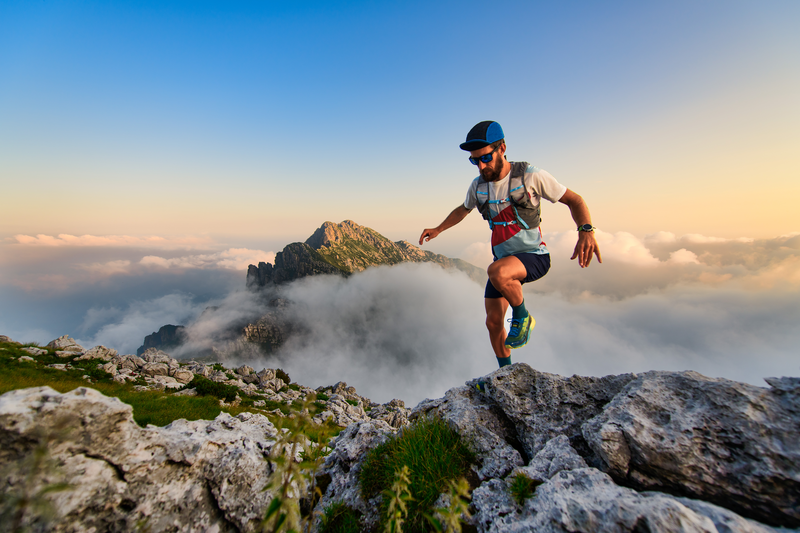
94% of researchers rate our articles as excellent or good
Learn more about the work of our research integrity team to safeguard the quality of each article we publish.
Find out more
ORIGINAL RESEARCH article
Front. Environ. Sci.
Sec. Environmental Economics and Management
Volume 13 - 2025 | doi: 10.3389/fenvs.2025.1552533
This article is part of the Research Topic Promoting Sustainable Urban Development: Cultivating Climate-Resilient Cities and Nurturing an Environmentally Conscious Lifestyle View all 5 articles
The final, formatted version of the article will be published soon.
You have multiple emails registered with Frontiers:
Please enter your email address:
If you already have an account, please login
You don't have a Frontiers account ? You can register here
Scientific understanding of China's ecological resilience and urban-rural dynamics supports comprehensive environmental and socio-economic advancement. This research utilizes an integrated coupling coordination framework to examine the relationship among ecological resilience and ruralurban dynamics in 31 Chinese provinces from 2011 to 2022. The spatiotemporal dynamics of ecological resilience-urban-rural coupling are examined through kernel density estimation and complementary methods. An LSTM network is used to forecast trends (2023-2030) and identify underlying patterns. Panel VAR is applied to explore the dynamic interactions between ecological resilience and urban-rural dynamics. The findings reveal regional disparities, with urban-rural dynamics consistently outperforming ecological resilience across all regions, while exhibiting lower variability. The coordination between ecological resilience and urban-rural dynamics shows an upward trend with moderate concentration and distinct regional variations. Projections for 2023-2030 indicate fluctuating yet upward trends in provincial-level coordination. Provincial development transitions from near-imbalance and marginal coordination pre-2026 to primary and intermediate coordination phases post-2026. The coordination levels across the four regions are ranked in descending order as follows: the eastern part of China, followed by the western, midland, and northeast areas. Nationwide analysis reveals significant autocorrelation in ecological resilience (5% level) and urban-rural dynamics (1% level), with urban-rural dynamics exerting a stronger influence on ecological resilience (1% level). This study elucidates the ecological resilience-urban-rural nexus, offering empirical foundations for China's sustainable urban-rural development strategies.
Keywords: Ecological resilience, urban-rural integrated development, Coupling coordination degree, Long short term memory network, Panel vector autoregression
Received: 07 Jan 2025; Accepted: 03 Mar 2025.
Copyright: © 2025 Wang, Song, Zhu, Jiang and Zhang. This is an open-access article distributed under the terms of the Creative Commons Attribution License (CC BY). The use, distribution or reproduction in other forums is permitted, provided the original author(s) or licensor are credited and that the original publication in this journal is cited, in accordance with accepted academic practice. No use, distribution or reproduction is permitted which does not comply with these terms.
* Correspondence:
Zhihui Jiang, Tarim University, Aral, China
Disclaimer: All claims expressed in this article are solely those of the authors and do not necessarily represent those of their affiliated organizations, or those of the publisher, the editors and the reviewers. Any product that may be evaluated in this article or claim that may be made by its manufacturer is not guaranteed or endorsed by the publisher.
Research integrity at Frontiers
Learn more about the work of our research integrity team to safeguard the quality of each article we publish.