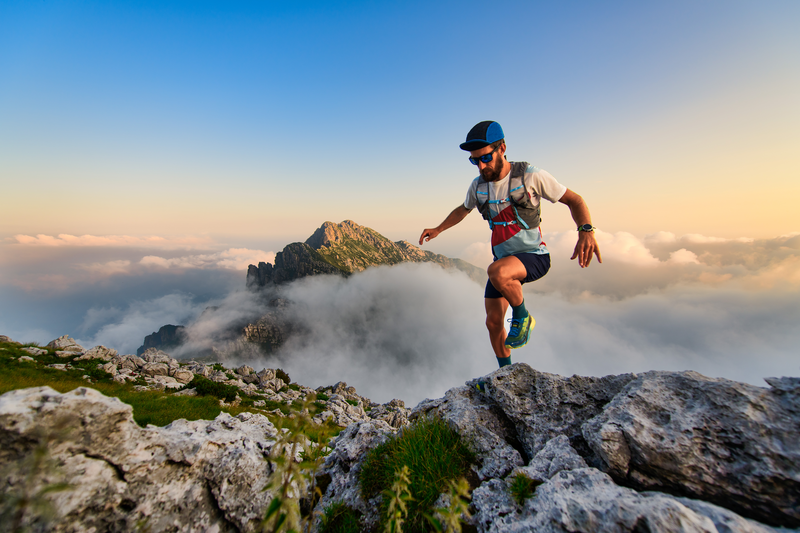
95% of researchers rate our articles as excellent or good
Learn more about the work of our research integrity team to safeguard the quality of each article we publish.
Find out more
ORIGINAL RESEARCH article
Front. Environ. Sci. , 26 March 2025
Sec. Soil Processes
Volume 13 - 2025 | https://doi.org/10.3389/fenvs.2025.1555622
Introduction: Tobacco, as an economic crop in our country, not only requires changes in cultivation practices but also benefits from reasonable fertilization to enhance yield and quality. Microbial organic fertilizers (MOFs) have received considerable attention in recent years; however, the relationship between the rhizosphere soil microorganisms of tobacco plants and the physicochemical properties of soil with MOFs remains poorly understood.
Methods: This study established experimental and control groups, employing a five-point sampling method to collect samples of the rhizosphere soil and tobacco leaves at four different growth stages. High-throughput 16S rRNA gene sequencing was conducted to analyze the microbial community structure, while the physicochemical properties of the soil and the physical characteristics of the tobacco leaves were also examined.
Results and Discussion: The results indicated that MOF reduced the uptake of ammonium nitrogen by tobacco at different growth stages, promoted the dispersion of the microbial community, and significantly altered the soil microbial community structure. At the phylum level, Actinobacteriota and Proteobacteria are the first and second dominant bacterial phyla in the soil microbial community. Significantly, during the prosperous growth stage of tobacco, the relative abundance of Actinobacteriota (14.90%) in the control group was lower than that of Proteobacteria (19.87%), whereas in the experimental group, the relative abundance of Actinobacteriota (22.99%) was higher than that of Proteobacteria (22.06%). Furthermore, an assessment of tobacco leaf morphology, yield, and quality demonstrated that the application of MOF resulted in a 21.93% increase in leaf yield and a 27.38% increase in yield value per unit area. Furthermore, nicotine and nitrogen content in the tobacco leaves slightly decreased. In summary, this study demonstrates that MOF can improve soil microbial communities and enhance the quality and yield of tobacco leaves, providing valuable insights into the effects of MOF on altering the physicochemical properties of tobacco soil and improving leaf quality.
The Asia-Pacific region is among the world’s largest producers and consumers of tobacco, with China recognized as one of the leading tobacco-growing countries (Su et al., 2020). Tobacco is a crucial economic crop in our nation, occupying approximately one-third of the global cultivation area (Zou et al., 2018), thereby playing an essential role in national development. It acts as a safeguard for national fiscal revenue while simultaneously promoting economic and social advancement. The use of fertilizers has significantly contributed to global agricultural prosperity (Jez et al., 2016) and has facilitated advancements in the tobacco cultivation sector. However, in their pursuit of higher yields, many tobacco farmers apply large quantities of fertilizers over extended periods. The excessive use of inorganic fertilizers can lead to various issues (Dang et al., 2022), including disruption of soil structure, alterations in soil physicochemical properties, and disturbances in the soil microbial ecological balance (Rahman et al., 2021a). Soil is home to a diverse array of microbial species that perform vital functions (Abed et al., 2013). Growing evidence highlights the critical roles of rhizosphere microorganisms in crop growth and development. Soils with appropriate microbial community structures are essential for robust plant growth and development (Van Bruggen et al., 2019).
In agricultural cultivation, the physicochemical properties of soil and the composition of soil microbial communities significantly influence crop appearance and quality (Zhang et al., 2012). As known, fertilization can alter soil microbial diversity and community composition, which in turn affects soil enzyme activity (Juan et al., 2008). Meanwhile, the investigation of soil microbial structure is vital under different cropping systems. Studies have demonstrated that continuous monoculture can lead to the occurrence of crop rotation obstacles, thereby disrupting the diversity of the soil microbial community (Zhou et al., 2014). Traditional fallow practices may slightly ameliorate degraded soils but could also result in nutrient leaching and the potential dissemination of pathogens within the soil (Ali et al., 2019). Cultivating plant diversity, such as through intercropping, companion planting, and relay cropping, can enhance ecosystem health and promote agricultural sustainability (Ali et al., 2021; Ghani et al., 2022). In China, tobacco cultivation is typically conducted through crop rotation systems. Crop rotation has been shown to enhance bacterial diversity and nitrogen cycling in tobacco soils, while also improving the growth efficiency of tobacco plants (Jin et al., 2024). However, during crop rotation, other crops (such as rice and maize) absorb substantial amounts of nutrients from the soil, leading to a decline in soil fertility (Wang et al., 2024). Therefore, the application of inorganic fertilizers to replenish these depleted nutrients is essential to meet the growth requirements of tobacco. Nevertheless, excessive use of inorganic fertilizers can reduce crop disease resistance and contribute to ecological degradation. In contrast, organic fertilizers, particularly microbial fertilizers, have been proven to improve soil quality, increase soil organic carbon input, and enhance microbial populations, thereby positively impacting crop production (Sabir et al., 2021). To promote optimal crop growth, it is essential to investigate the effects of fertilizers on crop development and soil microorganisms. Muhammad et al. demonstrated that a blend of 70% compost and 30% chemical fertilizer was more effective than pure chemical fertilizer. Additionally, organic fertilizers can increase the input of soil organic carbon, fostering the proliferation of heterotrophic microorganisms. By combining organic and microbial fertilizers, it is possible to achieve consistently high crop yields while maintaining soil ecological integrity (Hasnain et al., 2020). Sara et al. found that integrating low-concentration chemical fertilizers with high-concentration microbial fertilizers enhances the diversity of eukaryotic organisms in the soil (Sara et al., 2024). Yang et al. highlighted that appropriate cultivation practices and fertilization are crucial for improving soil structure, preserving moisture, and maintaining soil productivity (Yonghui et al., 2022). By exploring the effects of fertilizers on soil and soil microorganisms, valuable insights can be gained to support sustainable agricultural production.
Soil microorganisms enhance plant growth and strengthen plants’ resilience against both biotic and abiotic stressors, thereby playing crucial roles in crop protection and soil improvement (Ruan et al., 2020). Beneficial microbial communities can directly reduce disease incidence or lower disease risks by promoting the growth of tobacco plants (Huihui et al., 2021). Dang et al. demonstrated that incorporating manure significantly enhances bacterial α diversity in acidic and low-fertility soils (Rahman et al., 2021b). In contrast to conventional fertilizers, microbial organic fertilizer (MOFs) comprise a diverse range of functional probiotics, organic matter, and other beneficial components (Yan et al., 2024). These fertilizers are characterized by their rapid effectiveness and lasting impact. Wu et al. found that microbial formulations in composite microbial fertilizers can stabilize the ecological balance of soil (Xiong et al., 2017). Furthermore, Feng et al. reported that the application of microbial fertilizers significantly alleviates phosphorus limitations in soils used for tobacco cultivation (Feng et al., 2023). Shang et al. investigated the effects of applying Bacillus subtilis microbial fertilizer and plant growth-promoting rhizobacteria (PGPR) microbial fertilizer on tobacco plants, revealing that these applications not only alter the soil microbial community structure but also enhance the catalytic conversion efficiency of soil enzymes (Shang et al., 2023). Currently, MOFs are a focal point in the fertilizer industry; however, research on these fertilizers remains in its infancy, and their application at an industrial scale is limited. Additionally, the standalone use of MOFs can be costly, and their efficacy may be influenced by various environmental and geographical factors, necessitating adjustments in fertilization ratios across different regions.
Therefore, this study aimed to investigate the impact of MOF on tobacco growth in Hunan Province. Experiments were conducted in Changjiang Village, Renyi Town, Guiyang County. The control group consisted of conventional fertilizers containing cake fertilizer, while the experimental group comprised fertilizers containing MOF. The study assessed the effects of MOF on soil physicochemical properties and rhizosphere microbial communities throughout four growth stages of tobacco plants. We hypothesize that (1) the application of MOF could alter the soil microbial diversity at different growth stages of tobacco, (2) MOF improves the physicochemical properties of the soil to enhance soil fertility, and (3) the application of MOF could improve the quality and yield of tobacco leaves.
The selected experimental site is located in Changjiang Village, Renyi Town, Guiyang County, Chenzhou City, Hunan Province, China (112°23′26″E to 112°55′46″E and latitude 25°37′15″N to 26°13′30″N). Guiyang County has an average annual temperature that ranges from 16°C to 23°C, which is favorable for tobacco cultivation. The selected tobacco field is a tobacco-rice rotation system, and the soil is classified as red soil.
In this study, two distinct fertilization methods were established, namely, CK and MOF. The CK group served as the control group, receiving only the base fertilizer. In addition to the base fertilizer, the MOF group was designated as the experimental group, supplemented with MOF. The base fertilizer comprised a one-time application of 900 kg ha−1 of specialized tobacco compound fertilizer, 227 kg ha−1 of potassium silicate mineral fertilizer (a slow-release potassium fertilizer), and 136 kg ha−1 of specialized tobacco seedling fertilizer. The MOF applied in the experimental group was 600 kg ha−1, with a 1:1 ratio of Palygorskite to Bacillus. Three plots were selected for both the control and experimental groups, with each plot consisting of five rows, each measuring 10 m in length, for a total area of 65 m2. A 0.5-meter-wide walkway was incorporated between the plots to facilitate inspection and sampling, and protective measures were implemented on the experimental site. Each plot received base fertilizer before the transplanting period, with fertilization occurring once during the rosette stage, once during the prosperously growing stage, and once during the maturing stage. The timing of fertilization was carefully managed to ensure that the soil moisture levels in each plot were consistent with those observed during previous years’ fertilization practices in the local area. The daily management of tobacco is uniformly overseen by the Chenzhou Tobacco Company in accordance with the national standard GB/T 23221-2008 (Chen et al., 2024).
Soil and tobacco sampling were conducted using a five-point sampling method (Hu et al., 2023; Li X. et al., 2024). This approach begins with the selection of the midpoint of the diagonal of the experimental land as the central sampling point, followed by the identification of four equidistant points along the diagonal as additional sampling locations. During four distinct growth stages of the tobacco plants, we selected robust specimens exhibiting uniform growth and collected their rhizosphere soil. The specific procedure is as follows: first, gently uproot the tobacco plants along with their roots, then carefully shake to remove any loose soil from the roots. Next, utilize a sterile brush to collect the root soil, and finally, place the collected soil in sample bags for transportation back to the laboratory, ensuring refrigeration for subsequent testing. We collected a portion of fresh soil, weighed it, and then air-dried it to determine its moisture content. The dried soil was subsequently ground into a fine powder in preparation for the analysis of its physicochemical properties. Another aliquot of fresh soil was taken for microbial analysis. For the sampling of tobacco leaves, we sampled the tobacco leaves 28 days after applying the fertilizer during the maturing stage. The samples were immediately subjected to measurements of relevant physical properties. Afterward, some samples were used for curing, while the remaining samples were used for further analysis of physicochemical properties.
We utilized the established methodology to examine the soil’s physicochemical properties (Wang Z. et al., 2022; Yu X. et al., 2023) as outlined below: Each sample was divided into three parallel portions. The original soil samples were sieved using a 20-mesh sieve (1 mm diameter) to remove stones, plant roots, and residues. The physicochemical properties measured included available potassium (AK), available phosphorus (AP), organic matter (OM), pH, nitrate nitrogen (NO3−-N), and ammonium nitrogen (NH4+-N). Available potassium (AK) was quantified using flame photometry, while available phosphorus (AP) was assessed via the 0.5 mol/L NaHCO3 method. Organic matter (OM) was quantified using the potassium dichromate volumetric method with external heating. pH was measured with a pH meter (Sartorius Scientific Instruments) at a soil-to-water ratio of 1:2. Nitrate nitrogen (NO3−-N) was determined through the phenol disulfonic acid colorimetric method, and ammonium nitrogen (NH4+-N) was analyzed using the 2 mol/L KCl extraction method for ammonia-nitrogen.
Drawing from prior research (Yu X. et al., 2023), we implemented consistent DNA extraction and PCR amplification methodologies. This process entailed utilizing the DNeasy® PowerSoil® Pro Kit DNA kit (QIAGEN, United States) for extracting genomic DNA of soil microbial communities from 0.5 g soil samples. Subsequently, DNA purity and concentration were determined using a UV spectrophotometer, and the extracted DNA was assessed via 1% agarose gel electrophoresis. To amplify the highly variable regions of bacterial 16S rRNA V3-V4, the primer pairs 338F (5′-ACTCCTACGGGAGCAGCAGC-3′) and 806R (5′-GGACTACHVGGGTWTCTAAT-3′) were adopted (Zhang et al., 2022). The amplification protocol consisted of a pre-denaturation step at 95°C for 3 min, followed by denaturation at 95°C for 30 s, annealing at 55°C for 30 s, and extension at 72°C for 45 s. This cycle was repeated 30 times, with a final storage step at 10°C. For the identification of PCR products, three replicate PCR samples were pooled, and the resulting products were analyzed using 2% agarose gel electrophoresis. Finally, the PCR products underwent purification using the AxyPrep DNA Gel Extraction Kit.
Sequencing was performed using the Illumina MiSeq PE300 platform. In this process, one end of the DNA segment is immobilized on a chip, while the other end is hybridized with a complementary buried adapter that is anchored nearby. Subsequent PCR amplification results in the formation of DNA clusters, where the amplified DNA strands are linearized into single strands. Modified DNA polymerase and fluorescently labeled dNTPs are introduced to synthesize one base per cycle. The reaction plate surface is scanned with a laser to read the nucleotide type incorporated during the initial round of template sequencing. Chemical cleavage of the “fluorescent group” and “terminator group” restores the 3′ hydroxyl groups, allowing for the addition of the subsequent nucleotide. The fluorescence signals obtained in each cycle are then analyzed to determine the sequence of the template DNA segment.
Initially, the raw sequencing data underwent quality control using fastp version 0.20.0 software. Following this, the FLASH software (version 1.2.7) was utilized for sequence assembly. The assembly process comprised several steps: (1) filtering out bases with quality scores below 20 from the ends of the reads using a 50 bp sliding window, (2) merging paired-end reads based on their overlap relationships, requiring a minimum overlap length of 10 bp, (3) permitting a maximum mismatch rate of 0.2 within the overlap region of the merged sequences and discarding those that did not satisfy this criterion, and (4) distinguishing samples using barcodes and primers located at both ends of the sequences, adjusting sequence orientation while allowing zero mismatches for barcodes and a maximum of two mismatches for primers. After completing quality control and assembly, the optimized sequences were denoised using the Deblur plugin in the Qiime2 pipeline with default parameters.
Analysis of tobacco physical characteristics was conducted following the national standard GB 2635-1992 (Ling et al., 2020). The appearance quality of tobacco encompasses the sensory and experiential factors through which individuals assess its overall quality. Typically, the evaluation of tobacco grade quality involves visual inspection, tactile examination, auditory assessment, and olfactory perception. The primary factors influencing tobacco quality include color, maturity, leaf structure, oil content, color intensity, width, and length. The external appearance of tobacco not only reflects its internal quality but also serves as the principal criterion for grading during the procurement process. Generally, high-quality tobacco is characterized by optimal maturity, a loose texture, moderate thickness, golden or orange coloration, adequate oil content, a strong luster, and good elasticity. Tobacco can be classified into upper, middle, and lower leaves, each exhibiting distinct external traits. Leaf length is defined as the straight measurement from the base of the main vein to the leaf tip. Grading standards categorize leaf lengths into five groups: equal to or greater than 45 cm, 40 cm, 35 cm, 30 cm, and 25 cm, with increments of 5 cm. These specifications align with different grade requirements, ensuring that a specific grade meets or exceeds the designated length.
α-diversity was analyzed using the mothur software platform (v.1.30.2). α-diversity was measured using Hill numbers and Pielou’s evenness index. The Shannon index and Simpson index are indicators of community diversity; the ACE index and Chao index are indicators of community richness; and the Coverage index is an indicator of community coverage (Hill, 1973). Statistical analyses and graphical representations, including Venn diagrams, community composition analysis plots, PCoA, RDA, and correlation heatmaps, were performed using R (3.3.1). LEfSe software was used for multilevel species differential analysis. FAPROTAX (version 1.2.1) was employed for differential testing of functional groups. BugBase software was used for BugBase phenotypic prediction. Further comparative analysis of species annotation results was conducted based on the Majorbio Biocloud analysis platform. Variance significance tests for soil physicochemical properties were conducted using SPSS software.
All sequence data were submitted to the Sequence Read Archive (Accession Number: PRJNA1205160) and can be freely accessed through the NCBI website (https://www.ncbi.nlm.nih.gov/sra/PRJNA1205160).
This study examined the effects of MOF on the physicochemical properties of soil (Table 1). Relative to the control group, the application of MOF had a minimal impact on soil pH, which was consistently maintained at approximately 6.5. However, significant differences (p < 0.05) were observed in soil organic matter (OM), available potassium (AK), and available phosphorus (AP) across the four distinct growth stages. In the Experimental group, the AK levels increased during the transplanting stage, peaked at 778.90 mg/kg during the rosette stage, and subsequently declined at the maturation stage. The nitrate nitrogen levels decreased during both the transplanting and maturation stages but remained relatively stable during the vigorous growth stage, at approximately 11 mg/kg. Compared to the CK group, the ammonium nitrogen concentration in the Experimental group increased during all three stages.
To assess the impact of MOF on soil microbial composition, this study conducted α-diversity analysis to evaluate the richness and diversity of microbial communities during different stages: transplanting period, rosette stage, prosperously growing stage, and maturing stage (Supplementary Table S1). The results revealed that the coverage of each sample approached 0.99, indicating sufficient coverage. During the transplanting period and maturing stage, the Shannon index was higher for MOF-treated soil (6.980) compared to CK (7.110) and MOF (7.110) compared to CK (6.803), indicating that MOF promote increased community diversity during these stages. The Simpson index showed lower values for MOF during the transplanting period [MOF (0.002) < CK (0.007)] and maturing stage [MOF (0.004) < CK (0.006)], suggesting that MOF enhance ecosystem diversity in tobacco plant rhizospheric soil. Analysis of Shannon and Simpson indices indicated differences in microbial diversity during the transplanting and maturing stages due to MOF application. Compared to the CK control treatment, the Shannon index slightly increased and the Simpson index slightly decreased during the transplanting and maturing stages following MOF treatment, indicating an increase in the total quantity of microbial organisms in tobacco plant rhizospheric soil. However, we also observed that during the prosperously growing stage, the Shannon index decreased, while the Simpson index increased. The Ace and Chao indices were CK (5589.205) > MOF (5265.152) and CK (5456.055) > MOF (5149.431), respectively, indicating that the diversity of the soil microbial community and bacterial richness declined when plants treated with MOF entered the prosperously growing stage.
The Neutral Community Model (NCM) effectively estimated the primary relationship between the occurrence frequency of operational taxonomic units (OTUs) and changes in their relative abundance (Supplementary Figure S1). The turnover rates of the bacterial community in response to MOF treatment and the Control Check (CK) at four time points were 90.26%, 87.20%, and 92.76%, respectively. The effective population size (Nm) of the bacterial community following MOF treatment (Nm = 31,035.46) was greater than that of the CK control (Nm = 23,814.63). Both groups exhibited a sequence number of 49,233, with an m value of 0.6304 for MOF treatment and 0.4837 for the CK control. These findings indicate that bacterial species dispersal in the soil significantly increased after MOF treatment compared to the CK control. To identify the specific microbes influenced by MOF, we analyzed the microbial composition in the soil (Figure 1). The results from the Venn diagram indicate that MOF significantly influences the composition of bacteria in the rhizosphere soil of tobacco plants (Figure 1A). At the species level, variations in the number of unique bacteria were observed between the control (CK) and experimental group (MOF) groups across four time points. During the transplanting period, the CK group exhibited 45 unique bacterial genera, whereas the Experimental group displayed 50. In the rosette stage, the counts increased to 56 for CK and 84 for MOF. However, during the prosperously growing stage, the CK group had a notable decline to 187 unique genera, while the Experimental group decreased to 91. In the maturing stage, the numbers were 53 for CK and 58 for MOF. The total number of shared bacteria across all groups was 1,214. An analysis of community composition at the phylum level (Figure 1B) revealed that the predominant bacterial taxa in the soil included Actinobacteriota, Proteobacteria, Chloroflexi, Acidobacteriota, Firmicutes, Bacteroidota, and Myxococcota, collectively accounting for over 80% of the total soil bacteria. Actinobacteriota emerged as the dominant phylum, comprising approximately 19%–26% of the overall count. Proteobacteria was the second most dominant, accounting for about 20%, with specific percentages of 20.26% (T-CK), 19.54% (T-MOF), 19.66% (R-CK), 21.28% (R-MOF), 19.87% (P-CK), 22.06% (P-MOF), 22.03% (M-CK), and 19.96% (M-MOF). Although the proportions of each bacterial taxon differed between the experimental and control groups, these bacterial taxa remained dominant in the soil overall, indicating their relatively stable presence in the soil.
Figure 1. Analysis of species composition. (A) Venn diagram analysis at the species level. (B) Analysis of community composition at the phylum level.
Principal Coordinate Analysis (PCoA) at the species level was conductedto examine the differences in soil bacterial community composition between the MOF treatment group and the control group (Figure 2). The results (R = 0.6799, P = 0.0010) demonstrated significant differences in bacterial communities between the treatment and control groups, particularly during the transplanting period and prosperous growth stage. A distinct separation between the treatment and control groups was observed at these two stages, indicating that the application of MOF influenced the composition of soil bacterial communities.
Indicative microbes refer to microbial communities that exhibit statistically significant differences. To identify microbial communities with significant features in soil following the application of MOF, we performed LEfSe analysis (Figure 3). Among the bacterial community taxa-level classification units, 189 were identified (LDA score >3, P < 0.05). The taxa Blastocatellales, Micrococcaceae, Nitrosomonadaceae, and norank_c_bacteriap25 in the soil treated with MOF displayed significant features during the transplanting period, rosette stage, prosperous growth stage, and maturation stage, respectively. In contrast, the soil of the control group exhibited significant features of Actinobacteriota, Thermoleophilia, Bacilli, and Oxalobacteraceae across the four periods.
To elucidate the relationship between microbial communities and environmental factors, a Redundancy Analysis (RDA) was conducted (Figure 4A). RDA1 and RDA2 accounted for 23.75% and 5.84% of the variance in bacterial community structure, respectively. In comparison to the control (CK), the bacterial communities in the MOF treatment at four different periods were more significantly influenced by available phosphorus (AP), with this regulation being particularly pronounced during the rosette stage. To assess the impact of species abundance, an analysis of the top 50 species at the family classification level, in conjunction with physicochemical properties, was performed (Figure 4B), revealing significant correlations. Notably, norank_o_norank_c_bacteriap25, norank_o_norank_c_TK10,norank_o_Rokubacteriales, unclassified_c_Anaerolineae,Micromonosporaceae, and JG30-KF-CM45 exhibited strong positive correlations with available potassium (AK) and AP, while Sutterellaceae demonstrated a significant negative correlation with both AK and AP. Additionally, Nitrospiraceae showed a significant positive correlation with organic matter (OM) and a positive correlation with AK, whereas Blastocatellaceae exhibited a significant negative correlation with OM. Ilumatobacteraceae displayed a significant negative correlation with ammonium nitrogen (NH4+-N), and Blastocatellaceae also showed a negative correlation with both NH4+-N and OM. These findings suggest that MOF play a crucial role in influencing the abundance of bacterial species.
Figure 4. Environmental factor correlation analysis. (A) RDA analysis at the OTU level (B) Correlation Heatmap at the family level.
To further investigate the effects of MOF on the functioning of soil bacterial communities in tobacco inter-roots, we utilized the FAPROTAX database for predictions (Figure 5A). The findings indicated an enhancement in the fermentation and nitrate reduction functions of the rhizosphere bacterial community following the application of MOF during the transplanting period, rosette stage, and maturing stage, with a decrease observed during the prosperously growing stage. Conversely, chitinolysis, aromatic compound degradation, and chemoheterotrophy exhibited significant increases during the prosperously growing stage, while experiencing declines during the other three periods. Furthermore, the analysis of the phenotypes of bacterial communities in tobacco rhizosphere soil post-application of MOF, conducted using the BugBase database (Figure 5B), revealed significant differences across four aspects: Gram-Positive, Potentially Pathogenic, Aerobic, and Gram-Negative.
Figure 5. Functional prediction analysis. (A) Differential testing of functional groups in FAPROTAX functional prediction. (B) Phenotype groups in BugBase phenotype prediction.
The application of MOF was able to cause a more pronounced effect on tobacco leaf traits (Figure 6). Although compared to the CK group, MOF increased the length and width of the upper and middle leaves and reduced the length and width of the lower leaves, statistical analysis revealed that there were no significant differences between the two groups. The application of MOF leads to a 21.93% increase in yield and a 27.38% increase in yield value per unit area while maintaining the average price per plot (Supplementary Table S2). The analysis revealed significant differences between the experimental and control groups in terms of yield and yield value (p < 0.05). Furthermore, the appearance quality of cured tobacco leaves (Table 2), revealing an enhancement in leaf color after MOF treatment, with increases of 2.95% for upper leaves, 4.22% for middle leaves, and 3.42% for lower leaves. The total score of leaf quality varies: upper leaves increase by 2.95%, middle leaves by 2.51%, but lower leaves decrease by 1.35%. Oil content in leaves remains relatively unchanged in upper leaves but decreases in lower and middle leaves. Regarding color intensity, all three leaf segments show improvement after MOF treatment, with the greatest increase observed in lower leaves at 9.76%. Finally, chemical composition analysis of cured tobacco leaves (Supplementary Table S3) reveals an increase in potassium content and a decrease in nicotine and nitrogen content. The reduction of nicotine and total nitrogen content in tobacco is conducive to improving tobacco quality.
The research suggests that the soil’s physicochemical properties underwent changes following the application of MOF. Soil pH remained relatively stable across four distinct stages, aligning with Jiang et al.’s results (Jiang et al., 2021a). When the soil pH is around 6.5, the availability of the nutrients in the soil is relatively high. The root system of tobacco plants can absorb these nutrients more effectively, which thereby promotes the growth of the tobacco plants (Nagao, 1971; Zhao et al., 2024). Nitrogen and potassium are vital elements for plant development (Feng et al., 2018). The slow-release mechanism of convex-concave rods and Bacillus’s ability to convert insoluble potassium into soluble forms led to an increase in available potassium content during the transplanting period, rosette stage, and prosperously growing stage compared to the control (El-Hadad et al., 2011). Concurrently, Bacillus facilitated potassium utilization in the soil (Abdel-Megeed, 2012), with potassium ions extensively utilized during the maturing stage, resulting in a decline in soil potassium ion concentration. This decline is corroborated by the augmented potassium ion content in the final leaves. Tobacco is a plant that prefers nitrate nitrogen (Duan et al., 2022). When the nitrogen demand of tobacco plants is relatively low, they preferentially utilize nitrate nitrogen. The use of ammonium nitrogen as a nitrogen source may inhibit plant growth, reduce tobacco leaf yield, and impair quality. However, MOF can mitigate the absorption of ammonium nitrogen by plants, ultimately resulting in a decrease in soil nitrate nitrogen content and an increase in ammonium nitrogen content in the treated group.
This study investigates the rhizospheric soil microbial composition of tobacco plants across four distinct growth stages, based on the strong correlation between plant growth and microbial ecology characteristics. α-Diversity analysis revealed that MOF significantly influenced the bacterial diversity in the rhizosphere soil of tobacco plants, particularly during the prosperously growing stage. The increased nutrient demand by the plants intensified competition among microbial communities. Additionally, the application of MOF accelerated the succession process of bacterial communities, such as Bacillus, further exacerbating inter-bacterial competition, which led to a decline in diversity and richness. Previous studies have demonstrated that the application of Bacillus can reduce soil species diversity (Li et al., 2022), and that plants, when faced with increased nutrient demands, compete with microorganisms for limited nutrient resources through root exudates and other mechanisms (Ruan et al., 2024). These findings are consistent with our observations. NCM analysis reveals that the R2 value of the Experimental group exceeds that of the control (CK) group, with both the m value and Nm value also elevated in comparison to the control (CK) group. A higher R2 value indicates a closer alignment with a neutral model, suggesting that stochastic processes have a more significant influence on community assembly. Furthermore, an increased m value reflects a reduction in constraints on species dispersal, while a higher Nm value denotes a more uniform distribution of species across communities (Zhou and Ning, 2017). This implies that a greater number of species can migrate among different communities, thereby enhancing species diversity and richness within these communities. Consequently, the application of MOF appears to enhance microbial dispersal, corroborating the results of Liu et al.’s study on soil microbial communities (Liu X. et al., 2022).
Analyzing the rhizospheric soil microbial communities of tobacco plants at the phylum level reveals that Actinobacteriota and Proteobacteria are the first and second dominant phyla, respectively, comprising 39%–46% of the total soil bacterial population. This finding is consistent with previous reports (Verzeaux et al., 2016). Actinobacteriota serve as crucial decomposers in the soil, playing a significant role in nutrient transformation and utilization (Jenkins et al., 2009). In contrast, Proteobacteria display remarkable environmental adaptability and possess metabolic degradation functions (Emenike et al., 2023; Gong et al., 2023; Liu B. et al., 2022). Chloroflexi is the third dominant phylum, primarily involved in the decomposition of organic matter and carbon cycling in soil. They are capable of degrading complex organic compounds, thereby promoting the cycling of soil organic matter and the maintenance of soil fertility (Xian et al., 2020). Acidobacteriota, as the fourth dominant phylum, can solubilize minerals in the soil, releasing phosphorus and other trace elements essential for plant growth (Yu Y. et al., 2023). Furthermore, Principal Coordinate Analysis (PCoA) indicates that the application of MOF influences the bacterial communities in the soil, corroborating findings from previous studies (Jenkins et al., 2009; Sun et al., 2015; Wang B. et al., 2022).
LEfSe analysis revealed the microbial communities associated with specific functions and exhibiting significant features following the application of MOF (Du et al., 2022). For example, during the transplanting period after the application of these fertilizers, Blastocatellales displayed significant features. As a key player in the network of organic and natural farming systems (Matteoli et al., 2022), Blastocatellales not only decompose organic matter in the soil but also participate in the nitrogen cycling process, indicating a notable association between the reduction of nitrate nitrogen during the transplanting period and Blastocatellales. Additionally, Micrococcaceae exhibited significant features during the rosette stage; according to Lu et al., Micrococcaceae, recognized as a salt-tolerant bacterium, can promote plant growth (Lu et al., 2023). Furthermore, Nitrosomonadaceae, which are ammonia-oxidizing bacteria, facilitate soil nitrification by converting nitrites to nitrates (Cheng et al., 2017; Chun et al., 2021).
The shape, yield, and quality of tobacco leaves are the most direct and effective indicators for evaluating the efficacy of MOF. In addition to improving crop yield, MOF can enhance fertilizer utilization efficiency and reduce environmental pollution associated with chemical fertilizers (Liu et al., 2015; Shao et al., 2025). In this study, the application of MOF significantly increased both the yield and value of tobacco, while also improving the quality of the leaves in comparison to the control group (CK). This finding is consistent with previous research (Zhang et al., 2013). Moreover, nicotine is one of the main sources of nitrogen in tobacco, and the reduction in nitrogen content is usually associated with a reduction in nicotine content. Therefore, the reduction in nicotine and total nitrogen content in tobacco leaves could contributes to lowering the toxicity of tobacco and improving the quality of the leaves (Jiang Y. et al., 2021; Li Z. J. et al., 2024). The improvement in the quality of tobacco leaves could further enhance their economic value.
The bacterial community in this study underwent further analysis for functional predictions using FAPROTAX and phenotypic predictions using BugBase. In the FAPROTAX functional prediction, significant differences were observed in the chitinolysis and chemoheterotrophy functions of soil microorganisms. With the exception of the prosperously growing stage, during which the control group surpassed the experimental group, the experimental group exhibited higher levels than the control group in the other three periods. This suggests that organic fertilizers can significantly enhance the chitinolysis and chemoheterotrophy functions of soil microbial communities in the tobacco rhizosphere. BugBase phenotype prediction primarily indicated that, apart from the prosperously growing stage, the Gram-positive and aerobic phenotype types of microorganisms in the experimental group were higher than those in the control group, while the potentially pathogenic and Gram-negative phenotype types of microorganisms in the experimental group were lower than those in the control group. However, these hypotheses lacked experimental validation, resulting in disparities between predicted outcomes and actual observations (Du et al., 2022; Tao et al., 2020; Wang T. et al., 2022). Therefore, subsequent experiments should aim to validate the impact of changes in microbial communities on microbial community functions. It is worth noting that this study was conducted on the same land within the same year, and did not explore the effects of MOF in tobacco planting soils across different regions, which may limit its geographical applicability. In addition to altering soil microbial communities and improving tobacco yield and quality through MOF, interspecific planting of different plants has been shown to modify the composition of rhizospheric soil microbes (Zhou et al., 2023). Additionally, altering planting systems is another effective approach (Liu et al., 2020). Furthermore, balancing microbial community structure by reducing nitrogen fertilizer application can promote tobacco plant growth (Shen et al., 2022). Hence, various methods can be employed in subsequent studies to investigate the effects on soil microbes. The most effective approach to studying the effects of MOF on soil microbes in tobacco rhizospheric soil would involve conducting experiments in different regions, with different tobacco plants, and across different years. However, given the complexity of microbial interactions with soil physicochemical properties and plants, there remains substantial research potential in using MOF to improve soil quality and enhance plant growth (Gu et al., 2021; Li et al., 2021).
This study investigated the effects of MOF on microbial community structure, soil physicochemical properties, and tobacco leaf quality in rhizosphere soils. The results indicated that MOF can enhance levels of soil organic matter, available phosphorus, and ammonium nitrogen, while also increasing microbial diversity, particularly by promoting beneficial bacterial groups such as Actinobacteria and Proteobacteria. These changes in microbial communities contribute to improved soil fertility, which subsequently enhances the quality and yield of tobacco leaves. These findings may provide valuable insights for further research into the role of MOFs in promoting crop growth and increasing agricultural yields. Future studies should focus on long-term field trials to assess the persistence of these effects, explore microbial functional traits through metagenomic analysis, and investigate plant-microbe interactions to optimize the application of microbial fertilizers within agricultural systems.
The datasets presented in this study can be found in online repositories. The names of the repository/repositories and accession number(s) can be found below: https://www.ncbi.nlm.nih.gov/sra/PRJNA1205160.
ShW: Conceptualization, Formal Analysis, Methodology, Supervision, Writing–review & editing. BZ: Conceptualization, Formal Analysis, Investigation, Methodology, Writing–review & editing. SM: Data curation, Investigation, Methodology, Writing–review & editing. JHa: Investigation, Methodology, Writing–review & editing. LZ: Investigation, Methodology, Writing–review & editing. CG: Methodology, Supervision, Writing–review & editing. JHo: Investigation, Methodology, Writing–review & editing. HD: Data curation, Writing–review & editing. YZ: Investigation, Writing–review & editing. YW: Data curation, Methodology, Writing–review & editing. WW: Data curation, Methodology, Writing–original draft. JS: Writing–original draft. SX: Writing–original draft. JY: Conceptualization, Funding acquisition, Supervision, Writing–review & editing. GS: Funding acquisition, Resources, Supervision, Writing–review & editing.
The author(s) declare that financial support was received for the research and/or publication of this article. This work was supported by Technology development (commissioning) project from Inner Mongolia Kunming Cigarette Limited Liability Company (Grant No. 202315010534-JS-062, 202315010534-JS-061).
The authors would also like to thank the “Laboratory for Agricultural Molecular Biology” of Qingdao Agricultural University for providing laboratory apparatus.
Authors ShW, BZ, SM, JHa, LZ, CG, JHo, HD, and YZ were employed by Inner Mongolia Kunming Cigarette Co., Ltd.
The remaining authors declare that the research was conducted in the absence of any commercial or financial relationships that could be construed as a potential conflict of interest.
The author(s) declare that no Generative AI was used in the creation of this manuscript.
All claims expressed in this article are solely those of the authors and do not necessarily represent those of their affiliated organizations, or those of the publisher, the editors and the reviewers. Any product that may be evaluated in this article, or claim that may be made by its manufacturer, is not guaranteed or endorsed by the publisher.
The Supplementary Material for this article can be found online at: https://www.frontiersin.org/articles/10.3389/fenvs.2025.1555622/full#supplementary-material
Abdel-Megeed, A. E. S. (2012). Impact of rock materials and biofertilizations on P and K availability for maize (Zea Maize) under calcareous soil conditions. Saudi J. Biol. Sci. doi:10.1016/j.sjbs.2011.09.001
Abed, R. M. M., Al-Sadi, A. M., Al-Shehi, M., Al-Hinai, S., and Robinson, M. D. (2013). Diversity of free-living and lichenized fungal communities in biological soil crusts of the Sultanate of Oman and their role in improving soil properties. Soil Biol. Biochem. 57, 695–705. doi:10.1016/j.soilbio.2012.07.023
Ali, A., Ghani, M. I., Elrys, A. S., Ding, H., Iqbal, M., Cheng, Z., et al. (2021). Different cropping systems regulate the metabolic capabilities and potential ecological functions altered by soil microbiome structure in the plastic shed mono-cropped cucumber rhizosphere. Agric. Ecosyst. Environ. 318, 107486. doi:10.1016/j.agee.2021.107486
Ali, A., Ghani, M. I., Li, Y., Ding, H., Meng, H., and Cheng, Z. (2019). Hiseq base Molecular Characterization of soil microbial community, diversity structure, and predictive functional Profiling in continuous cucumber planted soil affected by diverse cropping systems in an intensive greenhouse region of Northern China. Int. J. Mol. Sci. 20 (11), 2619. doi:10.3390/ijms20112619
Chen, K., Wang, D., Zhang, J., Yang, M., dong, X., Wang, D., et al. (2024). Study on growth characteristics and differences of upper leaves of China's main flue-cured tobacco cultivars. Jiangsu Agric. Sci. 52 (01), 76–82.
Cheng, J., Chen, Y., He, T., Liao, R., Liu, R., Yi, M., et al. (2017). Soil nitrogen leaching decreases as biogas slurry DOC/N ratio increases. Appl. Soil Ecol. 111, 105–113. doi:10.1016/j.apsoil.2016.12.001
Chun, S.-J., Kim, Y.-J., Cui, Y., and Nam, K.-H. (2021). Ecological network analysis reveals distinctive microbial modules associated with heavy metal contamination of abandoned mine soils in Korea. Environ. Pollut. 289, 117851. doi:10.1016/j.envpol.2021.117851
Dang, P., Li, C., Lu, C., Zhang, M., Huang, T., Wan, C., et al. (2022). Effect of fertilizer management on the soil bacterial community in agroecosystems across the globe. Agric. Ecosyst. and Environ. 326, 107795. doi:10.1016/j.agee.2021.107795
Du, T.-Y., He, H.-Y., Zhang, Q., Lu, L., Mao, W.-J., and Zhai, M.-Z. (2022). Positive effects of organic fertilizers and biofertilizers on soil microbial community composition and walnut yield. Appl. Soil Ecol. 175, 104457. doi:10.1016/j.apsoil.2022.104457
Duan, Y., Yang, H., Wu, W., and Li, W. (2022). Molecular mechanisms of nitrogen absorption, Transport, and Assimilation in plants. Fujian J. Agric. 37 (04), 547–554.
El-Hadad, M. E., Mustafa, M. I., Selim, S. M., El-Tayeb, T. S., Mahgoob, A. E. A., and Abdel, A. N. H. (2011). The nematicidal effect of some bacterial biofertilizers on Meloidogyne incognita in sandy soil. Braz. J. Microbiol. 42 (1), 105–113. doi:10.1590/s1517-83822011000100014
Emenike, C., Agamuthu, P., Fauziah, S., Omo-Okoro, P., and Jayanthi, B. (2023). Enhanced bioremediation of metal-contaminated soil by consortia of proteobacteria. Water, Air, and Soil Pollut. 234 (12), 731. doi:10.1007/s11270-023-06729-3
Feng, J., Chen, L., Xia, T., Ruan, Y., Sun, X., Wu, T., et al. (2023). Microbial fertilizer regulates C:N:P stoichiometry and alleviates phosphorus limitation in flue-cured tobacco planting soil. Sci. Rep. 13 (1), 10276. doi:10.1038/s41598-023-37438-w
Feng, J., Chu, S., Wang, J., Wu, D., Mo, Q., and Zeng, S. (2018). Comprehensive evaluation of soil fertility of five typical forest stands in South China. J. South China Agric. Univ. 39 (3), 73–81.
Ghani, M. I., Ali, A., Atif, M. J., Pathan, S. I., Pietramellara, G., Ali, M., et al. (2022). Diversified crop rotation improves continuous monocropping eggplant production by altering the soil microbial community and biochemical properties. Plant Soil 480 (1-2), 603–624. doi:10.1007/s11104-022-05606-y
Gong, Y., Yang, S., Chen, S., Zhao, S., Ai, Y., Huang, D., et al. (2023). Soil microbial responses to simultaneous contamination of antimony and arsenic in the surrounding area of an abandoned antimony smelter in Southwest China. Environ. Int. 174, 107897. doi:10.1016/j.envint.2023.107897
Gu, S., Lian, F., Yang, H., Han, Y., Zhang, W., Yang, F., et al. (2021). Synergic effect of microorganism and colloidal biochar-based organic fertilizer on the growth and fruit quality of tomato. Coatings 11 (12), 1453. doi:10.3390/coatings11121453
Hasnain, M., Chen, J., Ahmed, N., Memon, S., Wang, L., Wang, Y., et al. (2020). The effects of fertilizer type and application time on soil properties, plant traits, yield and quality of tomato. Sustainability 12, 9065. doi:10.3390/su12219065
Hill, M. O. (1973). Diversity and evenness: A unifying notation and its consequences. Ecology. 54(2), 231–466. doi:10.2307/1934352
Hu, L., Huang, R., Zhou, L., Qin, R., He, X., Deng, H., et al. (2023). Effects of magnesium-modified biochar on soil organic carbon mineralization in citrus orchard. Front. Microbiol. 14, 1109272. doi:10.3389/fmicb.2023.1109272
Huihui, D., Rui, W., Xiaohong, R., Jianqiang, D., Xiaohua, D., and Guijun, B. (2021). Co-composting of fresh tobacco leaves and soil: an exploration on the utilization of fresh tobacco waste in farmland. Environ. Sci. Pollut. Res. Int. 29 (6), 8191–8204. doi:10.1007/s11356-021-16189-z
Jenkins, S. N., Waite, I. S., Blackburn, A., Husband, R., Rushton, S. P., Manning, D. C., et al. (2009). Actinobacterial community dynamics in long term managed grasslands. Ant. Van Leeuwenhoek 95, 319–334. doi:10.1007/s10482-009-9317-8
Jez, J. M., Lee, S. G., and Sherp, A. M. (2016). The next green movement: plant biology for the environment and sustainability, 6305. American Association for the Advancement of Science.
Jiang, C.-q., Shen, J., Yan, Y.-f., and Zu, C.-l. (2021a). Straw return with dolomite application increase flue-cured tobacco leaf yield and quality. Int. J. Agric. Biol. 25, 291–297. doi:10.17957/ijab/15.1669
Jiang, Y., Gong, J., Chen, Y., Hu, B., Sun, J., Zhu, Y., et al. (2021b). Biodegradation of nicotine and TSNAs by bacterium sp. Strain J54. Iran. J. Biotechnol. 19 (3), e2812. doi:10.30498/ijb.2021.240460.2812
Jin, X., Yang, X., Peng, S., Ma, E., Zhang, H., Lin, X., et al. (2024). Cropping rotation improved the bacterial diversity and N-cycling genes in tobacco fields through a 19-year long-term experiment. Appl. Soil Ecol. 193, 105165. doi:10.1016/j.apsoil.2023.105165
Juan, L., Bing-Qiang, Z., Xiu-Ying, L., Rui-Bo, J., and Bing, S. (2008). Effects of long-term combined application of organic and mineral fertilizers on microbial biomass, soil enzyme activities and soil fertility. Agric. Sci. China 7, 336–343. doi:10.1016/s1671-2927(08)60074-7
Li, Q., Xing, Y., Huang, B., Chen, X., Ji, L., Fu, X., et al. (2022). Rhizospheric mechanisms of Bacillus subtilis bioaugmentation-assisted phytostabilization of cadmium-contaminated soil. Sci. Total Environ. 825, 154136. doi:10.1016/j.scitotenv.2022.154136
Li, X., Xie, Y., Zhu, X., Wang, G., Bai, Y., Du, Y., et al. (2024a). Soil quality evaluation and its correlation with tobacco leaf quality under different previous crops. Crop J. (05), 167–174.
Li, Y., Zhao, Y., Song, Z., Deng, Y., Wang, H., Xu, L., et al. (2021). Effect of microbial combination with organic fertilizer on elymus dahuricus. Open Geosci. 13 (1), 233–244. doi:10.1515/geo-2020-0230
Li, Z. J., Yang, D. D., Wei, Z. Y., Huang, J., Chi, Y. Q., Lu, Y. X., et al. (2024b). Reduction of nicotine content in tobacco through microbial degradation: research progress and potential applications. Biotechnol. Biofuels Bioprod. 17 (1), 144–213. doi:10.1186/s13068-024-02593-3
Ling, Y., Qi, L., Xingbing, F., Liuchen, Z., Xiaopeng, D., Erdeng, M., et al. (2020). Effects of planting density and nitrogen application rate on the growth and the quality characteristics of mountain tobacco. J. Yunnan Agric. Univ. 35 (05), 810–817.
Liu, B., Yao, J., Chen, Z., Ma, B., Li, H., Wancheng, P., et al. (2022a). Biogeography, assembly processes and species coexistence patterns of microbial communities in metalloids-laden soils around mining and smelting sites. J. Hazard. Mater. 425, 127945. doi:10.1016/j.jhazmat.2021.127945
Liu, H., Li, J., Li, X., Zheng, Y., Feng, S., and Jiang, G. (2015). Mitigating greenhouse gas emissions through replacement of chemical fertilizer with organic manure in a temperate farmland. Sci. Bull. 60, 598–606. doi:10.1007/s11434-014-0679-6
Liu, X., Shi, Y., Kong, L., Tong, L., Cao, H., Zhou, H., et al. (2022b). Long-term application of bio-compost increased soil microbial community diversity and altered its composition and network. Microorganisms 10 (2), 462. doi:10.3390/microorganisms10020462
Liu, Z., Liu, J., Yu, Z., Yao, Q., Li, Y., Liang, A., et al. (2020). Long-term continuous cropping of soybean is comparable to crop rotation in mediating microbial abundance, diversity and community composition. Soil Tillage Res. 197, 104503. doi:10.1016/j.still.2019.104503
Lu, T., Xu, N., Lei, C., Zhang, Q., Zhang, Z., Sun, L., et al. (2023). Bacterial biogeography in China and its association to land use and soil organic carbon. Soil Ecol. Lett. 5 (4), 230172. doi:10.1007/s42832-023-0172-8
Matteoli, F. P., Silva, A. M., Feiler, H. P., de Araujo, V. L., and Cardoso, E. J. (2022). Predicting soil farming system and attributes based on soil bacterial community. Appl. Soil Ecol. 171, 104335. doi:10.1016/j.apsoil.2021.104335
Nagao, T. (1971). Studies on the Growth of Tobacco Roots: IX. On the respiratory character of root apex. Jpn. J. Crop Sci. 40 (3), 351–355. doi:10.1626/jcs.40.351
Rahman, M. M., Khanom, A., and Biswas, S. K. (2021a). Effect of Pesticides and chemical fertilizers on the nitrogen cycle and functional microbial communities in Paddy soils: Bangladesh Perspective. Bull. Environ. Contam. Toxicol. 106 (2), 243–249. doi:10.1007/s00128-020-03092-5
Rahman, M. M., Khanom, A., and Biswas, S. K. (2021b). Effect of Pesticides and chemical fertilizers on the nitrogen cycle and functional microbial communities in Paddy soils: Bangladesh Perspective. Bull. Environ. Contam. Toxicol. 106 (2), 243–249. doi:10.1007/s00128-020-03092-5
Ruan, W., Ren, J., Guo, M., and Yi, K. (2024). Advances in the study of plant-microbial interactions coordinated by nitrogen and phosphorus nutrients. J. Plant Nutr. Fertilizers 30 (07), 1322–1328.
Ruan, Y. N., Nan, R. Y., Xin, T. Z., Yuan, X. T., and Bin, C. Z. (2020). Application progress of microbial fertilizers in flue-cured tobacco production in China. IOP Conf. Ser. Earth Environ. Sci. 615 (1), 012084. doi:10.1088/1755-1315/615/1/012084
Sabir, M. S., Shahzadi, F., Ali, F., Shakeela, Q., Niaz, Z., and Ahmed, S. (2021). Comparative effect of fertilization practices on soil microbial diversity and activity: an Overview. Curr. Microbiol. 78 (10), 3644–3655. doi:10.1007/s00284-021-02634-2
Sara, H.A.-H., Dana, A. A., Talaat, A., Amer, F.A.-k., Sowaid, A.A.-M., Mahmoud, Y., et al. (2024). The effect of type and combination of fertilizers on eukaryotic microbiome of date palm rhizosphere. Plant Growth Regul. 103 (2), 439–451. doi:10.1007/s10725-024-01121-5
Shang, X., Fu, S., Guo, X., Sun, Z., Liu, F., Chen, Q., et al. (2023). Plant growth-promoting rhizobacteria microbial fertilizer changes soils’ microbial structure and promotes Healthy growth of Cigar tobacco plants. Agronomy 13 (12), 2895. doi:10.3390/agronomy13122895
Shao, D., He, Y., Zhai, Y., Yang, X., Guo, Z., Tan, J., et al. (2025). Mechanisms of tomato growth promotion in three soils after applying Bacillus combinations. Soil Tillage Res. 249, 106477. doi:10.1016/j.still.2025.106477
Shen, M.-C., Zhang, Y.-Z., Bo, G.-D., Yang, B., Wang, P., Ding, Z.-Y., et al. (2022). Microbial responses to the reduction of chemical fertilizers in the rhizosphere soil of flue-cured tobacco. Front. Bioeng. Biotechnol. 9, 812316. doi:10.3389/fbioe.2021.812316
Su, J., Chen, Y., Zhu, Y., Xiang, J., Zou, C., Hu, B., et al. (2020). The response of Hongda, a flue-cured tobacco cultivar, to nitrogen fertilizer rate. Archives Agron. Soil Sci. 67, 536–550. doi:10.1080/03650340.2020.1739658
Sun, R., Zhang, X.-X., Guo, X., Wang, D., and Chu, H. (2015). Bacterial diversity in soils subjected to long-term chemical fertilization can be more stably maintained with the addition of livestock manure than wheat straw. Soil Biol. Biochem. 88, 9–18. doi:10.1016/j.soilbio.2015.05.007
Tao, C., Li, R., Xiong, W., Shen, Z., Liu, S., Wang, B., et al. (2020). Bio-organic fertilizers stimulate indigenous soil Pseudomonas populations to enhance plant disease suppression. Microbiome 8, 137–214. doi:10.1186/s40168-020-00892-z
Van Bruggen, A. H. C., Goss, E. M., Havelaar, A., Van Diepeningen, A. D., Finckh, M. R., and Morris, J. G. (2019). One Health - cycling of diverse microbial communities as a connecting force for soil, plant, animal, human and ecosystem health. Sci. Total Environ. 664, 927–937. doi:10.1016/j.scitotenv.2019.02.091
Verzeaux, J., Alahmad, A., Habbib, H., Nivelle, E., Roger, D., Lacoux, J., et al. (2016). Cover crops prevent the deleterious effect of nitrogen fertilisation on bacterial diversity by maintaining the carbon content of ploughed soil. Geoderma 281, 49–57. doi:10.1016/j.geoderma.2016.06.035
Wang, B., Sun, M., Yang, J., Shen, Z., Ou, Y., Fu, L., et al. (2022a). Inducing banana Fusarium wilt disease suppression through soil microbiome reshaping by pineapple–banana rotation combined with biofertilizer application. Soil 8 (1), 17–29. doi:10.5194/soil-8-17-2022
Wang, T., Cheng, K., Huo, X., Meng, P., Cai, Z., Wang, Z., et al. (2022b). Bioorganic fertilizer promotes pakchoi growth and shapes the soil microbial structure. Front. plant Sci. 13, 1040437. doi:10.3389/fpls.2022.1040437
Wang, Y., Tang, X., Jiang, Z., Zhou, J., Zhang, S., Liu, J., et al. (2024). Effects of long-term combined application of organic-inorganic fertilizers on rice yield, nitrogen uptake and utilization in a rice-wheat rotation system. Chin. J. Soil Sci. 55 (02), 401–411.
Wang, Z., Zhang, Y., Bo, G., Zhang, Y., Chen, Y., Shen, M., et al. (2022c). Ralstonia solanacearum Infection disturbed the microbiome structure throughout the Whole tobacco crop Niche as Well as the nitrogen metabolism in soil. Front. Bioeng. Biotechnol. 10, 903555. doi:10.3389/fbioe.2022.903555
Xian, W., Zhang, X., and Li, W. (2020). Research status and prospect on bacterial phylum Chloroflexi. Acta Microbiol. Sin. 60 (09), 1801–1820.
Xiong, W., Guo, S., Jousset, A., Zhao, Q., Wu, H., Li, R., et al. (2017). Bio-fertilizer application induces soil suppressiveness against Fusarium wilt disease by reshaping the soil microbiome. Soil Biol. Biochem. 114, 238–247. doi:10.1016/j.soilbio.2017.07.016
Yan, L., Jianlin, W., Lei, M., Xiaobin, W., Fuli, Z., Rongzong, C., et al. (2024). Enhancing wheat yield through microbial organic fertilizer Substitution for partial chemical fertilization: regulation of nitrogen conversion and utilization. J. Soil Sci. Plant Nutr. 24 (1), 935–943. doi:10.1007/s42729-023-01597-6
Yonghui, Y., Minjie, L., Jicheng, W., Xiaoying, P., Cuimin, G. S., and Tang, D. W. S. (2022). Impact of combining long-term subsoiling and organic fertilizer on soil microbial biomass carbon and nitrogen, soil enzyme activity, and water use of winter wheat. Front. Plant Sci. 12, 788651. doi:10.3389/fpls.2021.788651
Yu, X., Zhang, Y., Shen, M., Dong, S., Zhang, F., Gao, Q., et al. (2023a). Soil Conditioner affects tobacco rhizosphere soil Microecology. Microb. Ecol. 86 (1), 460–473. doi:10.1007/s00248-022-02030-8
Yu, Y., Liu, H., Kuang, C., Gong, M., Dong, L., and Cao, H. (2023b). Structural and functional Differentiation of soil bacterial Sub-communities under different Gardens. Soils 55 (05), 1035–1043.
Zhang, J., Zheng, L., Shi, Y., Zhang, Z., Ma, X., Shen, G., et al. (2012). Effects of different planting patterns on soil microbial community, yield and quality of flue-cured tobacco leaves. Trans. Chin. Soc. Agric. Eng. 28 (19), 93–102.
Zhang, L., Liu, H., Gu, J., Lei, Q., Yin, Y., Qu, J., et al. (2013). Co-effect of compound microbial inoculum and organic-inorganic fertilizer on growth, yield and quality of flue-cured tobacco. Tob. Sci. Technol. 12, 67–73.
Zhang, Y., Bo, G., Shen, M., Shen, G., Yang, J., Dong, S., et al. (2022). Differences in microbial diversity and environmental factors in ploughing-treated tobacco soil. Front. Microbiol. 13, 924137. doi:10.3389/fmicb.2022.924137
Zhao, Y., Fu, C., Liu, Y., Xiang, D., Wang, Z., Qin, H., et al. (2024). Effects of adding Microalgae and Cow manure organic fertilizer on soil chemical properties and bacterial community, yield and quality of flue-cured. J. Yunnan Agric. Univ. Nat. Sci. 39 (03), 172–181.
Zhou, J., and Ning, D. (2017). Stochastic community assembly: Does it matter in microbial ecology? Microbiol. Mol. Biol. Rev. 81 (4), e00002-17–e00017. doi:10.1128/mmbr.00002-17
Zhou, X., Gao, D., Liu, J., Qiao, P., Zhou, X., Lu, H., et al. (2014). Changes in rhizosphere soil microbial communities in a continuously monocropped cucumber (Cucumis sativus L.) system. Eur. J. Soil Biol. 60, 1–8. doi:10.1016/j.ejsobi.2013.10.005
Zhou, X., Zhang, J., u Rahman, M. K., Gao, D., Wei, Z., Wu, F., et al. (2023). Interspecific plant interaction via root exudates structures the disease suppressiveness of rhizosphere microbiomes. Mol. plant 16 (5), 849–864. doi:10.1016/j.molp.2023.03.009
Keywords: microbial organic fertilizer, tobacco cultivation, soil physicochemical properties, soil bacterial community, sustainable agriculture
Citation: Wang S, Zhang B, Ma S, Hao J, Zhang L, Guo C, Hong J, Ding H, Zhang Y, Wu Y, Wang W, Sun J, Xing S, Yang J and Shen G (2025) Effects of microbial organic fertilizers on soil microbial communities and physicochemical properties in tobacco cultivation. Front. Environ. Sci. 13:1555622. doi: 10.3389/fenvs.2025.1555622
Received: 05 January 2025; Accepted: 05 March 2025;
Published: 26 March 2025.
Edited by:
Zhiguang Liu, Shandong Agricultural University, ChinaReviewed by:
Ahmad Ali, Florida Agricultural and Mechanical University, United StatesCopyright © 2025 Wang, Zhang, Ma, Hao, Zhang, Guo, Hong, Ding, Zhang, Wu, Wang, Sun, Xing, Yang and Shen. This is an open-access article distributed under the terms of the Creative Commons Attribution License (CC BY). The use, distribution or reproduction in other forums is permitted, provided the original author(s) and the copyright owner(s) are credited and that the original publication in this journal is cited, in accordance with accepted academic practice. No use, distribution or reproduction is permitted which does not comply with these terms.
*Correspondence: Jianming Yang, eWptaW5nODg4QDEyNi5jb20=; Guoming Shen, c2hlbmd1b21pbmdAY2Fhcy5jbg==
Disclaimer: All claims expressed in this article are solely those of the authors and do not necessarily represent those of their affiliated organizations, or those of the publisher, the editors and the reviewers. Any product that may be evaluated in this article or claim that may be made by its manufacturer is not guaranteed or endorsed by the publisher.
Research integrity at Frontiers
Learn more about the work of our research integrity team to safeguard the quality of each article we publish.