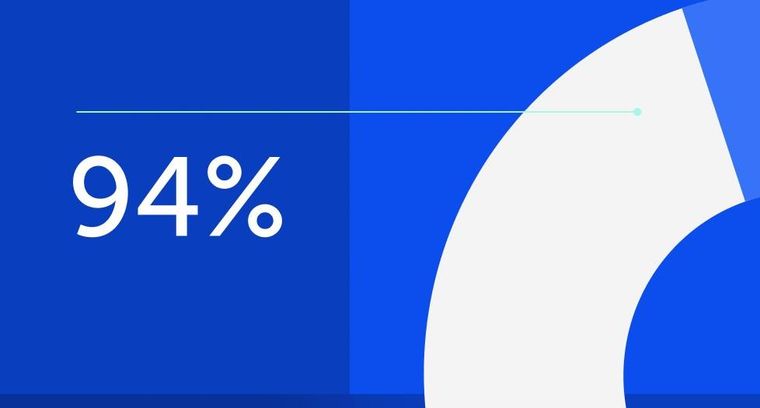
94% of researchers rate our articles as excellent or good
Learn more about the work of our research integrity team to safeguard the quality of each article we publish.
Find out more
ORIGINAL RESEARCH article
Front. Environ. Sci., 07 April 2025
Sec. Toxicology, Pollution and the Environment
Volume 13 - 2025 | https://doi.org/10.3389/fenvs.2025.1535294
This article is part of the Research TopicAgro-Environmental Nutrient Management Rooted in Nature-Based SolutionsView all articles
To better explore the resistance of garden plants to heavy metals and to achieve the ecological remediation of garden plants in heavy metal contaminated soils, the study analyzed their resistance under heavy metals and combined with ameliorated soils for experimental design. The soil and plant analyzer development values of rhododendrons and heather were reduced by more than 15% when treated with heavy metals, and the malondialdehyde of gardenia was increased by 6.42% on Zinc (Zn). The gardenia plant had significant Zn and Cadmium (Cd) accumulation ability, the enrichment coefficient of rhododendron root system under Copper (Cu) was 6.38, and the transfer coefficient of Cu metal of weigela was about 2.0. Compared with the control, the difference of proline content of rhododendron and gardenia under the treatment of the improved soil was more than 9 times. Meanwhile, the reduction trend of Pb, Zn, and Cu in the cultivated soil of cuckoo with diethylenetriaminepentaacetic acid applied was 28% higher than that of the blank control, which indicated that gardenia had a better effect of diethylenetriaminepentaacetic acid-Cu applied. The research results show that the physiological response and accumulation of garden plants to heavy metals demonstrate their tolerance to heavy metals, and the addition of stabilizers in heavy metal contaminated soil is feasible. This research content can promote the excavation of garden plants with high tolerance potential, and has reference technical value for the restoration of ecological landscapes in mining areas.
Mineral resources are an important material basis for social development, but their irrational exploitation has led to the increasingly serious problem of heavy metal pollution (Yaashikaa et al., 2022). According to the National Soil Pollution Survey Bulletin, China’s soil pollution exceeds the standard by more than 10 per cent, and the main pollutants are heavy metals such as lead, copper, chromium, zinc and nickel (Nie et al., 2023; Chaturvedi et al., 2018). These harmful substances migrate through water washing, rainfall and sedimentation and eventually accumulate in the soil, causing serious impacts on plant growth and the ecological environment. Although small amounts of heavy metals are beneficial to plant growth, excessive accumulation can inhibit or poison plants (Wang et al., 2018; Hatamian et al., 2019). Some plants have developed heavy metal tolerance mechanisms through evolution, such as extraction, volatilisation and filtration, and other remediation processes, among which the plant extraction technology shows great promise in heavy metal pollution management (Souri et al., 2018). For example, Bertini et al. (Bertini et al., 2019) found that plants with strong antioxidant capacity can effectively reduce heavy metal concentrations, while Jin-Hua et al. (Jin-Hua et al., 2022) showed that bryophytes have significant remediation effects on iron, manganese, and other heavy metal contamination in the Changgou Manganese Mine in Zunyi, Guizhou. Bai et al. conducted a performance evaluation of Pinus massoniana with strong manganese tolerance. The results showed that the increased activity of antioxidant enzymes in Pinus sylvestris was effective in remediating Mn-contaminated soils and that it could alter ambient gas parameters to maintain photosynthesis (Bai et al., 2021). The increase in temperature, exposure time, and initial metal concentration can affect the metal absorption capacity of floating plants such as water lilies (Pang et al., 2023). However, most of the current studies focus on laboratory simulation, and the remediation techniques in practical applications still need to be explored in depth. As an important medium for heavy metal pollution, mine soil not only affects local ecology, but also inhibits photosynthesis, antioxidant capacity and enzyme activity of garden plants. Therefore, accelerating vegetation restoration in mining areas has become a hot issue in environmental management.
Garden plants are widely used for ecological improvement and soil restoration in mining areas because of their adaptability, fast growth rate and remarkable absorption effect. The resistance of plants to heavy metals is mainly realised through two forms of exclusion and enrichment, but the tolerance mechanism varies according to plant species (Pandey et al., 2019; Meena et al., 2021). When heavy metals exceed the tolerance limit of plants, they will have toxic effects on plants, therefore, combining phytoremediation technology with other pollution control means (such as chelating agents, voltage, microorganisms and amendments) can effectively make up for the limitations of a single remediation technology (Pachaiappan et al., 2022; Abubakar et al., 2024). Based on the serious status of soil heavy metal pollution and the urgency of ecological remediation, the study aimed to explore the physiological response mechanism and tolerance characteristics of garden plants to heavy metal pollution, and to analyse the remediation mechanism through hydroponic and soil cultivation experiments, combined with diethylenetriacetic acid (DTPA) amendment. DTPA is a multi-purpose compound with strong chelating properties and is widely used in various industrial, medical and environmental fields. In environmental remediation, DTPA can form stable complexes with metal ions to remove heavy metals from contaminated soil and water, thereby reducing metal toxicity and improving soil quality. The study screened the garden plant materials suitable for planting, combined with the amendment to achieve the stabilisation and remediation of soil heavy metals, explored the growth condition, heavy metal fixation effect and uptake efficiency of plants under the action of stabiliser, and analysed the tolerance mechanism of plants at the molecular level. The research aims to explore the tolerance mechanism of garden plants to heavy metal pollution, evaluate the role of amendments in soil remediation, propose comprehensive remediation strategies, provide theoretical basis for screening and cultivating garden plants with greater remediation potential, and provide practical reference for ecological restoration in mining areas.
The study selected four common garden tree species as experimental trees in a particular seedling breeding site and conducted the experiments in an intelligent greenhouse at a university. This study on plants is complied with relevant institutional, national, and international guidelines and legislation. The field studies on plants were approved by Henan Polytechnic. In the experiments, the seedlings of four garden trees were kept at the same height (basically located around 20 cm). The cultivation process started by contextualizing the roots of the seedlings in distilled and condensed pure water to remove impurities such as soil, and then placing them in light-proof buckets with 1.8 L of nutrient solution. Three seedlings were placed in each bucket, the nutrient solution was Hoagland’s (the main components were 1 mmol/L ammonium phosphate solution ionised (NH4H2PO4), 5 mmol/L calcium nitrate 4 hydrate (Ca(NO3)2.4H2O), and 5 mmol/L potassium nitrate (KNO3). 2 mmol/L magnesium sulphate heptahydrate (MgSO4.7H2O) was diluted after 10 times configuration and the pre-culture time was 10 days. Table 1 shows the information of the garden plants selected for the experiment. The environmental conditions during the experimental period were all carried out in greenhouse, and the soil samples were sterilized for 30 min at 105°C.
After pre-culturing the seedlings, the garden plants were treated with heavy metals. The heavy metal sources chosen were from Pb nitrate Pb(No3)2, Zn sulphate heptahydrate ZnSO4-7(H2O), Cu sulphate pentahydrate CuSO-5H2O, Cd chloride CdCl2-2.5H2O, Cr trioxide Cr2O3, and Ni sulphate NiSO4, with high purity and from which Pb2+, Zn2+, Cu2+, Cd2+, Cr3+, and Ni2+. The concentration ratios of heavy metals were set for different garden plants, and the concentrations of heavy metals Pb2+, Zn2+, Cu2+, Cd2+, Cr3+, and Ni2+ for the four plants were 100 mg/L-1 (Pb), 100 mg/L-1 (Zn), 15 mg/L-1 (Cu), 5 mg/L-1 (Cd), 5 mg/L-1 (Cr), and 5 mg/L-1 (Ni) (Peng et al., 2022; Abbas et al., 2021). During the experiment, the nutrient solution was renewed every 5 h and the gas flow in the greenhouse was kept normal, and the corresponding plant plants were obtained after 2 weeks.
The relative chlorophyll content of the plants during growth was determined with the aid of a soil and plant analyzer development (SPAD)-502 chlorophyll meter, i.e., the SPAD values were determined for each plant on selected leaves at three different locations. Measurements were taken at two-thirds of the leaf and readings were taken while the leaf was out of the body to reduce the instability of the data, with the average of three repeated readings being the final value. To ensure the authenticity of chlorophyll measurement values, visible near-infrared spectroscopy technology is applied to assist in detecting plant chlorophyll content. The spectra of leaves are collected through reflection sampling, and the spectral data are preprocessed using smoothing, differentiation, and wavelet transform methods. Then, a quantitative analysis model of leaf chlorophyll content and leaf absorption spectrum is established using the partial least squares method. After group verification, the prediction accuracy of the model meets the requirements of practical measurement applications. Malonicdialdehyde (MDA) is one of the most important products of membrane lipid peroxidation and its increased levels can cause damage to membranes and cells, resulting in impairment of cell structure and function. MDA indirectly reflects the severity of free radical attack on the body’s cells, which is used to determine the degree of ageing of plant organs (Rai et al., 2021; Hoang et al., 2021a). Thiobarbituric acid (TBA) can be used to determine the MDA content. The principle is that MDA and TBA condense to form a red product, which forms a peak aggregate with maximum absorption at 532 nm. Free proline (F-proline) content is a measure of plant growth and development and is primarily used to help the body break down proteins (Hoang et al., 2021b; Jin et al., 2022). This level can be determined by the acidic ninhydrin method, which involves the formation of a stable purple-blue product of F-proline and ninhydrin under acidic or heated conditions. The shade of this coloured product can be read colourimetrically under spectrophotometry, with darker shades indicating higher levels of F-proline and artificial permutation removing interfering impurities of amino acids (El Rasafi et al., 2021). The determination of heavy metal content in plants is carried out in a sterile environment. The plants are washed in deionized water and placed in a solution of 20 mM Ethylenediaminetetraacetic acid (EDTA) disodium salt to remove the metal ions, followed by a secondary cleaning with pure water. The cleaned plants are divided into three parts according to their organ parts, which are disinfected, dried, and ground. The heavy metal content is determined with the aid of an inductively coupled plasma emission spectrometer. The main measurement time for experimental samples is once every 2 days, and the analysis of plant organs mainly involves the three parts of roots, stems, and leaves. The repeated treatments for groups are three.
Test environment and materials: the test area was located in an intelligent greenhouse of an agricultural and forestry university, and the test soil came from a lead-zinc mine area 0–20 cm soil layer (pH = 4.0–6.5), and the soil was discarded using natural air drying method after removing rocks and biological residues, and the particles were screened with a hundred-mesh sieve. In the soil sample of this experiment, the copper content is 120–450 mg/kg and the zinc content is 800–1800 mg/kg. Soil pH was monitored regularly during the experiment and maintained in a stable range by adding buffers (e.g., calcium carbonate or sulphuric acid) to minimise the effect on the solubility and chemical form of copper and zinc. The moisture content of the soil was also maintained at 70% of the field water holding capacity) at a constant temperature of 25°C during the control experiments. It was ensured that soil treatments, watering frequency, and light conditions were consistent between the experimental and control groups, and the experimental data were regularly monitored and adjusted accordingly.
Modifier: the modifier selected for the study was zeolite, constructed from a technology limited company, in which modified zeolite had a pH value of 9.03, and phosphorus and potassium content reached 1.74 and 2.08, respectively.
Experimental design and treatment: the protocol design was carried out with indoor potting experiments, selecting the plant materials mentioned in the study, and the group with 3% zeolite in the injection mode was the experimental group, and the group without any modifier was the blank control group.
Specifically, the contaminated soil and modifier were mixed well and filled into pots to which urea and dipotassium phosphate were added as basal fertiliser. The weight of soil in each basin was the same, the water content was 70% of the field capacity, and the watering time during the experiment was set at every 2 days, with three replications for each treatment group. The whole experiment lasted for 2 months.
Determination of heavy metals: Traditional soil agrochemical analysis and DTPA extraction methods were used. DTPA, as an important amino chelator, can combine with metal ions to form chelates, which indirectly reflect the heavy metal content and pollution degree in soil. Ltd. produces ICP-5000 Inductively Coupled Plasma Emission Spectrometer for material analysis and testing. The setting of heavy metals is based on the national soil environment quality level 2 standard (GB15618-1995“Soil Environment Quality Standard”).
Excel 2007 was used to organize and analyze the data during the experimental process. SPSS 22.0 was used for data statistical analysis, significance difference analysis, and analysis of variance (ANOVA). Duncan’s new complex range (SSR) method was used for difference significance analysis (P < 0.05). The experimental data are presented in the form of mean
Equation 1 represents the formula for calculating the study sample mean
Equation 2 represents the expression for calculating the standard deviation
The equations for the enrichment factor
In Equation 3,
As an element necessary for photosynthesis in plants, chlorophyll plays an important role in energy conversion. The intensity of photosynthesis is related to the amount of chlorophyll content. The study measured the relative chlorophyll content of four plants during the experiment to better reflect the mechanism of the effect of medium and heavy metals on the chlorophyll content of plants. The results of the experiments are shown in Table 2.
The results in Table 2 show that there is some variation in the SPAD values exhibited by the state of the plants without adding any heavy metals. The values of chlorophyll content were as follows: Ea > Gj > Pf > Rr, with the values fluctuating between (44,120) and the maximum chlorophyll of 115.22 for the Ea plants, and the SPAD values of the garden plants were reduced to different degrees by the addition of different heavy metals. Specifically, the four plants treated with Pb metal showed a decrease in SPAD of 24.3%, 14.5%, 18.2% and 12.7%, respectively, compared to the control, while the four plants treated with Zn metal showed a decrease in SPAD of 27.1%, 1.5%, 24.3%, and 18.6%, respectively, compared to the control. The effects were similar to those of Cu. Ea showed the most significant SPAD values for the six metals, indicating that it was more susceptible to heavy metal contamination, and the most significant changes in chlorophyll content of the plant leaves. gj plants were more tolerant to heavy metals. In Table 2, the four plants except azalea, whose SPAD values with plants under different heavy metal interventions, all showed statistically significant differences (P < 0.05) with the blank group without adding anything. The statistical difference between gardenia plants under Pb and Cu interventions and the control group was very obvious (P < 0.001), and the data of SPAD change in weigelia plants under the heavy metal interferences of Zn and Cr were significant (P < 0.001) and the difference of their mean SPAD scores was 30 and above. The MDA content and proline of the plant leaves are subsequently measured and the results are shown in Table 3.
In Table 3, the MDA content in the leaves of plants treated with heavy metals increased to different degrees, and all of them had different degrees of peroxidation reaction. There was a statistically significant difference between them and the control group (P < 0.05). The data of azalea and gardenia under Pb and Cu heavy metals in particular changed significantly, and the statistically significant difference from the control group showed P < 0.001. The mean Rr decreased from 0.231 (µ/mol/g)−1 to 0.221 (µ/mol/g)−1 under heavy metal Zn, which was significant compared to the decrease under the influence of Pb and Cu metals. However, MDA of Rr plants increased by 3.95% in Cd group. The peroxide values of Gj and Ea plants increased by 6.42% and 1.72%, respectively under the effect of heavy metal Cr, while Pf plants increased by 54.16%. Statistically significant difference was observed between Pf plants under Cu and Cr metals and control group. The above results indicated that their cell damage under the corresponding metals was severe. And the differential effect between the control and heavy metal treatment groups indicated that the differential effect of their MDA values was not significant and the plants under Cd treatment were better tolerated and more resistant to the toxicity. Proline can achieve the inhibitory effect on plant growth through reactive oxygen signal. The proline index of the three plant tissues Gj, Ea, and Pf increased under the influence of heavy metal concentrations, indicating a certain resistance to heavy metals. The plant leaves of Ea were affected by the heavy metal Pb by well over 50% and there were significant data differences (P < 0.05) between their values and those of the control group. The accumulation of heavy metals was then compared for different parts of the organs of the garden plants and the results of the data are shown in Table 4.
In Table 4, the plants under the influence of different heavy metals all exhibit an accumulation of elements mainly in the roots, while the accumulation of elements in the stem and leaves is less. This may be due to the fact that heavy metals are repelled during uptake and transfer, thus preventing excessive accumulation of metals. The accumulation of metals in all parts of the plant was mainly Pb > Zn > Cu > Cd, with the accumulation of Pb in the root and stem parts of the Rr plant exceeding 300 mg/kg-1, and the accumulation of Zn and Cd in the Gj plant being significantly higher. The accumulation of metals in each plant part specifically was further explored and four distinct heavy metals, Pb, Zn, Cu, and Cd, were selected for experimental analysis, the results of which were shown in Figure 1.
Figure 1. Accumulation of heavy metals in different parts of plants. (a) Pb; (b) Zn; (c) Cu; (d) Cd.
Figure 1 shows the heavy metal enrichment factors (Biological Enrichment Factor, BCF) of four garden plants. The results in Figure 1 showed that heavy metals were mainly concentrated in the roots and stems of the plants, and their enrichment factors were above 2.0 in the roots. The accumulation effect of Pb in Ea, and the accumulation of Zn, Cu, and Cd in the roots, stems, and leaves of Gj were obvious. The results of the assessment of the heavy metal uptake and transport capacity of the garden plants are shown in Figure 2.
Figure 2. Heavy metal transport of different parts of plants. Note: “*” indicates significant differences between different treatment groups under the modifier (P < 0.05).
In Figure 2, Rr and Gj plants were significantly more capable of transposing Pb metals than other plants and that their values were significantly different from those of the blank control. The transfer coefficient of Rr reached 1.15 in Zn and Ea had the highest transfer coefficient in the case of Cu uptake and transfer, approximating 2.0. Overall, plants with transfer coefficients greater than 1 all showed a strong uptake and transfer capacity under the corresponding heavy metals. The Lead zinc mine soil used for experiments was improved by adding artificial permutation to investigate the mechanism of its joint action with the garden plants, and the experimental results were shown in Figure 3.
Figure 3. Ecological response mechanism of landscape plants under heavy metal improvement. (a) SPAD; (b) MAD; (c) Proline.
The results of Figure 3 showed that the chlorophyll content of the plants increased by more than 60% under the amended soil compared to the conventional group. And that there was a significant difference in the variation of the values, with Rhododendron plants showing less variation than the other plants. In terms of MDA values, the heavy metals were less damaging to the plants in the amended soil, with Rhododendron in particular tending to show no change in this indicator. The proline of the garden plants in Figure 3C increased to varying degrees, with Rhododendron and gardenia showing more than a 9-fold difference in value compared to the control group. These results indicated that the soil amendment effectively reduced the toxic effects of heavy metals on the plants. Further analysis of the accumulation of heavy metals in the roots of each garden plant was carried out and the results were shown in Table 5.
From Table 5, the accumulation of Pb in the garden plants under the amended soil was improved to varying degrees. The values for Rr, Gj, Ea, and Pf plants differed significantly (P < 0.05) from those of the control group by 41.26%, 16.28%, 21.76%, and 32.64%, with Rr showing a decrease in the concentrations of Pb, Cu, Zn, and Cd in the amended soil. The effect of heavy metal accumulation by Gj and Ea in the presence of Cu was highly significant (P < 0.001). Gj showed higher tolerance to Pb, with improved and control mean values of 267.14 and 192.33, respectively, and showed a higher enrichment effect on Zn. The results of the analysis of the heavy metal situation under the amended soil combined with garden plants are shown in Figure 4.
DTPA is an important amino chelator that binds to metal ions to form chelates, which can give a side view of the heavy metal content of the soil and the degree of contamination (Li et al., 2023). Figure 4 shows that the amendments are effective in reducing the effective state of the soil, including heavy metals. Among them, DTPA-Pb, DTPA-Zn and DTPA-Cu decreased significantly in the Rr planted soil. They decreased by 42.16%, 28.54%, and 29.21%, respectively. Compared to the blank control, the decreasing dynamics of DTPA-Cu in the Gj planted soil reached 15.38%. The results of the subsequent correlation analysis between the accumulation in the roots of the landscape plants and the DTPA state were shown in Figure 5.
Figure 5. Correlation analysis between root accumulation of garden plants and DTPA state. (a) Pb; (b) Zn; (c) Cu; (d) Cd.
Figure 5 showed that soil amendment agents significantly reduced the heavy metal content of Rr and Ea. The DTPA-Pb and DTPA-Zn correlations for Rr were significant (P < 0.05), while Gj had a degree of negative correlation for Pb and Zn metals under amended soil, and the values varied less at small doses.
Heavy metal soil pollution can cause changes in the growth mechanism and stability of garden plants (Cui and Jin, 2023). This study analyzed the accumulation ability and physiological response of four garden plants under heavy metal stress, and conducted experimental design in combination with soil improvement. The results showed that without adding any heavy metals, the maximum chlorophyll content of Ea plants reached 115.22, indicating that their photosynthetic intensity was greater than that of other plants. The addition of heavy metals can affect the SPAD values of garden plants. Among them, the four plants with Pb metal input showed a SPAD decrease of more than 12% compared to the control group, and the SPAD values of Rr and Pf decreased by much more than 15% compared to the control group. Ea is more susceptible to heavy metal pollution, while Gj plants have better tolerance to heavy metals. The three plant tissues Gj, Ea, and Pf showed an increasing trend in proline values under the influence of heavy metal concentration, indicating their resistance to heavy metals. The increase in the influence of heavy metal Pb on Ea’s plant leaves far exceeds 50%. The reason is that azaleas belong to typical acidic soil plants, with well-developed root systems and high ability to accumulate heavy metals in the soil, resulting in a high ability to accumulate heavy metals. Research has found that heavy metals mainly accumulate in the roots of plants, followed by the stems and leaves. The metal accumulation in various parts of plants is mainly Pb > Zn > Cu > Cd, and the Pb accumulation value in the roots and stems of Rr plants exceeds 300 mg kg-1. This result is consistent with Li’s (Li et al., 2022) research, which found that heavy metals mainly accumulate in the roots of duckweed plants under Zn and Cd pollution conditions. The reason may be that heavy metals exhibit repulsive behavior during absorption and transfer, leading to a more pronounced accumulation in the roots. The enrichment coefficient of Rr roots under Cu is 6.38, indicating its strong enrichment ability for Cu, while the migration rate of Ea in Cu is about 2.0, demonstrating high heavy metal transport capacity. In contrast, Pb mainly exists in the soil in the form of precipitation, making its upward transport in plants more difficult. This is similar to the research results of Rasheed et al. (Rasheed et al., 2020), who found that the roots of lychee fruit trees have a stronger ability to accumulate Zn, Pb, and Cd. In addition, the MDA values of Gj and Ea increased by 6.42% and 1.72%, respectively, under Zn stress, while the MDA value of Pf increased by 54.16% under Cr stress. This indicates significant differences in the tolerance of different plants to heavy metals. Pf has poor tolerance to Cr, possibly due to its antioxidant system failing to effectively respond to heavy metal stress, leading to increased membrane lipid peroxidation. In contrast, Ea exhibits higher tolerance, possibly due to the activation of its flavonoid metabolites, which can degrade and metabolize heavy metals through the myristic acid system, thereby reducing damage (Zhao et al., 2023; Sun et al., 2022).
The application of zeolite amendments significantly reduces the available content of heavy metals in soil. The DTPA Pb, DTPA Zn, and DTPA Cu in Rr planting soil decreased by 42.16%, 28.54%, and 29.21%, respectively, while the DTPA Cu in Gj planting soil decreased by 15.38%. This indicates that zeolite converts heavy metals in soil from active to inactive states through adsorption and ion exchange, thereby reducing plant uptake of heavy metals. This result is consistent with the study by Raklami et al. (Raklami et al., 2022), which found that microbial remediation and amendments can effectively reduce the bioavailability of heavy metals in soil. After improving the soil, the chlorophyll content of the four garden plants increased by more than 60% compared to the conventional group, among which the chlorophyll content of Rr, Gj, Ea, and Pf increased by 41.26%, 16.28%, 21.76%, and 32.64%, respectively. This indicates that zeolite amendments not only reduce the bioavailability of heavy metals, but also improve the photosynthetic capacity of plants. This is similar to the findings of Aboubakar et al. (Aboubakar et al., 2023), which found that Pinus massoniana maintains photosynthesis by adjusting gas parameters under high manganese stress. Rhododendron shows a significant correlation between DTPA Pb and DTPA Zn in improved soil, indicating its strong selectivity in absorbing Pb and Zn. The correlation may be related to the organic acids or chelating agents secreted by their roots, which can activate Pb and Zn in the soil and increase their bioavailability. The root system of Rhododendron may secrete organic acids such as oxalic acid and citric acid to convert Pb and Zn in the soil from inactive to active states, thereby increasing their absorption. Zeolite amendments significantly reduced the heavy metal content in plants of the Euonymus genus, which may be related to their antioxidant mechanism and heavy metal fixation ability. The absorption of Pb and Zn by Gardenia jasminoides showed a certain degree of positive correlation, but the difference in changes was small at low doses. Zeolite improver reduces the absorption of heavy metals by Gardenia jasminoides by lowering the effective state content. Gardenia may reduce the accumulation of heavy metals in the body by enhancing antioxidant capacity or heavy metal rejection mechanisms (Nejad et al., 2018).
There are significant differences in the physiological response mechanisms and stress resistance characteristics of four types of garden plants. Ea exhibits high tolerance, which may be related to the activation of its flavonoid metabolites, which can degrade and metabolize heavy metals through the myristic acid system. In contrast, Pf has poor tolerance to Cr, possibly due to its antioxidant system failing to effectively respond to heavy metal stress. The MDA value of Gj increased less under Zn stress, indicating its strong antioxidant capacity. The enrichment coefficient of Rr under Cu stress was higher, but its SPAD value decreased by more than 15%, indicating that its photosynthesis was inhibited under heavy metal stress. These results are consistent with the study by Natasha et al. (Natasha et al., 2020), which found significant differences in the tolerance and detoxification mechanisms of different plants to heavy metals. For example, nickel (Ni) has the strongest toxicity to plants, while plants with high-fat oxidation exhibit certain variability in different parts. In addition, the study by Panda et al. (Panda et al., 2018) showed that lemongrass exhibited different tolerances in fly ash improved soil, especially at a 25% fly ash ratio, where its photosynthetic rate and photosystem II activity were significantly reduced, but oxidase activity increased, demonstrating good ecological remediation potential. Research has found that zeolite amendments can significantly reduce the available levels of heavy metals in soil and improve the photosynthetic capacity of plants. In addition, the study also found that Ea plants have strong heavy metal tolerance, which may be related to the activation of their flavonoid metabolites. These results provide a theoretical basis for screening and cultivating high tolerance garden plants, and have important technical reference value for the restoration of ecological landscapes in mining areas. The study analyzed the accumulation ability and physiological response of four garden plants to heavy metals, revealing the tolerance mechanisms of different plants to heavy metal stress, and providing new ideas and methods for the remediation of heavy metal contaminated soil. Future research can further explore the tolerance mechanism of garden plants to heavy metals under the action of amendments, especially the role of flavonoid metabolites in heavy metal detoxification. In addition, microbial remediation technology can be combined to study the impact of plant microbe interactions on the remediation of heavy metal contaminated soil.
The original contributions presented in the study are included in the article/supplementary material, further inquiries can be directed to the corresponding author.
NLi: Data curation, Formal Analysis, Investigation, Writing – original draft, Writing – review & editing. NLu: Data curation, Writing – original draft. JZ: Investigation, Writing – review & editing. YY: Investigation, Methodology, Supervision, Writing – review & editing.
The author(s) declare that no financial support was received for the research and/or publication of this article.
Author YY was employed by Co-Innovation Center for Sustainable Forestry in Southern China.
The remaining authors declare that the research was conducted in the absence of any commercial or financial relationships that could be construed as a potential conflict of interest.
The author(s) declare that no Generative AI was used in the creation of this manuscript.
All claims expressed in this article are solely those of the authors and do not necessarily represent those of their affiliated organizations, or those of the publisher, the editors and the reviewers. Any product that may be evaluated in this article, or claim that may be made by its manufacturer, is not guaranteed or endorsed by the publisher.
Abbas, N., Butt, T., Ahmad, M., Deeba, F., and Hussaun, N. (2021). Phytoremediation potential of Typha latifolia and water hyacinth for removal of heavy metals from industrial wastewater. Chem. Int. 7 (2), 103–111. doi:10.5281/zenodo.4559406
Aboubakar, A., El Hajjaji, S., Douaik, A., Labjar, N., Birang a Madong, R. C., Dahchour, A., et al. (2023). Heavy metal concentrations in soils and two vegetable crops (Corchorus olitorius and Solanum nigrum L.), their transfer from soil to vegetables and potential human health risks assessment at selected urban market gardens of Yaoundé, Cameroon. Int. J. Environ. Anal. Chem. 103 (15), 3522–3543. doi:10.1080/03067319.2021.1910251
Abubakar, M. Y., Kaugama, A. A., Japhet, A. T., Ataitiya, H., Ahmad, K. B., Idris, S. A., et al. (2024). Effects and remediation of heavy metals contamination in soil and vegetables from different areas: a review. Earthline J. Chem. Sci. 11 (3), 445–456. doi:10.34198/ejcs.11324.445456
Bai, Y., Zhou, Y., and Gong, J. (2021). Physiological mechanisms of the tolerance response to manganese stress exhibited by Pinus massoniana, a candidate plant for the phytoremediation of Mn-contaminated soil. Environ. Sci. Pollut. Res. 28 (1), 45422–45433. doi:10.1007/s11356-021-13912-8
Bertini, L., Focaracci, F., Proietti, S., Papetti, P., and Caruso, C. (2019). Physiological response of Posidonia oceanica to heavy metal pollution along the Tyrrhenian coast. Funct. Plant Biol. 46 (10), 933–941. doi:10.1071/fp18303
Chaturvedi, R., Favas, P. J. C., Pratas, J., Varun, M., and Paul, M. S. (2018). Effect of Glomus mosseae on accumulation efficiency, hazard index and antioxidant defense mechanisms in tomato under metal (loid) stress. Int. J. Phytoremediation 20 (9), 885–894. doi:10.1080/15226514.2018.1438360
Cui, S., and Jin, H. (2023). Research progress of heavy metal pollution in community garden soil at home and abroad. Chin. Agric. Sci. Bull. 39 (18), 67–74. doi:10.11924/j.issn.1000-6850.casb2022-05-53
El Rasafi, T., Nouri, M., and Haddioui, A. (2021). Metals in mine wastes: environmental pollution and soil remediation approaches–a review. Geosystem Eng. 24 (3), 157–172. doi:10.1080/12269328.2017.1400474
Hatamian, M., Nejad, A. R., Kafi, M., Souri, M. K., and Shahbazi, K. (2019). Growth characteristics of ornamental judas tree (Cercis siliquastrum L.) seedlings under different concentrations of lead and cadmium in irrigation water. Acta Sci. Pol. Hortorum Cultus 18 (2), 87–96. doi:10.24326/asphc.2019.2.8
Hoang, H. G., Chiang, C. F., Lin, C., Wu, C. Y., Lee, C. W., Cheruiyot, N. K., et al. (2021b). Human health risk simulation and assessment of heavy metal contamination in a river affected by industrial activities. Environ. Pollut. 285, 117414. doi:10.1016/j.envpol.2021.117414
Hoang, H. G., Lin, C., Chiang, C. F., Bui, X. T., Lukkhasorn, W., Bui, T. P. T., et al. (2021a). The individual and synergistic indexes for assessments of heavy metal contamination in global rivers and risk: a review. Curr. Pollut. Rep. 7, 247–262. doi:10.1007/s40726-021-00196-2
Ievinsh, G., Dišlere, E., Karlsons, A., Osvalde, A., and Vikmane, M. (2020). Physiological responses of wetland species rumex Hydrolapathum to increased concentration of biogenous heavy metals Zn and Mn in substrate. Proc. Latv. Acad. Sci. Sect. B. Nat. Exact, Appl. Sci. 74 (1), 35–47. doi:10.2478/prolas-2020-0006
Jin, H., Zhao, Z., and Zhi, W. (2022). Responses of acrocarpous moss communities to heavy metal (Fe, Mn, Cd) and sulfur pollution in the Changgou carbonate manganese ore, SW China. J. Mt. Sci. 19 (5), 1292–1306. doi:10.1007/s11629-021-7023-9
Jin-Hua, H., Zhao-Hui, Z., and Zhi-Hui, W. (2022). Responses of acrocarpous moss communities to heavy metal (Fe, Mn, Cd) and sulfur pollution in the Changgou carbonate manganese ore, SW China. J. Mt. Sci. 19 (5), 1292–1306. doi:10.1007/s11629-021-7023-9
Li, Q., Li, X., Bu, C., and Wu, P. (2023). Distribution, risk assessment, and source apportionment of heavy metal pollution in cultivated soil of a typical mining area in southwest China. Environ. Toxicol. Chem. 42 (4), 888–900. doi:10.1002/etc.5586
Li, Y., Xin, J., Ge, W., and Tian, R. (2022). Tolerance mechanism and phytoremediation potential of Pistia stratiotes to zinc and cadmium co-contamination. Int. J. Phytoremediation 24 (12), 1259–1266. doi:10.1080/15226514.2021.2025201
Liu, T., Wang, S., Chen, Y., Luo, J., Hao, B., Zhang, Z., et al. (2023). Bio-organic fertilizer promoted phytoremediation using native plant leymus chinensis in heavy Metal (loid) s contaminated saline soil. Environ. Pollut. 327, 121599. doi:10.1016/j.envpol.2023.121599
Meena, M., Sonigra, P., and Yadav, G. (2021). Biological-based methods for the removal of volatile organic compounds (VOCs) and heavy metals. Environ. Sci. Pollut. Res. 28 (3), 2485–2508. doi:10.1007/s11356-020-11112-4
Natasha, S. M., Saleem, M., Anwar, H., Khalid, S., Tarig, T. Z., Murtaza, B., et al. (2020). A multivariate analysis of comparative effects of heavy metals on cellular biomarkers of phytoremediation using Brassica oleracea. Int. J. Phytoremediation 22 (6), 617–627. doi:10.1080/15226514.2019.1701980
Nejad, A. R., Hatamian, M., Kafi, M., Souri, M. K., and Shahbazi, K. (2018). Interaction of lead and nitrate on growth characteristics of ornamental judas tree (Cercis siliquastrum L.). Open Agric. 3 (1), 670–677. doi:10.1515/opag-2018-0070
Nie, M., Wu, C., Tang, Y., Shi, G., Wang, X., Hu, C., et al. (2023). Selenium and Bacillus proteolyticus SES synergistically enhanced ryegrass to remediate Cu–Cd–Cr contaminated soil. Environ. Pollut. 323, 121272. doi:10.1016/j.envpol.2023.121272
Pachaiappan, R., Cornejo-Ponce, L., Rajendran, R., Manavalan, K., Femilaa Rajan, V., and Awad, F. (2022). A review on biofiltration techniques: recent advancements in the removal of volatile organic compounds and heavy metals in the treatment of polluted water. Bioengineered 13 (4), 8432–8477. doi:10.1080/21655979.2022.2050538
Panda, D., Panda, D., Padhan, B., and Biswas, M. (2018). Growth and physiological response of lemongrass (Cymbopogon citratus (DC) Stapf.) under different levels of fly ash-amended soil. Int. J. Phytoremediation 20 (6), 538–544. doi:10.1080/15226514.2017.1393394
Pandey, J., Verma, R. K., and Singh, S. (2019). Suitability of aromatic plants for phytoremediation of heavy metal contaminated areas: a review. Int. J. Phytoremediation 21 (5), 405–418. doi:10.1080/15226514.2018.1540546
Pang, Y. L., Quek, Y. Y., Lim, S., and Shuit, S. H. (2023). Review on phytoremediation potential of floating aquatic plants for heavy metals: a promising approach. Sustainability 15 (2), 1290. doi:10.3390/su15021290
Peng, S., Jin, Y., Chen, Y., Wu, C., Wang, Y., Wang, X., et al. (2022). Growth response, enrichment effect, and physiological response of different garden plants under combined stress of polycyclic aromatic hydrocarbons and heavy metals. Coatings 12 (8), 1054–1055. doi:10.3390/coatings12081054
Rai, G. K., Bhat, B. A., Mushtaq, M., Tariq, L., Rai, P. K., Basu, U., et al. (2021). Insights into decontamination of soils by phytoremediation: a detailed account on heavy metal toxicity and mitigation strategies. Physiol. Plant. 173 (1), 287–304. doi:10.1111/ppl.13433
Raklami, A., Meddich, A., Oufdou, K., and Baslam, M. (2022). Plants—microorganisms-based bioremediation for heavy metal cleanup: recent developments, phytoremediation techniques, regulation mechanisms, and molecular responses. Int. J. Mol. Sci. 23 (9), 5031. doi:10.3390/ijms23095031
Rasheed, F., Zafar, Z., Waseem, Z. A., Rafay, M., Abdullah, M., Abdus Salam, M. M., et al. (2020). Phytoaccumulation of Zn, Pb, and Cd in Conocarpus lancifolius irrigated with wastewater: does physiological response influence heavy metal uptake? Int. J. Phytoremediation 22 (3), 287–294. doi:10.1080/15226514.2019.1658711
Souri, M. K., Alipanahi, N., Hatamian, M., Ahmadi, M., and Tesfamariam, T. (2018). Elemental profile of heavy metals in garden cress, coriander, lettuce and spinach, commonly cultivated in Kahrizak, South of Tehran-Iran. Open Agric. 3 (1), 32–37. doi:10.1515/opag-2018-0004
Sun, S., Dong, C., Zhang, H., Yang, H., Zhang, N., and Bao, L. (2022). Heavy metal risk assessment of soils and crops in the intensive mining area of Gejiu, Yunnan Province. J. Geoscience Environ. Prot. 10 (11), 128–139. doi:10.4236/gep.2022.1011008
Wang, Y., Lv, N., Mao, X., Yan, Z., Wang, J., Tan, W., et al. (2018). Cadmium tolerance and accumulation characteristics of wetland emergent plants under hydroponic conditions. RSC Adv. 8 (58), 33383–33390. doi:10.1039/c8ra04015j
Yaashikaa, P. R., Kumar, P. S., Jeevanantham, S., and Saravanan, R. (2022). A review on bioremediation approach for heavy metal detoxification and accumulation in plants. Environ. Pollut. 301, 119035. doi:10.1016/j.envpol.2022.119035
Zhao, D., Wu, Q., Zeng, Y., Zhang, J., Mei, A., Zhang, X., et al. (2023). Contamination and human health risk assessment of heavy metal(loid)s in topsoil and groundwater around mining and dressing factories in Chifeng, North China. Int. J. Coal Sci. Technol. 10 (1), 8–47. doi:10.1007/s40789-023-00568-7
Keywords: garden plants, enrichment factor, ecological restoration, SPAD, MDA, proline
Citation: Li N, Lu N, Zhang J and Yu Y (2025) Physiological response mechanism and stress resistance characteristics of four garden plants under heavy metal stress. Front. Environ. Sci. 13:1535294. doi: 10.3389/fenvs.2025.1535294
Received: 27 November 2024; Accepted: 26 March 2025;
Published: 07 April 2025.
Edited by:
Tao Zhang, China Agricultural University, ChinaReviewed by:
Huu Tuan Tran, Văn Lang University, VietnamCopyright © 2025 Li, Lu, Zhang and Yu. This is an open-access article distributed under the terms of the Creative Commons Attribution License (CC BY). The use, distribution or reproduction in other forums is permitted, provided the original author(s) and the copyright owner(s) are credited and that the original publication in this journal is cited, in accordance with accepted academic practice. No use, distribution or reproduction is permitted which does not comply with these terms.
*Correspondence: Yuanchun Yu, eXVhbmNodW55dTF4MnlAMTYzLmNvbQ==
Disclaimer: All claims expressed in this article are solely those of the authors and do not necessarily represent those of their affiliated organizations, or those of the publisher, the editors and the reviewers. Any product that may be evaluated in this article or claim that may be made by its manufacturer is not guaranteed or endorsed by the publisher.
Research integrity at Frontiers
Learn more about the work of our research integrity team to safeguard the quality of each article we publish.