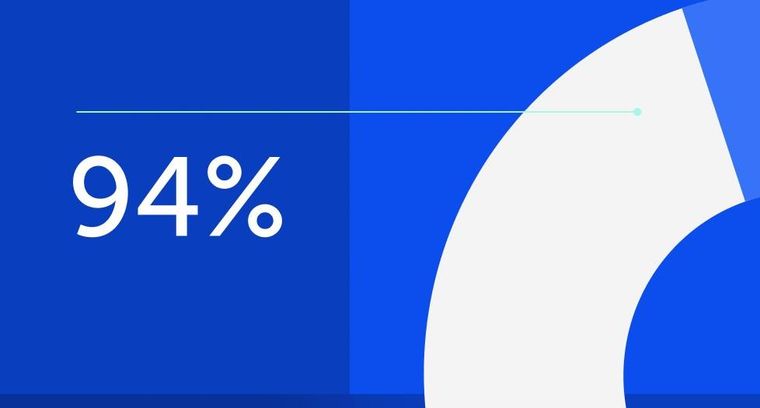
94% of researchers rate our articles as excellent or good
Learn more about the work of our research integrity team to safeguard the quality of each article we publish.
Find out more
ORIGINAL RESEARCH article
Front. Environ. Sci., 06 February 2025
Sec. Water and Wastewater Management
Volume 13 - 2025 | https://doi.org/10.3389/fenvs.2025.1520934
This article is part of the Research TopicSustainable Water Use and Management in Urban AreasView all 4 articles
In the current climate change scenario, Mediterranean cities face heatwaves and reduced availability of freshwater alternated with intense precipitation events in short periods. The demand for water, especially for urban and tourism purposes, is rising, particularly in coastal cities. The importance of integrating nature into cities, particularly trees, has been studied for its benefits in adapting to climate change and improving quality of life. However, water scarcity in cities remains a limiting factor for ensuring the water needs of urban trees and, consequently, the ecosystem services they provide. In this study, we quantified the ecosystem services (CO2 sequestration and storage, O2 production, air pollutants removal, and hydrological effects as transpiration, water intercepted and avoided runoff) provided by urban vegetation in Faro (Algarve, Portugal), with a detailed analysis of three green spaces. We analyzed the importance given to green spaces and the community preferences. Rainwater harvesting was studied as an alternative water source for irrigation and its advantages for the urban water cycle. We found that urban vegetation across the city sequesters 1.09 × 103 t. yr−1 CO2, stores 4.01 × 103 t C, contributes to air pollutant removal (CO = 114; O3 = 3.56 × 103; NO2 = 313; SO2 = 224; PM10 = 872; PM2.5 = 70) kg. yr−1, and prevents 861 m3. yr−1 of surface runoff. In general, people inquired use to visit and value the city’s green spaces, enjoy activities in nature, have definite preferences regarding green spaces, and are available to suggest actions to improve these spaces. It was confirmed that in order to maintain urban green spaces and the ecosystem services that their vegetation provides to the community, rainwater harvesting is of utmost relevance representing a reduction in drinking water for irrigation of 4.20 × 103 m3. yr−1 in the three studied green spaces.
Although urban areas account for less than 2% of the world’s territory, since 2007 they have been home to more than half of the world’s population and it is expected that 68% of the world’s population will live there in 2050 (UNEP, 2022). They concentrate people, infrastructures and services, and account for 80% of global energy consumption and 75% of greenhouse gases (GHGs) emissions, including carbon dioxide (CO2) (Kennedy et al., 2014; Wei et al., 2021). In addition to GHGs emissions, cities are responsible for the emission of other pollutants such as carbon monoxide (CO), sulfur dioxide (SO2), nitrogen oxides (NOx: NO and NO2) and particulate matter (PM10 and PM2.5), among others. These pollutants often coexist in the emissions from vehicles, power plants, factories, and other sources of pollution (Marcantonio et al., 2021; Xiao et al., 2024). Human exposure to these pollutants can promote the development of respiratory and cardiovascular diseases (Sicard et al., 2019; Wei et al., 2024; Yadav et al., 2024), exacerbate existing health problems, and even increase the risk of cancer (Ciabattini et al., 2021). GHGs are major drivers of global warming and climate change (Khalil et al., 2024). Moreover, cities are particularly vulnerable to the effects of climate change (Kumar, 2021). Rising temperatures lead to more frequent heatwaves (Rossiello and Szema, 2019; Wang C. et al., 2024) and intensify the urban heat island effect, which is linked to the presence of buildings, roads, and other infrastructures that absorb and retain heat (Kamal et al., 2023; Ma et al., 2023). Additionally, climate change increases the frequency of heavy rainfall events (Calvin et al., 2023), leading to urban flooding, which is mainly due to large areas of impermeable surfaces found in cities (Han et al., 2024; Wang L. et al., 2024). Heatwaves and the urban heat island effect, exacerbate the impact of air pollutants (CO, SO2, NOx and PM) (Wei et al., 2024), whose concentrations are also higher in cities (Jonidi Jafari et al., 2021; Manisalidis et al., 2020; Stanišić and Stojić, 2020). Climate change has led to water scarcity in Mediterranean areas (Soares and Lima, 2022). Consequently, water use efficiency must increase and, whenever possible, alternative water sources must be implemented for agriculture (Ingrao et al., 2023; Matias et al., 2024; Molénat et al., 2023; Moreira da Silva et al., 2022), tourism (Drius et al., 2019) (including golf courses) (Benlouali et al., 2017; Ortuño et al., 2015), industry and urban uses (Farreny et al., 2011; Marteleira et al., 2014; Reyes-Paecke et al., 2019).
Trees and water are two closely related and fundamental resources for quality of life, particularly in urban areas (Hamel et al., 2021; Pan et al., 2021). Urban trees can benefit people, namely, by improving the water and carbon cycles in cities (Graça et al., 2018; Spotswood et al., 2021). Trees also contribute to carbon dioxide (CO2) sequestration and oxygen (O2) production through photosynthesis (Baslam et al., 2020), and to air pollutants removal by absorbing them through their stomata or simply retaining them on the surface of their tissues (Fares et al., 2020; Nowak, 2023). NO2, SO2, and O3 are absorbed through the stomata of leaves and then either utilized in metabolic processes or converted into less harmful compounds, such as nitrates and sulfates (Hu and Sun, 2010; Shang et al., 2021; Wang et al., 2019). Particles such as PM10 and PM2.5 are captured by the surfaces of leaves and branches (Lu et al., 2018). These particles are trapped until they are removed by natural processes, such as washing by rainwater or the degradation of leaves (Gao et al., 2022). Part of the absorbed water by the plant is released as water vapor into the atmosphere through the leaf stomata (transpiration). This leads to an increase in the air relative humidity and the consumption of energy in the form of latent heat that is used to convert water from the liquid to the gaseous state, leading to a local decrease in temperature (Hernandez Candia and Michaelian, 2010; Ranawana et al., 2023). Therefore, tree canopies provide cool shaded areas, mitigating the effect of heat waves in cities (Liu and Jensen, 2018; Shamsipour et al., 2024). The temperature reduction caused by urban trees improves the quality of life of the people who use the space and, if close to buildings, leads to a reduction in the energy needed to cool it (Gao et al., 2020; Gratani et al., 2016; Winbourne et al., 2020).
In addition to the previously discussed ecosystem services, trees play a crucial role in mitigating urban flooding (Armson et al., 2013). If appropriate management measures and planning solutions are adopted, green spaces including different trees species, can collect and temporarily retain water. This allows peak discharges to flatten, reducing the likelihood of flash floods that could cause human and material damage (Mentens et al., 2006; Smith et al., 2024; Yousofpour et al., 2024). During rainfall, the tree canopy intercepts and retains water on its leaves and branches (Baptista et al., 2018a). A portion of this water evaporates back into the atmosphere, never reaching the ground, while the rest eventually falls, but with a delay compared to the onset of the rain (Yang et al., 2019). This delayed and reduced water flow helps to minimize surface runoff, effectively reducing the risk of flooding in urban areas. In the Mediterranean, rainwater interception is ensured mainly by evergreen species.
Urban green spaces as parks, gardens or squares, are a way to bring nature into cities and offer benefits to individuals and communities. They promote physical activity by offering space for exercise, improving cardiovascular health, reducing obesity, supporting mental wellbeing, and helping to reduce stress and anxiety through exposure to nature (Almeida et al., 2018). Economically, urban green spaces can increase property values and attract tourism, providing recreational opportunities and boosting local businesses. Additionally, they serve as spaces for community events and social interaction, fostering a sense of belonging. Green spaces offer valuable educational opportunities, particularly in environmental awareness, where visitors can learn about biodiversity, sustainability, and conservation efforts, enhancing public knowledge in ecology. Even in highly urbanized areas where urban green spaces cannot be implemented, nature can still be incorporated into cities to promote carbon sequestration and other ecosystem services through alternative solutions as green walls (Jozay et al., 2024) or green roofs (Kafy et al., 2024).
Implementing the Sustainable Development Goals (SDG) at the city level requires a new vision for the urban water cycle (Poch et al., 2023; Willaarts et al., 2024) incorporating actions across the five domains defined by the United Nations: people; planet; prosperity; peace; and partnerships (Tremblay et al., 2020). The traditional focus on large, centralized infrastructures and conventional water sources (surface and groundwater), with emphasis on quantity, quality, and cost efficiency, is no longer sufficient for addressing the needs of current and future generations. It is now essential to incorporate the socio-ecological dimensions, promote innovative technological and social solutions, engage communities, protect biodiversity and respect the territorial specificities of each city (Gain et al., 2021). The challenges of freshwater scarcity and rapid urban expansion have driven the need to define alternative water sources, such as rainwater harvesting and water reuse (Duckett et al., 2024). Even in urban areas with safe water distribution for human consumption, these alternative sources can be used for non-potable purposes, such as irrigating urban trees (Campisano et al., 2017; Lupia et al., 2017; Wang et al., 2021) or peri-urban orchards (Moreira da Silva et al., 2022).
The objectives of this study were: i) to quantify the ecosystem services provided by urban vegetation in a Mediterranean coastal city (Faro, Algarve—Portugal), facing heatwaves and water scarcity, with a detailed analysis of three public green spaces; ii) to assess the importance given to green spaces and the community preferences; iii) to study the feasibility of rainwater harvesting for green spaces irrigation decreasing the drinking water consumption and contributing to the urban water cycle improvement.
The study was conducted in Faro, the capital of the Algarve region, in southern Portugal (Figure 1), with more detailed analysis of three urban green spaces of this city (Figure 2): an urban park—Parque Ribeirinho (37.02451°N; −7.94667°W), an urban garden—Jardim da Alameda (37.01205°N; −7.93227°W), and a public square—Largo de S. Francisco (37.01514°N; −7.92664°W).
Faro has a resident population of around 42,000 (Instituto Nacional de Estatística, 2024), with significant seasonal variation due to tourism. The city’s main economic activities are related to tourism and commerce, along with fisheries, aquaculture and salt production in the nearby Ria Formosa. The city has 96% of its surface covered by impervious materials, including buildings, roads, sidewalks and parking areas.
The Parque Ribeirinho (Figure 2) is an urban park located along the Ria Formosa, which is a coastal lagoon classified as Natural Park and Ramsar site (Istomina et al., 2016). This urban park is distinguished by its scenic views and trails conducive to outdoor exercise, such as walking, running and cycling, while also serving as a sanctuary for birdwatching. The Jardim da Alameda (Figure 2) is the city’s oldest garden, provides a densely wooded environment ideal for leisurely walks, family activities, and cultural events, featuring a small playground and a fitness circuit. In contrast, the Largo de São Francisco (Figure 2) is a public square, which functions as a parking facility and as a social hub, hosting markets and temporary events. Each of these green spaces plays a unique role in the daily life of Faro’s residents and visitors. These green spaces differ also in area (Table 1). The urban garden is the smallest space and the one with the highest percentage of paths. It also includes impermeable areas like a skating rink and a playground. The urban park, the largest space, features the most lawn coverage and 32% biodiverse meadows, unique to this site. It also has fewer impermeable surfaces, mainly an amphitheater for public events. In contrast, the public square has over 86% of its area as impermeable roads and parking, with 13% as lawns near the medieval city wall and railway line.
The city is characterized by a Mediterranean Csa climate (Köppen-Geiger classification), with a mean annual precipitation of 483 mm, based on data since 1966 (PORDATA, 2024). Recent trends show a 20%–35% decrease in annual precipitation and a 20%–30% increase in extreme rainfall events (Soares et al., 2015). The typical wet season occurs between October to April, marked by intense, short rainfall events that often lead to urban flooding, causing significant material and human damage (Veloso et al., 2015), followed by long dry periods.
According to Portuguese Environment Agency, the emissions of air pollutants in Faro are of CO = 0.55 kg. yr−1, PM10 = 0.18 kg. yr−1, NO2 = 0.10 kg. yr−1, PM2.5 = 0.09 kg. yr−1, and SO2 = 0.03 kg. yr−1 (QUALAR, 2024).
The Algarve’s Multimunicipal Water Supply System is managed by Águas do Algarve (AdA) and abstracts surface water from Odelouca dam (Odelouca Stream), Odeleite-Beliche dams (Guadiana River basin) and Bravura dam (Odiáxere Stream), and groundwater from the Almádena\Odiáxere and Querença\Silves aquifers. Water abstraction varies based on hydrological conditions, water availability, treatment plant capacity, and distribution needs. AdA oversees four advanced Water Treatment Plants with a total capacity of 5.65 m3 s−1, along with 34 pumping stations, 18 storage tanks, 490.8 km of pipelines, and 78 delivery points. In 2022, 72.6 Mm3 of water were abstracted for municipal use in the Algarve, with 93% from surface sources and 7% from groundwater. During the 2022–2023 hydrological year, the Algarve Stream Basin, home to the Odelouca and Bravura dams, faced extreme drought, while the Guadiana River basin, where the Odeleite-Beliche dams are located, experienced moderate drought, insufficient for necessary volume regularization. There is a Water Safety Plan that ensures rigorous quality control from abstraction to consumer’s tap, managing the various risks to consistently provide safe drinking water (World Health Organization, 2023). The water supply system in Faro is managed by Fagar, includes 19 tanks with a total storage capacity of 28,680 m3 and a distribution network that extends over 574.86 km, covering 96% of the municipality and serving around 40,500 customers. The average daily consumption is 17,144 m3 for urban uses. In 2022, Fagar purchased 6.37 Mm3 of treated water from AdA, losing about 22.8% in distribution before reaching consumers’ taps. These water losses equated to 148,1407 kWh of energy and 203.33 t CO2e emissions (FAGAR, 2023).
Faro’s urban catchment covers 4.7 km2, divided into seven subcatchments linked to seven discharge points. Rainwater is managed by a 109 km drainage network, separate from the wastewater system, and discharged into streams or directly into the Ria Formosa saltmarsh. Fagar is also the company responsible for collecting and draining wastewater from the municipality of Faro, sending it to two municipal Wastewater Treatment Plants (WWTP), Faro-Noroeste and Faro-Olhão. These facilities are managed by AdA and provide secondary treatment enabling the reuse of treated water for some non-potable purposes (Moreira da Silva et al., 2022). Faro’s sanitation system covers 90% of the municipality, with 17 pumping stations and a 302 km long wastewater collection network. In 2022, 41.258 Mm3 of wastewater were collected and treated but only about 5% of the treated effluent was reused for irrigation of golf courses and gardens, ecosystem support and WWTP internal purposes.
To quantify the ecosystem services provided by the urban vegetation in the studied green spaces and across the city of Faro, an extensive georeferenced inventory of tree, palm and shrub specimens, provided by the municipality of Faro, was used. The diameter at breast height (DBH) and total height were recorded for all specimens. We used i-Tree Eco v6 software, developed by the USDA Forest Service Northern Research Station, accessible at itreetools.org. The meteorological data considered by the model was collected by a local weather station of the national authority, Portuguese Institute for Sea and Atmosphere (IPMA). Pollutant data were collected by the local air quality station of the national authority, the Portuguese Environment Agency (APA). Carbon storage, carbon sequestration, oxygen production and air pollutant (CO, O3, NO2, SO2, PM10, and PM2.5) removal were quantified. The hydrological effects were also estimated, including the amount of water intercepted by the canopy during rainfall, avoided runoff, transpiration, and evapotranspiration.
To assess the relevance of public green spaces for the Faro community and understand their preferences/suggestions, an anonymous survey was created on Google Forms and distributed through social networks, with the following questions: 1. Do you visit public green spaces in Faro?; 2. What are the reasons that lead you to visit (or not) public green spaces?; 3. What are your favorite public green spaces in Faro?; 4. What kind of improvements do you think should be implemented in Faro’s green spaces?.
Transpiration and evapotranspiration values were obtained through ecosystem services quantification (Section 2.2) and allowed a rough quantification of irrigation water for urban vegetation. Fagar provided the accounting of drinking water consumption for irrigation of the three green spaces between January 2017 and December 2021. The data were analyzed to calculate monthly averages for each green space and the results were compared with corresponding transpiration and evapotranspiration values. To assess the feasibility of rainwater harvesting as an alternative water source for irrigation of the studied green spaces, we identified the nearest public buildings (Table 2). We calculated the size of storage tanks considering annual volume of useable rainwater, according to the average annual of accumulated precipitation in Faro (483 mm, according to IPMA), the catchment areas, and roof characteristics. The annual volume of useable rainwater (Va) was calculated according to ETA ANQIP 0701 (ANQIP, 2022):
where,
Table 2. Public building’s location (coordinates) close to the studied green spaces, and respective storage tanks sizing for rainwater harvesting.
C is the runoff coefficient considering water retention, absorption and diversion on the collection surface (80% for flat or low slope waterproof roof, according to TA ANQIP 0701 and EN 16941-1:2018 (ANQIP, 2022; European Committee for Standardization, 2018); P is the average annual precipitation in Faro (483 mm); A is the coverage catchment area measured in horizontal projection; and nf is the hydraulic filtration efficiency (in general close to 90%).
The studied urban green spaces present different plant diversity and relative abundances (Table 3). The urban garden, despite being the smallest of the three spaces, has the highest species diversity, featuring 72 different species of trees, palms and shrubs, containing a total of 375 specimens and corresponding to the space with higher density of specimens. The public square is the green space with the least species diversity (17 species) and the lowest specimens density. Globally the city contains 13,985 specimens of 246 different species, among these, just 10% are native, the same percentage observed in the urban spaces except for the public square, where it was 6%. The evergreen species range from 62% to 65% in the urban spaces and correspond to 65% across the city. However, the proportion of evergreen specimens decreases from 72% in the urban garden, to 41% in the urban park, and to 30% in the public square. Between 88% and 93% of the specimens in the urban green spaces and across the city are flowering species (Angiosperms), while the remaining 7%–12% are Gymnosperms.
The 10 most abundant species vary among the green space and across the city (Table 4). In the urban garden, the most common species is Washingtonia robusta, which has the fourth most common in the urban park and the fifth most common in the city (with 624 specimens). The Tipuana tipu is the most abundant species in the urban park and in the public square, corresponding respectively, to 22% and 60% of the specimens. Among the 145 specimens present in the public square, 87 are T. tipu, and the second most abundant species has only 8 specimens. It is also the second most common species citywide.
The performance of the urban vegetation for CO2 sequestration, carbon storage, O2 production, and air pollutant removal is presented in Table 5. Among the three studied urban spaces, the urban park demonstrated the highest values for sequestered CO2 per year and stored carbon, produced O2 per year, and removed air pollutants per year, followed by the urban garden, and lastly the public square. However, the urban garden presents greater efficiency in CO2 sequestration and O2 production per area, since it has a greater tree density (375 trees in 21,189 m2) than the urban park (488 trees in 95,620 m2). The urban square was the green space with the lowest contribution to the quantified ecosystem services, as it has the lowest tree density (145 trees in 43,260 m2). Our results showed that globally, the urban vegetation in Faro has an annual capacity to sequester 1.09 × 103 t of CO2 and to produce 793 t of O2. The biomass of the studied vegetation represents 4.01 × 103 t of stored carbon in the city.
Table 5. Ecosystem services provided by urban vegetation and hydrological effects in the studied green spaces and in the Faro city.
Regarding the amount of air pollutants removed by urban vegetation, it was higher in the urban park, followed by the urban garden and lastly the urban square, and the citywide removed 5.15 × 103 kg. yr−1 of different air pollutants, as described in Table 5. Different air pollutants were systematically removed in different amounts, in descending order: O3 > PM10 > NO2 > SO2 > CO > PM2.5.
Our results showed that vegetation in Faro city promotes important hydrological effects, intercepting the rainwater and reducing the runoff. The vegetation intercepts 290 m3. yr−1 of rainwater in the urban garden, 170 m3. yr−1 in the urban park, and 18.9 m3. yr−1 in the public square. Across the city, this value rises to around 3.88 × 103 m3. yr−1. These green spaces are important for water-flow regulation, avoiding runoff, and preventing/reducing urban floods. Due to its highest density of trees, the urban garden presented the highest avoided runoff (65 m3. yr−1), while in the urban park the avoided runoff was 39 m3. yr−1, and in the public square the value was 4 m3. yr−1. Globally, the urban vegetation across the city is responsible for 860 m3. yr−1 of avoided runoff.
A total of 649 completed surveys were received and validated, with 79% of the respondents being residents in Faro. Among those who chose to respond, 66% identified as female and 34% as male. The age distribution of respondents was: 13% between 18 and 30 years old; 43% between 31 and 45 years old; 41% between 46 and 65 years old; and 3% over 65 years old. Among the respondents, 80% held higher education qualifications, 18% high school, and 2% just basic education. Out of all respondents, 19% indicated that they do not visit any green spaces in the city of Faro, and 81% use to do it (Figure 3). Among respondents who do use green spaces, 25% use them for sports and exercise, while the majority, 55%, visit to walk, connect with nature, read, and/or relax.
Figure 3. Answers to the questions: 1. Do you visit public green spaces in Faro?; and 2. What are the reasons that lead you to visit (or not) public green spaces?.
When it was asked What are your favorite public green spaces in Faro? 25% of respondents chose Jardim da Alameda (the urban garden), making it the second most popular green space in the city. In fourth place was Largo de S. Francisco (the public square), with 8% of respondents identifying it as their most frequented green space. In sixth place, Parque Ribeirinho (the urban park) was favored by 3% of respondents as the green space they most visit. Respondents were given a short-response section to suggest improvements for the green spaces. Suggestions included adding more vegetation, particularly trees for shading, and installing water drinking fountains. They also requested public restrooms or kiosks for immediate needs. Other suggestions focused on upgrading the spaces through expansion, improved pavement, new or replacement equipment, and better lighting. Finally, they emphasized the need to improve maintenance and cleaning, as well as installing more waste containers.
From 2017 to 2021, the mean monthly temperature ranged between 12°C in January and 25°C and in August (Figure 4). March was the wettest month, with a mean precipitation of 49 mm, while in Summer, July and August presented precipitation values close to zero (0.1 mm).
During the Summer, in the hottest months (May to August) the urban green spaces presented the highest values for transpiration and evapotranspiration, with the maximum values in July and August (Figure 5), when the precipitation was close to zero. The irrigation of the urban park (Figure 5A) and the urban square (Figure 5C) was exclusively ensured by drinking water, while in the urban garden there is a hole to provide groundwater for irrigation, and which is not counted (Figure 5B). Thus, the average monthly irrigation in the urban park and in the public square, reached the maximum values from June to September and from July to September, respectively. Figure 5C shows that drinking water irrigation levels in the urban garden have remained consistently lower than transpiration, and this occurrence is due to the use of groundwater for irrigation.
Figure 5. Average monthly transpiration, evapotranspiration, and irrigation with drinking water in the studied green spaces from 2017 to 2021: (A) urban park (Parque Ribeirinho); (B) – urban garden (Jardim da Alameda); and (C) – public square (Largo S. Francisco).
Considering the period 2017 to 2021, if we integrated rainwater harvesting from the roofs of public buildings in the vicinity of green spaces (Table 2), we could meet a significant part of irrigation needs and significantly reduce drinking water consumption, as presented in Table 6. In the case of the urban garden, considering the difference between evapotranspiration and harvested rainwater we could reduce the groundwater abstraction in 10,099 m3. yr−1, and use only rainwater for irrigation and for other non-potable uses, as washing of waste containers and impermeable surfaces (e.g., skating rink). Groundwater could be used as an alternative reserve for long periods without precipitation, meaning the use of drinking water would no longer be necessary to irrigate this urban garden.
Several evidences have been demonstrated on the physical and social contribution of green spaces to climate change mitigation and adaptation, but the multifunctional and multiscale nature of urban green infrastructure complicates the categorization of services and benefits, as in reality the interactions between different benefits are multiple and occur at different spatial scales, i.e., city, neighborhood and specific site (Demuzere et al., 2014). The differences in the areas of the three urban spaces are closely related to their characteristics, location (Table 1), and the activities that provide to the community. The urban garden (Jardim da Alameda), is the smallest green space with great tree density, located in the city center and nearby schools, where the contact with nature is limited. This is the second most appreciated by the community, mainly for connect with nature, read, and/or relax. Among the studied green spaces, the urban garden also has 7% more of impermeable surfaces which correspond to infrastructures as a playground and a skating rink, that promote physical activity, social interaction, and overall wellbeing, making this public space more attractive and useful for diverse age groups (Oosterbroek et al., 2024; Wang Y. et al., 2024). There are several approaches to finding space and favorable conditions to green infrastructure in cities, some of which have already been studied at European scale, such as the implementation of green roofs on buildings, which have demonstrated several benefits for the urban water cycle, energy consumption and climate resilience (Quaranta et al., 2021) The urban park (ranked as the sixth most appreciated green space) is located outside the city centre, in a less urbanized area along the Ria Formosa saltmarsh, with an extensive area and vegetation cover (including biodiverse meadows and higher percentage of lawn) suitable for physical exercise and pathways for family walks. Biodiverse meadows offer significant ecological advantages over traditional lawns, enhancing biodiversity by supporting a wide range of plant and animal species, and improving ecosystem services as pollination (Norton et al., 2019; Paudel and States, 2023). In contrast to lawns, which require intensive maintenance and inputs as synthetic fertilizers and frequent mowing, biodiverse meadows are self-sustaining, contributing to soil health and reducing environmental damages. The studied public square (ranked as the fourth most appreciated green space) comprises roads and parking lots (86% of the total area), lawn areas (13%), and two lakes/water features (0.9% of area). The trees present in this space provide shade for the parking lot or are scattered throughout the lawn area. This public space functions as a parking facility, crucial for the community in the urban mobility model currently existing in Faro, but also as a social hub, hosting markets and other temporary events. The studied urban spaces have different designs and sizes, which leads to different plant diversity and relative abundance (Table 3). Among the studied green spaces, the urban park is smaller than the urban garden but has a greater diversity of species, with 72 different species of which 90% are exotic. The public square has the lowest species diversity and the fewest trees among the three green spaces studied. The use of exotic plants in the studied urban spaces and across the city appears to be common. Among the non-native trees, some were introduced several centuries ago and are already part of the Mediterranean landscape and traditions, such as citrus, which are present in almost all Mediterranean cities (Duarte et al., 2016; Matias et al., 2021). Some authors have shown that exotic urban tree species can play an important role in the ecosystem services they provide (Martin et al., 2014; Muvengwi et al., 2024; Riley et al., 2018), while others have found that native species can provide greater benefits (Arcos-LeBert et al., 2021). However, in urban areas facing water scarcity, previous studies demonstrate that native species provide more ecosystem services than exotic ones, as they are more water-efficient and promote greater soil carbon storage (Dhanya et al., 2014; Guillen-Cruz et al., 2021). Although 65% of the species in the public square are evergreen, only 30% of the specimens are evergreen. In the Mediterranean climate, the cold and rainy season corresponds to the period in which deciduous species lose their leaves (Deitch et al., 2017). In urban spaces where most of the surface is impermeable, the use of evergreen trees should be prioritized to take advantage of the trees’ ability to intercept rainwater through their canopy, reducing surface runoff and helping to prevent or mitigate urban flooding (Baptista et al., 2018b; Li et al., 2023; Ma et al., 2022). Additionally, the leaves shed by deciduous trees must be cleared from surfaces to avoid clogging rainwater drainage systems, which requires more labor (Yeom and Ahn, 2024). In contrast, when used in urban spaces, deciduous species can be strategically placed in areas with bare soil, where fallen leaves do not need to be removed, do not cause blockages, and decompose naturally, contributing to organic matter and water retention in the soil (Bakhmatova et al., 2022; Yang et al., 2014).
Urban green spaces play a crucial role in carbon sequestration, helping pave the way towards carbon neutrality, especially in urban environments where emissions are higher (Fan et al., 2022; Moreira da Silva et al., 2024). The ability of the studied green spaces for CO2 sequestration is related to its characteristics, including the species composition and respective density. Previous studies have shown that different tree species supply ecosystem services with different efficiency and that within each species, the size and condition of trees are important aspects, namely, for carbon sequestration and storage (Graça et al., 2018; Sæbø et al., 2012; Snehlata et al., 2021; Wang et al., 2021). The urban garden presents greater species diversity with 375 specimens in 2.1 ha, and annually sequesters 16.5 t.ha−1 of CO2. The urban park presents lower species diversity with 488 specimens in 9.6 ha and annually sequesters 5.41 t.ha−1 of CO2. Finally, the public square presents the lowest species diversity with 145 specimens in 43.260 ha, and has the lowest capacity for annual CO2 sequestration, 4.11 t.ha−1. However, in absolute quantity, the urban park sequesters more CO2 (51.7 t. yr−1) than the urban garden (35.0 t. yr−1) and public square (17.0 t. yr−1). Overall, the studied vegetation across the city sequesters 1.09 ×103 t. yr−1 of CO2, which offsets the emissions of 188 Portuguese (5.8 t per capita), only about 0.5% of the residents in Faro (Instituto Nacional de Estatística, 2024). There are still few studies on CO2 sequestration in urban green spaces, with similar species and under comparable edaphoclimatic conditions to those of this work. Previous works, report 47.5 t.ha−1 of CO2 sequestration per year for smaller urban parks with fewer trees, in Dhaka–Bangladesh with a subtropical climate (Shadman et al., 2022). Some of CO2 sequestered by the vegetation is converted in biomass in the trunks, branches, leaves, and roots (Varshney et al., 2022), and remains stored as long as the specimen is alive, preventing its release to the atmosphere and contributing to a medium or long-term carbon storage (Nunes et al., 2020). The studied urban spaces also function as carbon sinks, in decreasing order: urban garden (20.6 C t.ha−1) > urban park (86.4 C t.ha−1) > urban square (12.5 C t.ha−1). Recently, some authors (Aguilar-Tello et al., 2023) reported C storage values between 2.5 and 8.0 t.ha−1 for urban parks, in South America (Peru). Green biomass and arboreal area ratio are considered the most important factors for CO2 concentration (Jiang et al., 2023). Spatial patterns, as canopies density positively contribute to carbon sinks, whereas areas like parkways have a negative effect on CO2 distribution. During photosynthesis (6 CO2 + 6 H2O → 6 C6H12O6 + 6 O2), plants sequester CO2 and release O2 to the atmosphere in a direct ratio. Therefore, among the studied green spaces, the urban park presents the highest O2 production (37.7 t. yr−1), followed by the urban garden (25.5 t. yr−1), and the lowest value was quantified in the public square (12.9 t. yr−1). Urban vegetation also contributes to the removal of air pollutants as CO, O3, NO2, SO2, PM10, and PM2.5, and its efficiency is affected by the characteristics of the species as leaf size and structure, wax content, ultrastructure, thickness, pubescence, and surface roughness (Vigevani et al., 2022; Weyens et al., 2015; Yousofpour et al., 2024). Climatic conditions (precipitation and wind), and quantity and composition of atmospheric pollutants also can affect their removal by vegetation. According to Portuguese Environment Agency, air quality is normally classified as good (https://www.iqair.com/portugal/faro). Our results show that the amount of air pollutants removed in the studied green spaces is directly related to the number of trees (Table 5), in decreasing order kg. yr−1: urban park (CO = 6.9; O3 = 201; NO2 = 19.1; SO2 = 13.7; PM10 = 53.2; PM2.5 = 4.3) > urban garden (CO = 4.9; O3 = 135; NO2 = 13.4; SO2 = 9.6; PM10 = 37.3; PM2.5 = 3.0) > urban square (CO = 2.1; O3 = 49.9; NO2 = 5.7; SO2 = 4.1; PM10 = 15.8; PM2.5 = 1.3). The planning and installation of urban green spaces must consider their vegetation and ecosystem services, as important tools for adapting to anthropogenic and climate change. Certain trees species can function as natural biodevices for air pollutant removal, particularly in urban hot spots, where emissions are higher due to human activities, as transportation or industry (Bressane et al., 2024). The studied urban park is located near railway track, where fuel combustion by trains results in air pollutants emission, as NO2, PM10, and PM2.5 (Font et al., 2020; Lalive et al., 2018). To improve the urban park performance in that air pollutants removal, corridors of native tree species can be installed within the park along the tracks, to phytoremediate the pollutants released by trains, functioning as visual and sound barriers (Gharibi and Shayesteh, 2024), and contributing to the aesthetics of the green space. Among the native tree species, previous studies in Algarve region (Moreira da Silva et al., 2024) recommended the use of Ceratonia siliqua and Pinus pinea which are drought-tolerant species with high capacity for air pollutant removal. Flooding in urban areas is becoming more frequent, and different and complementary preventive approaches are needed to reduce or even eliminate its impact, including, for example, deep learning modelling (Zhao and Zhiwen, 2024). Our results confirm that urban trees in green spaces can improve the urban water cycle, contributing to flooding risks mitigation in cities, particularly during extreme precipitation events, increasingly frequent in regions such as the Algarve, in the Mediterranean. The trees of the studied green spaces intercepted the rainwater through their canopies reducing the volume of water that immediately runs off. Among the urban green spaces, the urban garden located in a flood-prone area in the city center intercepted 287 m3. yr−1, preventing 64.5 m³ of surface runoff annually. Urban planning should also prioritize the potential of certain urban tree species to prevent surface runoff during rainfall. In flood-prone areas and regions where rainwater runoff converges, evergreen trees should be strategically selected and placed on sidewalks, parking lots, and close to other expansive impermeable surfaces. In the studied public square most of surface is impermeable, where 86% of the total area is roads and parking spaces, and the trees intercept 18.9 m³ of rainwater annually, preventing 4.1 m³ year−1 of surface runoff. However, 70% of the specimens in this space are deciduous trees. In flood-prone areas, with few permeable zones, evergreen tree species with higher capacity to rainwater interception should be preferred to deciduous tree species. Currently, 60% of the specimens in this public square are of T. tipu, an exotic and deciduous species that is highly valued for its aesthetic appeal and the shade it provides, mainly in Spring and Summer. However, T. tipu has a low capacity to intercept water during the months with higher precipitation, in Fall and Winter, when its leaves have already fallen. If T. tipu were replaced, for example, by C. siliqua, an additional water volume of 61.5 m3. yr−1 could be intercepted, resulting in a further reduction of 13.6 m3. yr−1 of surface runoff. Maintenance practices in public green spaces affect the ecosystem services provided by trees and thus their role for climate resilience. Lawn mowing cause GHGs emissions primarily by biogenic soil processes and requires the use of equipment that also contributes to GHG emissions (Lerman and Contosta, 2019). While pruning trees can stimulate new growth, if done properly, it reduces the tree canopy (Jacinto et al., 2024; Matias et al., 2023), making them less effective at providing shade, at retaining rainwater and by removing stored carbon, which is then released into the atmosphere (Nero et al., 2024; Speak and Salbitano, 2023). The use of synthetic fertilizers releases nitrous oxide (N2O), a potent GHG (Mendoza Beltran et al., 2022; Yang et al., 2024). Organic waste management (like grass clippings, leaves, and branches) affects the carbon balance of public green spaces. Although decomposition releases GHGs into the atmosphere (Yasmin et al., 2022), applying this organic matter on-site avoids the GHG emissions associated with transportation and landfill decomposition (Grossi et al., 2024; Nordahl et al., 2023; Zhazhkov et al., 2024). When applied to urban soils, it improves water retention, enhances carbon storage, and reduces the need for synthetic fertilizers (Baldi et al., 2018; De Lucia et al., 2013; Li et al., 2021; Oldfield et al., 2014). Our results confirm the benefits of trees in the studied green spaces, related to the quality of life for Faro’s community and to climate resilience. The ecosystem services provided by urban green spaces can be significantly enhanced if considered in their planning or redesignated with this aim (Xie et al., 2019). Also, local communities should be listened to and engaged in the co-design of the public green spaces.
Despite all the benefits, green spaces in cities require water for irrigation, which is a scarce resource in many Mediterranean cities, as is the case of Faro. To maintain the vegetation’s water needs, irrigation must be calculated based on evapotranspiration. In the studied urban green spaces, between 2017 and 2021 there were situations in which this did not occur. From July to October, in the urban park, irrigation exceeded evapotranspiration, as well as in the public square, from March to October, with a peak in September. These occurrences must be related to the irrigation of lawn areas, whose transpiration was not considered in this study. However, the September peak observed in the public square must correspond to a specific social event. To reduce the drinking water consumption for non-potable uses, such as green spaces irrigation, cities should explore alternative water sources, in this case rainwater harvesting. According to our results, capturing rainwater on the roofs of public buildings in the vicinity of green spaces can be an important efficiency measure in the urban water cycle in Faro, reducing the use of potable water for irrigating green spaces and maintaining the ecosystem services provided by trees. The reduction in annual consumption of drinking water for irrigation of urban green spaces was 4,199 m3, varying between 30% and 100% depending on the green space. This reduction corresponds to savings of 4,283 kWh, avoided emissions of 798 kg CO2e, or the volume of drinking water that 59 Portuguese people consumed in 2022 (https://www.ersar.pt/pt/setor/factos-e-numeros). Although this study did not include the financial analysis of systems for rainwater harvesting and storing, this should be considered in a subsequent phase.
The quantification of ecosystem services provided by urban vegetation (trees and shrubs) in the three studied green spaces highlighted their importance for the local community health and wellbeing and for the city’s climate resilience. Overall, urban vegetation sequesters 1.09 × 103 t. yr−1 of CO2, stores 4.01 × 103 t C, produces 793 t. yr−1 of O2, contributes to air pollutant removal (CO = 114; O3 = 3.56 × 103; NO2 = 313; SO2 = 224; PM10 = 872; PM2.5 = 70) kg. yr−1, and prevents 861 m3. yr−1 of surface runoff. According to this study, the CO2 sequestration offsets the emissions of less than 1% of Faro residents. The contribution of each studied green space for the quantified ecosystem services, depends on their characteristics, including the area of each land use, species composition and tree density, and must be improved. The community consultation showed that most people value urban green spaces, enjoy carrying out activities in contact with nature, have defined preferences regarding green spaces, and are available to suggest actions to improve these spaces. According to the obtained results, in the current scenario of water scarcity that the Algarve region faces, to maintain urban green spaces and the ecosystem services that its vegetation provides to the community, rainwater harvesting is an alternative water source for irrigation that should be used to improve the efficiency of the urban water cycle. The use of rainwater to urban green spaces irrigation is relevant to maintain their functioning, allowing this Mediterranean city to: reduce the consumption of drinking water; improve the CO2 sequestration and accelerate carbon neutrality; improve air quality; increase resilience to drought and extreme precipitation events; decrease the severity of heatwaves; and maintain healthier daily activities.
The data analyzed in this study is subject to the following licenses/restrictions: They are property of Faro Municipality. Requests to access these datasets should be directed to ZmN1bmhhQGNtLWZhcm8ucHQ=.
PM: Conceptualization, Data curation, Investigation, Methodology, Software, Writing–original draft, Writing–review and editing. MM: Investigation, Methodology, Writing–original draft, Writing–review and editing, Conceptualization, Data curation, Funding acquisition, Project administration, Supervision, Validation. JT: Conceptualization, Data curation, Investigation, Methodology, Resources, Writing–original draft, Writing–review and editing. AD: Supervision, Writing–original draft, Writing–review and editing.
The author(s) declare that financial support was received for the research, authorship, and/or publication of this article. This work supported by CIMA–Centre for Marine and Environmental Research (https://doi.org/10.54499/UIDP/00350/2020) MED UIDB/05183/2020 (https://doi.org/10.54499/UIDB/05183/2020) and CHANGE LA/P/0121/2020 (https://doi.org/10.54499/LA/P/0121/2020).
Arch. Filipe Cunha—Director of the Department of Infrastructure and Urbanism, Faro Municipality, for providing data on urban vegetation and drinking water consumption in urban green spaces.
The authors declare that the research was conducted in the absence of any commercial or financial relationships that could be construed as a potential conflict of interest.
The author(s) declare that no Generative AI was used in the creation of this manuscript.
All claims expressed in this article are solely those of the authors and do not necessarily represent those of their affiliated organizations, or those of the publisher, the editors and the reviewers. Any product that may be evaluated in this article, or claim that may be made by its manufacturer, is not guaranteed or endorsed by the publisher.
Aguilar-Tello, A., Aponte, H., and Alegre, J. (2023). CO2 sequestration and O2 production in urban parks: the case of an introduced species in tropical south America. J. Urban Environ. Eng., 13–21. doi:10.4090/juee.2023.v17n1.13-21
Almeida, C. M. V. B., Mariano, M. V., Agostinho, F., Liu, G. Y., and Giannetti, B. F. (2018). Exploring the potential of urban park size for the provision of ecosystem services to urban centres: a case study in São Paulo, Brazil. Build. Environ. 144, 450–458. doi:10.1016/j.buildenv.2018.08.036
ANQIP (2022). Technical specification ETA 0701-rainwater harvesting systems in buildings (version 11). Aveiro, Portugal: ANQIP.
Arcos-LeBert, G., Aravena-Hidalgo, T., Figueroa, J. A., Jaksic, F. M., and Castro, S. A. (2021). Native trees provide more benefits than exotic trees when ecosystem services are weighted in Santiago, Chile. Trees 35, 1663–1672. doi:10.1007/s00468-021-02144-5
Armson, D., Stringer, P., and Ennos, A. R. (2013). The effect of street trees and amenity grass on urban surface water runoff in Manchester, UK. Urban For Urban Green 12, 282–286. doi:10.1016/j.ufug.2013.04.001
Bakhmatova, K. A., Matynyan, N. N., and Sheshukova, A. A. (2022). Anthropogenic soils of urban parks: a review. Eurasian Soil Sci. 55, 64–80. doi:10.1134/S1064229322010021
Baldi, E., Cavani, L., Margon, A., Quartieri, M., Sorrenti, G., Marzadori, C., et al. (2018). Effect of compost application on the dynamics of carbon in a nectarine orchard ecosystem. Sci. Total Environ. 637–638, 918–925. doi:10.1016/j.scitotenv.2018.05.093
Baptista, M. D., Livesley, S. J., G. Parmehr, E., Neave, M., and Amati, M. (2018a). Terrestrial laser scanning to predict canopy area metrics, water storage capacity, and throughfall redistribution in small trees. Remote Sens. (Basel) 10, 1958. doi:10.3390/rs10121958
Baptista, M. D., Livesley, S. J., Parmehr, E. G., Neave, M., and Amati, M. (2018b). Variation in leaf area density drives the rainfall storage capacity of individual urban tree species. Hydrol. Process 32, 3729–3740. doi:10.1002/hyp.13255
Baslam, M., Mitsui, T., Hodges, M., Priesack, E., Herritt, M. T., Aranjuelo, I., et al. (2020). Photosynthesis in a changing global climate: scaling up and scaling down in crops. Front. Plant Sci. 11, 882. doi:10.3389/fpls.2020.00882
Benlouali, H., Harrouni, M. C., Fallah, M., Hirich, A., and Choukr-Allah, R. (2017). Current situation of reclaimed wastewater reuse in golf courses in Marrakech (Morocco): problems and solutions. Desalination Water Treat. 91, 273–280. doi:10.5004/dwt.2017.21567
Bressane, A., Loureiro, A. I. S., Medeiros, L.C. de C., Negri, R. G., and Goulart, A. P. G. (2024). Overcoming barriers to managing urban green spaces in metropolitan areas: prospects from a case study in an emerging economy. Sustainability 16, 7019. doi:10.3390/su16167019
Calvin, K., Dasgupta, D., Krinner, G., Mukherji, A., Thorne, P. W., and Trisos, C. (2023). “IPCC, 2023: Climate Change 2023: Synthesis Report,” in Contribution of working groups I, II and III to the sixth assessment report of the intergovernmental panel on climate change. Editor Core Writing Team, H. Lee, and J. Romero (Geneva, Switzerland: IPCC). doi:10.59327/ipcc/ar6-9789291691647
Campisano, A., Butler, D., Ward, S., Burns, M. J., Friedler, E., DeBusk, K., et al. (2017). Urban rainwater harvesting systems: research, implementation and future perspectives. Water Res. 115, 195–209. doi:10.1016/j.watres.2017.02.056
Ciabattini, M., Rizzello, E., Lucaroni, F., Palombi, L., and Boffetta, P. (2021). Systematic review and meta-analysis of recent high-quality studies on exposure to particulate matter and risk of lung cancer. Environ. Res. 196, 110440. doi:10.1016/j.envres.2020.110440
Deitch, M., Sapundjieff, M., and Feirer, S. (2017). Characterizing precipitation variability and trends in the world’s mediterranean-climate areas. Water (Basel) 9, 259. doi:10.3390/w9040259
De Lucia, B., Cristiano, G., Vecchietti, L., and Bruno, L. (2013). Effect of different rates of composted organic amendment on urban soil properties, growth and nutrient status of three Mediterranean native hedge species. Urban For Urban Green 12, 537–545. doi:10.1016/j.ufug.2013.07.008
Demuzere, M., Orru, K., Heidrich, O., Olazabal, E., Geneletti, D., Orru, H., et al. (2014). Mitigating and adapting to climate change: multi-functional and multi-scale assessment of green urban infrastructure. J. Environ. Manage 146, 107–115. doi:10.1016/j.jenvman.2014.07.025
Dhanya, B., Sathish, B. N., Viswanath, S., and Purushothaman, S. (2014). Ecosystem services of native trees: experiences from two traditional agroforestry systems in Karnataka, Southern India. Int. J. Biodivers. Sci. Ecosyst. Serv. Manag. 10, 101–111. doi:10.1080/21513732.2014.918057
Drius, M., Bongiorni, L., Depellegrin, D., Menegon, S., Pugnetti, A., and Stifter, S. (2019). Tackling challenges for Mediterranean sustainable coastal tourism: an ecosystem service perspective. Sci. Total Environ. 652, 1302–1317. doi:10.1016/j.scitotenv.2018.10.121
Duarte, A., Fernandes, J., Bernardes, J., and Miguel, G. (2016). Citrus as a component of the mediterranean diet. J. Spatial Organ. Dyn. IV, 289–304.
Duckett, D., Troldborg, M., Hendry, S., and Cousin, H. (2024). Making waves: promoting municipal water reuse without a prevailing scarcity driver. Water Res. 249, 120965. doi:10.1016/j.watres.2023.120965
European Committee for Standardization (2018). European standard EN 1694-1 - on-site non-potable water systems - Part 1: systems for the use of rainwater. Brussels, Belgium: CEN.
FAGAR (2023). Relatório & Contas 2023: FAGAR - Faro, gestão de águas e resíduos, E.M. Available at: https://fagar.pt/sites/default/files/documentos/rc2023.pdf (Accessed September 9, 24).
Fan, L., Wang, J., Han, D., Gao, J., and Yao, Y. (2022). Research on promoting carbon sequestration of urban green space distribution characteristics and planting design models in xi’an. Sustainability 15, 572. doi:10.3390/su15010572
Fares, S., Conte, A., Alivernini, A., Chianucci, F., Grotti, M., Zappitelli, I., et al. (2020). Testing removal of carbon dioxide, ozone, and atmospheric particles by urban parks in Italy. Environ. Sci. Technol. 54, 14910–14922. doi:10.1021/acs.est.0c04740
Farreny, R., Gabarrell, X., and Rieradevall, J. (2011). Cost-efficiency of rainwater harvesting strategies in dense Mediterranean neighbourhoods. Resour. Conserv. Recycl 55, 686–694. doi:10.1016/j.resconrec.2011.01.008
Font, A., Tremper, A. H., Lin, C., Priestman, M., Marsh, D., Woods, M., et al. (2020). Air quality in enclosed railway stations: quantifying the impact of diesel trains through deployment of multi-site measurement and random forest modelling. Environ. Pollut. 262, 114284. doi:10.1016/j.envpol.2020.114284
Gain, A. K., Hossain, S., Benson, D., Di Baldassarre, G., Giupponi, C., and Huq, N. (2021). Social-ecological system approaches for water resources management. Int. J. Sustain. Dev. and World Ecol. 28, 109–124. doi:10.1080/13504509.2020.1780647
Gao, K., Santamouris, M., and Feng, J. (2020). On the efficiency of using transpiration cooling to mitigate urban heat. Climate 8, 69. doi:10.3390/cli8060069
Gao, Z., Qin, Y., Yang, X., and Chen, B. (2022). PM10 and PM2.5 dust-retention capacity and leaf morphological characteristics of landscape tree species in the northwest of hebei province. Atmos. (Basel) 13, 1657. doi:10.3390/atmos13101657
Gharibi, S., and Shayesteh, K. (2024). Evaluation of flow, supply, and demand for noise reduction in urban area, Hamadan in Iran. PLoS One 19, e0303581. doi:10.1371/journal.pone.0303581
Graça, M., Alves, P., Gonçalves, J., Nowak, D. J., Hoehn, R., Farinha-Marques, P., et al. (2018). Assessing how green space types affect ecosystem services delivery in Porto, Portugal. Landsc. Urban Plan. 170, 195–208. doi:10.1016/j.landurbplan.2017.10.007
Gratani, L., Catoni, R., Puglielli, G., Varone, L., Crescente, M. F., Sangiorgio, S., et al. (2016). Carbon dioxide (CO2) sequestration and air temperature amelioration provided by urban parks in rome. Energy Procedia 101, 408–415. doi:10.1016/j.egypro.2016.11.052
Grossi, G., Bernini, R., Lacetera, N., Vona, T., Papaleo, F., and Vitali, A. (2024). Carbon footprint associated with two organic waste management: tunnel composting system versus landfill. Int. J. Environ. Sci. Technol. 21, 5499–5512. doi:10.1007/s13762-023-05392-9
Guillen-Cruz, G., Rodríguez-Sánchez, A. L., Fernández-Luqueño, F., and Flores-Rentería, D. (2021). Influence of vegetation type on the ecosystem services provided by urban green areas in an arid zone of northern Mexico. Urban For Urban Green 62, 127135. doi:10.1016/j.ufug.2021.127135
Hamel, P., Hamann, M., Kuiper, J. J., Andersson, E., Arkema, K. K., and Silver, J. M. (2024). Blending ecosystem service and resilience perspectives in planning of natural infrastructure: lessons from the san francisco bay area. Front. Environ. Sci. 9. doi:10.3389/fenvs.2021.601136
Han, F., Yu, J., Zhou, G., Li, S., and Sun, T. (2024). Projected urban flood risk assessment under climate change and urbanization based on an optimized multi-scale geographically weighted regression. Sustain Cities Soc. 112, 105642. doi:10.1016/j.scs.2024.105642
Hernandez Candia, C. N., and Michaelian, K. (2010). Transpiration in plants: a thermodynamic imperative. Nat. Preced. doi:10.1038/npre.2010.5463.1
Hu, Y., and Sun, G. (2010). Leaf nitrogen dioxide uptake coupling apoplastic chemistry, carbon/sulfur assimilation, and plant nitrogen status. Plant Cell Rep. 29, 1069–1077. doi:10.1007/s00299-010-0898-5
Ingrao, C., Strippoli, R., Lagioia, G., and Huisingh, D. (2023). Water scarcity in agriculture: an overview of causes, impacts and approaches for reducing the risks. Heliyon 9, e18507. doi:10.1016/j.heliyon.2023.e18507
Instituto Nacional de Estatística (2024). Estatísticas territoriais. Available at: https://www.ine.pt/xportal/xmain?xpid=INE&xpgid=ine_unid_territorial&menu (Accessed August 20, 24).
Istomina, E. A., Luzhkova, N. M., and Khidekel’, V. V. (2016). Birdwatching tourism infrastructure planning in the Ria Formosa natural park (Portugal). Geogr. Nat. Resour. 37, 371–378. doi:10.1134/S1875372816040120
Jacinto, C., Matias, P., Oliveira, C. M., and Duarte, A. (2024). Effect of heading cuts on branch growth of ‘Encore’ Mandarin. Acta Hortic., 241–246. doi:10.17660/ActaHortic.2024.1399.31
Jiang, Y., Liu, Y., Sun, Y., and Li, X. (2023). Distribution of CO2 concentration and its spatial influencing indices in urban park green space. Forests 14, 1396. doi:10.3390/f14071396
Jonidi Jafari, A., Charkhloo, E., and Pasalari, H. (2021). Urban air pollution control policies and strategies: a systematic review. J. Environ. Health Sci. Eng. 19, 1911–1940. doi:10.1007/s40201-021-00744-4
Jozay, M., Zarei, H., Khorasaninejad, S., and Miri, T. (2024). Maximising CO2 sequestration in the city: the role of green walls in sustainable urban development. Pollutants 4, 91–116. doi:10.3390/pollutants4010007
Kafy, A.Al, Crews, K. A., and Thompson, A. E. (2024). Exploring the cooling potential of green roofs for mitigating diurnal heat island intensity by utilizing Lidar and Artificial Neural Network. Sustain Cities Soc. 116, 105893. doi:10.1016/j.scs.2024.105893
Kamal, A., Mahfouz, A., Sezer, N., Hassan, I. G., Wang, L. L., and Rahman, M. A. (2023). Investigation of urban heat island and climate change and their combined impact on building cooling demand in the hot and humid climate of Qatar. Urban Clim. 52, 101704. doi:10.1016/j.uclim.2023.101704
Kennedy, C. A., Ibrahim, N., and Hoornweg, D. (2014). Low-carbon infrastructure strategies for cities. Nat. Clim. Chang. 4, 343–346. doi:10.1038/nclimate2160
Khalil, M., AlSayed, A., Elsayed, A., Sherif Zaghloul, M., Bell, K. Y., Al-Omari, A., et al. (2024). Advances in GHG emissions modelling for WRRFs: from State-of-the-Art methods to Full-Scale applications. Chem. Eng. J. 494, 153053. doi:10.1016/j.cej.2024.153053
Kumar, P. (2021). Climate change and cities: challenges ahead. Front. Sustain. Cities 3. doi:10.3389/frsc.2021.645613
Lalive, R., Luechinger, S., and Schmutzler, A. (2018). Does expanding regional train service reduce air pollution? J. Environ. Econ. Manage 92, 744–764. doi:10.1016/j.jeem.2017.09.003
Lerman, S. B., and Contosta, A. R. (2019). Lawn mowing frequency and its effects on biogenic and anthropogenic carbon dioxide emissions. Landsc. Urban Plan. 182, 114–123. doi:10.1016/j.landurbplan.2018.10.016
Li, Y., Shao, X., Yu, J., Zhou, C., and Li, H. (2023). Morphological characteristics and water harvesting function of six urban shrub species: implications on sustainable greenspaces in a semi-humid region. Urban Ecosyst. 26, 1155–1168. doi:10.1007/s11252-023-01373-5
Li, Y., Zheng, Q., Yang, R., Zhuang, S., Lin, W., and Li, Y. (2021). Evaluating microbial role in reducing N2O emission by dual isotopocule mapping following substitution of inorganic fertilizer for organic fertilizer. J. Clean. Prod. 326, 129442. doi:10.1016/j.jclepro.2021.129442
Liu, L., and Jensen, M. B. (2018). Green infrastructure for sustainable urban water management: practices of five forerunner cities. Cities 74, 126–133. doi:10.1016/j.cities.2017.11.013
Lu, S., Yang, X., Li, S., Chen, B., Jiang, Y., Wang, D., et al. (2018). Effects of plant leaf surface and different pollution levels on PM2.5 adsorption capacity. Urban For Urban Green 34, 64–70. doi:10.1016/j.ufug.2018.05.006
Lupia, F., Baiocchi, V., Lelo, K., and Pulighe, G. (2017). Exploring rooftop rainwater harvesting potential for food production in urban areas. Agriculture 7, 46. doi:10.3390/agriculture7060046
Ma, C., Yao, Q., Meng, H., Zhou, B., Wang, Q., and Luo, Y. (2022). Rainfall partitioning by evergreen and deciduous broad-leaved xerophytic tree species: influence of rainfall, canopy characteristics, and meteorology. Water (Basel) 14, 3723. doi:10.3390/w14223723
Ma, Y., Lauwaet, D., Kouti, A., and Verbeke, S. (2023). A toolchain to evaluate the impact of urban heat island and climate change on summer overheating at district level. Urban Clim. 51, 101602. doi:10.1016/j.uclim.2023.101602
Manisalidis, I., Stavropoulou, E., Stavropoulos, A., and Bezirtzoglou, E. (2020). Environmental and health impacts of air pollution: a review. Front. Public Health 8, 14. doi:10.3389/fpubh.2020.00014
Marcantonio, R., Javeline, D., Field, S., and Fuentes, A. (2021). Global distribution and coincidence of pollution, climate impacts, and health risk in the Anthropocene. PLoS One 16, e0254060. doi:10.1371/journal.pone.0254060
Marteleira, R., Pinto, G., and Niza, S. (2014). Regional water flows – assessing opportunities for sustainable management. Resour. Conserv. Recycl 82, 63–74. doi:10.1016/j.resconrec.2013.10.016
Martin, L. M., Polley, H. W., Daneshgar, P. P., Harris, M. A., and Wilsey, B. J. (2014). Biodiversity, photosynthetic mode, and ecosystem services differ between native and novel ecosystems. Oecologia 175, 687–697. doi:10.1007/s00442-014-2911-0
Matias, P., Barrote, I., Azinheira, G., Continella, A., and Duarte, A. (2023). Citrus pruning in the Mediterranean climate: a review. Plants 12, 3360. doi:10.3390/plants12193360
Matias, P., Coelho, L., and Reis, M. (2024). Efficacy of slow sand filtration enriched with Trichoderma atroviride in the control of Rhizoctonia solani in soilless culture. Crop Prot. 186, 106917. doi:10.1016/j.cropro.2024.106917
Matias, P., Duarte, B., and Duarte, A. (2021). Citrinos na dieta mediterrânica: frutos com sumo e com história. Rev. Assoc. Port. Hortic., 39–41.
Mendoza Beltran, A., Jepsen, K., Rufí-Salís, M., Ventura, S., Madrid Lopez, C., and Villalba, G. (2022). Mapping direct N2O emissions from peri-urban agriculture: the case of the Metropolitan Area of Barcelona. Sci. Total Environ. 822, 153514. doi:10.1016/j.scitotenv.2022.153514
Mentens, J., Raes, D., and Hermy, M. (2006). Green roofs as a tool for solving the rainwater runoff problem in the urbanized 21st century? Landsc. Urban Plan. 77, 217–226. doi:10.1016/j.landurbplan.2005.02.010
Molénat, J., Barkaoui, K., Benyoussef, S., Mekki, I., Zitouna, R., and Jacob, F. (2023). Diversification from field to landscape to adapt Mediterranean rainfed agriculture to water scarcity in climate change context. Curr. Opin. Environ. Sustain 65, 101336. doi:10.1016/j.cosust.2023.101336
Moreira da Silva, M., Ferreira, L., Sarmento, T., and Selada, C. (2024). Engaging young people in the development of innovative nature-inspired technologies for carbon sequestration in cities: case studies from Portugal. Smart Cities 7, 445–459. doi:10.3390/smartcities7010017
Moreira da Silva, M., Resende, F. C., Freitas, B., Aníbal, J., Martins, A., and Duarte, A. (2022). Urban wastewater reuse for citrus irrigation in Algarve, Portugal—environmental benefits and carbon fluxes. Sustainability 14, 10715. doi:10.3390/su141710715
Muvengwi, J., Ndagurwa, H. G. T., Witkowski, E. T. F., and Mbiba, M. (2024). Woody species composition, diversity, and ecosystem services of yards along an urban socioeconomic gradient. Sci. Total Environ. 912, 168976. doi:10.1016/j.scitotenv.2023.168976
Nero, B. F., Kuusaana, E. D., Ahmed, A., and Campion, B. B. (2024). Carbon storage and tree species diversity of urban parks in Kumasi, Ghana. City Environ. Interact. 24, 100156. doi:10.1016/j.cacint.2024.100156
Nordahl, S. L., Preble, C. V., Kirchstetter, T. W., and Scown, C. D. (2023). Greenhouse gas and air pollutant emissions from composting. Environ. Sci. Technol. 57, 2235–2247. doi:10.1021/acs.est.2c05846
Norton, B. A., Bending, G. D., Clark, R., Corstanje, R., Dunnett, N., Evans, K. L., et al. (2019). Urban meadows as an alternative to short mown grassland: effects of composition and height on biodiversity. Ecol. Appl. 29, e01946. doi:10.1002/eap.1946
Nowak, D. J. (2023). “Improved air quality and other services from urban trees and forests,” in Engineering and ecosystems (Cham: Springer International Publishing), 215–245. doi:10.1007/978-3-031-35692-6_10
Nunes, L. J. R., Meireles, C. I. R., Gomes, C. J. P., and Ribeiro, N. M. C. A. (2020). Forest contribution to climate change mitigation: management oriented to carbon capture and storage. Climate 8, 21. doi:10.3390/cli8020021
Oldfield, E. E., Felson, A. J., Wood, S. A., Hallett, R. A., Strickland, M. S., and Bradford, M. A. (2014). Positive effects of afforestation efforts on the health of urban soils. For Ecol Manage 313, 266–273. doi:10.1016/j.foreco.2013.11.027
Oosterbroek, B., de Kraker, J., Huynen, M. M. T. E., and Martens, P. (2024). Integrated assessment of health benefits and burdens of urban greenspace designs. Sustainability 16, 7534. doi:10.3390/su16177534
Ortuño, A., Hernández, M., and Civera, S. (2015). Golf course irrigation and self-sufficiency water in Southern Spain. Land use policy 44, 10–18. doi:10.1016/j.landusepol.2014.11.020
Pan, H., Page, J., Cong, C., Barthel, S., and Kalantari, Z. (2021). How ecosystems services drive urban growth: integrating nature-based solutions. Anthropocene 35, 100297. doi:10.1016/j.ancene.2021.100297
Paudel, S., and States, S. L. (2023). Urban green spaces and sustainability: exploring the ecosystem services and disservices of grassy lawns versus floral meadows. Urban For Urban Green 84, 127932. doi:10.1016/j.ufug.2023.127932
Poch, M., Aldao, C., Godo-Pla, L., Monclús, H., Popartan, L. A., Comas, J., et al. (2023). Increasing resilience through nudges in the urban water cycle: an integrative conceptual framework to support policy decision-making. Chemosphere 317, 137850. doi:10.1016/j.chemosphere.2023.137850
PORDATA (2024). Ambiente: Precipitação e temperatura. Available at: https://www.pordata.pt/pt/documentos-indicadores (Accessed October 31, 24).
QUALAR (2024). Informação sobre a qualidade do ar. Available at: https://qualar.apambiente.pt/downloads (Accessed October 31, 24).
Quaranta, E., Dorati, C., and Pistocchi, A. (2021). Water, energy and climate benefits of urban greening throughout Europe under different climatic scenarios. Sci. Rep. 11, 12163. doi:10.1038/s41598-021-88141-7
Ranawana, S. R. W. M. C. J. K., Bramley, H., Palta, J. A., and Siddique, K. H. M. (2023). “Role of transpiration in regulating leaf temperature and its application in physiological breeding,” in Translating physiological tools to augment crop breeding (Singapore: Springer Nature Singapore), 91–119. doi:10.1007/978-981-19-7498-4_5
Reyes-Paecke, S., Gironás, J., Melo, O., Vicuña, S., and Herrera, J. (2019). Irrigation of green spaces and residential gardens in a Mediterranean metropolis: gaps and opportunities for climate change adaptation. Landsc. Urban Plan. 182, 34–43. doi:10.1016/j.landurbplan.2018.10.006
Riley, C. B., Herms, D. A., and Gardiner, M. M. (2018). Exotic trees contribute to urban forest diversity and ecosystem services in inner-city Cleveland, OH. Urban For Urban Green 29, 367–376. doi:10.1016/j.ufug.2017.01.004
Rossiello, M. R., and Szema, A. (2019). Health effects of climate change-induced wildfires and heatwaves. Cureus 11, e4771. doi:10.7759/cureus.4771
Sæbø, A., Popek, R., Nawrot, B., Hanslin, H. M., Gawronska, H., and Gawronski, S. W. (2012). Plant species differences in particulate matter accumulation on leaf surfaces. Sci. Total Environ. 427–428, 347–354. doi:10.1016/j.scitotenv.2012.03.084
Shadman, S., Ahanaf Khalid, P., Hanafiah, M. M., Koyande, A. K., Islam, Md.A., Bhuiyan, S. A., et al. (2022). The carbon sequestration potential of urban public parks of densely populated cities to improve environmental sustainability. Sustain. Energy Technol. Assessments 52, 102064. doi:10.1016/j.seta.2022.102064
Shamsipour, A., Heidari, H., and Avatefi, R. (2024). Estimating of the climate effects of urban gardens with an emphasis on carbon sequestration in Shiraz, Iran. Int. J. Environ. Sci. Technol. 21, 1379–1388. doi:10.1007/s13762-023-05334-5
Shang, B., Feng, Z., Peng, J., Xu, Y., and Paoletti, E. (2021). Effects of elevated ozone and nitrogen addition on leaf nitrogen metabolism in poplar. J. Plant Ecol. 14, 555–568. doi:10.1093/jpe/rtab010
Sicard, P., Khaniabadi, Y. O., Perez, S., Gualtieri, M., and De Marco, A. (2019). Effect of O3, PM10 and PM2.5 on cardiovascular and respiratory diseases in cities of France, Iran and Italy. Environ. Sci. Pollut. Res. 26, 32645–32665. doi:10.1007/s11356-019-06445-8
Smith, I. A., Templer, P. H., and Hutyra, L. R. (2024). Water sources for street trees in mesic urban environments. Sci. Total Environ. 908, 168411. doi:10.1016/j.scitotenv.2023.168411
Snehlata, , Rajlaxmi, A., and Kumar, M. (2021). Urban tree carbon density and CO2 equivalent of National Zoological Park, Delhi. Environ. Monit. Assess. 193, 841. doi:10.1007/s10661-021-09619-5
Soares, P. M. M., Cardoso, R. M., Ferreira, J. J., and Miranda, P. M. A. (2015). Climate change and the Portuguese precipitation: ENSEMBLES regional climate models results. Clim. Dyn. 45, 1771–1787. doi:10.1007/s00382-014-2432-x
Soares, P. M. M., and Lima, D. C. A. (2022). Water scarcity down to earth surface in a Mediterranean climate: the extreme future of soil moisture in Portugal. J. Hydrol. (Amst) 615, 128731. doi:10.1016/j.jhydrol.2022.128731
Speak, A. F., and Salbitano, F. (2023). The impact of pruning and mortality on urban tree canopy volume. Urban For Urban Green 79, 127810. doi:10.1016/j.ufug.2022.127810
Spotswood, E. N., Beller, E. E., Grossinger, R., Grenier, J. L., Heller, N. E., and Aronson, M. F. J. (2021). The biological deserts fallacy: cities in their landscapes contribute more than we think to regional biodiversity. Bioscience 71, 148–160. doi:10.1093/biosci/biaa155
Stanišić, S., and Stojić, A. (2020). Urban air pollution and environmental health, 1–8. doi:10.1007/978-3-319-71061-7_120-1
Tremblay, D., Fortier, F., Boucher, J., Riffon, O., and Villeneuve, C. (2020). Sustainable development goal interactions: an analysis based on the five pillars of the 2030 agenda. Sustain. Dev. 28, 1584–1596. doi:10.1002/sd.2107
UNEP (2022). United Nations environment program. Annual report 2022. Available at: https://www.unep.org/resources/annual-report-2022 (Accessed August 14, 24).
Varshney, K., Pedersen Zari, M., and Bakshi, N. (2022). “Carbon sequestration through building-integrated vegetation,” in The palgrave encyclopedia of urban and regional futures (Cham: Springer International Publishing), 1–9. doi:10.1007/978-3-030-51812-7_319-1
Veloso, N., Cruz, P., Carvalho, H., and Moreira da Silva, M. (2015). Monitoring urban storm water: facing climate changes in a Mediterranean coastal city. 1, 93–103. doi:10.2495/SC150091
Vigevani, I., Corsini, D., Mori, J., Pasquinelli, A., Gibin, M., Comin, S., et al. (2022). Particulate pollution capture by seventeen woody species growing in parks or along roads in two European cities. Sustainability 14, 1113. doi:10.3390/su14031113
Wang, C., Li, Z., Chen, Y., Ouyang, L., Zhao, H., Zhu, J., et al. (2024). Characteristic changes in compound drought and heatwave events under climate change. Atmos. Res. 305, 107440. doi:10.1016/j.atmosres.2024.107440
Wang, Y., Chang, Q., and Li, X. (2021). Promoting sustainable carbon sequestration of plants in urban greenspace by planting design: a case study in parks of Beijing. Urban For Urban Green 64, 127291. doi:10.1016/j.ufug.2021.127291
Wang, Y., Desha, C., Caldera, S., and Beer, T. (2024). Roles of urban green spaces for children in high-density metropolitan areas during pandemics: a systematic literature review. Sustainability 16, 988. doi:10.3390/su16030988
Wang, Y., Jin, W., Che, Y., Huang, D., Wang, J., Zhao, M., et al. (2019). Atmospheric nitrogen dioxide improves photosynthesis in mulberry leaves via effective utilization of excess absorbed light energy. Forests 10, 312. doi:10.3390/f10040312
Wang, L., Huang, Z., Gan, B., Zhang, Z., Fu, H., Fang, D., et al. (2024). Climate change impacts on magnitude and frequency of urban floods under scenario and model uncertainties. J. Environ. Manage 366, 121679. doi:10.1016/j.jenvman.2024.121679
Wei, T., Wu, J., and Chen, S. (2021). Keeping track of greenhouse gas emission reduction progress and targets in 167 cities worldwide. Front. Sustain. Cities 3. doi:10.3389/frsc.2021.696381
Wei, Y., Amini, H., Qiu, X., Castro, E., Jin, T., Yin, K., et al. (2024). Grouped mixtures of air pollutants and seasonal temperature anomalies and cardiovascular hospitalizations among U.S. Residents. Environ. Int. 187, 108651. doi:10.1016/j.envint.2024.108651
Weyens, N., Thijs, S., Popek, R., Witters, N., Przybysz, A., Espenshade, J., et al. (2015). The role of plant–microbe interactions and their exploitation for phytoremediation of air pollutants. Int. J. Mol. Sci. 16, 25576–25604. doi:10.3390/ijms161025576
Willaarts, B. A., Novais, M. H., González Navarro, J. M., López Lara, G., Morais, M., Zilliox, E., et al. (2024). The governance of the water-energy nexus: Co-produced narratives to take stock and address energy dependencies of the urban water cycle in Atlantic Europe. Environ. Sci. Policy 160, 103835. doi:10.1016/j.envsci.2024.103835
Winbourne, J. B., Jones, T. S., Garvey, S. M., Harrison, J. L., Wang, L., Li, D., et al. (2020). Tree transpiration and urban temperatures: current understanding, implications, and future research directions. Bioscience 70, 576–588. doi:10.1093/biosci/biaa055
World Health Organization (2023). Water safety plan manual: step-by-step risk management for drinking-water suppliers. 2nd ed. Geneva, Switzerland: World Health Organization (WHO). Available at: https://www.who.int/publications/i/item/9789240067691.
Xiao, Y., Liu, Y., and Cai, W. (2024). Spatial and temporal evolution and drivers of GHG emissions from urban domestic wastewater treatment in China: a review at the provincial level. Environ. Sci. Pollut. Res. 31, 21028–21043. doi:10.1007/s11356-024-32358-2
Xie, Q., Yue, Y., Sun, Q., Chen, S., Lee, S.-B., and Kim, S. W. (2019). Assessment of ecosystem service values of urban parks in improving air quality: a case study of wuhan, China. Sustainability 11, 6519. doi:10.3390/su11226519
Yadav, V., Yadav, A. K., Singh, V., and Singh, T. (2024). Artificial neural network an innovative approach in air pollutant prediction for environmental applications: a review. Results Eng. 22, 102305. doi:10.1016/j.rineng.2024.102305
Yang, B., Lee, D. K., Heo, H. K., and Biging, G. (2019). The effects of tree characteristics on rainfall interception in urban areas. Landsc. Ecol. Eng. 15, 289–296. doi:10.1007/s11355-019-00383-w
Yang, H., Guo, Y., Fang, N., Dong, B., and Wu, X. (2024). Greenhouse gas emissions of sewage sludge land application in urban green space: a field experiment in a Bermuda grassland. Sci. Total Environ. 926, 172106. doi:10.1016/j.scitotenv.2024.172106
Yang, L., Li, Y., Peng, K., and Wu, S. (2014). Nutrients and heavy metals in urban soils under different green space types in Anji, China. Catena (Amst) 115, 39–46. doi:10.1016/j.catena.2013.11.008
Yasmin, N., Jamuda, M., Panda, A. K., Samal, K., and Nayak, J. K. (2022). Emission of greenhouse gases (GHGs) during composting and vermicomposting: measurement, mitigation, and perspectives. Energy Nexus 7, 100092. doi:10.1016/j.nexus.2022.100092
Yeom, S., and Ahn, J. (2024). An experimental study of the retention effect of urban drainage systems in response to grate inlet clogging. Sustainability 16, 7596. doi:10.3390/su16177596
Yousofpour, Y., Abolhassani, L., Hirabayashi, S., Burgess, D., Sabouhi Sabouni, M., and Daneshvarkakhki, M. (2024). Ecosystem services and economic values provided by urban park trees in the air polluted city of Mashhad. Sustain Cities Soc. 101, 105110. doi:10.1016/j.scs.2023.105110
Zhao, R., and Zhiwen, Z. (2024). Enhancing streamflow prediction in the Wujiang River basin: a two-stage decomposition approach with deep learning integration. J. Water Clim. Change 15, 5683–5697. doi:10.2166/wcc.2024.599
Keywords: ecosystem services, urban trees, urban water cycle, carbon sequestration, air pollutants, rainwater harvesting, community engagement
Citation: Matias P, Moreira da Silva M, Teigão J and Duarte A (2025) Urban vegetation benefits in mediterranean cities for climate change adaptation and water usage efficiency – a case study in Algarve, Portugal. Front. Environ. Sci. 13:1520934. doi: 10.3389/fenvs.2025.1520934
Received: 31 October 2024; Accepted: 21 January 2025;
Published: 06 February 2025.
Edited by:
Cristina Matos, University of Trás-os-Montes and Alto Douro, PortugalReviewed by:
Bhuvan Vemuri, Micron, United StatesCopyright © 2025 Matias, Moreira da Silva, Teigão and Duarte. This is an open-access article distributed under the terms of the Creative Commons Attribution License (CC BY). The use, distribution or reproduction in other forums is permitted, provided the original author(s) and the copyright owner(s) are credited and that the original publication in this journal is cited, in accordance with accepted academic practice. No use, distribution or reproduction is permitted which does not comply with these terms.
*Correspondence: Manuela Moreira da Silva, bXNhbnRpQHVhbGcucHQ=
Disclaimer: All claims expressed in this article are solely those of the authors and do not necessarily represent those of their affiliated organizations, or those of the publisher, the editors and the reviewers. Any product that may be evaluated in this article or claim that may be made by its manufacturer is not guaranteed or endorsed by the publisher.
Research integrity at Frontiers
Learn more about the work of our research integrity team to safeguard the quality of each article we publish.