- African Sustainable Agriculture Research Institute (ASARI), Mohammed VI Polytechnic University (UM6P), Laayoune, Morocco
Phosphate mining is essential for global agriculture but poses significant environmental challenges, particularly in landscape degradation. This study reviews the current state and trends of phosphate mining, highlighting economic importance, environmental impacts, and sustainability issues. It evaluates restoration techniques, focusing on the effectiveness of various plant species in restoring mined areas. Key factors influencing successful rehabilitation include topsoil management, physico-chemical site characteristics, nutrient bioavailability, topography, and water availability. The study advocates for integrated land management strategies that combine tailored nutrient management plans, erosion control, and water conservation, particularly in arid climates. Strengthening and enforcing regulatory frameworks is crucial to ensure accountability in land rehabilitation, with a focus on sustainability. Recent legal advancements in Morocco aim to modernize mining operations and enhance environmental accountability. The findings recommend increased investment in research to develop innovative rehabilitation techniques and long-term monitoring programs to ensure adaptive management and continuous improvement of restoration outcomes.
1 Rationale and objectives
The rationale for this review stems from the critical need to balance the economic importance of phosphate mining with its environmental consequences, particularly landscape degradation. Phosphate is indispensable for global agriculture, serving as a key component in fertilizers that sustain food production. However, the environmental impacts, especially in regions like Morocco where phosphate mining is extensive, are profound and multifaceted. The degradation of mined lands presents significant ecological and socio-economic challenges, necessitating effective rehabilitation strategies.
Given the growing global emphasis on sustainability and environmental stewardship, there is an urgent need to assess and improve current rehabilitation practices. This review is motivated by the recognition that while phosphate mining is vital for agricultural productivity, its long-term sustainability depends on the successful restoration of mined landscapes. Additionally, the review addresses the lack of comprehensive assessments that integrate various rehabilitation techniques, environmental factors, and socio-economic considerations, particularly in the context of phosphate mining in Morocco.
The objective of this review is to provide a comprehensive evaluation of the current state and trends in phosphate mining, with a specific focus on the environmental impacts and rehabilitation strategies employed globally and in Morocco. The review aims to:
• Assess the effectiveness of various rehabilitation techniques; including revegetation, reclamation, and remediation, with a particular focus on plant species suited for restoring mined areas.
• Identify key factors influencing the success of rehabilitation efforts, such as topsoil management, site characteristics, nutrient availability, topography, and water resources.
• Examine the socio-economic dimensions of mining rehabilitation, emphasizing the role of community engagement and the impact of legal and regulatory frameworks.
• Highlight recent legal advancements in Morocco aimed at modernizing mining operations and enhancing environmental accountability.
• Advocate for integrated restoration strategies that balance ecological resilience with operational efficiency, contributing to the creation of sustainable post-mining landscapes.
This review ultimately seeks to contribute to the ongoing discourse on sustainable mining practices, offering insights that can inform both policy and practice in the rehabilitation of phosphate mining lands. We explored 132 sources from the literature, of which 77% are indexed scientific papers. Figure 1 shows a clear tendency of the publications repartition over time, with 52% (69 out of 102) of the articles published withing the 2011-2024 period. This reveals a growing interest for the restoration of degraded lands, especially those resulting from phosphate mining activities.
2 Status of phosphate mining in the world
The contemporary landscape of global phosphate mining is marked by its crucial role in national economies and the associated environmental challenges. While contributing significantly to the GDP of many nations, phosphate mining raises environmental concerns, with discernible alterations to natural landscapes observed across extraction, processing, transportation, and smelting processes (Matschullat and Gutzmer, 2012). By 1974, the global production of phosphate rock (PR) reached 110 million metric tons, with the United States, USSR, and Morocco accounting for 75% of the total, prompting questions about the sustainability of these patterns. Forecasts suggest potential shifts in production shares for Morocco and the USSR, with Australia emerging as a significant PR producer. The United States is also anticipated to potentially transition into a net importer of PR in the 21st century, highlighting evolving international trade dynamics (Van Kauwenbergh, 2010).
The complexity of phosphate mining is further accentuated by challenges associated with the primary phosphorus source—phosphate rock. Non-renewability, high extraction costs, and depletion concerns characterize this essential resource, with predictions indicating depletion within 50–100 years at current extraction rates. The allocation of 82% of mined phosphorus to agriculture underscores its indispensable role in critical sectors (Cieślik and Konieczka, 2017). To address dependency on the global phosphate rock market, initiatives like phosphorus recovery from mining wastewater emerge as viable solutions. Phosphate, a key resource mined globally, is influenced by geopolitical dynamics, emphasizing the interconnectedness of global trade and resource dynamics among major producing countries such as China, Russia, the USA, and Morocco (Cieślik and Konieczka, 2017).
According to the United States Geological Survey (USGS, 2023; 2024a), China is currently the world’s largest producer of phosphate rock, followed by Morocco, which holds the largest global reserves (Figure 2).
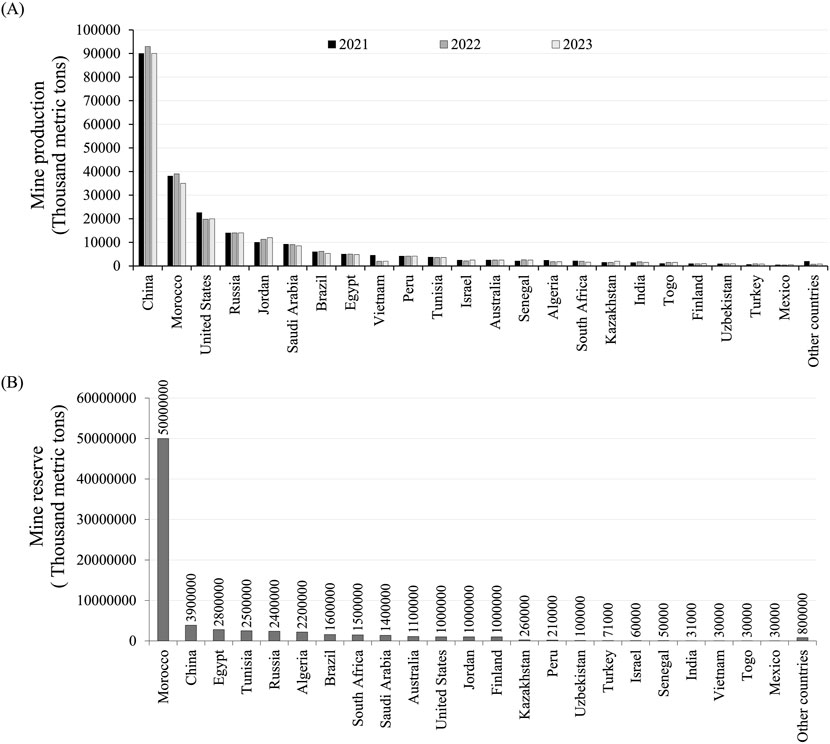
Figure 2. World rock phosphate production (A) (USGS, 2023; USGS, 2024a) and reserve (B) (USGS, 2024b).
Worldwide, phosphate rock resources, primarily found as sedimentary marine phosphorites, are concentrated in northern Africa, the Middle East, China, and the United States, with additional significant occurrences in Brazil, Canada, Finland, Russia, and South Africa. Substantial resources are also identified on continental shelves and seamounts in the Atlantic and Pacific Oceans, collectively surpassing 300 billion tons and emphasizing the vast reservoirs available for extraction. Importantly, current assessments, supported by USGS (2024a) and Cisse and Mrabet (2004), indicate the absence of imminent shortages in phosphate rock, affirming the robustness of global reserves to meet sustained demand.
3 Status of phosphate mining in Morocco
Morocco holds a dominant position in the global phosphate market, commanding 30% of market share, with significant sedimentary rock reserves (USGS, 2023). The mining activities of the Office Chérifien des Phosphates (OCP Group), operating in the regions of Khouribga, Gantour, and Boucraâ, encompass the extraction, processing, enrichment, and delivery of phosphates to over thirty countries. These activities are organized into three main production region: the North production region, consisting of the Khouribga mining site and the Jorf Lasfar chemical site, connected by a slurry pipeline; the Central production region, including the Gantour basin (Benguerir and Youssoufia regions in Morocco) and the chemical site of Safi; and the South production region, comprising the Phosboucraâ mining site (OCP, 2022a).
Phosphate reserves are distributed across the Khouribga, Youssoufia, Boucraâ, and Essaouira basins. Estimates indicate that Khouribga’s reserves represent about 43% of the total for the Group, while those of Gantour, Meskala, and Boucraâ represent approximately 37%, 18%, and 2%, respectively (OCP, 2021). According to Benjelloun and Saoud (2021) and USGS (2023), Morocco holds more than 70% of the world’s phosphate reserves, around 50,000 thousand million tons, and the OCP Group estimates that these economically exploitable reserves equate to several centuries of global consumption at current levels. The map of the main deployment sites in Morocco is presented in Figure 3.
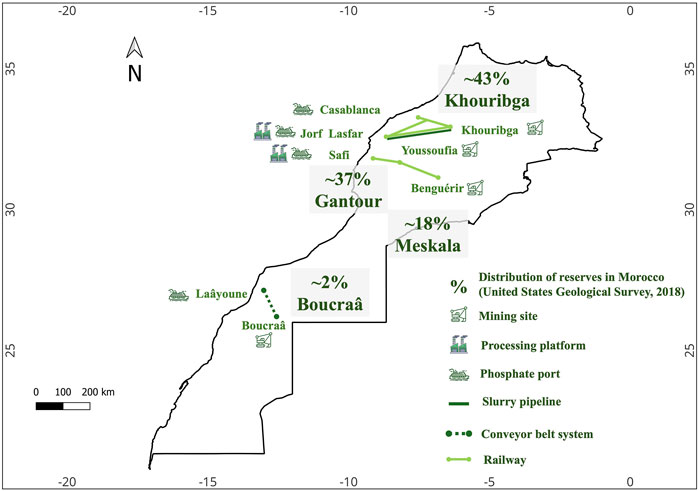
Figure 3. Main phosphate production and processing sites in Morocco adapted from OCP (2021).
According to USGS (2024b), there is an annual upswing in mining output, as illustrated in Figure 4. This elevation is attributed to a surge in demand both domestically and internationally.
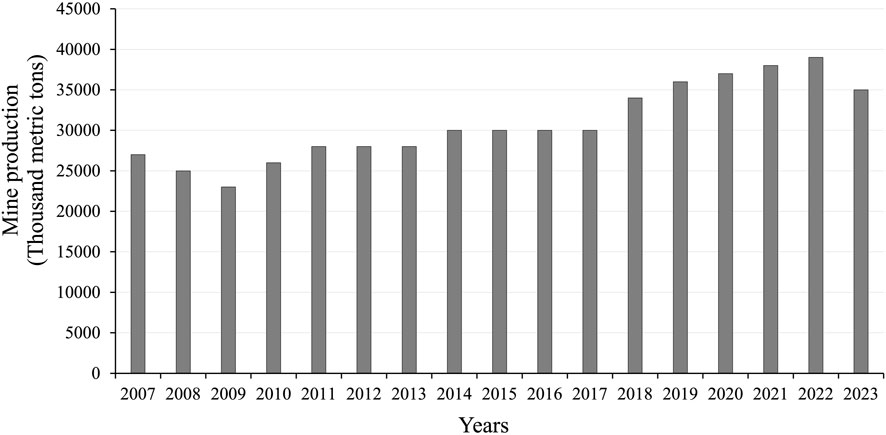
Figure 4. Mine production in Morocco between 2007 and 2023 (USGS, 2024a).
4 Restoration strategies of phosphate mining sites
Phosphate mining, a crucial industry for global agriculture, often leaves lasting impacts on landscapes. In the pursuit of mitigating these effects, restoration becomes imperative, encompassing several key terms and concepts including revegetation, rehabilitation, reclamation, and remediation. This article examines these terms, exploring their definitions and highlighting their significance in the broader context of environmental restoration efforts following phosphate-mining operations.
4.1 Revegetation: cultivating life amidst desolation
Revegetation emerges as a fundamental restorative strategy for phosphate mining sites (Bradshaw, 1996). This multifaceted approach involves the deliberate reintroduction of robust botanical life to areas adversely impacted by mining activities. Indigenous vegetation or seeds are discerningly introduced, facilitating the establishment of resilient and diverse plant species. This process not only serves to stabilize susceptible soil but also acts as a preventative measure against erosion, ultimately revitalizing the previously degraded terrain (Bradshaw, 1996; Zine et al., 2018). Revegetation, an integral facet of the restoration paradigm, denotes the intentional reintroduction of plant life to erstwhile mined or disturbed lands, with the overarching objective of reinstating a sustainable ecosystem (Hobbs and Harris, 2001). Generally, revegetation helps to stabilize soils, enhance biodiversity, and restore the aesthetic value of previously mined areas.
4.2 Rehabilitation: nurturing ecosystem vitality
In ecological restoration, rehabilitation, highlighted by Bradshaw (1996), complements revegetation. Unlike the latter’s focus on replicating the original landscape, rehabilitation prioritizes improving soil quality and crafting habitats tailored to native species’ needs. This multifaceted strategy revitalizes ecosystem functionality, ensuring renewed vitality in harmony with surrounding biodiversity. From a managerial standpoint, rehabilitation is a term within the purview of assessing the economic and environmental considerations associated with maintaining ecological quality and optimizing local land management capabilities. While sharing affinities with the concept of restoration, rehabilitation uniquely prioritizes “soil rehabilitation” and site decontamination as its principal objectives (Lima et al., 2016).
4.3 Reclamation: transforming depletion into productivity
Reclamation is centred on the metamorphosis of degraded lands into a functional state, typically for advantageous land uses such as agriculture or forestry, with the overarching goal of augmenting the land’s overall productivity and value (Gerwing et al., 2022). As a broader concept within the field of restoration, reclamation focuses on the geotechnical stabilization of land through coordinated operations. It envisions the conversion of depleted and discarded mining sites into productive landscapes. Soil, once infertile and abandoned, undergoes revitalization to sustain diverse agricultural crops or resilient forests. This transformative process reshapes the terrestrial environment, providing newfound potential and opportunities for sustainable land use (Bradshaw, 1996). Generally, the main goal of reclamation is to augment the land’s overall productivity and ecological value, thereby enhancing the sustainability and utility of these previously degraded areas. This multidisciplinary approach combines principles of soil science, hydrology, engineering, and ecosystem management to achieve a comprehensive restoration of the land’s physical, chemical, and biological integrity (Feng et al., 2023).
4.4 Remediation: addressing environmental contamination
Simultaneously, within the broader context of environmental renewal, remediation plays a crucial role. This process identifies and neutralizes hazardous substances and pollutants stemming from mining activities. The meticulous endeavour aims to cleanse contaminated soils and water sources, safeguarding ecosystems and human health. Employing diverse techniques, remediation ensures the comprehensive “cleaning up” of the site (Lima et al., 2016).
Together, these terms constitute a comprehensive framework for the restoration of phosphate mining sites, integrating ecological, social, and economic considerations to achieve sustainable outcomes. Figure 5 presents an overview of the post-mining restoration processes. Ireland applied this R4 criteria to a peat mine and found it successful (Lima et al., 2016).
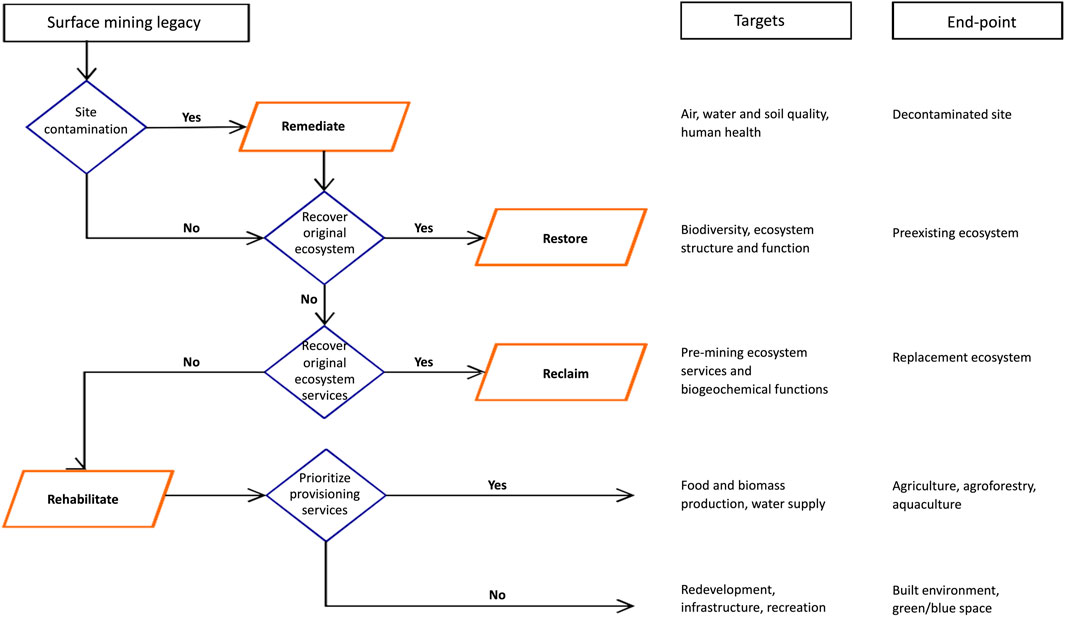
Figure 5. Proposed R4 decision tree based on targets and end-goals for post mining recovery (Lima et al., 2016).
5 Status and progress of restoration of phosphate mining sites in Morocco
Morocco’s pivotal role as the world’s second-largest phosphate producer is accentuated by the OCP Group’s initiatives in soil rehabilitation, showcasing a steadfast commitment to sustainable development through topsoil preservation and the enhancement of agricultural practices for socio-economic advantages (Annual Report of the Court of Audit, 2018; OCP, 2022). Since 2013, OCP has actively pursued environmental excellence, adhering to rigorous international standards and undertaking the rehabilitation of 3870 ha of land. This commitment persists, as evidenced by plans to double annual land rehabilitation efforts and collaborate with the Mohammed VI Polytechnic University for a comprehensive land rehabilitation strategy (Annual Report of the Court of Audit, 2018).
At the Gantour and Khouribga sites, the OCP Group endeavours to annually rehabilitate 1,000 ha, twice the area utilized for phosphate extraction (Annual Report of the Court of Audit, 2018). Figure 6 shows the area under rehabilitation of mining sites each year since 2018, while Figure 7 depicts the evolution of the planted area over time (OCP, 2020; OCP, 2022a). By the end of 2020, OCP had successfully planted 4.5 million trees across 4,500 ha of former mining areas, witnessing the flourishing of hundreds of thousands of olive and argan trees on once-abandoned sites (OCP, 2022).
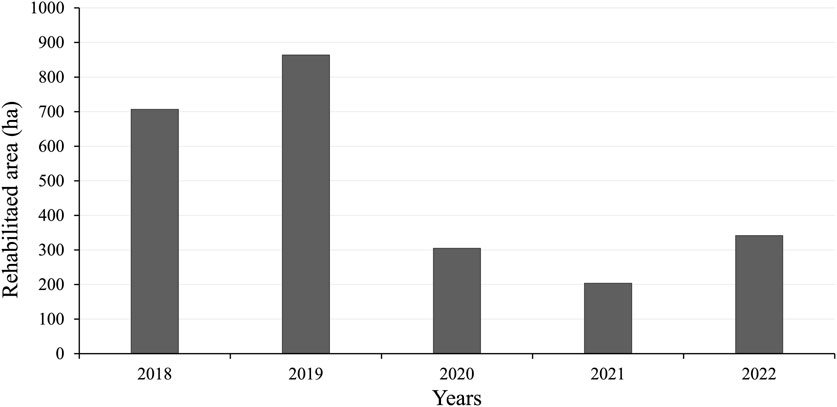
Figure 6. Evolution of rehabilitated phosphate mined land area in Morocco (OCP S.a, 2022).
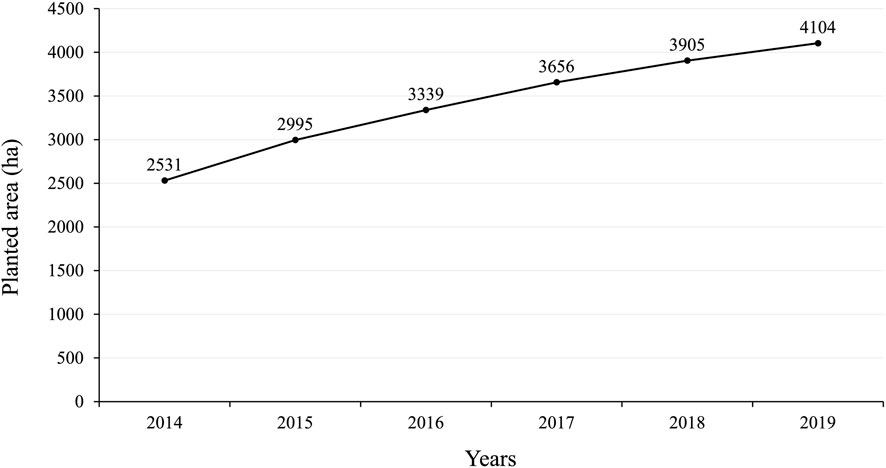
Figure 7. Evolution of planted phosphate mined area in Morocco (ha) (OCP, 2020).
The OCP Group has further demonstrated its commitment through the release of 152 million USD for the “Green Mine” project which is part of a sustainable rehabilitation of an old mining site extending over an area of 294 ha inside the urban perimeter of Khouribga. This extensive rehabilitation initiative consists of the establishment of a sociocultural complex, a residential and tourist complex, alongside multidisciplinary sports fields and extreme sports facilities (Donsimoni and Labaronne, 2014). A wastewater treatment plant is incorporated to process and recycle wastewater for optimized irrigation (Benjelloun and Saoud, 2021). Simultaneously, a central library and mall, housing offices and businesses, contribute to the economic development and cultural vibrancy of the city (Donsimoni and Labaronne, 2014).
6 Gaps in restoration research regarding phosphate mined areas in Morocco
Gaps in restoration research concerning phosphate-mined areas in Morocco are discernible across various dimensions, as revealed by an analysis of relevant literatures. The first gap relates to the exploration of synergistic solutions, specifically the interaction between iron and potassium oxide, aiming at mitigating heavy metal levels in agriculture. While studies like those conducted by Swift et al. (2016) thoroughly explore the alterations in land use post-mining, there remains a conspicuous gap in comprehensive investigations concerning the enduring success of restoration endeavours (Hobbs and Harris, 2001).
The translocation and absorption of nutrients in plants cultivated in the presence of high phosphorus (P) content and heavy metals on phosphate mining lands represent significant gaps in current research. High levels of phosphorus can lead to nutrient imbalances and affect the uptake of other essential nutrients by plants, potentially impacting their growth and development (Sabiha-Javied et al., 2023). Additionally, the presence of heavy metals in phosphate mining areas can further exacerbate these issues, as they may interfere with nutrient absorption mechanisms in plant roots and disrupt physiological processes (Sandeep et al., 2019). Research addressing these gaps is essential for understanding the complex interactions between phosphorus, heavy metals, and nutrient uptake in plants (Gupta et al., 2014), thereby informing more effective management strategies for sustainable agriculture on phosphate mining lands.
Furthermore, the need for adaptive management strategies and a comprehensive understanding of the socioeconomic benefits arising from successful restoration initiatives remains inadequately addressed. Jellinek et al. (2019) underscored this gap in understanding the broader impacts of restoration beyond ecological aspects. Moreover, innovative remediation techniques tailored to the unique challenges of phosphate-mined landscapes and the role of microbial communities in rehabilitated soils, particularly in the context of phosphate clay cover, represent additional gaps in the existing literature (EPA, 2020).
Integration of indigenous knowledge in restoration practices, community participation, and decision-making processes related to phosphate-mined site restoration is also an understudied area (Zine et al., 2018). Comparative analyses of reclamation methods, deficient heavy metal analysis in soil control with a call for comparative norms, and comprehensive gaps in agricultural rehabilitation practices are further identified gaps (Lima et al., 2016).
One noteworthy omission relates to the advancement of environmental monitoring in mine site rehabilitation, proposing a holistic approach that analyses roots, leaves, and fruits to gauge heavy metal mobility within trees. This approach, as advocated by Damayanti and Handayani (2023), enhances our understanding of the ecological impact of mining activities and contributes to the development of effective rehabilitation strategies. These identified gaps collectively highlight the need for comprehensive and multifaceted research initiatives to address the complexities of restoring phosphate-mined areas in Morocco.
7 Restoration techniques of phosphate mining sites
Phosphate mining is essential for global agriculture, but it often leaves a lasting impact on the environment (OCP, 2022a). To mitigate the ecological consequences, restoration techniques are crucial. Figure 8 presents various restoration techniques used in the context of phosphate mining sites.
7.1 Chemical restoration
Chemical restoration techniques involve the application of chemicals to amend soil properties and support vegetation growth (Guéablé et al., 2021). This may include the addition of lime, gypsum, compost, biosolids fertilizers or other soil conditioners to address pH imbalances and nutrient deficiencies. Chemical amendments such as fertilizers play a vital role in improving soil quality and creating conditions conducive to plant establishment (Li and Huang, 2015).
7.2 Physical restoration
Physical restoration focuses on manipulating the landscape to promote ecological recovery (Cacciuttolo and Cano, 2022). This may involve reshaping landforms, contouring, and creating water retention features. Physical techniques aim to control erosion, enhance soil structure, and create microclimates that support plant colonization. The restoration of mining sites can be approached through diverse and innovative methods, including the integration of photovoltaic panels, the creation of recreational parks, residential development, and the harnessing of rainwater. Employing photovoltaic panels on reclaimed mining lands not only contributes to sustainable energy generation but also serves as a dual-purpose strategy by providing shade and promoting biodiversity beneath the panels (Lambert et al., 2022; Pouresmaieli et al., 2023). Transforming former mining areas into recreational parks enhances the ecological and social value of the landscape, offering a harmonious blend of green spaces and leisure facilities (Kalybekov et al., 2019). Simultaneously, designing residential spaces on reclaimed sites ensures a responsible and efficient use of land resources, promoting eco-friendly living in proximity to restored natural habitats (Donsimoni and Labaronne, 2014). Additionally, the incorporation of rainwater harvesting systems aids in sustainable water management, minimizing runoff and replenishing local aquifers (Oweis, 2017). This multifaceted approach not only addresses the environmental impact of mining activities but also strives to create resilient, functional, and community-centric spaces within the restored landscapes.
7.3 Biological-phytoremediation
Biological restoration through phytoremediation involves the use of plants to remediate contaminated soils. Phytoremediation utilizes plants to efficiently clean up diverse contaminants in polluted environments, ranging from metals and pesticides to explosives and oil (EPA, 2001). Phytoremediation processes emerge as a promising strategy for mining sites restoration. This approach involves selecting indigenous plants that accumulate heavy metals and thrive in the region’s specific conditions, reducing contaminant mobility (Mendez and Maier, 2008a). Acting as an alternative to capping, phytoremediation establishes a vegetative cap within tailings, immobilizing metals and curbing erosion (Mendez and Maier, 2008b). Assisted phytoremediation, which relies on organic matter, root exudates, and microbial communities, stabilizes contaminants effectively (Mendez and Maier, 2008a). Despite limited field studies, the potential of assisted phytoremediation is underscored by research in semi-arid environments (Brown et al., 2009; Pardo et al., 2014). Integrating phytoremediation into mine site restoration offers a sustainable and effective remediation solution. Phytoremediation deploys some strategies to address metal pollution as shown in Figure 8.
Phytoremediation has proven to be particularly successful in addressing land degradation caused by mining in Morocco. The country’s phosphate mining activities have resulted in soils contaminated with metallic trace elements (MTEs) such as cadmium (Cd), lead (Pb), zinc (Zn), and copper (Cu) (Diallo et al., 2024). Research has shown that various plant species can mitigate these effects. For example, Italian ryegrass (Lolium multiflorum) significantly reduced MTE concentrations in polluted soils at sites like Mibladen by accumulating these metals in its roots and shoots (Elouadihi et al., 2022). Additionally, native species such as Hirschfeldia incana, Citrullus vulgaris, Aizoon hispanicum, and Stipa capensis have demonstrated their ability to stabilize heavy metals, preventing further environmental damage (Midhat et al., 2019). These efforts, which combine strategies like phytoextraction and phytostabilization with techniques such as organic mulching and drip irrigation, have shown great potential in rehabilitating mined lands, contributing to sustainable agriculture and ecological restoration (Nouri et al., 2013).
In Morocco, phosphate-mined lands have been a focal point for phytoremediation initiatives. Studies by Zine et al. (2020) and others highlight the use of native plants such as Atriplex semibaccata and Vicia sativa for phytostabilization. These plants immobilize heavy metals like cadmium, copper, and zinc in their roots, reducing their mobility and environmental risks. Organic amendments, such as compost and peat, have further enhanced soil properties, fostering plant growth and reducing metal bioavailability (El Berkaoui et al., 2021). Moreover, the integration of phosphate industry by-products, such as phosphogypsum, into rehabilitation practices has boosted soil recovery and plant growth (Guéablé et al., 2024). The inclusion of nitrogen-fixing plants and diverse vegetation, including argan, olive, and carob trees, has also promoted soil fertility and stability, enhancing long-term ecological balance (Mghazli et al., 2021). These combined efforts underscore the potential of phytoremediation in restoring phosphate-mined lands in Morocco, demonstrating its role in achieving sustainable land use and ecological restoration.
7.4 Advancements in innovation technologies for optimizing phytoremediation strategies
Innovation serves as a key driver in advancing novel strategies within the field of phytoremediation, enabling the creation of sustainable and efficient methods for remediating contaminated soils. For instance, a recent patent outlines an eco-friendly approach to removing heavy minerals from soils, sediments, and mine tailings. This technique avoids the use of water and minimizes dust generation, presenting an effective solution for restoring sites affected by heavy mineral contamination while mitigating environmental impacts during the remediation process (Snyder and See, 2022).
Another noteworthy innovation involves a specialized soil conditioner tailored for heavy metal-contaminated soils. This formulation incorporates components such as plant ash, calcium magnesium phosphate, quick lime, zeolite, hydroxyapatite, and fertilizers in specific ratios. These elements interact synergistically to stabilize heavy metals, improve soil fertility, and enhance soil structure. This approach has demonstrated high efficiency, cost-effectiveness, and environmental compatibility, making it particularly suitable for rehabilitating soils degraded by industrial and mining activities (Chen et al., 2014).
Additionally, a patented formulation for woody plant seeding has been developed to support phytoremediation and the ecological restoration of disturbed lands. This formulation combines woody plant seeds, organic mulch, beneficial microorganisms, mineral fertilizers, absorbent polymers, and adhesives. It also includes a pre-treatment process involving organic biomass to improve soil conditions. This integrated approach promotes robust plant establishment, enhances soil properties, and facilitates long-term ecological recovery (Beaudoin Nadeau et al., 2022).
8 Advantage and disadvantage of restoration techniques and their cost implications
Table 1 provides a concise overview of the advantages and disadvantages of three key restoration techniques: Chemical, Physical (including various applications), and Biological-Phytoremediation employed in phosphate mining sites.
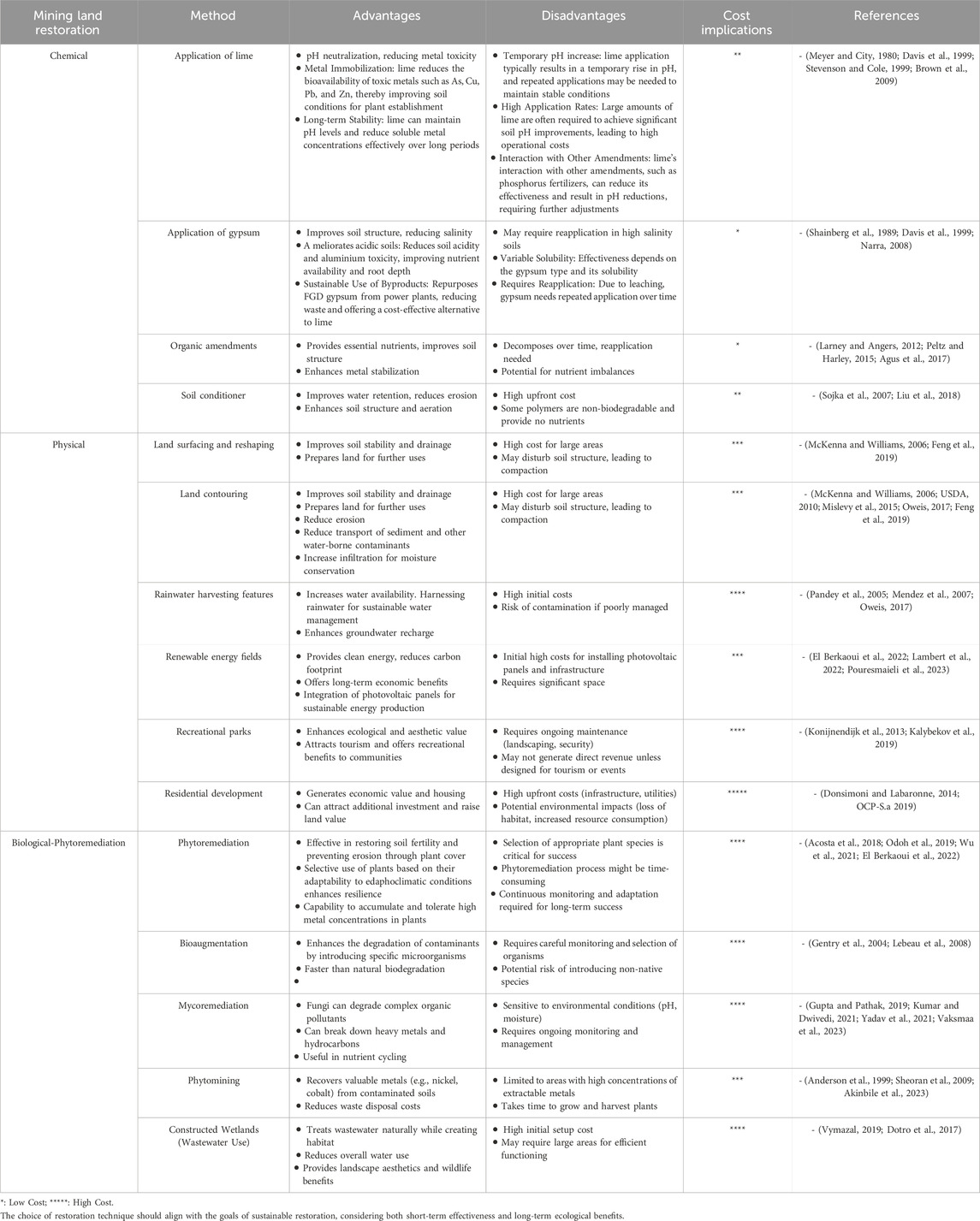
Table 1. Comparison of restoration techniques for phosphate mining sites: Advantages, disadvantages, and cost implications.
9 Plant species for mining sites restoration
Successful mine site restoration requires a holistic approach for plant species selection integrating trees, grasses, and shrubs in a balanced manner, while considering the advantages of both monoculture and mixed plantation, and evaluating the suitability of indigenous versus introduced species, is essential. The choice of plant species should align with restoration goals, ecological principles, and long-term sustainability.
9.1 Use of trees, grasses and shrubs
Efficient phytoremediation strategies for mining site reclamation necessitate a judicious selection of vegetation, encompassing trees, grasses, and shrubs, to address the multifaceted challenges of contamination (Cetinkaya and Sozen, 2011). Grasses play a pivotal role by swiftly establishing ground cover, transiently mitigating tailings dispersion, while concurrently, shrubs and trees instigate enduring erosion prevention (Padmavathiamma et al., 2014). In arid contexts, the arboreal and shrubbery presence not only fosters a nutrient-rich milieu for grasses but also ameliorates soil attributes. The incorporation of halophytes, adept at salt tolerance, assumes critical importance in saline soil environments for the efficacious realization of phytostabilization endeavours (Mendez and Maier, 2008b). Tree species such as Acacia cyanophylla Lindl., Pinus brutia Ten., and Schinus molle L. exhibit notable promise, demonstrating adaptability and a propensity for metal accumulation. Populus sp. emerges as a commendable choice due to its commendable traits of deep-rootedness and rapid growth (Cetinkaya and Sozen, 2011). Concurrently, shrubs, including Pistacia terebinthus L., Capparis spinosa L. var. canescens Coss., Ziziphus lotus (L.) Lam., and Lycium ferocissimum Miers, are posited as viable options for re-vegetation, leveraging their wide colonization. An assessment of native plant species in the mining site suggests their resilience to drought, salinity, and contamination challenges (Cetinkaya and Sozen, 2011).
Noteworthy recommendations include the utilization of members from the Chenopodiaceae family, particularly Atriplex spp., and halophytic shrubs such as Larrea tridentate and Baccharis sarothroides, renowned for their salt tolerance in arid regions (Glenn et al., 1999). Exemplifying this, the United Arab Emirates (UAE) demonstrates the physiological capacity of plants, including mangroves and halophytes, to accumulate essential ions, thereby mitigating soil salinity and metal concentrations (Padmavathiamma et al., 2014). Furthermore, introducing salt-tolerant species such as Conocarpus erectus and Atriplex lentiformis in arid regions holds promise for augmenting agricultural production (Padmavathiamma et al., 2014). The integration of drought-tolerant species, such as Ceratonia siliqua, Eucalyptus camaldulensis, and Moringa oleifera, stands as a prospective strategy to address the challenges posed by arid and semi-arid climates (Ezzine et al., 2023). Table 2 presents examples of plans with their phytoremediation mechanisms regarding heavy metals removal.
9.2 Monoculture and mixed plantation
The debate between monoculture and mixed plantation strategies in mining site restoration revolves around balancing ecological resilience with operational efficiency. Monocultures, historically efficient in simplifying management practices (Nichols et al., 2006), often lack ecological robustness, being more susceptible to pests and diseases, as noted by Kanowski and Catterall (2010). In contrast, mixed plantations incorporating diverse native species can mitigate these risks by enhancing biodiversity and ecosystem stability (Alexander et al., 2011). This diversity not only promotes sustainable forest ecosystems but also enhances phytoremediation efficiency. Mixed plantations, which include a variety of species, promote biodiversity, enhance ecosystem services, and reduce the risk of catastrophic events (Nichols et al., 2006; Kanowski and Catterall, 2010). Research by Vamerali et al. (2010) and Fagorzi et al. (2018) demonstrates that mixed plantations foster synergistic plant interactions, thereby improving metal uptake and soil health. By combining hyperaccumulators with non-hyperaccumulators, these plantations optimize biomass production and metal extraction capabilities, contributing to a more resilient and environmentally sustainable approach to mining land rehabilitation (Jha et al., 2017). Therefore, the choice between monoculture and mixed plantation methods should consider site-specific conditions and restoration goals, aiming to maximize both ecological resilience and phytoremediation effectiveness in restoring degraded mining landscapes.
9.3 Indigenous vs. introduced species
Selecting between indigenous and introduced species for mine site restoration is a critical decision that affects the effectiveness of re-establishing vegetation, a vital component of rehabilitating degraded landscapes (Wong and Bradshow, 2002). Indigenous species, adapted to local conditions, are often preferred due to their ability to enhance survival and growth rates (Wu et al., 2021). Species like kanuka (Kunzea ericoides) and manuka (Leptospermum scoparium) can thrive on mine wastes (Craw et al., 2007). These species contribute to soil structure improvement, increased organic matter, and enhanced microbial activity, promoting long-term ecosystem recovery (Todd et al., 2009).
In contrast, introduced species can offer rapid soil stabilization and contribute to biodiversity (Towns et al., 1997), but they also carry risks such as dominating native flora and altering local ecosystems, potentially reducing biodiversity (Todd et al., 2009). Introduced species may impact populations and communities significantly and may not interact effectively with local fauna and soil microorganisms (Williams, 2007). Therefore, while introduced species may provide short-term benefits, careful consideration of the ecological context, potential invasiveness, and long-term sustainability is crucial to ensure that the restoration efforts align with goals for long-term ecological stability and biodiversity (Simberloff et al., 2005).
10 Agricultural management of restored sites
The successful restoration of degraded sites demands a comprehensive and scientifically informed approach to management. This article explores the nuances of managing restored sites, with a keen focus on water management regimes, fertilizer application and management, and agronomic measures. By delving into these components, our aim is to provide an in-depth understanding of the scientific principles essential for effective site management and the enhancement of long-term ecological health.
10.1 Water management regimes
Effective water management is crucial in arid and semi-arid regions where water scarcity poses significant challenges. Drip irrigation is a highly efficient method that delivers water directly to the plant roots, significantly reducing water wastage compared to traditional surface irrigation (Dong et al., 2022). Subsurface drip irrigation (SDI) further enhances water use efficiency by placing the irrigation lines below the soil surface, which minimizes evaporation and nitrate leaching while promoting better crop health and yield (Thompson et al., 2009). Organic mulching is another vital practice that conserves water by retaining soil moisture, regulating soil temperature, and reducing evaporation (Kader et al., 2019). This technique is particularly beneficial in rain-fed systems and arid environments. Additionally, rainwater harvesting captures and stores rainwater for irrigation, helping to supplement water supplies and reduce reliance on conventional source (Oweis, 2017). Understanding the hydrological characteristics of the site and implementing comprehensive water management plans are crucial for mitigating issues such as waterlogging, drought stress and other water -related challenges (Cerdà and Doerr, 2005).
10.2 Fertilizer application and management
The judicious application of fertilizer is vital for replenishing soil nutrients depleted by degradation. Organic fertilizers, in particular, enhance soil fertility and structure while minimizing environmental impacts (Bhatt et al., 2019). In restoring degraded lands, particularly in sites like abandoned mines plagued by nutrient scarcity and toxic substances, chemical fertilizers play a pivotal role (Zhou et al., 2015). By introducing nutrients like nitrogen, phosphorus, and potassium, these fertilizers promote plant growth and enhance soil fertility, thus aiding in restoration (Mensah, 2015). When coupled with physical restoration techniques, chemical fertilizer can rectify soil pH, improve nutrient absorption, and enhance soil structure, thereby facilitating ecosystem recovery (Forján et al., 2019). However, careful consideration is necessary when applying chemical fertilizers, given potential environmental risks and high costs (Wu et al., 2010). Integrating chemical methods with other restoration strategies is crucial for achieving sustainable and effective outcomes (Barrow, 2012). Essential steps in ensuring successful restoration efforts include adopting ecologically-sound application methods and selecting appropriate fertilizers based on comprehensive nutrient deficiency assessments of the target site.
10.3 Agronomic measures
Agronomic measures are crucial for restoring soil health and productivity in the rehabilitation of mining land. Practices such as crop rotation, intercropping, and cover cropping play a significant role in soil conservation and ecosystem resilience (Quintarelli et al., 2022). Implementing deep ripping or tilling (Figure 9A) can help alleviate soil compaction caused by heavy machinery, while conservation tillage practices, including minimum tillage and no-till, minimize soil disturbance and reduce erosion risk (Mendez and Maier, 2008a; USDA, 2010; Swift et al., 2016). Effective crop management strategies, such as selecting appropriate crop hybrids and rotations, mitigate stress on crops and enhance soil health. Regular soil testing for nutrients and maintaining proper soil pH are essential for optimizing productivity. The use of cover crops and organic matter sources like crop residue and animal manure (Figure 9B), along with water management practices such as terracing (Figure 9C) and contour farming (Figure 9D), improve soil organic matter, support microorganisms, and ensure adequate water retention. These combined agronomic measures facilitate the sustainable restoration and management of reclaimed mining land, promoting its return to productive agricultural use (USDA, 2010).
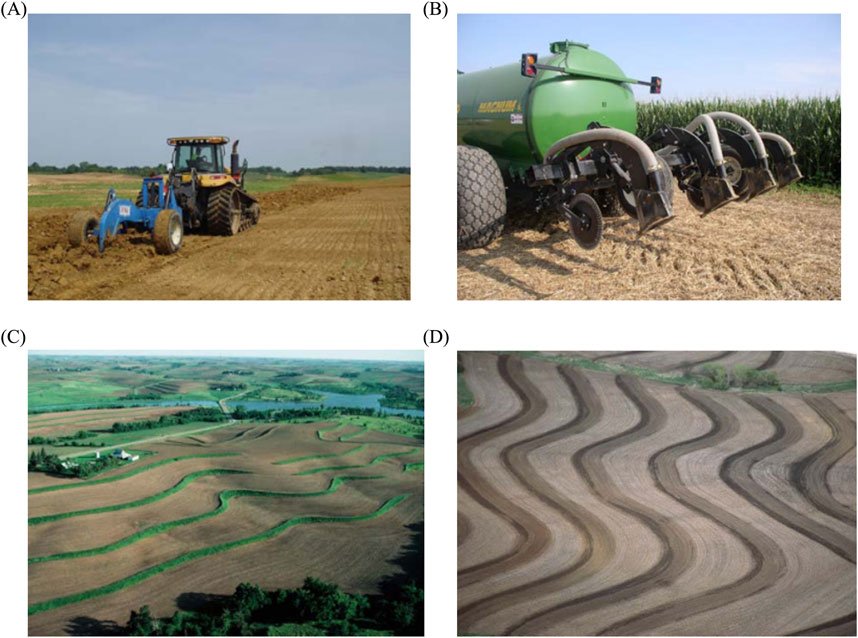
Figure 9. Soil restauration trough agronomic measures: Using a ripper to break up compaction on reclaimed land (A); Subsurface application of Animal waste (B); Terraces installed in a crop field to slow runoff and reduce erosion (C); Contour tillage used to reduce runoff and increase water infiltration (D).
11 Factors to consider for restoration of phosphate mined sites
11.1 Availability of topsoil and management
The availability and management of topsoil are pivotal considerations in the restoration of phosphate mined sites (Ghose, 2001). Topsoil serves as the foundation for vegetation establishment and nutrient cycling, providing essential organic matter and microbial communities necessary for soil health (Maiti, 2013). Effective management practices such as topsoil stockpiling, preservation, and redistribution are essential to ensure the successful reintegration of topsoil into the restored landscape (Ghose, 2001; Maiti, 2013). By prioritizing the preservation and strategic use of topsoil, restoration practitioners can facilitate the establishment of diverse and resilient plant communities, contributing to the long-term stability and ecological functionality of the site (Maiti, 2013; Evans, 2022).
11.2 Physico-chemical characteristics of the site
Understanding the physico-chemical characteristics of phosphate mined sites is paramount for implementing suitable restoration strategies (Yang et al., 2019). Factors such as soil texture, pH, and nutrient content significantly influence vegetation growth and ecosystem recovery dynamics (Liang et al., 2020). Soil texture determines water retention capacity and drainage, while pH levels affect nutrient availability and microbial activity (Maiti, 2013). A scientific understanding of these characteristics guides the selection of appropriate restoration techniques. Additionally, a comprehensive analysis should address potential contaminants and the application of soil amendments tailored to the specific needs of the site (Clewell and Aronson, 2006).
11.3 Bioavailability of the nutrient
The bioavailability of phosphate nutrients is a critical factor in promoting plant growth and facilitating ecosystem recovery on phosphate mined sites (Siebielec et al., 2018). Soil properties, microbial activity, and the chemical forms of phosphorus present in the soil influence phosphorus availability (Chen et al., 2021). Residual phosphorus from mining activities may exist in various forms, including organic and inorganic compounds, each with different rates of release and uptake by plants (Maiti, 2013; Siebielec et al., 2018). Assessing the bioavailability of phosphorus informs fertilizer application strategies and nutrient management plans, ensuring optimal nutrient utilization by vegetation during the restoration process (Duan et al., 2021). By addressing nutrient availability constraints, restoration practitioners can enhance plant establishment and growth, accelerating the recovery of disturbed ecosystems.
11.4 Topography
The topography of phosphate mined sites plays a crucial role in shaping water drainage patterns, erosion susceptibility, and overall landscape stability. Understanding the topographic features such as slope gradient, aspect, and drainage pathways is essential for designing effective erosion control measures and water management strategies (Cerdà and Doerr, 2005). Steep slopes increase the risk of soil erosion and surface runoff, necessitating the implementation of terracing, contouring, and vegetative buffers to mitigate erosion and sedimentation (Stefǎnescu et al., 2011). Conversely, flat or depressional areas may experience waterlogging and ponding, requiring drainage infrastructure and soil amendments to improve water infiltration and aeration (Oweis, 2017). By considering topographic characteristics, restoration practitioners can implement site-specific interventions that minimize erosion risk and enhance ecosystem resilience.
11.5 Water availability
Water availability is a critical determinant of vegetation establishment and ecosystem recovery on phosphate mined sites, particularly in regions characterized by arid or semi-arid climates (Northey et al., 2016). Adequate water supply is essential for sustaining plant growth, supporting soil microbial activity, and facilitating nutrient uptake (Dong et al., 2022). Techniques such as rainwater harvesting, irrigation, and soil moisture conservation are instrumental in maximizing water availability and minimizing moisture stress during the restoration process (Pandey et al., 2005; Oweis, 2017). By prioritizing water availability considerations, restoration practitioners can optimize plant survival and growth, accelerating the restoration of degraded landscapes.
11.6 Socio-economic context and local population need
The socio-economic context and local population needs profoundly influence the success and sustainability of restoration efforts on phosphate mined sites (Song et al., 2018). Engaging local communities, stakeholders, and indigenous groups in the restoration process fosters a sense of ownership, enhances project acceptance, and promotes long-term stewardship (Dubey, 2017). Understanding the socio-economic dynamics, cultural values, and livelihood dependencies of nearby communities is essential for integrating restoration objectives with local development priorities (Hajkowicz et al., 2011). By incorporating socio-economic considerations into restoration planning and implementation, practitioners can foster socio-ecological resilience and promote sustainable land management practices that benefit both people and the environment (Dubey, 2017; Song et al., 2018).
11.7 Climate
Climate exerts a profound influence on the ecological dynamics and restoration trajectories of phosphate mined sites (Odell et al., 2018). Climatic factors such as temperature, precipitation, and seasonal variability influence vegetation growth, soil moisture dynamics, and ecosystem resilience (Odell et al., 2018; Yuan et al., 2021). By considering climate considerations in restoration planning and implementation, practitioners can future-proof restoration efforts and promote ecosystem sustainability in a changing climate.
12 Institutional and regulatory aspects of phosphate mining restoration in the world and specifically in Morocco
12.1 Worldwide
Faced with the environmental impact of mining and quarrying, most countries have enacted legislation, albeit with varying degrees of specificity and stringency. In France, financial guarantees are mandatory to ensure site restoration following quarry exploitation. However, in mining operations, while there is no requirement for such guarantees, the restoration process is outlined in the authorization application. Both quarries and mines are expected to undergo revegetation, though it is not explicitly mandated by law.
In overseas French territories, adoption of metropolitan mining codes is recent, with New Caledonia as an example where there is currently no legal obligation for mine rehabilitation, though this is expected to change with the formulation of mining policies.
In the United States, the Surface Mining Control and Reclamation Act (SMCRA) mandates the restoration of landscapes to control runoff and erosion, alongside establishing diverse vegetation cover. However, this has deterred tree planting, as herbaceous cover suffices for deposit refunds to companies. Reforestation programs are underway in several countries, including Bolivia, Colombia, Brazil, Jamaica, and Malaysia.
In Australia, rehabilitation guidelines align with local environmental needs, with detailed proposals required before operation begins, and ongoing monitoring, including revegetation progress. Rehabilitation may be obligatory in places like the UK or Brazil, albeit without a consistent requirement for vegetation cover restoration, as seen in Zimbabwe.
In many countries, particularly in the Global South, rehabilitation efforts are lacking due to small-scale operations or weak enforcement of existing laws, as exemplified in Brazil’s Macedo nickel mine. Large-scale operations, however, adhere to international environmental standards.
Governments often court mining companies for investment and export opportunities, leading to increased exploration and exploitation. Countries with lenient environmental regulations or nascent mining sectors, like Argentina, attract foreign investors. Argentina’s mining sector, mainly in quarries, has seen significant growth, influenced by a liberal legal framework like Chile’s, despite past environmental issues (Roux, 2002).
12.2 Morocco
The significance of the mining and geological sector in the Moroccan economy is clearly apparent, thereby granting it a pivotal role in public policy. The Moroccan government, through its designated authority overseeing mining affairs, is actively working to enhance this sector’s competitiveness and allure, aiming to stimulate economic activity, particularly in isolated regions. The government has placed emphasis on advancing phosphate production and its derivatives through the OCP and has shown a strong interest in promoting investment in the petroleum sector. Additionally, attention has been given to other minerals through the 2025 mining strategy.
Initiated in 2013, the development strategy for the non-phosphate mining sector by 2025 aims to modernize mining legislation to align with the aspirations of mining operators. It also seeks to attract further investment, particularly from foreign sources, while boosting employment, transactions, and overall investment volume.
Regarding the regulation of mining waste recovery, the 33–13 law includes a dedicated chapter (Title V) that introduces provisions concerning the authorization of mining waste exploitation, specifically mine dumps and spoil heaps (Articles 75–83). The operational details for meeting the requirements of mining waste exploitation are outlined in Decree No. 2-18-548, which lays down the procedure for granting exploitation authorizations in accordance with the law.
Authorization for mining waste exploitation is equipped with regulatory mechanisms to ensure environmentally responsible waste management, including:
• Mandating environmental impact assessments: The decree specifies that applicants must commit to conducting environmental impact assessments and submitting environmental acceptability decisions within 1 year of receiving the exploitation authorization.
• Enforcing environmental preservation during exploitation: Holders of exploitation authorizations are required to inform the mining administration of proposed measures for hygiene, safety, and environmental preservation at least 1 month before commencing exploitation activities.
• Requiring site restoration in case of renunciation: Authorization holders must provide details of security measures taken or planned to restore exploited areas. The mining administration or delegated individuals may condition renunciation acceptance on executing necessary restoration work for the exploited sites.
As for the mining sites’ rehabilitation, the 33–13 law introduced a new requirement mandating the rehabilitation of mining sites post-exploitation. According to Article 60 of the law, mining exploitation license holders are obligated to develop a closure plan. However, as of now, the specific regulations for enforcing this obligation have not been established.
This requirement is being strengthened by draft law No. 46-20, which amends and supplements Law 33-13 concerning mining permits and authorizations for the exploitation of mine dumps and spoil heaps. This draft law establishes a deposit for each type of mining permit or authorization for the exploitation of mine dumps and spoil heaps. These deposits are intended, in principle, to finance rehabilitation work by the administration in cases where such work has not been undertaken by the operator (Energy and Environment, 2021).
13 Conclusion and recommendations
The restoration of phosphate-mined lands presents multifaceted challenges that necessitate a comprehensive and integrated approach. Effective restoration relies on addressing several critical factors, including topsoil management, soil physico-chemical properties, nutrient bioavailability, and water availability. Proper preservation and redistribution of topsoil are essential for re-establishing soil health and supporting plant growth. Detailed assessments of soil texture, pH, and nutrient levels guide the selection of appropriate restoration techniques and nutrient management strategies. Additionally, managing phosphorus bioavailability and implementing targeted erosion control measures based on topographic features are crucial for optimizing restoration outcomes. Moroccan phosphate mining operations have demonstrated significant advancements in land restoration practices, including the application of innovative techniques and the incorporation of local knowledge. However, there are notable gaps that need addressing to improve restoration outcomes. These include the need for more precise nutrient management, enhanced erosion control measures, and better water management practices.
To advance restoration practices, it is recommended to:
• Focus on Nutrient Dynamics and Heavy Metal Contamination: Investigate the interactions between phosphorus, heavy metals, and nutrient uptake in plants grown on restored sites to optimize agricultural productivity and ensure environmental safety.
• Leverage Indigenous Knowledge: Integrate traditional ecological practices and local expertise into restoration projects. This approach will improve community engagement and tailor solutions to the unique environmental and cultural context of Morocco.
• Adopt Innovative Remediation Techniques: Explore and implement cutting-edge remediation technologies, including phytoremediation, bioremediation, and the use of soil amendments, to address specific contamination challenges in phosphate-mined areas.
• Conduct Comprehensive Soil Assessments: Perform detailed analyses of soil physico-chemical properties and nutrient dynamics to tailor restoration approaches and improve soil conditions.
• Optimize Water Management: Implement innovative water conservation and irrigation techniques to maintain adequate moisture levels, particularly in arid regions.
• Integrate Community Involvement: Engage local communities in restoration planning and execution to align projects with socio-economic needs and enhance community support and stewardship.
• Strengthen Regulatory Frameworks: Advocate for robust environmental regulations and their enforcement to ensure sustainable mining practices and effective restoration of degraded lands.
By addressing these recommendations, Morocco can enhance the ecological resilience and productivity of phosphate-mined lands, contributing to sustainable land management and the long-term recovery of degraded landscapes.
Author contributions
AB: Conceptualization, Data curation, Investigation, Methodology, Visualization, Writing–original draft, Writing–review and editing. AAHS: Investigation, Validation, Visualization, Writing–review and editing. BAF: Methodology, Writing–review and editing. AH: Conceptualization, Funding acquisition, Investigation, Methodology, Project administration, Resources, Supervision, Validation, Visualization, Writing–review and editing.
Funding
The author(s) declare that financial support was received for the research, authorship, and/or publication of this article. This study was funded by the OCP Group under Grant No. AS73.
Acknowledgments
The authors would like to express their sincere gratitude to the OCP Group for providing the financial support necessary to carry out the project activities. We also extend our appreciation to everyone who contributed to the success of this work, whether through technical support, collaboration, or encouragement.
Conflict of interest
The authors declare that the research was conducted in the absence of any commercial or financial relationships that could be construed as a potential conflict of interest.
Generative AI statement
The author(s) declare that no Generative AI was used in the creation of this manuscript.
Publisher’s note
All claims expressed in this article are solely those of the authors and do not necessarily represent those of their affiliated organizations, or those of the publisher, the editors and the reviewers. Any product that may be evaluated in this article, or claim that may be made by its manufacturer, is not guaranteed or endorsed by the publisher.
Abbreviations
Al, Aluminium; As, Arsenic; Cd, Cadmium; Cu, Copper; EPA, Environmental Protection Agency; Fe, Iron; FGD, Flue gas desulphurization; GDP, Gross Domestic Product; Mn, Manganese; Ni, Nickel; OCP, Office Chérifien des Phosphates; P, Phosphorus; Pb, Lead; pH, Power of Hydrogen ions; PR, Phosphate rock; SDI, Subsurface drip irrigation; Se, Selenium; USD, United States dollar; USDA, United States Department of Agriculture; USGS, United States Geological Survey; USSR, Union of Soviet Socialist Republics; Zn, Zin.
References
Acosta, J. A., Faz, A., Martínez-Martínez, S., Zornoza, R., Carmona, D. M., Kabas, S., et al. (2018). Phytoremediation of mine tailings with Atriplex halimus and organic/inorganic amendments: a five-year field case study. Chemosphere 204, 71–78. doi:10.1016/j.chemosphere.2018.04.027
Agus, C., Sari, A., Wulandari, D., Siregar, C. A., and Harianja, V. (2017). The role of soil amendment on tropical post tin mining area in bangka island Indonesia for dignified and sustainable environment and life. IOP Conf. Ser. Earth Environ. Sci. 83 (1), 012030. doi:10.1088/1755-1315/83/1/012030
Akinbile, B. J., Makhubela, B. C. E., and Ambushe, A. A. (2023). Phytomining of valuable metals: status and prospective-a review. Int. J. Environ. Anal. Chem. 103 (16), 3913–3933. doi:10.1080/03067319.2021.1917557
Alexander, S., Nelson, C. R., Aronson, J., Lamb, D., Cliquet, A., Erwin, K. L., et al. (2011). Opportunities and challenges for ecological restoration within REDD+. Restor. Ecol. 19 (6), 683–689. doi:10.1111/j.1526-100X.2011.00822.x
Anderson, C. W. N., Brooks, R. R., Stewart, R. B., Simcock, R., Leblanc, M., Robinson, B., et al. (1999). Phytomining for nickel, thallium and gold. J. Geochem. Explor. 67 (1–3), 407–415. doi:10.1016/S0375-6742(99)00055-2
Annual Report of the Court of Audit (2018). Annual Report of the court of Audit. L’activité minière sein l’Office chérifien phosphates. Available at: https://www.courdescomptes.ma/wp-content/uploads/2023/01/Lactivite-miniere-au-sein-de-lOffice-cherifien-des-phosphates.pdf.
Badr, N., Fawzy, M., and Al-Qahtani, K. M. (2012). Phytoremediation: an ecological to heavy metal polluted soil and evaluation of plant removal ability. World Appl. Sci. J. 16 (9), 1292–1301.
Barrow, C. J. (2012). Biochar: potential for countering land degradation and for improving agriculture. Appl. Geogr. 34, 21–28. doi:10.1016/j.apgeog.2011.09.008
Beaudoin Nadeau, M., Blouin, D., and Grenon, F. (2022). Seeding formulation for woody plants and pre-treatment of land surfaces.
Benjelloun, Z., and Saoud, K. (2021). Phosphate mining and the circular economy: Morocco’s OCP Group’s approach to sustainable water use, in Sustainable industrial water use: perspectives, incentives, and, eds. J. Smith, A. Brown, and C. Johnson (London: IWA Publishing), 63–72. doi:10.2166/9781789060676_0063
Bhatt, M. K., Labanya, R., and Joshi, H. C. (2019). Influence of long-term chemical fertilizers and organic manures on soil fertility - a review. Univers. J. Agric. Res. 7 (5), 177–188. doi:10.13189/ujar.2019.070502
Bradshaw, A. D. (1996). Underlying principles of restoration. Can. J. Fish. Aquatic Sci. 53 (Suppl. 1), 3–9. doi:10.1139/cjfas-53-s1-3
Brown, S., Svendsen, A., and Henry, C. (2009). Restoration of high zinc and lead tailings with municipal biosolids and lime: a field study. J. Environ. Qual. 38 (6), 2189–2197. doi:10.2134/jeq2008.0103
Cacciuttolo, C., and Cano, D. (2022). Environmental impact assessment of mine tailings spill considering metallurgical processes of gold and copper mining: case studies in the andean countries of Chile and Peru. WaterSwitzerl. 14 (19), 3057. doi:10.3390/w14193057
Cerdà, A., and Doerr, S. H. (2005). Influence of vegetation recovery on soil hydrology and erodibility following fire: an 11-year investigation. Int. J. Wildland Fire 14 (4), 423–437. doi:10.1071/WF05044
Cetinkaya, G., and Sozen, N. (2011). Plant species potentially useful in the phytostabilization process for the abandoned CMC mining site in Northern Cyprus. Int. J. Phytoremediation 13 (7), 681–691. doi:10.1080/15226514.2010.500155
Chen, W., Hou, X., and Liu, W. (2014). Compound heavy metal contaminated soil conditioner and application thereof and application process.
Chen, Y., Li, X., Zhang, Y., Wang, Y., Liu, Y., Lv, C., et al. (2021). Effects of different types of microbial inoculants on available nitrogen and phosphorus, soil microbial community, and wheat growth in high-P soil. Environ. Sci. Pollut. Res. 28 (18), 23036–23047. doi:10.1007/s11356-020-12203-y
Cieślik, B., and Konieczka, P. (2017). A review of phosphorus recovery methods at various steps of wastewater treatment and sewage sludge management. The concept of “no solid waste generation” and analytical methods. J. Clean. Prod. 142, 1728–1740. doi:10.1016/j.jclepro.2016.11.116
Cisse, L., and Mrabet, T. (2004). World phosphate production: overview and prospects. Phosphorus Res. Bull. 15 (0), 21–25. doi:10.3363/prb1992.15.0_21
Clewell, A. F., and Aronson, J. (2006). Motivations for the restoration of ecosystems. Conserv. Biol. 20 (2), 420–428. doi:10.1111/j.1523-1739.2006.00340.x
Craw, D., Rufaut, C. G., Haffert, L., Paterson, L., and Smith, C. M. S. (2007). Plant colonization and arsenic uptake on high arsenic mine wastes, New Zealand. Water, Air, Soil Pollut. 179 (1–4), 351–364. doi:10.1007/s11270-006-9238-3
Damayanti, R., and Handayani, S. (2023). Rehabilitation plan for coal pit revegetation area East Kalimantan. IOP Conf. Ser. Earth Environ. Sci. 1190 (1), 012016. doi:10.1088/1755-1315/1190/1/012016
Davis, A., Eary, L. E., and Helgen, S. (1999). Assessing the efficacy of lime amendment to geochemically stabilize mine tailings. Environ. Sci. Technol. 33 (15), 2626–2632. doi:10.1021/es9810078
Diallo, A., El Khalil, H., El Hachimi, M. L., El Hachimi, M., and El Hachimi, M. (2024). Native plant species growing on the abandoned Zaida lead/zinc mine site in Morocco: phytoremediation potential for biomonitoring perspective. PLOS ONE 19 (6), e0305053. doi:10.1371/JOURNAL.PONE.0305053
Dong, S., Li, Y., Wang, X., Zhang, Y., and Wan, S. (2022). Soil water and salinity dynamics under the improved drip-irrigation scheduling for ecological restoration in the saline area of Yellow River basin. Agric. Water Manag. 264 (January), 107255. doi:10.1016/j.agwat.2021.107255
Donsimoni, M., and Labaronne, D. (2014). L’OCP sur ses territoires. Available at: https://hal.univ-grenoble-alpes.fr/hal-00972642.
Dotro, G., Langergraber, G., Molle, P., Nivala, J., Puigagut, J., Stein, O., et al. (2017). Treatment wetlands. J. Environ. Qual. doi:10.2134/jeq1997.00472425002600050040x
Duan, S., Banger, K., and Toor, G. S. (2021). Evidence of phosphate mining and agriculture influence on concentrations, forms, and ratios of nitrogen and phosphorus in a Florida river. WaterSwitzerl. 13 (8), 1064. doi:10.3390/w13081064
Dubey, K. (2017). Socio economic impact study of mining and mining polices on the livelihoods of local population in the Vindhyan region of Uttar Pradesh. Centre Soc. For. Eco-Rehabilitation (ICFRE) 1 (2), 1–152. Available at: https://www.niti.gov.in/sites/default/files/2019-01/Socio-Economic-Impact-Study-of-Mining-and-Mining-Polices.pdf.
El Berkaoui, M., El Hachimi, M. L., El Hachimi, M., and El Hachimi, M. (2022). Assessment of the transfer of trace metals to spontaneous plants on abandoned pyrrhotite mine: potential application for phytostabilization of phosphate wastes. Plants 11 (2), 1–13. doi:10.3390/plants11020179
El Berkaoui, M., El Hachimi, M. L., El Hachimi, M., El Hachimi, M., Bendaou, N., and Smouni, A. (2021). Phytostabilization of phosphate mine wastes used as a store-and-release cover to control acid mine drainage in a semiarid climate. Plants 10 (5), 900. doi:10.3390/plants10050900
Elouadihi, N., El Hachimi, M. L., El Hachimi, M., and El Hachimi, M. (2022). View of Phytoremediation study of mining soils: case of the Mibladen and Zaida mine. High. Moulouya, Moroc. doi:10.18006/2022.10(6).1391.1400
Energy, M. O. F., and Environment, T. H. E. (2021). National action plan for the recovery of mining waste. Minist. energy, mines Environ. Environ. Dep. Available at: https://mtedd.gov.ma/images/PUBLICATIONS/plannationaldechets_miniers.pdf.
EPA (2001). A Citizen’s Guide to phytoremediation. United States Environmental Protection Agency. Available at: https://www.epa.gov/sites/default/files/2015-04/documents/acitizensguidetophytoremediation.pdf.
EPA (2020). Remediation technologies for cleaning up contaminated sites. United States Environmental Protection Agency. Available at: https://www.epa.gov/remedytech/remediation-technologies-cleaning-contaminated-sites (Accessed September 10, 2024).
Evans, K. (2022). From the ground up: prioritizing soil at the forefront of ecological restoration. doi:10.1111/rec.13453
Ezzine, H., El Hachimi, M. L., El Hachimi, M., and El Hachimi, M. (2023). Physiological responses of three field-grown species (Ceratonia siliqua, Eucalyptus camaldulensis, and Moringa oleifera) to water deficits in a Mediterranean semi-arid climate. Sci. Rep. 13 (1), 1–10. doi:10.1038/s41598-023-31664-y
Fagorzi, C., El Hachimi, M. L., El Hachimi, M., El Hachimi, M., Dziewit, L., Pini, F., et al. (2018). Harnessing rhizobia to improve heavy-metal phytoremediation by legumes. Genes. 9 (11), 542. doi:10.3390/genes9110542
Feng, Y., El Hachimi, M. L., El Hachimi, M., and El Hachimi, M. (2019). Effects of surface coal mining and land reclamation on soil properties: a review. Earth-Science Rev. 191 (November 2017), 12–25. doi:10.1016/j.earscirev.2019.02.015
Feng, Z., El Hachimi, M. L., El Hachimi, M., El Hachimi, M., Cui, R., and Lu, L. (2023). Integrated mining and reclamation practices enhance sustainable land use: a case study in huainan coalfield, China. Land 12 (11), 1994. doi:10.3390/land12111994
Flores-Tavizón, E., El Hachimi, M. L., El Hachimi, M., and El Hachimi, M. (2003). Arsenic tolerating plants from mine sites and hot springs in the semi-arid region of Chihuahua, Mexico. Acta Biotechnol. 23 (2–3), 113–119. doi:10.1002/abio.200390016
Forján, R., Rodríguez-Vila, A., and Covelo, E. F. (2019). Increasing the nutrient content in a mine soil through the application of technosol and biochar and grown with Brassica juncea L. Waste Biomass Valorization 10 (1), 103–119. doi:10.1007/s12649-017-0027-6
Gentry, T. J., Rensing, C., and Pepper, I. L. (2004). New approaches for bioaugmentation as a remediation technology. Crit. Rev. Environ. Sci. Technol. 34 (5), 447–494. doi:10.1080/10643380490452362
Gerwing, T. G., El Hachimi, M. L., El Hachimi, M., and El Hachimi, M. (2022). Restoration, reclamation, and rehabilitation: on the need for, and positing a definition of, ecological reclamation. Restor. Ecol. 30 (7), 0–2. doi:10.1111/rec.13461
Ghose, M. K. (2001). Management of topsoil for geoenvironmental reclamation of coal mining areas. Environ. Geol. 40 (11–12), 1405–1410. doi:10.1007/s002540100321
Glenn, E. P., Brown, J. J., and Blumwald, E. (1999). Salt tolerance and crop potential of halophytes. Crit. Rev. Plant Sci. 18, 227–255. doi:10.1080/07352689991309207
Guéablé, Y. K. D., El Hachimi, M. L., El Hachimi, M., El Hachimi, M., and El Mejahed, K. (2024). New sustainable strategy for rehabilitating phosphate mining sites using phosphate industry by-products and sludge integrating Argan, Carob, and Olive trees. Environ. Technol. Innovation 35, 103651. doi:10.1016/j.eti.2024.103651
Guéablé, Y. K. D., El Hachimi, M. L., El Hachimi, M., El Hachimi, M., Hafidi, M., El Gharouss, M., et al. (2021). New approach for mining site reclamation using alternative substrate based on phosphate industry by-product and sludge mixture. Sustain. Switz. 13 (19), 10751. doi:10.3390/su131910751
Gupta, D. K., Chatterjee, S., Datta, S., Veer, V., and Walther, C. (2014). Role of phosphate fertilizers in heavy metal uptake and detoxification of toxic metals. Chemosphere 108, 134–144. doi:10.1016/j.chemosphere.2014.01.030
Gupta, S., and Pathak, B. (2014). “Mycoremediation of polycyclic aromatic hydrocarbons,” in Abatement of environmental pollutants: trends and strategies. Editors K. Bauddh, B. Singh, and J. Korstad (Amsterdam: Elsevier), 127–149. doi:10.1016/B978-0-12-818095-2.00006-0
Hajkowicz, S. A., Heyenga, S., and Moffat, K. (2011). The relationship between mining and socio-economic well being in Australia’s regions. Resour. Policy 36 (1), 30–38. doi:10.1016/j.resourpol.2010.08.007
Hegazy, A. K., Abdel-Ghani, N. T., and El-Chaghaby, G. A. (2011). Phytoremediation of industrial wastewater potentiality by Typha domingensis. Int. J. Environ. Sci. Technol. 8 (3), 639–648. doi:10.1007/bf03326249
Hobbs, R. J., and Harris, J. A. (2001). Restoration ecology: repairing the earth’s ecosystems in the new millennium. Restor. Ecol. 9 (2), 239–246. doi:10.1046/j.1526-100X.2001.009002239.x
Ibrahim, M. M., Alsahli, A. A., and El-Gaaly, G. (2013). Evaluation of phytoremediation potential of six wild plants for metal in a site polluted by industrial wastes: a field study in Riyadh, Saudi Arabia. Pak. J. Bot. 45 (2), 571–576.
Jellinek, S., Parris, K. M., Driscoll, D. A., Dwyer, P. D., Gordon, A., Guerrero, A. M., et al. (2019). Integrating diverse social and ecological motivations to achieve landscape restoration. J. Appl. Ecol. 56 (1), 246–252. doi:10.1111/1365-2664.13248
Jha, A. B., Misra, A. N., and Sharma, P. (2017). “Phytoremediation of heavy metal-contaminated soil using bioenergy crops,” in Phytoremediation potential of bioenergy plants. Editors K. Bauddh, B. Singh, and J. Korstad (Singapore: Springer), 63–96. doi:10.1007/978-981-10-3084-0_3
Kader, M. A., Senge, M., Mojid, M. A., Ito, K., Khan, F. H., and Khan, N. I. (2019). Mulching as water-saving technique in dryland agriculture: review article. Bull. Natl. Res. Centre 43 (1), 147. doi:10.1186/s42269-019-0186-7
Kalybekov, T., Zhakupov, Z., Kalybekova, G., and Zhakypbek, Y. (2019). Substantiation of ways to reclaim the space of the previously mined-out quarries for the recreational purposes. E3S Web Conf. 123, 01004. doi:10.1051/e3sconf/201912301004
Kanowski, J., and Catterall, C. P. (2010). Carbon stocks in above-ground biomass of monoculture plantations, mixed species plantations and environmental restoration plantings in north-east Australia. Ecol. Manag. Restor. 11 (2), 119–126. doi:10.1111/j.1442-8903.2010.00529.x
Konijnendijk, C. C., Annerstedt, M., Nielsen, A. B., and Maruthaveeran, S. (2013). Benefits of urban parks - a systematic review, 1–68.Int. Fed. Parks Recreat. Adm. (January)
Kumar, V., and Dwivedi, S. K. (2021). Mycoremediation of heavy metals: processes, mechanisms, and affecting factors. Environ. Sci. Pollut. Res. 28 (9), 10375–10412. doi:10.1007/s11356-020-11491-8
Lambert, Q., Gros, R., and Bischoff, A. (2022). Ecological restoration of solar park plant communities and the effect of solar panels. Ecol. Eng. 182 (February), 106722. doi:10.1016/j.ecoleng.2022.106722
Larney, F. J., and Angers, D. A. (2015). The role of organic amendments in soil reclamation: a review. Can. J. Soil Sci. 92, 19–38. doi:10.4141/CJSS2010-064
Lebeau, T., Braud, A., and Jézéquel, K. (2008). Performance of bioaugmentation-assisted phytoextraction applied to metal contaminated soils: a review. Environ. Pollut. 153 (3), 497–522. doi:10.1016/j.envpol.2007.09.015
Li, X., and Huang, L. (2015). Toward a new paradigm for tailings phytostabilization - nature of the substrates, amendment options, and anthropogenic pedogenesis. Crit. Rev. Environ. Sci. Technol. 45 (8), 813–839. doi:10.1080/10643389.2014.921977
Liang, J. L., Liu, J., Wang, X., and Li, L. (2020). Novel phosphate-solubilizing bacteria enhance soil phosphorus cycling following ecological restoration of land degraded by mining. ISME J. 14 (6), 1600–1613. doi:10.1038/s41396-020-0632-4
Lima, A. T., Mitchell, K., O’Connell, D. W., Verhoeven, J., and Van Cappellen, P. (2016). The legacy of surface mining: remediation, restoration, reclamation and rehabilitation. Environ. Sci. Policy 66, 227–233. doi:10.1016/j.envsci.2016.07.011
Liu, J., Wang, Z., and Li, Y. (2018). Efficacy of natural polymer derivatives on soil physical properties and erosion on an experimental loess hillslope. Int. J. Environ. Res. Public Health 15 (1), 9–14. doi:10.3390/ijerph15010009
Maiti, S. K. (2013). “Topsoil management,” in Ecorestoration of the coalmine degraded lands. doi:10.1007/978-81-322-0851-8
Matschullat, J., and Gutzmer, J. (2012). Mining and its environmental impacts. Environ. Geol., 353–366. doi:10.1007/978-1-4939-8787-0_205
McKenna, P., and Williams, E. (2006). Mine rehabilitation: leading practice sustainable development program for the mining industry. Available at: https://www.industry.gov.au/publications/leading-practice-handbooks-sustainable-mining/mine-rehabilitation.
Mendez, M. O., Glenn, E. P., and Maier, R. M. (2007). Phytostabilization potential of quailbush for mine tailings: growth, metal accumulation, and microbial com. J. Environ. Qual. 36 (1), 245–253. doi:10.2134/jeq2006.0197
Mendez, M. O., and Maier, R. M. (2008a). Phytoremediation of mine tailings in temperate and arid environments. Rev. Environ. Sci. Biotechnol. 7 (1), 47–59. doi:10.1007/s11157-007-9125-4
Mendez, M. O., and Maier, R. M. (2008b). Phytostabilization of mine tailings in arid and semiarid environments - an emerging remediation technology. Environ. Health Perspect. 116 (3), 278–283. doi:10.1289/ehp.10608
Mensah, A. K. (2015). Role of revegetation in restoring fertility of degraded mined soils in Ghana: a review. Int. J. Biodivers. Conservation 7 (2), 57–80. doi:10.5897/ijbc2014.0775
Meyer, D. R., and City, C. (1980). Nutritional problems associated with the establishment of vegetation on tailings from an asbestos mine. Environ. Pollut. Ser. A, Ecol. Biol. 23, 287–298. doi:10.1016/0143-1471(80)90071-9
Mghazli, N., El Hachimi, M. L., El Hachimi, M., El Hachimi, M., El Adnani, M., and Bruneel, O. (2021). Description of microbial communities of phosphate mine wastes in Morocco, a semi-arid climate, using high-throughput sequencing and functional prediction. Front. Microbiol. 12 (July), 1–17. doi:10.3389/fmicb.2021.666936
Midhat, L., El Hachimi, M. L., El Hachimi, M., El Hachimi, M., and Mandi, L. (2019). Accumulation of heavy metals in metallophones from three mining sites (Southern Centre Morocco) and evaluation of their phytoremediation potential. Ecotoxicol. Environ. Saf. 169, 150–160. doi:10.1016/J.ECOENV.2018.11.009
Mislevy, P., El Hachimi, M. L., El Hachimi, M., and El Hachimi, M. (2015). Phosphate mining and reclamation. Reclam. Drastically Disturb. Lands 1980 (41), 961–1005. doi:10.2134/agronmonogr41.c38
Narra, S. (2008). Structural amelioration of clay-sand substrate using FGD Gypsum as a substitute for Lime in recultivation of virgin soil at post-mining field Noche (Latia) under soil physical and plant growth aspects.
Nichols, J. D., Bristow, M., and Vanclay, J. K. (2006). Mixed-species plantations: prospects and challenges. For. Ecol. Manag. 233, 383–390. doi:10.1016/j.foreco.2006.07.018
Northey, S. A., El Hachimi, M. L., El Hachimi, M., El Hachimi, M., and Haque, N. (2016). Water footprinting and mining: where are the limitations and opportunities? J. Clean. Prod. 135, 1098–1116. doi:10.1016/j.jclepro.2016.07.024
Nouri, M., El Hachimi, M. L., El Hachimi, M., El Hachimi, M., Ksibi, M., Pereira, R., et al. (2013). Metal and phosphorus uptake by spontaneous vegetation in an abandoned iron mine from a semiarid area in center Morocco: Implications for phytoextraction. Environ. Res. Eng. Manag. 64 (2), 59–71. doi:10.5755/J01.EREM.64.2.3866
OCP (2020). Sustainability Report 2020. Encycl. Sustain. Manag., 3365. doi:10.1007/978-3-031-25984-5_302063
OCP (2022a). OCP sustainability integrated Report 2022. Available at: https://ocpsiteprodsa.blob.core.windows.net/media/2023-08/OCPSustainabilityIntegratedReport2022_1.pdf.
OCP (2022b). Document de reference relatif a L’exercice 2021. Available at: https://www.ammc.ma/sites/default/files/DROCP022_2022.pdf.
OCP (2019). Plaquette réhabilitation minière. Available at: http://www.ocp-siam.com/fr/content/plaquette-réhabilitation-minière.
Odell, S. D., Bebbington, A., and Frey, K. E. (2018). Mining and climate change: a review and framework for analysis. Extr. Industries Soc. 5 (1), 201–214. doi:10.1016/j.exis.2017.12.004
Odoh, C. K., El Hachimi, M. L., El Hachimi, M., and El Hachimi, M. (2019). Status, progress and challenges of phytoremediation - an African scenario. J. Environ. Manag. 237 (February), 365–378. doi:10.1016/j.jenvman.2019.02.090
Oweis, T. Y. (2017). Rainwater harvesting for restoring degraded dry agro-pastoral ecosystems: a conceptual review of opportunities and constraints in a changing climate. Environ. Rev. 25 (2), 135–149. doi:10.1139/er-2016-0069
Padmavathiamma, P. K., Ahmed, M., and Rahman, H. A. (2014). Phytoremediation - a sustainable approach for contaminant remediation in arid and semi-arid regions -a review. Emir. J. Food Agric. 26 (9), 757–772. doi:10.9755/ejfa.v26i9.18202
Pandey, D. N., El Hachimi, M. L., El Hachimi, M., and El Hachimi, M. (2005). Mine spoil restoration: a strategy combining rainwater harvesting and adaptation to random recurrence of droughts in Rajasthan. Int. For. Rev. 7 (3), 241–249. doi:10.1505/ifor.2005.7.3.241
Pardo, T., El Hachimi, M. L., El Hachimi, M., El Hachimi, M., and Bernal, M. P. (2014). The use of olive-mill waste compost to promote the plant vegetation cover in a trace-element-contaminated soil. Environ. Sci. Pollut. Res. 21 (2), 1029–1038. doi:10.1007/s11356-013-1988-z
Parker, D. R., El Hachimi, M. L., El Hachimi, M., and El Hachimi, M. (2003). Selenium phytoremediation potential of Stanleya pinnata. Plant Soil 249 (1), 157–165. doi:10.1023/A:1022545629940
Peltz, C. D., and Harley, A. (2015). Biochar application for abandoned mine land reclamation. Agric. Environ. Appl. Biochar Adv. Barriers 80439, 325–339. doi:10.2136/sssaspecpub63.2014.0047.5
Pouresmaieli, M., El Hachimi, M. L., El Hachimi, M., and El Hachimi, M. (2023). Integration of renewable energy and sustainable development with strategic planning in the mining industry. Results Eng. 20 (September), 101412. doi:10.1016/j.rineng.2023.101412
Quintarelli, V., El Hachimi, M. L., El Hachimi, M., El Hachimi, M., Haider, G., Abideen, Z., et al. (2022). Cover crops for sustainable cropping systems: a review. Agric. Switz. 12 (12), 2076. doi:10.3390/agriculture12122076
Roux, C.Le (2002). La réhabilitation des mines et carrières à ciel ouvert: Restauration des sites miniers. Bois forêts des tropiques 272 (272), 5–19. Available at: http://cat.inist.fr/?aModele=afficheN&cpsidt=13586759.
Sabiha-Javied, S., Khan, M., Iqbal, M., uz Zaman, Q., Aslam, A., Tufail, M., et al. (2023). Uptake of heavy metal in wheat from application of different phosphorus fertilizers. J. Food Compos. Analysis 115 (October 2022), 104958. doi:10.1016/j.jfca.2022.104958
Sandeep, G., Vijayalatha, K. R., and Anitha, T. (2019). Heavy metals and its impact in vegetable crops. Int. J. Chem. Stud. 7 (1), 1612–1621. Available at: https://www.chemijournal.com/archives/?year=2019&vol=7&issue=1&ArticleId=4944&si=false.
Shainberg, I., Sumner, M. E., Miller, W. P., Farina, M. P. W., Pavan, M. A., Fey, M. V., et al. (1989). Use of gypsum on soils: a review. A Rev. 9, 1–111. doi:10.1007/978-1-4612-3532-3_1
Sheoran, V., Sheoran, A. S., and Poonia, P. (2009). Phytomining: a review. Miner. Eng. 22 (12), 1007–1019. doi:10.1016/j.mineng.2009.04.001
Siebielec, G., Ukalska-Jaruga, A., and Kidd, P. (2018). “Bioavailability of trace elements in soils amended with high-phosphate materials,” in Phosphate in soils. doi:10.1201/9781351228909
Simberloff, D., Parker, I. M., and Windle, P. N. (2005). Introduced species policy, management, and future research needs. Front. Ecol. Environ. 3 (1 SPEC. ISS.), 12–20. doi:10.2307/3868440
Snyder, M. J., and See, J. R. (2022). System and method for suppressing dust during the collection of heavy minerals.
Sojka, R. E., Entry, J. A., Orts, W. J., and Lentz, R. (2007). Polyacrylamide in agriculture and environmental land management. Adv. Agron. 92 (04), 75–162. doi:10.1016/S0065-2113(04)92002-0
Song, Y., Zhao, Y., Tong, R., Zhang, J., Li, G., Gu, Q., et al. (2018). Environmental and socio-economic sustainability appraisal of contaminated land remediation strategies: a case study at a mega-site in China. Sci. Total Environ. 610–611, 391–401. doi:10.1016/j.scitotenv.2017.08.016
Stefǎnescu, L., Popescu, C., and Bălteanu, D. (2011). Assessment of soil erosion potential by the USLE method in Rosia Montana mining area and associated Natech events. Carpathian J. Earth Environ. Sci. 6 (1), 35–42.
Stevenson, F. J., and Cole, M. A. (1999). Cycles of soil: carbon, nitrogen, phosphorus, sulfur. Micronutrients.
Swift, R., McGowan, A., and Smith, J. (2016). Potential for agricultural production on disturbed soils mined for apatite using legumes and beneficial microbe. SOIL Discuss. (May), 1–21. doi:10.5194/soil-2016-33
Thompson, T. L., Huan-cheng, P., and Yu-ji, L. (2009). The potential contribution of Subsurface drip irrigation to water-saving agriculture in the western USA. Agric. Sci. China 8 (7), 850–854. doi:10.1016/S1671-2927(08)60287-4
Todd, A. J., Smith, R., and Brown, P. (2009). Indigenous plant species establishment during rehabilitation of an opencast coal mine, South-East Otago, New Zealand. N. Z. J. For. Sci. 39 (1), 81–98.
Towns, D. R., Simberloff, D., and A. E. Atkinson, I. (1997). Restoration of New Zealand islands: redressing the effects of introduced species. Pac. Conserv. Biol. 3 (2), 99–124. doi:10.1071/pc970099
USDA (2010). Farm management practices for reclaimed cropland. United States Department of Agriculture, Natural Resources Conservation Service, 1–26. Available at: http://www.techtransfer.osmre.gov/MRsite/Documents/InHousePublications/FarmMgtPractices1-11-10.pdf.
USGS (2023). Phosphate rock. U.S. Geological Survey. Available at: https://pubs.usgs.gov/periodicals/mcs2023/mcs2023-phosphate.pdf.
USGS (2024a). Phosphate rock. U.S. Geol. Surv. Available at: https://pubs.usgs.gov/periodicals/mcs2024/mcs2024-phosphate.pdf.
USGS (2024b). Phosphate rock statistics and information. U.S. Geol. Surv. Available at: https://www.usgs.gov/centers/national-minerals-information-center/phosphate-rock-statistics-and-information.
Vaksmaa, A., El Hachimi, M. L., El Hachimi, M., El Hachimi, M., Hernando-Morales, V., and Niemann, H. (2023). Role of fungi in bioremediation of emerging pollutants. Front. Mar. Sci. 10 (March), 1–21. doi:10.3389/fmars.2023.1070905
Vamerali, T., Bandiera, M., and Mosca, G. (2010). Field crops for phytoremediation of metal-contaminated land. A review. Environ. Chem. Lett. 8 (1), 1–17. doi:10.1007/s10311-009-0268-0
Van Kauwenbergh, S. J. (2010). World phosphate rock reserves and resources. International Fertilizer Development Center.
Vymazal, J. (2019). “Constructed wetlands for wastewater treatment,”. Encyclopedia of ecology. Editors S. E. Jørgensen, and B. D. Fath, 1-4, Second Edition (Oxford: Elsevier), 1, 14–21. doi:10.1016/B978-0-12-409548-9.11238-2
Williams, S. L. (2007). Introduced species in seagrass ecosystems: status and concerns. J. Exp. Mar. Biol. Ecol. 350, 89–110. doi:10.1016/j.jembe.2007.05.032
Wong, M. H., and Bradshow, A. D. (2002). “The restoration and management of derelict land: modern approaches,” in Restoration ecology (Singapore: World Scientifi Publishing Co.Pte.Ltd), 476–479. doi:10.2307/4131445
Wu, B., El Hachimi, M. L., El Hachimi, M., El Hachimi, M., Wang, X., Zhang, R., et al. (2021). Evaluation of phytoremediation potential of native dominant plants and spatial distribution of heavy metals in abandoned mining area in Southwest China. Ecotoxicol. Environ. Saf. 220 (24), 112368. doi:10.1016/j.ecoenv.2021.112368
Wu, G., El Hachimi, M. L., El Hachimi, M., El Hachimi, M., Chu, L., and Ruan, C. (2010). A critical review on the bio-removal of hazardous heavy metals from contaminated soils: issues, progress, eco-environmental concerns and opportunities. J. Hazard. Mater. 174 (1–3), 1–8. doi:10.1016/j.jhazmat.2009.09.113
Yadav, P., El Hachimi, M. L., El Hachimi, M., and El Hachimi, M. (2021). Mycoremediation of environmental pollutants: a review with special emphasis on mushrooms. Environ. Sustain. 4 (4), 605–618. doi:10.1007/s42398-021-00197-3
Yang, S. X., Li, X., Zhang, Y., Wang, Y., and Liu, Y. (2019). Effects of amendments on soil microbial diversity, enzyme activity and nutrient accumulation after assisted phytostabilization of an extremely acidic metalliferous mine soil. Appl. Sci. Switz. 9 (8), 1552. doi:10.3390/app9081552
Yuan, Y., Li, X., Zhang, Y., Wang, Y., Liu, Y., Guo, H., et al. (2021). Assessing vegetation stability to climate variability in Central Asia. J. Environ. Manag. 298 (April), 113330. doi:10.1016/j.jenvman.2021.113330
Zhou, L., Li, X., Zhang, Y., Wang, Y., Liu, Y., Zhong, L., et al. (2015). Restoration of rare earth mine areas: organic amendments and phytoremediation. Environ. Sci. Pollut. Res. 22 (21), 17151–17160. doi:10.1007/s11356-015-4875-y
Zine, H., El Hachimi, M. L., El Hachimi, M., and El Hachimi, M. (2018). Screening for native plant species potential revegetation of phosphatic clay applied as a cover to abandoned Kettara mine tailings Marrakech. Morocco 1, 83–88.
Keywords: heavy metals, integrated land management, phosphate mined lands, phytoremediation, rehabilitation
Citation: Bourazza A, Hassane Sidikou AA, Fenta BA and Hirich A (2025) Restoration of phosphate mined lands: literature review with insights from Morocco. Front. Environ. Sci. 13:1519868. doi: 10.3389/fenvs.2025.1519868
Received: 30 October 2024; Accepted: 02 January 2025;
Published: 22 January 2025.
Edited by:
Chong Jiang, Guangdong Academy of Science (CAS), ChinaReviewed by:
Ashwini A. Waoo, AKS University, IndiaDeepu Pandita, Government department of School Education, India
Copyright © 2025 Bourazza, Hassane Sidikou, Fenta and Hirich. This is an open-access article distributed under the terms of the Creative Commons Attribution License (CC BY). The use, distribution or reproduction in other forums is permitted, provided the original author(s) and the copyright owner(s) are credited and that the original publication in this journal is cited, in accordance with accepted academic practice. No use, distribution or reproduction is permitted which does not comply with these terms.
*Correspondence: Anass Bourazza, YW5hc3MuYm91cmF6emFAdW02cC5tYQ==