- 1Department of Biology, Concordia University, Montréal, QC, ;Canada
- 2Department of Biology, Carleton University, Ottawa, ON, Canada
Due to its unforgiving nature, predation pressure exerts strong selection pressure on the behaviour of prey animals. As a result, prey are forced to balance the conflicting demands of successfully detecting and avoiding predators and the need to engage in other fitness-related activities such as foraging, mating and social behaviour. Here, we provide an overview of the role that individual predator avoidance decisions plays in constraining behavioural phenotypes and how past experience with risks shapes current (and future) trade-offs, physiological and life history investments. Critically, access to reliable risk assessment information allows prey to respond to spatially and temporally variable predation risks. Uncertainty of predation risks is expected to limit the ability of prey to make short- and longer-term adjustments responses to predation threats, potentially increasing the indirect costs of predation. We describe a ‘landscape of information’ in which prey rely on publicly available risk assessment information to reduce the uncertainty of predation risks associated with variable threats and the potential impact of natural and anthropogenic environmental factors which may limit information availability. Despite a long tradition of research into the antipredator trade-offs made by prey animals, there remain a number of important unanswered questions.
Introduction
Predation is a pervasive and unforgiving selection pressure on prey populations. A large and diverse body of research has explored the impacts of predation on the life history (e.g. Chivers et al., 1999), morphology (e.g. Brönmark and Pettersson, 1994; Chivers et al., 2008; Brönmark et al., 2011), physiology (e.g. Cooke et al., 2003) and behaviour (e.g. Lima and Dill, 1990) of prey on both ecological and evolutionary time scales. Predators can exert adverse effects on their prey either directly by killing them or indirectly by instilling ‘fear’ in them (Zanette et al., 2011; Zanette and Clinchy, 2019; Allen et al., 2022), which induces behavioural, physiological and neurobiological costs on individuals attempting to avoid predation (Zanette and Clinchy, 2019). Of the multitude of possible responses to the fear of predation, behaviour is by far the most plastic and offers prey individuals an immediate response to acute predation threats (Lima and Dill, 1990; Sih et al., 2000). Antipredator behavioural responses to perceived acute predation threats (i.e. fear of predation) include increased vigilance, grouping behaviour, avoidance (through camouflage, reduced activity, seeking refuge or fleeing, for example), deterrence behaviour and aggressive defence (e.g. Lima and Dill, 1990; Dugatkin and Godin, 1992; Godin, 1997; Krause and Ruxton, 2002; Ruxton et al., 2004). The potential fitness benefits to an individual expressing one or more of these antipredator behaviours is increased survivorship and reproductive success (Figure 1), but at the potential fitness-related costs of expended energy and lost behavioural opportunities such as foraging, mating, parental care and territorial defence (Figure 1). Vice versa, engaging in any of the latter competing behavioural activities may increase an individual’s susceptibility to predation (e.g. Godin and Smith, 1988; Magnhagen, 1991; Jakobsson et al., 1995; Krause and Godin, 1996). Hence, individual prey are faced with trade-offs between such benefits and costs when ‘deciding’ on whether, when and how to respond to a perceived acute threat of predation (e.g. Lima and Dill, 1990). The optimal or adaptive decision, the one that maximises the individual prey’s fitness, depends on a number of factors including the magnitude of the perceived predation threat, the expected payoff of the antipredator response adopted, the prey’s vulnerability to predation, its current condition, its ‘personality’ and constraints imposed by correlated behaviours (Lima and Dill, 1990; Godin, 1997; Sih and Bell, 2008; Jones and Godin, 2010).
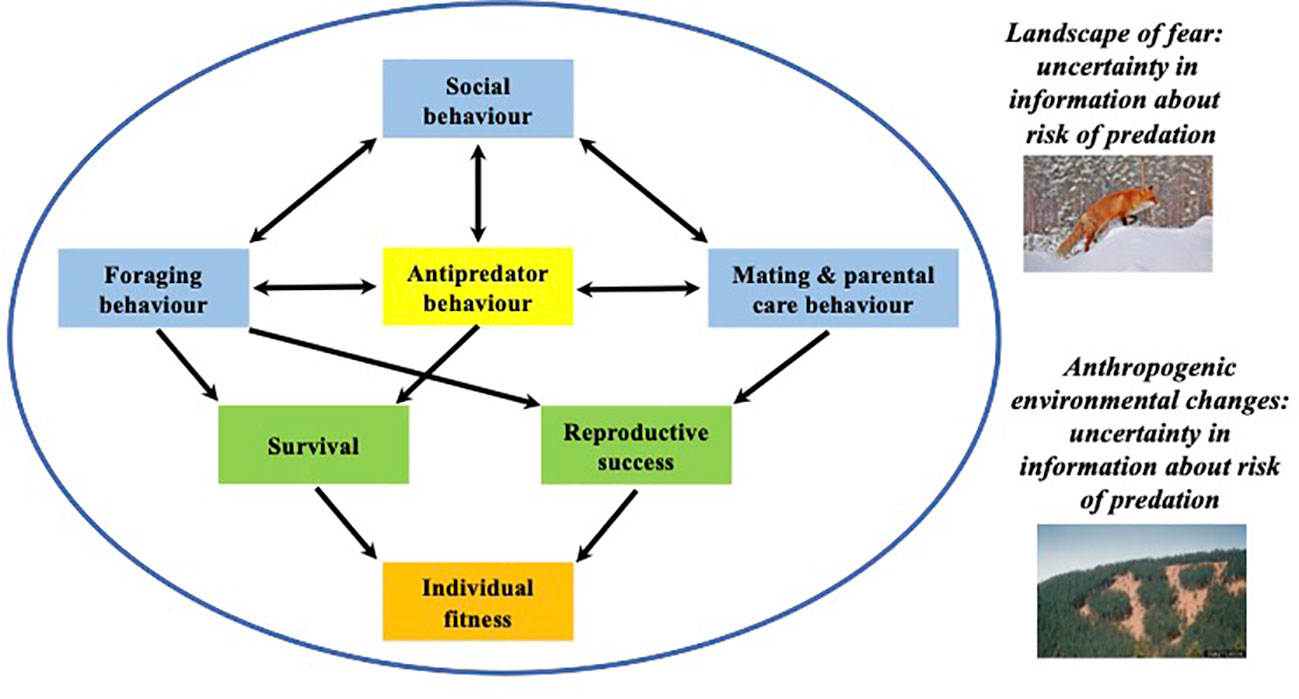
Figure 1 Conceptualisation of the functional categories of behaviour (blue boxes) that can interact with each other (bidirectional arrows) and be traded-off against the benefits associated with antipredator behaviour (yellow box). The accumulation of these trade-offs will impact individual survival and reproduction (green boxes), ultimately determining an individual’s lifetime fitness (orange box). Such trade-offs are expected to be influenced by (i) the spatial and temporal variation in predation risk (Landscape of Fear) and (ii) in risk assessment information (Landscape of Information) and its potential loss due to anthropogenic environmental changes.
Critically, the costs associated with antipredator behavioural decisions are asymmetric. Failing to respond to an ecologically relevant predation threat may result in death, whereas responding to an irrelevant threat result in wasted time and energy expended and lost opportunities to engage in other fitness-related activities (Figure 1). For example, prey that engage in antipredator behaviours in response to predation risk may have fewer opportunities to forage (e.g. Fraser and Gilliam, 1987; Lima and Dill, 1990), mate (Lima and Dill, 1990; Magnhagen, 1991; Sih, 1994), or engage in social behaviour (Kim et al., 2011) (Figure 1). The cumulation of such lost-opportunity costs over time are non-negligible and may lead to adverse non-consumptive effects (NCE) of the fear of predation on individual prey, such as reduced food intake, growth and fecundity, and resulting population-level impacts (Preisser et al., 2005; Preisser and Bolnick, 2008). Interestingly, Preisser et al. (2005) estimate that the impacts of NCEs on prey population dynamics can far exceed the consumptive effects due to the costs associated with predator-avoidance trade-offs (see also Zanette and Clinchy, 2019; Allen et al., 2022).
In recent years, the focus of individual behavioural responses to predation risk has shifted from the aforementioned discrete behavioural trade-offs to how past experiences with ambient predation shapes the individual prey’s ‘perception’ of risk. For example, Lima and Bednekoff, (1999) predation risk allocation hypothesis predicts that that prey exposed to frequent predation risks will respond less intensely to future acute predation threats and forage at higher rates during rare safe periods than those exposed to infrequent risks (e.g. Ferrari et al., 2009). Likewise, Clark (1994) proposed the asset protection model which posits that the behavioural decisions made by prey depend on their past success at accruing fitness. For example, Reinhardt and Healey (1999) found that faster growing coho salmon (Oncorhynchus kisutch) fry took longer to resume foraging following a predator exposure than did slower growing conspecifics. Similarly, gravid female Trinidadian guppies (Poecilia reticulata) exhibited stronger antipredator responses to a standardised predation cue compared to virgin conspecifics (Katwaroo-Andersen et al., 2016). In both these cases, individuals with higher ‘accrued fitness’ exhibited more risk averse tactics than those with lower ‘accrued fitness’. Effectively, an individual’s past experiences shapes the value of potential future trade-offs associated with responding to its perception of the ambient fear of predation.
The concept of the “Landscape of Fear” (LoF) predicts the impacts of spatial and temporal variation in the risks of predation on individual fitness, the population dynamics of prey (and predator), and the resulting community dynamics (e.g. Zanette et al., 2011; Zanette and Clinchy, 2019; Allen et al., 2022). Recent models have extended the LoF paradigm to highlight the spatial patterns of risk (Gaynor et al., 2019). In effect, these models predict that the presence of predation risks can dramatically alter the behaviour, physiology and life history of prey, leading to decreased recruitment and population growth. Because predation risk varies in space and time, its adverse impacts on prey populations will also vary over a ‘landscape of fear’. LoF models generally posit that, as the energetic costs associated with detecting and avoiding predators increases, prey fecundity and survival decreases (Zanette and Clinchy, 2019). Moreover, the impacts of these effects are expected to vary according to the temporal-spatial variability in risks (Gaynor et al., 2019).
Here, we propose that the spatial and temporal variation in predation risks in nature, inherent in the Landscape of Fear models (Gaynor et al., 2019; Zanette and Clinchy, 2019), can be reframed conceptually as a “Landscape of Information” about the fear of predation (Figure 2). This reframing emphasises the importance of understanding the types of ecological information, and their spatiotemporal variation, that predict the ambient risk of predation, how individual prey gain and assess such information, and how they use acquired information to make appropriate antipredator behavioural decisions. Additionally, we highlight the multiple interacting effects and complexity associated with predicting the responses of prey to predation and identify several critical areas of future study.
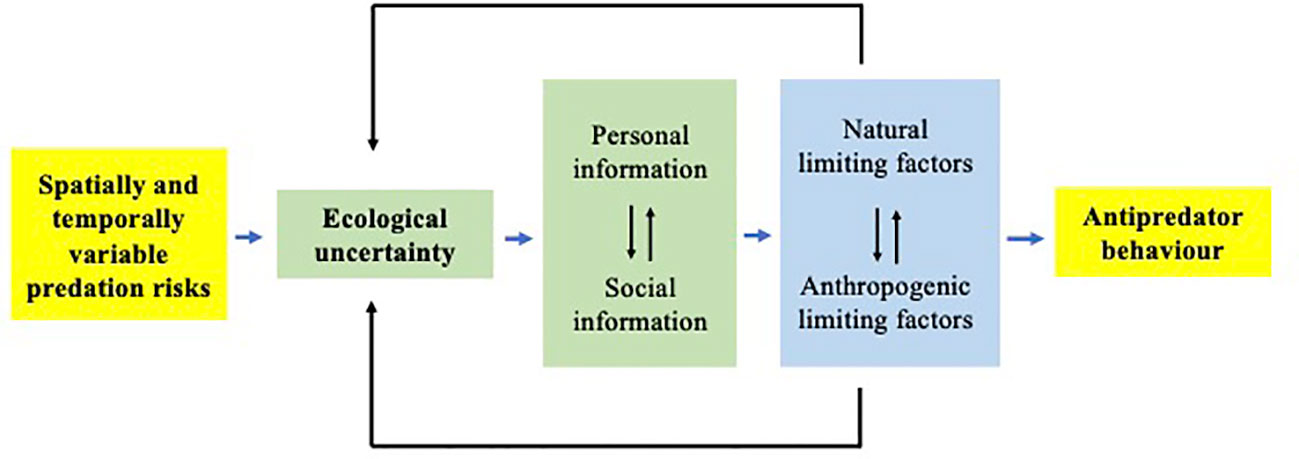
Figure 2 Conceptualisation of the proposed Landscape of Information concept. Spatially and temporally variable predation risks lead to ecological uncertainty within local habitats. Prey can reduce the costs of uncertainty by increasing their use of personal and(or) social information about their ambient risk of predation (risk assessment cues; green box) and respond with appropriate antipredator behaviour (yellow box). However, the availability of this information is dictated by the interacting effects of natural and anthropogenic factors (blue box) that may limit the quantity and(or) quality of information, thus increasing ecological uncertainty (see text for details).
Landscape of information about the fear of predation
The ability of prey to make context-appropriate behavioural decisions assumes the availability of reliable and honest indicators of local predation risks in order to avoid ecologically relevant threats and ignore ecologically irrelevant information (Brown et al., 2011; Brown et al., 2013). Individuals can obtain information about various aspects of their environment either directly via direct sampling (personal information) or indirectly by acquiring inadvertent social information from others (Danchin et al., 2004; Dall et al., 2005). The acquisition of personal information is considered more costly, but more accurate and reliable, than cheaper and potentially less reliable social information (Kendal et al., 2005; Feyten et al., 2021). Prey animals have a suite of publicly available information cues and social information cues (Brown et al., 2011; Weissburg et al., 2014) that can predict their ambient predation risk. However, these cues involve multiple sensory modalities which may differ in reliability and detectability (Brown et al., 2011; Weissburg et al., 2014), potentially giving rise to variability in the quantity and(or) quality of risk assessment. This variation in information could give rise to a ‘Landscape of Information’ (Figure 2).
Such a ‘Landscape of Information’ can best be represented by spatiotemporal variation in the individual prey’s level of certainty versus uncertainty of the local risk of mortality to predation versus safety (Luttbeg et al., 2020; Crane et al. in review). The concept of ecological uncertainty of risks is broadly defined as ambiguity regarding the risk of predation associated with a specific microhabitat owing to incomplete or unreliable information (Dall et al., 2005; Munoz and Blumstein, 2012; Feyten and Brown, 2018). At the same time, prey can also be exposed to uncertainty of rewards associated with occupying a specific microhabitat (Feyten et al., 2021; Brusseau et al. in press; Brown et al., 2022). Generally speaking, as prey are increasingly uncertain about local risks, the relative costs associated with failing to recognise a threat disproportionately increase, leading to an overestimation of acute threats (Johnson et al., 2013). While ‘uncertainty’ is a complex and poorly understood concept (Crane et al. in review), it does highlight the need for prey to acquire additional information regarding risks and (or) rewards in order to make appropriate adaptive behavioural decisions. For example, Brusseau et al. (in press) have recently shown that Trinidadian guppies engage in increased predator inspection behaviour (i.e. information gathering, Dugatkin and Godin, 1992; Fishman, 1999) in sites along rivers disturbed by human recreation relative to undisturbed sites. Certain human activities (Candolin and Rahman, 2023) likely increase uncertainty regarding the identity of potential predation risks and potential rewards (i.e. novel foraging opportunities). This uncertainty is argued to lead to increased information gathering among prey.
Factors driving availability of ecological information
Given the critical importance of the availability of risk assessment cues, there are at least two general categories of environmental factors that can influence the behavioural decision-making process within prey populations. First, variability in naturally occurring factors, such as microhabitat structural complexity, current velocity, water depth, and ambient light might shape the quantity and/or quality of information available to prey and the resulting perception of predation risk. For example, young-of-the-year Atlantic salmon defend smaller foraging territories and exhibit reduced reaction distances towards standardised predation threats (i.e. reduced perceived predation risk) in complex versus more homogeneous habitats (Venter et al., 2008). Likewise, fathead minnows (Pimephales promelas) show lower levels of baseline predator vigilance in structured microhabitats than in barren ones (Crane et al., 2020). However, the effects of habitat complexity may differ. Trinidadian guppies, for example, exhibit higher levels of perceived predation risk in highly structured microhabitats (Feyten, 2023), likely due to limitations of visual risk assessment. Another environmental factor is ambient light levels, resulting from either diel cycles or shading. Australian house geckos (Gehyra dubia) show higher activity and foraging rates on bright (i.e. high moon brightness) nights than on darker nights (Norberg and Schwarzkopf, 2022). Conversely, brown-headed cowbirds (Molothus ater) are impaired in their ability to detect predators in full sunlight compared with shaded patches, presumably due to increased glare (Fernández-Juricic et al., 2012). Additionally, prey may be capable of compensating for reduced information via one sensory modality by increasing their reliance on other modalities. Juvenile Atlantic salmon (Salmo salar), for example, exhibit stronger responses to conspecific alarm cues at night (low visual information) than during daylight (high visual information; Leduc et al., 2010). Within aquatic ecosystems, water depth and current velocity can also influence information availability and resulting behavioural trade-offs. For example, Hazlett et al. (2009) demonstrated that the ability of crayfish (Orconectes virilis) to detect and respond to predator odours is negatively related to current velocity, resulting in reduced foraging activity and increased time spend immobile. Similarly, juvenile chub (Leuciscus cephalus) shift from fast moving riffles to slower moving stream sections following the presentation of a simulated avian predator (Allouche and Gaudin, 2003). This shift in habitat results in reduced foraging opportunities and growth (Allouche and Gaudin, 2003).
Second, in addition to natural variation in environmental factors, multiple researchers have examined the impact of anthropogenic disturbances on the quantity and/or reliability of ecological information. Presumably, any anthropogenic factor that alters the availability of information will impair the ability of prey to make reliable behavioural decisions (Leduc et al., 2013; Candolin, 2019; Candolin and Rahman, 2023), potentially leading to increased uncertainty. However, these studies have often focussed on variation within in a single environmental stressor (Hale et al., 2017; O’Brien et al., 2019). For example, Leduc et al. (2009) demonstrated that juvenile Atlantic salmon do not respond to reliable indicators of predation risk under weakly acidic stream conditions (i.e. impacts of acid rain), leading to increased predation mortality (Elvidge and Brown, 2014). Similarly, increased water turbidity limits the ability of fathead minnows to generalise the learned recognition of novel predators (Chivers et al., 2013). More important, however, are the likely interacting effects of multiple environmental stressors on information availability. While any number of anthropogenic factors (e.g. pollutants, turbidity, habitat degradation; Candolin and Rahman, 2023) may alter predation risk assessment information and individual behavioural decisions, such as antipredator responses, foraging patterns, habitat choices and (or) mating decisions, relatively few studies have examined the interacting effects of multiple stressors. Moreover, anthropogenic stressors may (co) vary independently, making it increasingly difficult to predict their impacts on behavioural decisions. While community ecologists have long recognised the potential for complex interactions among multiple stressors (e.g. Benedetti-Cecchi, 2003; Benedetti-Cecchi et al., 2006; Koussorpolis and Wacker, 2015; Norberg and Schwarzkopf, 2022), few studies have taken such an approach to individual behaviour (e.g. Brodin et al., 2014).
Discussion
In this brief perspective article, we have proposed that the “Landscape of Fear” paradigm can be reframed as a “Landscape of Information” paradigm, emphasising the importance of understanding the types of ecological information, and their spatiotemporal variation, that predict the ambient risk of predation, how individual prey gain and assess such information, and how they use acquired information to make appropriate antipredator behavioural decisions. We have additionally attempted to highlight that behavioural trade-offs are complex and dependent on a variety of interacting factors. There are a number of critical research questions and challenges that remain.
First, future studies need to examine the links between individual predator avoidance trade-offs under a wide range of conditions (e.g. variable predation risk, different life history stages, variable information availability), so as to forge empirical links with the predictions of population and community models. Many ‘top-down’ approaches underestimate the importance of behavioural decision making in prey animals. It is apparent from the Non-consumptive Effects of Predation (NCE) and Landscape of Fear models that the behaviour of individual prey has considerable impact on population viability and community structure. However, the potential impact of day-to-day variation in the behavioural decisions of individual animals on higher-order processes (at the population and community levels) remains poorly understood.
Models of the NCE assume that prey that are forced to balance predator avoidance versus foraging (for example) might have less energy available for other fitness-related activities (e.g. courtship) (Figure 1). Indeed, individual behavioural decisions likely have highly complex (and poorly understood) interacting affects among a suite of functional behaviours. If we aim to scale models from individual behaviour to long-term fitness (i.e. a bottom-up approach), much needed empirical data are required.
Second, behavioural decisions are known to be highly plastic (see above) and, as we have argued here, influenced by variability in factors such as uncertainty, information availability, and the form and intensity of predation pressure. Integrating this variance into models of population and/or community dynamics is a critical step in fine tuning future models. This is particularly important in the use of such models to address practical issues in conservation and management. As described above, our understanding of how multiple interacting anthropogenic stressors constrain the ability of prey to make appropriate behavioural decisions is currently limited, which in turn hinders our ability to make informed conservation/management policies.
Finally, long-term behavioural observations on prey have historically proven logistically difficult. However, new (and more affordable) technological solutions are becoming increasingly available to researchers, allowing them to track individual prey or subpopulations of prey and their predators as they move in space and over time. For example, visible implant elastomer tags, radio frequency identification (RFID) and passive integrative transponder (PIT) technologies are now available for even very small bodied prey. Biomimetic robots have been employed to manipulate, for example, behaviour and signals in small-bodied prey fish (e.g. Heathcote et al., 2018). Radio and GPS collars are widely used for remotely tracking and quantifying behaviour patterns, such as foraging and mating, in ungulates (e.g. Body et al., 2012; 2014). Likewise, inexpensive and dependable camera traps and data loggers allow researchers to quantify the presence of predators and a suite of environmental variables over time (e.g. McCarthy et al., 2018). Such technologies can be employed to quantify longer-term impacts of behavioural trade-offs, in situ.
Data availability statement
The original contributions presented in the study are included in the article/supplementary material. Further inquiries can be directed to the corresponding author.
Author contributions
All authors listed have made a substantial, direct, and intellectual contribution to the work and approved it for publication.
Funding
GB and J-GG are supported by the Natural Sciences and Engineering Research Council of Canada.
Acknowledgments
We would like to thank the numerous colleagues and students who have contributed to our research programs.
Conflict of interest
The authors declare that the research was conducted in the absence of any commercial or financial relationships that could be construed as a potential conflict of interest.
Authors J-GG and GB declared that they were editorial board members of Frontiers at the time of submission. This had no impact on the peer review process and the final decision.
Publisher’s note
All claims expressed in this article are solely those of the authors and do not necessarily represent those of their affiliated organizations, or those of the publisher, the editors and the reviewers. Any product that may be evaluated in this article, or claim that may be made by its manufacturer, is not guaranteed or endorsed by the publisher.
References
Allen M., Clinchy M., Zanette L. Y. (2022). Fear of predators in free-living wildlife reduces population growth over generations. Proc. Natl. Acad. Sci. USA 119, e2112404119. doi: 10.1073/pnas.2112404119
Allouche S., Gaudin P. (2003). Effects of avian predation threat, water flow and cover on growth and habitat use by chub, Leuciscus cephalus, in an experimental stream. Oikos 94, 481–492. doi: 10.1034/j.1600-0706.2001.940310.x
Benedetti-Cecchi L. (2003). The importance of the variance around the mean effect size of ecological processes. Ecology 84, 2335–2346. doi: 10.1890/02-8011
Benedetti-Cecchi L., Bertocci I., Vaselli S., Maggi E. (2006). Tempoal variance reverses the eimpact of high mean intensity of stress in climate change experiments. Ecology 87, 2489–2499. doi: 10.1890/0012-9658(2006)87[2489:TVRTIO]2.0.CO;2
Body G., Weladji R. B., Holand Ø. (2012). The recursive model as a new approach to validate and monitor activity sensors. Behav. Ecol. Sociobiol 66, 1531–1541. doi: 10.1007/s00265-012-1414-4
Body G., Weladji R. B., Holand Ø, Nieminen M. (2014). Highly competitive reindeer males control female behavior during the rut. PloS One 9, e95618. doi: 10.1371/journal.pone.0095618
Brodin T., Piovano S., Fick J., Klaminder J., Heynen M., Jonsson M. (2014). Ecological effects of pharmaceuticals in aquatic systems – impacts through behavioural alterations. Phil. Trans. R. Soc. B. 369, 20120580. doi: 10.1098/rstb.2013.0580
Brönmark C., Lakowitz T., Hollander J. (2011). Predator-induced morphological plasticity across local populations of a freshwater snail. PloS One 6, e21773. doi: 10.1371/journal.pone.0021773
Brönmark C., Pettersson L. B. (1994). Chemical cues from piscivores induce a change in morphology in crucian carp. Oikos 70, 396–402. doi: 10.2307/3545777
Brown G. E., Crane A. L., Demes E. E. M., Chivers D. P., Ferari M. C. O. (2022). Uncertain foraging opportunities and predation risk exert additive effects on induced neophobia in cichlids. Anim. Behav. 186, 21–28. doi: 10.1016/j.anbehav.2022.01.013
Brown G. E., Ferrari M. C. O., Chivers D. P. (2011). “Learning about danger: chemical alarm cues and threat-sensitive assessment of predation risk by fishes,” in Fish cognition and behaviour, 2nd ed. Eds. Brown C., Laland K. N., Krause J. (London: Blackwell), 59–80.
Brown G. E., Ferrari M. C. O., Elvidge C. K., Ramnarine I. W., Chivers D. P. (2013). Phenotypically plastic neophobia: a response to variable predation risk. Proc. R. Soc. Lond. B 280, 20122712. doi: 10.1098/rspb.2012.2712
Brusseau A., Feyten L. E. A., Crane A. L., Brown G. E. Exploring the effects of anthropogenic disturbance on predator inspection activity in Trinidadian guppies. Curr. Zool. doi: 10.1093/cz/zoad002
Candolin U. (2019). Mate choice in a changing world. Biol. Rev. 94, 1246–1260. doi: 10.1111/brv.12501
Candolin U., Rahman T. (2023). Behavioural responses of fishes to anthropogenic disturbances: adaptive value and ecological consequences. J. Fish Biol. doi: 10.1111/jfb.15322
Chivers D. P., Al-Batati F., Brown G. E., Ferrari M. C. O. (2013). The effect of turbidity on recognition and generalization of predators and non-predators in aquatic ecosystems. Ecol. Evol. 3, 268–277. doi: 10.1002/ece3.454
Chivers D. P., Kiesecker J. M., Marco A., Wildy E. L., Blaustein A. R. (1999). Shifts in life history as a response to predation in western toads (Bufo boreas). J. Chem. Ecol. 25, 2455–2463. doi: 10.1023/A:1020818006898
Chivers D. P., Zhao X. X., Brown G. E., Marchant T. A., Ferrari M. C. O. (2008). Predator-induced changes in morphology of a prey fish: the effects of food level and temporal frequency of predation risk. Evol. Ecol. 22, 561–574. doi: 10.1007/s10682-007-9182-8
Clark C. W. (1994). Anti-predator behaviour and the asset-protecdtion principle. Behav. Ecol. 5, 153–170. doi: 10.1093/beheco/5.2.159
Cooke S. J., Steinmetz J., Degner J. F., Grant E. C., Philipp D. P. (2003). Metabolic fright responses of different-sized largemouth bass Micropterus salmoides to two avian predators show variations in nonlethal energetic costs. Can. J. Zool 81, 699–709. doi: 10.1139/z03-044
Crane A. L., Ferrari M. C. O., Rivera-Hernández I. A. E., Brown G. E. (2020). Microhabitat complexity influences fear acquisition in fathead minnows. Behav. Ecol. 31, 261–266. doi: 10.1093/beheco/arz187
Crane A. L., Feyten L. E. A., Preagola A., Ferrari M. C. O., Brown G. E. Uncertainty in antipredator decisions: a conceptual review. Biol. Rev.
Dall S. R. X., Giraldeau L.-A., Olsson O., McNamara J. M., Stephens D. W. (2005). Information and its use by animals in evolutionary ecology. Trends Ecol. Evol. 20, 187–193. doi: 10.1016/j.tree.2005.01.010
Danchin E., Giraldeau L.-A., Valone T. J., Wagner R. H. (2004). Public information: from nosy neighbors to cultutal evolution. Science 305, 487–491. doi: 10.1126/science.1098254
Dugatkin L. A., Godin J.-G. J. (1992). Prey approaching predators: a cost-benefit perspective. Ann. Zool Fennici 29, 233–252.
Elvidge C. K., Brown G. E. (2014). Predation costs of impaired chemosensory risk assessment on acid-impacted juvenile Atlantic salmon (Salmo salar). Can. J. Fish Aquat Sci. 71, 756–762. doi: 10.1139/cjfas-2013-0633
Fernández-Juricic E., Deisher M., Stark A. C., Randolet J. (2012). Predator detection is limited in microhabitats with high light intensity: An experiment with brown-headed cowbirds. Ethology 118, 341–350. doi: 10.1111/j.1439-0310.2012.02020.x
Ferrari M. C. O., Sih A., Chivers D. P. (2009). The paradox of risk allocation: a review and prospectus. Anim. Behav. 78, 579–585. doi: 10.1016/j.anbehav.2009.05.034
Feyten L. E. A. (2023). Uncertainty of risk and information limitations shape neophobic antipredator responses in Trinidadian guppies.PhD thesis (Monteal, Quebec: Concordia University).
Feyten L. E. A., Brown G. E. (2018). Ecological uncertainty impacts vigilance as a marker of fear. Anim. Sentience. 15 (7).
Feyten L. E. A., Crane A. L., Ramnarine I. W., Brown G. E. (2021). Predation risk shapes the use of conflicting personal and social safety information in guppies. Behav. Ecol. 32, 1296–1305. doi: 10.1093/beheco/arab096
Fishman M. A. (1999). Predator inspection: Closer approach as a way to improve assessment of potential threats. J. Theor. Biol. 196, 225–235. doi: 10.1006/jtbi.1998.0834
Fraser D. F., Gilliam J. F. (1987). Feeding under predation hazard: Response of the guppy and Hart’s rivulus from sites with contrasting predation hazard. Behav. Ecol. Sociobiol. 21, 203–209. doi: 10.1007/BF00292500
Gaynor K. M., Brown J. S., Middleton A. D., Power M. E., Brashares J. S. (2019). Landscapes of fear: Spatial patterns of risk perception and response. Trends Ecol. Evol. 34, 355–368. doi: 10.1016/j.tree.2019.01.004
Godin J.-G. J. (1997). “Evading predators,” in Behavioural ecology of teleost fishes. Ed. Godin J.-G. J. (Oxford: Oxford University Press), 191–236.
Godin J.-G. J., Smith S. A. (1988). A fitness cost of foraging in the guppy. Nature 333, 69–71. doi: 10.1038/333069a0
Hale R., Piggott J. J., Swearer S. E. (2017). Describing and understanding behavioral responses to multiple stressors and multiple stimuli. Ecol. Evol. 7, 38–47. doi: 10.1002/ece3.2609
Hazlett B. A., Acquistapace P., Gherardi F. (2009). Responses of the crayfish orconectes virilis to chemical cues depend upon flow conditions. J. Crustacean Biol. 26, 94–98. doi: 10.1651/C-2595.1
Heathcote R. J. P., Darden S. K., Troscianko J., Lawson M. R. M., Brown A. M., Laker P. R., et al. (2018). Dynamic eye colour as an honest signal of aggression. Curr. Biol. 28, R635–R655. doi: 10.1016/j.cub.2018.04.078
Jakobsson S., Brick O., Kullberg C. (1995). Escalated fighting behaviour incurs increased predation risk. Anim. Behav. 49, 235–239. doi: 10.1016/0003-3472(95)80172-3
Johnson D. D. P., Blumstein D. T., Fowler J. H., Hasleton M. G. (2013). The evolution of error: error management, cognitive constraints, and adaptive decision making biases. Trends Ecol. Evol. 28, 474–481. doi: 10.1016/j.tree.2013.05.014
Jones K. A., Godin J.-G. J. (2010). Are fast explorers slow reactors: Linking personality type and anti-predator behaviour. Proc. R Soc. B 277, 625–632. doi: 10.1098/rspb.2009.1607
Katwaroo-Andersen J., Elvidge C. K., Ramnarine I. W., Brown G. E. (2016). Interactive effects of reproductive assets and ambient predation risk on the threat-sensitive decisions of Trinidadian guppies. Curr. Zool. 62, 221–226. doi: 10.1093/cz/zow062
Kendal R. L., Coolen I., van Bergen Y., Laland K. N. (2005). Trade-offs in the adaptive use of social and asocial leanring. Adv. Study Behav. 35, 333–379. doi: 10.1016/S0065-3454(05)35008-X
Kim J.-W., Grant J. W. A., Brown G. E. (2011). Do juvenile Atlantic salmon (Salmo salar) use chemosensory cues to detect and avoid risky habitats in the wild? Can. J. Fish Aquat Sci. 68, 655–662. doi: 10.1139/f2011-011
Koussoroplis A.-M., Wacker A. (2015). Covariance modulates the effect of joint temperature and food variance on ectotherm life-history traits. Ecol. Lett. 19, 143–152. doi: 10.1111/ele.12546
Krause J., Godin J.-G. J. (1996). Influence of prey foraging posture on flight behavior and predation risk: predators take advantage of unwary prey. Behav. Ecol. 7, 264–271. doi: 10.1093/beheco/7.3.264
Leduc A. O. H. C., Kim J.-W., Macnaughton C. J., Brown G. E. (2010). The sensory complement model helps predict diel alarm response patterns in juvenile Atlantic salmon (Salmo salar) under natural conditions. Can. J. Zool 88, 398–403. doi: 10.1139/Z10-016
Leduc A. O. H. C., Munday P. L., Brown G. E., Ferrari M. C. O. (2013). Effects of acidification on olfactory-mediated behaviour in freshwater and marine ecosystems: a synthesis. Phil Trans. R Soc. B 368, 20120447. doi: 10.1098/rstb.2012.0447
Leduc A. O. H. C., Roh E., Brown G. E. (2009). Effects of acid rainfall on juvenile Atlantic salmon (Salmo salar) antipredator behaviour: loss of chemical alarm function and potential survival consequences during predation. Mar. Freshw. Res. 60, 1223–1230. doi: 10.1071/MF08323
Lima S. L., Bednekoff P. A. (1999). Temporal variation in danger drives antipredator behaviour: the predation risk allocation hypothesis. Am. Nat. 153, 649–659. doi: 10.1086/303202
Lima S. L., Dill L. M. (1990). Behaivoral decisions made under the risk of predation: a review and prospectus. Can. J. Zool 68, 619–640. doi: 10.1139/z90-092
Luttbeg B., Ferrari M. C. O., Blumstein D. T., Chivers D. P. (2020). Safety cues can give prey more valuable information than danger cues. Am. Nat. 195, 636–648. doi: 10.1086/707544
Magnhagen C. (1991). Predation risk as a cost of reproduction. Trends Ecol. Evol. 6, 183–186. doi: 10.1016/0169-5347(91)90210-O
McCarthy M. S., Després-Einspenner M.-L., Samuni L., Mundy R., Lemoine S., Preis A., et al. (2018). An assessment of the efficacy of camera traps for studying demographic composition and variation in chimpanzees (Pan todlodytes). Am. J. Pimatol 80, e22904. doi: 101002/ajp.22904
Munoz N. E., Blumstein D. T. (2012). Multisensory perception in uncertain environments. Behav. Ecol. 23, 457–462. doi: 10.1093/beheco/arr220
Norberg E. J., Schwarzkopf L. (2022). Afraid of the dard? The influence of natural and artificial light at night on the behavioral activity of a nocturnal gecko. Front. Ecol. Evol. 10, 821335. doi: 10.3389/fevo.2022.821335
O’Brien A. L., Dafforn K. A., Cariton A. A., Johnston E. L., Mayer-Pinto M. (2019). After decades of stessor research in urban estuine ecosystems the focus is still on single stessors: A systematic literature review and meta-analysis. Sci. Tot Environ. 684, 753–764. doi: 10.1016/j.scitotenv.2019.02.131
Preisser E. L., Bolnick D. I. (2008). When predators don’t eat their prey: Nonconsumptive predator effects on prey dynamics. Ecology 89, 2414–2415. doi: 10.1890/08-0522.1
Preisser E. L., Bolnick D. I., Benard M. F. (2005). Scared to death? The effects of intimidation and consumption in predator-prey interactions. Ecology 86, 501–509. doi: 10.1890/04-0719
Reinhardt U. G., Healey M. C. (1999). Size-dependent foraging behaviour and use of cover in juvenile Coho salmon under predation risk. Can. J. Zool 75, 1642–1651. doi: 10.1139/z97-791
Ruxton G. D., Sherratt T. N., Speed M. P. (2004). Avoiding attack. The evolutionary ecology of crypsis, warning signals, and mimicry (Oxford: Oxford University Press).
Sih A. (1994). Predation risk and the evolutionary ecology of reproductive behaviour. J. Fish Biol. 45 (Suppl. A), 111–130. doi: 10.1111/j.1095-8649.1994.tb01087.x
Sih A., Bell A. M. (2008). Insights for behavioral ecology from behavioral syndromes. Adv. Study Behav. 38, 227–281. doi: 10.1016/S0065-3454(08)00005-3
Sih A., Ziemba R., Harding K. C. (2000). New insights on how temporal variation in predation risk shapes prey behavior. Trends Ecol. Evol. 15, 3–4. doi: 10.1016/S0169-5347(99)01766-8
Venter O., Grant J. W. A., Noël M. V., Kim J.-W. (2008). Mechanisms underlying the incease in young-of-the-year Atlantic salmon (Salmo salar) density with habitat complexity. Can. J. Fish Aquat Sci. 65, 1956–1964. doi: 10.1139/F08-106
Weissburg M., Smee D. L., Ferner M. C. (2014). The sensory ecology of nonconsumptive predator effects. Am. Nat. 184, 141–157. doi: 10.1086/676644
Zanette L. Y., Clinchy M. (2019). Ecology of fear. Curr. Biol. 29, R309–R313. doi: 10.1016/j.cub.2019.02.042
Keywords: predator-prey interactions, behavioural decision making, anthropogenic stressor, risk assessment cues, ecological uncertainty
Citation: Brown GE and Godin J-GJ (2023) Ecological uncertainty and antipredator behaviour: an integrative perspective. Front. Ethol. 2:1238167. doi: 10.3389/fetho.2023.1238167
Received: 10 June 2023; Accepted: 10 July 2023;
Published: 26 July 2023.
Edited by:
Rulon Clark, San Diego State University, United StatesReviewed by:
Gazzola Andrea, University of Pavia, ItalyCopyright © 2023 Brown and Godin. This is an open-access article distributed under the terms of the Creative Commons Attribution License (CC BY). The use, distribution or reproduction in other forums is permitted, provided the original author(s) and the copyright owner(s) are credited and that the original publication in this journal is cited, in accordance with accepted academic practice. No use, distribution or reproduction is permitted which does not comply with these terms.
*Correspondence: Grant E. Brown, Z3JhbnQuYnJvd25AY29uY29yZGlhLmNh