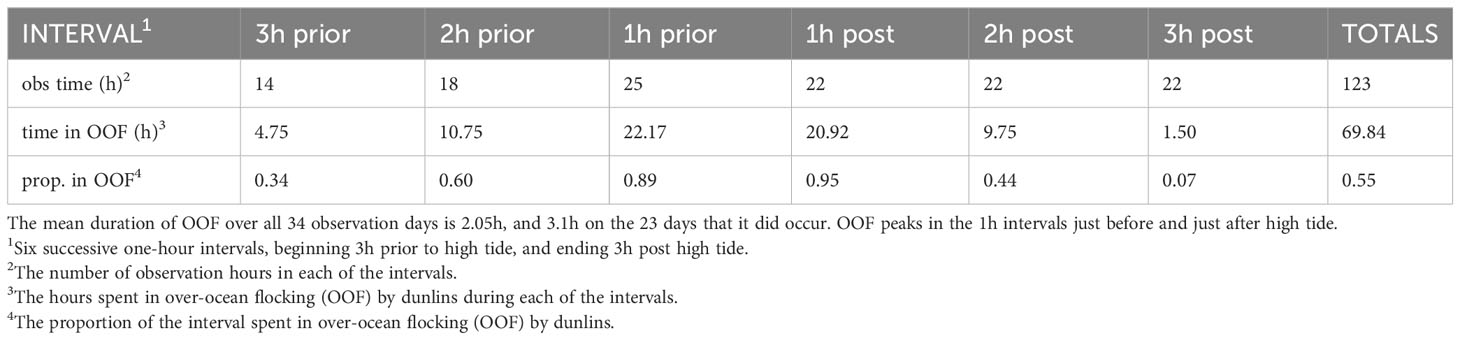
Table 1 The occurrence of over-ocean flocking (OOF) by dunlins during the 6h window centered on high tide, in Boundary Bay, British Columbia.
Predation by peregrines
A total of 139 kills of dunlins by peregrines was recorded during 905 observation hours, for an overall average risk of 0.15 h-1. The temporal distribution of kills over the 6h high tide period is uneven, and is summarized in Table 2. Kills occurred at a rate lower than the average during the intervals 2h- and 3h- prior to high tide, slightly less often than the average around high tide, and at a rate higher than the average 2h- and 3h- after high tide. As noted by both Dekker and Ydenberg (2004) and by Dekker (2013); (see his Figure 3) there is a sharp peak in the kill rate during the 2h- post-high tide interval. This feature is of special interest.
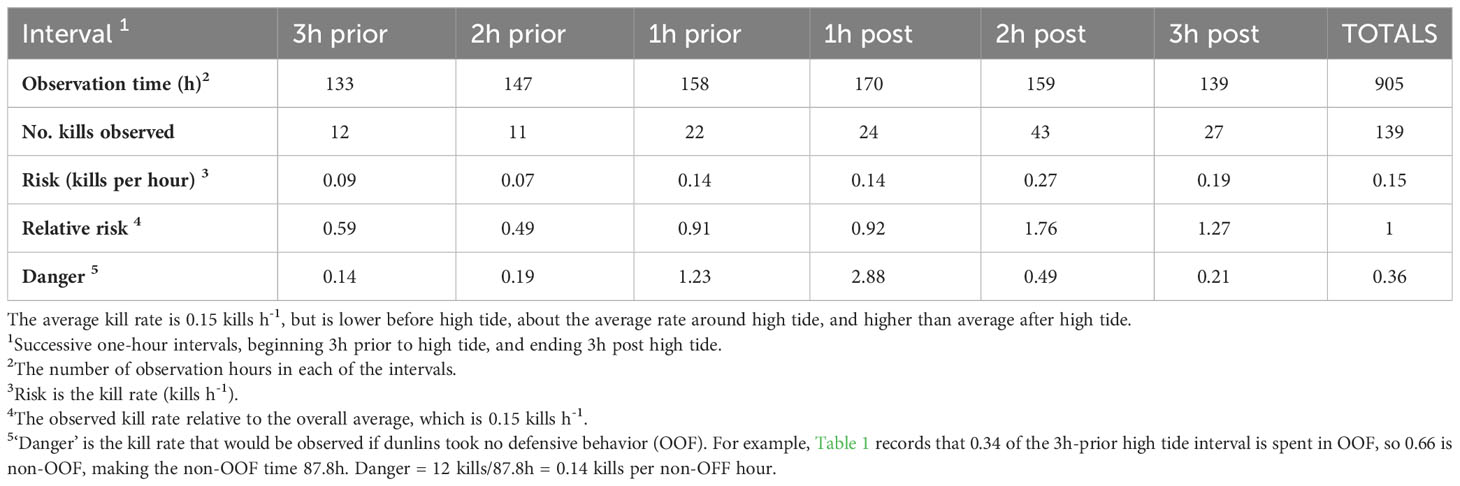
Table 2 The occurrence of dunlin kills by peregrines during the 6h window centered on high tide, in Boundary Bay, British Columbia.
The key concept in our analysis is ‘danger’ – the kill rate that would be observed if dunlins did not engage in OOF (i.e. took no defensive measures). In each of the six intervals danger (overall average 0.36h-1) is higher than the risk (overall average 0.15h-1), as expected if the function of OOF is to provide safety from peregrines (Table 3). As previously asserted (Dekker and Ydenberg, 2004; Dekker, 2013), the intervals just before and especially that just after the high tide point are the most dangerous (Table 3).
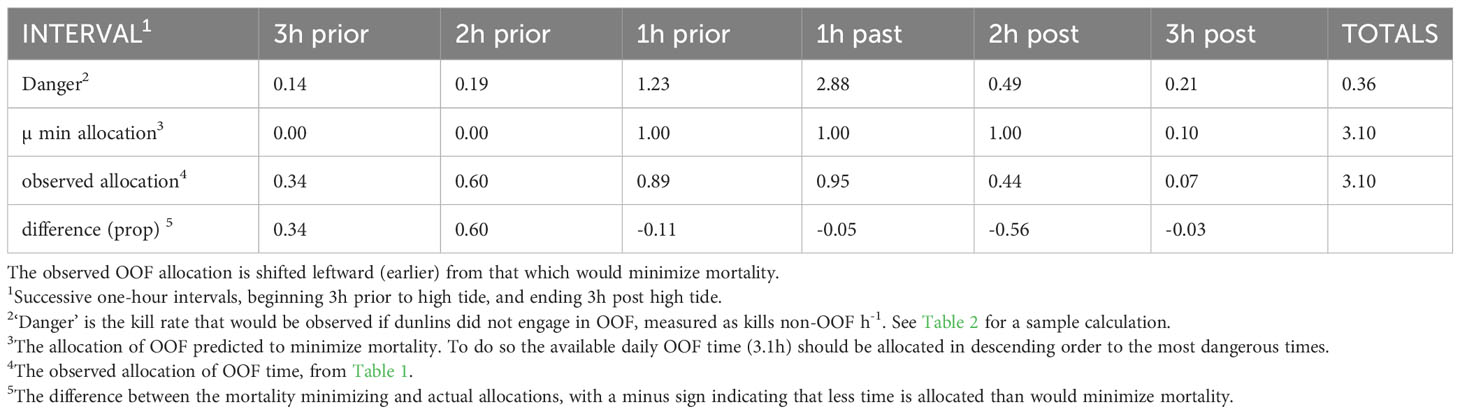
Table 3 Danger and the observed temporal allocation of over-ocean flocking by dunlins, compared with that predicted to minimize mortality (‘µ min’).
Danger and risk both vary strongly among the six hourly intervals, but their levels are not at all related (r = 0.007), because dunlins devote a varying amount of time to OOF in each interval. The allocation (prop. of time) to OOF in each of the intervals is correlated with the danger level (r = 0.82; Table 3), as expected if the amount of defensive behavior increases with the level of danger. To minimize risk, the limited amount of OOF available (3.1h) should be allocated to the most dangerous intervals, which in descending order are the 1h- post-tide interval (danger 2.88, from Table 3), the 1h- pre-tide interval (danger 1.23), and the 2h- post-tide interval (danger 0.49). The small remaining time (0.1h) could be allocated to either the 3h- post-tide (danger 0.21) or the 2h- pre-tide interval (danger 0.19), which are almost equally dangerous. We allocated this to the former, because it has slightly higher danger. The match between the observed allocation and this pattern is moderate (r = 0.65; Table 3). The intervals just prior to and just after high tide are as predicted devoted (almost) entirely to OOF. Much more time than predicted is allocated to the intervals 3h- and 2h- prior to high tide, and much less than predicted to the 2h-post tide interval (Table 3).
Discussion
Over-ocean flocking is safer but energetically more expensive than traditional roosting (Ydenberg et al., 2010), analogous to the long flights shorebirds sometimes make to distant but safer roosting sites (Brennan et al., 1985; Rogers et al., 2006; Rosa et al., 2006; Johnston-González and Abril, 2019). We observed that OOF occurred on 68% of winter days, and lasted on average 3.1h. Two other data sets collected at Boundary Bay totalling an additional 62 observation days report OOF timing closely matching these measures (Out, 2010; Hentze, 2012).
An hour of OOF is estimated to add ~3.0 – 4.3% to the daily energy requirement of wintering dunlins at Boundary Bay (Hentze, 2012; see his Table 3.2), even when taking into account attributes that lower the energetic cost of time in the air (i.e. much gliding, minimum power speed; see Hedenström and Alerstam, 1996). The increased daily energy expenditure resulting from OOF is presumably financed by additional foraging, which could occur at night (Shepherd and Lank, 2004), or at other portions of the tidal cycle (Jiménez et al., 2015). As expected if a trade-off is operating, the incidence of OOF is reduced under conditions that make meeting the daily energetic requirement more challenging. For example, OOF does not occur on days without wind (flight is more expensive) or with heavy rain (foraging on mudflats is less successful; dunlins may forage at upland sites in such weather; Shepherd and Lank, 2004), and it lasts less long on cold days (higher daily energy expenditure; Dekker, 2013). OOF does not occur at all when safe roosting options are available, as when ice (Dekker, 2013) or driftwood (pers. obs.) floating in the bay provides roost sites distant from shore. Also as expected if OOF functions to counter the danger posed by (diurnal) falcons, Hentze (2012) confirmed using radar that OOF does not occur nocturnally. If ongoing, it ends abruptly at dusk and begins abruptly at dawn.
The Wolf and Mangel (2007); see Introduction) hypothesis holds that ‘false’ attacks made prior to high tide induce dunlins to initiate OOF sooner than they otherwise would. The energetic expense of OOF limits its duration, so an early start results in correspondingly early termination, which because the tide is still high creates stealth hunting opportunities. The peak in mortality during the 2h- post-high tide interval (0.27 kills h-1 Table 2) results from the relatively low allocation to OOF (0.44) during a time of high danger (0.49 kills h-1).
One test of the hypothesis would measure the incidence of half-hearted and serious attacks in relation to the tidal cycle. But even experienced observers cannot easily differentiate such hunts, making any such classification subjective. In his detailed descriptions of peregrine hunting tactics, Dekker (2009) does not use the term ‘false’ attack, but he does describe ‘half-hearted’ and ‘aborted’ attacks, as does the other veteran observer of wild peregrines (Ratcliffe, 1962). Dekker (1980) explains these attacks as part of ‘ …… an extended process, lasting several hours with frequent pauses …. The initial phase might be considered as a “warming-up” period, until it merges into a relatively brief period of serious hunting.” This verbal description matches the Wolf - Mangel hypothesis.
Nevertheless, it is possible that the temporal pattern we observed is not a consequence of peregrine tactics. For example, attacks by less skilled individual predators early in the high tide period may force dunlins into OOF, while skilled individuals concentrate their hunting just after high tide, raising the kill rate in that interval. Testing this hypothesis would require that individual predators be identifiable, but peregrines were not marked in Dekker’s studies. The frequency of observed hunts by peregrines at Boundary Bay (as well as in the Bay of Fundy, where semipalmated sandpipers Calidris pusilla also engage in OOF; Dekker at al., 2012) shows a marked peak 2 - 4 hours before high tide (unpubl. data). This is consistent with the Wolf – Mangel hypothesis, and does not seem readily explained by variation in skill among individuals.
A further hypothesis that does not invoke prescient predatory tactics by peregrines is that dunlins have a relatively low level of OOF during the 2h- post-tide interval because foraging is especially valuable at this time. For example, food availability may be highest on a falling tide (Esselink and Zwarts, 1989). Foraging during this dangerous time would be advantageous if the intake rate is high enough that the amount of foraging (and hence exposure to peregrines) required at other times of day is so reduced that total mortality is lowered. For this condition to hold, the reduction in mortality at other times would have to be larger than that incurred during the 2h-post high tide interval. This seems unlikely, as this interval accounts for 31% (43/139) of the kills observed. The danger more than 2 hours from high tide is very low, making it improbable that enough savings could be generated to compensate.
To minimize risk dunlins should allocate the daily quota of OOF (~3.1h) to the intervals with greatest danger, namely from 1h- pre-high tide to 2h- post-high tide (Table 2). This is when the tide level forces dunlins closest to the shoreline where peregrines can hunt by stealth. The observed allocation of OOF does not match this predicted pattern (Table 3) very closely. While the intervals just before and after high tide are as predicted devoted almost entirely to OOF, it occupies less than half of the 2h-post high tide interval (Table 1). Further, much of the 2h- and 3h-pre high tide intervals are spent in OOF (0.60 and 0.34 respectively, together ~66 minutes), in spite of the fact that danger is low (0.14 and 0.19 kills h-1, respectively). The mortality-minimizing allocation predicts no OOF at all during these intervals.
The relative kill rate in each interval is inversely proportional to the mismatch between the observed and predicted OOF allocations, with the mortality higher when too little time is spent in OOF (Figure 2). Had dunlins used the presumed mortality-minimizing allocation, the total number of kills that would have been observed (assuming that the kill rate during OOF is 0) is estimated at 75.7, which is 63.3 (45.5%) fewer than observed. Even if we allow that OOF reduces mortality by just 50%, the mortality-minimizing allocation would result in 18.8 (13.5%) fewer deaths. These estimates are consistent with Wolf and Mangel’s (2007) model.
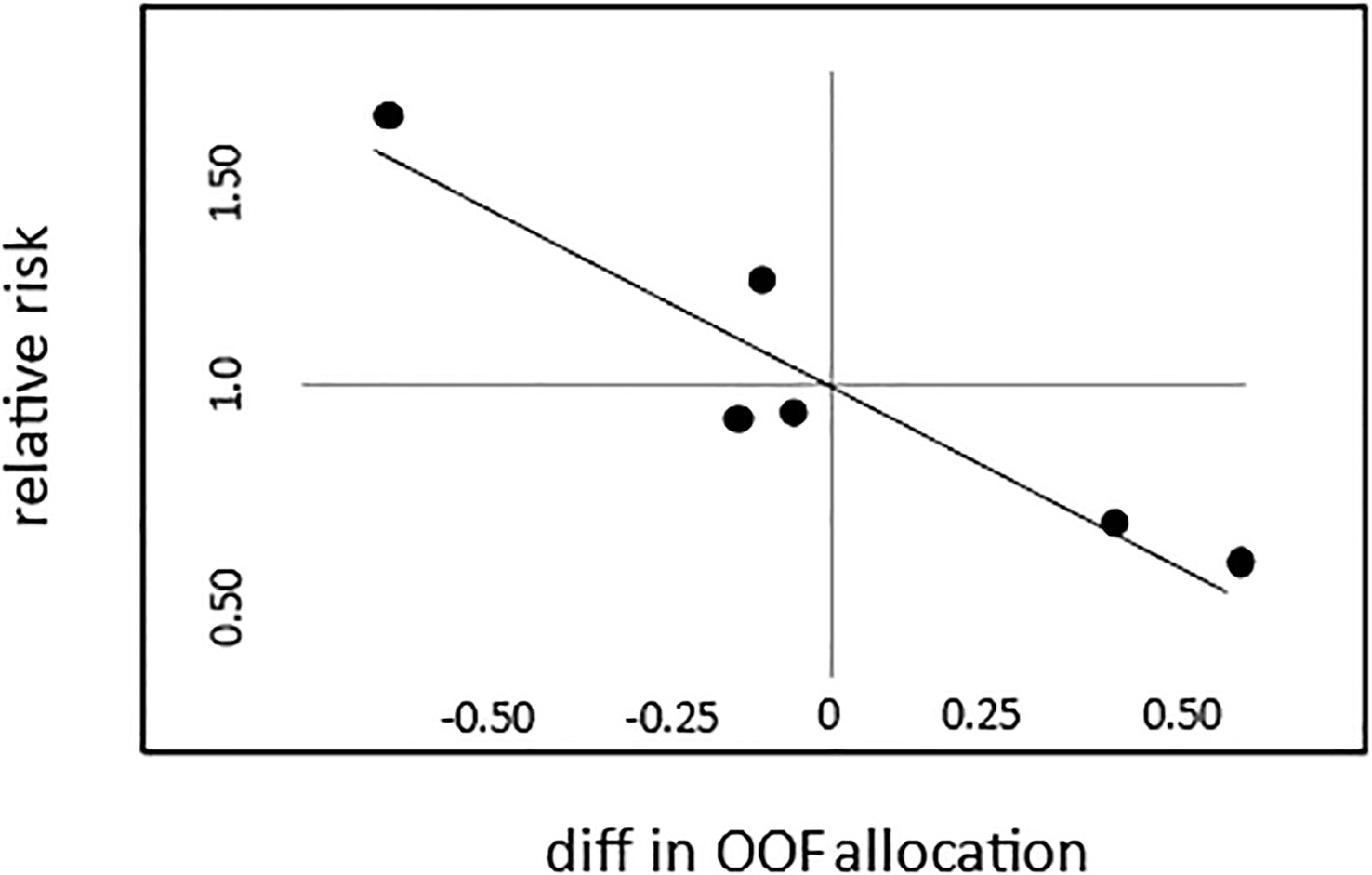
Figure 2 OOF allocation and the mortality imposed by peregrines on wintering dunlins at Boundary Bay, British Columbia. Points represent the 6 one-hour intervals from 3h pre- to 3h post-high tide. The x-axis is the difference between the observed OOF allocation (proportion in each interval) and that which would minimize mortality, with negative numbers representing less time spent in OOF than is optimal. The relative risk (y-axis; from Table 2) is inversely proportional to this difference, high when too little time is spent in OOF, and low when too much time is spent in OOF.
A general tactical consideration for predators is to match hunting effort to times and places at which prey are most active (Lang et al., 2019). The idea developed here is that predators could also hunt (or feign hunting) in order to shift some of their prey’s activity to times or places at which hunting is more successful. Such tactics may feature in many predator-prey interactions. Solitary predators with large territories such as pumas Felis concolor (Laundre, 2010) prowl about when or where captures are unlikely. The mere threat of their presence may be enough to require extra caution by prey, forcing them to take risks at other times or places. For example, Suselbeek et al. (2014) found that ocelots Leopardus pardalis are diurnally active even though captures of their main prey, tropical rainforest agoutis Dasyprocta punctata, are seldom made during daylight. Most agoutis are caught during twilight, before they enter the safety of their nocturnal burrows. However, the possible presence of ocelots during daytime requires agoutis to forage cautiously (i.e. more slowly), which results in the activity of some extending into twilight when ocelots have the advantage. Those with poor (low fruit availability) territories are forced to forage longest, enter their burrows latest, and are as a consequence at greatest risk. Predators in other systems may use analogous tactics. Prominent perching and soaring, unpredictable appearances, loud calls and odoriferous or otherwise salient signals that advertise a predator’s presence may all function as forms of ‘false attack’ that induce prey to alter behavior, possibly making them more vulnerable at other times or places.
Data availability statement
The original contributions presented in the study are included in the article/supplementary materials. Further inquiries can be directed to the corresponding author.
Ethics statement
The animal study was approved by Animal Care Committee, Simon Fraser University. The study was conducted in accordance with the local legislation and institutional requirements.
Author contributions
RY: Conceptualization, Data curation, Formal Analysis, Funding acquisition, Methodology, Writing – original draft, Writing – review & editing. RS-L: Conceptualization, Writing – review & editing. SY: Conceptualization, Writing – review & editing.
Acknowledgments
We dedicate this paper to Dick Dekker on his 90th birthday. At age 75 Dick successfully defended, in a memorable public event, a Ph.D. thesis submitted to Wageningen University entitled ‘Hunting by wild peregrines and other falcons’. The degree was hard-earned. His thesis, beautified by his own illustrations, was based on a lifetime of observation, much undertaken at great personal expense, meticulously and systematically recording data from each of thousands of field days. Armed with keen eyesight, vision and tenacity Dick became the world’s foremost watcher of wild falcons.
Conflict of interest
The authors declare that the research was conducted in the absence of any commercial or financial relationships that could be construed as a potential conflict of interest.
Publisher’s note
All claims expressed in this article are solely those of the authors and do not necessarily represent those of their affiliated organizations, or those of the publisher, the editors and the reviewers. Any product that may be evaluated in this article, or claim that may be made by its manufacturer, is not guaranteed or endorsed by the publisher.
References
Baird R. W., Dill L. M. (1995). Occurrence and behaviour of transient killer whales: seasonal and pod-specific variability, foraging behaviour, and prey handling. Can. J. Zool. 73 (7), 1300–1311. doi: 10.1139/z95-154
Beauchamp G. (2016). Function and structure of vigilance in a gregarious species exposed to threats from predators and conspecifics. Anim. Behav. 116, 195–201. doi: 10.1016/j.anbehav.2016.04.008
Beauchamp G., Ruxton G. D. (2012). Changes in anti-predator vigilance over time caused by a war of attrition between predator and prey. Behav. Ecol. 23, 368–374. doi: 10.1093/beheco/arr194
Brennan L. A., Buchanan J. B., Herman S. G., Johnson T. M. (1985). Interhabitat movements of wintering dunlins in Western Washington. Murrelet 66, 11–16. doi: 10.2307/3535459
Conklin J. R., Colwell M. A. (2007). Diurnal and nocturnal roost site fidelity of Dunlin (Calidris alpina pacifica) at Humboldt Bay, California. Auk 124 (2), 677–689. doi: 10.1093/auk/124.2.677
Creel S., Becker M., Droge E., M’soka J., Matandiko W., Rosenblatt E., et al. (2019). What explains variation in the strength of behavioral responses to predation risk? A standardized test with large carnivore and ungulate guilds in three ecosystems. Biol. Conserv. 232, 164–172. doi: 10.1016/j.biocon.2019.02.012
Cresswell W. (1996). Surprise as a winter hunting strategy in Sparrowhawks, Peregrines, and Merlins. Ibis 138, 684–692. doi: 10.1111/j.1474-919X.1996.tb04770.x
Dekker D. (1998). Over-ocean flocking by Dunlins (Calidris alpina) and the effect of raptor predation at Boundary Bay, British Columbia. Can. Field-Nat. 112, 694–697.
Dekker D. (2009). Hunting tactics of Peregrines and other falcons. (Wageningen University and Research).
Dekker D. (2013). High-tide flight by wintering Dunlin (Calidris alpina): a weather-dependent trade-off between energy loss and predation risk. Can. J. Zool. 91, 25–29. doi: 10.1139/cjz-2012-0213
Dekker D., Out M., Tabak M., Ydenberg R. (2012). The effect of kleptoparasitic Bald Eagles and Gyrfalcons on the kill rate of Peregrine Falcons hunting Dunlins wintering in British Columbia. Condor 114, 290–294. doi: 10.1525/cond.2012.110110
Dekker D., Ydenberg R. C. (2004). Raptor predation on wintering dunlins in relation to the tidal cycle. Condor 106, 415–419. doi: 10.1093/condor/106.2.415
Dierschke V. (2003). Predation hazard during migratory stopover: are light or heavy birds under risk? J. Avian Biol. 34, 24–29. doi: 10.1034/j.1600-048X.2003.03049.x
Esselink P., Zwarts L. (1989). Seasonal trend in burrow depth and tidal variation in feeding activity of Nereis diversicolor. Mar. Ecol. Prog. Ser. 56, 243–254. doi: 10.3354/meps056243
Gaston A. J., Ydenberg R. C., Smith G. E. J. (2008). Ashmole’s halo and population regulation in seabirds. Mar. Ornithol. 35, 119–126.
Hedenström A., Alerstam T. (1996). Skylark optimal flight speeds for flying nowhere and somewhere. Beh Ecol. 7, 121–126. doi: 10.1093/beheco/7.2.121
Hentze N. T. (2012). Characteristics of over-ocean flocking by Pacific dunlins (Calidris alpina pacifica) at Boundary Bay, British Columbia. (MSc) (Burnaby: Simon Fraser University).
Houston A. I., McNamara J. M. (1999). Models of Adaptive Behaviour: An Approach Based on State (Cambridge: Cambridge University Press).
Humphreys R. K., Ruxton G. D. (2020). Avian distraction displays: a review. Ibis (London, England), 162 (4), 1125–1145. doi: 10.1111/ibi.12814
Jiménez A., Elner R. W., Favaro C., Rickards K., Ydenberg R. C. (2015). Intertidal biofilm distribution underpins differential tide-following behavior of two sandpiper species (Calidris mauri and Calidris alpina) during northward migration. Estuar. Coast. Shelf Sci. 155, 8–16. doi: 10.1016/j.ecss.2014.12.038
Johnston-González R., Abril E. (2019). Predation risk and resource availability explain roost locations of Whimbrel Numenius phaeopus in a tropical mangrove delta. Ibis 161, 839–853. doi: 10.1111/ibi.12678
Jukema J., Piersma T. (2006). Permanent female mimics in a lekking shorebird. Biol. Lett. 2, 161–164. doi: 10.1098/rsbl.2005.0416
Lang S. D., Mann R. P., Farine D. R. (2019). Temporal activity patterns of predators and prey across broad geographic scales. Beh Ecol. 30, 172–180. doi: 10.1093/beheco/ary133
Lank D. B., Butler R. W., Ireland J., Ydenberg R. C. (2003). Effects of predation danger on migratory strategies of sandpipers. Oikos 103, 303–319. doi: 10.1034/j.1600-0706.2003.12314.x
Lank D. B., Ydenberg R. C. (2003). Death and danger at migratory stopovers: problems with "predation risk". J. Avian Biol. 34, 225–228. doi: 10.1034/j.1600-048X.2003.03250.x
Laundre J. W. (2010). Behavioral response races, predator-prey shell games, ecology of fear, and patch use of pumas and their ungulate prey. Ecology 91, 2995–3007. doi: 10.1890/08-2345.1
McNamara J. M., Gasson C. E., Houston A. I. (1999). Incorporating rules for responding into evolutionary games. Nature 401, 368–371. doi: 10.1038/43869
McNamara J. M., Houston A. I. (1990). The value of fat reserves and the tradeoff between starvation and predation. Acta Biotheor. 38, 37–61. doi: 10.1007/BF00047272
Out M. (2010). Escape from the Falcon's claws: how Dunlins manage timing of over-ocean flocking to lower predation risk. M.Sc. Thesis (Simon Fraser University).
Page G., Whitacre D. F. (1975). Raptor predation on wintering shorebirds. Condor 77, 73–83. doi: 10.2307/1366760
Ratcliffe D. A. (1962). Breeding density in the Peregrine Falco peregrinus and Raven Corvus corax. Ibis 104, 13–39. doi: 10.1111/j.1474-919X.1962.tb08625.x
Reurink F., Hentze N., Rourke J., Ydenberg R. (2016). Site-specific flight speeds of nonbreeding Pacific dunlins as a measure of the quality of a foraging habitat. Beh Ecol. 27, 803–809. doi: 10.1093/beheco/arv223
Rogers D. I., Piersma T., Hassell C. J. (2006). Roost availability may constrain shorebird distribution: exploring the energetic costs of roosting and disturbance around a tropical bay. Biol. Conserv. 133, 225–235. doi: 10.1016/j.biocon.2006.06.007
Rosa S., Encarnação A. L., Granadeiro J. P., Palmeirim J. M. (2006). High water roost selection by waders: maximizing feeding opportunities or avoiding predation? Ibis 148, 88–97. doi: 10.1111/j.1474-919X.2006.00497.x
Shepherd P. C. F., Lank D. B. (2004). Marine and agricultural habitat preferences of Dunlin wintering in British Columbia. J. Wildl Manage. 68, 61–73. doi: 10.2193/0022-541X(2004)068[0061:MAAHPO]2.0.CO;2
Suselbeek L., Emsens W.-J., Hirsch B. T., Kays R., Rowcliffe J. M., Zamora-Gutierrez V., et al. (2014). Food acquisition and predator avoidance in a Neotropical rodent. Anim. Behav. 88, 41–48. doi: 10.1016/j.anbehav.2013.11.012
Wolf N., Mangel M. (2007). Strategy, compromise, and cheating in predator–prey games. Evol. Ecol. Res. 9, 1293–1304.
Ydenberg R. C., Barrett J., Lank D. B., Xu C., Faber M. (2017). The redistribution of non-breeding dunlins in response to the post-DDT recovery of falcons. Oecologia 183, 1101–1110. doi: 10.1007/s00442-017-3835-2
Ydenberg R. C., Dekker D., Kaiser G., Shepherd P. C. F., Evans Ogden L., Rickards K., et al. (2010). Winter body mass and over-ocean flocking as components of danger management by Pacific dunlins. BMC Ecol. 10, 1. doi: 10.1186/1472-6785-10-1
Ydenberg R. C., Tavera E. A., Lank D. B. (2022). Danger, risk and anti-predator behavior in the life history of long-distance migratory sandpipers. J. Avian Biol. 53, e03002. doi: 10.1111/jav.03002
Keywords: predator - prey games, escape, foraging, trade off, deception, compromise
Citation: Ydenberg R, Young S and Sullivan-Lord R (2023) Gaming the system: how predators induce prey to make themselves more vulnerable. Front. Ethol. 2:1256380. doi: 10.3389/fetho.2023.1256380
Received: 10 July 2023; Accepted: 28 August 2023;
Published: 11 October 2023.
Edited by:
Rulon Clark, San Diego State University, United StatesReviewed by:
Yann Henaut, ECOSUR Conservation and Biodiversity, MexicoWilliam Pitt, Smithsonian Conservation Biology Institute (SI), United States
Copyright © 2023 Ydenberg, Young and Sullivan-Lord. This is an open-access article distributed under the terms of the Creative Commons Attribution License (CC BY). The use, distribution or reproduction in other forums is permitted, provided the original author(s) and the copyright owner(s) are credited and that the original publication in this journal is cited, in accordance with accepted academic practice. No use, distribution or reproduction is permitted which does not comply with these terms.
*Correspondence: Ron Ydenberg, ydenberg@sfu.ca