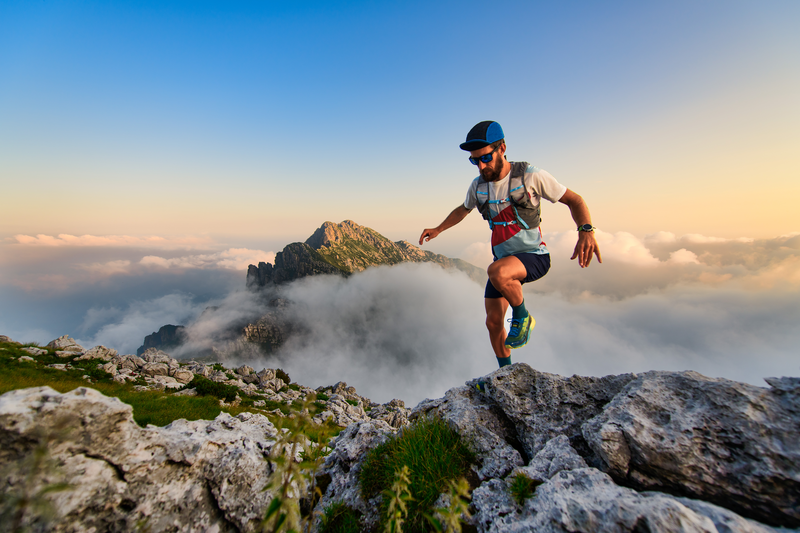
95% of researchers rate our articles as excellent or good
Learn more about the work of our research integrity team to safeguard the quality of each article we publish.
Find out more
ORIGINAL RESEARCH article
Front. Environ. Sci. , 07 April 2025
Sec. Environmental Economics and Management
Volume 13 - 2025 | https://doi.org/10.3389/fenvs.2025.1555798
Accomplishing the coordination between economy and environment requires a series of efficient measure so that the Potter effect can be realized. This study, from the perspective of market-government coordination, empirically examines the Porter effect of China’s carbon emissions trading policy using a difference-in-differences approach. The findings reveal that: (1) The carbon emissions trading policy has significantly reduced local carbon emissions by 78.6%, increased regional green total factor productivity by 32.7%, and enhanced scientific and technological innovation by 43.9%, thereby demonstrating a pronounced and strong Porter effect. Robustness checks, including placebo tests and Propensity Score Matching-Difference in Differences estimation, confirmed the validity of these results. (2) Market mechanisms, as measured by carbon prices and market liquidity, only partially facilitate the realization of the Porter effect under the policy. (3) The stronger the administrative intervention by local governments, the more pronounced the policy’s Porter effect. Based on these findings, this study proposes the following policy recommendations: to achieve the dual carbon goals, the government should further expand the carbon emissions trading policy and progressively establish a unified national carbon trading market with cross-sector participation. Additionally, policymakers should address market failures in environmental governance, uphold the synergy between market mechanisms and administrative interventions, thereby fostering a virtuous cycle between environmental protection and economic development.
The process of reform and openness has delivered substantial economic benefits to China, leading to the phenomenon of the China economic miracle. China’s economy has quickly grown over 4 decades of reform and openness. However, this rapid development model has concurrently resulted in significant environmental pollution and ecological decline. As the world’s leading emitter of CO2, China faces formidable challenges in reducing said emissions while advancing industrialization and urbanization. Environmental pollution is a consistent concern across all sectors of society (Tu and Chen, 2013). The 19th Party Congress report emphasizes that building an ecologically balanced society is a key millennium strategy for the nation’s sustained advancement. It is imperative to instill the principle that verdant landscapes and clean waters hold the same value as revenue, as well as to adhere to the state policy of conserving resources and safeguarding the environment (Ren et al., 2019). On examining China’s history in air pollution control, it seems clear that the carbon emissions trading policy (CETP) has played a substantial role in curbing rising energy consumption and greenhouse gas emissions. Since 2011, China has implemented the CETP across seven provinces and cities, namely, Beijing, Tianjin, Shanghai, Chongqing, Shenzhen, Hubei, and Guangdong. The primary objective of this initiative is to accumulate a synergistic approach—leveraging market mechanisms—to managing greenhouse gas emissions. Additionally, it aims to enhance the efficacy of carbon and pollution reduction efforts, drawing from advanced practices and insights (Zhang et al., 2014; Pang and Duan, 2016). Simultaneously, China’s economic expansion has tended to rely on substantial inputs, high energy consumption, and elevated pollution levels. This underscores the need to focus more heavily on core technologies and bolster international competitiveness. In recent years, China’s industrial productivity has experienced a decline, thus impeding overall macroeconomic growth. The 19th CPC National Congress report emphasizes the urgency of shifting toward quality, efficiency, and new energy sources, all while enhancing total factor productivity, so as to achieve a superior, more efficient, and sustainable form of economic development (Cai, 2017). Therefore, in the context of increasingly severe environmental challenges and the pursuit of high-quality development, the influence of environmental regulations on total factor productivity has emerged as a pivotal subject within economics research. In terms of ascertaining whether the CETP can effectively reduce carbon emissions and bolster total factor productivity to realize the Porter effect, existing studies have primarily investigated said effect through isolated examinations of the CETP’s impact on total factor productivity or scientific and technological innovation (STI) (Hu et al., 2023; Ren et al., 2019). Notably, the existing literature lacks comprehensive evaluations of the CETP’s Porter effect on pilot provinces by considering such factors as carbon emission reduction, efficiency enhancement, and advancements in STI in an integrated manner.
Furthermore, unlike the developed nations of North America and Europe (Nabernegg et al., 2019), China’s CETP was launched at a later stage, primarily targeting state-owned enterprises (SOEs). This delay has resulted in various issues, such as legislative delays and underdeveloped market mechanisms (Liu et al., 2015; Zhao et al., 2016). However, existing research indicates that despite these challenges, China’s CETP continues to wield a significant impact on the designated pilot regions (Zhao et al., 2019). This implies that, aside from market mechanisms, other factors may influence the pilot regions through the CETP—which current research has yet to adequately explain. Given the predominance of China’s traditional administrative, intervention-based environmental regulatory tools, the preponderance of SOEs among the CETP’s pilot participants, and the CETP’s relatively weak policy mechanisms, it is reasonable to hypothesize that compliant subjects in the pilot areas are motivated by two primary factors. Indeed, they respond to the market-based incentives embedded in the CETP, and are subject to government-administered controls. The latter may be reflected in the direct supervision of compliance through administrative intervention (e.g., monitoring behavior in the relevant environmental responsibility assessment system or formulating corresponding administrative penalties for non-compliance). The market characteristics of the current emission control subjects, mostly SOEs, provide the government with a realistic basis for effectively supervising compliance (Wu et al., 2021). In order to verify this speculation, this paper further studies the mechanism of the CETP’s Porter effect based on the synergistic effect of the market mechanism and administrative interventions based on existing research.
In sum, while an increasing number of studies have begun to focus China’s CETP—with scholars examining it from various angles and yielding a wealth of research findings—there are notable theoretical and empirical gaps regarding the robustness of the CETP’s Porter hypothesis. These studies have generated inconclusive results, meaning that there is a shortage of comprehensive research regarding the CETP’s influence on the Porter effect in pilot provinces, taking into account such factors as green total factor productivity (GTFP) and STI. Accordingly, there is a need to further deepen theoretical and empirical research in this area. Moreover, the methodologies that have previously been used require refinement. Based on this, the potential marginal contributions of this paper are as follows: (1) While existing studies have often adopted a single perspective, this paper comprehensively analyzes the strong version of the Porter hypothesis by integrating carbon emissions, GTFP, and STI. (2) This paper innovatively examines the strong Porter hypothesis from the perspective of both “market mechanisms” and “administrative regulations.” It incorporates key carbon trading indicators, such as carbon price and market liquidity, into the policy evaluation model to assess the effectiveness of the CETP in promoting the Porter effect in pilot provinces. Additionally, it uses such indicators as the proportion of SOEs to measure the government’s regulatory capacity, thereby evaluating the impact of administrative intervention on the CETP’s Porter effect.
The relationship between environmental regulation and economic growth has long been a contentious topic in academic research. According to neoclassical economics, while environmental regulation can improve environmental quality, it also increases production costs for enterprises, thereby reducing their international competitiveness and negatively impacting economic growth (Tu and Chen, 2015). However, the Porter hypothesis posits that well-designed environmental regulation policies can incentivize technological innovation, enhance environmental performance and competitiveness, and achieve a win-win outcome for both environmental protection and economic growth (Ambec et al., 2013). The Porter hypothesis provides a theoretical framework for studying the interplay between environmental regulation, environmental protection, efficiency, and competitiveness. Scholars have since validated its effects from the perspectives of environmental impact and technological innovation (Tu and Chen, 2015). Jaffe and Palmer (1997) further advanced the strong Porter hypothesis, arguing that environmental regulation not only stimulates innovation, but also enhances corporate competitiveness. Previous studies have often used GTFP as a comprehensive indicator of corporate competitiveness to explore how it is impacted by environmental regulation. However, the findings remain inconclusive: some studies have suggested that environmental regulation positively promotes GTFP (Hamamoto, 2006; Shen et al., 2019), while others have indicated a negative impact (Gray and Shadbegian, 1995; Lanoie et al., 2008). Additionally, other scholars have argued that there is no definitive relationship between the two (Ferjani and Gray, 2011).
As a form of environmental regulation policy, the CETP, implemented in seven regions of China since 2011, has become a focal point of research. Existing studies have demonstrated that this policy has achieved positive outcomes in various aspects, including carbon emission reduction (Zhang et al., 2017), technological advancement (Chen et al., 2021), improved production efficiency (Yang et al., 2021), and the optimization of corporate investments (Qi et al., 2023). Furthermore, Jiang et al. (2022) analyzed the impact of the industry coverage of the carbon trading system on total oil consumption using a computable general equilibrium (CGE) model. They found that covering all high oil-consuming industries can lead to the most significant reductions in total oil consumption and external dependence, providing valuable insights for the design of carbon trading systems. However, most existing studies have focused on a single dimension, failing to comprehensively analyze its Porter effect. Jiang et al. (2023) further highlighted that incorporating the co-benefits of air quality into carbon emission reduction task allocation can significantly enhance regional emission reduction efficiency and offer a more comprehensive perspective for policy design.
In this context, green technological innovation has emerged as a critical driver for enhancing environmental performance and corporate competitiveness. As the primary force behind green development and the ultimate solution for achieving carbon neutrality, green technological innovation is pivotal. However, due to the externalities inherent in technology and financial markets, market incentives for environmentally beneficial technological innovations are often insufficient, making policy intervention essential. In contrast to generalized science and technology policies, the Porter hypothesis posits that well-designed environmental policies can incentivize enterprises to engage in green technological innovation, thereby achieving both environmental protection and improved corporate competitiveness.
Therefore, the formulation of rational environmental policies is crucial for guiding and motivating innovation entities to pursue and disseminate green technological innovations. As a significant environmental policy, can the CETP promote the Porter hypothesis in the pilot provinces? What are the mechanisms through which it influences the Porter effect? Existing research has yet to provide a comprehensive explanation. Given that most pilot regions have integrated special funds to support major low-carbon development projects and established mechanisms for public participation in green consumption within their implementation plans, it is reasonable to hypothesize that the pilot provinces, driven by government guidance and societal participation, have actively invested in low-carbon technologies and promoted green and low-carbon transitions, thereby realizing the Porter effect. To validate this hypothesis, this study builds on existing research and examines the specific impact of the CETP on the Porter effect in the pilot provinces from the perspective of the synergistic interaction between government intervention and market mechanisms.
Jaffe and Palmer (1997) categorized the Porter hypothesis into three distinct versions: the “weak” version claims that environmental regulation stimulates eco-innovation, although the net impact of regulation and innovation on corporate benefits remains uncertain in direction and magnitude; the “narrow” version emphasizes that flexible environmental policy instruments are more effective than command-and-control regulations in incentivizing corporate innovation; and the “strong” version asserts that well-designed regulations can induce innovations sufficient to fully offsetting compliance costs, potentially enhancing corporate productivity. This study focuses on examining the CETP’s strong Porter effect and explores the influence of market mechanisms and administrative interventions on this effect. Figure 1 presents the theoretical framework of the research.
The CETP assigns emissions rights as tradable commodities in an open market. The implementation of this policy can positively impact carbon emission reduction. Research by both domestic and international scholars has substantiated the carbon emission reduction benefits of the CETP (Zhang et al., 2023; Liu et al., 2019). The CETP operates through a specific mechanism, whereby the government initially allocates carbon emission quotas to different enterprises. Subsequently, it permits the free trade of carbon emission rights in the market. Surplus carbon emission allowances are rewarded to technology-oriented enterprises through subsidies, while resource-oriented enterprises with insufficient carbon emission allowances are subject to taxation. This arrangement serves to incentivize and promote carbon emission reduction effects. Since the inception of the CETP, while the mechanism’s construction remains an ongoing process, the persistent expansion of trading volume and market liquidity underscores its active role in facilitating value discovery. This, in turn, offers a practical foundation for the pilot provinces to secure emission reduction benefits for the primary trading entities. Consequently, the study posits the following hypothesis:
H1. Implementing the CETP can effectively promote carbon emission reduction in pilot provinces.
Traditional economists have contended that escalating environmental costs can adversely impact a company’s productivity and competitive edge (Jorgenson and Wilcoxen, 1990). However, the Porter hypothesis suggests that this only holds true when firms remain entirely passive and static. Given that businesses inherently strive for profitability, they tend to adopt a more proactive stance in response to environmental regulations. Under the pressure of such regulations, enterprises often focus on enhancing production efficiency. They achieve this by optimizing resource allocation, refining production processes, and driving technological innovation. These efforts not only bolster their position in the product market by reducing carbon intensity per unit, but also align with the requirements of total emission control within the emissions rights trading framework. Moreover, the reduction incentives offered by carbon emissions trading mechanisms prompt firms to further optimize resource allocation or upgrade their processes. This, in turn, enhanced GTFP (Hu et al., 2023). In contrast to other control-command-style environmental regulations, the CETP, being market-driven, presents notable advantages. One key advantage is that the environmentally responsible actions taken by enterprises not only enable them to meet the total emissions control stipulations within the carbon market and lower their carbon emissions costs, but also to capitalize on the surplus carbon emission allowances by trading and selling them in the carbon market, thereby realizing additional emission reduction benefits (Clarkson et al., 2015). The rise in potential benefits serves to diminish the positive external impact associated with enterprises’ emission reduction efforts. This serves to more cohesively align the costs and benefits of emission reduction within enterprises (Matisoff, 2010). When examining the allocation of enterprise resources, it is generally assumed that management efficiently allocates available resources with the overarching objective of maximizing the enterprise’s value. Nonetheless, as previously discussed, the implementation of an emissions trading mechanism can impact enterprises’ current production efficiency. In this context, by enhancing production efficiency to decrease the carbon emissions per unit of production, businesses not only reap the usual benefits associated with improved productivity, but can also generate additional profits through the sale of excess carbon emission allowances in the carbon emissions trading market. Consequently, under the influence of the carbon emissions trading mechanism, enterprise management is more incentivized to optimize resource allocation or upgrade production processes, thereby enhancing the overall productivity of enterprises in the pilot provinces (Hu et al., 2023). Accordingly, the study posits the following:
H2. Implementing the CETP has helped enhance GTFP in the pilot provinces.
In recent years, China’s environmental regulation has gradually shifted from administrative intervention to market incentives in support of global climate action objectives (Wu et al., 2021). The CETP has the capacity to encourage businesses to proactively cut carbon emissions and abatement expenses. This can be achieved by enhancing production efficiency, optimizing resource allocation, and prioritizing the adoption of clean energy sources. In line with the Porter hypothesis, environmental regulations can stimulate enterprises to engage in green technological innovation, thereby reaping the dual benefits of carbon emission reduction and economic development. In theoretical terms, the CETP has the potential to incentivize enterprises to advance various carbon technologies, including those with lower carbon emissions, those with zero carbon emissions, and even carbon-negative technologies that can offset and absorb the emissions necessarily generated during the production process. This can reduce the expenditures associated with the purchase of carbon emissions rights or generating additional income by selling surplus emission rights. Furthermore, considering the escalating carbon emission reduction targets set by China in recent years, the market value of carbon credits has steadily increased. Consequently, the CETP has evolved into a growing incentive for enterprises’ investments into non-negative and negative carbon technologies (Cao and Su, 2023). Consequently, the following hypothesis is proposed:
H3. Implementing the CETP will help enhance the level of STI in the pilot provinces.
Prior research has uncovered significant effects of the CETP within the designated regions, including substantial reductions in carbon emissions (Zhang et al., 2023; Liu et al., 2019), enhancements in production efficiency (Jun Hu et al., 2023), and a boost in STI (Chen et al., 2021). This paper also posits that the CETP can indeed yield a strong Porter effect. However, when considering the broader context of China’s CETP, the carbon market system has yet to be fully established. There exist substantial disparities in carbon prices among different pilot regions, suggesting a degree of inflexibility. Additionally, the trading dynamics within the CETP display distinctive characteristics. Given that China’s carbon market system remains incomplete and at a relatively nascent stage of development, government oversight is crucial to ensure that the market, which is currently operating inefficiently, maintains a high level of compliance with emission control standards. Researchers aiming to analyze the operational dynamics of the CETP during its developmental phase must consider both market mechanisms and administrative interventions. Presently, the CETP’s emission reduction mechanism functions through two distinct pathways. The first involves the carbon trading market system, which is centered around price mechanisms. The second entails government oversight to ensure contractual compliance through administrative interventions, thus ensuring the market’s smooth operation. Through the combined influence of administrative intervention and market mechanisms, it is imperative to apply more robust administrative control measures within the pilot market to compensate for its inherent inefficiencies and ensure the CETP’s effective implementation. The effective coordination between the government and the market is pivotal in establishing the carbon trading market. China’s carbon market has yet to meet the fundamental criteria of efficiency, resulting in a lack of a functioning price mechanism. The government plays a vital role in addressing these market failures. Furthermore, the primary objective of the CETP is to commodify emission rights, enabling carbon prices to mirror the marginal cost of corporate emissions. Through this market-driven mechanism, it allows high- and low-efficiency enterprises to participate in carbon trading, thereby enhancing resource use efficiency. This, in turn, empowers the CETP to stimulate carbon emission reductions, improve productivity, and elevate STI levels within pilot regions. As such, the study proposes the following hypotheses.
H4. Local governments are incentivized to offset the operational inefficiencies of the carbon market through administrative intervention. Consequently, carbon emissions trading entities can operate efficiently within a less developed carbon market environment, thereby advancing the attainment of the Porter hypothesis effect.
H5. In the presence of both market mechanisms and administrative intervention, as operational efficiency diminishes within the carbon market, the extent of government control over emission management becomes a key determinant in the magnitude of the CETP-induced Porter effect.
Before using the difference-in-differences (DID), the parallel trend test must first be satisfied, meaning that the CO2 emissions, business performance, and carbon reduction-efficiency synergy index of the energy industry in both pilot and non-pilot regions should exhibit the same trend. Based on this, and following the practices found in the general literature, this paper took the year of policy implementation as the benchmark and used the event study method to test the parallel trends before the CETP. Figure 1 reports the results of the parallel trend test and dynamic effects (Beck et al., 2010). Before the policy’s implementation, the coefficients for all years were neither significant nor showed a significant trend, indicating that the pre-policy trends were essentially parallel. The results of the parallel trend indicate that, in the years prior to the CETP, there were no significant differences in the CO2 emissions, business performance, and carbon reduction-efficiency synergy index between the experimental and control groups, thus satisfying the parallel trend assumption.
The examination of the CETP-induced Porter effect hinges on a comprehensive grasp of the concept of policy impact assessment, which entails evaluating the outcomes of policy implementation based on corresponding criteria and employing scientifically sound technical evaluation methodologies. Policy impact is often gauged by contrasting sample characteristics before and after policy implementation. However, this approach is limited by a need to account for inherent fluctuations within the sample itself. Moreover, some studies assess a policy’s effectiveness by comparing the samples it directly affects. Nevertheless, this method overlooks the disparities in the initial samples. Both of these approaches fall short of capturing the pure effects of a policy.
Princeton University initially introduced the DID model in 1985. Scholars have since been dedicated to developing and enhancing this research model, whose fundamental concept involves creating time and group dummy variables for policy implementation, along with interaction terms between them. This approach effectively mitigates the impact of other factors, resulting in a more accurate assessment of policy outcomes. The DID model has proven to be highly fruitful in econometric analysis, offering the advantage of addressing endogeneity issues and measuring policy increments. The basic form of the DID model is depicted in Equation 1:
In Equation 1, the subscripts i and t represent the region and year, respectively. The variable y signifies the explanatory variable. DID represents the core explanatory variable. The variable du differentiates between the experimental and control groups, where it equals 1 and 0 for the treatment and control groups, respectively. The variable dt distinguishes between periods before and after policy implementation, equaling 0 before the intervention and 1 after. DID represents the interaction term, equal to 1 for the sample in the treatment group after the policy intervention, and 0 otherwise. Finally, ε represents a disturbance term.
Selecting the most suitable research method is crucial for measuring GTFP. In the existing literature, scholars have predominantly employed the Solow residual, SFA (stochastic frontier analysis), and DEA (data envelopment analysis) methods for measurement. After evaluating the distinctions among these research methods and considering the specifics of this paper, including its research subjects, samples, and objectives, it was imperative to opt for the most appropriate measurement technique for empirical research. Given the multi-input and -output data, including non-desired outputs in this study, the DEA method’s advantages made it the most suitable choice. Furthermore, prior researchers have noted that the SBM (super-efficiency-based data envelopment analysis) model within the DEA framework is better suited for studying China’s total factor productivity. Within this framework, the super-efficient SBM model (an enhanced version of the SBM) is capable of evaluating the effective decision-making units more accurately. Additionally, this research takes both resource and environmental factors into account, addressing the limitations of the traditional Malmquist-Lenberger (ML) index when dealing with non-desired outputs. It should be noted that the ML index can encounter difficulties in solving linear programming problems when measuring the inter-period directional distance function, and it lacks transmissibility and circular cumulativeness. Conversely, the geometric mean logarithmic loss (GML) index exhibits transmissibility, circularity, and the capability for inter-period comparisons. Consequently, this paper employed the GML index to assess the alteration and breakdown of GTFP within the mining industry, using the super-efficient SBM model as the foundation.
Tone, (2001) introduced a non-radial, non-angular SBM model that relies on relaxation vectors. In this scenario, assuming there are n decision-making units (DMUs), the input and output indicator matrices for these are represented as
In Equation 2, ρ* (0 < ρ* < 1) represents the efficiency value within the SBM model, signifying the assessed DMU. In Equation 3, S- ≥ 0 and S+> 0 represent the input and output slacks, respectively. Meanwhile, xλ and yλ refer to the input and output quantities along the frontier, respectively.
The traditional SBM model measures efficiency up to 1, which does not allow for comparisons between efficient DMUs. However, Tone, (2001) introduced enhanced the original model by proposing the super-efficient SBM model, which calculates efficiency values for all DMUs and facilitates comparisons between efficient DMUs. The formula for the super-efficient SBM model is structured as Equations 4, 5:
As the tension between resources, the environment, and the economy becomes increasingly apparent, scholars have recognized the significance of sustainable development in their studies on the quality of economic growth. To enhance the measurement of economic growth quality, the super-efficient SBM model considers the inclusion of non-desired outputs.
Using the super-efficient SBM model as a foundation, each DMU encompasses not only input and expected output vectors, but also non-desired output vectors. These are designated as the input, desired output, and non-desired output vectors, denoted as
In Equation 6, α* denotes the efficiency measure for the evaluated DMU, whereas λ indicates the importance assigned to each DMU in Equation 7. Nevertheless, this model is limited to yielding a singular static efficiency value, which represents a one-time evaluation. This outcome, on its own, lacks comprehensiveness. Accordingly, we employed the GML productivity index to compute the GTFP growth rate, capturing the dynamic efficiency alterations.
Since the inception of the ML index by Chung et al. (1997) and its subsequent adoption in GTFP measurement by various researchers, it has become a prevalent method. Nevertheless, the ML index carries clear limitations. Firstly, it employs a geometric mean formulation, potentially leading to situations where linear programming cannot find a solution when assessing the inter-period directional distance function. Secondly, it lacks transmissibility and cyclic cumulativity. In contrast, Oh (2010) introduced the GML productivity index, which is founded on the production possibilities of the entire domain. This index possesses the desirable qualities of being transmissible, cyclic, and amenable to inter-period comparisons. The specific mathematical expression for this index is provided in Equation 8:
When GMLt+1 > 1, it signifies an increase in GTFP, indicating that the desired output in the production process is growing or the undesired output is diminishing. Conversely, a value of less than 1 indicates a decline in GTFP. When GMLt+1 = 1, GTFP remains constant. Furthermore, the GML index can be broken down into two components: technical progress change (TC) and technical efficiency change (EC). The decomposition formula for these components is presented in Equation 9:
EC can be further subdivided into two distinct components: pure technical efficiency (PEC) and scale efficiency (SEC). PEC represents the efficiency of production influenced by such factors as management and technology, while SEC signifies the efficiency of production linked to scale-related factors. The specific formula expression is provided in Equation 10:
When EC > 1, it signifies that the respective DMU has moved closer to the production frontier compared to the previous period, indicating an improvement in technical efficiency. Conversely, when EC < 1, a decrease in technical efficiency is implied. When EC = 1, the technical efficiency remains unaltered.
If TC > 1, it denotes that the technical level of this DMU has advanced in comparison to the prior period; conversely, it has regressed when TC < 1. When TC = 1, the technical level remains static.
To accurately gauge efficiency and productivity, it is imperative to carefully choose relevant input-output data, as exemplified by this paper’s GTFP measurement. The computation of the GTFP index necessitates the consideration of factor input, desired output, and non-desired output indicators. Among these, the factor input indicators primarily encompass such metrics as the year-end urban workforce count, fixed capital investments, and total energy consumption. Notably, the capital stock is commonly estimated using the perpetual inventory method, and this estimation process incorporates such data points as initial capital stock, new fixed assets, and the rate of capital depreciation (Li and Bai, 2018). The desired output is represented by the GDP at constant 2004 prices, while total energy consumption signifies the non-desired output. The production function is formally defined in Equation 11:
K represents capital inputs, L represents labor inputs, E represents energy inputs, Y represents desired outputs, and U represents undesired outputs.
This study employed the widely accepted IPCC reference method to compute CO2 emissions stemming from fossil fuel usage in Chinese provinces between 2004 and 2020. To evaluate GTFP within the mining industry, we employed a combined approach using the SBM-GML model through MaxDEA software. This approach yields results that offer the advantage of inter-period comparisons. The level of STI within each province was measured through internal research and development (R&D) funding.
Given the strong connection between carbon emissions and carbon intensity with economic development, it is imperative to account for the varying economic development characteristics within the treatment and control groups. This adjustment ensures that the carbon emissions of both groups are comparable. In this paper, we selected certain control variables to assess the diverse attributes of regional economic development. These include: (1) Industrial composition, particularly the contribution of the secondary industry’s value added to GDP (strind); (2) Extent of external engagement, as represented by foreign direct investment (strwz); (3) Level of market development, quantified by the market index (market index); and (4) Fiscal reliance, indicated by the ratio of local public budget revenue to GDP (strpub).
We employed two metrics, carbon trading price and market liquidity, to assess the state of carbon trading, serving as proxies for the market mechanism. Specifically, the carbon trading price was calculated as the logarithmic transformation of the annual average of daily closing prices (price), while market liquidity was determined as the logarithmic transformation of the annual count of non-zero trading days (liqui). Moreover, we considered government control to be synonymous with administrative intervention. Therefore, an indicator reflecting the extent of government control can also serve as a measure of the level of administrative intervention. Given that the primary entities responsible for emissions control in the pilot area are predominantly SOEs (Zhao et al., 2016), as well as that the government possesses greater potential control over such enterprises compared to their non-state-owned counterparts (e.g., by linking compliance behavior of emissions control entities with the SOEs’ performance appraisal and evaluation system), this paper used the proportion of SOEs (strgygz) as a measure of administrative intervention. The proportion of fixed assets of SOEs in industrial enterprises above the designated size precisely measures the proportion of SOEs. In addition, taking into account that the higher the government’s share in the initial distribution of the national economy, the closer the government’s relationship with market players, and the stronger the market players can be controlled, we selected the degree of fiscal dependence (strpub) instead of the proportion of SOEs to conduct a robustness test.
This paper evaluates the Porter effect of the CETP using panel data from 30 provinces in China between 2004 and 2020. Carbon emission data were sourced from the China Carbon Emission Accounting Database (Chen et al., 2021), the marketization index from the China Sub-Province Marketization Index Report, the carbon price was taken from the China Carbon Emission Trading Network.,and other data were come from the China Statistical Yearbook and China Industrial Statistical Yearbook of the past years. Descriptive statistics were analyzed as shown in Table 1.
Meeting the parallel trends assumption is a prerequisite when employing the DID method. Essentially, this assumption dictates that, prior to policy implementation, the experimental and control groups must exhibit similar trends. This alignment is crucial because it implies that the control group’s trend can serve as a suitable counterfactual for estimating what might have happened to the experimental group had it not been impacted by the policy. Failing to meet the parallel trends assumption can result in the DID method either overestimating or underestimating the policy’s effect. Accordingly, we conducted a parallel trend analysis between the experimental and control groups to validate the accuracy of our DID estimation results. Additionally, following Beck’s (2010) approach, we generated a graphical representation of the parallel trend test, as illustrated in Figure 2. The findings showed that the coefficient representing the difference between the control and experimental groups consistently hovered around zero before the CETP’s implementation. This indicates that the experimental and control groups maintained a roughly parallel growth trajectory. Consequently, we could reasonably compare these two groups, confirming their compliance with the parallel trend test. Furthermore, it should be noted that the selected indicators in this paper met the assumed conditions for parallel trends.
The DID model is a fixed effects estimation method based on quasi-natural experiments, and most studies apply the DID estimate regression parameters by controlling for time and individual effects. Hence, we also adopted the DID to estimate the Porter effect of the CETP.
Table 2 shows the outcomes of the baseline regressions outlined in Equation 13, where all regressions account for year-fixed effects, region-fixed effects, and region-by-year interaction effects. In Table 2, Columns (1), (3), and (5) do not include the control variables, while these are included in Columns (2), (4), and (6). Upon reviewing Table 2, it becomes apparent that, when solely considering year-fixed effects, region-fixed effects, and region-year interaction effects, the DID coefficient related to CO2 emissions is notably and significantly negative at the 1% confidence level, with a coefficient value of −0.786. Furthermore, the DID coefficients influencing GTFP and STI are also significantly negative, reaching the 1%–5% confidence level, with coefficient values of 0.327 and 0.439, respectively. Upon introducing control variables, the DID coefficient linked to CO2 emissions remains significantly negative at the 1% confidence level, with a coefficient value of −1.259. Similarly, the DID coefficients affecting GTFP and STI continue to exhibit significance, albeit at the 1%–10% confidence level, with coefficient values of 0.257 and 0.243, respectively. It can thus be inferred that the CETP concurrently achieves a reduction in CO2 emissions within the pilot area, while also fostering enhancements in both GTFP and the level of STI in the same region. This substantiates a robust manifestation of the CETP’s Porter effect, thereby validating hypotheses H1, H2, and H3. Therefore, we can posit that the CETP policy effectively promotes carbon emission reduction, enhances production efficiency, and boosts technological innovation levels in the pilot provinces. The primary reasons for this are as follows: (1) By setting a cap on total carbon emissions and allocating quotas, the policy incentivizes enterprises to adopt emission reduction measures. Firms can trade carbon emission quotas in the market, allowing those with lower abatement costs to profit from selling surplus quotas, while those with higher costs are motivated to reduce emissions to lower expenses, thereby directly driving carbon emission reduction (Liu et al., 2019). (2) The CETP policy increases the cost of carbon emissions for enterprises, compelling them to optimize resource allocation, gradually eliminate outdated production capacities, and adopt more efficient production technologies and equipment, thereby improving production efficiency (Hu et al., 2023). (3) The policy also encourages enterprises to increase investment in R&D in such areas as low-carbon technologies and clean energy, thereby fostering collaboration between industry, academia, and research institutions, which, in turn, elevates the level of technological innovation in the pilot provinces (Hu et al., 2020).
To further examine whether this study’s outcomes were influenced by unobservable factors at the province-industry-year level, we conducted a placebo test wherein the pilot provinces were randomly assigned, with 6 of the 30 provinces designated as the treatment group. It was assumed that these six provinces had implemented the CETP, with the remainder of the country serving as the control group. Through this random allocation, it could be ensured that the independent variables constructed in this research exerted no impact on CO2 emissions, GTFP, and STI. In simpler terms, any noteworthy discovery would suggest that the regression findings presented in this study may have been influenced by bias. To address this concern, we performed 500 random samples and executed benchmark regressions. Figure 3 illustrates the average values of the regression estimates across these 500 random assignments. Notably, the mean values of all estimated coefficients were revealed to be almost zero. Furthermore, Figure 3 plots the distribution of the 500 estimated coefficients alongside their corresponding p-values. It is evident that these distributions are tightly clustered around zero, with the majority of estimates possessing p-values greater than 0.1. Simultaneously, the actual estimates related to CO2 emissions, GTFP, and STI stood out as clear anomalies in the placebo test. Taken together, these findings strongly suggest that the estimates presented in this paper are unlikely to have been influenced by unobservable factors specific to the province-industry-year context.
To ensure the accuracy of the regression outcomes and account for inter-province variability’s impact on the results, we employed the PSM-DID method for a robustness examination. Initially, the indicator signifying the treatment group (Treat = 1) served as the dependent variable. Covariates such as the share of value added by the secondary industry in GDP (strind), foreign direct investment (strwz), marketization index (marketindex), and the proportion of local general public budget revenue to GDP (strpub) were taken into consideration. For the matching process, we opted for the non-replacement method employing Mahalanobis distance matching to ensure that matched observations would exhibit similarity across all dimensions. Table 3 presents the standard support test results for all variables both before and after propensity score matching, while Table 4 showcases the outcomes of the PSM-DID analysis.
The study employed PSM-DID as a robustness test for the model, with the finding reaffirming the earlier conclusions. The results of the PSM-DID test once again validated the notion that the CETP in China concurrently reduces CO2 emissions within the pilot area, while also fostering enhancements in both GTFP and STI levels. This reaffirms the presence of a strong Porter hypothesis effect resulting from the CETP.
The process of reforming environmental regulation policies in China is intricate and ever-evolving. It frequently unfolds alongside a multitude of concurrent economic policies and government regulations that run in parallel. This intricate landscape can complicate efforts to accurately pinpoint the specific impacts of the CETP. In an effort to bolster the robustness of our estimation results, we sought to isolate the influence of other pertinent policies. Beyond the CETP, the introduction of the Ecological Civilization Pilot Area policy in 2013 is another factor affecting the pilot regions. To ensure the exclusion of any interference stemming from these related policies, we chose to omit Guizhou, Jiangxi, and Fujian provinces from the DID test. The regression findings demonstrate that, even after excluding the impact of these concurrent policies, the regression coefficient for the DID variable DID remained statistically significant. Consequently, the benchmark regression results in this paper were substantiated as robust. Table 5 shows the relevant regression results.
The preceding benchmark regression and robustness findings suggest that the CETP has a notable and consistent impact: it significantly reduces CO2 emissions while concurrently boosting GTFP and STI in the pilot areas. These well-supported empirical conclusions serve to substantiate a strong Porter effect within these pilot provinces. However, it should be noted that the Porter effect observed in the CETP’s impact on the pilot regions may not be solely attributable to the functioning of the market mechanism. This is due to the fact that the development of the CETP in China is not yet fully mature, and the market mechanism may not be functioning optimally. To assess the extent to which the pilot regions achieve the Porter effect through the market mechanism, we adjusted Equation 1 as follows:
Within these variables, perfit serves as an indicator to assess the effectiveness of the market mechanism. Specifically, it takes into account such factors as carbon pricing and market liquidity, with θ denoting its associated coefficient. When including both DIDit and its interaction with the perfit term simultaneously, the coefficient β1 signifies the absence of any market mechanism impact on the CETP. This could occur, for instance, if the carbon price were set at zero. On the other hand, θ represents the degree of heterogeneity in carbon emission reduction effects resulting from carbon trading. θ stands as a pivotal coefficient for gauging the effectiveness of the market mechanism. The significance of the remaining symbols aligns with that in Equation 1.
Table 6 presents the pertinent regression findings. First, when evaluating the market mechanism using carbon prices for CO2 emissions, the coefficient θ exhibited no statistically significant negative correlation at the 10% confidence level. However, it is notable that the absolute value of the coefficient β1 diminished and achieved statistical significance at the 1%–5% confidence level. This suggests that the CETP significantly drives CO2 emission reduction through means other than the market mechanism. Regarding GTFP, the coefficient θ was notably positive and reached statistical significance at the 1% confidence level. Additionally, the absolute value of the coefficient β1 decreased from 0.257 to 0.112, suggesting that the carbon trading price can indeed significantly enhance GTFP. Nonetheless, the CETP continues to significantly boost GTFP through avenues beyond the market mechanism. In the context of STI, the coefficient θ exhibited a substantial and statistically significant positive effect at the 1% confidence level. Furthermore, the absolute value of the coefficient β1 notably declined from 0.243 to 0.0337. This indicates that the carbon trading price can indeed significantly elevate STI. Nevertheless, it must be emphasized that the CETP continues to play a significant role in advancing STI through avenues that extend beyond the market mechanism. Second, when assessing the market mechanism using market liquidity for CO2 emissions, the coefficient θ registered as statistically significant and exhibited a negative correlation at the 10% confidence level. Furthermore, while the absolute value of the coefficient β1 diminished, it remained statistically significant at the 10% confidence level. This implies that higher market liquidity can indeed significantly augment the CETP’s carbon emission reduction impact. Nevertheless, it ought to be highlighted that the CETP continues to significantly reduce carbon emissions through means other than the market mechanism. When considering GTFP, the coefficient θ exhibited no statistically significant negative correlation at the 10% confidence level. However, it is noteworthy that the coefficient β1 does decrease from 0.257 to 0.211. While the coefficient θ, assessing the influence of market liquidity on GTFP, does not reach statistical significance, it is notable that the absolute value of β1 decreases. This suggests that the market mechanism, as gauged by market liquidity, may have some degree of effectiveness. In terms of STI, although the coefficient θ was positive, it did not achieve statistical significance. Nonetheless, the absolute value of coefficient β1 decreased and was statistically significant at the 1% confidence level. This implies that the carbon market substantially contributes to elevating levels of STI through mechanisms that extend beyond typical market channels.
Based on the empirical findings presented above, several key conclusions can be drawn. Indeed, the CETP’s Porter effect in the pilot regions can be partially attributed to the market mechanism centered around carbon trading. The carbon trading price exerts a significant influence on both GTFP and STI within the pilot provinces. Additionally, market liquidity plays a role in shaping carbon emission reductions in the pilot regions. However, it must be borne in mind that the CETP’s Porter effect in the pilot areas also originates from non-market mechanisms distinct from carbon trading.
Considering that this effect has origins beyond carbon trading, a pertinent question arises: Is this non-market mechanism closely linked to the extent of government administrative intervention in the market? In order to examine hypotheses H4 and H5, we made the following adjustments to Equation 12:
In these modifications, govit functions as the metric for gauging government administrative intervention, encapsulating such elements as fiscal dependence and the proportion of SOEs. The coefficient β1 corresponds to this metric. Furthermore, DIDit × govit represents the interaction term between the DID variable and the extent of administrative intervention. The coefficient θ linked to this term is vital to assessing whether the influence of administrative intervention impacts the carbon abatement effects of the carbon market. The meanings of the remaining symbols remain consistent with those outlined in Equation 13. The pertinent regression results are presented in Table 7.
Table 7. Regression results of the impact of the proportion of state-owned enterprises and fiscal dependence on the Porter effect of CETP.
In the context of CO2 emissions, GTFP, and STI, the interaction terms involving the DID variables and the percentage of SOEs all exhibited statistical significance, ranging from the 1%–10% confidence level. This implies that, as the proportion of SOEs increases, the CETP’s Porter effect in the pilot region strengthens. Essentially, this indicates that a more pronounced government capacity to regulate the market corresponds to a more significant Porter effect of the CETP in the pilot region. Substituting the proportion of SOEs with fiscal dependence, the regression coefficients of the pertinent interaction terms continued to exhibit statistical significance, falling within the 1%–10% confidence range. This ensured that the research outcomes remain unaltered. Furthermore, the DID variable can be substituted with carbon trading variables, such as carbon price and market liquidity, which provide a more comprehensive set of information. The regression findings showed that the regression coefficients related to the interaction between carbon trading variables and the proportion of SOEs were statistically significant within the 5%–10% confidence level. This further affirms that, within the collaborative framework of market mechanisms and administrative intervention, when the operational efficiency of the CETP decreases, the magnitude of government control over the main body responsible for emission control becomes a crucial factor. As this government control intensifies, the Porter effect of the CETP becomes more pronounced. Table 8 shows the relevant regression results.
Table 8. Further regression results on the synergistic Porter effect of market mechanisms and administrative intervention.
In sum, the empirical test results presented above demonstrate that the CETP does indeed manifest the Porter effect within the pilot area. However, the current carbon trading market mechanism plays a limited role in fostering this effect. On the other hand, the CETP’s Porter effect can be amplified effectively by the government actively exercising control over the market.
Consequently, the empirical results presented in this study corroborate that the current pilot regions within China’s CETP can indeed achieve the Porter effect. This accomplishment is predicated on the collaborative interplay between market mechanisms and administrative interventions, effectively incentivizing compliance among entities responsible for emission control. It should be highlighted that, while both the CETP and emissions trading fall within the realm of market-based environmental regulation, the energy-saving impact of China’s emissions trading pilot exhibits a notably positive correlation with the level of marketization (Shi et al., 2020). In contrast to emissions trading, which commenced its pilot phase in 2007, the policy implemented in these pilot regions had a later start date and a shorter implementation period. Furthermore, the construction of the corresponding market mechanisms still needs to be completed. Consequently, during the initial stages of development, the CETP still heavily relies on administrative intervention. However, as the CETP and its associated system within these regions continue to mature and gradually evolve, its Porter effect is anticipated to progressively intensify in the future.
Leveraging China’s inter-provincial panel data from 2004 to 2020, this paper used the DID method to assess the Porter effect arising from the CETP. Robustness tests were conducted through the use of a placebo test, PSM-DID analysis, and by excluding concurrent related policies. The empirical findings consistently demonstrated that the CETP substantially fosters carbon emission reduction within the pilot provinces. Additionally, it enhances GTFP and STI levels in these regions. These outcomes affirm the presence of a robust Porter effect attributed to the CETP. Secondly, based on the synergistic perspective of the market mechanism and administrative intervention, we explained and examined the CETP’s Porter effect from both theory and practice. Our findings suggest that the market mechanism has a limited impact on the Porter effect’s CETP, while regional administrative intervention can significantly enhance it. Accordingly, the current CETP in the pilot region is realized through the synergistic effect of the market mechanism and administrative intervention. Hence, the execution of the CETP should effectively use both market forces and government intervention. It is essential to proactively advance the development of the CETP while also maximizing the government’s role. Considering these findings, the paper offers the following policy suggestions.
The pilot policy has significantly improved carbon reduction, GTFP, and STI in the pilot regions. As such, the government would do well to further promote the expansion of the CETP and gradually establish a unified national carbon trading market with participation across industries so as to enhance its Porter effect. Financial institutions should be encouraged to participate in the carbon market, attracting a diverse range of market participants and gradually integrating high-energy-consuming industries into the trading system so as to improve market liquidity. Additionally, the carbon pricing mechanism should be refined by introducing carbon price adjustment tools to mitigate excessive price volatility and strengthen firms’ long-term market expectations.
While market mechanisms can partially enhance the Porter effect, administrative intervention plays a more critical role in significantly amplifying it. Therefore, the implementation of the CETP should effectively leverage both market forces and government intervention, ensuring a coordinated approach. Policymakers should avoid a “one-size-fits-all” approach by dynamically adjusting the intensity of administrative interventions (e.g., allowance allocation and regulatory enforcement) based on real-time market data, such as carbon prices and liquidity. Additionally, a regionally differentiated strategy should be adopted, with increased policy support and technology transfer initiatives tailored to underdeveloped regions in central and western China.
This study has several limitations. First, although we employed multiple methods (e.g., Difference-in-Differences, Propensity Score Matching-DID) to rigorously estimate the effects of the CETP policy, our analysis relied solely on provincial-level data due to the policy’s implementation at the provincial scale. Future research should extend this work by incorporating city-level data to capture localized impacts. Second, we focused on the direct effects of the CETP policy but did not explore its potential spatial spillover effects, which represents an avenue for further investigation.
Publicly available datasets were analyzed in this study. This data can be found here: https://libproxy.cug.edu.cn/https/443/cn/com/epsnet/olap/yitlink/#/datas_data?cubeId=891.
SK: Writing – original draft, Writing – review and editing, Data curation, Methodology. ZY: Data curation, Investigation, Writing – review and editing. LT: Conceptualization, Formal Analysis, Funding acquisition, Visualization, Writing – review and editing.
The author(s) declare that no financial support was received for the research and/or publication of this article.
The authors declare that the research was conducted in the absence of any commercial or financial relationships that could be construed as a potential conflict of interest.
The author(s) declare that no Generative AI was used in the creation of this manuscript.
All claims expressed in this article are solely those of the authors and do not necessarily represent those of their affiliated organizations, or those of the publisher, the editors and the reviewers. Any product that may be evaluated in this article, or claim that may be made by its manufacturer, is not guaranteed or endorsed by the publisher.
Ambec, S., Cohen, M. A., Elgie, S., and Lanoie, P. (2013). The Porter hypothesis at 20: can environmental regulation enhance innovation and competitiveness? Rev. Environ. Econ. Pol. 7, 2–22. doi:10.1093/reep/res016
Beck, T., Levine, R., and Levkov, A. (2010). Big bad banks? The winners and losers from bank deregulation in the United States. J. Finance 65 (5), 1637–1667. doi:10.1111/j.1540-6261.2010.01589.x
Cai, F. (2017). Analysis of China’s economic reform effects – the perspective of labor reallocation. Econ. Res. 52 (07), 4–17.
Cao, X., and Su, X. (2023). Does the carbon emission trading pilot policy promote carbon neutral technological innovation? China Population-Resources Environ. 33, 94–104. 07.
Chen, Z., Zhang, X., and Chen, F. (2021). Do carbon emission trading schemes stimulate green innovation in enterprises? Evidence from China. Technol. Forecast. Soc. Change. 168, 120744. doi:10.1016/j.techfore.2021.120744
Chung, Y. H., Färe, R., and Grosskopf, S. (1997). Productivity and undesirable outputs: a directional distance function approach. J. Environ. Manag. 51 (3), 229–240. doi:10.1006/jema.1997.0146
Clarkson, P. M., Li, Y., Pinnuck, M., and Richardson, G. D. (2015). The valuation relevance of greenhouse gas emissions under the European Union carbon emissions trading scheme. Eur. Acc. Rev. 24 (3), 551–580. doi:10.1080/09638180.2014.927782
Ferjani, A. (2011). Environmental regulation and productivity: a data envelopment analysis for Swiss dairy farms. Agric. Econ. Rev.
Gray, W. B., and Shadbegian, R. (1995). in Pollution abatement costs, regulation, and plant-level productivity (USA: National Bureau of Economic Research Cambridge, Mass.).
Hamamoto, M. (2006). Environmental regulation and the productivity of Japanese manufacturing industries. Res. Energ. Econ. 28 (4), 299–312. doi:10.1016/j.reseneeco.2005.11.001
Hu, J., Fang, Q., and Long, W. (2023). Carbon emission regulation, corporate emission reduction incentives and total factor productivity: a natural experiment based on China’s carbon emission trading mechanism. Econ. Res. 58 (04), 77–94.
Hu, J., Nan, H., and Shen, H. T. (2020). Can market-incentive environmental regulation promote corporate innovation? A natural experiment based on China’s carbon emissions trading mechanism. J. Finance. Res. 1, 171–189.
Jaffe, A. B., and Palmer, K. (1997). Environmental regulation and innovation: a panel data study. Rev. Econ. Stat. 79 (4), 610–619. doi:10.1162/003465397557196
Jiang, H. D., Liu, L. J., Dong, K., and Fu, Y. W. (2022). How will sectoral coverage in the carbon trading system affect the total oil consumption in China? A CGE-based analysis. Energ. Econ. 110, 105996. doi:10.1016/j.eneco.2022.105996
Jiang, H. D., Purohit, P., Liang, Q. M., Liu, L. J., and Zhang, Y. F. (2023). Improving the regional deployment of carbon mitigation efforts by incorporating air-quality co-benefits: a multi-provincial analysis of China. Ecol. Econ. 204, 107675. doi:10.1016/j.ecolecon.2022.107675
Jorgenson, D. W., and Wilcoxen, P. J. (1990). Environmental regulation and US economic growth. Rand. J. Econ. 21, 314–340. doi:10.2307/2555426
Lanoie, P., Patry, M., and Lajeunesse, R. (2008). Environmental regulation and productivity: testing the porter hypothesis. J. Prod. Anal. 30, 121–128. doi:10.1007/s11123-008-0108-4
Li, W., and Bai, Y. (2018). Can environmental regulation trigger the “innovation compensation” effect? – a game analysis based on Porter’s hypothesis. Audit. Econ. Res. 33 (06), 103–111.
Liu, L., Chen, C., Zhao, Y., and Zhao, E. (2015). China’s carbon-emissions trading: overview, challenges and future. Renew. Sust. Energ. Rev. 49, 254–266. doi:10.1016/j.rser.2015.04.076
Liu, , et al. (2019). Research on the effect of carbon emission reduction policy in China’s carbon emissions trading pilot[J]. China Population, Resour. Environ. 29 (11), 49–58.
Matisoff, D. C. (2010). Making cap-and-trade work: lessons from the European Union experience. Environ. Sci. Policy. Sustain. Dev. 52 (1), 10–19. doi:10.1080/00139150903479530
Nabernegg, S., Bednar-Friedl, B., Muñoz, P., Titz, M., and Vogel, J. (2019). National policies for global emission reductions: effectiveness of carbon emission reductions in international supply chains. Ecol. Econ. 158, 146–157. doi:10.1016/j.ecolecon.2018.12.006
Oh, D. (2010). A global Malmquist-Luenberger productivity index. J. Prod. Anal. 34, 183–197. doi:10.1007/s11123-010-0178-y
Pang, T., and Duan, M. S. (2016). Cap setting and allowance allocation in China’s emissions trading pilot programmes: special issues and innovative solutions. Clim. Policy. 16 (7), 815–835. doi:10.1080/14693062.2015.1052956
Qi, Y., Yuan, M., and Bai, T. (2023). Where will corporate capital flow to? Revisiting the impact of China’s pilot carbon emission trading system on investment. J. Environ. Manag. 336, 117671. doi:10.1016/j.jenvman.2023.117671
Ren, S., Zheng, J., Liu, D., and Chen, X,. (2019). Whether emissions trading mechanisms improve firms’ total factor productivity – evidence from listed companies in China. China. indust. Econ. (05), 5–23. doi:10.19581/j.cnki.ciejournal.2019.05.001
Shen, N., Liao, H., Deng, R., and Wang, Q. (2019). Different types of environmental regulations and the heterogeneous influence on the environmental total factor productivity: empirical analysis of China’s industry. J. Clean. Prod. 211, 171–184. doi:10.1016/j.jclepro.2018.11.170
Shi, B., Li, N., Gao, Q., et al. (2022). Market incentives, carbon quota allocation and carbon emission reduction: evidence from China’s carbon trading pilot policy [J]. J. Environ. Manage. 319, 115650. doi:10.1016/j.jenvman.2022.115650
Tone, K. (2001). A slacks-based measure of efficiency in data envelopment analysis. Eur. J. Oper. Res. 130 (3), 498–509. doi:10.1016/s0377-2217(99)00407-5
Tu, Z., and Chen, R. (2013). Study on the dynamic marginal carbon emissions of industrialization and urbanization – an analytical framework based on the LMDI “two-tier complete decomposition method.”. China. indust. Econ. (09), 31–43.
Tu, Z., and Chen, R. (2015). Can emissions trading mechanism realize the Porter effect in China? Econ. Res. 50 (07), 160–173. doi:10.19581/j.cnki.ciejournal.2013.09.004
Wu, Y., Qi, J., Xin, Q., and Chen, J., (2021). Study on carbon emission reduction effect of China’s carbon market-based on the synergistic perspective of market mechanism and administrative intervention. China. indust. Econ. (08), 114–132. doi:10.19581/j.cnki.ciejournal.2021.08.007
Yang, L., Li, Y., and Liu, H. (2021). Did carbon trade improve green production performance? Evidence from China. Energ. Econ. 96. doi:10.1016/j.eneco.2021.105185
Zhang, D., Karplus, V. J., Cassisa, C., and Zhang, X. (2014). Emissions trading in China: progress and prospects. Energ. Pol. 75, 9–16. doi:10.1016/j.enpol.2014.01.022
Zhang, Y., Liu, Y., and Zhao, J. (2023). Research on the effect of environmental regulation on carbon emission reduction – a quasi-natural experiment based on the pilot carbon emissions trading policy. Ecol. Econ. 39 (07), 29–44.
Zhang, Y. J., Peng, Y. L., Ma, C. Q., and Shen, B. (2017). Can environmental innovation facilitate carbon emissions reduction? Evidence from China. Energ. Pol. 100, 18–28. doi:10.1016/j.enpol.2016.10.005
Keywords: CETP, porter effect, market mechanism, administrative intervention, DID
Citation: Kebei S, Yaxin Z and Tongping L (2025) A study on the porter effect of China’s carbon emissions trading policy. Front. Environ. Sci. 13:1555798. doi: 10.3389/fenvs.2025.1555798
Received: 08 January 2025; Accepted: 24 March 2025;
Published: 07 April 2025.
Edited by:
Guoxiang Li, Nanjing Normal University, ChinaReviewed by:
Hong-Dian Jiang, China University of Geosciences, ChinaCopyright © 2025 Kebei, Yaxin and Tongping. This is an open-access article distributed under the terms of the Creative Commons Attribution License (CC BY). The use, distribution or reproduction in other forums is permitted, provided the original author(s) and the copyright owner(s) are credited and that the original publication in this journal is cited, in accordance with accepted academic practice. No use, distribution or reproduction is permitted which does not comply with these terms.
*Correspondence: Zhang Yaxin, emhhbmd5YXhpbkBjdWcuZWR1LmNu
Disclaimer: All claims expressed in this article are solely those of the authors and do not necessarily represent those of their affiliated organizations, or those of the publisher, the editors and the reviewers. Any product that may be evaluated in this article or claim that may be made by its manufacturer is not guaranteed or endorsed by the publisher.
Research integrity at Frontiers
Learn more about the work of our research integrity team to safeguard the quality of each article we publish.