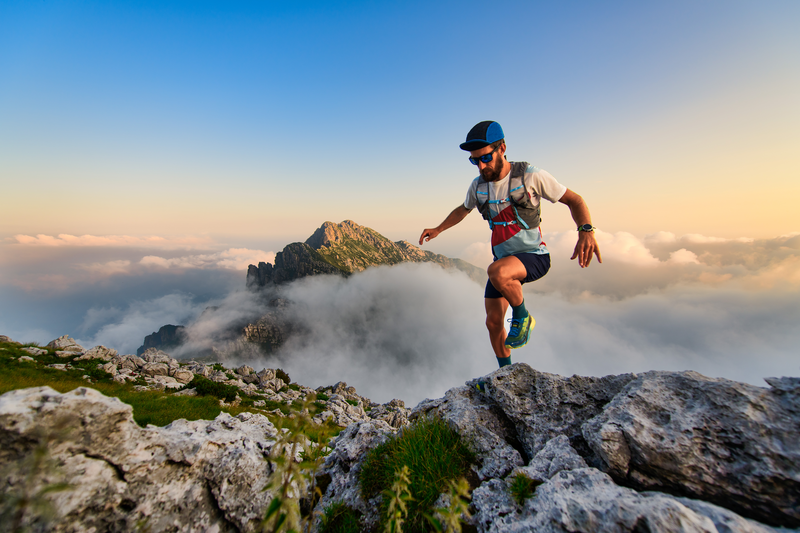
94% of researchers rate our articles as excellent or good
Learn more about the work of our research integrity team to safeguard the quality of each article we publish.
Find out more
ORIGINAL RESEARCH article
Front. Environ. Sci.
Sec. Toxicology, Pollution and the Environment
Volume 13 - 2025 | doi: 10.3389/fenvs.2025.1566507
This article is part of the Research Topic Life in the "Plastisphere": Linking the Biodiversity of Microbial Communities to the Risk of Micro-(nano-)plastics and Related New Contaminants View all articles
The final, formatted version of the article will be published soon.
You have multiple emails registered with Frontiers:
Please enter your email address:
If you already have an account, please login
You don't have a Frontiers account ? You can register here
Recent studies suggest that paper cups may also contribute to environmental pollution, particularly through the release of microplastics (MPs). The Nile River, one of the world's most vital water sources, faces alarming contamination levels, raising concerns about its ecological health. This study investigated whether paper cups release MPs, ions, and heavy metals into water and assessed the potential impact of MPs on fish. In order to completely comprehend the nature and scope of the issue, one liter of water was collected from the Nile River in Assiut, Egypt and the paper cups were ripped into tiny pieces. Paper cups were similarly soaked in similar volumes of distilled and tap water. Four months later, the leachate from each trail (three replicates for each) was analyzed to determine and compare the distribution of specific ions, heavy metals and microplastics. In order to clarify the availability of MPs in freshwater fish, the intestines of two common fish species (Oreochromis niloticus and Bagrus bajad) were collected from the River Nile in Assiut and examined. Polyethylene, polystyrene and polypropylene were the three main forms of microplastics identified in water samples from the Nile. Also, paper cups soaked in tap water leached the same three groups of MPs, but in lower amounts. Some microplastics may take longer to biodegrade in water, as evidenced by the absence of other forms of microplastics like rayon and polyvinyl chloride in any of the water samples under investigation. The present findings also indicate a noteworthy accumulation of MPs in the intestines of Oreochromis niloticus and Bagrus bajad. In conclusion, these results indicated release of some ions, heavy metals, and microplastics from paper cups into water and the River Nile water is polluted with paper cups which have a negative effect on aquatic organisms. This study brings us one step closer to investigating and fully understanding the nature and extent of the problem posed by paper cups and their effects on the River Nile and freshwater fish, which will ultimately be reflected in human health risks.
Keywords: Paper cups, Leachates, Microplastics, River Nile, Assiut City, fish
Received: 24 Jan 2025; Accepted: 11 Mar 2025.
Copyright: © 2025 Eid, Mahmoud and Sayed. This is an open-access article distributed under the terms of the Creative Commons Attribution License (CC BY). The use, distribution or reproduction in other forums is permitted, provided the original author(s) or licensor are credited and that the original publication in this journal is cited, in accordance with accepted academic practice. No use, distribution or reproduction is permitted which does not comply with these terms.
* Correspondence:
Alaa El-Din H. Sayed, Assiut University, Assiut, Egypt
Disclaimer: All claims expressed in this article are solely those of the authors and do not necessarily represent those of their affiliated organizations, or those of the publisher, the editors and the reviewers. Any product that may be evaluated in this article or claim that may be made by its manufacturer is not guaranteed or endorsed by the publisher.
Research integrity at Frontiers
Learn more about the work of our research integrity team to safeguard the quality of each article we publish.