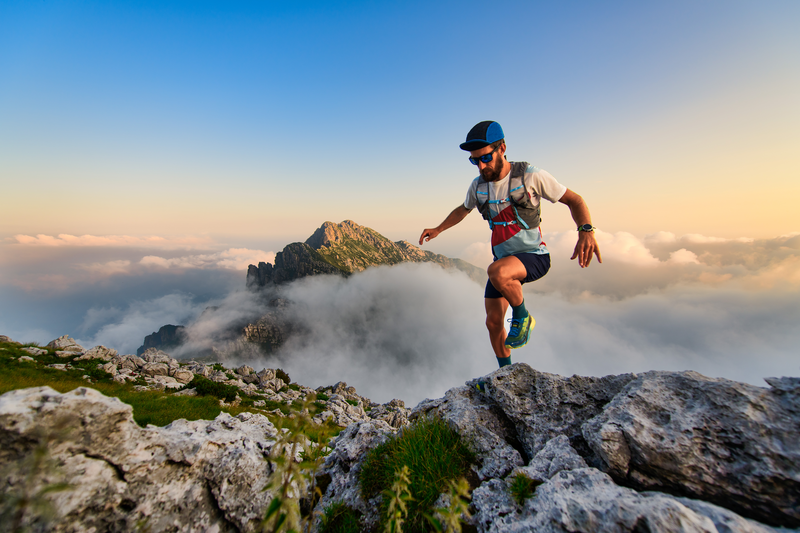
95% of researchers rate our articles as excellent or good
Learn more about the work of our research integrity team to safeguard the quality of each article we publish.
Find out more
ORIGINAL RESEARCH article
Front. Environ. Sci. , 24 March 2025
Sec. Soil Processes
Volume 13 - 2025 | https://doi.org/10.3389/fenvs.2025.1548881
Introduction: Soil erosion and land degradation pose significant threats to sustainable resource management in the Beshilo Watershed, Northeastern Ethiopia. These challenges are driven by steep slopes, unsustainable agricultural practices, and deteriorating soil health, necessitating a comprehensive assessment to inform effective mitigation strategies.
Methods: This study integrates geospatial analysis and field surveys to evaluate land degradation severity and identify soil erosion hotspots. The Revised Universal Soil Loss Equation (RUSLE) model, land use/land cover (LULC) analysis, and normalized difference vegetation index (NDVI) mapping are employed alongside FAO soil data, including soil organic matter (SOM), texture, and permeability assessments. GPS-documented inventories of active landslides and gullies further enhance the analysis.
Results: The findings reveal alarming soil erosion rates, with RUSLE values ranging from 0 to 1,288 t/ha/year, an average annual loss of 68.3 t/ha/year, and a total soil loss of 664,333 tonnes-far exceeding Ethiopia’s tolerable limits of 12-18 t/ha/year. Approximately 38.5% of the watershed is categorized as high or severe erosion risk. GIS analysis reports a standard deviation of 100.06 for soil loss, highlighting spatial variability. LULC analysis shows that 73.4% of the area is under cultivation, while forest cover has declined to 7%. NDVI values range from -0.24 to 0.58, indicating severe vegetation degradation. Soil assessments report low SOM levels (1-3%), weak soil structure ratings (1-4), and a predominance of sandy loam texture (78%), all contributing to heightened erosion vulnerability.
Discussion and Conclusion: The results highlight the urgent need for targeted Sustainable Land Management (SLM) interventions to mitigate soil erosion and rehabilitate degraded lands. Recommended measures include terracing, agroforestry, vegetative buffer strips, and integrated soil and water conservation strategies. These findings provide essential data to support evidence-based policymaking and the implementation of effective land management practices in erosion-prone regions.
Soil erosion and Land degradation represent critical global environmental challenges, threatening food security, biodiversity, and socio-economic stability (Gupta, 2019; Hossain et al., 2020). The Food and Agriculture Organization (FAO) estimates that approximately one-third of the Earth’s surface is degraded, with the loss of millions of hectares of arable land each year (Food and Agriculture Organization of the United Nations, 2019). Soil erosion, as the most pervasive form of land degradation, exacerbates poverty, contributes to climate change, and disrupts ecosystem services, making it one of the most urgent threats to global sustainability (Akça et al., 2022; Trivedi et al., 2018; Tunçay and Başkan, 2022). Globally, about 23–42 billion tons of fertile topsoil are lost annually, undermining agricultural productivity and amplifying food insecurity (Pimentel and Burgess, 2013). Regions like sub-Saharan Africa, where economies rely heavily on rain-fed agriculture, are disproportionately affected. For instance, Africa loses an estimated 280 million tons of topsoil each year, further entrenching poverty and food scarcity (UNEP, 2016).
Ethiopia, known as the “water tower” of Eastern Africa, exemplifies these challenges with its highland regions facing intense soil erosion due to steep slopes, deforestation, overgrazing, and erratic rainfall patterns. Annual soil loss in Ethiopia ranges from 20 to 100 tons per hectare in the highlands, causing a significant 2% decline in the nation’s agricultural GDP (Taye et al., 2018). The Beshilo Watershed in northeastern Ethiopia, located within the Blue Nile Basin, is a critical hotspot for soil erosion. Unsustainable land-use practices, combined with population pressures, exacerbate the loss of fertile soil, impair water quality, and increase sedimentation in key reservoirs like the Grand Ethiopian Renaissance Dam (Wodaje, 2016; Mekonnen et al., 2022). These cascading effects have far-reaching implications for both local livelihoods and regional energy and water resource management.
Despite global recognition of land degradation as a critical environmental issue, significant gaps persist in the spatial identification and monitoring of soil erosion hotspots, particularly in data-scarce regions like Ethiopia. While the Universal Soil Loss Equation (USLE) and its revised version (RUSLE) provide reliable models for estimating erosion, their application in Ethiopia has been limited by the lack of high-resolution data and comprehensive field validation (Ayalew and Selassie, 2015; Pal and Chakrabortty, 2019a; Teku et al., 2024). Moreover, there is insufficient integration of advanced geospatial technologies such as Geographic Information Systems (GIS) and Remote Sensing (RS) in erosion assessments within the Ethiopian Highlands. Previous studies, such as those by Taye et al. (2018), Bewket and Teferi (2009), and Wolka (2014), have predominantly employed the Revised Universal Soil Loss Equation (RUSLE) or the Soil and Water Assessment Tool (SWAT) using remotely sensed data. While these approaches have provided valuable insights, they often faced limitations in accuracy due to the lack of ground-truthing, site-specific rainfall data, and high-resolution land cover information. Moreover, most existing research has been conducted at regional or national scales, such as the work by Abate (2016) and Hurni et al. (2015), which lacked the micro-watershed-level analyses necessary for designing localized land management interventions. Additionally, studies by Hagos et al. (2023) and Nigussie et al. (2017) highlighted soil erosion risk zones but failed to incorporate historical land-use changes, which are critical for understanding long-term degradation patterns. Similarly, research by Demissie et al. (2022) and Desalegn et al. (2023) often relied on coarse-resolution Digital Elevation Models (DEMs), leading to underestimation or overestimation of slope-based erosion risks.
The absence of systematic field validation further limited the reliability of erosion modeling outputs in most cases. Although numerous studies have been conducted to assess soil erosion across Ethiopia, including works by Haregeweyn et al. (2017), Gashaw et al. (2018), and Mekonnen et al. (2022), many of these investigations primarily relied on empirical models, often overlooking the integration of field-based validation with advanced geospatial techniques. Moreover existing studies often focus on broader regions without detailed analysis of specific watersheds like Beshilo, which is critical for developing localized and actionable interventions. Therefore, this study aims to address these knowledge gaps by integrating geospatial techniques and field surveys to: (1) Map and quantify soil erosion hotspots within the Beshilo Watershed, (2) Assess the underlying drivers of soil erosion and land degradation in the region. (3) Provide evidence-based recommendations for sustainable land management practices tailored to the local context. This study presents a more refined and high-precision approach to assessing soil erosion hotspots and land degradation in the Beshilo Watershed by integrating advanced geospatial techniques with rigorous field validation. Unlike previous studies that relied on broad-scale datasets and generalized models, this research utilizes satellite-derived rainfall data from CHIRPS (2000–2023), validated with ground-based observations, to enhance the accuracy of rainfall erosivity estimations. Additionally, elevation, slope, and drainage characteristics are analyzed using the high-resolution SRTM Digital Elevation Model (30 m), providing a more detailed representation of topographic influences on soil erosion. A notable distinction of this study is its exclusive use of Landsat imagery (TM, ETM+, OLI) for generating the 2024 Land Use/Land Cover (LULC) map, avoiding machine-learning-based classification and omitting Sentinel-2 data. The LULC classification is systematically verified using ground control points obtained from field surveys, topographical maps, and high-resolution Google Earth imagery, ensuring greater accuracy and reliability.
By integrating site-specific rainfall erosivity factors, high-resolution terrain analysis, and detailed LULC classification, this research significantly enhances the precision of erosion risk assessments. Unlike interpolated regional datasets, the long-term ground-based rainfall data incorporated here provides more site-relevant insights into erosive rainfall patterns. Moreover, the localized sub-watershed analysis enables the identification of micro-watersheds most susceptible to soil erosion, allowing for more targeted and effective conservation interventions. Through systematic validation and robust methodological improvements, this study fills critical knowledge gaps in soil erosion modeling. The findings offer actionable insights for policymakers, land managers, and development practitioners, supporting evidence-based soil and water conservation strategies that are crucial for sustainable land management in Ethiopia and other ecologically vulnerable regions. By employing GIS and RS tools, coupled with field surveys, this research delivers a scalable and replicable methodology for assessing soil erosion risks in similar regions globally. The findings are directly relevant to policymakers, researchers, and local communities, offering actionable insights to enhance agricultural productivity, safeguard ecosystem services, and build climate resilience. This research is particularly significant in light of global efforts to combat land degradation and promote sustainable development, as reflected in initiatives like the United Nations Sustainable Development Goals (SDGs), specifically Goal 15, which calls for combating desertification and restoring degraded land. By contributing to these broader objectives, this study not only addresses local challenges in Ethiopia but also provides a framework for tackling land degradation in other vulnerable regions worldwide.
The Beshilo Watershed, located in the northeastern highlands of Ethiopia within the Amhara region, is an ecologically and economically vital area characterized by its diverse landscapes and complex topography. Spanning an expansive area, the watershed lies between 420,000m and 570,000 m North latitude and 1,200,000 m to 1,320,000 m East longitude (Figure 1). It features steep mountainous terrains, undulating hills, and interconnected valley systems. Elevations vary significantly, ranging from approximately 1,208 m in the lowlands to 4,266 m in the highlands. This dramatic elevation gradient not only shapes the region’s geomorphology and hydrology but also strongly influences land use patterns and agricultural activities. The watershed is traversed by a well-developed drainage network, dominated by the Beshilo River and its tributaries, which carve deep valleys and steep slopes as they flow into the Blue Nile. The hydrology of the watershed is highly seasonal, with high water flow during the June–September rainy season and reduced flow during the dry months (Ahmad, et al., 2020). The steep terrain, coupled with intense seasonal rainfall, exacerbates soil erosion, particularly in areas with insufficient vegetation cover (Mohammed and Yimam, 2022). This erosion contributes to sedimentation in downstream reservoirs and rivers, negatively impacting water availability and quality (Mekonnen et al., 2022).
Geologically, the Beshilo watershed is underpinned by volcanic formations, including basaltic and tuffaceous rocks typical of the Ethiopian highlands. Over time, these formations have weathered into diverse soil types, each exhibiting distinct erosion behaviors. Dominant soils include Eutric Cambisols, Eutric Leptosols, Eutric Vertisols, and Lithic Leptosols (Figure 2a). Eutric Cambisols, often found on highland slopes, are moderately fertile and well-drained, while Lithic Leptosols, located in valley bottoms, are nutrient-rich but poorly drained. Shallow Eutric Leptosols, prone to erosion, dominate steep slopes. Texturally, sandy loam is the most common soil type, followed by clay, with the combination of steep topography and fragile soils intensifying erosion and land degradation processes. Climatically, the Beshilo watershed falls within the tropical highland zone, experiencing a bimodal rainfall pattern. The primary rainy season, from June to September, provides the majority of the annual precipitation, while a shorter rainy period occurs between March and May. Annual rainfall ranges from approximately 600 mm in the lowlands to over 1,400 mm in the highlands (Figure 2b), while average temperatures also vary with elevation, creating distinct agro-ecological zones (Figure 2c). The highlands are classified as moist or wet Dega zones, supporting crops like barley and wheat, while mid-elevation areas, or Woina Dega zones, are suitable for teff, maize, and sorghum. The lower elevations, classified as Kolla zones, are characterized by less reliable rainfall, limiting agriculture to drought-resistant crops.
Figure 2. Soil type (a), Rainfall distribution (b), traditional agroecological zone (c), and LULC (d) map of the study area.
Despite its ecological diversity, the Beshilo watershed faces significant challenges due to high population pressure. It supports a predominantly rural population heavily reliant on subsistence agriculture, which combines crop cultivation and livestock rearing. Approximately 73% of the land is cultivated, while forested areas account for only 7% of the total area (Figure 2d). Agriculture has expanded onto steep slopes, driven by limited arable land, contributing to deforestation and exacerbating soil degradation. The lack of adequate soil conservation practices, coupled with unsustainable farming and grazing, has accelerated erosion, threatening agricultural productivity and livelihoods in the region.
To comprehensively capture land cover dynamics and vegetation health, this study relies on high-resolution satellite imagery from platforms such as Landsat. Landsat imagery provides detailed multispectral and hyperspectral data, enabling robust vegetation analyses, including the derivation of the Normalized Difference Vegetation Index (NDVI). The NDVI serves as a critical indicator of vegetation cover changes, highlighting areas experiencing significant degradation. To enhance the spatial resolution and validate erosion features, Google Earth Pro, combined with field verification, is used to create an inventory of active gullies and landslides, particularly in inaccessible regions. This hybrid approach ensures reliable mapping and enhances the accuracy of spatial datasets. Additionally, Digital Elevation Models (DEMs) sourced from the Shuttle Radar Topography Mission (SRTM) provide foundational terrain parameters, including: Slope steepness, Slope length, Flow accumulation, and Drainage patterns.
These topographic metrics are essential for modeling soil erosion dynamics as they directly influence sediment transport, water flow paths, and erosion potential. Gaps and sinks in the DEM data are addressed using advanced processing techniques to ensure accuracy in hydrological modeling. Supplementary spatial layers, such as agro-ecological zones, elevation gradients, and watershed boundaries, are integrated to refine the analysis. By combining satellite imagery, remote sensing tools, and DEM-derived data, the study adopts a multi-faceted approach to identify the drivers and extents of land degradation, laying the groundwork for evidence-based conservation strategies.
Structured field surveys were a critical component of this study, designed to validate and enhance the accuracy of remote sensing outputs. A systematic stratified sampling approach was employed to select field survey sites, ensuring comprehensive representation across varying land cover types, slope gradients, and erosion-prone areas. The study area was divided into homogeneous land units based on satellite-derived land use and topographic classifications, from which sampling sites were randomly selected within each unit. In total, 57 field sites were surveyed across the Beshilo Watershed, encompassing areas with varying erosion risk levels, land use patterns, and topographical conditions. At each site, detailed observations were conducted, including visual assessments of erosion features (such as rills, gullies, and sheet erosion), vegetation cover, soil stability, and land management practices. GPS coordinates were recorded for each observation point, enabling precise spatial correlation with remote sensing datasets. Furthermore, a comprehensive gully and landslide inventory was developed using a hybrid approach that combined high-resolution imagery from Google Earth Pro with on-ground field validation. This inventory identified and mapped 671 gullies and 578 individual landslides, with GPS-referenced measurements recorded for each feature.
Geographic Information Systems (GIS) form the cornerstone of the analytical framework for assessing land degradation and soil erosion. By enabling the seamless integration and analysis of spatial datasets, GIS facilitates the generation of thematic maps that capture key factors influencing soil erosion dynamics. These include the R-Map, which represents the rainfall erosivity factor; the K-Map, reflecting soil erodibility; the C-Map, illustrating land cover and vegetation influence; the LS-Map, highlighting topographic features such as slope length and steepness; and the P-Map, which accounts for conservation practices. The integration of these thematic maps culminates in the development of a comprehensive erosion risk map, providing a spatially explicit evaluation of soil erosion hotspots and land degradation risks. Moreover, the gully and landslide inventory map further enhances the analysis by offering insights into the spatial distribution of erosion features, thereby supporting targeted mitigation strategies.
The study applies the Revised Universal Soil Loss Equation (RUSLE) to estimate annual soil loss and identify soil erosion risk areas. The RUSLE model is defined as Equation 1:
Where, A = Average annual soil loss (t/ha/yr), R = Rainfall-runoff erosivity factor (MJ mm ha−1 h−1 yr−1), K = Soil erodibility factor (t h MJ−1 mm−1), LS = Slope length and steepness factor (dimensionless), C = Cover management factor (dimensionless), P = Support practice factor (dimensionless).
The R-factor quantifies the erosive potential of rainfall. Given the scarcity of detailed rainfall intensity data in Ethiopia, the study adopts Hurni’s (1985) erosivity model, which is tailored to Ethiopian highland conditions:
where, P = Mean annual rainfall (mm).
Hurni’s model is widely validated in Ethiopian studies and offers a practical alternative for data-scarce regions.
The K-factor represents the susceptibility of soil to erosion, determined by its texture, structure, and organic matter content. To quantify this factor, soil data is sourced from the Harmonized World Soil Database (HWSD). Considering the challenges associated with field-based measurements, the study employs the empirical equation proposed by Wischmeier and Smith (1978):
where, clay = Clay fraction (%), OM = Organic matter content (%), sand = Sand fraction (%).
This method provides a pragmatic balance between accuracy and data availability, allowing spatial mapping of soil erodibility.
The LS-factor accounts for slope length and steepness, which are critical in the rugged terrains of the Ethiopian highlands. DEMs from SRTM with a 12.5 m resolution are processed using GIS algorithms developed by Moore and Wilson (1992). The LS-factor is calculated as:
Where, S represents the slope in percentage, and m is an empirically determined constant that depends on slope length, typically 1.4 for topographically diverse regions like Ethiopia. This method ensures accurate quantification of terrain impacts on soil erosion.
The C-factor represents the protective effect of vegetation cover on soil, mitigating erosion by reducing the impact of rainfall and surface runoff. To accurately estimate the C-factor, a detailed maximum likelihood supervised Land Use Land Cover (LULC) classification was conducted using Landsat imagery, followed by local adjustments informed by field observations, GPS data, and Google Earth analysis. Subsequently, C-factor values were assigned to each LULC category based on literature specific to Ethiopian conditions. This approach ensures a more realistic and contextually accurate representation of the C-factor, thereby enhancing the model’s reliability, as supported by Oliveira et al. (2013).
In this study, the P-factor, which represents the effect of conservation practices on reducing soil erosion, was assigned based on the integration of Land Use/Land Cover (LULC) types and slope categories for the Beshilo watershed in Northeastern Ethiopia. This integration of LULC data with slope categories derived from a Digital Elevation Model (DEM) offers an efficient and robust approach for estimating the P-factor at a regional scale. Following the methodology outlined by Wischmeier and Smith (1978) and tailored to Ethiopian conditions, LULC was categorized into agricultural and non-agricultural classes. This approach ensures that the P-factor estimation reflects the unique land use patterns and topographic characteristics of the region, enhancing the model’s accuracy and applicability.
To effectively identify and evaluate areas at high risk of soil erosion and land degradation, this study employs the Revised Universal Soil Loss Equation (RUSLE) integrated with advanced spatial analysis techniques. The RUSLE model is used to classify soil erosion severity into distinct categories based on thresholds established by Food and Agriculture Organization of the United Nations (2018). This classification enables the identification of areas where soil loss surpasses tolerable limits, designating them as erosion hotspots. This approach provides critical insights for prioritizing intervention strategies in regions most vulnerable to land degradation.
This study employs an integrated approach combining GIS, remote sensing techniques, and field observations to comprehensively assess the extent and severity of land degradation in the Beshilo watershed, Northeastern Ethiopia. Key indicators analyzed include soil health parameters from the FAO World Soil Database, the Normalized Difference Vegetation Index (NDVI), Revised Universal Soil Loss Equation (RUSLE)-based soil erosion estimates, and field-documented gully and landslide data. Using a GIS framework, the RUSLE parameters (R, K, LS, C, and P) were integrated to produce a spatially explicit soil erosion risk map. By combining field observations with remote sensing data and geospatial analysis, the study effectively identifies erosion-prone areas and land degradation hotspots within the watershed. This comprehensive methodology ensures accurate and actionable results, providing a critical foundation for designing and implementing targeted conservation strategies.
This study utilized a wide array of primary and secondary data sources, ensuring a comprehensive analysis of the Beshilow watershed. Key datasets included satellite images, geological and hydrogeological maps, topographic maps, administrative boundaries, and field observations. Secondary data were obtained from peer-reviewed scientific literature, official government records, census reports, and project documentation. A summary of the data and their sources is presented in Table 1, and the general work flow of the study is illustrated in Figure 3.
Figure 3. General work flow of the study area. R-map (a), K-map (b), LS–map (c), C-map (d) of the study area. P-map (e), NDVI-map (f) of the study area.
The R-factor values in the Beshilo watershed were spatially analyzed using Equation 2, as illustrated in the R-factor map. The results reveal a significant spatial variability in rainfall erosivity across the watershed, with values ranging between 428 and 770 MJ mm ha−1 h−1 year−1(Figure 3a). This variation underscores the heterogeneity of rainfall intensity within the region. The southeastern and southwestern zones of the watershed exhibit the highest R-factor values, ranging from 601 to 770 MJ mm ha−1 h−1 year−1. These elevated values are attributed to the higher rainfall intensity and prolonged precipitation events characteristic of these areas, as corroborated by the spatial rainfall distribution map. In contrast, the northern and central regions demonstrate lower R-factor values, ranging from 428 to 550 MJ mm ha−1 h−1 year−1, indicating reduced rainfall erosivity and comparatively lower erosion risks.
The observed R-factor values align with findings from previous studies in Ethiopian highlands. For instance, Hurni (1985) reported R-factor values between 500 and 800 MJ mm ha−1 h−1 year−1 for high rainfall regions, which corresponds closely to the results observed in the Beshilo watershed, particularly in its southern zones. Similarly, studies by Bewket and Teferi (2009) and Mekonnen et al. (2023) have identified analogous R-factor ranges within highland watersheds, further affirming the direct correlation between rainfall intensity and erosivity potential. The spatial variability of R-factor in the Beshilo watershed also resonates with the findings of Gessesse et al. (2015), who emphasized the influence of micro-climatic conditions and elevation gradients on rainfall erosivity. Specifically, the pronounced erosivity in the southern portions of the watershed highlights the urgent need for targeted soil and water conservation interventions in these areas to mitigate erosion risks effectively. Overall, the spatial distribution of R-factor values in the Beshilo watershed provides critical insights into the rainfall-driven erosion processes, serving as a foundation for implementing site-specific erosion control measures and sustainable land management strategies.
The K-factor, representing soil erodibility, was calculated using Equation 3, and the results are displayed spatially in the provided map. The K-factor values in the Beshilo watershed range from 0.1328 to 0.1629 t h MJ−1 mm−1 (Figure 3b), indicating varying levels of soil susceptibility to erosion across the watershed. The areas with higher K-values (0.1395–0.1629) are concentrated in the northern and eastern parts of the watershed, where soil characteristics such as silt and low organic matter content contribute to increased erodibility. Conversely, lower K-values (0.1328–0.1346) are predominant in the southern and central regions, reflecting soils with higher clay content and structural stability.
The observed spatial distribution of K-factor values highlights the influence of soil properties, including texture, structure, organic matter content, and permeability, on soil erodibility. The higher K-values in the northern and eastern regions suggest these areas are more prone to erosion, which aligns with findings from earlier studies in similar environments. For instance, Wischmeier and Smith (1978) emphasized that soils with high silt content and low organic matter are particularly vulnerable to erosion, which corresponds to the characteristics of the soils in the northern and eastern parts of the watershed. The results also align with studies conducted by Gessesse et al. (2015) and Taye et al. (2013), who reported similar K-factor ranges [0.10–0.20 t·ha·h/(ha·MJ·mm)] in Ethiopian highlands, emphasizing the inherent vulnerability of soils in these regions to erosion. The spatial variability observed in the Beshilo watershed further supports the findings of Bewket and Teferi (2009), who noted that variations in soil properties significantly influence erosion potential across micro-watersheds in Ethiopia. When compared to global studies, the K-factor values in the Beshilo watershed fall within the range typically reported for tropical and subtropical regions, as noted by Renard et al. (1997). However, the relatively low K-values in the southern and central regions indicate a comparative advantage in terms of soil resilience, which can be leveraged for sustainable land management.
The LS-factor, which represents the combined influence of slope length (L) and slope steepness (S) on soil erosion, was derived for the Beshilo watershed using Equation 4. This factor plays a pivotal role in evaluating erosion susceptibility by capturing the topographic attributes that influence runoff velocity and erosive energy across the landscape. In the Beshilo watershed, LS values exhibit a significant range, varying between 0 and 30.39 (Figure 3c), indicative of the heterogeneous topography and associated erosion potential within the study area. Low LS values are predominantly observed in areas characterized by gentle slopes and shorter slope lengths, where the potential for erosion is minimal due to limited runoff concentration and reduced flow velocity. In contrast, high LS values (approaching 30.39) are concentrated in regions with steep gradients and extended flow paths, conditions that exacerbate surface runoff velocity and subsequently amplify soil erosion risk. These high LS zones, primarily located in the steep upper reaches of the watershed, align with findings from prior studies in the Ethiopian highlands (Hurni, 1988), which underscore the direct correlation between steep slopes, longer slope lengths, and increased soil erosion vulnerability.
The calculated LS-factor for the Beshilo watershed falls within ranges reported for similar highland terrains in Ethiopia (Desale et al., 2023; Fenta et al., 2021), reinforcing the consistency of the results. Specifically, regions with slopes exceeding 30° exhibit disproportionately high LS values, reflecting their heightened susceptibility to erosion due to the intensified gravitational forces acting on surface runoff. This aligns with the observations of Fentaw and Abegaz. (2024), who highlighted the critical role of slope steepness in driving erosion dynamics in the Ethiopian highlands. The spatial variability of LS values across the Beshilo watershed underscores the need for targeted soil conservation interventions in erosion-prone areas. High LS zones, where steep and long slopes prevail, demand immediate and robust soil management measures such as terracing, afforestation, and check dam construction to mitigate runoff velocity and soil loss. As emphasized by Hurni (1988), integrating such conservation strategies in regions with high LS values is paramount to reducing soil erosion impacts and ensuring sustainable land productivity in fragile highland environments. Conversely, areas with low LS values, predominantly in flatter terrains, exhibit limited erosion risk due to lower runoff energy. Nevertheless, these zones also warrant attention for maintaining soil stability and preventing degradation over time. The LS-factor assessment in this study thus highlights the critical influence of topography on erosion processes and serves as a foundational tool for identifying erosion hotspots and prioritizing land management efforts in the Beshilo watershed.
The C-factor, representing the cover and management factor in the Revised Universal Soil Loss Equation (RUSLE), was assigned based on literature specific to Ethiopian and spatially mapped for the Beshilo watershed. The C-factor values exhibit significant spatial variability, ranging from 0 to 0.6 (Figure 3d; Table 2), which reflects the heterogeneity of land cover and management practices across the watershed. Low C-factor values (0–0.1) are concentrated in areas with dense vegetation cover, such as forests (7.12%) and certain portions of shrubland (12.70%), which provide effective soil protection through their vegetative cover. These regions intercept raindrops, reduce surface runoff velocity, and enhance infiltration, minimizing soil erosion risks. Moderate C-factor values (0.1–0.15) are predominantly observed in cultivated lands (73.43%) with partial vegetation cover or intermittent cropping systems. Although these areas retain some vegetation cover during cropping seasons, their soil remains vulnerable to erosion during fallow periods or periods of intense rainfall. On the other hand high C-factor values (0.6) are concentrated in barren lands and areas with minimal vegetation cover, particularly in the western and southwestern regions of the watershed. These areas are characterized by degraded landscapes, poor land management practices, and limited protective ground cover, making them most susceptible to soil erosion.
The spatial variability in C-factor values across the Beshilo watershed highlights the critical role of land use and vegetation cover in mitigating soil erosion risks. The high C-factor values observed in degraded and poorly managed areas align with findings from Gashaw et al. (2017), who reported similar patterns in regions with sparse vegetation and intensive human activities in the Ethiopian highlands. These findings are further corroborated by Bewket and Teferi (2009), who linked high C-factor values to overgrazed lands, unprotected slopes, and barren terrains, particularly in watersheds subjected to intensive agricultural pressure. In contrast, the low C-factor values in forested and well-vegetated regions of the Beshilo watershed resonate with observations by Haregeweyn et al. (2015). Their study emphasized the significant role of vegetation in reducing soil erosion by intercepting rainfall, diminishing surface runoff energy, and enhancing soil stability. This pattern is particularly notable in the northern and eastern zones of the watershed, where ongoing forest conservation measures and sustainable land management practices have been partially implemented. When compared to other Ethiopian watersheds, the C-factor values of the Beshilo watershed align with findings in the Chemoga watershed (Bewket and Teferi, 2009) and the Upper Blue Nile Basin (Hurni et al., 2005). These studies reported C-factor ranges of 0.0 to 0.65, with higher values corresponding to degraded and barren lands, and lower values linked to areas with dense vegetation or sustainable management practices. The results underscore the necessity for targeted vegetation restoration and land management interventions in regions with high C-factor values to mitigate soil erosion risks and enhance watershed sustainability.
In this study, the P-factor was assigned based on land use/land cover (LULC) types and slope categories for the Beshilo watershed, Northeastern Ethiopia. The P-factor reflects the impact of soil conservation practices on soil erosion, where lower values indicate more effective erosion control. The values were adopted from literature on Ethiopian highlands and adjusted to the study area as shown in Table 3; Figure 3e. The P-factor values used in this study follow the general framework for the Ethiopian highlands, where the risk of soil erosion is influenced by the combination of land use practices and slope gradients. Agricultural lands, especially those with slopes ranging from 0°–5° to 30°–50°, show varying degrees of protection against erosion due to conservation practices. The lower P-values for agricultural land (ranging from 0.1 to 0.33) are indicative of moderate erosion control in these areas. However, as slope increases, the effectiveness of conservation measures tends to decrease, leading to higher erosion risks. For agricultural areas with slopes greater than 30°, the P-factor increases to 0.25 and 0.33, reflecting a higher vulnerability to soil erosion due to the limited effectiveness of common conservation measures, such as terracing and contour farming, at steep gradients. This finding is consistent with the study by Hurni (1988), who identified steeper slopes in Ethiopian highlands as areas requiring more intensive soil conservation practices due to increased runoff and soil loss.
Conversely, non-agricultural lands have a P-value of 1, which assumes no active erosion control. These areas, often characterized by natural vegetation or barren land, face a higher erosion risk, particularly in regions with low vegetation cover or poor land management practices. Ejegu and Yegizaw (2021) highlighted that such lands, without proper conservation measures, are more susceptible to soil erosion, especially during heavy rainfall events. These findings align with regional studies such as Teshome et al. (2020), which pointed out that agricultural lands with moderate slopes benefit from effective conservation techniques, reducing the risk of erosion. However, lands with steep gradients require more advanced conservation strategies, such as check dams or improved agroforestry, to mitigate soil loss.
The spatial distribution of annual soil loss in the Beshilo watershed was analyzed using the Revised Universal Soil Loss Equation (RUSLE) model integrated with GIS. The analysis revealed significant spatial variability, with soil loss values ranging from 0 to 1,288 t/ha/year (Figure 4a). The total annual gross soil loss was estimated at 664,333 tonnes, with an average soil loss rate of 68.3 t/ha/year. This average far exceeds the tolerable limits of 12–18 t/ha/year for Ethiopia (Hurni, 1985) and the global benchmark of 40 t/ha/year (Pimentel and Kounang, 1998). The GIS analysis quantified a standard deviation of 100.06, highlighting pronounced heterogeneity in soil loss across the watershed. This spatial variability is attributed to differences in topography, land use/land cover (LULC), soil properties, rainfall intensity, and land management practices. Steep slopes, unsustainable agricultural activities, and degraded vegetation cover in particular contribute to heightened erosion risks in specific areas.
The findings align with studies conducted in similar Ethiopian highland watersheds, where topography and land management practices have been identified as major determinants of soil loss. For instance, Tebebu et al. (2015) reported comparable soil loss patterns in the Anjeni watershed, emphasizing that steep slopes and agricultural intensification are primary drivers of erosion in the Ethiopian highlands. Similarly, Hurni (1988) highlighted that soil erosion rates in Ethiopia’s highlands could exceed 300 t/year in cultivated lands with inadequate conservation measures, consistent with the upper ranges identified in this study. The extremely high soil loss rates in specific locations of the Beshilo watershed (above 1,288 t/year) are alarming. These values suggest severe land degradation, which not only threatens agricultural productivity but also contributes to sedimentation in downstream water bodies. Tamene and Vlek (2008) emphasized that such levels of soil erosion significantly hinder sustainable land management and exacerbate food insecurity in the region. Despite efforts to implement soil and water conservation measures, the results suggest that these interventions are either insufficient or poorly maintained, particularly in areas of high erosion risk. This finding resonates with the observations of Bewket and Sterk (2003), who noted that the effectiveness of conservation practices in Ethiopian highlands is often compromised by socioeconomic and institutional challenges.
Compared to global soil loss studies, the annual soil loss rates observed in the Beshilo watershed are among the highest, reflecting the unique challenges of managing erosion in the Ethiopian highlands. Pimentel and Kounang (1998) noted that global average soil loss on agricultural lands is approximately 10–40 t/ha/year, significantly lower than the average values observed in this study. The results also highlight the critical role of slope in exacerbating soil erosion. Similar observations were made by Wischmeier and Smith (1978), whose foundational work on RUSLE emphasized the nonlinear relationship between slope steepness and soil loss. In the Beshilo watershed, the steepest slopes are associated with the highest soil loss, a finding that aligns with these theoretical expectations. The discrepancy underscores the urgent need for targeted erosion control measures tailored to the region’s steep topography and intensive land use. Sustainable Land Management (SLM) strategies such as contour terracing, agroforestry, and vegetative buffer strips should be prioritized to mitigate soil loss and enhance land productivity in erosion-prone areas of the watershed.
This study integrated GIS, remote sensing techniques, and field observations to comprehensively assess the extent and severity of land degradation in the Beshilo watershed, Northeastern Ethiopia. The analysis employed critical indicators, including soil health parameters, Normalized Difference Vegetation Index (NDVI), Revised Universal Soil Loss Equation (RUSLE)-based soil erosion estimates, and field-documented gully and landslide data. The findings underscore significant spatial variability in soil health, erosion susceptibility, and vegetation cover, reflecting the area’s heightened vulnerability to land degradation.
Due to the large scale of the watershed, no field survey was conducted for soil health indicators; instead, the analysis relied on the FAO World Soil Database. The assessment of key soil health indicators, such as soil organic matter (SOM), soil texture, structure, and permeability, revealed significant variations across the watershed, strongly correlating with erosion dynamics. Soil organic matter, a critical indicator of soil fertility and structural stability, ranged from 1% to 3% (Table 4). Eutric Vertisols exhibited the highest SOM levels (3%), indicating better fertility and strong soil structure, while Lithic Leptosols showed critically low SOM levels (1%), reflecting weak soil structure and increased erosion susceptibility. These findings align with global benchmarks, where SOM levels below 2% are considered a threshold for poor soil health and heightened erosion risk (Lal, 2004). Soil permeability assessments further highlighted the watershed’s vulnerability. Eutric Leptosols demonstrated poor infiltration rates (0.3 mm/h), intensifying runoff and soil detachment, while Lithic Leptosols showed relatively higher permeability (7.5 mm/h). The weak soil structure (grade 1) and poor infiltration capacity of Eutric and Lithic Leptosols exacerbate erosion susceptibility, particularly during intense rainfall events (Pimentel, 2006). These results align with field GPS observations, where extensive gully formations and landslides were concentrated in areas dominated by soils with low SOM, weak structure, and limited permeability, reinforcing the strong link between soil health degradation and active erosion processes.
Table 4. Soil organic matter, texture, structure and permeability of the study area (FAO, 2015).
The RUSLE model estimated a mean annual soil loss of 68.3 t/ha/year, significantly exceeding global and national thresholds for tolerable soil loss. Globally, soil loss rates above 10–12 t/ha/year are considered unsustainable for agriculture (Pimentel, 2006), while within Ethiopia, the tolerable soil loss rate ranges from 2 to 18 t/ha/year (Hurni, 1985). Spatial analysis revealed pronounced variability in erosion severity across the watershed. Areas with minimal soil loss (<5 t/ha/year) accounted for 28.2% of the total area and were characterized by flat terrain and dense vegetative cover. Conversely, severe soil loss areas (>30 t/ha/year), constituting 38.3% of the watershed (Figure 4b, Table 5), were concentrated on steep slopes with sparse vegetation cover. These erosion hotspots were predominantly associated with active gully formations and landslide-prone zones, highlighting the interplay between terrain steepness, degraded soil structure, and reduced vegetation cover as key drivers of land degradation.
Table 5. Soil loss range and severity class (FAO, 2015).
The NDVI analysis further substantiated the severity of land degradation by quantifying vegetation cover variability across the watershed. NDVI values ranged from −0.24 to 0.58 (Figure 3F), with higher values (up to 0.58) observed in areas with dense vegetative cover, corresponding to lower erosion rates and improved soil stability. In contrast, areas with NDVI values as low as −0.24 exhibited sparse or absent vegetation, particularly on steep slopes, aligning closely with severe soil loss zones identified through RUSLE. Globally, NDVI values below 0.2 indicate poor vegetation health and increased soil erosion risk (Tucker and Sellers, 1986). The NDVI-based assessment thus reinforces the critical role of vegetation cover in mitigating erosion and land degradation.
Field GPS data revealed active gully formations and landslide occurrences concentrated in areas underlain by Lithic Leptosols and Eutric Leptosols. These soils, characterized by weak structure and low organic matter, exhibited poor resistance to detachment and transport processes, particularly under high-intensity rainfall events. Gullies and landslides further exacerbate the loss of topsoil, reduce agricultural productivity, and accelerate land degradation, reinforcing the findings from soil health and RUSLE-based erosion analyses.
The Beshilo watershed is significantly affected by land degradation, driven by unsustainable land use, deforestation, and steep terrain. To mitigate these challenges, adopting sustainable land management (SLM) practices is critical for preserving soil health, enhancing agricultural productivity, and reducing erosion. This section outlines a suite of SLM practices tailored to the unique biophysical and socio-economic conditions of the watershed, supported by evidence from similar contexts in Ethiopia and globally.
Agroforestry combines trees and shrubs with agricultural crops and/or livestock, creating multifunctional landscapes that stabilize soils, improve water infiltration, and provide additional income sources (Gebremedhin et al., 2023). Studies in the Ethiopian highlands have demonstrated that agroforestry systems reduce soil loss by up to 30% compared to monocropping systems (Tadesse and Negash, 2023). In the Beshilo watershed, integrating indigenous tree species, such as Cordia africana and Faidherbia albida, with staple crops like maize and sorghum could enhance soil structure while offering economic benefits to farmers.
Terracing is an effective practice for steep slopes, reducing runoff velocity and providing an opportunity for sediment deposition (Adimassu and Tamene, 2023). Contour farming, wherein crops are planted along natural elevation contours, complements terracing by further minimizing surface runoff. In a study by Hurni (1988), terracing reduced soil erosion by 40% in highland regions of Ethiopia. Implementing bench terraces along the steep slopes of the Beshilo watershed, coupled with proper maintenance to prevent terrace degradation, is critical to achieving long-term benefits.
Physical SWC structures, such as check dams, stone bunds, and gabions, are essential in mitigating gully erosion, a severe issue in the Beshilo watershed. Research by Adimassu and Assefa Abera (2017) revealed that stone bunds reduced soil loss by up to 68% on degraded lands in Ethiopia. These measures can be implemented in active erosion hotspots identified through RUSLE modeling, ensuring site-specific interventions to stabilize gullies and rehabilitate degraded lands.
Vegetative buffer strips composed of grasses, such as Vetiveria zizanioides (vetiver grass) and local shrubs, can trap sediments, reduce runoff, and enhance soil fertility. Reforestation with native species can further strengthen soil conservation efforts. Studies in the Ethiopian highlands show that vegetative strips reduce sediment transport by over 50% in degraded watersheds (Gessesse et al., 2015). For the Beshilo watershed, prioritizing degraded riparian zones for reforestation could restore ecological functions while enhancing biodiversity.
Diversifying cropping systems through rotation and intercropping enhances soil fertility and reduces erosion. Leguminous crops, such as beans and peas, enrich soils with nitrogen, improving overall productivity. Research by Teshome et al. (2022), found that crop rotation reduced soil loss by 25% and increased yields by 15% in Ethiopian highland farming systems. Promoting rotational practices, combined with erosion-resistant crops in the Beshilo watershed, can contribute to sustainable land use.
Community engagement is vital for the success of SLM practices. Participatory watershed management approaches, as implemented in the Tigray region, demonstrate the importance of local involvement in designing and maintaining conservation structures (Tesfaye et al., 2022). For the Beshilo watershed, organizing community-led committees to monitor and maintain SWC structures, combined with education and training programs, can foster long-term ownership and sustainability.
The success of SLM practices requires enabling policies that provide financial incentives, technical support, and institutional coordination. Integrating SLM into regional development plans, as outlined in Ethiopia’s Sustainable Land Management Program (SLMP), can address barriers to implementation. Subsidies for adopting SWC structures, combined with access to credit and market opportunities, are critical for scaling up SLM interventions in the Beshilo watershed.
Understanding the uncertainties associated with soil erosion modeling is crucial, particularly given the sensitivity of the Revised Universal Soil Loss Equation (RUSLE) to its input parameters rainfall erosivity (R), soil erodibility (K), topographic factors (LS), cover management (C), and conservation practices (P). While a formal sensitivity analysis was not conducted in this study, alternative approaches were employed to assess the credibility of the model outputs and minimize potential uncertainties. To ensure contextual validation, the estimated soil erosion rates for the Beshilo Watershed were compared with findings from existing literature in Ethiopia and other comparable regions. The mean annual soil loss obtained in this study aligns closely with previous assessments in the neighboring Blue Nile Basin (Haregeweyn et al., 2017; Tadesse and Negash, 2023) and the upper Awash Basin (Meshesha et al., 2018), reinforcing the reliability of the model estimates. This comparative analysis serves as an indirect validation method, increasing confidence in the results despite the absence of direct sediment yield calibration.
Further validation was achieved through extensive field observations and ground-truthing. Erosion hotspots identified by the RUSLE model were cross-verified with on-site assessments, revealing strong spatial agreement with observable erosion features such as active gullies, rills, and severely degraded landscapes. This consistency between modeled outputs and real-world conditions underscores the model’s effectiveness in identifying high-risk erosion zones. Recognizing the importance of formal sensitivity analyses and direct sediment yield calibration, we acknowledge these as valuable areas for future research. Incorporating such approaches could further enhance the precision and applicability of soil erosion assessments in Ethiopia’s diverse agro-ecological landscapes. This study, however, provides a strong foundation for informed conservation planning by integrating robust geospatial techniques with field-based validation. By transparently addressing uncertainties and model limitations, this research offers meaningful insights for policymakers, land managers, and researchers, supporting the development of targeted and sustainable soil conservation strategies.
This study acknowledges several limitations that must be considered when interpreting the findings. Firstly, due to the large scale of the Beshilo watershed, a detailed field investigation of critical soil health indicators such as soil organic matter (SOM), soil texture, permeability, and structure was not feasible. Instead, these parameters were estimated using the FAO World Soil Database, which, while widely recognized, lacks the specificity and localized accuracy of field-based data collection. As a result, the derived soil-related data may not fully capture the spatial and temporal variability of soil conditions within the watershed. Secondly, the assessment of land degradation extent in the Beshilo watershed was constrained by the absence of productivity data at the watershed scale. Productivity data, such as crop yields and biomass outputs, are essential for quantifying land degradation impacts on agricultural systems. The lack of such data limited the ability to establish a direct correlation between land degradation and productivity losses. This challenge is particularly significant in regions like the Ethiopian highlands, where land productivity is a key indicator of degradation severity.
Furthermore, the integration of geospatial techniques with field surveys, while effective for large-scale assessments, comes with inherent limitations. Geospatial analyses, including remote sensing and digital elevation modeling, are subject to data resolution and processing constraints, which can affect the accuracy of erosion hotspot identification and land degradation mapping. Field surveys, though valuable for ground-truthing, were limited to specific accessible areas within the watershed, which may have introduced spatial biases in the interpretation of results. Additionally, the temporal dynamics of soil erosion and land degradation could not be comprehensively assessed due to the lack of long-term observational data. Soil erosion is influenced by seasonal variations in rainfall, land use changes, and anthropogenic activities, which were not fully captured within the study period. This limitation underscores the need for sustained monitoring efforts to analyze trends and patterns over extended timeframes. Lastly, while this study provides critical insights into the spatial extent of soil erosion and land degradation in the Beshilo watershed, it does not account for socio-economic factors such as land tenure systems, community practices, and resource management challenges. These factors play a vital role in influencing land management decisions and erosion control measures, and their omission represents an area for further research. Despite these limitations, the study offers a valuable foundation for understanding soil erosion hotspots and land degradation dynamics in the Beshilo watershed. It highlights the importance of integrating geospatial techniques with field-based validation to inform sustainable land management practices and guide targeted interventions in erosion-prone areas.
This study provides a comprehensive assessment of land degradation and soil erosion hotspots in the Beshilo watershed, Northeastern Ethiopia, by integrating geospatial analysis and field survey techniques. The findings underscore the severity of soil erosion in the region, with RUSLE-based soil loss values ranging up to an alarming 1,288 t/ha/year, and a mean annual soil loss of 68.3 t/ha/year—far exceeding the tolerable limits of 12–18 t/ha/year in Ethiopia and the global standard of 40 t/ha/year. The classification of erosion severity indicates that 38.3% of the watershed is under severe to very severe risk, revealing the critical need for targeted interventions. The study identifies a confluence of factors driving these erosion rates, including steep terrain, unsustainable agricultural practices, limited vegetation cover, and poor soil health. Land use and land cover analysis revealed that 73.4% of the watershed is dominated by cultivated land, with only 7% covered by forest, while NDVI values as low as −0.24 further reflect the extent of vegetation loss. Soil quality indicators, including low soil organic matter (SOM), poor soil structure, and high sand content (78% sandy loam), coupled with documented instances of landslides and gully formations, further corroborate the extent of land degradation.
To mitigate these challenges, the study emphasizes the urgent need for the adoption of Sustainable Land Management (SLM) practices tailored to the local context. Proposed measures include terracing, agroforestry, vegetative buffer strips, and integrated soil and water conservation techniques. Implementing these strategies will require multi-stakeholder collaboration, capacity building, and policy support to ensure sustainable land use while balancing ecological integrity and agricultural productivity. This assessment provides critical data and actionable insights for stakeholders, enabling evidence-based decision-making and the design of targeted interventions. The study serves as a vital contribution toward addressing the pressing issue of land degradation in the Beshilo watershed and offers a model for similar contexts in Ethiopia and beyond. Immediate action is imperative to reverse the current trends, protect livelihoods, and ensure the sustainability of this fragile landscape. To address these issues, the following Sustainable Land Management (SLM) practices are recommended.
To address these issues, the following Sustainable Land Management (SLM) practices are recommended.
➢ Targeted Implementation of SLM Practices.
• Focus on priority areas identified as severe erosion hotspots for the implementation of terracing, agroforestry, and vegetative buffer strips.
• Encourage the use of native species in agroforestry systems to enhance soil stability and biodiversity.
➢ Community-Based Watershed Management
• Establish participatory watershed management committees to ensure local engagement and ownership of SLM interventions.
• Provide training and capacity-building programs to equip communities with knowledge of sustainable practices and maintenance of conservation structures.
➢ Policy and Institutional Support.
• Integrate SLM into regional and national development plans, ensuring alignment with Ethiopia’s Sustainable Land Management Program (SLMP).
• Develop policies that provide financial incentives, such as subsidies or access to credit, to encourage the adoption of conservation practices.
➢ Monitoring and Evaluation Framework
• Establish a robust monitoring system using geospatial tools to track the effectiveness of implemented SLM practices over time.
• Incorporate soil health indicators and erosion metrics as key components of monitoring to assess progress and refine strategies.
➢ Integration of Research and Practice
• Promote collaborative research initiatives to refine RUSLE parameters and improve the accuracy of erosion predictions.
• Encourage knowledge sharing between researchers, policymakers, and practitioners to bridge the gap between science and field implementation.
➢ Reforestation and Vegetation Restoration.
• Prioritize degraded areas for reforestation using native tree species, particularly in riparian zones and steep slopes.
• Combine reforestation efforts with livelihood diversification strategies to ensure socio-economic benefits for local communities.
By implementing these recommendations, the Beshilo watershed can achieve a sustainable balance between environmental conservation and socio-economic development, serving as a model for addressing land degradation challenges across Ethiopia.
The original contributions presented in the study are included in the article/supplementary material, further inquiries can be directed to the corresponding authors.
MW: Methodology, Validation, Writing–review and editing. DW: Conceptualization, Visualization, Writing–original draft, Writing–review and editing.
The author(s) declare that no financial support was received for the research, authorship, and/or publication of this article.
We, the authors of this manuscript titled “Assessing Soil Erosion Hotspots and Land Degradation Extent in Beshilo Watershed, Northeastern Ethiopia: Integrating geospatial and field survey techniques, for Sustainable Land Management”, extend our deepest gratitude to all who contributed to the successful completion of this work. We sincerely appreciate the invaluable efforts and assistance of Mr. Abdi Ame, Mr. Bie Gatwech, Mss. Ruth Solomon, Mr. Siraj Abdurahman, Mr. Wendimu Tassew, and Mss Zinash Gezmu during the data collection phase of our fieldwork.
The authors declare that the research was conducted in the absence of any commercial or financial relationships that could be construed as a potential conflict of interest.
The author(s) declare that no Generative AI was used in the creation of this manuscript.
All claims expressed in this article are solely those of the authors and do not necessarily represent those of their affiliated organizations, or those of the publisher, the editors and the reviewers. Any product that may be evaluated in this article, or claim that may be made by its manufacturer, is not guaranteed or endorsed by the publisher.
Abate, T. (2016). Indigenous ecological knowledge and pastoralist perception on rangeland management and degradation in Guji Zone of south Ethiopia. Consilience (15), 192–218.
Adimassu, Z., and Assefa Abera, B. (2017). Synthesis of land management effects on crop productivity and soil properties in Ethiopia. Ethiop. J. Nat. Resour. 17 (2), 1563–3705.
Adimassu, Z., and Tamene, L. (2023). Benefits of selected land management practices on ecosystem services: case studies in four watersheds of Ethiopia. Int. J. Environ. Sci. Technol. 20 (2), 1919–1936. doi:10.1007/s13762-022-04136-5
Ahmad, I., Dar, M. A., Andualem, T. G., and Teka, A. H. (2020). GIS-based multi-criteria evaluation of groundwater potential of the Beshilo River basin, Ethiopia. J. Afr. earth Sci. 164, 103747. doi:10.1016/j.jafrearsci.2019.103747
Akça, E., Büyük, G., İnan, M., and Kırpık, M. (2022). “Sustainable management of land degradation through legume-based cropping system,” in Advances in legumes for sustainable intensification (Academic Press), 267–280.
Ayalew, G., and Selassie, Y. G. (2015). Soil loss estimation for soil conservation planning using geographic information system in Guang watershed, Blue Nile basin. J. Environ. Earth Sci. 5 (1), 126–134.
Bewket, W., and Sterk, G. (2003). Assessment of soil erosion in cultivated fields using a survey methodology for rills in the Chemoga watershed, Ethiopia. Agric. Ecosyst. and Environ. 97 (1-3), 81–93. doi:10.1016/s0167-8809(03)00127-0
Bewket, W., and Teferi, E. (2009). Assessment of soil erosion hazard and prioritization for treatment at the watershed level: case study in the Chemoga watershed, Blue Nile basin, Ethiopia. Land Degrad. and Dev. 20 (6), 609–622. doi:10.1002/ldr.944
Demissie, S., Meshesha, D. T., Adgo, E., Haregeweyn, N., Tsunekawa, A., Ayana, M., et al. (2022). Effects of soil bund spacing on runoff, soil loss, and soil water content in the Lake Tana Basin of Ethiopia. Agric. Water Manag. 274, 107926. doi:10.1016/j.agwat.2022.107926
Desale, T., Metaferia, G., Shifaw, E., Abebe, S., Molla, W., and Asmare, M. (2023). Identification and prioritization of sub-watersheds to soil erosion and sediment yield susceptibility using RUSLE, remote sensing, and GIS (case study: abbay—Awash Basin in Wollo Area, Ethiopia). Water Conservation Sci. Eng. 8 (1), 1. doi:10.1007/s41101-023-00179-y
Desalegn, M. Y., Miheretu, B. A., and Gobezie, T. (2023). Impact of land use/land cover changes on soil erosion risk in upper Mile River sub-watershed, North Eastern highlands of Ethiopia. Geol. Ecol. Landscapes, 1–13. doi:10.1080/24749508.2023.2206063
Ejegu, M. A., and Yegizaw, E. S. (2021). Modeling soil erosion susceptibility and LULC dynamics for land degradation management using geoinformation technology in Debre Tabor district, Northwestern highlands of Ethiopia. J. Degr. Min. Land. Manage. 8, 2623–2633. doi:10.15243/jdmlm.2021.082.2623
Fao, F. (2015). Agriculture organization: status of the world’s soil resources (SWSR)–Main report. Food Agric. Organ. U. N. Intergov. Tech. Panel Soils, Rome, Italy.
Fenta, A. A., Tsunekawa, A., Haregeweyn, N., Tsubo, M., Yasuda, H., Kawai, T., et al. (2021). Agroecology-based soil erosion assessment for better conservation planning in Ethiopian river basins. Environ. Res. 195, 110786. doi:10.1016/j.envres.2021.110786
Fentaw, A. E., and Abegaz, A. (2024). Soil erosion assessment and identification of erosion hotspot areas in the upper Tekeze Basin, Northern Ethiopia. Heliyon 10, e32880. doi:10.1016/j.heliyon.2024.e32880
Food and Agriculture Organization of the United Nations (2018). The state of the world’s land and water resources for food and agriculture: managing systems at risk. Rome, Italy: FAO. Available online at: https://www.fao.org.
Food and Agriculture Organization of the United Nations (FAO) (2019). The state of the world's biodiversity for food and agriculture. Rome, Italy: FAO.
Gashaw, T., Tulu, T., and Argaw, M. (2018). Erosion risk assessment for prioritization of conservation measures in Geleda watershed, Blue Nile basin, Ethiopia. Environ. Syst. Res. 6 (1), 1–14. doi:10.1186/s40068-016-0078-x
Gashaw, T., Tulu, T., Argaw, M., and Worqlul, A. W. (2017). Evaluation and prediction of land use/land cover changes in the Andassa watershed, Blue Nile Basin, Ethiopia. Environ. Syst. Res. 6, 17–15. doi:10.1186/s40068-017-0094-5
Gebremedhin, B., Tadesse, T., Hadera, A., Tesfay, G., and Rannestad, M. M. (2023). Risk preferences, adoption and welfare impacts of multiple agroforestry practices. For. Policy Econ. 156, 103069. doi:10.1016/j.forpol.2023.103069
Gessesse, G. D., Mansberger, R., and Klik, A. (2015). Assessment of rill erosion development during erosive storms at Angereb watershed, Lake Tana sub-basin in Ethiopia. J. Mt. Sci. 12, 49–59. doi:10.1007/s11629-014-3151-9
Gupta, G. S. (2019). Land degradation and challenges of food security. Rev. Eur. Stud. 11, 63. doi:10.5539/res.v11n1p63
Hagos, Y. G., Andualem, T. G., Sebhat, M. Y., Bedaso, Z. K., Teshome, F. T., Bayabil, H. K., et al. (2023). Soil erosion estimation and erosion risk area prioritization using GIS-based RUSLE model and identification of conservation strategies in Jejebe watershed, Southwestern Ethiopia. Environ. Monit. Assess. 195 (12), 1501. doi:10.1007/s10661-023-12136-2
Haregeweyn, N., Tsunekawa, A., Nyssen, J., Poesen, J., Tsubo, M., Tsegaye Meshesha, D., et al. (2015). Soil erosion and conservation in Ethiopia: a review. Prog. Phys. Geogr. 39 (6), 750–774. doi:10.1177/0309133315598725
Haregeweyn, N., Tsunekawa, A., Poesen, J., Tsubo, M., Meshesha, D. T., Fenta, A. A., et al. (2017). Comprehensive assessment of soil erosion risk for better land use planning in river basins: case study of the Upper Blue Nile River. Sci. Total Environ. 574, 95–108. doi:10.1016/j.scitotenv.2016.09.019
Hossain, A., Krupnik, T. J., Timsina, J., Mahboob, M. G., Chaki, A. K., Farooq, M., et al. (2020). “Agricultural land degradation: processes and problems undermining future food security,” in Environment, climate, plant and vegetation growth (Cham: Springer International Publishing), 17–61.
Hurni, H. (1985). “Erosion-productivity-conservation systems in Ethiopia,” in IV international conference on soil conservation. Soil conservation and productivity: proceedings ( Bern, Switzerland: Maracay, Venezuela).
Hurni, H. (1988). Degradation and conservation of the resources in the Ethiopian highlands. Mt. Res. Dev. 8, 123–130. doi:10.2307/3673438
Hurni, H., Tato, K., and Zeleke, G. (2005). The implications of changes in population, land use, and land management for surface runoff in the upper Nile basin area of Ethiopia. Mt. Res. Dev. 25 (2), 147–154. doi:10.1659/0276-4741(2005)025[0147:tiocip]2.0.co;2
Hurni, K., Zeleke, G., Kassie, M., Tegegne, B., Kassawmar, T., Teferi, E., et al. (2015). Economics of Land Degradation (ELD) Ethiopia Case Study: soil degradation and sustainable land management in the rainfed agricultural areas of Ethiopia: an assessment of the economic implications.
Kebede, B., Tsunekawa, A., Haregeweyn, N., Adgo, E., Ebabu, K., Meshesha, D. T., et al. (2021). Determining C-and P-factors of RUSLE for different land uses and management practices across agro-ecologies: case studies from the Upper Blue Nile basin, Ethiopia. Phys. Geogr. 42 (2), 160–182. doi:10.1080/02723646.2020.1762831
Lal, R. (2004). Soil carbon sequestration impacts on global climate change and food security. science 304 (5677), 1623–1627. doi:10.1126/science.1097396
Mekonnen, A. A., Raghuvanshi, T. K., Suryabhagavan, K. V., and Kassawmar, T. (2022). GIS-based landslide susceptibility zonation and risk assessment in complex landscape: a case of Beshilo watershed, northern Ethiopia. Environ. Challenges 8, 100586. doi:10.1016/j.envc.2022.100586
Mekonnen, E., Kebede, A., Asfaw, S., and Feyissa, S. (2023). Optimizing soil erosion estimates of RUSLE model by analyzing land use/cover dynamics in upper Awash River Basin, Central Ethiopia. Geomatics, Nat. Hazards Risk 14 (1), 2257363. doi:10.1080/19475705.2023.2257363
Meshesha, D. T., Fisse, G., and Ayehu, R. (2018). Effects of soil and water conservation practices on selected bio-physical, and livelihood attributes and farmers perception at Akusti Micro Watershed, Northwest Ethiopia. J. Agric. Environ. Sci. 3 (2).
Mohammed, J. A., and Yimam, Z. A. (2022). Spatiotemporal variability and trend analysis of rainfall in Beshilo sub-basin, upper Blue nile (abbay) basin of Ethiopia. Arabian J. Geosciences 15 (16), 1387. doi:10.1007/s12517-022-10666-6
Moore, I. D., and Wilson, J. P. (1992). Length-slope factors for the revised universal soil loss equation: simplified method of estimation. J. soil water conservation 47 (5), 423–428.
Nigussie, Z., Tsunekawa, A., Haregeweyn, N., Adgo, E., Nohmi, M., Tsubo, M., et al. (2017). Farmers' perception about soil erosion in Ethiopia. Land Degrad. and Dev. 28 (2), 401–411. doi:10.1002/ldr.2647
Oliveira, P. T. S., Wendland, E., and Nearing, M. A. (2013). Rainfall erosivity in Brazil: a review. Catena 100, 139–147. doi:10.1016/j.catena.2012.08.006
Pal, S. C., and Chakrabortty, R. (2019a). Modeling of water induced surface soil erosion and the potential risk zone prediction in a sub-tropical watershed of Eastern India. Model. Earth Syst. Environ. 5 (2), 369–393. doi:10.1007/s40808-018-0540-z
Pimentel, D. (2006). Soil erosion: a food and environmental threat. Environ. Dev. Sustain. 8, 119–137. doi:10.1007/s10668-005-1262-8
Pimentel, D., and Burgess, M. (2013). Soil erosion threatens food production. Agriculture 3 (3), 443–463. doi:10.3390/agriculture3030443
Pimentel, D., and Kounang, N. (1998). Ecology of soil erosion in ecosystems. Ecosystems 1, 416–426. doi:10.1007/s100219900035
Renard, K. G., McCool, D. K., Cooley, K. R., Foster, G. R., Istok, J. D., and Mutchler, C. K. (1997). “Rainfall-runoff erosivity factor (R),” in Predicting soil erosion by water–A guide to conservation planning with the revised universal soil loss equation (RUSLE).
Tadesse, E., and Negash, M. (2023). Impacts of indigenous agroforestry practices and elevation gradient on ecosystem carbon stocks in smallholdings’ farming system in South-Central Ethiopia. Agrofor. Syst. 97 (1), 13–30. doi:10.1007/s10457-022-00781-4
Tamene, L., and Vlek, P. L. (2008). Soil erosion studies in northern Ethiopia. Land use soil Resour., 73–100. doi:10.1007/978-1-4020-6778-5_5
Taye, G., Poesen, J., Wesemael, B. V., Vanmaercke, M., Teka, D., Deckers, J., et al. (2013). Effects of land use, slope gradient, and soil and water conservation structures on runoff and soil loss in semi-arid Northern Ethiopia. Phys. Geogr. 34 (3), 236–259. doi:10.1080/02723646.2013.832098
Taye, G., Vanmaercke, M., Poesen, J., Van Wesemael, B., Tesfaye, S., Teka, D., et al. (2018). Determining RUSLE P-and C-factors for stone bunds and trenches in rangeland and cropland, North Ethiopia. Land Degrad. and Dev. 29 (3), 812–824. doi:10.1002/ldr.2814
Tebebu, T. Y., Steenhuis, T. S., Dagnew, D. C., Guzman, C. D., Bayabil, H. K., Zegeye, A. D., et al. (2015). Improving efficacy of landscape interventions in the (sub) humid Ethiopian highlands by improved understanding of runoff processes. Front. Earth Sci. 3, 49. doi:10.3389/feart.2015.00049
Teku, D., Kesete, N., and Abebe, A. (2024). GIS based annual soil loss estimation with revised universal soil loss equation (RUSLE) in the upper Meki sub-catchment, rift valley sub-basin, Ethiopia. Cogent Food and Agric. 10 (1), 2311802. doi:10.1080/23311932.2024.2311802
Tesfaye, B., Lengoiboni, M., Zevenbergen, J., and Simane, B. (2022). Land preservation uptakes in the escarpments of north-eastern Ethiopia: drivers, sustainability, and constraints. Land 11 (5), 676. doi:10.3390/land11050676
Teshome, D. S., Moisa, M. B., Gemeda, D. O., and You, S. (2022). Effect of land use-land cover change on soil erosion and sediment yield in Muger Sub-Basin, Upper Blue Nile Basin, Ethiopia. Land 11 (12), 2173. doi:10.3390/land11122173
Teshome, M., Mohammed, M., and Asfaw, Z. (2020). Pattern of functional diversity along the elevation gradient in the dry evergreen Afromontane forest of Hararghe Highland, Southeast Ethiopia. Biosyst. Divers. 28 (3), 257–264. doi:10.15421/012034
Trivedi, P., Singh, B. P., and Singh, B. K. (2018). “Soil carbon: introduction, importance, status, threat, and mitigation,” in Soil carbon storage (Academic Press), 1–28.
Tucker, C. J., and Sellers, P. J. (1986). Satellite remote sensing of primary production. Int. J. remote Sens. 7 (11), 1395–1416. doi:10.1080/01431168608948944
Tunçay, T., and Başkan, O. (2022). “Assessment of land degradation factors,” in Vegetation dynamics, changing ecosystems and human responsibility (IntechOpen).
United Nations Environment Programme (2016). Global environmental outlook: GEO-6 regional assessment for Africa. Nairobi, Kenya: UNEP. Available online at: https://wedocs.unep.org.
Wischmeier, W. H., and Smith, D. D. (1978). Predicting rainfall erosion losses: a guide to conservation planning (No. 537). Washington, DC: United States Department of Agriculture, Science and Education Administration.
Wodaje, S. T. (2016). Land degradation vulnerability assessment using GIS and remote sensing in Beshilo River Basin, Ethiopia. Master's thesis, Addis Ababa, Ethiopia: NTNU.
Keywords: soil erosion hotspots, land degradation assessment, beshilo watershed, geospatial analysis, sustainable land management
Citation: Workie MD and Teku D (2025) Assessing soil erosion hotspots and land degradation extent in Beshilo Watershed, Northeastern Ethiopia: integrating geospatial and field survey techniques, for sustainable land management. Front. Environ. Sci. 13:1548881. doi: 10.3389/fenvs.2025.1548881
Received: 20 December 2024; Accepted: 05 March 2025;
Published: 24 March 2025.
Edited by:
Zhiguang Liu, Shandong Agricultural University, ChinaReviewed by:
Berhanu Sinshaw, University of California, Merced, CA, United StatesCopyright © 2025 Workie and Teku. This is an open-access article distributed under the terms of the Creative Commons Attribution License (CC BY). The use, distribution or reproduction in other forums is permitted, provided the original author(s) and the copyright owner(s) are credited and that the original publication in this journal is cited, in accordance with accepted academic practice. No use, distribution or reproduction is permitted which does not comply with these terms.
*Correspondence: Melak Desta Workie, bWVsYWtkZXN0YTI4QGdtYWlsLmNvbQ==; Degfie Teku, ZGVnZmllMjAyM0BnbWFpbC5jb20=
Disclaimer: All claims expressed in this article are solely those of the authors and do not necessarily represent those of their affiliated organizations, or those of the publisher, the editors and the reviewers. Any product that may be evaluated in this article or claim that may be made by its manufacturer is not guaranteed or endorsed by the publisher.
Research integrity at Frontiers
Learn more about the work of our research integrity team to safeguard the quality of each article we publish.