- 1Shelf Sea System Ecology, Biologische Anstalt Helgoland, Alfred Wegener Institute Helmholtz Centre for Polar and Marine Research, Helgoland, Germany
- 2Centre for Scientific Diving, Biologische Anstalt Helgoland, Alfred Wegener Institute Helmholtz Centre for Polar and Marine Research, Helgoland, Germany
- 3Shelf Sea System Ecology, Alfred Wegener Institute Helmholtz Centre for Polar and Marine Research, Bremerhaven, Germany
- 4Thünen Institute of Fisheries Ecology, Bremerhaven, Germany
Oyster reefs are biodiversity hotspots with multiple ecosystem functions and services that are declining worldwide. Historic populations of European oysters (Ostrea edulis) have been decimated by overfishing and are nowadays considered functionally extinct in European waters. To halt and reverse the associated biodiversity loss, oyster reef restoration was implemented into marine conservation measures and several reef restoration projects started across Europe. Following ecological restoration standards, it is crucial to identify reef-associated predators and predator-prey interactions influencing reef recovery as predators can control prey populations. Therefore, this study examined consumptive and nonconsumptive interactions among common North Sea predators, brown crabs (Cancer pagurus) and European lobsters (Homarus gammarus), and European oysters on Helgoland island (German Bight, North Sea) for the first time. Field surveys and monitorings in offshore pilot oyster reefs and experimental seafloor areas showed (i) that brown crabs, lobsters and oysters co-occur in these subtidal environments and (ii) interact with each other. Manipulative experiments indicated (iii, iv) that both predators consume oysters, (v) that medium-sized to large oysters are safe from brown crabs, and (vi) that large oysters are relatively safe from lobsters. They also found (vii) that the presence of common mussels (Mytilus spp.), as an alternative and more profitable prey, and (viii) the formation of larger and heavier oyster clumps, that are more difficult to handle, can reduce predation on oysters. Furthermore, they showed (ix) that the presence of brown crab conspecifics and (x) lobsters in natural abundances can nonconsumptively limit oyster consumption of brown crabs through intimidation mediated by (xi) brown crab- and (xii) lobster-released waterborne predator cues detected by brown crabs which indicates naturally underlying mechanisms regulating and limiting predation on oysters. Thereby, this study provides fundamental knowledge that is essential to understand predator-prey interactions in offshore oyster reefs and to facilitate ecological reef restoration.
1 Introduction
Oyster reefs are known as biodiversity hotspots with multiple ecosystem functions and services. Unfortunately, drastic declines of oyster reef habitats have been recognized worldwide (Cannon et al., 2022; zu Ermgassen et al., 2024). Historic populations of European oysters (Ostrea edulis) have been decimated by overfishing, collapsed in the 1920s and are nowadays considered functionally extinct in several European ecoregions of the North Sea (Pogoda, 2019; Thurstan et al., 2024). Against the background of preventing, halting and reversing biodiversity loss (United Nations Sustainable Development Goals, 2024), oyster reef restoration is implemented into marine conservation measures (BfN, 2024a; BfN, 2024b). Following ecological restoration standards and the recovery wheel concept (Gann et al., 2019), it is important to identify relevant reef-associated species and predator-prey interactions impacting ecosystem recovery and eventually guiding the practical implementation of restoration. This is crucial as predators can control prey populations (Menge et al., 2023; Meira et al., 2024) and were shown to have negative impacts on populations of ecologically and economically important American oysters (Crassostrea virginica) in North America (Johnson and Smee, 2014; Pickering et al., 2017).
In the North Sea, brown crabs (Cancer pagurus) and European lobsters (Homarus gammarus, formerly Homarus vulgaris) are common predators (Tonk and Rozemeijer, 2019; Jurrius and Rozemeijer, 2022). Brown crabs are very abundant in the German Bight (Tonk and Rozemeijer, 2019). Increases in artificial hard substrates (e.g., wind turbine foundations and wrecks) contribute to brown crab population growth (Krone et al., 2017; ter Hofstede et al., 2022) and constitute lobster habitats (Jurrius and Rozemeijer, 2022; Krone and Schröder, 2011; Thatcher et al., 2023). Lobster stocks are also supported by regional hatcheries (Schmalenbach et al., 2011; Hinchcliffe et al., 2021) and lobster sightings have increased in recent years (Helgoland Lobster, 2022; Stamp et al., 2024). Both predators prey on bivalves and snails (Hallbäck and Warén, 1972; Shelton et al., 1979; Hall et al., 1991; Karlsson and Christiansen, 1996; Mascaró and Seed, 2001; Silva et al., 2010; Leiknes, 2023) but information on co-occurrence and predator-prey interactions among brown crabs, lobsters and native oysters is largely missing (OSPAR, 2023) and limited to a single lab study which focused on juvenile brown crabs interacting with juvenile oysters (Mascaró and Seed, 2001). Therefore, it is unclear whether brown crabs and lobsters influence oyster reef restoration.
To address these knowledge gaps, (i) pilot oyster reefs in the Natura 2000 Borkum Reef Ground Marine Protected Area, North Sea were surveyed for brown crabs and lobsters and (ii) predator-oyster interactions in Helgoland harbor and off Helgoland, North Sea were monitored. Based on these field observations, we focused on consumptive and nonconsumptive interactions among predators and prey (Holt et al., 2009; Ferrari et al., 2010). We experimentally examined whether (iii) brown crabs and (iv) lobsters consume oysters and whether (v, vi) both predators prefer certain oyster sizes. Specifically, we tested the hypothesis that brown crabs and lobsters prefer relatively small oysters as shell-breaking predators tend to avoid potential claw damage that can result from handling over-sized prey (Juanes, 1992). As brown crabs also consume other molluscan prey (Shelton et al., 1979; Mascaró and Seed, 2001; Silva et al., 2010) and we found that individual large oysters are relatively safe from predation (Figures 2A, B, F), we investigated whether (vii) brown crabs preferred common mussels (Mytilus spp.), as an alternative prey, over oysters and whether (viii) large oyster clumps are safe from lobster predation. Moreover, as cannibalism occurs among brown crabs (Lawton, 1989; Amaral et al., 2009) and lobster stomachs contained brown crabs (Hallbäck and Warén, 1972), we tested (ix) whether brown crab and (x) lobster presence intimidates brown crabs and, thereby, reduces their oyster consumption. Finally, as brown crab and lobster presence limited oyster consumption of brown crabs (Figures 5A, B), we tested whether these nonconsumptive predator effects are mediated by waterborne cues released by (xi) brown crabs and (xii) lobsters. Waterborne predator cues can influence multiple consumers simultaneously and, thereby, have strong indirect effects on prey populations (Werner and Peacor, 2003; Preisser et al., 2005). Thus, we examined waterborne cues as such brown crab and lobster cues trigger predator defense responses in mussels (Côté, 1995; Côté and Jelnikar, 1999; Wang et al., 2024) (Supplementary Figure S1) and as waterborne predator cues can limit consumer feeding activity and, indirectly, facilitate prey survival (Trussell et al., 2003; Molis et al., 2011). Thereby, this study provides fundamental knowledge of consumptive and nonconsumptive interactions among brown crabs, lobsters and oysters, that is essential to understand predator-prey relationships in offshore oyster reef habitats and to facilitate ecological reef restoration.
2 Materials and methods
2.1 Field surveys and monitorings in seafloor environments
2.1.1 Predator abundance in offshore pilot oyster reefs at Borkum Reef Ground
Two pilot oyster reefs (53.894362, 6.464667; at 30 m depth) at Borkum Reef Ground were surveyed in September 2021 to examine whether brown crabs and lobsters occur. Both reefs consist of limestone boulders partially covered in oyster shells and were established in July 2020 to examine natural reef development (Pineda-Metz et al., 2023; Pogoda et al., 2024). During these surveys, occupational scientific divers took pictures across two transects on each reef using a camera (EOS M6 with an EF-M 22 mm lens; Cannon, Krefeld, Germany) and a quadrat (50 cm × 50 cm) (Pineda-Metz et al., 2022). After enhancing the picture quality in Lightroom (www.adobe.com) and removing low quality pictures, the remaining 44 pictures (11 m2 seafloor) were examined for both predators. Since both reefs cover a total area of circa 100 m2 (Pineda-Metz et al., 2023) these surveys captured 11% of the two reefs. Additionally, three oyster baskets (15 L, 6 mm grid width; Seapa, Edwardstown, SA, Australia), that were used for monitoring the growth of juvenile oysters next to the reefs (Pineda-Metz et al., 2023), were collected from the seafloor and examined for predators.
2.1.2 Predator-oyster interactions in the Helgoland harbor
To observe whether predators interact with oysters, a monitoring was conducted in the Helgoland harbor (54.170500, 7.891667; at 10 m depth) in September 2021 using a benthic lander equipped with two illuminated and camera-monitored oyster loading areas (Supplementary Figure S2). After deploying the lander on the seafloor, divers started the monitoring by putting nine medium-sized oysters (7–10 cm shell length, SL; i.e., the distance between the umbo hinge and the widest edge) (Pineda-Metz et al., 2023b) on each of the two loading areas (50 cm × 50 cm). During the monitoring, both cameras took pictures of the oysters every 15 s for approximately 40 h. To test the illumination and camera settings, the lander had previously been installed in the same location without oysters for a 24 h testing phase. After enhancing the picture quality in Lightroom, we examined 26,112 pictures taken over 104 h and 7 min for predators and predator-oyster interactions.
2.1.3 Predator-oyster spat-on-reef interactions off Helgoland
To check whether predators interact with oyster spat-on-reef, 500 small oysters (1–2 cm SL; purchased at Morecambe Bay Oysters, Barrow-in-Furness, England) were cemented to two 3D-sandstone reefs (each 50 cm in diameter and 50 cm in height) (Colsoul et al., 2019) using Prompt natural cement (2:1 cement/sea water ratio; Vicat, France; Supplementary Figure S3). Both sandstone reefs were deployed at MarGate (54.194062, 7.878252; at 10 m depth), an experimental seafloor area off Helgoland, with approximately 1 m distance to each other in August 2023. Spat-on-reef survival and predator abundance per sandstone reef were examined by the divers directly after reef deployment, after 1 day, and after 22 days.
2.2 Predator and prey collection, sizes, and husbandry at the Helgoland Oyster Hatchery
For manipulative experiments, we collected large brown crabs (10–12 cm carapace width, CW) by dredging the seafloor off Helgoland, small brown crabs (4–6 cm CW) using traps in the Helgoland rocky intertidal zone (54.177036, 7.884547) and lobsters (9–16 cm carapace length, CL; Supplementary Table S1) using traps off Helgoland. We purchased small (1 cm SL) and somewhat larger oysters (2 cm SL) at Morecambe Bay Oysters, medium-sized oysters (5–7 cm SL) at Bodes’s fish shop (Bremen, Germany) and large oysters (10–13 cm SL) at Rossmore Oysters (Stranraer, Scotland). We removed individual medium-sized and large Pacific oysters (Crassostrea gigas) and larger Pacific oyster clumps (9–15 cm SL) from low intertidal boulders in the Helgoland harbor (54.177178, 7.893714) using hammer and chisel and collected small mussels (Mytilus spp.) from pontons floating nearby (54.176808, 7.893872). We maintained all organisms in permanent seawater flow systems in the hatchery outdoor area. We fed crabs with large mussels (5–7 cm SL, five mussels/week) from subtidal ropes off Helgoland, lobsters with medium-sized Pacific oysters (five Pacific oysters/week) from the Helgoland harbor, and oysters with instant algae (Shellfish Diet 1800, Reed Mariculture Inc., Campbell, CA, United States; 250 mL/tank twice a week).
2.3 Manipulative experiments at the Biological Institute Helgoland
We conducted manipulative experiments in lab aquaria, lab mesocosms and outdoor tanks from June to November 2022 and from June to September 2023. Cuboid aquaria (31.5 cm × 18.5 cm × 16.5 cm, length × width × height, 9.6 L) were filled with 8 L water and received a constant flow of water (15 L/h). Mesocosms consisted of cylindrical basins (diameter: 126 cm, height: 50 cm, 620 L) containing 560 L water under constant flow (375 L/h) (Mackay-Roberts et al., 2024). Cuboid outdoor tanks (75 cm × 50 cm × 30 cm; 112 L) held 100 L water under constant flow (80 L/h). These dimensions ensured that all predators frequently encountered the offered prey. All aquaria and tank experiments were conducted under the natural light/dark rhythm. All mesocosm experiments were performed under red-filtered light, that does not influence brown crab and lobster activity (Conan et al., 1984; Davenport et al., 2023), with a 12 h light/dark rhythm. Basic information on all 19 experiments (hereafter: Exp1-17, S1-S2) are summarized in Supplementary Figures S4, S5.
2.3.1 Oyster consumption and preferences of brown crabs and lobsters
We performed four experiments (Exp1-4) to test whether large brown crabs consume oysters and to test our hypothesis that brown crabs prefer smaller oyster sizes. In general, we put a predator in an experimental unit (EU, e.g., aquarium or mesocosm) and offered it oysters of one size (one-choice experiment) or two sizes (two-choice experiment). As control, we put caged oysters, that could not be attacked by the predator, into the same EU (Exp1,2,4–8) or included oysters in a separate predator-free EU (Exp3). We started these experiments by adding the oysters and crabs to the EUs. We stopped them once oyster consumption of crabs had become apparent by removing the crabs from the EUs and counted the number of oysters consumed by each crab. Specifically, these experiments tested whether large crabs consume small oysters (Exp1) and differentiate between small and somewhat larger oysters (Exp2), small and large oysters (Exp3) and medium-sized and large oysters (Exp4). We chose these brown crab and oyster sizes as such organism sizes occur in the pilot oyster reefs (Pineda-Metz et al., 2023; Pogoda et al., 2024), as lab experiments had indicated that small brown crabs (2–4 cm CW) consume small oysters (0.3–1.8 cm SL) (Mascaró and Seed, 2001), and as such oyster sizes are commonly used in aquaculture and reef restoration (Pineda-Metz et al., 2023; Pogoda et al., 2024; Miron et al., 2005; Poirier et al., 2017; FAO, 2023b). We also used these approaches in four experiments (Exp5-8) that examined whether small and large lobsters consume small oysters (Exp5), somewhat larger oysters (Exp6) and medium-sized oysters (Exp7) and whether lobsters differentiate between medium-sized and large oysters (Exp8). Additionally, we took pictures of predator-oyster interactions and crushed oyster shells (Supplementary Figure S6).
2.3.2 Oyster and mussel preferences of brown crabs
As small juvenile brown crabs (2–4 cm CW) consume small oysters and mussels (Mascaró and Seed, 2001), we investigated whether crabs (4–6 cm CW) differentiate between oysters and mussels through a two-choice experiment (Exp9). To examine oyster and mussel profitability for crabs, we compared oyster and mussel shell thickness at the shell lip, center and base (Exp9) as well as oyster and mussel soft tissue dry weight (Exp9). We also estimated crab handling times for oysters (Exp10) and mussels (Exp11) through one-choice experiments. To measure shell thickness, we equipped digital calipers (Digi-Met, Helios Preisser, Gammertingen, Germany) with accessory metal extensions (Universal Measuring Tool Kit, www.fortis-tools.com) that we attached to the tip of each caliper jaw (Pickering and Quijón, 2011; Sherker et al., 2017). All oysters and mussels were picked at random. We measured the shell thickness of both valves of 30 oysters and 30 mussels each. As mussel shell thickness for the left and right mussel shell valves was highly correlated at the shell lip, center and base (Pearson correlation: r ≥ 0.57, p < 0.005; Supplementary Figure S7), we used the left mussel valve and both oyster shell valves for our comparisons. We dried the soft tissues of all oysters and mussels in a heating cabinet (Memmert, Schwabach, Germany) at 60°C for 72 h and measured individual soft tissue dry weight using a lab balance (Secura 224-1CEU, Sartorius, Göttingen, Germany). To estimate prey handling time, we counted the number of days until the crabs started consuming oysters and mussels. Oysters and mussels in separate EUs without crabs served as controls.
2.3.3 Individual Pacific oyster and Pacific oyster clump consumption of lobsters
To test whether clumping protects oysters from predation, we offered medium-sized individual Pacific oysters and larger Pacific oyster clumps, consisting of four to six oysters each, to large lobsters (11–16 cm CL; Exp12). We used Pacific oysters as model organisms because European oyster clumps were not available. We measured individual oyster shell length and oyster clump length (i.e., the longest axis of a clump) using the calipers. We determined individual oyster weight and oyster clump weight using a lab balance (EW 6200-2NM, Kern, Balingen, Germany). We also tested whether lobsters differentiate between medium-sized individual Pacific oysters and similar-sized individual European oysters (Exp13). Caged individual oysters and oyster clumps were the controls.
2.3.4 Effects of conspecific and lobster presence on oyster consumption of brown crabs
We tested whether conspecific presence influences oyster consumption of crabs (Exp14). For that, we placed six cages (36.5 cm × 26.5 cm × 21 cm, grid width: 1.2 cm) in each of 12 mesocosms. These cage dimensions enabled that the crabs frequently encountered their prey. To start the experiment, we added six medium-sized oysters and one large crab each to three cages in three mesocosms (conspecific presence), six medium-sized oysters each to the remaining cages in these three mesocosms (conspecific presence control), six medium-sized oysters and one large brown crab each to one cage in the other nine mesocosms (conspecific absence), and six medium-sized oysters each to the remaining cages in these nine mesocosms (conspecific absence control). After oyster consumption of crabs had become visible, we stopped the experiment by removing the oysters from the cages and counting the number of oysters consumed. We then compared the numbers of oysters consumed by crabs in conspecific presence and absence. The controls allowed testing whether the presence of three crabs (conspecific presence control) or one crab per mesocosm (conspecific absence control) had any effect on oyster mortality. Additionally, we examined whether the mesocosms affected oyster survival. For that, we had placed six cages in each of three mesocosms and included six medium-sized oysters in each cage (Supplementary Figure S8).
To examine whether lobster presence affects oyster consumption of brown crabs (Exp15), we used 12 mesocosms. We included one lobster in each of six mesocosms (predator presence) but no lobsters in the remaining six mesocosms (predator absence). We started the experiment by adding two cages to each mesocosm. One cage contained six medium-sized oysters and a large crab, while the other cage contained only six medium-sized oysters. The lobsters could freely move in the mesocosms but could not reach the caged oysters and crabs. The caged oysters in lobster presence and absence (as well as crab absence) served as controls. To examine whether the mesocosms affected oyster survival, we put two cages (each containing six medium-sized oysters) in each of three mesocosms (Supplementary Figure S9). We terminated and sampled this experiment as Exp14 above. To check the crab condition at the end of the experiment, we grabbed all crabs to check whether they clung to the cages and examined them externally.
2.3.5 Effects of conspecific and lobster water on oyster consumption of brown crabs
To examine whether the detected effects of conspecific and lobster presence on oyster consumption of brown crabs (Figures 5A, B) were mediated by conspecific- and lobster-released waterborne cues detected by the crabs, we measured oyster consumption of crabs exposed to conspecific water (Exp16) and lobster water (Exp17). For that, we produced crab water by keeping three large crabs in individual cages (36.5 cm × 26.5 cm × 21 cm, grid width: 1.2 cm) in a tank (250 L) containing 200 L water for 24 h and lobster water by keeping one large lobster in another tank (300 L) containing 200 L water for the same duration. We constantly aerated the stagnant water in both tanks using an automatic air pump (Medo-Blower LA-45C, Nitto Kohki Co., Ltd., Japan). We prepared 20 aquaria each containing 8 L stagnant crab (or lobster) water and 20 aquaria each containing 8 L stagnant water and placed 20 small oysters into each aquarium. We started both experiments by adding one small crab in each of ten crab (or lobster) water-filled aquaria and ten water-filled aquaria. The remaining aquaria (that contained only oysters in crab water, lobster water or water) served as controls (Supplementary Figure S10). We constantly aerated all aquaria using air pumps. We stopped and sampled both experiments as Exp14 (Section 2.3.4).
2.3.6 Randomization, procedural controls, oyster maintenance, temperature and salinity monitoring
We arranged all aquaria in the lab and all cages and organisms in the tanks and mesocosms at random. All organisms were intact and free from epibionts. All crabs and lobsters were in intermolt judging from their carapace hardness (Amaral et al., 2009). To standardize predator hunger state, all crabs and lobsters were fed regularly (Section 2.2) but subjected to a 3-day starvation period before most experiments (Amaral et al., 2009). Only the five largest Exp5 lobsters were exposed to a 10-day starvation period since they had not eaten any oysters in the previous Exp6 (Supplementary Figure S4). As procedural controls, we briefly touched each crab and made sure that each lobster responded to our presence when measuring their oyster consumption. To confirm that small oysters were alive, we gently rubbed their two valves back and forth between our fingers (Supplementary Figure S11) before (n = 1744 oysters in total) and after the experiments (n = 1,352). When doing that with dead oysters, both valves easily fell apart. The valves of dead oysters also did not contain any soft bodies but often sediment. Alive medium-sized and large oysters have firmly closed valves, while the valves of dead oysters of such sizes usually gape open (Poirier et al., 2017). During mesocosm and tank experiments that lasted longer than 48 h (Supplementary Figure S4), we fed the oysters every second day. For that, we diluted 15 mL Shellfish Diet in 24 L water and added 1.6 L of this dilution to each mesocosm or tank. We measured temperature and salinity in each EU daily using an infrared thermometer (Lasergrip 774, Etekcity Corporation, Anaheim, CA, United States) and a salinity tester (HI98319, Hanna Instruments Inc., Woonsocket, RI, United States; Supplementary Figure S5).
2.4 Data analysis
We visually confirmed that brown crabs and lobsters co-occur with oysters in the pilot oyster reefs, that lobsters interact with oysters in Helgoland harbor, that both predators interact with oyster spat-on-reef off Helgoland, that both predators crush and consume oysters in the lab, and that large lobsters consume medium-sized and large but not small oysters. We used one-sample t-tests to analyze one-choice experiments, two-choice experiments in which one oyster size (or prey) was not consumed and effects of conspecific presence on oyster consumption of crabs which did not consume oysters in conspecific presence. In all these cases, we compared the obtained mean number of consumed prey individuals (±SE) with zero. We used Student’s t-tests to analyze two-choice experiments and effects of lobster presence and waterborne predator cues on oyster consumption of brown crabs. Previously, we had checked whether the data met the assumptions for one-sample t-tests (normality) and Student’s t-tests (normality, variance homogeneity) using Kolmogorov-Smirnov and Levene tests (Sokal and Rohlf, 2012). In two cases, these assumptions were not met until the data were square root transformed after the constant “0.5” had been added (Sokal and Rohlf, 2012). When variance homogeneity could not be achieved through data transformation, we used Welch’s t-test for unequal variances (Ruxton, 2006). In one case, we omitted the one-sample t-test because the number of consumed oysters was very low (Figure 3D). In the Results section, we specified the type of each t-test when providing the corresponding result. We calculated Hedge’s g to compare the effect sizes of predator nonconsumptive effects and waterborne predator cues on oyster consumption of crabs. Hedge’s g ≥ 0.2 is a small effect, g ≥ 0.5 is a medium effect and g ≥ 0.8 is a large effect (Grizzard and Shaw, 2017). Since the duration of the experiments ranged between four and 264 h, we calculated standardized oyster consumption rates for 4 h to facilitate comparisons among the experiments (Figures 2, 3B–D, 5). We provide the raw data in Supplementary Tables S2–S23. We used Pearson correlation analyses to examine the shell thickness relationships between the left and right mussel valves (Section 2.3.2; Supplementary Figure S5) after we had confirmed that the shell thickness data met the required normality assumptions through Kolmogorov-Smirnov tests (Sokal and Rohlf, 2012). We performed these tests using GraphPad (www.graphpad.com) and Statistica (www.staftsoft.de) and plotted all data using Sigma Plot (www.grafiti.com).
3 Results
3.1 Field surveys and monitorings in seafloor environments
3.1.1 Predator abundance in offshore pilot oyster reefs at Borkum Reef Ground
There were 2.0 ± 0.9 brown crabs/m2 (mean ± SE) in the western reef, 3.1 ± 0.7 brown crabs/m2 in the eastern reef (Figure 1A), no lobsters in the western reef and one lobster (0.1 ± 0.1 lobster/m2) in the eastern reef (Figure 1B) on 18 September 2021. Brown crab size was 10–12 cm CW. Lobster size was 7 cm CL. In the three oyster baskets, there were two brown crabs in total (0–1 crab/basket; 4–5 cm CW). Lobsters did not occur in the baskets.
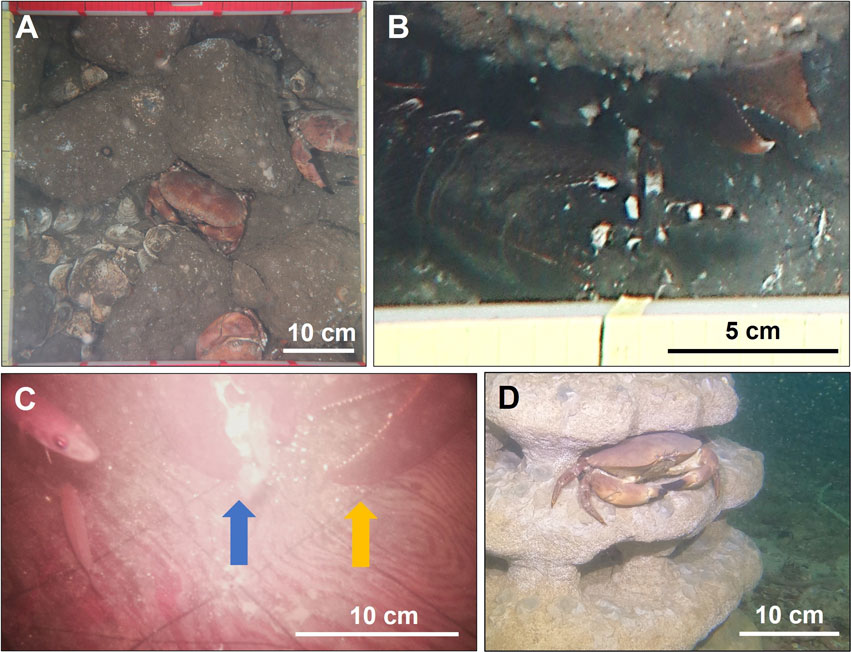
Figure 1. Field observations in the North Sea. (A) Brown crabs, oysters and limestones surveyed in the offshore pilot oyster reefs at Borkum Reef Ground on 18 September 2021 (picture source: Pineda-Metz et al., 2022). (B) A European lobster detected under a limestone at Borkum Reef Ground on 18 September 2021 (picture source: Pineda-Metz et al., 2022). (C) A lobster feeding on an oyster in Helgoland harbor on 23 September 2021. The blue arrow points at the lobster crushing claw crushing the oyster, while the orange arrow points at the lobster cutting claw (picture: S. E. A. Pineda-Metz). (D) A brown crab on an artificial sandstone reef with oyster spat-on-reef off Helgoland on 24 August 2023 (picture: M. Brand).
3.1.2 Predator-oyster interactions in the Helgoland harbor
We observed 18 lobster-oyster interactions in 14 h and 20 min from 6:41 p.m. on 25 September 2021 to 9:09 a.m. on 26 September 2021 (Supplementary Table S24; Supplementary Figures S2B–E). Most oysters were transported away from the lander loading area by a lobster. Only one oyster was consumed on the loading area by a lobster (Figure 1C). We also observed three lobster visits on the loading areas during which the lobster did not interact with the oysters and nine lobster visits after the last oyster had been carried away from the lander (Supplementary Table S24). We could not identify individual lobsters as the camera was mounted close to the loading area (for good picture quality) and, therefore, often photographed only individual lobster body parts. Still, most pictures showed large lobsters and only one picture showed a smaller lobster indicating that at least two lobsters were present and that the larger one(s) was/were predominant. While one camera showed a lobster at a given time, the other camera captured no lobster at the same time, showing that the lander was visited by only one lobster at the same time. Most lobster observations (77%) were made from 7 to 9 a.m. and from 7 to 9 p.m. (Supplementary Table S24; Supplementary Figure S12). Brown crabs did not occur during the monitoring and previous testing phase. One green crab (Carcinus maenas) was observed during the testing phase. Additionally, we note that all six lobsters13-18 collected for the experiments in June 2023 (Supplementary Table S1) were caught in traps deployed in a relatively small area (circa 25,000 m2; center: 54.176367, 7.898411) near the Helgoland harbor within 24 h.
3.1.3 Predator-oyster spat-on-reef interactions off Helgoland
Directly after the reef deployment, oyster spat-on-reef survival was 100% on both reefs on 2 August 2023. The following day, spat-on-reef survival was 25% on the western reef and 100% on the eastern reef. On the western reef, three very large brown crabs (15–20 cm CW) were observed preying on spat-on-reef, one small lobster and one swimming crab (Liocarcinus spp.) were seen attempting to prey on spat-on-reef and numerous detached and broken spat-on-reef shell pieces were found next to the reef. No predators were observed on the eastern reef but four lobsters were seen under two benthic landers near the reefs. After 22 days, almost all spat-on-reef was consumed. One very large brown crab (15 cm CW) was observed resting on each reef (Figure 1D) and one large brown crab (10 cm CW) was detected next to the eastern reef. One small lobster was found below a nearby lander.
3.2 Manipulative experiments at the Biological Institute Helgoland
All brown crabs and lobsters survived the experiments. Crabs and lobsters did not molt during the experiments. All crabs that were exposed to conspecific (Exp9) or lobster presence (Exp10) were intact and did not show any signs of predator attacks. In the controls, almost all oysters (99.9%) survived and oyster mortality was restricted to Exp8 (Section 3.2.1; Figure 2F). Details on water temperature and salinity during the experiments are provided in Supplementary Figure S5.
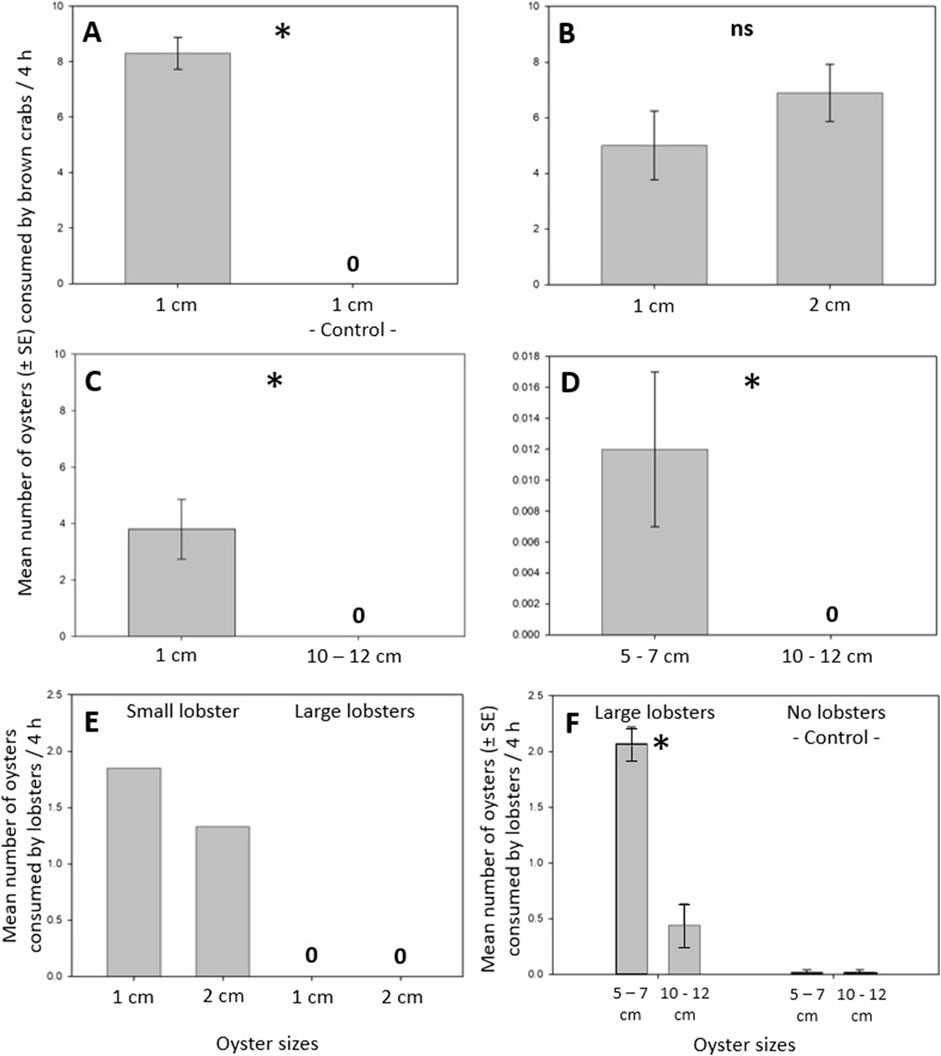
Figure 2. Oyster consumption of brown crabs and lobsters. (A) Crabs consumed small oysters (1 cm shell length, SL). (B) Crabs consumed small (1 cm, SL) and somewhat larger (2 cm SL) oysters and did not prefer small over somewhat larger oysters. (C) Crabs consumed small oysters but did not consume large oysters (10–12 cm SL). (D) Crabs consumed few medium-sized oysters (5–7 cm SL) but did not consume large oysters. (E) A small lobster (9 cm CL) consumed small and somewhat larger oysters, whereas large lobsters (12–16 cm CL) did not consume such oysters. (F) Large lobsters (11–15 cm CL) consumed medium-sized and large oysters and preferred medium-sized over large oysters. Oyster mortality in the controls was very low, identical for both oyster sizes and, thus, negligible. An “asterisk” indicates a significant difference (p < 0.05) between two corresponding bars, while “ns” denotes a non-significant result (p ≥ 0.05). A “0” indicates that no oyster died in the controls (A) or that no oyster individuals of a specific size were consumed by the predators (C–E).
3.2.1 Oyster consumption of brown crabs and lobsters
Crabs consumed small oysters (Exp1: one-sample t-test: t9 = 14.35, p < 0.001, Figure 2A), small and somewhat larger oysters (Figure 2B) and did not prefer small over somewhat larger oysters (Exp2: Student’s t-test: t16 = 1.18, p = 0.256, Figure 2B). Crabs preferred small over large oysters (Exp3: one-sample t-test: t9 = 3.53, p < 0.006, Figure 2C) and medium-sized over large oysters (Exp4: one-sample t-test: t8 = 2.40, p < 0.043, Figure 2D). Crabs consumed 500 times as many small oysters as medium-sized oysters (compare Figures 2C, D) indicating that they also preferred small over medium-sized oysters. Crabs did not consume large oysters (Figures 2C, D). No oyster mortality occurred in the controls.
A small lobster13 (9 cm CL) consumed all offered small and somewhat larger oysters during the first night (Exp5, 6, Figure 2E), whereas the remaining five large lobsters14-18 (12–16 cm CL) did not consume any oysters of these two sizes during the two 7-day experiments (Exp5, 6; Figure 2E). Large lobsters1-12 (11–15 cm CL) consumed medium-sized oysters (Exp7; Figure 2E). Hereby, lobster10 consumed all three oysters within a day, lobsters1, 2 and 4 consumed all oysters in 6 days, lobsters6-9 consumed all oysters in 8 days, and lobsters3, 5, 11 and 12 consumed all oysters in 9 days (Exp7). Large lobsters1-12 consumed medium-sized and large oysters (Exp8, Figure 2F) but strongly preferred medium-sized over large oysters (Exp8: Student’s t-test: t22 = 6.76, p < 0.001, Figure 2F). Oyster mortality in the controls was negligible and identical in medium-sized and large oysters (Exp8: 0.08 ± 0.08 medium-sized and large oysters, mean ± SE, Figure 2F). Additionally, we frequently observed that lobsters1-12 had piled up their oysters along the mesocosms walls.
3.2.2 Oyster and mussel preferences of brown crabs
When offered oysters (2–5 cm SL) and somewhat larger mussels (3–6 cm SL; Exp9: Student’s t-test: t58 = 7.29, p < 0.001; Figure 3A) simultaneously, crabs preferred mussels over oysters (Exp9: one-sample t-test: t8 = 2.54, p < 0.032) and did not consume any oysters over three consecutive days (Figure 3B). When offered such oysters and mussels separately, crabs readily consumed mussels on the first day of the experiment (Exp10) but started consuming oysters only during the third day (Exp11). Concerning the number of mussels and oysters consumed on the third day, crabs, again, consumed mussels (Exp10: one-sample t-test: t8 = 5.90, p < 0.001; Figure 3C) but hardly consumed oysters (Exp11; 0.22 ± 0.15 oysters; Figure 3D). Mussel shells were thinner than oyster shells at the shell lip (Exp9: Welch’s t-tests: left oyster valve: t36 = 4.48, p < 0.001; right oyster valve: t39 = 5.14, p < 0.001; Figure 3E), center (Exp9: Welch’s t-tests: left oyster valve: t33 = 5.77, p < 0.001; right oyster valve: t40 = 6.77, p < 0.001; Figure 3F), and base (Exp9: Welch’s t-tests: left oyster valve: t30 = 16.32, p < 0.001; right oyster valve: t35 = 6.55, p < 0.001; Figure 3G). Mussels contained more soft tissue than oysters (Welch’s t-test: t29 = 10.20, p < 0.001; Figure 3H). No oyster and mussel mortality occurred in the controls.
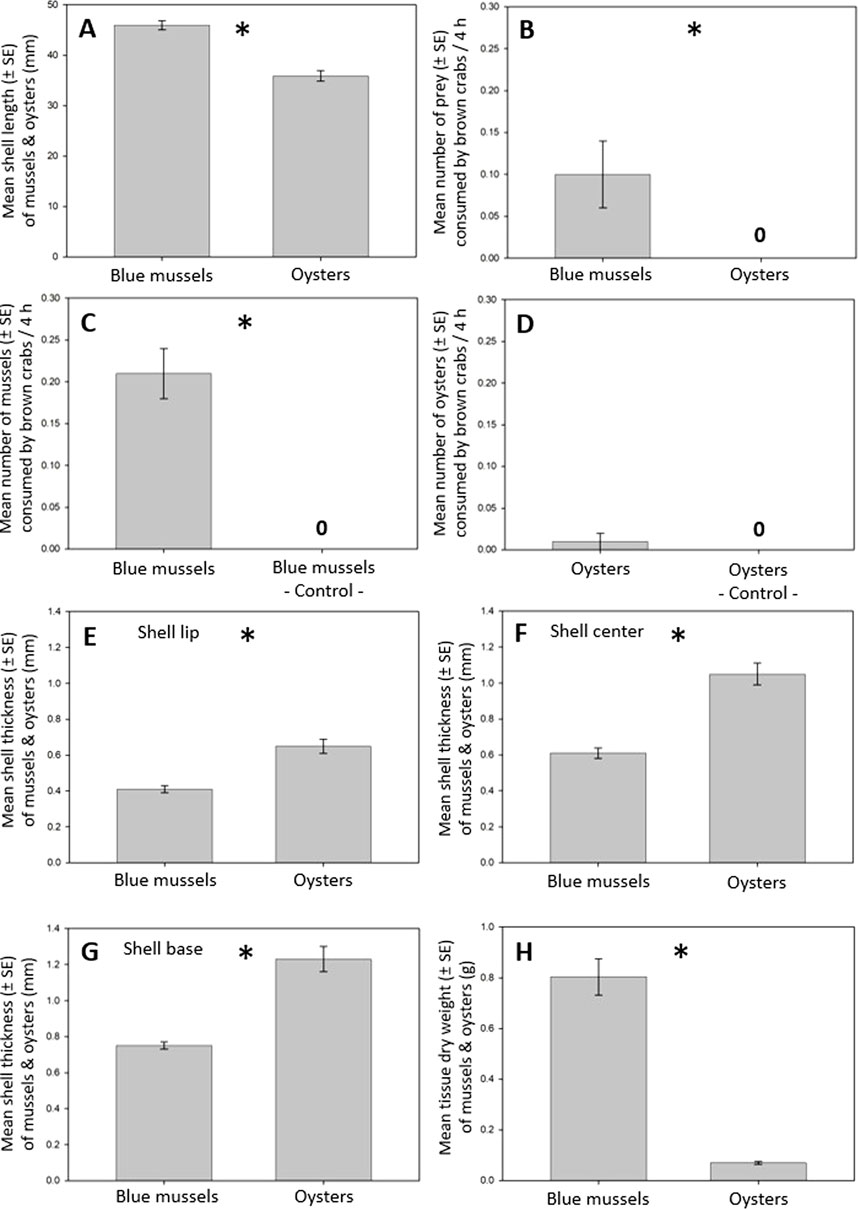
Figure 3. Mussel and oyster consumption of brown crabs. (A) Mussels were somewhat larger than oysters. (B) Crabs preferred mussels over oysters. (C) Crabs consumed mussels. (D) Crabs hardly consumed oysters. Therefore, no t-test was performed. (E) Shell lip thickness in mussels was thinner than in oysters. (F) Shell center thickness was thinner in mussels than in oysters. (G) Shell base thickness was thinner in mussels than in oysters. (H) Mussels contained more flesh than oysters. An “asterisk” indicates a significant difference (p < 0.05) between two corresponding bars and a “0” indicates that no oyster was consumed (B) or that no mussel or oyster mortality occurred in the controls (C, D).
3.2.3 Individual Pacific oyster and Pacific oyster clump consumption of lobsters
Lobsters1-12 attacked individual Pacific oysters and oyster clumps (Figure 4A) and preferred individual oysters over oyster clumps (Exp12: Student’s t-test: t22 = 2.65, p < 0.015, Figure 4A). All attacked individual oysters were consumed entirely, whereas only oysters protruding from the oyster clumps were eaten (Figure 4A). Individual oysters were smaller (Exp12: Student’s t-test: t142 = 12.99, p < 0.001, Figure 4B) and lighter than oyster clumps (Exp12: Student’s t-test: t142 = 15.94, p < 0.001, Figure 4C). Lobsters1-12 did not prefer medium-sized individual Pacific oysters over similar-sized individual European oysters (Exp13: Student’s t-test: t22 = 0.24, p = 0.813, Figure 4D). No individual oyster or oyster clump died in the controls.
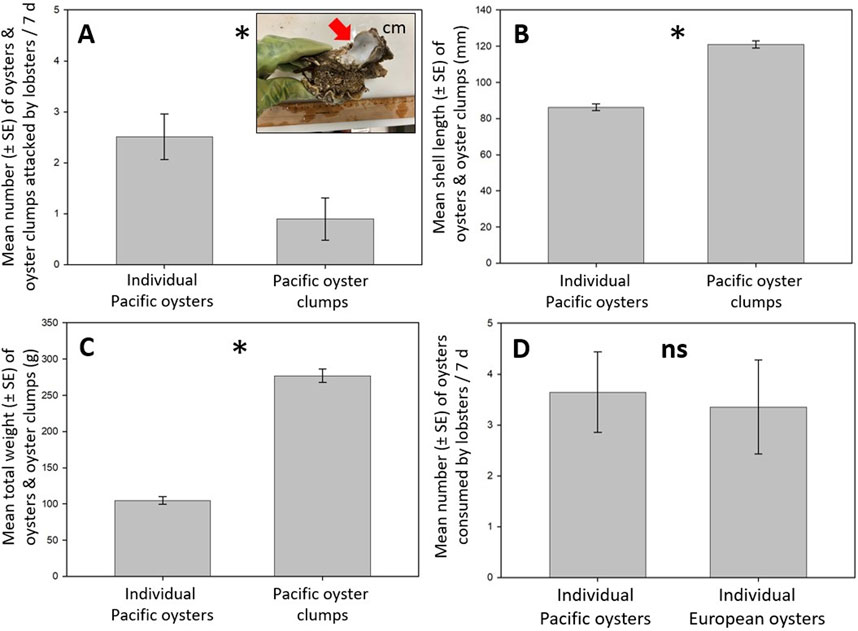
Figure 4. Individual Pacific oyster and Pacific oyster clump consumption of lobsters. (A) Lobsters attacked individual oysters and oyster clumps and preferred individual oysters over oyster clumps. Attacked individual oysters were consumed entirely (Supplementary Figure S6D), whereas only oyster individuals protruding from the oyster clumps were consumed (see red arrow in the picture inset for an example; picture: J. A. Ellrich). (B) Individual oysters were smaller than oyster clumps. (C) Individual oysters were lighter than oyster clumps. (D) Lobsters consumed medium-sized individual Pacific oysters and similar-sized European oysters and did not prefer Pacific over European oysters. An “asterisk” indicates a significant difference (p < 0.05) between two corresponding bars, whereas “ns” denotes a non-significant result (p ≥ 0.05).
3.2.4 Effects of conspecific and lobster presence on oyster consumption of brown crabs
Conspecific presence reduced crab consumption of medium-sized oysters by 100% (Exp14: one-sample t-test: t8 = 2.40, p < 0.043, Hedge’s g = 1.137; Figure 5A). No oyster mortality occurred in the controls which indicated that the presence of crabs does not affect oyster survival (Figure 5A).
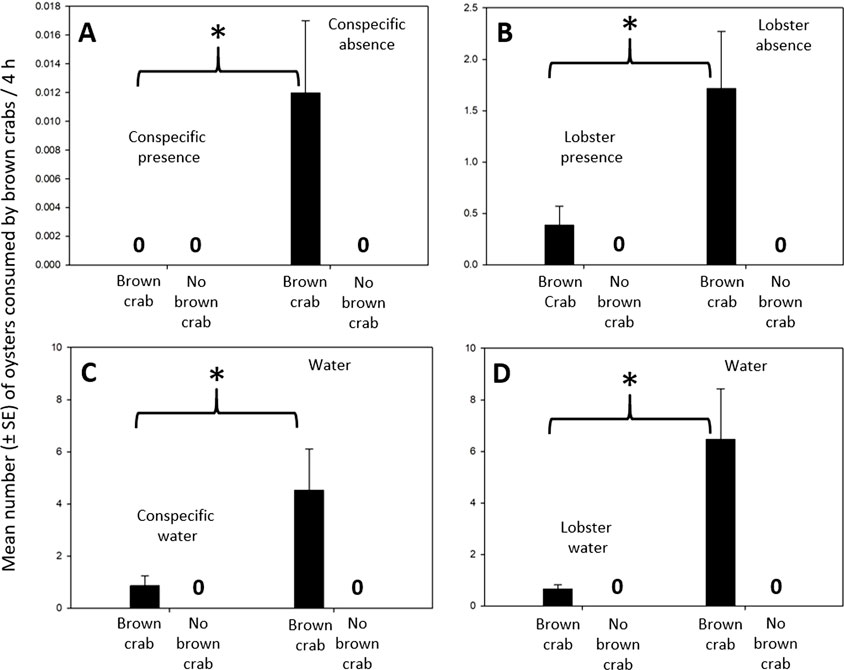
Figure 5. Oyster consumption of brown crabs in predator presence and absence, predator water and water. (A) Oyster consumption of crabs was lower in conspecific presence than in conspecific absence. (B) Oyster consumption of crabs was lower in lobster presence than in lobster absence. (C) Oyster consumption of crabs was lower in conspecific water than in water. (D) Oyster consumption of crabs was lower in lobster water than in water. An “asterisk” indicates a significant difference (p < 0.05) between two corresponding bars. A “0” indicates that no oysters were consumed by the crabs (in “brown crab” treatments) or that no oysters died (in “no brown crab” treatments/controls).
Lobster presence reduced crab consumption of medium-sized oysters by 77% (Exp15: Student’s t-test: t10 = 2.74, p < 0.021, Hedge’s g = 1.334; Figure 5B). No oyster mortality occurred in the controls. Therefore, lobster presence did not affect oyster survival (Figure 5B). Additionally, we observed that crabs kept in lobster presence were rather weak towards the end of the experiment as these crabs did not hold on to their cages during our procedural controls implying the physiological consequences for crabs being exposed to lobsters.
3.2.5 Effects of conspecific and lobster water on oyster consumption of brown crabs
Conspecific water reduced crab consumption of small oysters by 81% (Exp16: Student’s t-test: t18 = 2.27, p < 0.036, Hedge’s g = 1.013; Figure 5C). No oyster mortality occurred in the controls (Figure 5C). Thus, waterborne cues released by conspecifics reduced crab predation on oysters but did not influence oyster survival.
Lobster water reduced crab consumption of small oysters by 89% (Exp17: Welch’s t-test: t9 = 3.14, p < 0.012, Hedge’s g = 1.406; Figure 5D). There was no oyster mortality in the controls (Figure 5D). Thus, lobster nonconsumptive limitation of crab predation was mediated by lobster waterborne cues and such cues do not influence oyster survival.
4 Discussion
Within the framework of the project RESTORE on the restoration of European oyster reef habitat in the German North Sea (Pogoda et al., 2024), this study investigated, for the first time, consumptive and nonconsumptive interactions among common North Sea predators, brown crabs and European lobsters, and European oysters. As predators can control prey populations (Menge et al., 2023; Meira et al., 2024; Johnson and Smee, 2014; Pickering et al., 2017) and information on interactions between predators and European oysters is largely missing (Mascaró and Seed, 2001; OSPAR, 2023), our results provide fundamental knowledge of predator-prey interactions in offshore oyster reefs that is crucial for oyster reef restoration in European waters (zu Ermgassen et al., 2021).
4.1 Brown crabs, lobsters and oysters co-occur in offshore seafloor environments
The field surveys detected large brown crabs and a small lobster co-occurring in the two Borkum Reef Ground pilot oyster reefs (Figures 1A,B). Thus, mobile predators, that are considered early habitat colonizers (Kraufvelin et al., 2023; Smith et al., 2023) and typically occur on natural and artificial hard substrates (Krone et al., 2017; ter Hofstede et al., 2022; Krone and Schröder, 2011; Thatcher et al., 2023; Helgoland Lobster, 2022; Dybern, 1973), have colonized these reefs within 14 months (July 2020 to September 2021). Moreover, the occurrence of small brown crabs (4–5 cm CW) in oyster baskets (0.6 cm mesh width) next to the reefs (Section 3.1.1) indicates that crab megalopa larvae (0.2–0.4 cm CL (Ingle, 1981)) settling from the plankton (Sheehy and Prior, 2009) and the resulting tiny juvenile crabs (≥0.2 cm CW (Ingle, 1981)), that use such structurally rich habitats as protective nurseries (Mesquita et al., 2021), became recruited to the reef surroundings during that period. We also note that small and large lobsters were observed with the monitored oysters in Helgoland harbor (Section 3.1.2) and that large brown crabs and large lobsters were seen on (or near) both sandstone reefs and around oyster baskets kept on benthic landers off Helgoland 1 day and 22 days after sandstone reef deployment (Section 3.1.3). These findings show that early and later stages of oyster reef restoration projects are subject to predators from small to large sizes.
4.2 Brown crabs and lobsters interact with oysters and consume them in the field
As lab experiments found that small brown crabs consume small oysters (Mascaró and Seed, 2001) and small to large brown crabs occurred in the pilot oyster reefs (Section 3.1.1), we examined whether these predators consume small to large oysters. Our experiments confirmed that small brown crabs consume small oysters (Figures 5C,D) and found, for the first time, that large brown crabs consume small (Figures 2A–C), somewhat larger (Figure 2B) and medium-sized (Figure 2D) but no large oysters (Figures 2C,D). Moreover, our field observations off Helgoland showed that very large brown crabs consumed even oyster spat-on-reef by chipping cemented oyster spat off the sandstone reef (Section 3.1.3). These findings show that brown crabs consume small to medium-sized oysters.
It was also examined whether predators interact with medium-sized oysters by deploying a camera-monitored oyster-lander on the Helgoland harbor seafloor (Section 2.1.2) which showed that at least one large lobster interacted with the oysters and consumed them in the field (Figure 1C). Interestingly, previous field observations in the Oslofjord (Norway) showed that lobsters transport their prey to their shelters for later consumption (Steen and Ski, 2014). However, as our camera was mounted relatively close to the lander, we could not determine whether all oysters were transported away from the lander by one or more lobsters and whether these oysters were consumed. Still, the three (i-iii) facts (i) that all six lobsters caught for the experiments in June 2023 were trapped within a relatively small area (approximately 25,000 m2) right outside Helgoland harbor within 1 day (Section 3.1.2), (ii) that we counted four large lobsters in proximity to each other near benthic landers off Helgoland in August 2023 (Section 3.1.3), and (iii) that territorial lobster individuals show overlapping home ranges elsewhere in the North Sea (Stamp et al., 2024; Moland et al., 2011; Skerritt et al., 2015) suggests that the Helgoland harbor lander was visited by more than one lobster. Similar to the aforementioned Oslofjord observations (Steen and Ski, 2014), we saw that the lobsters in the mesocosms collected oysters from the floors and stored them along the walls for later consumption (Section 3.2.1). We also observed one small lobster13 feeding on small oysters in an outdoor tank (Section 3.2.1) and another small lobster attempting to feed on the oyster spat-on-reef off Helgoland (Section 3.1.3). Additionally, we note that most lobster-oyster interactions (77%) in the Helgoland harbor were observed under dim daylight conditions within 2 h after sunrise (7–9 a.m.) and sunset (7–9 p.m.; Supplementary Figure S12) (Sunrise and Sunset Helgoland, 2024) which corresponds with the current opinion that lobsters are no nocturnal but primarily crepuscular predators (Davenport et al., 2023). Overall, these findings show that lobsters interact with small to medium-sized oysters and consume them in the lab and in the field. Accordingly, predation by brown crabs and lobsters poses a risk for small to medium-sized oysters in restoration projects almost instantly after reef deployment.
4.3 Prey size refuges: medium-sized to large oysters are safe from brown crabs and large oysters are relatively safe from lobsters
Our lab experiments showed that large crabs consumed on average (±SE) 3.80 ± 1.06 (Figure 2C) to 8.30 ± 0.58 small oysters (Figure 2A) and 6.89 ± 1.02 somewhat larger oysters (Figure 2B) within 4 h. In contrast, large crabs consumed only 0.01 ± 0.01 oysters (2–5 cm SL; Figure 3D) and 0.01 ± 0.32 medium-sized oysters within the same time (Figure 2D). Thus, large crabs consumed 380 to 830 times more small oysters and 689 times more somewhat larger oysters than medium-sized oysters. We also repeatedly found that large crabs did not consume large oysters (Figures 2C, D), even over an 11-day experiment (Figure 2D; Supplementary Figure S4). These results indicate strong crab predation pressure on smaller oysters. Previous lab work with small brown crabs (2–4 cm CW) and small European oysters (0.3–3 cm SL) had shown that small crabs consumed small oysters (0.3–2.1 cm SL) but did not consume larger oysters (2.1–3 cm SL) (Mascaró and Seed, 2001). When such crabs were offered mussels, Pacific oysters or cockles (Cerastoderma edule) of similar sizes (0.2–3 cm SL), crabs consistently consumed smaller prey individuals (mussels: 0.4–2.4 cm SL; Pacific oysters: 0.3–2.4 cm SL; cockles: 0.2–2.1 cm SL) (Mascaró and Seed, 2001). Likewise, crabs (7.8–15 cm CW) offered horse mussels (Modiolus modiolus, 0.7–9.9 cm SL) preferred smaller horse mussels (1.5–5.9 cm SL) (Seed et al., 1975). Also, crabs (6.1–14.1 cm CW) fed scallops (Pecten maximus, 3.0–9.0 cm SL) preferentially consumed smaller scallops (3.0–4.0 cm SL) (Lake et al., 1987). Furthermore, crabs (7.6–8.6 cm CW) offered dogwhelks (Nucella lapillus, 1.0–2.5 cm SL) preferred smaller dogwhelks (1.0–1.5 cm SL), and crabs (6.4–9.8 cm CW) offered periwinkles (Littorina littorea, 1.0–3.0 cm SL) preferentially ate smaller periwinkles (1.0–2.0 cm SL) (Lawton and Hughes, 1985). All these preferences support the notion that brown crabs consistently select smaller bivalve and other molluscan prey to limit prey handling time to reduce risk of competition and predation (Juanes, 1992; Seed and Hughes, 1995) and to avoid claw wear and damage resulting from handling larger prey that can lead to energy loss, poorer agility and defense ability (Juanes, 1992; Juanes and Hartwick, 1990). Altogether, these results clearly show that brown crabs strongly prefer smaller oysters (and other small molluscs), whereas their consumption of medium-sized and large oysters is negligible.
Concerning lobsters, a positive (but flattening) correlation between predator size and prey size preference appears plausible. For example, we observed that a small lobster13 readily consumed all offered small and somewhat larger oysters within the first night of both experiments, whereas large lobsters14-18 did not consume such small oyster sizes over two consecutive 7-day experiments (Section 3.2.1; Figure 2E). We also found that large lobsters1-12 strongly preferred medium-sized oysters over large oysters (Figure 2F). Similarly, small American lobsters (Homarus americanus, 5.5–9.8 cm CL) offered American oysters (1.0–3.5 cm SL) preferred small (1.0–2.5 cm SL) over larger oysters (Elner and Lavoie, 1983). Information on European lobster preferences for sizes in other prey species (e.g., bivalves and snails (Hallbäck and Warén, 1972)) seemingly does not exist. However, prey size preferences in other lobster species, including American, Californian (Panulirus interruptus), Caribbean (Panulirus argus), New Zealand (Jasus edwarsii) and South African lobsters (Jasus lalandii), often increase with lobster size (Elner and Jamieson, 1979; Griffiths and Seiderer, 1980; Tegner and Levin, 1983; Robles et al., 1990; James and Tong, 1998; van Zyl et al., 1998; Sainte-Marie and Chabot, 2002; Hanson, 2009; Eurich et al., 2014; Gnanalingam and Butler IV, 2018) which resembles our findings and likely reflects the changing ability of lobsters to handle prey up to a certain prey size as lobsters grow (Boudreau and Worm, 2012). Overall, these findings indicate that medium-sized to large oysters have reached a prey size refuge from brown crab but not lobster predation, and that large oysters experience lower lobster predation.
4.4 Alternative prey can reduce predation pressure on oysters
Large crabs offered mussels and slightly smaller oysters simultaneously (Figure 3A) consumed mussels but did not consume oysters (Figure 3B). Likewise, small crabs preferred small mussels over similar-sized oysters (Mascaró and Seed, 2001). These preferences for mussels are probably driven by mussels being a more profitable prey than oysters. For instance, our mussel shells were thinner than our oyster shells at the shell lip (Figure 3E), center (Figure 3F) and base (Figure 3G) and, thus, easier to crack. Mussels also have a somewhat higher energy content (range: 5.30–5.66 cal/mg ash-free dry weight; AFDW) than oysters (5.21 cal/mg AFDW) (Beukema et al., 1997) and our mussels contained 11 times as much flesh as our oysters (Figure 3H) indicating that our mussels were more nutritious than our oysters. When offered mussels and oysters separately, crabs consumed almost 15 times as many mussels as oysters within the same time period (Figures 3C,D). Furthermore, crabs started consuming mussels already on the first day of the experiment but did not consume any oysters before the third day (Section 3.2.2) showing that prey handling time for mussels is shorter than for oysters. Accordingly, we estimated that our mussels (0.8033 g/d; average tissue dry weight/estimated handling time of 1 day) were 34 times as profitable as our oysters (0.023311 g/d; average tissue dry weight/estimated handling time of 3 days). Similarly, preferences of invasive green crabs and native red rock crabs (Cancer irroratus) in Prince Edward Island (PEI, Atlantic Canada) for soft-shell clams (Mya arenaria) and mussels over American oysters (Miron et al., 2005; Pickering and Quijón, 2011) were negatively related to shell thickness differences among these three bivalve species (Pickering and Quijón, 2011). Finally, we note that small crabs did neither prefer small mussels over similar-sized cockles nor small Pacific oysters over similar-sized European oysters (Mascaró and Seed, 2001) and that lobsters (based on stomach content analyses) tend to prefer crab, snail, and polychaete prey over bivalve prey (Hallbäck and Warén, 1972) but did not prefer medium-sized Pacific oysters over similar-sized European oysters (Figure 4D). Considered together, these findings indicate that prey profitability drives brown crab preferences for prey and that the presence of alternative prey, such as mussels, cockles and Pacific oysters, can reduce predation pressure on European oysters.
4.5 Clumping protects Pacific oysters from predation
Individual oysters can form oyster clumps by overgrowing conspecifics (Reise et al., 2017a; Merk et al., 2020). Such oyster clumps are typically larger than individual oysters and may, thus, have reached a prey size refuge from predation. To test this prediction, we conducted an experiment in which lobsters were offered individual medium-sized Pacific oysters and larger Pacific oyster clumps (Section 2.3.3). We used Pacific oysters, which are widespread in the North Sea (Troost, 2010; Reise et al., 2017b) and common around Helgoland (Zwerschke et al., 2013; Ellrich et al., 2023), as model organisms because European oyster clumps were not available. Our 6.5-day experiment found that lobsters preferred individual oysters over oyster clumps (Figure 4A) and that lobsters barely consumed clumped oysters. Actually, only a few oyster clumps showed traces of lobster attacks during which only oysters protruding from the clumps were consumed (Figure 4A) likely as the clumps were larger and heavier than the individual oysters (Figures 4B, C) and, thus, more difficult for the lobsters to handle. These results indicate that oyster clumps are relatively safe from large predators. Interestingly, paired juvenile American oysters (i.e., two small individuals grown together) in PEI experienced lower predation by invasive green crabs than individual juvenile American oysters (Poirier and Quijón, 2022). We also found that lobsters do not prefer medium-sized Pacific over similar-sized European oysters (Figure 4D) suggesting that European oyster clumps are also safer from predation than individual European oysters which should be examined through future manipulative experiments.
4.6 Brown crab and lobster presence regulate and limit oyster consumption of brown crabs under natural predator abundances
As cannibalism occurs among brown crabs (Lawton, 1989; Amaral et al., 2009), lobsters prey on brown crabs (Hallbäck and Warén, 1972) and predator presence often reduces prey feeding activity since prey typically ceases feeding to decrease predation risk (Trussell et al., 2003; Molis et al., 2011; Pessarrodona et al., 2019; Catalán et al., 2021; McCall et al., 2023; Curtis and Wing, 2024), we examined whether such nonconsumptive effects (NCEs) by conspecifics and lobsters would reduce oyster consumption of crabs (Section 2.3.4). Working with a crab abundance in the mesocosms (2.4 crabs/m2) which closely corresponded with the natural crab abundance in the pilot oyster reefs (2.0–3.1 crabs/m2; Section 3.1.1), we found that conspecific presence essentially “switched off” crab consumption of medium-sized oyster (Figure 5A). This shows that the presence of cannibalistic conspecifics heavily diminishes oyster consumption of crabs likely as the crabs spend more time avoiding conspecifics when detecting conspecific cues. Interestingly, mutualistic interferences among interacting conspecifics can also limit prey consumption of brown crabs and, thereby, cause a disproportional increase in prey consumption with crab density (Amaral et al., 2009). Thus, predator NCEs (this study) and mutualistic interferences (Amaral et al., 2009) are two different mechanisms underlying such a disproportional increase that ultimately facilitates prey survival. In contrast, such a disproportional increase may not occur among non-cannibalistic predators. This notion is supported by field experiments which showed a positive relationship between the number of adult predatory dogwhelks (that only show slight cannibalistic tendencies as juveniles (Largen, 1967; Crothers, 1985; Gul’bin and Shadrin, 1991; Gosselin and Chia, 1994)) and the number of mussels consumed by them (Meister et al., 2023). The presence of only one lobster also limited brown crab consumption of medium-sized oysters by 77% (Figure 5B) which indicates that even individual lobsters, like the one in the eastern pilot oyster reef (Figure 1B), contribute to nonconsumptive predator regulation and limitation of brown crab feeding activity in the field. Finally, we detected that conspecific presence (Hedge’s g = 1.137) and lobster presence (g = 1.334) had similarly large negative effects on oyster consumption of crabs. However, as predator NCEs on prey typically increase with predator abundance (Silberbush and Blaustein, 2011; Hill and Weissburg, 2013; Ellrich et al., 2015; Büchner-Miranda et al., 2024) and both mesocosm experiments (Exp14, 15) used different predator abundances (i.e., three crabs/mesocosm versus one lobster/mesocosm), these findings suggest that individual crab NCEs are likely somewhat weaker than the detected individual lobster NCEs. Overall, these results show that brown crab and lobster presence can regulate and limit oyster consumption of brown crabs under natural predator abundances and suggest (in combination with the strong brown crab preferences for small and somewhat larger oysters; Section 4.3) that medium-sized and larger oysters are safe from brown crab predation when exposed to such natural brown crab and lobster abundances.
4.7 Waterborne brown crab and lobster cues mediate predator nonconsumptive regulation and limitation of brown crab predation
Waterborne cues released by brown crabs and lobsters trigger defense responses in mussels (Côté, 1995; Côté and Jelnikar, 1999; Wang et al., 2024). Therefore, we examined whether the detected conspecific and lobster NCEs on oyster consumption of brown crabs (Figures 5A, B) are mediated by such cues. For that, we measured oyster consumption of brown crabs in conspecific water, lobster water and water (Section 2.3.5). Conspecific and lobster water reduced oyster consumption of crabs by 81% and 89%, respectively (Figures 5C, D), indicating that waterborne conspecific and lobster cues mediate the detected nonconsumptive regulation and limitation of crab predation. Conspecific and lobster water had large effects on oyster consumption of crabs (conspecific water: Hedge’s g = 1.013; lobster water: g = 1.406). Yet, predator NCEs on prey intensify with predator cue concentration (Büchner-Miranda et al., 2024; Loose and Dawidowicz, 1994; von Elert and Pohnert, 2000; Ferland-Raymond et al., 2010) and we used three crabs but only one lobster to produce conspecific and lobster water (Section 2.3.5). This suggests, as the aforementioned effects of conspecific and lobster presence on crab predation (Section 4.6), that individual crab NCEs are likely somewhat weaker than the detected individual lobster NCEs. This conclusion is corroborated by our two pilot studies which found that crab water, that derived from three crabs, had a weaker effect on mussel byssal thread production (g = 1.373, Supplementary Figure S1A) than lobster water from an individual lobster (g = 1.884, Supplementary Figure S1B). Finally, predator NCEs on prey can also be triggered by tactile and visual predator cues (Tapia-Lewin and Pardo, 2014; Boudreau et al., 2018). However, the effects of conspecific presence (g = 1.137) and conspecific water (g = 1.013) as well as lobster presence (g = 1.334) and lobster water (g = 1.406) on oyster consumption of crabs were quite similar which further corroborates that the detected predator NCEs were mediated by waterborne predator cues.
4.8 Applicability for other predator-prey systems and restoration projects in Europe and beyond
Although our study focused on consumptive and nonconsumptive interactions among common predators and European oysters in the North Sea, we note that brown crabs and lobsters are NE Atlantic predators that occur from northern Norway (Europe) to Western Sahara (northern Africa) (González, 2022; FAO, 2023a) which encompasses the entire historic European oyster distribution range from southern Norway to southern Spain (Pogoda, 2019; Thurstan et al., 2024) where several oyster reef restoration projects are currently underway (Pogoda, 2019; Pogoda et al., 2024; zu Ermgassen et al., 2021). Accordingly, our findings provide fundamental knowledge of predator-prey interactions that are relevant for oyster reef restoration in Europe.
Prey size refuges may protect oysters also from other common shell crushing NE Atlantic predators such as juvenile green crabs, that interacted with juvenile oysters and preferred small over large juvenile oysters in lab experiments (Mascaró and Seed, 2001), and swimming crabs (Liocarcinus spp.), that prey on thin-shelled bivalves (Choy, 1986) and were, for the first time, observed attempting to prey on oyster spat-on-reef off Helgoland (Section 3.1.3). This conclusion is corroborated by the seven (i–vii) findings in North America which indicated (i, ii) that larger American oysters (≥5.5 cm SL) showed lower mortalities when exposed to invasive green crabs and native red rock crabs than smaller American oysters (≤5.5 cm SL) (Poirier et al., 2017), (iii, iv) that large American oysters (2.5–4.0 cm SL) were not consumed by these two predators when offered together with small (≤1.5 cm SL) and medium-sized American oysters (1.5–2.5 cm SL) (Miron et al., 2005), (v) that medium-sized (2.5–3.5 cm SL) and large American oysters (3.5–5.5 cm SL) showed lower mortalities under green crab predation than small American oysters (1.5–2.5 cm SL) (Pickering et al., 2017; Tummon Flynn et al., 2015) with (vi) large oysters not being consumed by green crabs (Pickering et al., 2017), and (vii) that medium-sized American oysters (2.5–3.5 cm SL) suffered lower mortality from green crab predation than small American oysters (1.5–2.5 cm SL) (Poirier and Quijón, 2022). Accordingly, larger European oysters are likely safe from predation by green crabs and swimming crabs that have similar claw morphologies, claw strengths and shell crushing methods (ap Rheinallt and Hughes, 1985). Also, the presence of brown crabs and lobsters, that prey on green crabs and other predatory crab species (Hallbäck and Warén, 1972; Lawton, 1989) and show interspecific agnostic behaviour towards other predatory crab species (Davenport et al., 2023), may regulate and limit European oyster consumption by other predatory crab species, such as green crabs, through predator consumptive and nonconsumptive effects.
Our results also provide important information for other shellfish restoration projects in Europe (Carranza and zu Ermgassen, 2020; Fitzsimons et al., 2019) focusing, for example, on declining horse mussel populations (Strong et al., 2015; Fariñas-Franco and Roberts, 2017) in Denmark (BioReef, 2024) and Northern Ireland (Modiolus Restoration Research Group, 2024) whose subtidal mussel beds are biodiversity hotspots across northern European waters (Fariñas-Franco et al., 2023). Brown crabs and lobsters consume horse mussels (Hallbäck and Warén, 1972; Shelton et al., 1979; Seed et al., 1975) and brown crabs prefer small over larger horse mussels (Seed et al., 1975). Thus, prey size refuges and predator consumptive and nonconsumptive effects may limit predation on other shellfish habitats under restoration, such as horse mussel beds, as well.
Finally, as green crabs and red rock crabs have cannibalistic tendencies (Ojeda and Dearborn, 1991; Stehlik, 1993; Baeta et al., 2006; Chaves et al., 2010; Rondeau et al., 2014; Gehrels et al., 2017; Geburzi et al., 2018) and green crab ethograms show intraspecific agonistic behaviour and intraspecific kleptoparasitism (Davenport et al., 2023), it would be interesting to investigate whether mutualistic interferences among interacting conspecifics (Amaral et al., 2009), conspecific presence and waterborne cues (this study) also regulate and limit American oyster consumption by green crabs and red rock crabs and, thereby, facilitate American oyster survival in the field.
4.9 Recommendations for reef restoration and future research: measures to regulate predation
Based on this study, we recommend to identify predator-prey relationships and the corresponding predator-prey interactions to understand offshore reef community ecology and to develop and test measures regulating predation in ecological reef restoration. As first steps, literature reviews and field surveys should determine predators, prey, alternative prey and top-predators (that prey on predators) occurring in the respective region. In Europe, these can include, for example, green crabs and brown crabs (predators), oysters (prey), mussels (alternative prey) and lobsters (Mascaró and Seed, 2001, this study) or large fish such as skates or rays (top-predators) (Poiesz et al., 2021). Next, field observations and manipulative experiments should establish the predator hierarchy and corresponding predator-prey interactions (this study). Building on this, measures for natural regulation of predation (increasing in complexity concerning feasibility and efficiency) can be developed: from one-species (oyster) over two-species (oyster, mussel) to multi-species approaches (oyster, mussel, lobster, fish). To test and compare these approaches, we suggest to monitor them with regard to reef development over time (Pineda-Metz et al., 2023; zu Ermgassen et al., 2021).
For the one-species approach and as oyster reef building blocks, we recommend to use larger individual oysters which have reached a size refuge protecting them from predators (i.e., ≥7 cm oyster SL for brown crabs, ≥12 cm oyster SL for lobsters, Section 4.3) and oyster spat-on-large-shells or oyster clumps (spat-on-clumps) as oyster size (Section 4.3) and clumping reduced predation pressure (Section 4.5). These larger oyster sizes certainly mean higher economic costs due to more effort and longer production phases for oyster hatcheries and nurseries. However, predators consistently preferred crushing the shells of small oysters and other molluscan prey (Sections 4.3, 4.5, 4.8). Accordingly, larger single oysters, spat-on-large-shells and spat-on-clumps are likely better protected from shell-crushing predators than small and single seed oysters. Using them in restoration projects, should, therefore, increase oyster survival in the field and oyster reef restoration success in the long-term.
Concerning the two-species approach, we suggest to add an alternative prey, such as mussels (Section 4.4), if native to the restoration region. As mussel beds as biodiversity hotspots are also declining (Meister et al., 2023; Banke et al., 2024; Little et al., 2024; Cameron and Scrosati, 2023), the ecological restoration of both systems, oyster reefs and mussel beds, can go hand in hand where applicable and ecologically relevant. Some predators should prefer mussels as more profitable alternative prey over oysters (Section 4.4), so that the alternative prey can distract and nourish these predators and, thereby, regulate and limit predation pressure on oyster reefs.
Finally, regarding the multi-species approach, we recommend to include native top-predators (if not already present), such as European lobsters or large fish, such as skates, that prey on predators, tend to prefer crab, snail and polychaete prey over bivalve prey (Section 4.4) and limit oyster consumption of predators, in the case of lobsters also through predator NCEs (Section 4.6) mediated by waterborne predator cues (Section 4.7).
4.10 Summary and conclusions
We conclude that common brown crabs and European lobsters are predators of European oysters and that oyster size refuges, the presence of alternative prey, oyster clumping, as well as intra- and interspecific regulation and limitation of oyster consumption by predators mediated through predator NCEs, that are triggered by waterborne predator cues, can regulate and reduce predation pressure on European oysters. Since brown crabs and lobsters co-occur across the entire historic European oyster distribution range (from southern Norway to southern Portugal) where several oyster reef restoration projects are conducted, our study provides fundamental knowledge of predator-prey interactions that is required for developing measures to regulate and reduce oyster predation and increase oyster reef restoration success in European waters.
Data availability statement
The original contributions presented in the study are included in the article/Supplementary Material, further inquiries can be directed to the corresponding author.
Ethics statement
The animal study was approved by Professor Dr. Philipp Fischer, Biologische Anstalt Helgoland, Alfred Wegener Institute Helmholtz Centre for Polar and Marine Research, 27498 Helgoland, Germany. The study was conducted in accordance with the local legislation and institutional requirements.
Author contributions
JE: Conceptualization, Data curation, Formal Analysis, Investigation, Methodology, Project administration, Resources, Supervision, Visualization, Writing–original draft. CK-F: Data curation, Investigation, Writing–review and editing. MB: Data curation, Formal Analysis, Investigation, Methodology, Supervision, Writing–review and editing. BC: Funding acquisition, Resources, Writing–review and editing. BP: Conceptualization, Funding acquisition, Project administration, Resources, Supervision, Writing–review and editing.
Funding
The author(s) declare that financial support was received for the research, authorship, and/or publication of this article. This study was conducted within the framework of the research and development project RESTORE Wissenschaftliche Begleitung (15 August 2020 – 15 August 2025; FKZ: 3520892013) with funds provided by the German Federal Agency for Nature Conservation (BfN).
Acknowledgments
We thank the two reviewers and Markus Molis (The Arctic University of Norway, UiT) for helpful comments on our manuscript, Anna-Lena Bohlen (Alfred Wegener Institute for Polar and Marine Research, AWI), Tanja Hausen (AWI), Silke Henkel (AWI), Stefanie Klüver (AWI), Marcus Meierdierks (AWI), Miriam C. Niewöhner (Carl von Ossietzky University), and Corina Peter (AWI) for logistic support, Santiago E. A. Pineda-Metz (AWI) for conducting the predator-oyster monitoring in Helgoland harbor, Katja Fiegener (Carl von Ossietzky University), Ulrich Hoge (AWI), Johannes Lemburg (AWI), Matthias Littmann (AWI) and Andreas Wagner (AWI) for technical support, Nicolás Araujo Piñeiro (AWI), Lennard Klingforth (AWI), Dominique C. Noetzel (University of Rostock) and Martin Sackmann (AWI) for oyster husbandry support, the AWI Scientific Diving Team for diving support, Ute Kieb (AWI) and Uwe Nettelmann (AWI) for providing brown crabs, Dieter Klings (AWI) and Kai Siemens (AWI) for ship support and Nicholas J. Mackay-Roberts (AWI) and Gunnar Gerdts (AWI) for letting us use the mesocosms.
Conflict of interest
The authors declare that the research was conducted in the absence of any commercial or financial relationships that could be construed as a potential conflict of interest.
Generative AI statement
The author(s) declare that no Generative AI was used in the creation of this manuscript.
Publisher’s note
All claims expressed in this article are solely those of the authors and do not necessarily represent those of their affiliated organizations, or those of the publisher, the editors and the reviewers. Any product that may be evaluated in this article, or claim that may be made by its manufacturer, is not guaranteed or endorsed by the publisher.
Supplementary material
The Supplementary Material for this article can be found online at: https://www.frontiersin.org/articles/10.3389/fenvs.2025.1509318/full#supplementary-material
References
Amaral, V., Paula, J., Hawkins, S., and Jenkins, S. (2009). Cannibalistic interactions in two co-occurring decapod species: effects of density, food, alternative prey and habitat. J. Exp. Mar. Biol. Ecol. 368, 88–93. doi:10.1016/j.jembe.2008.10.025
ap Rheinallt, T., and Hughes, R. N. (1985). Handling methods used by the velvet swimming crab Liocarcinus puber when feeding on molluscs and shore crabs. Mar. Ecol. Prog. Ser. 25, 63–70. doi:10.3354/meps025063
Baeta, A., Cabral, H. N., Marques, J. C., and Pardal, M. A. (2006). Feeding ecology of the green crab, Carcinus maenas (L., 1758) in a temperate estuary, Portugal. Crustaceana 79, 1181–1193. doi:10.1163/156854006778859506
Banke, T. L., Steinfurth, R. C., Lange, T., Canal-Vergés, P., Svane, N., and Flindt, M. R. (2024). Dislodgement and mortality challenges when restoring shallow mussel beds (Mytilus edulis) in a Danish Estuary. Restor. Ecol. 32, e14160. doi:10.1111/rec.14160
Beukema, J. J. (1997). “Caloric values of marine invertebrates with an emphasis on the soft parts of marine bivalves,” in Oceanography and marine biology. An annual review. Editors H. Barnes, A. D. Ansell, R. N. Gibson, and M. Barnes, 35, 407–435. doi:10.1201/b12590
BfN (2024a). “Bundesamt für Naturschutz. Rahmenvorgaben Kompensationsmaßahmen – BfN-Maßnahmendatenblatt Europäische Auster Ostrea edulis,” in Wiederansiedlung der Europäischen Auster im Naturschutzgebiet „Borkum Riffgrund“. Februar 2024. Bonn, Germany. doi:10.19217/brs244
BioReef (2024). BioReef: marine restoration with WWF and DTU Aqua. Available at: https://orsted.com/en/who-we-are/sustainability/nature/net-positive-biodiversity-impact/working-with-wwf-for-ocean-biodiversity/bioreef (Accessed November 24, 2024).
Boudreau, M. L., Scrosati, R. A., and Wong, M. C. (2018). Predator (Carcinus maenas) nonconsumptive limitation of prey (Nucella lapillus) feeding depends on prey density and predator cue type. J. Ethol. 36, 259–264. doi:10.1007/s10164-018-0557-9
Boudreau, S. A., and Worm, B. (2012). Ecological role of large benthic decapods in marine ecosystems: a review. Mar. Ecol. Prog. Ser. 469, 195–213. doi:10.3354/meps09862
Bundesamt für Naturschutz. Rahmenvorgaben Kompensationsmaßahmen – BfN-Maßnahmendatenblatt Wiederherstellung geogener Riffe in der deutschen AWZ der Nordsee. Teilbereich: Naturschutzgebiet Borkum Riffgrund. Bonn, Germany. doi:10.19217/brs246
Büchner-Miranda, J. A., Salas-Yaquin, L. P., Valdivia, N., Scrosati, R. A., Riedemann-Saldivia, B., Cubillos, V. M., et al. (2024). Responses of mussels (Perumytilus purpuratus) to waterborne exudate cues from predatory snails (Acanthina monodon) depend on cue intensity. Mar. Biol. 171, 107. doi:10.1007/s00227-024-04419-0
Cameron, N. M., and Scrosati, R. A. (2023). Mass disappearance of intertidal mussels after an unusual winter cold snap in eastern Canada. Ecology 104, e4179. doi:10.1002/ecy.4179
Cannon, D., Kibler, K., Walters, L., and Chambers, L. (2022). Hydrodynamic and biogeochemical evolution of a restored intertidal oyster (Crassostrea virginica) reef. Sci. Total Environ. 831, 154879. doi:10.1016/j.scitotenv.2022.154879
Carranza, A., and zu Ermgassen, P. (2020). A global overview of restorative shellfish mariculture. Front. Mar. Sci. 7, 722. doi:10.3389/fmars.2020.00722
Catalán, A. M., Büchner-Miranda, J., Riedemann, B., Chaparro, O. R., Valdivia, N., and Scrosati, R. A. (2021). Community-wide consequences of nonconsumptive predator effects on a foundation species. J. Anim. Ecol. 90, 1307–1316. doi:10.1111/1365-2656.13455
Chaves, M. L., Horta, M. S., Chainho, P., Costa, M. J., and Costa, J. L. (2010). New additions to the feeding ecology of Carcinus maenas (L., 1758) in a South-western Europe estuary (Portugal). Cah. Biol. Mar. 51, 229–238. doi:10.21411/CBM.A.86A0CE1C
Choy, S. C. (1986). Natural diet and feeding habits of the crabs Liocarcinus puber and L. holsatus (Decapoda, Branchyura, Portunidae). Mar. Ecol. Prog. Ser. 31, 87–99. doi:10.3354/meps031087
Colsoul, B., Pouvreau, S., Di Poi, C., Pouil, S., Merk, V., Peter, C., et al. (2019). Addressing critical limitations of oyster (Ostrea edulis) restoration: identification of nature-based substrates for hatchery production and recruitment in the field. Aquat. Conserv. Mar. Freshw. Ecosyst. 30, 2101–2115. doi:10.1002/aqc.3454
Conan, G. Y., Maynard, D. R., Merrien, A., and Guennegan, Y. (1984). “Monitoring the foraging activity of lobsters and associated species by underwater television,” ICES Shellfish Committee C. M. 1984/K: 45, 13 p.
Côté, I. M. (1995). Effects of predatory crab effluent on byssus production in mussels. J. Exp. Mar. Biol. Ecol. 188, 233–241. doi:10.1016/0022-0981(94)00197-L
Côté, I. M., and Jelnikar, E. (1999). Predator-induced clumping behaviour in mussels (Mytilus edulis Linnaeus). J. Exp. Mar. Biol. Ecol. 235, 201–211. doi:10.1016/S0022-0981(98)00155-5
Crothers, J. H. (1985). Dog-whelks: an introduction to the biology of Nucella lapillus (L.). Field Stud. 6, 291–360.
Curtis, J. S., and Wing, S. R. (2024). Size-specific reduction in kelp consumption by New Zealand urchins exposed to chemical cues from the red rock lobster. Ecosphere 15, e4932. doi:10.1002/ecs2.4932
Davenport, J., Jessopp, M., Harman, L., Micaroni, V., and McAllen, R. (2023). Feeding, agonistic and cooperative behavioural responses of shallow water benthic marine scavengers. J. Nat. Hist. 57, 1049–1065. doi:10.1080/00222933.2023.2226372
Dybern, B. I. (1973). Lobster burrows in Swedish waters. Helgoländer Wiss. Meeresunters 24, 401–414. doi:10.1007/BF01609529
Ellrich, J. A., Ehlers, S. M., Furukuma, S., Pogoda, B., and Koop, J. H. E. (2023). Characterization of three plastic forms: plasticoncrete, plastimetal and plastisessiles. Sci. Total Environ. 895, 165073. doi:10.1016/j.scitotenv.2023.165073
Ellrich, J. A., Scrosati, R. A., and Petzold, W. (2015). Predator density affects nonconsumptive predator limitation of prey recruitment: field experimental evidence. J. Exp. Mar. Biol. Ecol. 472, 72–76. doi:10.1016/j.jembe.2015.07.005
Elner, R. W., and Jamieson, G. S. (1979). Predation of sea scallops, Placopecten magellanicus, by the rock crab, Cancer irroratus, and the American lobster, Homarus americanus. J. Fish. Res. Board Can. 36, 537–543. doi:10.1139/f79-077
Elner, R. W., and Lavoie, R. E. (1983). Predation on American oysters (Crassostrea virginica [Gmelin]) by American lobsters (Homarus americanus Milne-Edwards), rock crabs (Cancer irroratus Say), and mud crabs (Neopanopa sayi [Smith]). J. Shellfish Res. 3, 129–134.
Eurich, J. G., Selden, R. L., and Warner, R. R. (2014). California spiny lobster preference for urchins from kelp forests: implications for urchin barren persistence. Mar. Ecol. Prog. Ser. 498, 217–225. doi:10.3354/meps10643
FAO (2023b). “Fisheries and Aquaculture,”Cult.Aqu. Spec. Inform. Program. Rome, Italy. Available at: https://www.fao.org/fishery/en/culturedspecies/ostrea_edulis?lang=en (Accessed November 24, 2024).
FAO (2023a). Food and agriculture organization of the united Nations. Cancer pagurus (Linnaeus, 1758). Available at: https://www.fao.org/fishery/en/aqspecies/2627/en (Accessed November 24, 2024).
Fariñas-Franco, J. M., Cook, R. L., Gell, F. R., Harries, D. B., Hirst, N., Kent, F., et al. (2023). Are we there yet? Management baselines and biodiversity indicators for the protection and restoration of subtidal bivalve shellfish habitats. Sci. Total Environ. 863, 161001. doi:10.1016/j.scitotenv.2022.161001
Fariñas-Franco, J. M., and Roberts, D. (2017). The relevance of reproduction and recruitment to the conservation and restoration of keystone marine invertebrates: a case study of sublittoral Modiolus modiolus reefs impacted by demersal fish. Aquatic. Conserv. Mar. Freshw. Ecosyst. 28, 672–689. doi:10.1002/aqc.2882
Ferland-Raymond, B., March, R. E., Metcalfe, C. D., and Murray, D. L. (2010). Prey detection of aquatic predators: assessing the identity of chemical cues eliciting prey behavioral plasticity. Biochem. Syst. Ecol. 38, 169–177. doi:10.1016/j.bse.2009.12.035
Ferrari, M. C. O., Wisenden, B. D., and Chivers, D. P. (2010). Chemical ecology of predator–prey interactions in aquatic ecosystems: a review and prospectus. Can. J. Zool. 88, 698–724. doi:10.1139/Z10-029
Fitzsimons, J. A., Branigan, S., Gillies, C. L., Brumbaugh, R. D., Cheng, J., DeAngelis, B. M., et al. (2019). Restoring shellfish reefs: global guidelines for practitioners and scientists. Conserv. Sci. Pract. 2, e198. doi:10.1111/csp2.198
Gann, G. D., McDonald, T., Walder, B., Aronson, J., Nelson, C. R., Jonson, J., et al. (2019). International principles and standards for the practice of ecological restoration. Second edition. Restore. Ecol. 27, 1–46. doi:10.1111/rec.13035
Geburzi, J. C., Brandis, D., and Buschbaum, C. (2018). Recruitment patterns, low cannibalism and reduced interspecific predation contribute to high invasion success of two Pacific crabs in northwestern Europe. Estuar. Coast. Shelf Sci. 200, 460–472. doi:10.1016/j.ecss.2017.11.032
Gehrels, H., Tummon Flynn, P., Cox, R., and Quijón, P. A. (2017). Effect of habitat complexity on cannibalism rates in European green crabs (Carcinus maenas Linnaeus, 1758). Mar. Ecol. 38, e12448. doi:10.1111/maec.12448
Gnanalingam, G., and Butler IV, M. J. (2018). Examining the relationship between size and feeding preferences in the Caribbean spiny lobster Panulirus argus (Latreille, 1804) (Decapoda: achelata: Palinuridae). J. Crustac. Biol. 38, 245–249. doi:10.1093/jcbiol/rux122
González, J. A. (2022). Southern distribution limit of the European lobster, Homarus gammarus (Decapoda, Nephropidae) on the coast of northwest Africa with remarks on its habitat, and new record from the Canary Islands. Crustaceana 95, 511–516. doi:10.1163/15685403-bja10198
Gosselin, L. A., and Chia, F.-S. (1994). Feeding habits of newly hatched juveniles of an intertidal predatory gastropod, Nucella emarginata (Deshayes). J. Exp. Mar. Biol. Ecol. 176, 1–13. doi:10.1016/0022-0981(94)90193-7
Griffiths, C. L., and Seiderer, J. L. (1980). Rock-lobsters and mussels – limitations and preferences in a predator-prey interaction. J. Exp. Mar. Biol. Ecol. 44, 95–109. doi:10.1016/0022-0981(80)90104-5
Grizzard, M., and Shaw, A. Z. (2017). Effect size. Int. Encycl. Commun. Res. Methods, 1–8. doi:10.1002/9781118901731.iecrm0076
Gul’bin, V. V., and Shadrin, N. V. (1991). Feeding ecology of the predatory gastropod Nucella heyseana in peter the great Bay, sea of Japan. Asian Mar. Biol. 8, 95–102.
Hall, S. J., Basford, D. J., Robertson, M. R., Raffaelli, D. G., and Tuck, I. (1991). Patterns of recolonization and the importance of pit-digging by the crab Cancer pagurus in a subtidal sand habitat. Mar. Ecol. Prog. Ser. 72, 93–102. doi:10.3354/meps072093
Hallbäck, H., and Warén, A. (1972). Food ecology of lobster, Homarus vulgaris, in Swedish waters. Some preliminary results. ICES Shellfish Committee C. M. 1972/K: 29, 11 p.
Hanson, J. M. (2009). Predator-prey interactions of American lobster (Homarus americanus) in the southern gulf of st. Lawrence, Canada. N. Z. J. Mar. Freshw. Res. 43, 69–88. doi:10.1080/00288330909509983
Helgoland lobster. (2022). Available at: https://www.helgoland-lobster.de/index.html [Accessed November 24, 2024].
Hill, J. M., and Weissburg, M. J. (2013). Predator biomass determines the magnitude of non-consumptive effects (NCEs) in both laboratory and field environments. Oecologia 172, 79–91. doi:10.1007/s00442-012-2488-4
Hinchcliffe, J., Agnalt, A.-L., Daniels, C. L., Drengstig, A., Lund, I., McMinn, J., et al. (2021). European lobster Homarus gammarus aquaculture: technical developments, opportunities and requirements. Rev. Aquacult. 14, 919–937. doi:10.1111/raq.12634
Holt, R. D. (2009). “Predation and community organization,” in The princeton guide to ecology. Editors S. A. Levins, S. R. Carpenter, H. Charles, J. Godfrey, A. P. Kinzig, M. Loreauet al. (Princeton, NJ: Princeton University Press), 274–281.
Ingle, R. W. (1981). The larval and post-larval development of the Edible Crab, Cancer pagurus Linnaeus (Decapoda: Branchyura). Bull. Br. Mus. Nat. Hist. 40 (5), 211–236.
James, P. J., and Tong, L. J. (1998). Feeding technique, critical size, and size preference of Jasus edwarsii fed cultured and wild mussels. Mar. Freshw. Res. 49, 151–156. doi:10.1071/MF97138
Johnson, K. D., and Smee, D. L. (2014). Predators influence the tidal distribution of oysters (Crassostrea virginica). Mar. Biol. 161, 1557–1564. doi:10.1007/s00227-014-2440-8
Juanes, F. (1992). Why do decapod crustaceans prefer small-sized prey? Mar. Ecol. Prog. Ser. 87, 239–249. doi:10.3354/meps087239
Juanes, F., and Hartwick, E. B. (1990). Prey size selection in dungeness crabs: the effect of claw damage. Ecology 71, 744–758. doi:10.2307/1940327
Jurrius, L. H., and Rozemeijer, M. J. C. (2022). The role of the European lobster (Homarus gammarus) in the ecosystem. An inventory as part of a feasibility study for passive fisheries on European lobster Homarus gammarus in offshore winds farms. Wageningen Mar. Res. Rep. C070/22. doi:10.18174/580457
Karlsson, K., and Christiansen, M. E. (1996). Occurrence and population composition of the edible crab (Cancer pagurus) on rocky shores of an islet on the south coast of Norway. Sarsia 81, 307–314. doi:10.1080/00364827.1996.10413628
Kraufvelin, P., Bergström, L., Sundqvist, F., Ulmestrand, M., Wennhage, H., Wikström, A., et al. (2023). Rapid re-establishment of top-down control at a no-take artificial reef. Ambio 52, 556–570. doi:10.1007/s13280-022-01799-9
Krone, R., Dederer, G., Kanstinger, P., Krämer, P., Schneider, C., and Schmalenbach, I. (2017). Mobile demersal megafauna at common offshore wind turbine foundations in the German Bight (North Sea) two years after deployment – increased production rate of Cancer pagurus. Mar. Environ. Res. 123, 53–61. doi:10.1016/j.marenvres.2016.11.011
Krone, R., and Schröder, A. (2011). Wrecks as artificial lobster habitats in the German Bight. Helgol. Mar. Res. 65, 11–16. doi:10.1007/s10152-010-0195-2
Lake, N. C. H., Jones, M. B., and Paul, J. D. (1987). Crab predation on scallop (Pecten maximus) and its implications for scallop cultivation. J. Mar. Biol. Ass. U.K. 67, 55–64. doi:10.1017/S0025315400026357
Largen, M. J. (1967). The diet of the dog-whelk, Nucella lapillus (Gastropoda Prosobranchia). J. Zool. 151, 123–127. doi:10.1111/j.1469-7998.1967.tb02867.x
Lawton, P. (1989). Predatory interaction between the branchyuran crab Cancer pagurus and decapod crustacean prey. Mar. Ecol. Prog. Ser. 52, 169–179. doi:10.3354/meps052169
Lawton, P., and Hughes, R. N. (1985). Foraging behaviour of the crab Cancer pagurus feeding on the gastropods Nucella lapillus and Littorina littorea: comparissons with optimal foraging theory. Mar. Ecol. Prog. Ser. 27, 143–154. doi:10.3354/meps027143
Leiknes, S. M. (2023). The effect of Marine Protected Areas on the diet of Lillesand European lobster (Homarus gammarus): a DNA-based diet analysis approach. Trondheim, Norway: Norwegian University of Science and Technology. Available at: https://hdl.handle.net/11250/3080050.
Little, C., Trowbridge, C. D., Pilling, G. M., Williams, G. A., Morritt, D., and Stirling, P. (2024). Long-term fluctuations and recent decline of mussel populations in an Irish sea lough. J. Molluscan Stud. 90, eyae002. doi:10.1093/mollus/eyae002
Loose, C. J., and Dawidowicz, P. (1994). Trade-offs in diel vertical migration by zooplankton: the costs of predator avoidance. Ecology 75, 2255–2263. doi:10.2307/1940881
Mackay-Roberts, N. J., Bock, C., Lannig, G., Lucassen, M., Paul, N., Schaum, E., et al. (2024). A benthic mesocosm system for long-term multi-factorial experiments applying predicted warming and realistic microplastic pollution scenarios. Limnol. Oceanogr. Methods 22, 910–929. doi:10.1002/lom3.10653
Mascaró, M., and Seed, R. (2001). Foraging behavior of juvenile Carcinus maenas (L.) and Cancer pagurus L. Mar. Biol. 139, 1135–1145. doi:10.1007/s002270100677
McCall, M., Albins, M. A., and Powers, S. P. (2023). The common predator, Sheepshead (Archosargus probatocephalus), enhances survival of the Eastern oyster (Crassostrea virginica) in an experimental setting. J. Exp. Mar. Biol. Ecol. 568, 151945. doi:10.1016/j.jembe.2023.151945
Meira, A., Byers, J. E., and Sousa, R. (2024). A global synthesis of predation on bivalves. Biol. Rev. 99, 1015–1057. doi:10.1111/brv.13057
Meister, N., Langbehn, T. J., Varpe, Ø., and Jørgensen, C. (2023). Blue mussels in western Norway have vanished where in reach of crawling predators. Mar. Ecol. Prog. Ser. 721, 85–101. doi:10.3354/meps14416
Menge, B. A., Gravem, S. A., Richmond, E., and Noble, M. M. (2023). A unified meta-ecosystem dynamics model: integrating herbivore-plant subwebs with the intermittent upwelling hypothesis. Ecosphere 14, e4531. doi:10.1002/ecs2.4531
Merk, V., Colsoul, B., and Pogoda, B. (2020). Return of the native: survival, growth and condition of European oysters reintroduced to German offshore waters. Aquat. Conserv. Mar. Freshw. Ecosyst. 30, 2180–2190. doi:10.1002/aqc.3426
Mesquita, C., Dobby, H., Pierce, G. J., Jones, C. S., and Fernandes, P. G. (2021). Abundance and spatial distribution of brown crab (Cancer pagurus) from fishery-independent dredge and trawl surveys in the North Sea. ICES J. Mar. Sci. 78, 597–610. doi:10.1093/icesjms/fsaa105
Miron, G., Audet, D., Landry, T., and Moriyasu, M. (2005). Predation potential of the invasive green crab (Carcinus maenas) and other common predators on commercial bivalve species found on Prince Edward Island. J. Shellfish Res. 24, 579–586. doi:10.2983/0730-8000(2005)24[579:PPOTIG]2.0.CO;2
Modiolus Restoration Research Group (2024). Modiolus restoration plan. Available at: https://www.qub.ac.uk/research-centres/ModiolusRestorationResearchGroup/ModiolusRestorationPlan/(Accessed November 24, 2024).
Moland, E., Moland Olsen, E., Andvord, K., Knutsen, J. A., and Stenseth, N. C. (2011). Home range of European lobster (Homarus gammarus) in a marine reserve: implications for future reserve design. Can. J. Fish. Aquat. Sci. 68, 1197–1210. doi:10.1139/f2011-053
Molis, M., Preuss, I., Firmenich, A., and Ellrich, J. (2011). Predation risk indirectly enhances survival of seaweed recruits but not intraspecific competition in an intermediate herbivore species. J. Ecol. 99, 807–817. doi:10.1111/j.1365-2745.2011.01800.x
Ojeda, F. P., and Dearborn, J. H. (1991). Feeding ecology of benthic mobile predators: experimental analyses of their influence in rocky subtidal communities of the Gulf of Maine. J. Exp. Mar. Biol. Ecol. 149, 13–44. doi:10.1016/0022-0981(91)90114-C
OSPAR (2023). Status Assessment 2020 – European flat oysters and Ostrea edulis beds. Available at: https://oap.ospar.org/en/ospar-assessments/committee-assessments/biodiversity-committee/status-assesments/european-flat-oyster/ (Accessed November 24, 2024).
Pessarrodona, A., Boada, J., Pagès, J. F., Arthur, R., and Alcoverro, T. (2019). Consumptive and non-consumptive effects of predators vary with the ontogeny of their prey. Ecology 100, e02649. doi:10.1002/ecy.2649
Pickering, T., and Quijón, P. A. (2011). Potential effects of a non-indigenous predator in its expanded range: assessing green crab, Carcinus maenas, prey preference in a productive coastal area of Atlantic Canada. Mar. Biol. 158, 2065–2078. doi:10.1007/s00227-011-1713-8
Pickering, T. R., Poirier, L. A., Barrett, T. J., McKenna, S., Davidson, J., and Quijón, P. A. (2017). Non-indigenous predators threaten ecosystem engineers: interactive effects of green crab and oyster size on American oyster mortality. Mar. Environ. Res. 127, 24–31. doi:10.1016/j.marenvres.2017.03.002
Pineda-Metz, S. E. A., Brand, M., Merk, V., Feuring, A., and Pogoda, B. (2022). Seabed images taken at pilot oyster reefs in the Borkum Reef Ground marine protected area during September 2021. Bremerhaven, Germany: PANGAEA. doi:10.1594/PANGAEA.941836
Pineda-Metz, S. E. A., Colsoul, B., Niewöhner, M., Hausen, T., Peter, C., and Pogoda, P. (2023). Setting the stones to monitor European flat oyster reefs in the German North Sea. Aquat. Conserv. Mar. Freshw. Ecosyst. 2023, 1–17. doi:10.1002/aqc.3945
Pineda-Metz, S. E. A., Merk, V., and Pogoda, B. (2023b). A machine learning model and biometric transformations to facilitate European oyster monitoring. Aquat. Conserv. Mar. Freshw. Ecosyst. 2023, 708–720. doi:10.1002/aqc.3912
Pogoda, B. (2019). Current status of European oyster decline and restoration in Germany. Humanities 8, 9. doi:10.3390/h8010009
Pogoda, B., Colsoul, B., Hausen, T., Merk, V., and Peter, C. (2024). Restoration of European oyster (Ostrea edulis) in the German North Sea (RESTORE preliminary investigation). BfN-Schriften 684. doi:10.19217/skr684
Poiesz, S. S. H., van Elderen, T., Witte, J. I. J., and van der Veer, H. W. (2021). Historical trophic ecology of some divergent shark and skate species in the Dutch coastal North Sea zone. Mar. Biol. 168, 165. doi:10.1007/s00227-021-03974-0
Poirier, L. A., and Quijón, P. A. (2022). Natural attachment and size of cultured oysters limit mortality from a non-indigenous predator. Aquac. Res. 53, 1121–1126. doi:10.1111/are.15613
Poirier, L. A., Symington, L. A., Davidson, J., St-Hillaire, J., and Quijón, P. A. (2017). Exploring the decline of oyster beds in Atlantic Canada shorelines: potential effects of crab predation on American oysters (Crassostrea virginica). Helgol. Mar. Res. 71, 13. doi:10.1186/s10152-017-0493-z
Preisser, E. L., Bolnick, D. I., and Benard, M. F. (2005). Scared to death? The effects of intimidation and consumption in predator-prey interactions. Ecology 86, 501–509. doi:10.1890/04-0719
Reise, K., Buschbaum, C., Büttger, H., Rick, J., and Wegner, K. M. (2017b). Invasion trajectory of Pacific oysters in the northern Wadden Sea. Mar. Biol. 164, 68. doi:10.1007/s00227-017-3104-2
Reise, K., Buschbaum, C., Büttger, H., and Wegner, K. M. (2017a). Invading oysters and native mussels: from hostile takeover to compatible bedfellows. Ecosphere 8 (9), e01949. doi:10.1002/ecs2.1949
Robles, C., Sweetnam, D., and Eminike, J. (1990). Lobster predation on mussels: shore-level differences in prey vulnerability and predator preference. Ecology 71, 1564–1577. doi:10.2307/1938292
Rondeau, A., Hanson, J. M., and Comeau, M. (2014). Rock crab, Cancer irroratus, fishery and stock status in the southern Gulf of St. Lawrence: LFA 23, 24, 25, 26A and 26B. DFO Can. Sci. Advis. Sec. Res. Doc. 2014/032. vi + 52 p.
Ruxton, G. D. (2006). The unequal variances t-test is an underused alternative to Student`s t-test and the Mann-Whitney U test. Behav. Ecol. 17, 688–690. doi:10.1093/beheco/ark016
Sainte-Marie, B., and Chabot, D. (2002). Ontogenetic shifts in natural diet during benthic stages of American lobsters (Homarus americanus) off the Magellan Islands. Fish. Bull. 100, 106–116. Available at: https://aquadocs.org/items/6f567a68-1588-44c0-a634-df796ded6db6.
Schmalenbach, I., Mehrtens, F., Janke, M., and Buchholz, F. (2011). A mark-recapture study of hatchery-reared juvenile European lobsters, Homarus gammarus, released at the rocky island of Helgoland (German Bight, North Sea) from 2000 to 2009. Fish. Res. 108, 22–30. doi:10.1016/j.fishres.2010.11.016
Seed, R., and Brown, R. A. (1975). “The influence of reproductive cycle, growth, and mortality on population structure in Modiolus modiolus (L.), Cerastoderma edule (L.), and Mytilus edulis L., (Mollusca: Bivalvia),” in Proc. 9th europ. Mar. Biol. Symp. Editor H. Barnes, 257–274.
Seed, R., and Hughes, R. N. (1995). Criteria for prey size-selection in molluscivorous crabs with contrasting claw morphologies. J. Exp. Mar. Biol. Ecol. 193, 177–195. doi:10.1016/0022-0981(95)00117-4
Sheehy, M. R. J., and Prior, A. E. (2009). Progress on an old question for stock assessment of the edible crab Cancer pagurus. Mar. Ecol. Prog. Ser. 353, 191–202. doi:10.3354/meps07229
Shelton, R. G., Kinnear, J. A. M., and Livingstone, K. (1979). A preliminary account of the feeding habits of the edible crab Cancer pagurus L. off N.W. Scotland. ICES Shellfish Committee C. M. 1979/K: 35, 6 p.
Sherker, Z. T., Ellrich, J. A., and Scrosati, R. A. (2017). Predator-induced shell plasticity in mussels hinders predation by drilling snails. Mar. Ecol. Prog. Ser. 573, 167–175. doi:10.3354/meps12194
Silberbush, A., and Blaustein, L. (2011). Mosquito females quantify risk of predation to their progeny when selecting an oviposition site. Funct. Ecol. 25, 1091–1095. doi:10.1111/j.1365-2435.2011.01873.x
Silva, A. C. F., Hawkins, S. J., Boaventura, D. M., Brewster, E., and Thompson, R. C. (2010). Use of the intertidal zone by mobile predators: influence of wave exposure, tidal phase and elevation on abundance and diet. Mar. Ecol. Prog. Ser. 406, 197–210. doi:10.3354/meps08543
Skerritt, D. J., Robertson, P. A., Mill, A. C., Polunin, N. V. C., and Fitzsimmons, C. (2015). Fine-scale movement, activity patterns and home-ranges of European lobster Homarus gammarus. Mar. Ecol. Prog. Ser. 536, 203–219. doi:10.3354/meps11374
Smith, R. S., Cheng, S. L., and Castorani, M. C. N. (2023). Meta-analysis of ecosystem services associated with oyster restoration. Conserv. Biol. 37, e13966. doi:10.1111/cobi.13966
Sokal, R. R., and Rohlf, F. J. (2012). Biometry: the principles and practices of statistics in biological research. Fourth Edition. New York: W.H. Freeman and Company.
Stamp, T., Pittman, S. J., Holmes, L. A., Rees, A., Ciotti, B. J., Thatcher, H., et al. (2024). Restorative function of offshore longline mussel farms with ecological benefits for commercial crustacean species. Sci. Total Environ. 951, 174987. doi:10.1016/j.scitotenv.2024.174987
Steen, R., and Ski, S. (2014). Video-surveillance system for remote long-term in situ observations: recording diel cavity use and behaviour of wild European lobsters (Homarus gammarus). Mar. Freshw. Res. 65, 1094–1101. doi:10.1071/MF13139
Stehlik, L. L. (1993). Diets of the branchyuran crabs Cancer irroratus, C. borealis, and Ovalipes ocellatus in the New York Bight. J. Crustac. Biol. 13, 723–735. doi:10.2307/1549103
Strong, J. A., Service, M., and Moore, H. (2015). Estimating the historical distribution, abundance and ecological contribution of Modiolus modiolus in Strangford Lough, Northern Ireland. Biol. Environ. Proc. R. Ir. Acad. 116, 1–16. doi:10.3318/BIOE.2016.1
Sunrise and sunset Helgoland (2024). Available at: https://www.sunrise-and-sunset.com/en/sun/germany/helgoland/2022/september [Accessed November 15, 2024].
Tapia-Lewin, S., and Pardo, L. M. (2014). Field assessment of the predation risk - food availability trade-off in crab megalopae settlement. PLoS One 9, e95335. doi:10.1371/journal.pone.0095335
Tegner, M. J., and Levin, L. A. (1983). Spiny lobsters and sea urchins: analysis of a predator-prey interaction. J. Exp. Mar. Biol. Ecol. 73, 125–150. doi:10.1016/0022-0981(83)90079-5
ter Hofstede, R., Driessen, F. M. F., Elzinga, P. J., Van Koningsveld, M., and Schutter, M. (2022). Offshore windfarms contribute to epibenthic biodiversity in the North Sea. J. Sea Res. 185, 102229. doi:10.1016/j.seares.2022.102229
Thatcher, H., Stamp, T., Wilcockson, D., and Moore, P. J. (2023). Residency and habitat use of European lobster (Homarus gammarus) within an offshore wind farm. ICES J. Mar. Sci. 2023, 1410–1421. doi:10.1093/icesjms/fsad067
Thurstan, R. H., McCormick, H., Preston, J., Ashton, E. C., Bennema, F. P., Bratoš Cetinić, A., et al. (2024). Records reveal the vast historical extent of European oyster reef ecosystems. Nat. Sustain. 7, 1719–1729. doi:10.1038/s41893-024-01441-4
Tonk, L., and Rozemeijer, M. J. C. (2019). Ecology of the brown crab (Cancer pagurus) and production potential for passive fisheries in Dutch offshore windfarms. Wageningen Mar. Res. Rep. C064/19A. doi:10.18174/496176
Troost, K. (2010). Causes and effects of a highly successful marine invasion: case-study of the introduced Pacific oyster Crassostrea gigas in continental NW European estuaries. J. Sea. Res. 64, 145–165. doi:10.1016/j.seares.2010.02.004
Trussell, G. C., Ewanchuk, P. J., and Bertness, M. D. (2003). Trait-mediated effects in rocky intertidal food chains: predator risk cues alter prey feeding rates. Ecology 84, 629–640. doi:10.1890/0012-9658(2003)084[0629:TMEIRI]2.0.CO;2
Tummon Flynn, P. S., Mellish, C. L., Pickering, T. R., and Quijón, P. A. (2015). Effects of claw autotomy on green crab (Carcinus maenas) feeding rates. J. Sea Res. 103, 113–119. doi:10.1016/j.seares.2015.07.002
United Nations Sustainable Development Goals (2024). United Nations general assembly economic and social council. Prog. towards Sustain. Dev. Goals. Rep. Secretary-General. A/79/79-E/2024/54. Available at: https://sdgs.un.org/goals (Accessed November 24, 2024).
van Zyl, R. F., Mayfield, S., Pulfrich, A., and Griffiths, C. L. (1998). Predation by West Coast rock lobsters (Jasus lalandii) on two species of winkle (Oxystele sinensis and Turbo cidaris). S. Afr. J. Zool. 33, 203–209. doi:10.1080/02541858.1998.11448473
von Elert, E., and Pohnert, G. (2000). Predator specificity of kairomones in diel vertical migration of Daphnia: a chemical approach. Oikos 88, 119–128. doi:10.1034/j.1600-0706.2000.880114.x
Wang, S. V., Ellrich, J. A., Beermann, J., Pogoda, B., and Boersma, M. (2024). Musseling through: Mytilus byssal thread production is unaffected by continuous noise. Mar. Environ. Res. 200, 106661. doi:10.1016/j.marenvres.2024.106661
Werner, E. E., and Peacor, S. D. (2003). A review of trait-mediated indirect interactions in ecological communities. Ecology 84, 1083–1100. doi:10.1890/0012-9658(2003)084[1083:arotii]2.0.co;2
zu Ermgassen, P., Bos, O., Debney, A., Gamble, C., Glover, A., Pogoda, B., et al. (2021). European native oyster habitat restoration monitoring handbook. London: The Zoological Society of London. Available at: https://nativeoysternetwork.org/wp-content/uploads/sites/27/2021/11/European%20Native%20Oyster%20Habitat%20Monitoring%20Handbook_WEB_Final.pdf.
zu Ermgassen, P., McCormick, H., Debney, A., Fariñas-Franco, J., Gamble, C., Gillies, C., et al. (2024). European native oyster reef ecosystems are universally collapsed. EcoEvoRxiv. doi:10.32942/X2HP52
Keywords: ecological restoration, predation, European oyster (Ostrea edulis), brown crab (Cancer pagurus), European lobster (Homarus gammarus), German Bight
Citation: Ellrich JA, Kozian-Fleck C, Brand M, Colsoul B and Pogoda B (2025) Ecological reef restoration: consumptive and nonconsumptive interactions among common North Sea predators and European oysters. Front. Environ. Sci. 13:1509318. doi: 10.3389/fenvs.2025.1509318
Received: 10 October 2024; Accepted: 03 January 2025;
Published: 22 January 2025.
Edited by:
Martin Siegert, University of Exeter, United KingdomReviewed by:
Baoquan LI, Chinese Academy of Sciences (CAS), ChinaRoberto Simonini, University of Modena and Reggio Emilia, Italy
Copyright © 2025 Ellrich, Kozian-Fleck, Brand, Colsoul and Pogoda. This is an open-access article distributed under the terms of the Creative Commons Attribution License (CC BY). The use, distribution or reproduction in other forums is permitted, provided the original author(s) and the copyright owner(s) are credited and that the original publication in this journal is cited, in accordance with accepted academic practice. No use, distribution or reproduction is permitted which does not comply with these terms.
*Correspondence: Julius A. Ellrich, anVsaXVzLmVsbHJpY2hAYXdpLmRl