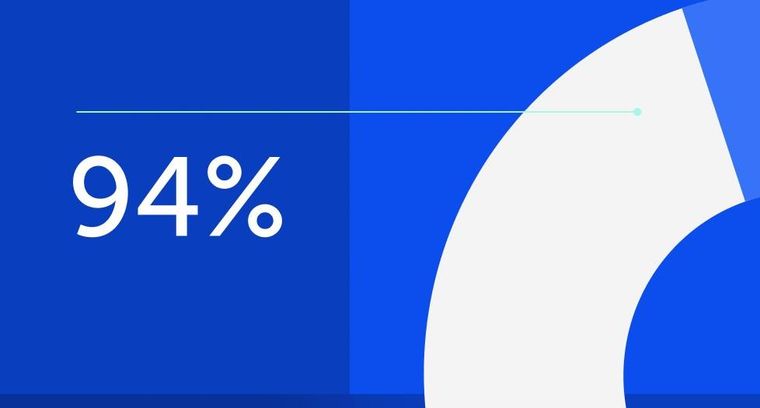
94% of researchers rate our articles as excellent or good
Learn more about the work of our research integrity team to safeguard the quality of each article we publish.
Find out more
SYSTEMATIC REVIEW article
Front. Environ. Sci., 26 February 2025
Sec. Toxicology, Pollution and the Environment
Volume 13 - 2025 | https://doi.org/10.3389/fenvs.2025.1509156
This article is part of the Research TopicResource Conversion and Safe Disposal of Solid Wastes Containing Heavy MetalsView all 4 articles
Phosphogypsum (PG), phosphate sludge (PS), and sewage sludge (SS) are regarded by-products produced in huge amounts. However, PG, PS and SS are no longer considered as waste, but as valued resources in accordance with the circular economy's rules. Their management provides a serious environmental problem. In order to assess the impacts of SS, PS, and PG on soil physico-chemical parameters (pH, EC, OM, nutrients, and heavy metals) in response to diverse experimental settings, the purpose of the current study was to conduct a meta-analysis on previously published results. The VOSviewer program was used to construct bibliometric maps using the VOS mapping and grouping techniques. The findings indicated that there were statistically significant changes (P < 0.05) in electrical conductivity (EC), organic matter (OM), and pH in connection to the different by-products employed. The application of SS considerably elevated pH by 46.15% compared to the control. Furthermore, a beneficial effect on P and K was detected, regardless of the by-product used. Moreover, Cd, Pb, and Ni concentrations in SS treatments had a substantial reduction of 30.46%, 30.70%, and 18.07%, respectively. Cd, Pb, and Cu concentrations in PG treatments revealed a substantial decrease of 47.71%, 36.14%, and 46.01%, respectively. Based on the acquired data, PG, PS, and SS need to be regularly monitored and regulated. This study serves as an early investigation for the construction of a new approach to restore damaged land on mine sites by employing phosphate industry by-products and sludge for revegetation objectives.
• Meta-analysis was conducted to assess effect of SS, PS and PG application to mining soil/degraded land.
• SS are the most economical for raising pH and OM.
• SS, PS and PG application increased nutrients.
• By-products should be further improved to remedy heavy metals contaminated soils.
• Recommendation to use SS, PS and PG to substitute the soil.
The phosphate industry’s production of phosphorus (P) is a critical component with great economic value in fertilizer manufacturing. However, population growth, concurrent with increased food demand and urbanization, has noted a major increase in the usage of mined phosphate rock relative to other sources of P since the middle of the twentieth century (Sheoran et al., 2010; Gupta et al., 2019). Fertilizers are essential for crop growth. However, some estimates suggest that we may consume the existing rock phosphate reserves within 50–120 years (Cordell et al., 2009; Wendling et al., 2013). An efficient use of P, as well as its recovery and reuse through different processes, are necessary to face this future P shortage. Phosphate mining is the main source of phosphorus for industry (Derhy et al., 2020). However, all the elements in phosphate ores, except phosphorus, are considered impurities that must be removed by suitable processes (Mariana Campos Assunção, 2017). There are many beneficiation procedures for extracting phosphate ores, depending on the kind of ore and accompanying gangue minerals. We learned about attrition scrubbing and classification, size reduction and screening, electrostatic separation, chemical dissolution of carbonates, magnetic separation, and flotation (Gharabaghi et al., 2010; Bakopoulou et al., 2013; Hakkou et al., 2016). Following different extraction and phosphoric acid production operations, mining inevitably results in by-products (phosphate sludges and phosphogypsum). Sulfuric acid reacts with phosphate rocks to produce phosphogypsum (PG) as a by-product (Saadaoui et al., 2017; Es-Said et al., 2020). As a result, one tonne of phosphorus produces 4–5 tonne of PG (Javied et al., 2009; Contreras et al., 2018).
Phosphate sludge (PS) is a residue produced by phosphate mining, typically obtained through the phosphate beneficiation process involving washing and flotation (Moukannaa et al., 2018). Considering that it generates a significant amount, PS storage occupies huge regions and poses a significant challenge in terms of storage capacity (Tabrika et al., 2021; Guéablé et al., 2022a). Furthermore, according to several authors, the large quantities of these by-products, which are generally stored in the open air, alter the landscape and arable land. Therefore, without a sustainable management approach, these by-products have the potential to have direct effects on the natural environment as well as the health of the nearby population (Reta et al., 2018; Boutaleb et al., 2020). As a result, reusing these by-products (PG and PS) from rock phosphate processing becomes a critical issue in terms of minimizing the footprint and the environmental implications. Numerous researchers have produced a wide range of solutions that are not only effective but also environmentally benign. These solutions include those that are related to the valorization of by-products and the rehabilitation of phosphate mining sites. When seen in this light, the literature suggests a variety of approaches to the reuse of by-products resulting from activities within the phosphate sector. In agriculture, PG is utilized as an amendment for soils that have been degraded and as a fertilizer for the development of crops (Ahmed et al., 2010; Kammoun et al., 2017; Outbakat et al., 2022), as well as in the brick and cement industry (Loutou et al., 2017; Moukannaa et al., 2018; Bayoussef et al., 2021), and in road construction (Saadaoui et al., 2019; Amrani et al., 2019).
Sewage sludge (SS) is a by-product that arises from the municipal wastewater treatment process (Ondreičková et al., 2019; An-nori et al., 2020). Its production is increasing globally due to industrialization and population growth (Usman et al., 2012; Aricak et al., 2019). One common use of SS is as an amendment for agricultural soil. Numerous studies have shown that SS is a valuable source of nutrients, including organic matter, nitrogen, phosphorus, potassium, calcium, sulfur, and magnesium (Guéablé et al., 2021). Therefore, applying SS to soil can enhance its quality, particularly in degraded soils with low agricultural productivity (Asensio et al., 2013).
Heavy metals, which at high concentrations could become harmful and limit their valorizations, especially when employed as a soil amendment in large quantities, may be present in PG, PS, and SS. Heavy metals are permanent and non-biodegradable, and unlike organic molecules, they can be digested or eliminated. As a result, they may cause pollution in the food chain (Lyczko et al., 2014). Thus, even at low concentrations, these heavy metals may pose serious risks to living things because to their capacity to induce mutagenesis and aggregation characteristics (Issaad et al., 2019).
While numerous studies have examined the nutrient and heavy metal contents of PG, PS, and SS, little research has been done on how these materials might be used for mining rehabilitation. Therefore, in order to offer a thorough interpretation of research findings, a systematic review may be helpful. Thus, the goal of the current study was to conduct a meta-analysis on the nutrient and heavy metal contents of soil-plant systems associated with a mixture of PG, PS, and SS utilized as substrates for degraded soils or mine site rehabilitation, utilizing several tree species.
The bibliography for this study was completed following the PRISMA (Preferred Reporting Items for Systematic Reviews and Meta-Analyses) procedure (Karimi et al., 2021). A global study was conducted using Scopus databases between 2000 and 2023, focused on articles primarily from the “Agricultural and Biological Sciences” category in Scopus, which encompasses a large portion of the literature relevant to the topic at hand. In this sense, four processes comprised the comprehensive search, which included publications based on PRISMA guidelines: identification, screening, eligibility, and selection of articles. For searching, the keywords used were: “Mining Rehabilitation,” “Phosphogypsum,” “Phosphate Sludge,” “Sewage Sludge.”
In bibliometrics, science mapping is a method used to create a visual depiction of network structures. Science mapping is an emerging field that combines scientometrics and information metrology. It encompasses the interdisciplinary areas of applied mathematics, information science, and computer science (Apori et al., 2022). In the past few decades, numerous researchers have employed a variety of scientific mapping approaches to analyze the possible dynamic mechanism of discipline change (Smyrnova-Trybulska et al., 2018; Vagelas and Leontopoulos, 2023). Because of their power and complementarity, CiteSpace and VOSviewer are the two tools for scientific mapping analysis. CiteSpace (https://sourceforge.net/projects/citespace, accessed on 11 June 2024) is a Java-based application software that was proposed by Professor Chen of Drexel University in 2004 (Apori et al., 2022). It uses the theory of co-citation analysis and the pathfinder algorithm, minimum spanning trees, to perform a quantitative analysis of literature in specific fields. This method is used to analyze and visualize trends and emerging patterns in scientific publications’ knowledge (Smyrnova-Trybulska et al., 2018). It provides unique advantages in literature, such as keyword analysis, cluster analysis, subject words, and author information. In addition, the software tool for literature analysis and knowledge visualization developed by van Eck and Waltman is VOSviewer (https://www.vosviewer.com/, accessed on June 11 June 2024) (Van Eck, 2011). It is capable of creating and visualizing keyword co-occurrence networks across a variety of fields. VOSviewer software has advantages over other visualization tools in terms of processing massive data and creating graphics, allowing it to more clearly depict hot spots and study subjects (Vagelas and Leontopoulos, 2023).
The articles underwent a thorough screening process in order to meet a number of inclusion requirements, including: 1) availability of full-text articles; 2) publication of articles in the English language; 3) The number of study sites sampled and the study site’s size were stated. 4) The investigations were either descriptive or cross-sectional in nature; 5) The research solely focused on mining, damaged soils, or agriculture; 6) We displayed the types of by-product sources, including phosphate sludge (PS), sewage sludge (SS), and phosphogypsum (PG), and their individual or combined utilization; 7) We displayed the physico-chemical parameter values (pH, organic matter, and conductivity) from PG, PS, and SS; 8) We explicitly indicated the nutrient and heavy metal values (mean value and standard deviation value) in PG, PS, and SS. We also applied the following exclusion criteria to the articles. We excluded: 1) books and review articles; 2) articles that presented figures of low quality; 3) not using English language; 4) no relevant outcomes. Lastly, we extracted the parameters of the heavy metal contents (As, Pb, Ni, Al, Cd, Cr, Cu, Zn, and Fe) and nutrients (N, P, K, Ca, and Mg) from PG, PS, and SS. We used the GetData Graph Digitizer (Version 2.22) (http://getdata-graph-digitizer.com) to extract the data for articles that contained graphical results.
An extensive analysis was conducted to increase the sample size and enhance the reliability of the findings. This was achieved by combining the results from various studies, focusing on the nutrient and heavy metal contents in PG, PS, and SS, using the mean and standard error (SE) measurements (Equation 1).
The SE was determined using the following Equation 1:
SD represents the standard deviation, while N denotes the sample size.
This study collected and managed data using Microsoft Office 2016. The correlation study was performed using Metawin 3, and the graphs were created with SigmaPlot (version 14.5). A categorical meta-analysis was performed to reduce data heterogeneity and clarify the impacts of PG, PS, and SS treatments on nutrient and physicochemical parameter changes. The impacts of PG, PS, and SS applications on changes in nutrient and physicochemical parameters were evaluated using the “effect size” among the several subgroups (pH, EC, OM, N, P, K, Ca, and Mg). The effect size (Equation 2) of the various by-products was estimated using a natural logarithm of the response ratio (R = Xt/Xc) (Bohoussou et al., 2022).
Where Xt and Xc represent the average values of PG, PS, and SS of the treatment (t) and control group (c) respectively.
The values were subsequently transformed into percentages by multiplying them by (R-1) and then multiplying the result by 100 (Bohoussou et al., 2023). Positive numbers imply a gain in physico-chemical qualities such as pH, electrical conductivity (EC), organic matter (OM), nutrients, and heavy metals relative to the baseline values. Conversely, negative values indicate a reduction. Bootstrapping procedures within the ‘boot’ package were used to generate 95% confidence intervals for weighted mean effect sizes using 4.999 iterations. Statistical significance was determined using 95% confidence intervals (CI), and if the value of the 95% CI overlapped with 0, it was considered nonsignificant.
Up until 1 February 2020, searches from Scopus in the “Agricultural and Biological Sciences” field area were conducted as part of the research selection procedure. 2,864 articles were found at first. Then, we eliminated 2,524, 74, 61, and 141 papers because they were duplicates or ineligible by automation tools, abstract, or complete text, books and review, figures of low quality, not using English language. Ultimately, 50 publications were determined to be suitable research for this review’s inclusion. In accordance with Cochrane procedures, the selection procedure for the examined studies is depicted in Figure 1.
A research hotspot is a network of links formed by researchers concentrating on a certain set of research problems or subjects for a predetermined amount of time. This drastically increases the number of publications in related literature. Keywords in an article serve to concisely define, extract, and summarize the main idea, as well as visually represent the direction and significance of the study findings, which are the article’s essential components (Apori et al., 2022).
In this study, we created a knowledge graph by visually analyzing the keywords in the published literature on the research topic using the VOSviewer software. A circular node represents each term, with its greater area and longer diameter indicating a higher frequency of the term in the study domain. The association curves that connect the circular nodes represent the terms’ co-occurrence. Furthermore, to differentiate between node clusters, VOSviewer uses a knowledge graph with distinct colors, each of which represents a distinct cluster.
The keyword co-occurrence map (Figure 2) clearly shows that “Sewage Sludge,” located in the middle of the image, is the focus of the keyword knowledge map for the entire topic area. Circular nodes of various diameters radiate outward from the center, encircling it. With “sewage sludge” (1052), “heavy metals” (392), “compost” (159), “biosolid” (153), and “soil” (119) reflecting the total link strength, we set the threshold value to 276 in order to clearly display the high-frequency keywords. The grouping of keywords in various hues inside the graph indicates that these high-frequency words function as a type of binding hub. These high-frequency words, which combine a variety of low-frequency phrases, have been a prominent frontier study issue in the discipline for the past 20 years.
Figure 2. VOSviewer cluster analysis between the keywords found in the Scopus publication record (search string: “Mining Rehabilitation,” “Phosphogypsum,” “Phosphate Sludge,” “Sewage Sludge.”) records from 2000 to 2023.
We use the VOSviewer program’s special clustering density view function to further demonstrate the keyword clustering findings in order to more quickly, intuitively, and clearly identify and distill popular study topics in the field from the densely connected nodes and linkages (Figure 3). In the clustering density view, we can see the highlighted focal section very clearly, and the density of each node varies depending on the high and low frequency of the keywords that surround it. Both the number of nodes and the weights of the scattered nodes around each node directly influence the density of the nodes. The color of the nodes is closer to yellow (i.e., the darker the color) as the density of nodes in the center increases, indicating a higher research intensity for the topic and the key area of research. On the other hand, as the density of nodes on the edge decreases, the color becomes closer to blue, indicating that the research topic is not particularly popular. Consequently, the shift from the yellow area in the center to the blue area at the edge is indicative of the evolution in the research topic’s popularity. Based on the density clustering results in Figure 3, we extracted four frontier trending topics in the current research field (Table 1). We then analyze and discuss the research content and significant findings for each topic.
The utilization of different by-products resulted in significant variations (P < 0.05) in electrical conductivity (EC), organic matter (OM), and pH (Figure 4). Across all experiments, we discovered a positive EC effect on SS (56.36% increase), PS (285.74% increase), and PG (224.18% increase) applications. PS produced the most sensitive EC response when compared to SS and PG. The application of SS elevated pH by 46.15% when compared to the control. However, PS and PG are not relevant because they intersect with zero (the red line). Furthermore, our meta-analysis revealed that both SS (103.70%) and PG (87.88%) treatments resulted in significant increases in OM. This revealed that SS and PG applications was the most effective way to improve OM.
Figure 4. Phosphogypsum (PG), phosphate sludge (PS), and sewage sludge (SS) influences on soil pH, Electrical Conductivity (EC), and Organic Matter (OM): analysis of statistically significant effect sizes via 95% bootstrap confidence intervals.
Variations in the soil EC value can be used to infer changes in the amount of soluble salt in the soil because the two quantities are directly proportional (Chi et al., 2012; Alam et al., 2020; Guéablé et al., 2022b). Applying PS causes the soil’s EC value to rise noticeably, which helps to explain this most sensitive response. Baiz et al. (2021) speculate that the elevated calcium and potassium content of PS may be the cause of the EC increase.
Soil pH is a key indicator of soil characteristic with a major influence on soil fertility (Neina, 2019). This pH value is higher than the increases in pH following the application of amendments found in another meta-analysis. Indeed, the addition of lime, biochar, by-products (phosphogypsum and caustic sludge), manure, straw, and a combination of amendments improved the pH of the soil by 15%, 12%, 15%, 13%, and 17%, respectively (Zhang et al., 2023). According to Balduíno et al. (2019), applying SS to the mined area increased substrate fertility by changing pH. Due to this modification, the research area’s edaphic environment is chemically different from the original soil. Zanuzzi et al. (2009) found that a single application of industrial waste such as SS caused a pH rise in mine soils. This mining site played a crucial role in extracting valuable mineral ores like sphalerite, galena, and pyrite. This is supported by the study conducted by Żukowska et al. (2023) which demonstrated that the use of SS as a substrate led to notable enhancements in soil properties. Applying SS can help by neutralizing the strong acidity or alkalinity, which can reduce the potential toxicity of heavy metals (Zanuzzi et al., 2013; Bolaños-Guerrón et al., 2021).
In addition, organic matter (OM) has been found to enhance the soil’s ability to retain nutrients and water, creating a favorable environment for plant growth and microbial activity (Zanuzzi et al., 2009). Our findings align with the meta-analysis conducted by Zhang et al. (2023). Soil OM showed a positive response to the application of biochar (50%), by-product (12%), manure (26%), straw (26%) and combined materials (40%). Many previous studies expressed increased organic matter concentration with increased amounts of SS (Wu et al., 2013; Luna et al., 2016; Khan et al., 2023). Nevertheless, the OM present in the mixture with PG originates from the addition of organic matter as a soil enhancer and fertilizer. Therefore, the studies conducted by, Guéablé et al. (2021) and Mahmoud et al. (2021) demonstrated that using SS along with PG greatly increased the amount of organic matter in the soil and was an important part of restoring land that had been damaged.
We have distinguished between macronutrients (N, P, K) (Figure 5) and secondary nutrients (Ca, Mg) (Figure 6). We observed a positive effect on P and K, regardless of the by-product. The most reliable effects were observed on SS (205.22%) and PG (113.28%), with a positive effect on P and K respectively. Additionally, we observed a general positive effect of N on SS and PG, and a non-significant effect of N on PS (Figure 5). While by-products altered the soil’s nutrient composition, PS and PG did not significantly alter P levels. By addition of PG, Ca increased significantly by 36.12%. Additionally, SS significantly increased Mg by 161.52% compared to PG (Figure 6).
Figure 5. Nitrogen (N), phosphorus (P), and potassium (K) contents from phosphogypsum (PG), phosphate sludge (PS), and sewage sludge (SS): effect sizes using bootstrap (95%) confidence intervals.
Figure 6. Calcium (Ca) and magnesium (Mg) contents from phosphogypsum (PG), phosphate sludge (PS), and sewage sludge (SS): effect sizes using bootstrap (95%) confidence intervals.
The sludge application enhanced the soil’s total N, P, and Ca concentrations, as well as its pH. Rao et al (2001) also found that that all organic additions improved nitrogen, phosphorus, potassium, and magnesium contents. The most significant enhancement was achieved with SS. Utilizing SS is a viable approach to swiftly enhance soil fertility by introducing organic matter, nitrogen, and phosphorus (Moreno-Barriga et al., 2017). In their work, Cox and Whelan (2000) reforested an industrial site using an anthroposol that included SS and a combination of industrial wastes. Their investigation indicated that significant levels of N and P remained after 11 years, demonstrating the possibility for nutrient cycling. Furthermore, according to Czekala (1999) studies, one ton of SS contains an average of 543 kg of OM, 32 kg of N, and 16.6 kg of P. Kelly (2006) found that SS had similar characteristics to topsoil, concluding that SS can be a potential substitute for topsoil.
With over 92% calcium sulphate, PG is an excellent calcium (Ca) source; it is a by-product of the wet acid synthesis of phosphoric acid from rock phosphate (Bossolani et al., 2022). According to Takasu et al. (2006), found that using PG instead of Ca is a valuable fertilization method. The addition of PG greatly enhances the soil’s chemical and physical properties due to its high P, Ca, and S contents (Nayak et al., 2011; Mahmoud et al., 2021). Al-Enazy et al. (2018) pointed out PG application increased soil available P. However, no significant effect was observed on soil available K. The nutrient-rich nature of PG has led to an interest in using it both in agriculture and for rehabilitating degraded land or mining sites, rather than disposing of it in tailings ponds or heaps (Singh et al., 2018; Provenzano et al., 2020).
Although by-products change the soil’s nutrient contents, PS and PG do not significantly alter the P content. Our research showed that PG and PS did not significantly enhance the P content. This could be linked to the PS or PG method or the phosphate rock content used in the studies we selected for our work.
By-products (SS and PG) decreased the average concentrations of heavy metals in soil, as shown in Figure 7. The mean Cd, Pb, and Ni concentrations in SS significantly reduced by 30.46%, 30.70%, and 18.07%, respectively, across all investigations. Additionally, there was a considerable decrease in the mean amounts of Cd, Pb, and Cu in PG, with 47.71%, 36.14%, and 46.01%, respectively. However, we could not find any information on Cd, Pb, Cu, and Ni for PS, nor on Ni for PG. Metal pollution is a major concern due to its potential environmental effects (Li et al., 2019; Iyyappan et al., 2023). Heavy metals such as Pb, Zn, Cu, Cd, and Ni pose significant environmental hazards due to their indestructibility and hazardous effects on living creatures when their concentrations exceed a specific threshold (Mahmoud and Abd El-Kader, 2015; Guéablé et al., 2022a). The reductions in soil heavy metals were mostly attributable to their immobilization onto PG or SS particles, in addition to their indirect impacts on soil characteristics. This was the primary reason for the observations. There have been multiple investigations that have demonstrated that the by-products have a considerable impact on the levels of heavy metals that are present in the soil (Smaoui-Jardak et al., 2017; Turkyilmaz et al., 2020). There are several mechanisms that are responsible for the immobilization of heavy metals by by-products. These mechanisms include adsorption (Violante et al., 2010), ion exchange (Ding et al., 2017), complexation (Wijesekara et al., 2016), and precipitation processes (Guerrero et al., 2021). Nayak et al. (2013) reported that using PG as an amendment can dilute heavy metals. Additionally, the accumulation of heavy metals in the soil during site remediation is influenced by various physico-chemical parameters (Al-Lami et al., 2022; Qian et al., 2023). The current meta-analysis’s findings showed that adding SS and/or PG significantly raised soil pH, EC, OM, and nutrient levels, all of which are beneficial for reducing heavy metals pollution.
Figure 7. Impact of sewage sludge (SS), phosphate sludge (PS), and phosphogypsum (PG) additions on soil heavy metal concentrations: mean effect sizes with 95% bootstrap confidence intervals.
The solubility of large amounts of heavy metals is decreased when the pH of the environment is raised, as shown by Zanuzzi et al. (2009). An increase in pH causes a decrease in the solubility of contaminants in soil, including cadmium, copper, nickel, and zinc (Illera et al., 2004; Gosh and Singh, 2005). In addition, in dry regions, carbonate plays a crucial role in trapping heavy metals by creating a surface for adsorption or nucleation. It also helps maintain a stable pH level, which promotes the hydrolysis and precipitation of metals (Almendro-Candel et al., 2007). In a study conducted by Mahmoud and Abd El-Kader. (2015), it was found that the presence of PG in soil treatment leads to the formation of surface metal-complexes, which helps immobilize heavy metals. These complexes can be formed when the metal interacts with the surface sites of oxides found in the PG, such as Ca-OH, Fe-OH, Al-OH, and Si-OH. Furthermore, PG includes phosphate rocks that help to immobilize soil Pb by causing the formation of pyromorphite minerals (Mahmoud and El-Kader, 2013). One of the most important buffering mechanisms that governs lead movement and fixation in water, soils, and by-products is the interaction between lead and phosphorus, which occurs through the production of pyromorphite. Additionally, it decreases the bioavailability and solubility of lead (Mahmoud and El-Kader, 2013; Wijesekara et al., 2016). It is possible to use immobilization of inorganic contaminants as a restorative approach for soils that are contaminated with heavy metals (Li et al., 2022). According to Zanuzzi et al. (2013), SS is also capable of successfully reducing heavy metals through different mechanisms, including chelation, complexation, and adsorption between metals, and applied organic matter. It has been demonstrated through research carried out by Guéablé et al. (2021) that a mixture consisting of 65% PG, 30% PS, and 5% SS has the potential to facilitate the successful immobilization of heavy metals through the mine rehabilitation process. The conclusion that can be drawn from this finding is that the incorporation of by-products such as PG into SS has the potential to limit the solubility of heavy metals through the formation of complicated reactions. The use of by-products and their combinations must be beneficial to the soils or substrates with which they are mixed.
Meanwhile, in a study conducted by Liang et al. (2012), it was discovered that the effectiveness of immobilizing Cd, Zn, and Pb was 100%, 85.7%, and 62.2%, respectively, when 5% red mud was combined with SS. Moreover, the immobilization efficiencies of Cd, Zn, and Pb were found to be 100%, 92%, and 82%, respectively, when there was a combination of 10% red mud with SS. These by-products should contribute to the rehabilitation of soils or mines by representing no source of contamination.
Phosphogypsum (PG), a by-product of the phosphate fertilizer industry, includes naturally occurring radioactive materials (NORM), such as uranium (U), thorium (Th), and radium (Ra), which may pose environmental and health risks (Hamza et al., 2019; Akfas et al., 2024). The presence of radium-226 is concerning due to its extended half-life and its capacity to emit radon gas, a recognized carcinogen. Application of PG to soils for land rehabilitation may result in radon emissions into the atmosphere, thereby increasing the risk of lung cancer with extended exposure (Al-Hwaiti et al., 2010). Furthermore, PG may bring additional radionuclides into the soil, potentially impacting plant development and the food chain (Chakraborty et al., 2013). The radioactive characteristics must be meticulously evaluated when considering the application of PG for soil rehabilitation, as they pose significant safety and environmental challenges necessitating comprehensive assessment and mitigating strategies.
The rehabilitation of degraded land in general, and of mines in particular, requires a global consideration of the efficiency of the by-products to be used. Previous studies have similarly reported that SS, PS, and PG applications can significantly improve physico-chemical properties parameters of soils (Peregrina Alonso et al., 2006; Adusei-Gyamfi et al., 2019; Guéablé et al., 2022b). In order to address the degradation of arid and semi-arid areas that experience high erosion rates, it is crucial to establish a robust vegetation cover and restore the mining soil (Guéablé et al., 2022b). Plants in arid and semi-arid environments experience severe water stress as a result of the minimal rainfall that occurs in these regions (Outbakat et al., 2022). Usually, the species that are utilized are seedlings or greenhouse plants, both of which are susceptible to posttransplant shock upon sowing, which can be attributed to either inadequate moisture or inadequate nutrients (Peregrina Alonso et al., 2006; Bateman et al., 2021). It is thus vital to use plant species that are adapted to the water stress, nutrients available in the restored region, and the site’s climatology, as they have acquired morphological and physiological adaptations that allow them to live and flourish in challenging conditions (Alvarenga et al., 2018). For the purpose of preserving the ecological equilibrium, it is of interest to make use of species that are often found in the area that is going to be rehabilitated.
Furthermore, these plants serve as the foundation for organic ecological transition by adapting to climate and soil circumstances (Grandlic et al., 2008). Compared to plants imported from other ecosystems, they have higher chances of surviving, growing, and reproducing under stressful environmental conditions (Hindersah et al., 2018). Having a seed bank of species to exploit, or in the absence of one, preparing one using plants from the nearby environment under conditions similar to those originally present on the mine site, is a crucial and basic strategy in all mining operations. This will allow for the utilization of local species, which will increase the likelihood of effective revegetation. From the perspective of the soil and landscape, the advantages of revegetation are crucial (Yang et al., 2014). According to Feng et al. (2020) plants boost water penetration, decrease water erosion by catching rainwater, and encourage soil regeneration. Because there are more nutrients available when organic matter is used as a restoration approach by adding SS, plants develop more quickly (Moreno-Barriga et al., 2017). Therefore, the right mix of by-products and revegetation promotes plant development and, eventually, the restoration of the rehabilitated area’s landscape (Peñaranda Barba et al., 2020). By-product application did, in fact, result in more vegetation, which is important for land rehabilitation since vegetation enhances soil organic matter, stabilizes soil structure, reduces erosion, and minimizes it (Guéablé et al., 2022b; Guerrero et al., 2021).
Furthermore, establishing and supporting of vigorous plant growth requires irrigation in most climates. Thus, plant selection is an essential element and must be consistent with the site’s climate. Moreover, irrigation is required at the beginning of a vegetation project to support plant growth. It is typically supplied through drip irrigation systems (Scanlon et al., 2005; Venkatraman and Ashwath, 2010). Abandoned mines face further problems, as in most cases, they are located in very remote areas and may not have a local water supply. As observed at the webbs consols and mole river sites in Australia, the water associated with the site is often contaminated by a range of metal contaminants, high acidity, and high salinity (Bolan et al., 2017). In order to successfully revegetate damaged land, rehabilitation techniques must strike a compromise between immediate goals like soil stabilization and erosion management and long-term goals like boosting native biodiversity or growing woody vegetation. The unfavourable environmental conditions that frequently impede establishment can be lessened via site preparation and sowing (Wong, 2003; Li, 2006; Baethke et al., 2020; Kragt et al., 2019). Furthermore, higher nitrogen and phosphate availability may be the cause of the increased cover on seeded locations where biosolids have been applied (Meyer et al., 2009). When revegetating severely disturbed landscapes, agronomic species are often utilized to generate rapid cover by quickly utilizing available nutrients (Baethke et al., 2020). It will be crucial to choose plant species that can compete in this nutrient-rich environment when creating an anthroposol because there is currently little research on the application of species combinations in the rehabilitation of degraded land or former mines (Galfati et al., 2011; Santos et al., 2019). According to Kelly (2006), the by-products and their combinations must improve the spoil or current soil in order to promote revegetation without contaminating the soil. Thus, by-products should be characterized by:
- Rich in macronutrients (NPK) with certain trace elements such as Cu, Zn, and Mg.
- A pH that should be more or less neutral,
- A reasonable humidity level, providing water for regions with low rainfall.
- A high organic matter content to promote plant growth and restore soil structure
- Less concentrations of pollutants, which include heavy metals, that are in excess of what the plant needs to survive.
For the purpose of revegetating an industrial site, Cox and Whelan (2000) developed an artificial soil combination that was prepared by combining SS and waste from industrial processes. The results of this study demonstrated that the soil underwent development over both the short and long term, with the first year exhibiting the most rapid alterations. Following 11 years, the nutrient cycling potential revealed significant levels of nitrogen and phosphorus, together with an abundance of organic carbon for the decomposition of microorganisms.
The mining sector is one of the most significant in the twenty-first century since it supplies vital resources for economic growth. On the other hand, significant efforts need to be done in order to improve the economic, social, and environmental elements of this industry. The results of this meta-analysis and study have shown that the utilization of sewage sludge and by-products from the phosphate industry has a great deal of promise for the rehabilitation of mining soils or degraded land. Its purpose is to bring attention to the enormous potential that has been found in the study and work that has been done over the past 20 years. In a semi-arid environment, the most important things to focus on are increasing the amount of organic matter in the soil, optimizing the processes of revegetation, and managing water. One of the most important issues is the creation and management of by-products, which are made feasible by the utilization of these by-products in the rehabilitation of mining sites or degraded land. Nevertheless, the utilization of sewage sludge (SS), phosphate sludge (PS), and phosphogypsum (PG) for the rehabilitation of mining soils or degraded land has some limitations that should be addressed. These include potential concerns related to the presence of heavy metals, the variability in physicochemical properties of the by-products, and the environmental risks associated with their long-term use. So, it is necessary to take into account not only the positive features, but also the bad ones, such as the potential pollutants that are released because of production activities in the industrial sector. Consequently, we are investigating different ways to integrate plant species into by-products that are not harmful to the environment and might be reintroduced into the economy as part of the circular economy.
Future lines of research, complementing the aspects linked to the Valorization of by-products from the phosphate industry and sewage sludge, are proposed. While adding these by-products have advantages, it accentuates the differences between by-products applications in different studies. It complicates the ability of researchers to synthesize and understand how nutrient and heavy metal contents in by-products affect the rehabilitation process. As a proposal for future lines of work, it could be agreed to work within the following objectives:
• Valorization of phosphogypsum (PG), phosphate sludge (PS), and sewage sludge (SS), in the rehabilitation of mining sites to reduce their handling and storage costs.
• Conducting experiments to assess the efficacy of phosphogypsum (PG), phosphate sludge (PS), and sewage sludge (SS) in improving soil quality and encouraging plant growth.
• Evaluating the impact of phosphogypsum (PG), phosphate sludge (PS), and sewage sludge (SS) when applied either alone or in combination at a certain ratio.
• Optimizing the conditions under which by-products are used in the transfer dynamics of the water-soil-plant system.
The datasets presented in this study can be found in online repositories. The names of the repository/repositories and accession number(s) can be found below: Data will be made available on request.
YKDG: Conceptualization, Data curation, Methodology, Visualization, Writing–original draft. NYB: Formal Analysis, Methodology, Visualization, Writing–original draft. MEG: Funding acquisition, Supervision, Writing–review and editing. KEM: Conceptualization, Funding acquisition, Supervision, Writing–review and editing. MH: Conceptualization, Supervision, Writing–review and editing.
The author(s) declare that financial support was received for the research, authorship, and/or publication of this article. This work was supported by the Gantour OCP phosphate production plant in Ben Guerir, Morocco, under the “specific agreement OCP-UM6P no. RE02.”
The authors declare that the research was conducted in the absence of any commercial or financial relationships that could be construed as a potential conflict of interest.
The author(s) declare that no Generative AI was used in the creation of this manuscript.
All claims expressed in this article are solely those of the authors and do not necessarily represent those of their affiliated organizations, or those of the publisher, the editors and the reviewers. Any product that may be evaluated in this article, or claim that may be made by its manufacturer, is not guaranteed or endorsed by the publisher.
Adusei-Gyamfi, J., Ouddane, B., Rietveld, L., Cornard, J. P., and Criquet, J. (2019). Natural organic matter-cations complexation and its impact on water treatment: a critical review. Water Res. 160, 130–147. doi:10.1016/j.watres.2019.05.064
Ahmed, H. K., Fawy, H. A., and Abdel-Hady, E. S. (2010). Study of sewage sludge use in agriculture and its effect on plant and soil. Agric. Biol. J. N. Am. 1, 1044–1049. doi:10.5251/abjna.2010.1.5.1044.1049
Akfas, F., Elghali, A., Aboulaich, A., Munoz, M., Benzaazoua, M., Bodinier, J. L., et al. (2024). Exploring the potential reuse of phosphogypsum: a waste or a resource?. Sci. Total. Environ. 908, 168196. doi:10.1016/j.scitotenv.2023.168196
Alam, S. I., Hammoda, H., Khan, F., Enazi, R., and Goktepe, I. (2020). Electrical conductivity, pH, organic matter and texture of selected soils around the Qatar university campus. Res. Agric. Livest. Fish. 7 (3), 403–409. doi:10.3329/ralf.v7i3.51359
Al-Enazy, A. A., Al-Barakah, F., Al-Oud, S., and Usman, A. (2018). Effect of phosphogypsum application and bacteria co-inoculation on biochemical properties and nutrient availability to maize plants in a saline soil. Archives Agron. Soil Sci. 64 (10), 1394–1406. doi:10.1080/03650340.2018.1437909
Al-Hwaiti, M. S., Ranville, J. F., and Ross, P. E. (2010). Bioavailability and mobility of trace metals in phosphogypsum from Aqaba and Eshidiya, Jordan. Chem. Erde-Geochem. 70, 283–291. doi:10.1016/j.chemer.2010.03.001
Al-Lami, M. K., Oustriere, N., Gonzales, E., and Burken, J. G. (2022). Phytomanagement of Pb/Zn/Cu tailings using biosolids-biochar or -humus combinations: enhancement of bioenergy crop production, substrate functionality, and ecosystem services. Sci. Total Environ. 836 (February), 155676. doi:10.1016/j.scitotenv.2022.155676
Almendro-Candel, M. B., Jordán, M. M., Navarro-Pedreño, J., Mataix-Solera, J., and Gómez-Lucas, I. (2007). Environmental evaluation of sewage sludge application to reclaim limestone quarries wastes as soil amendments. Soil Biol. Biochem. 39 (6), 1328–1332. doi:10.1016/j.soilbio.2006.12.008
Alvarenga, P., Clemente, R., Garbisu, C., and Becerril, J. M. (2018). Indicators for monitoring mine site rehabilitation. Bio-Geotechnologies Mine Site Rehabilitation, 49–66. doi:10.1016/B978-0-12-812986-9.00003-8
Amrani, M., Taha, Y., Kchikach, A., Benzaazoua, M., and Hakkou, R. (2019). Valorization of phosphate mineWaste rocks as materials for road construction. Minerals 9, 237. doi:10.3390/su131910751
An-nori, A., El Fels, L., Ezzariai, A., El Hayani, B., El Mejahed, K., El Gharous, M., et al. (2020). Effectiveness of helminth egg reduction by solar drying and liming of sewage sludge. Environ. Sci. Pollut. Res. 28, 14080–14091. doi:10.1007/s11356-020-11619-w
Apori, S. O., Mcmillan, D., Giltrap, M., and Tian, F. (2022). Mapping the restoration of degraded peatland as a research area: a scientometric review. Front. Environ. Sci. 10 (October), 1–22. doi:10.3389/fenvs.2022.942788
Aricak, B., Cetin, M., Erdem, R., Sevik, H., and Cometen, H. (2019). The change of some heavy metal concentrations in scotch pine (Pinus Sylvestris) depending on traffic density, organelle and washing. Appl. Ecol. Environ. Res. 17 (3), 6723–6734. doi:10.15666/aeer/1703_67236734
Asensio, V., Vega, F. A., Andrade, M. L., and Covelo, E. F. (2013). Technosols made of wastes to improve physico-chemical characteristics of a copper mine soil. Pedosphere 23 (1), 1–9. doi:10.1016/S1002-0160(12)60074-5
Baethke, K. A., Ploughe, L. W., Gardner, W. C., and Fraser, L. H. (2020). Native seedling colonization on stockpiled mine soils is constrained by site conditions and competition with exotic species. Minerals 10 (4), 361. doi:10.3390/min10040361
Baiz, Z., Azim, K., Tabrika, I., Abbas, Y., Ibijbijen, J., Hamza, A., et al. (2021). Solid phosphate sludge composted with organic horticultural residues improves the growth of citrus and forest seedlings. Org. Agric. 11 (4), 577–587. doi:10.1007/s13165-021-00358-y
Bakopoulou, A., Antonarakou, A., Lozios, S., and Zambetakis - Lekkas, A. (2013). Development and precodification of a lithosphere questionnaire as a tool in educational research (geosciences). Bull. Geol. Soc. Greece 50, 201–208. doi:10.12681/bgsg.11720
Balduíno, A., Corrêa, R., Munhoz, C. B. R., Chacon, R., and Pinto, J. R. R. (2019). Edaphic filters and plant colonization in a mine revegetated with sewage sludge. Floresta Ambiente 26 (2), 1–12. doi:10.1590/2179-8087.103917
Bateman, A. M., Erickson, T. E., Merritt, D. J., Veneklaas, E. J., and Muñoz-Rojas, M. (2021). Native plant diversity is a stronger driver for soil quality than inorganic amendments in semi-arid post-mining rehabilitation. Geoderma 394 (March), 115001. doi:10.1016/j.geoderma.2021.115001
Bayoussef, A., Loutou, M., Taha, Y., Mansori, M., Benzaazoua, M., Manoun, B., et al. (2021). Use of clays by-products from phosphate mines for the manufacture of sustainable lightweight aggregates. J. Clean. Prod. 280, 124361. doi:10.1016/j.jclepro.2020.124361
Bohoussou, Y. N. D., Kou, Y. H., Yu, W. B., Lin, B. jian, Virk, A. L., Zhao, X., et al. (2022). Impacts of the components of conservation agriculture on soil organic carbon and total nitrogen storage: a global meta-analysis. Sci. Total Environ. 842 (July), 156822. doi:10.1016/j.scitotenv.2022.156822
Bohoussou, N. D. Y., Han, S.-W., and Li, H.-R. (2023). Effects of fertilizer application strategies on soil organic carbon and total nitrogen storage under different agronomic practices: a meta-analysis. Land. Degrad. Dev. 34 (18), 5889–5904.
Bolan, N. S., Kirkham, M. B., and Ok, Y. S. (2017). “Spoil to soil mine site rehabilitation and revegetation,” in Spoil to soil. doi:10.1201/9781351247337
Bolaños-Guerrón, D., Capa, J., and Flores, L. C. (2021). Retention of heavy metals from mine tailings using Technosols prepared with native soils and nanoparticles. Heliyon 7 (7), e07631. doi:10.1016/j.heliyon.2021.e07631
Bossolani, J. W., Crusciol, C. A. C., Moretti, L. G., Garcia, A., Portugal, J. R., Bernart, L., et al. (2022). Improving soil fertility with lime and phosphogypsum enhances soybean yield and physiological characteristics. Agron. Sustain. Dev. 42 (2), 26. doi:10.1007/s13593-022-00765-9
Boutaleb, F. N., Boutaleb, B. B., Deblij, S., and El, A. S. (2020). Effect of phosphate mine tailings from Morocco on the mechanical properties of ceramic tiles. Int. J. Eng. Res. And V9 (02), 140–145. doi:10.17577/ijertv9is020092
Chakraborty, S. R., Azim, R., Rezaur Rahman, A. K. M., and Sarker, R. (2013). Radioactivity concentrations in soil and transfer factors of radionuclides from soil to grass and plants in the chittagong city of Bangladesh. J. Physi. Sci. 24 (1), 95–113.
Chi, C. M., Zhao, C. W., Sun, X. J., and Wang, Z. C. (2012). Reclamation of saline-sodic soil properties and improvement of rice (Oriza sativa L.) growth and yield using desulfurized gypsum in the west of Songnen Plain, northeast China. Geoderma 187–188, 24–30. doi:10.1016/j.geoderma.2012.04.005
Contreras, M., Teixeira, S. R., Santos, G. T. A., Gázquez, M. J., Romero, M., and Bolívar, J. P. (2018). Influence of the addition of phosphogypsum on some properties of ceramic tiles. Constr. Build. Mater. 175, 588–600. doi:10.1016/j.conbuildmat.2018.04.131
Cordell, D., Drangert, J. O., and White, S. (2009). The story of phosphorus: global food security and food for thought. Glob. Environ. Change 19 (2), 292–305. doi:10.1016/j.gloenvcha.2008.10.009
Cox, J. A., and Whelan, R. J. (2000). Soil development of an artificial soil mix: nutrient dynamics, plant growth, and initial physical changes. Aust. J. Soil Res. 40 (38), 465–477. doi:10.1071/sr99048
Czekala, J. (1999). Sewage sludges as a source of organic matter and nutrients. Folia. Univer. Agri. Steti. Agri. (77), 33–37.
Derhy, M., Taha, Y., Hakkou, R., and Benzaazoua, M. (2020). Review of the main factors affecting the flotation of phosphate ores. Minerals 10 (12), 1109–1122. doi:10.3390/min10121109
Ding, Q., Cheng, G., Wang, Y., and Zhuang, D. (2017). Effects of natural factors on the spatial distribution of heavy metals in soils surrounding mining regions. Sci. Total Environ. 578, 577–585. doi:10.1016/j.scitotenv.2016.11.001
Es-Said, A., Nafai, H., Hamdaoui, L. E., Bouhaouss, A., and Bchitou, R. (2020). Adsorptivity and selectivity of heavy metals cd(Ii), cu(ii), and zn(ii) toward phosphogypsum. Desalination Water Treat. 197, 291–299. doi:10.5004/dwt.2020.25964
Feng, J., Wei, W., and Pan, D. (2020). Effects of rainfall and terracing-vegetation combinations on water erosion in a loess hilly area, China. J. Environ. Manag. 261, 110247. doi:10.1016/j.jenvman.2020.110247
Galfati, I., Bilal, E., Sassi, A. B., Abdallah, H., and Zaier, A. (2011). Accumulation of heavy metals in native plants growing near the phosphate treatment industry, Tunisia. Carpathian J. Earth Environ. Sci. 6 (2), 85–100.
Gharabaghi, M., Irannajad, M., and Noaparast, M. (2010). A review of the beneficiation of calcareous phosphate ores using organic acid leaching. Hydrometallurgy 103 (1–4), 96–107. doi:10.1016/j.hydromet.2010.03.002
Gosh, M., and Singh, S. P. (2005). A review on phytoremediation of heavy metals and utilization of it’s by products. Euphytica 6 (04), 214–231. doi:10.1007/s10681-014-1088-2
Grandlic, C. J., Mendez, M. O., Chorover, J., Machado, B., and Maier, R. M. (2008). Plant growth-promoting bacteria for phytostabilization of mine tailings. Environ. Sci. Technol. 42 (6), 2079–2084. doi:10.1021/es072013j
Guéablé, Y. K. D., Bezrhoud, Y., Aké, A. H. J., Moulay, H., An-nori, A., Soulaimani, A., et al. (2022a). New approach for mining site reclamation using alternative substrate based on phosphate industry by-product and sludge (Part 2): metals transfer to plant and soil microbial density. Sustainability 14 (11359), 11359. doi:10.3390/su141811359
Guéablé, Y. K. D., Bezrhoud, Y., Moulay, H., Moughli, L., Hafidi, M., El Gharouss, M., et al. (2021). New approach for mining site reclamation using alternative substrate based on phosphate industry by-product and sludge mixture. Sustain. Switz., 13(10751), 10751. doi:10.3390/su131910751
Guéablé, Y. K. D., Uke, O. D., Bezrhoud, Y., Moulay, H., Moughli, L., Hafidi, M., et al. (2022b). Study of the sodicity of phosphate by-products and sludge mixture for large-scale application in mine site reclamation. Environ. Sci. Proc. 18, 18. doi:10.3390/environsciproc2022016018
Guerrero, J. L., Pérez-Moreno, S. M., Gutiérrez-Álvarez, I., Gázquez, M. J., and Bolívar, J. P. (2021). Behaviour of heavy metals and natural radionuclides in the mixing of phosphogypsum leachates with seawater. Environ. Pollut. 268, 115843. doi:10.1016/j.envpol.2020.115843
Gupta, N., Yadav, K. K., Kumar, V., Kumar, S., Chadd, R. P., and Kumar, A. (2019). Trace elements in soil-vegetables interface: translocation, bioaccumulation, toxicity and amelioration - a review. Sci. Total Environ. 651, 2927–2942. doi:10.1016/j.scitotenv.2018.10.047
Hakkou, R., Benzaazoua, M., and Bussière, B. (2016). Valorization of phosphate waste rocks and sludge from the Moroccan phosphate mines: challenges and perspectives. Procedia Eng. 138, 110–118. doi:10.1016/j.proeng.2016.02.068
Hamza, Z. M., Kadhim, S. A., and Hussein, H. H. (2019). Assessment the norms for agricultural soils in Ghammastown. Iraq: Plant Arch 19, 1483–1490.
Hindersah, R., Handyman, Z., Indriani, F. N., Suryatmana, P., and Nurlaeny, N. (2018). Azotobacter population, soil nitrogen and groundnut growth in mercury-contaminated tailing inoculated with Azotobacter. J. Degrade. Min. Land Manage 5 (53), 2502–2458. doi:10.15243/jdmlm
Illera, V., Garrido, F., Vizcayno, C., and García-González, M. T. (2004). Field application of industrial by-products as Al toxicity amendments: chemical and mineralogical implications. Eur. J. Soil Sci. 55 (4), 681–692. doi:10.1111/j.1365-2389.2004.00640.x
Issaad, M., Boutaleb, A., Kolli, O., Edahbi, M., Benzaazoua, M., and Hakkou, R. (2019). Environmental characterization of mine waste at the Pb–Zn Sidi Kamber abandoned mine (NE Algeria). Rendiconti Lincei 30 (2), 427–441. doi:10.1007/s12210-019-00806-8
Iyyappan, J., Baskar, G., Deepanraj, B., Anand, A. V., Saravanan, R., and Awasthi, M. K. (2023). Promising strategies of circular bioeconomy using heavy metal phytoremediated plants – a critical review. Chemosphere 313, 137097. doi:10.1016/j.chemosphere.2022.137097
Javied, S., Waheed, S., Siddique, N., Chaudhry, M. M., Irfan, N., and Tufail, M. (2009). Arsenic pollution from phosphogypsum produced at multan, Pakistan. Nucl. 46 (3), 219–224.
Kammoun, M., Ghorbel, I., Charfeddine, S., Kamoun, L., Gargouri-Bouzid, R., and Nouri-Ellouz, O. (2017). The positive effect of phosphogypsum-supplemented composts on potato plant growth in the field and tuber yield. J. Environ. Manag. 200, 475–483. doi:10.1016/j.jenvman.2017.06.016
Karimi, P., Yazdanbakhsh, A., Atamaleki, A., Kariminejad, F., Ahmadian, M., and Fallah, S. (2021). Global evaluation of potentially harmful elements (PHEs) in potato and carrot irrigated by wastewater: a systematic review, meta-analysis, and health risk assessment. Environ. Health Eng. Manag. 8 (4), 245–255. doi:10.34172/EHEM.2021.28
Kelly, G. (2006). Recycled organics in mine site rehabilitation: a review of the scientific literature. Parramatta, NSW: Department of Environment and Conservation NSW. Available at: http://141.243.32.146/resources/warr/2006184_ORG_MineLitReview.pdf.
Khan, R., Shukla, S., Kumar, M., Zuorro, A., and Pandey, A. (2023). Sewage sludge derived biochar and its potential for sustainable environment in circular economy: advantages and challenges. Chem. Eng. J. 471 (April), 144495. doi:10.1016/j.cej.2023.144495
Kragt, M., Lison, C., Manero, A., and Hawkis, J. (2019). Mine site rehabilitation conditions in Western Australia. Proc. 13th Int. Conf. Mine Clos. 2018, 95–110. doi:10.36487/acg_rep/1915_09_kragt
Li, J., Zhang, J., Larson, S. L., Ballard, J. H., Guo, K., Arslan, Z., et al. (2019). Electrokinetic-enhanced phytoremediation of uranium-contaminated soil using sunflower and Indian mustard. Int. J. Phytoremediation 21 (12), 1197–1204. doi:10.1080/15226514.2019.1612847
Li, M. S. (2006). Ecological restoration of mineland with particular reference to the metalliferous mine wasteland in China: a review of research and practice. Sci. Total Environ. 357 (1–3), 38–53. doi:10.1016/j.scitotenv.2005.05.003
Li, Q., Wang, Y., Li, Y., Li, L., Tang, M., Hu, W., et al. (2022). Speciation of heavy metals in soils and their immobilization at micro-scale interfaces among diverse soil components. Sci. Total Environ. 825, 153862. doi:10.1016/j.scitotenv.2022.153862
Liang, Z., Peng, X., and Luan, Z. (2012). Immobilization of Cd, Zn and Pb in sewage sludge using red mud. Environ. Earth Sci. 66 (5), 1321–1328. doi:10.1007/s12665-011-1341-0
Loutou, M., Hajjaji, M., Babram, M. A., Mansori, M., Favotto, C., and Hakkou, R. (2017). Phosphate sludge-based ceramics: microstructure and effects of processing factors. J. Build. Eng. 11, 48–55. doi:10.1016/j.jobe.2017.04.002
Luna, L., Pastorelli, R., Bastida, F., Hernández, T., García, C., Miralles, I., et al. (2016). The combination of quarry restoration strategies in semiarid climate induces different responses in biochemical and microbiological soil properties. Appl. Soil Ecol. 107, 33–47. doi:10.1016/j.apsoil.2016.05.006
Lyczko, N., Nzihou, A., and Sharrok, P. (2014). Calcium phosphate sorbent for environmental application. Procedia Eng. 83, 423–431. doi:10.1016/j.proeng.2014.09.051
Mahmoud, E., and Abd El-Kader, N. (2015). Heavy metal immobilization in contaminated soils using phosphogypsum and rice straw compost. Land Degrad. Dev. 26 (8), 819–824. doi:10.1002/ldr.2288
Mahmoud, E., Ghoneim, A., El Baroudy, A., Abd El-Kader, N., Aldhumri, S. A., Othman, S., et al. (2021). Effects of phosphogypsum and water treatment residual application on key chemical and biological properties of clay soil and maize yield. Soil Use Manag. 37 (3), 494–503. doi:10.1111/sum.12583
Mahmoud, E. K., and El-Kader, N. A. (2013). Soil immobilization of heavy metal using soil amendments in a greenhouse study. Compost Sci. Util. 21 (3–4), 156–163. doi:10.1080/1065657X.2013.859531
Mariana Campos Assunção, P. (2017). Université Pierre et Marie Curie École doctorale ED388 Étude physicochimique et formulation d’un nouveau solvant d’extraction pour la purification de l’acide phosphorique.
Meyer, S., Burger, D., and Jürgens, N. (2009). “Analysis and evaluation of ecological restoration experiments in the Succulent Karoo (South Africa). Diplom thesis in geoecology,” in Institute für Geographie und Geoökologie, Hamburg, Germany (Karlsruhe: University of Karlsruhe, August), 92.
Moreno-Barriga, F., Díaz, V., Acosta, J. A., Muñoz, M. Á., Faz, Á., and Zornoza, R. (2017). Organic matter dynamics, soil aggregation and microbial biomass and activity in Technosols created with metalliferous mine residues, biochar and marble waste. Geoderma 301, 19–29. doi:10.1016/j.geoderma.2017.04.017
Moukannaa, S., Loutou, M., Benzaazoua, M., Vitola, L., Alami, J., and Hakkou, R. (2018). Recycling of phosphate mine tailings for the production of geopolymers. J. Clean. Prod. 185, 891–903. doi:10.1016/j.jclepro.2018.03.094
Nayak, A. K., Mishra, V. K., Sharma, D. K., Jha, S. K., Singh, C. S., Shahabuddin, M., et al. (2013). Efficiency of phosphogypsum and mined gypsum in reclamation and productivity of rice-wheat cropping system in sodic soil. Commun. Soil Sci. Plant Analysis 44 (5), 909–921. doi:10.1080/00103624.2012.747601
Nayak, S., Mishra, C. S., Guru, B., and Rath, M. (2011). Effect of phosphogypsum amendment on soil physico-chemical properties, microbial load and enzyme activities. J. Environ. Biol. 32, 613–617. Available at: www.jeb.cowww.jeb.co
Neina, D. (2019). The role of soil pH in plant nutrition and soil remediation. Appl. Environ. Soil Sci. 2019 (5794869), 1–9. doi:10.1155/2019/5794869
Ondreičková, K., Gubišová, M., Gubiš, J., Klčová, L., and Horník, M. (2019). Rhizosphere bacterial communities of arundo Donax grown in soil fertilised with sewage sludge and agricultural by-products. Agric. (Pol’nohospodarstvo) 65 (1), 37–41. doi:10.2478/agri-2019-0004
Outbakat, M. B., El Mejahed, K., El Gharous, M., El Omari, K., and Beniaich, A. (2022). Effect of phosphogypsum on soil physical properties in Moroccan salt-affected soils. Sustain. Switz. 14 (20), 1–14. doi:10.3390/su142013087
Peñaranda Barba, M. A., Alarcón Martínez, V., Gómez Lucas, I., and Navarro Pedreño, J. (2020). Methods of soil recovery in quarries of arid and semiarid areas using different waste types. Span. J. Soil Sci. 10 (2), 101–122. doi:10.3232/SJSS.2020.V10.N2.01
Peregrina Alonso, F., Santano Arias, J., Ordónez Fernández, R., González Fernández, P., and Espejo Serrano, R. (2006). Agronomic implications of the supply of lime and gypsum by-products to Palexerults from western Spain. Soil Sci. 171 (1), 65–81. doi:10.1097/01.ss.0000200557.253069.50
Provenzano, R. S., Moreira, A., Moraes, L. A. C., and Cabrera, R. A. D. (2020). Agronomic efficiency of sulfur sources and interaction of sulfur with micronutrients. J. Plant Nutr. 43 (11), 1674–1680. doi:10.1080/01904167.2020.1739300
Qian, L., Lin, H., Li, B., and Dong, Y. (2023). Physicochemical characteristics and microbial communities of rhizosphere in complex amendment-assisted soilless revegetation of gold mine tailings. Chemosphere 320, 138052. doi:10.1016/j.chemosphere.2023.138052
Rao, A. V., Richa, T. A. K., and Tak, R. (2001). Influence of organic amendments on the growth of different trees and nutrient uptake in gypsum minespoils. J. Indi. Socie. Soil. Sci. 49 (2), 386–390.
Reta, G., Dong, X., Li, Z., Su, B., Hu, X., Bo, H., et al. (2018). Environmental impact of phosphate mining and beneficiation: review. Int. J. Hydrology 2 (4), 424–431. doi:10.15406/ijh.2018.02.00106
Saadaoui, E., Ghazel, N., Ben Romdhane, C., and Massoudi, N. (2017). Phosphogypsum: potential uses and problems–a review. Int. J. Environ. Stud. 74 (4), 558–567. doi:10.1080/00207233.2017.1330582
Saadaoui, H., Ghennioui, A., Ikken, B., Rhinane, H., and Maanan, M. (2019). Using GIS and photogrammetry for assessing solar photovoltaic potential on Flat Roofs in urban area case of the city of Ben Guerir/Morocco. Int. Archives Photogrammetry, Remote Sens. Spatial Inf. Sci. - ISPRS Archives 42 (4/W12), 155–166. doi:10.5194/isprs-archives-XLII-4-W12-155-2019
Santos, E. S., Abreu, M. M., and Macías, F. (2019). Rehabilitation of mining areas through integrated biotechnological approach: technosols derived from organic/inorganic wastes and autochthonous plant development. Chemosphere 224, 765–775. doi:10.1016/j.chemosphere.2019.02.172
Scanlon, B. R., Reedy, R. C., Keese, K. E., and Dwyer, S. F. (2005). Evaluation of evapotranspirative covers for waste containment in arid and semiarid regions in the southwestern USA. Vadose Zone J. 4 (1), 55–71. doi:10.2113/4.1.55
Sheoran, V., Sheoran, A., and Poonia, P. (2010). Soil reclamation of abandoned mine land by revegetation: a review. Int. J. Soil, Sediment Water 3 (2), 13.
Singh, Y. P., Arora, S., Mishra, V. K., Dixit, H., and Gupta, R. K. (2018). Effect of organic and inorganic amendments on amelioration of sodic soil and sustaining rice (Oryza sativa)-wheat (Triticum aestivum) productivity. Indian J. Agric. Sci. 88 (9), 1455–1462. doi:10.56093/ijas.v88i9.83515
Smaoui-Jardak, M., Kriaa, W., Maalej, M., Zouari, M., Kamoun, L., Trabelsi, W., et al. (2017). Effect of the phosphogypsum amendment of saline and agricultural soils on growth, productivity and antioxidant enzyme activities of tomato (Solanum lycopersicum L.). Ecotoxicology 26 (8), 1089–1104. doi:10.1007/s10646-017-1836-x
Smyrnova-Trybulska, E., Morze, N., Kuzminska, O., and Kommers, P. (2018). Mapping and visualization: selected examples of international research networks. J. Inf. Commun. Ethics Soc. 16 (4), 381–400. doi:10.1108/JICES-03-2018-0028
Tabrika, I., Mayad, E. H., Zaafrani, M., Guilli, M., and Azim, K. (2021). Optimization of solid phosphate sludge composting by integration of horticultural waste. Org. Agric. 11 (2), 319–325. doi:10.1007/s13165-020-00302-6
Takasu, E., Yamada, F., Shimada, N., Kumagai, N., Hirabayashi, T., and Saigusa, M. (2006). Effect of phosphogypsum application on the chemical properties of Andosols, and the growth and Ca uptake of melon seedlings. Soil Sci. Plant Nutr. 52 (6), 760–768. doi:10.1111/j.1747-0765.2006.00093.x
Turkyilmaz, A., Cetin, M., Sevik, H., Isinkaralar, K., and Saleh, E. A. A. (2020). Variation of heavy metal accumulation in certain landscaping plants due to traffic density. Environ. Dev. Sustain. 22 (3), 2385–2398. doi:10.1007/s10668-018-0296-7
Usman, K., Khan, S., Ghulam, S., Khan, M. U., Khan, N., Khan, M. A., et al. (2012). Sewage sludge: an important biological resource for sustainable agriculture and its environmental implications. Am. J. Plant Sci. 03 (12), 1708–1721. doi:10.4236/ajps.2012.312209
Vagelas, I., and Leontopoulos, S. (2023). A bibliometric analysis and a citation mapping process for the role of soil recycled organic matter and microbe interaction due to climate change using Scopus database. AgriEngineering 5 (1), 581–610. doi:10.3390/agriengineering5010037
van Eck, N. J. (2011). Methodological advances in bibliometric mapping of science. Available at: http://repub.eur.nl/res/pub/26509/.
Venkatraman, K., and Ashwath, N. (2010). Field performance of a phytocap at lakes creek landfill, rockhampton, Australia. Manag. Environ. Qual. An Int. J. 21 (2), 237–252. doi:10.1108/14777831011025571
Violante, A., Cozzolino, V., Perelomov, L., Caporale, A. G., and Pigna, M. (2010). Mobility and bioavailability of heavy metals and metalloids in soil environments. J. Soil Sci. Plant Nutr. 10 (3), 268–292. doi:10.4067/S0718-95162010000100005
Wendling, L. A., Blomberg, P., Sarlin, T., Priha, O., and Arnold, M. (2013). Phosphorus sorption and recovery using mineral-based materials: sorption mechanisms and potential phytoavailability. Appl. Geochem. 37, 157–169. doi:10.1016/j.apgeochem.2013.07.016
Wijesekara, H., Bolan, N. S., Kumarathilaka, P., Geekiyanage, N., Kunhikrishnan, A., Seshadri, B., et al. (2016). “Biosolids enhance mine site rehabilitation and revegetation,” in Environmental materials and waste: resource recovery and pollution prevention, 138. Elsevier Inc, 45–71. doi:10.1016/B978-0-12-803837-6.00003-2
Wong, M. H. (2003). Ecological restoration of mine degraded soils, with emphasis on metal contaminated soils. Chemosphere 50 (6), 775–780. doi:10.1016/S0045-6535(02)00232-1
Wu, Y., Li, Y., Zheng, C., Zhang, Y., and Sun, Z. (2013). Organic amendment application influence soil organism abundance in saline alkali soil. Eur. J. Soil Biol. 54, 32–40. doi:10.1016/j.ejsobi.2012.10.006
Yang, Y. Y., Wu, H. N., Shen, S. L., Horpibulsuk, S., Xu, Y. S., and Zhou, Q. H. (2014). Environmental impacts caused by phosphate mining and ecological restoration: a case history in Kunming, China. Nat. Hazards 74 (2), 755–770. doi:10.1007/s11069-014-1212-6
Zanuzzi, A., Arocena, J. M., van Mourik, J. M., and Faz Cano, A. (2009). Amendments with organic and industrial wastes stimulate soil formation in mine tailings as revealed by micromorphology. Geoderma 154 (1–2), 69–75. doi:10.1016/j.geoderma.2009.09.014
Zanuzzi, A., Faz, A., and Acosta, J. A. (2013). Chemical stabilization of metals in the environment: a feasible alternative for remediation of mine soils. Environ. Earth Sci. 70 (6), 2623–2632. doi:10.1007/s12665-013-2313-3
Zhang, S., Zhu, Q., de Vries, W., Ros, G. H., Chen, X., Muneer, M. A., et al. (2023). Effects of soil amendments on soil acidity and crop yields in acidic soils: a world-wide meta-analysis. J. Environ. Manag. 345, 118531. doi:10.1016/j.jenvman.2023.118531
Keywords: mining site, phosphate sludge, phosphogypsum, rehabilitation, sewage sludge
Citation: Guéablé YKD, Bohoussou NY, El Gharous M, El Mejahed K and Hafidi M (2025) Using by-products for rehabilitating mine sites or degraded lands: systematic review and meta-analysis on phosphogypsum, phosphate sludge and sewage sludge. Front. Environ. Sci. 13:1509156. doi: 10.3389/fenvs.2025.1509156
Received: 10 October 2024; Accepted: 27 January 2025;
Published: 26 February 2025.
Edited by:
Ming Xia, Jiangsu Ocean University, ChinaReviewed by:
Mirjana B. Radenkovic, University of Belgrade, SerbiaCopyright © 2025 Guéablé, Bohoussou, El Gharous, El Mejahed and Hafidi. This is an open-access article distributed under the terms of the Creative Commons Attribution License (CC BY). The use, distribution or reproduction in other forums is permitted, provided the original author(s) and the copyright owner(s) are credited and that the original publication in this journal is cited, in accordance with accepted academic practice. No use, distribution or reproduction is permitted which does not comply with these terms.
*Correspondence: Khalil El Mejahed, a2hhbGlsLmVsbWVqYWhlZEB1bTZwLm1h; Mohamed Hafidi, aGFmaWRpQHVjYS5hYy5tYQ==
Disclaimer: All claims expressed in this article are solely those of the authors and do not necessarily represent those of their affiliated organizations, or those of the publisher, the editors and the reviewers. Any product that may be evaluated in this article or claim that may be made by its manufacturer is not guaranteed or endorsed by the publisher.
Research integrity at Frontiers
Learn more about the work of our research integrity team to safeguard the quality of each article we publish.