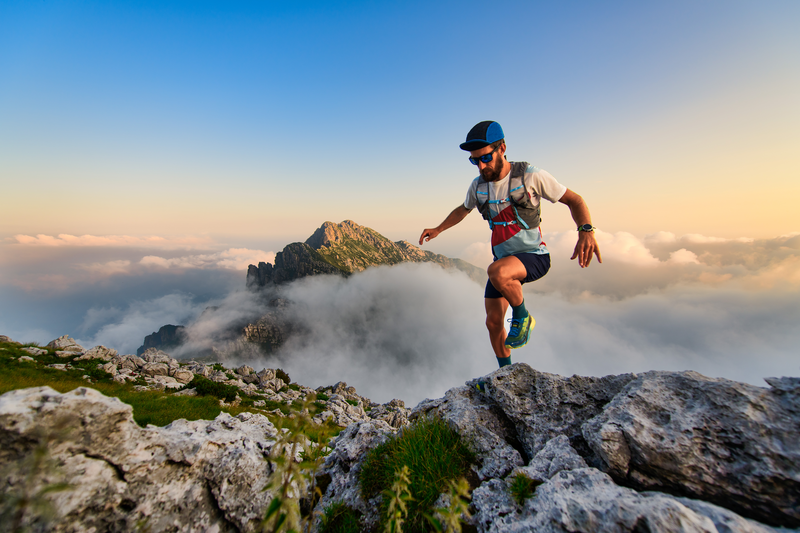
94% of researchers rate our articles as excellent or good
Learn more about the work of our research integrity team to safeguard the quality of each article we publish.
Find out more
BRIEF RESEARCH REPORT article
Front. Epidemiol.
Sec. Infectious Disease Epidemiology
Volume 5 - 2025 | doi: 10.3389/fepid.2025.1518559
This article is part of the Research Topic HTLV-1 and EBV-related disorders: pathogenesis and clinical advances View all 6 articles
The final, formatted version of the article will be published soon.
You have multiple emails registered with Frontiers:
Please enter your email address:
If you already have an account, please login
You don't have a Frontiers account ? You can register here
The probability of presenting with infectious mononucleosis (IM) upon primary Epstein-Barr virus infection increases dramatically at the start of puberty. Aiming to understand why that is, we assessed whether the number of infection-related health events during two specific time periodsages 10-12 years (pre-teen window) and the three most recent years (recent window)could predict the likelihood of individuals aged 13-19 years developing IM.We used sibship-stratified Cox regression to mitigate socio-demographic confounding and bias. Consequently, we only followed members of IM-affected sibships aged 13 to 19 years between 1999 and 2021 for IM, based on information from complete nationwide Danish administrative and health registers. Estimates were further adjusted for sex, age, birth order (1, 2, 3+) and sibship constellation (number of siblings and their signed [older/younger] age difference to the index person). Infection-related health events defining the exposures considered were either a category of antimicrobial prescription, or a hospital contact with an infectious disease diagnosis. We measured evidence/probability of the associations using asymptotic Bayes factors, rather than using p-value based testing.The adjusted hazard ratio (HR) for IM with 95% confidence limits for an additional antimicrobial prescription in the pre-teen exposure window was [1.01; 0.98-1.04], and the corresponding adjusted HR for an additional antimicrobial prescription in the recent exposure window was [1.02;0.99-1.06].Conclusions: IM was not preceded by unusual numbers of infections. Small effect sizes, together with small variation in exposure, did not render the assessed exposures useful for predicting IM for public health or the clinic.
Keywords: Epstein-Barr virus, Immune System, Infections, Infectious Mononucleosis, Puberty, Bayes factor
Received: 28 Oct 2024; Accepted: 28 Feb 2025.
Copyright: © 2025 Rostgaard, Kristjánsson, Davidsson, Biel-Nielsen Dietz, Søegaard, Stensballe and Hjalgrim. This is an open-access article distributed under the terms of the Creative Commons Attribution License (CC BY). The use, distribution or reproduction in other forums is permitted, provided the original author(s) or licensor are credited and that the original publication in this journal is cited, in accordance with accepted academic practice. No use, distribution or reproduction is permitted which does not comply with these terms.
* Correspondence:
Klaus Rostgaard, Danish Cancer Society, Copenhagen, Denmark
Disclaimer: All claims expressed in this article are solely those of the authors and do not necessarily represent those of their affiliated organizations, or those of the publisher, the editors and the reviewers. Any product that may be evaluated in this article or claim that may be made by its manufacturer is not guaranteed or endorsed by the publisher.
Research integrity at Frontiers
Learn more about the work of our research integrity team to safeguard the quality of each article we publish.