- 1Department of Earth and Environmental Sciences, Vanderbilt University, Nashville, TN, United States
- 2Department of Oceanography, Noakhali Science and Technology University, Noakhali, Bangladesh
- 3School of Physics, Chemistry and Earth Sciences, Faculty of Sciences, Engineering and Technology, The University of Adelaide, Adelaide, SA, Australia
- 4Department of Oceanography, University of Dhaka, Dhaka, Bangladesh
- 5Geologist, Bangladesh Water Development Board, Dhaka, Bangladesh
- 6Department of Geology, University of Dhaka, Dhaka, Bangladesh
- 7School of Geosciences, Faculty of Science, University of Sydney, Darlington, Australia
- 8Department of Disaster Science and Climate Resilience, University of Dhaka, Dhaka, Bangladesh
This study investigates the contamination of groundwater in Dhaka City, Bangladesh, focusing on six potentially toxic elements, including As, Cu, Mn, Cr, Al, and B, due to their implications for public health as groundwater serves as the primary source of drinking water in the region. 15 samples were taken into consideration and was analyzed for six elements (As, Cu, Mn, Cr, Al, and B) using an Atomic Absorption Spectrophotometer (AAS). Arsenic (As) and chromium (Cr) were undetectable in all samples. The average concentrations of Copper (Cu), Aluminum (Al), Boron (B), and Manganese (Mn) were measured at 0.075 mg/L, 0.087 mg/L, 1.14 mg/L, and 0.48 mg/L, respectively. Among these, only one sample (S-05) exceeded the WHO (2022) drinking water limits for Boron, and 10 samples surpassed the limits for Manganese. Pollution indices like MEI, NI, and Cd were used to evaluate contamination levels, revealing significant pollution in multiple samples. Metal evaluation index (MEI) values were found between 0.39 and 17.97 with the average of 6.71. The average values of the Nemerow pollution index (NI) and degree of contamination (Cd) were found sequentially to be 4.35 and 5.71. In both cases, eight samples were found to be highly contaminated. The observed hazard index (HI) values for adults varied from 0.20 to 2.47, whereas for children it ranged from 0.32 to 3.93. All samples indicate values of children exceed the HI values of adults which indicates that children are more susceptible than adults through oral exposure to drinking water. The elevated concentrations of manganese were the primary cause of the higher NI, Cd, and HI values in eight samples. This study highlights groundwater contamination as a critical public health concern and advocates the need for mitigation efforts to ensure safe drinking water access. The study underscores the urgent need for implementing stricter groundwater management policies and public health interventions to mitigate contamination risks.
1 Introduction
Groundwater has emerged as a paramount water source for several sectors globally, including drinking, irrigation, and industry, owing to its exceptional quality, reduced seasonal fluctuations, and convenient utilization (Barlow and Reichard, 2010; Güler et al., 2012; Carol et al., 2013; Huang et al., 2013; Patel et al., 2016). The quality of the groundwater is significantly influenced by natural phenomena, which encompass the geological and mineralogical characteristics of aquatic systems and subterranean aquifers, the dynamics of groundwater flow, interactions between water and geological formations, and the mixing of different water sources (Schot and Van der Wal, 1992; Appelo and Postma, 2004; Nagarajan et al., 2010; Alexakis, 2011). Yet, a variety of human activities can change the composition of groundwater, including excessive water withdrawal, fertilizer application, the impact of irrigation water circulation, and the infiltration of water from septic systems and municipal waste disposal sites (Kumar et al., 2011; Triki et al., 2014).
Through the chemical weathering of rocks and minerals, elements like arsenic (As), iron (Fe), copper (Cu), boron (B), manganese (Mn), cadmium (Cd), zinc (Zn), lead (Pb), chromium (Cr), nickel (Ni), aluminum (Al), and others are released into groundwater (Singh, 2005; Rango et al., 2009; Tajwar and Uddin, 2021). Potentially toxic elements releases into shallow groundwater are also caused by human activities such as urbanization, industrialization, dumping of solid waste, and agricultural practices (Kumar et al., 2016; Pugazhendhi et al., 2018; Bhattacharjee et al., 2019; Saha et al., 2021). These hazardous elements can pose a considerable risk to human health, even at low amounts (Ali et al., 2019). According to several studies (Muhammad et al., 2011; Lu et al., 2015; Nkpaa et al., 2018; Singh et al., 2018), consuming water contaminated with these metals can lead to several detrimental health consequences, including neurological diseases, gastrointestinal bleeding, vascular disease, hypertension, cancer, restrictive lung disorder, and reproductive concerns. Particularly arsenic has long-term harmful effects on health, including disorders of the digestive system, decreased blood cell synthesis, exhaustion, skin irritations, malignant skin growth, and a variety of cancers affecting the bladder, liver, and lungs in addition to neurological and cardiovascular conditions (Hughes, 2002). Excessive consumption of drinking water containing copper can lead to nausea, diarrhea, upset stomach, and damage to liver tissue (National Research Council et al., 2000). According to (Adimalla and Li, 2019) long-term chromium exposure can cause neurological, gastrointestinal, cardiovascular, renal, hepatic, and even fatal consequences on the hematopoiesis. Long-term high manganese exposure can damage DNA and cause neurological problems and diminished intellectual function (World Health Organization, 2021). High boron levels in drinking water are harmful to human health; in babies, they can cause vomiting, diarrhea, skin rashes, irritability, and convulsive convulsions. In adults, they can cause comparable symptoms such as nausea, vomiting, diarrhea, skin redness, and throat ulcers (Epa, 2011). Drinking water contaminated with aluminum has been linked to non-carcinogenic health hazards such as renal failure, Alzheimer’s disease, dementia, and disorders of the bones or brain (Ingerman et al., 2008; Lina and Shaharuddin, 2017). Moreover, groundwater containing iron (Fe), mercury (Hg), lead (Pb), and cadmium (Cd) may be harmful to people’s health. (Tirkey et al., 2017; Rahman et al., 2020; Tajwar et al., 2023).
Many studies have documented high levels of arsenic, iron, manganese, boron, and fluoride contamination in groundwater at different locations in Bangladesh (Islam and Mostafa, 2024). For instance, arsenic contamination in groundwater has been a well-documented issue, particularly in the southern regions of Bangladesh. A study by (Ahmed et al., 2004) identified significant arsenic levels in alluvial aquifers, with concentrations exceeding the World Health Organization’s (WHO) guidelines for safe drinking water. These findings were consistent with studies in the coastal regions, where arsenic, along with manganese and boron, were frequently detected at elevated levels, posing severe public health risks (Rahman et al., 2020). In urban areas like Chittagong and Khulna, similar patterns of contamination have been observed, particularly regarding manganese and boron, where industrial discharges and the use of fertilizers have contributed to the increasing contamination levels (Bodrud-Doza et al., 2019b).
Studies in the coastal region of Bangladesh have shown fluoride contamination along with manganese and boron as dominant pollutants in groundwater, further complicating the water quality scenario (Rahman et al., 2021b). The findings of elevated manganese levels in Dhaka City are consistent with those in other cities such as Khulna and Rajshahi, where high manganese concentrations were linked to both natural mineral weathering and industrial effluents (Hoque et al., 2014). Boron contamination, identified in both urban and coastal regions, was often attributed to agricultural runoff and the use of boron-based fertilizers, reflecting the widespread anthropogenic impact on groundwater quality (Mustafa, 2008).
Moreover, studies conducted in other South Asian countries, such as India and Pakistan, have documented similar patterns of contamination. In India’s Subarnarekha River Basin, high levels of arsenic and iron were reported, correlating with Bangladesh’s experience of naturally occurring arsenic contamination in groundwater (Giri and Singh, 2015). In the Kohistan region of Pakistan, elevated levels of manganese and boron were found to be closely associated with agricultural practices and poor waste management, similar to the situation in Dhaka (Muhammad et al., 2011). These findings highlight the regional nature of groundwater contamination in South Asia, emphasizing the need for collaborative mitigation strategies (Ahmed et al., 2004; Atikul Islam et al., 2017; Rahman et al., 2018; 2021b; 2021a; Tajwar and Uddin, 2022). The sources of the detected toxic elements in groundwater can be attributed to both natural and anthropogenic activities. Natural sources include the weathering of rocks and the dissolution of minerals containing elements like manganese, boron, and arsenic. Human activities, such as industrial discharge, agricultural runoff, and improper waste management, also contribute significantly to groundwater contamination (Ahmed et al., 2004; Rahman et al., 2020).
Like other urbanized regions of Bangladesh and its neighboring countries, the capital, Dhaka, is significantly reliant on groundwater resources for multiple purposes. This increased reliance is eliciting concerns regarding water quality. Although prior research has examined groundwater contamination in different regions of Bangladesh, there is a shortage of studies specifically addressing the occurrence of naturally occurring potentially toxic elements (PTEs) such as arsenic (As), manganese (Mn), chromium (Cr), copper (Cu), aluminum (Al), and boron (B) in the aquifers of Dhaka. Moreover, there is an absence of thorough risk assessments regarding the health effects of these contaminants on the residents of Dhaka. Hence, a substantial gap exists in the evaluation of particular contaminants and their role in detrimental health effects on the capital’s inhabitants. Addressing these deficiencies is crucial to protect public health and guide sustainable groundwater management strategies. To mitigate these issues, strategies such as improving industrial waste treatment, promoting best management practices in agriculture, and enhancing groundwater monitoring systems need to be implemented (Mustafa, 2008).
This study aims to evaluate whether the groundwater of Dhaka city is contaminated with naturally occurring potentially toxic elements (PTEs) and to assess their concentrations against national and international drinking water standards. Moreover, the target is to quantify pollution indices, hazard indices, and health risks associated with oral exposure routes by identifying the sources of these contaminants, whether geogenic or anthropogenic. The ultimate goal is to provide evidence-based recommendations for policymakers and stakeholders to mitigate risks and enhance sustainable groundwater management practices in Dhaka.
2 Materials and methods
2.1 Study area
This research emphasized some specific areas of Dhaka City, situated within the latitudinal range of 23°40′to 23°54′N and the longitudinal range of 90°20′to 90°28′E (Figure 1). Dhaka city covers about 370 square kilometers and is surrounded by interconnected streams, namely the Balu River to the east, the Turag River to the west, the Tongi Canal to the north, and the Buriganga River to the south. However, these rivers are not extensively utilized for drinking water compared to groundwater. Dhaka City comprises 40% of the Madhupur Tract, formed during the Pleistocene period, while the remaining 60% consists of recent alluvial deposits containing both ancient and recent floodplain and, river channel sediments (Hoque et al., 2007; 2014). Those cities surrounding rivers are influenced by several faults on the Madhupur Tract, which can impact the interaction between the aquifer and the rivers (Hoque et al., 2007).
The geological and hydrogeological condition of the Dhaka city is very complex. Geologically the main aquifer of Dhaka city lies within the Holocene and Pleistocene sediments of Ganges-Brahmaputra delta (Khan et al., 2011). Throughout the study area, the Madhupur formation is overlain by the Pliocene Dupi Tila sand formation that formulate the main aquifer system (Hasan et al., 2022). The main aquifer system in this region is characterized as semi-confined to confined. Groundwater recharge in Dhaka occurs primarily through rainfall, river infiltration, and surface water interactions (Hasan et al., 2019). However, urbanization has altered natural recharge patterns, with increased surface runoff and reduced infiltration due to extensive concrete cover. Groundwater extraction in Dhaka is high, especially for drinking water and irrigation, leading to concerns about depletion and the long-term sustainability of the aquifers (Ahmed et al., 2010; Akther et al., 2010).
The rapid urbanization of Dhaka, with its dense population and industrial growth, has significantly impacted groundwater quality (Bodrud-Doza et al., 2019b). Industrial effluents, untreated sewage, and landfill leachate are some of the major sources of groundwater contamination (Bodrud-Doza et al., 2020). Dhaka lacks a proper wastewater treatment infrastructure, and untreated wastewater from households and industries is often discharged directly into the environment, leading to contamination of both surface and groundwater (Bodrud-Doza et al., 2020).
2.2 Groundwater sampling and chemical analyses
Fifteen groundwater samples were collected from monitoring wells at depths of 60–90 m, representing the maximum feasible number given the constraints of this megacity, balancing the need for quality data and practical considerations, adhering to American Public Health Association (APHA) standards for sampling, preservation, and analysis. Given the circumstances that the study area’s groundwater table is at 30–78 m, residents of this megacity are likely to encounter this aquifer water while installing water wells at minimal cost. Sampling sites were selected by considering all the major residential, industrial and other contamination sources in the concerned area. Sampling locations are well distributed for best representation and a density of 0.6 samples per sq. km (15 samples for 25 sq. km) was maintained to meet the global standard (Luo et al., 2019) for similar studies. Samples were sorted by evaluating the borehole lithologs to maintain the same source of aquifer throughout the study. Stagnant water was pumped from the wells for at least ten minutes before sample collection. Two bottles were taken from each site—one acidified with 1% v/v concentrated HNO₃ and the other left unacidified. All samples were filtered through a 0.45 μm syringe-head membrane filter immediately after collection. A geologist from the Bangladesh Water Development Board supervised the process at the sampling locations. The samples were transported to the laboratory following APHA protocols, using pre-cleaned, high-density polyethylene containers to avoid contamination. They were stored at 4°C in a portable cooler to preserve their chemical and biological integrity, with minimal holding time before analysis. A strict chain of custody ensured the proper handling of samples, maintaining their accuracy in representing the groundwater conditions at the time of collection (Tajwar et al., 2023).
For metal analysis, Cu, Cr, Al, Mn, and As were quantified using Atomic Absorption Spectroscopy (AAS) on a Thermo Scientific iCE 3,000 Series instrument. Certified reference materials (CRMs) from Inorganic Ventures, Christiansburg, Virginia (ISO 17,034), were used to ensure accuracy. Standards traceable to the National Institute of Standards and Technology (NIST) (SRM 3114, SRM 3112a, SRM 3101a, SRM 3132, SRM 3103a) were employed to analyze Cu, Cr, Al, Mn, and As, respectively. Calibration curves were prepared using at least five points for each metal, and all correlation coefficients (R2) exceeded 0.995, indicating excellent linearity. Instrument settings were optimized for each element, including lamp current (Cu: 3.75 mA, Cr: 6.0 mA, Al: 10.0 mA, Mn: 5.0 mA, As: 10.0 mA), slit width (Cu: 1.0 nm, Cr: 1.0 nm, Al: 0.5 nm, Mn: 1.0 nm, As: 0.5 nm), and flame type (air-acetylene) (Ahmed et al., 2004).
Limits of detection (LD) were calculated using the formula:
where σ is the standard deviation of replicate blank measurements.
Limits of Quantification (LQ) were determined using the formula:
The limits of detection (LD) for copper (Cu), chromium (Cr), aluminum (Al), manganese (Mn), and arsenic (As) were determined to be between 0.001 and 0.005 mg/L, while the limits of quantification (LQ) ranged from 0.005 to 0.01 mg/L. Calibration curves for Cu, Cr, and Al spanned the range of 0.01–1 mg/L, for Mn the range was 0.005–0.5 mg/L, and for As, the range was 0.001–0.1 mg/L.
Boron was analyzed using a UV-VIS Spectrophotometer (HACH DR3900) with the Carmine Method 8,015, where boron forms a red complex with curcumin, measured by absorbance at 555 nm. The samples were acidified with sulfuric acid to stabilize boron, and calibration curves were generated using certified standards, with correlation coefficients (R2) above 0.999. The detection limit for boron was 0.01 mg/L, with a quantification range of 0.01–5 mg/L. All samples were analyzed in duplicate, and accuracy was ensured using analytical blanks and standard references. Additional testing at the University of Dhaka and the Bangladesh Council of Scientific and Industrial Research (BCSIR) yielded a high correlation coefficient of 0.975 between laboratories, demonstrating strong consistency and reliability in the analytical methods used.
Precautions were taken throughout the analysis to prevent contamination, including the use of clean latex gloves and talc-free lab coats. Functional standards were assessed after every ten samples. Calibration curves were optimized for signal intensity and sensitivity during the chemical analysis of each sample.
2.3 Pollution evaluation indices
The Metal Evaluation Index (MEI), Nemerow Pollution Index (NI), and Degree of Contamination (Cd) were selected for their ability to comprehensively assess groundwater contamination. MEI evaluates overall water quality, NI accounts for the severity of both average and maximum pollutant levels, and Cd quantifies the impact of elements exceeding safe limits. These indices provide a clear understanding of contamination and its health risks (Edet and Offiong, 2002). These metrics—MEI, NI, and Cd—are significant in assessing groundwater quality as they provide a detailed evaluation of contamination levels and associated health risks. MEI quantifies overall metal contamination, NI identifies the most critical pollutants by considering both mean and maximum concentrations, and Cd assesses the cumulative impact of elements exceeding permissible limits. This approach offers a comprehensive risk assessment that is crucial for formulating mitigation strategies and protecting public health (Wu et al., 2010; Ayejoto et al., 2023).
2.3.1 Metal Evaluation Index
The overall heavy metal quality of water is assessed by the Metal Evaluation Index (MEI). The following (Equation 1) was used to measure MEI (Edet and Offiong, 2002):
The observed value for parameter i is denoted as Hc, while the maximum permissible concentration (MAC) of parameter i is represented by Hmac. According to WHO (World Health Organization, 2021) acceptable thresholds for the amounts of As, Cu, Mn, Al, Cr, and B are 0.01, 2, 0.08, 0.9, 0.05, and 2.4 mg/L, respectively. According to (Edet and Offiong, 2002; Bodrud-Doza et al., 2019a) the metal contamination level of groundwater was assessed by categorizing the index into three distinct groups: low MEI ≥10, medium MEI (10–20), and high MEI > 20.
2.3.2 Nemerow Pollution Index
Groundwater metal contamination levels were assessed using the Nemerow Pollution Index (NI). This index integrates both the mean and the maximum readings from the single-factor pollution indices, giving precedence to factors that are significantly polluting (Equation 2).
Here, ‘n’ signifies the count of indices, ‘Ci’ represents the recorded concentration of each heavy metal ‘i’, and ‘Si’ denotes its corresponding standard value. According to the NI, groundwater’s heavy metal pollution is classified into six distinct categories: non-polluted (<0.5), safe (0.5–0.7), cautionary (0.7–1.0), minimally polluted (1.0–2.0), moderately polluted (2.0–3), and heavily polluted (>3.0). (Li et al., 2001).
2.3.3 Contamination index ( )
The contamination index has been employed in prior research to evaluate levels of metal contamination (Mustafa, 2008). It represents the aggregate of contamination factors for various parameters that surpass their allowable limits, as described in Equation 3.
where
2.4 Human health risk assessment
Quantitative assessment is conducted to evaluate the health hazards linked to metals or metalloids, which are categorized as either carcinogenic or non-carcinogenic health risks (U.S. Environmental Protection Agency U.S. EPA, 2009). This study assessed the non-carcinogenic health risks of chronic daily intake (CDI), hazard quotient (HQ), and hazard index (HI) using the standard approach recommended by (Epa, 2011).
2.4.1 The chronic daily intake (CDI)
The (Equation 4) considered for the oral exposure and the determination of the chronic daily intake (CDI) of components through this route was approximated as follows.
Where,
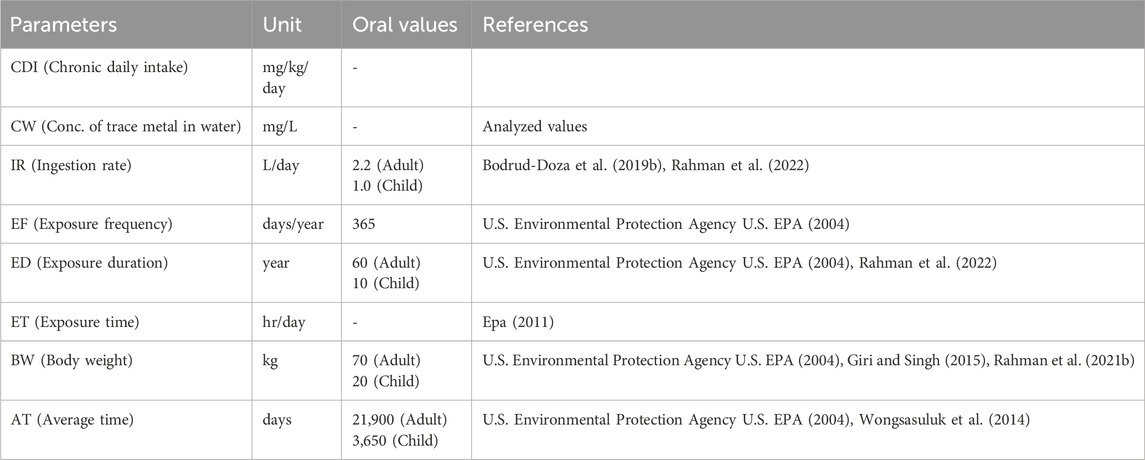
Table 1. Parameters for determining metal exposure by oral consumption and the dermal exposure pathways.
2.4.2 The hazard quotient (HQ)
The United States Environmental Protection Agency (Epa, 2011) assessed non-carcinogenic health risks associated with exposure to concerning metals by comparing the calculated quantity of pollutants from various exposure pathways (oral and dermal) against a predefined reference dose (RfD) to ascertain the hazard quotient (HQ). An HQ value below 1 signifies an absence of non-carcinogenic health risks, whereas an HQ value exceeding 1 denotes a health risk deemed unacceptable (U.S. Environmental Protection Agency U.S. EPA, 2001). HQ was calculated by Equation 5:
The calculation of HQ involves the hazard quotient (HQ) and the reference dose (RfD, in mg/kg/day). Specifically, the RfD values for oral toxicity of Cu, Fe, Mn, and Zn are set at 0.04, 0.3, 0.02, and 0.3 respectively (Wu et al., 2010; Bodrud-Doza et al., 2019b).
2.4.3 Hazard index (HI)
The Hazard Quotients (HQs) for each metal at a specific site are aggregated to form aHazard Index (HI) (Equation 6), which is used to assess the cumulative potential for non-carcinogenic effects resulting from exposure to multiple metals (Epa, 2011)
According to the USEPA (2011), health risks associated with potentially toxic elements are categorized based on the HI value: an HI greater than 1 indicates a condition of risk or unsafety, while an HI less than 1 suggests no detrimental impact on health safety.
2.5 Data analysis
Metal concentration data were initially assessed according to World Health Organization standards (World Health Organization, 2021). A comprehensive statistical evaluation of metal concentrations, encompassing minimum, maximum, average, and standard deviation measures, was performed utilizing Microsoft Excel 2019. Nemerow pollution index and degree of contamination were presented in graduated symbols using ArcGIS software 10.2.
3 Results and discussion
3.1 Metal concentrations in the groundwater
The findings of the research conducted on six potentially toxic elements (PTEs), namely arsenic (As), copper (Cu), manganese (Mn), boron (B), chromium (Cr), and aluminum (Al), are displayed in Table 2. The purpose of this analysis was to evaluate the levels of contamination in the groundwater area under investigation. Arsenic (As) and chromium (Cr) were below the detection limit in all the analyzed samples. Copper (Cu) was detected in all the samples except sample numbers S-02 and S-05 and other samples ranged between 0.02 and 0.2 mg/L with a mean value of 0.075 mg/L. No samples exceeded the WHO (2021) drinking water limits. Manganese (Mn) was found in all 15 samples, ranging from 0.001 to 1.39 mg/L, with a mean concentration of 0.48 mg/L. Among these samples, 10 samples exceeded the WHO (2021) drinking water limit of 0.08 mg/L. Aluminum (Al) concentrations ranged from 0.001 to 0.86 mg/L, with a mean value of 0.087 mg/L. No samples exceeded the WHO (2021) drinking water limits. Boron (B) was detected in all samples, ranging from 0.5 to 2.7 mg/L, with a mean concentration of 1.14 mg/L. Among these samples, only one sample (S-05) exceeded the WHO (2021) drinking water limits. In terms of mean concentrations, the analyzed metals followed a descending order: boron (B) > manganese (Mn) > aluminum (Al) > copper (Cu) > arsenic (As), chromium (Cr).
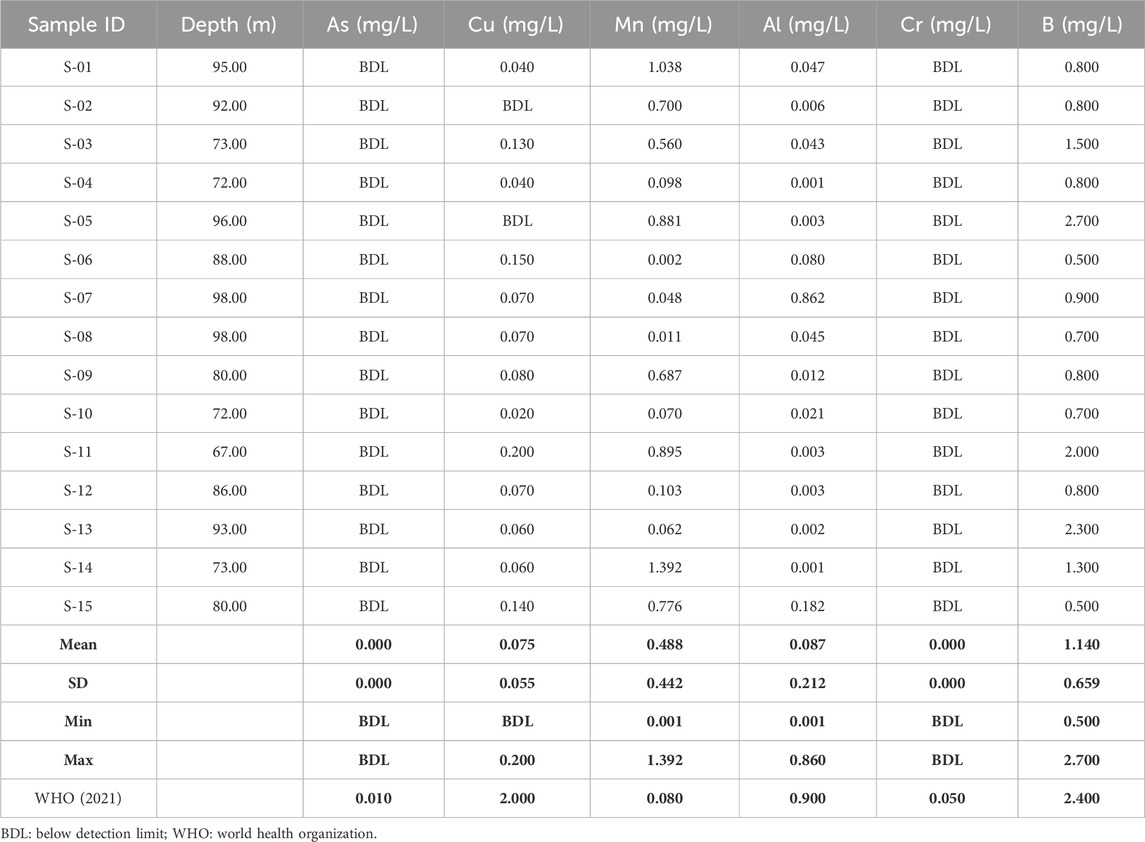
Table 2. An overview of the analyzed parameters and a comparison of drinking water standards to the studied parameters.
3.2 Pollution indices
A total of three pollution indices (Metal evaluation index, Nemerow pollution index, and Degree of contamination) were used to detect the contamination level of the analyzed groundwater. All three indexes showed more or less similar results (Figure 2). The Metal evaluation index measures the groundwater quality emphasizing metal quantity in the samples. MEI was calculated for As, Cu, Mn, Cr, B, and Al where the minimum and maximum MEI values were 0.39 and 17.97 with a mean MEI value of 6.71. MEI values of the analyzed samples were categorized according to (Edet and Offiong, 2002) classification. Among 15 samples, 10 samples fall in the low contamination category (<10) and 05 samples (S-1, 5, 11, 14, 15) in the medium contamination (10–20) category. No samples were found in the high contamination class (>20). The Nemerow Pollution Index is applied to measure the level of pollution by various heavy metals in groundwater within the sampled regions. The NI values for the groundwater samples varied, showing a range from 0.14 to 12.33, and an average value calculated at 4.35. Based on the NI categorization, eight out of fifteen groundwater samples (specifically S-1, 2, 3, 5, 9, 11, 14, 15) were classified under the severe pollution category. Furthermore, the Degree of Contamination metric was employed to assess the magnitude of metal pollution across the sampling locations. The minimum value of Cd was −0.60 and the maximum Cd value was 16.97. The mean value of all sampling points was 5.71. Like NI values, Cd values in this study revealed eight samples out of 15 samples (S-1, 2, 3, 5, 9, 11, 14, 15) were unsuitable for drinking purposes. Two indexes (NI and Cd) revealed that around 50% of the samples were severely contaminated but one index MEI showed less contamination in the groundwater by naturally occurring toxic elements.
3.3 Health risk assessment (HRA)
This research aimed to perform health risk assessments on both adults and children to assess the potential non-carcinogenic health hazards associated with the arsenic (As), copper (Cu), manganese (Mn), chromium (Cr), boron (B), and aluminum (Al) in groundwater, with a specific focus on oral consumption routes.
The hazard index (HI) values obtained from the analysis of the samples ranged from 0.20 to 2.47 for adults and 0.32 to 3.93 for children. For both adults and children, eight out of fifteen samples (S-1, 2, 3, 5, 9, 11, 14, 15) exhibited potential non-carcinogenic health risks, as HI values exceeded the safety limit of 01 (Figure 3). The samples which were found contaminant in NI and Cd index presented a noncarcinogenic risk for both adults and children. In all samples, HI values of children surplus the HI values of adults which indicates that the children are more vulnerable than adults through oral exposure to drinking water.
The reason for higher HI values in eight samples was mainly due to the concentration of manganese (Mn). This is the only element for which seven samples presented hazard quotient (HQ) values over 01. This indicates that Mn alone is a risky element in the groundwater of the study area. Other elements (As, Cu, Al, Cr, B) were not found risky where arsenic and chromium were below the detection limit for all the samples.
Previous research conducted in different parts of Bangladesh, including areas such as Khulna, Chittagong, and Rajshahi, has reported similar issues with elevated levels of toxic elements, particularly manganese and arsenic. Studies such as those by (Bodrud-Doza et al., 2019b; Rahman et al., 2020) in Dhaka have identified manganese and arsenic as key contaminants in groundwater, posing substantial health risks to local populations. The elevated manganese levels found in this study, particularly in 10 out of 15 samples, are consistent with previous findings, where manganese concentrations often exceeded the World Health Organization’s (WHO) guidelines in urban and industrial regions.
Moreover, in terms of pollution indices such as the Nemerow Pollution Index (NI) and the Degree of Contamination (Cd), previous studies in coastal and urban areas of Bangladesh have also demonstrated high contamination levels, particularly in groundwater systems heavily influenced by industrial runoff and agricultural practices. For instance (Ahmed et al., 2004), found that the NI and Cd values in their study areas similarly indicated severe contamination in more than 50% of the samples, aligning with the results of this study where eight samples were classified under the severe pollution category.
The higher hazard index (HI) values observed in children compared to adults also reflect findings from earlier health risk assessments, such as those by (Bouchard et al., 2011), which showed that children are more susceptible to neurotoxic elements like manganese due to their developing nervous systems. This comparison reinforces the critical public health implications of manganese contamination in Dhaka and other similar urban environments, emphasizing the need for immediate intervention to mitigate health risks, particularly for vulnerable populations such as children.
Manganese exposure poses significant health risks, particularly due to its neurotoxic effects. Chronic exposure to elevated levels of manganese can lead to neurological issues, including cognitive impairments, motor dysfunction, and Parkinson’s disease (Lucchini et al., 2012). The susceptibility to manganese toxicity is higher in children than in adults due to their developing nervous systems, which makes them more vulnerable to neurodevelopmental issues (Bouchard et al., 2011). In this study, the hazard index (HI) values for children exceeded those for adults, indicating that children are at greater risk through oral exposure to contaminated drinking water. Other elements such as aluminum and boron, while less acutely toxic than manganese, have been associated with potential long-term health effects, including neurodegenerative diseases and developmental delays in children when consumed in high concentrations (Flora et al., 2008).
4 Conclusion
This comprehensive study on groundwater quality in Dhaka City, Bangladesh, highlights a pressing environmental and public health issue stemming from elevated levels of certain metals. Specifically, our findings indicate that manganese concentrations exceeded World Health Organization (WHO) drinking water guidelines in 10 out of the 15 sampled locations, posing a clear risk to public health. Notably, these elevated levels of manganese are associated with potential non-carcinogenic risks, which are of particular concern for the pediatric population of Dhaka City. Children are shown to be disproportionately affected, with hazard index (HI) values reaching as high as 3.93, significantly higher than those observed in adults. This disparity underscores the heightened vulnerability of children to manganese exposure, which could lead to adverse health outcomes if not addressed promptly. Moreover, the analytical use of pollution indices such as the Nemerow Pollution Index (NI) and the Degree of Contamination (Cd) further illustrates the severity of the situation. Eight of the fifteen groundwater samples were classified as severely polluted based on NI values, which ranged from 0.14 to 12.33 with a mean of 4.35, categorizing the mean groundwater condition as being under severe pollution. Similarly, the Degree of Contamination (Cd) values, which varied significantly across samples, highlighted eight instances where the groundwater was considered unfit for drinking purposes. Local authorities such as the Bangladesh Water Development Board (BWDB) have initiated several measures to monitor groundwater quality. These efforts include hydrology projects aimed at tracking contamination levels, as was done for this study.
The results highlight the urgent necessity for proactive strategies to reduce the risks linked to groundwater contamination. Prompt measures, including adopting sophisticated treatment technologies such as adsorption, reverse osmosis, or electrocoagulation, could markedly diminish the concentrations of arsenic, manganese, and other hazardous elements. Additionally, the contamination should be reduced at its source by following industrial waste management norms, minimizing agricultural runoff, and improving natural filtration systems. Community-based initiatives, such as awareness campaigns regarding safe water practices and alternative water sources, could further mitigate exposure risks.
Policy development must be the primary means of addressing these challenges. Governments and regulatory bodies must implement more efficient groundwater quality standards and ensure adherence through consistent monitoring and penalties for noncompliance. Policies promoting sustainable agricultural practices and eco-friendly industrial processes are equally essential. Incorporating groundwater management into comprehensive national water policies can facilitate coordinated initiatives across various sectors. Subsequent research must emphasize the provision of evidence to guide these policies, concentrating on contamination pathways, socio-economic ramifications, and the cost-efficiency of mitigation strategies. Cooperative initiatives among policymakers, scientists, and local stakeholders are crucial for formulating region-specific, implementable solutions that protect public health and the environment. Future research could focus on long-term studies assessing the health impacts of chronic manganese exposure, as well as investigations into other potentially toxic elements, such as lead and cadmium, that were not covered in this study. The implementation of comprehensive water safety plans and regular monitoring programs across Dhaka could significantly mitigate the long-term risks posed by groundwater contamination.
Data availability statement
The original contributions presented in the study are included in the article/supplementary material, further inquiries can be directed to the corresponding author.
Author contributions
MT: Formal Analysis, Writing–original draft, Writing–review and editing, Conceptualization. MR: Formal Analysis, Writing–original draft, Writing–review and editing. SS: Formal Analysis, Writing–original draft, Writing–review and editing. NS: Data curation, Writing–original draft, Writing–review and editing. MG: Supervision, Writing–original draft, Writing–review and editing. MH: Formal Analysis, Methodology, Writing–original draft, Writing–review and editing. AS-A: Formal Analysis, Writing–review and editing. AZ: Supervision, Writing–review and editing.
Funding
The author(s) declare that financial support was received for the research, authorship, and/or publication of this article. The publication charge of this article will be covered by the University of Dhaka, Bangladesh.
Acknowledgments
The authors extend their gratitude to the Bangladesh Water Development Board (BWDB) for facilitating the laboratory analyses with support from the project of Bangladesh Weather and Climate Services Regional Project: Component-B.
Conflict of interest
The authors declare that the research was conducted in the absence of any commercial or financial relationships that could be construed as a potential conflict of interest.
The author(s) declared that they were an editorial board member of Frontiers, at the time of submission. This had no impact on the peer review process and the final decision.
Generative AI statement
The author(s) declare that no Generative AI was used in the creation of this manuscript.
Publisher’s note
All claims expressed in this article are solely those of the authors and do not necessarily represent those of their affiliated organizations, or those of the publisher, the editors and the reviewers. Any product that may be evaluated in this article, or claim that may be made by its manufacturer, is not guaranteed or endorsed by the publisher.
References
Adimalla, N., and Li, P. (2019). Occurrence, health risks, and geochemical mechanisms of fluoride and nitrate in groundwater of the rock-dominant semi-arid region, Telangana State, India. Hum. Ecol. Risk Assess. Int. J. 25, 81–103. doi:10.1080/10807039.2018.1480353
Ahmed, K. M., Bhattacharya, P., Hasan, M. A., Akhter, S. H., Alam, S. M. M., Bhuyian, M. A. H., et al. (2004). Arsenic enrichment in groundwater of the alluvial aquifers in Bangladesh: an overview. Appl. Geochem. 19, 181–200. doi:10.1016/j.apgeochem.2003.09.006
Ahmed, K. M., Sultana, S., Hasan, M. A., Bhattacharya, P., Hasan, M. K., Burgess, W. G., et al. (2010). “Groundwater quality of upper and lower Dupi Tila aquifers in the megacity Dhaka, Bangladesh,” in Proc 7th international groundwater quality conference held in Zurich, Switzerland, 13–18.
Akther, H., Ahmed, M. S., and Rasheed, K. B. S. (2010). Spatial and temporal analysis of groundwater level fluctuation in Dhaka City, Bangladesh. Asian J. Earth Sci. 3, 222–230. doi:10.3923/ajes.2010.222.230
Alexakis, D. (2011). Assessment of water quality in the Messolonghi–Etoliko and Neochorio region (West Greece) using hydrochemical and statistical analysis methods. Environ. Monit. Assess. 182, 397–413. doi:10.1007/s10661-011-1884-2
Ali, H., Khan, E., and Ilahi, I. (2019). Environmental chemistry and ecotoxicology of hazardous heavy metals: environmental persistence, toxicity, and bioaccumulation. J. Chem. 2019, 1–14. doi:10.1155/2019/6730305
Appelo, C. A. J., and Postma, D. (2004). in Geochemistry, groundwater and pollution. Editors C. A. J. Appelo, and D. Postma (London: CRC Press). Available at: https://www.taylorfrancis.com/books/mono/10.1201/9781439833544/geochemistrygroundwater-pollu-on-dieke-postma-appelo. doi:10.1201/9781439833544
Atikul Islam, M., Zahid, A., Rahman, Md. M., Rahman, Md. S., Islam, M. J., Akter, Y., et al. (2017). Investigation of groundwater quality and its suitability for drinking and agricultural use in the south central part of the coastal region in Bangladesh. Expo. Health 9, 27–41. doi:10.1007/s12403-016-0220-z
Ayejoto, D. A., Agbasi, J. C., Nwazelibe, V. E., Egbueri, J. C., and Alao, J. O. (2023). Understanding the connections between climate change, air pollution, and human health in Africa: insights from a literature review. J. Environ. Sci. Health Part C 41, 77–120. doi:10.1080/26896583.2023.2267332
Barlow, P. M., and Reichard, E. G. (2010). Saltwater intrusion in coastal regions of North America. Hydrogeol. J. 18, 247–260. doi:10.1007/s10040-009-0514-3
Bhattacharjee, S., Saha, B., Saha, B., Uddin, Md. S., Panna, C. H., Bhattacharya, P., et al. (2019). Groundwater governance in Bangladesh: established practices and recent trends. Groundw. Sustain Dev. 8, 69–81. doi:10.1016/j.gsd.2018.02.006
Bodrud-Doza, Md., Bhuiyan, M. A. H., Islam, S. M. D.-U., Quraishi, S. B., Muhib, Md. I., Rakib, M. A., et al. (2019a). Delineation of trace metals contamination in groundwater using geostatistical techniques: a study on Dhaka City of Bangladesh. Groundw. Sustain Dev. 9, 100212. doi:10.1016/j.gsd.2019.03.006
Bodrud-Doza, Md., Bhuiyan, M. A. H., Islam, S. M. D.-U., Rahman, M. S., Haque, Md. M., Fatema, K. J., et al. (2019b). Hydrogeochemical investigation of groundwater in Dhaka City of Bangladesh using GIS and multivariate statistical techniques. Groundw. Sustain Dev. 8, 226–244. doi:10.1016/j.gsd.2018.11.008
Bodrud-Doza, M., Islam, S. M. D.-U., Rume, T., Quraishi, S. B., Rahman, M. S., and Bhuiyan, M. A. H. (2020). Groundwater quality and human health risk assessment for safe and sustainable water supply of Dhaka City dwellers in Bangladesh. Groundw. Sustain Dev. 10, 100374. doi:10.1016/j.gsd.2020.100374
Bouchard, M. F., Sauvé, S., Barbeau, B., Legrand, M., Brodeur, M.-È., Bouffard, T., et al. (2011). Intellectual impairment in school-age children exposed to manganese from drinking water. Environ. Health Perspect. 119, 138–143. doi:10.1289/ehp.1002321
Carol, E., Mas-Pla, J., and Kruse, E. (2013). Interaction between continental and estuarine waters in the wetlands of the northern coastal plain of Samborombón Bay, Argentina. Appl. Geochem. 34, 152–163. doi:10.1016/j.apgeochem.2013.03.006
Edet, A. E., and Offiong, O. E. (2002). Evaluation of water quality pollution indices for heavy metal contamination monitoring. A study case from Akpabuyo-Odukpani area, Lower Cross River Basin (southeastern Nigeria). GeoJournal 57, 295–304. doi:10.1023/B:GEJO.0000007250.92458.de
Epa, U. S. (2011). Exposure factors handbook, 20460. Washington, DC: Office of research and Development, 2–6.
Flora, S. J. S., Mittal, M., and Mehta, A. (2008). Heavy metal induced oxidative stress and its possible reversal by chelation therapy. Indian J. Med. Res. 128, 501–523.
Giri, S., and Singh, A. K. (2015). Human health risk assessment via drinking water pathway due to metal contamination in the groundwater of Subarnarekha River Basin, India. Environ. Monit. Assess. 187, 63. doi:10.1007/s10661-015-4265-4
Güler, C., Kurt, M. A., Alpaslan, M., and Akbulut, C. (2012). Assessment of the impact of anthropogenic activities on the groundwater hydrology and chemistry in Tarsus coastal plain (Mersin, SE Turkey) using fuzzy clustering, multivariate statistics and GIS techniques. J. Hydrol. (Amst) 414, 435–451. doi:10.1016/j.jhydrol.2011.11.021
Hasan, M., Islam, M. A., Alam, M. J., Rahman, M., and Hasan, M. A. (2022). Hydrogeochemical characterization and quality assessment of groundwater resource in Savar — an industrialized zone of Bangladesh. Environ. Monit. Assess. 194, 549. doi:10.1007/s10661-022-10137-1
Hasan, M., Islam, Md. A., Aziz Hasan, M., Alam, Md. J., and Peas, M. H. (2019). Groundwater vulnerability assessment in Savar upazila of Dhaka district, Bangladesh — a GIS-based DRASTIC modeling. Groundw. Sustain Dev. 9, 100220. doi:10.1016/j.gsd.2019.100220
Hoque, M. A., Hoque, M. M., and Ahmed, K. M. (2007). Declining groundwater level and aquifer dewatering in Dhaka metropolitan area, Bangladesh: causes and quantification. Hydrogeol. J. 15, 1523–1534. doi:10.1007/s10040-007-0226-5
Hoque, M. A., McArthur, J. M., Sikdar, P. K., Ball, J. D., and Molla, T. N. (2014). Tracing recharge to aquifers beneath an Asian megacity with Cl/Br and stable isotopes: the example of Dhaka, Bangladesh. Hydrogeol. J. 22, 1549–1560. doi:10.1007/s10040-014-1155-8
Huang, G., Sun, J., Zhang, Y., Chen, Z., and Liu, F. (2013). Impact of anthropogenic and natural processes on the evolution of groundwater chemistry in a rapidly urbanized coastal area, South China. Sci. Total Environ. 463–464, 209–221. doi:10.1016/j.scitotenv.2013.05.078
Hughes, M. F. (2002). Arsenic toxicity and potential mechanisms of action. Toxicol. Lett. 133, 1–16. doi:10.1016/s0378-4274(02)00084-x
Ingerman, L., Jones, D. G., Keith, S., and Rosemond, Z. A. (2008). Toxicological profile for aluminum.
Islam, Md. Z., and Mostafa, M. G. (2024). Iron, manganese, and lead contamination in groundwater of Bangladesh: a review. Water Pract. Technol. 19, 745–760. doi:10.2166/wpt.2024.030
Khan, M. K. A., Alam, M., Islam, M. S., Hassan, M. Q., and Al-Mansur, M. A. (2011). Environmental pollution around Dhaka EPZ and its impact on surface and groundwater. Bangladesh J. Sci. Industrial Res. 46, 153–162. doi:10.3329/bjsir.v46i2.8181
Kumar, P. J. S., Jegathambal, P., and James, E. J. (2011). Multivariate and geostatistical analysis of groundwater quality in Palar river basin. Int. J. Geol. 4, 108–119.
Kumar, S. S., Malyan, S. K., Kumar, A., and Bishnoi, N. R. (2016). Optimization of Fenton’s oxidation by Box-Behnken design of response surface methodology for landfill leachate. J. Mater. Environ. Sci. 7, 4456–4466.
Li, J., Feng, M., and Yu, L. (2001). Assessment on the situation of water quality in Liaodong Bay shallow waters. Mar. Environ. science/Haiyang Huanjing Kexue. Dalian 20, 42–45.
Lina, A. J., and Shaharuddin, M. S. (2017). Health risk associated with aluminium exposure in groundwater: a cross-sectional study in an Orang Asli Village in Jenderam Hilir, Selangor, Malaysia. Malays. J. Public Health Med., 58–62.
Lu, S.-Y., Zhang, H.-M., Sojinu, S. O., Liu, G.-H., Zhang, J.-Q., and Ni, H.-G. (2015). Trace elements contamination and human health risk assessment in drinking water from Shenzhen, China. Environ. Monit. Assess. 187, 4220. doi:10.1007/s10661-014-4220-9
Lucchini, R. G., Dorman, D. C., Elder, A., and Veronesi, B. (2012). Neurological impacts from inhalation of pollutants and the nose–brain connection. Neurotoxicology 33, 838–841. doi:10.1016/j.neuro.2011.12.001
Luo, P. P., Kang, S. X., Zhou, M. M., Lyu, J. Q., Aisyah, S., Binaya, M., et al. (2019). Water quality trend assessment in Jakarta: a rapidly growing Asian megacity. PLoS One 14, e0219009. doi:10.1371/journal.pone.0219009
Muhammad, S., Shah, M. T., and Khan, S. (2011). Health risk assessment of heavy metals and their source apportionment in drinking water of Kohistan region, northern Pakistan. Microchem. J. 98, 334–343. doi:10.1016/j.microc.2011.03.003
Mustafa, O. M. (2008). Evaluating water quality of waraz mountaneous area, using contamination index, sulaimaniya governorate, northeast Iraq. Iraqi Bull. Geol. Min. 4, 59–66.
National Research CouncilCommission on Life Sciences; Board on Environmental Studies and ToxicologyCommittee on Copper in Drinking Water (2000). Copper in drinking water.
Nagarajan, R., Rajmohan, N., Mahendran, U., and Senthamilkumar, S. (2010). Evaluation of groundwater quality and its suitability for drinking and agricultural use in Thanjavur city, Tamil Nadu, India. Environ. Monit. Assess. 171, 289–308. doi:10.1007/s10661-009-1279-9
Nkpaa, K. W., Amadi, B. A., and Wegwu, M. O. (2018). Hazardous metals levels in groundwater from Gokana, Rivers State, Nigeria: non-cancer and cancer health risk assessment. Hum. Ecol. Risk Assess. Int. J. 24, 214–224. doi:10.1080/10807039.2017.1374166
Patel, P., Raju, N. J., Reddy, B. C. S. R., Suresh, U., Gossel, W., and Wycisk, P. (2016). Geochemical processes and multivariate statistical analysis for the assessment of groundwater quality in the Swarnamukhi River basin, Andhra Pradesh, India. Environ. Earth Sci. 75, 611–624. doi:10.1007/s12665-015-5108-x
Pugazhendhi, A., Ranganathan, K., and Kaliannan, T. (2018). Biosorptive removal of copper(II) by Bacillus cereus isolated from contaminated soil of electroplating industry in India. Water Air Soil Pollut. 229, 76. doi:10.1007/s11270-018-3734-0
Rahman, M., Khan, M. R., Ahmed, K. M., Zahid, A., and Siddique, M. A. M. (2018). An assessment of nitrate concentration in the groundwater of the central coastal region of Bangladesh. J. NOAMI 35, 107–116.
Rahman, M., Khan, Md. S. I., Hossain, M. S., Hossain, Md. I. S., Hasan, M., Hamli, H., et al. (2022). Groundwater contamination and health risk evaluation of naturally occurring potential toxic metals of hatiya island, Bangladesh. J. Ecol. Eng. 23, 223–236. doi:10.12911/22998993/148192
Rahman, M., Tushar, M. A. N., Zahid, A., Ahmed, K. M. U., Siddique, M. A. M., and Mustafa, M. G. (2021a). Spatiotemporal distribution of boron in the groundwater and human health risk assessment from the coastal region of Bangladesh. Environ. Sci. Pollut. Res. 28, 21964–21977. doi:10.1007/s11356-020-11682-3
Rahman, M., Tushar, M. A. N., Zahid, A., Mustafa, M. G., Siddique, M. A. M., and Ahmed, K. M. (2021b). Spatial distribution of manganese in groundwater and associated human health risk in the southern part of the Bengal Basin. Environ. Sci. Pollut. Res. 28, 41061–41070. doi:10.1007/s11356-021-13577-3
Rahman, Md. M., Bodrud-Doza, Md., Siddiqua, M. T., Zahid, A., and Islam, A. R.Md. T. (2020). Spatiotemporal distribution of fluoride in drinking water and associated probabilistic human health risk appraisal in the coastal region, Bangladesh. Sci. Total Environ. 724, 138316. doi:10.1016/j.scitotenv.2020.138316
Rango, T., Bianchini, G., Beccaluva, L., Ayenew, T., and Colombani, N. (2009). Hydrogeochemical study in the Main Ethiopian Rift: new insights to the source and enrichment mechanism of fluoride. Environ. Geol. 58, 109–118. doi:10.1007/s00254-008-1498-3
Saha, S. K., Gazi, M. Y., Tajwar, M., and Kumar, S. (2021). Soil contamination assessment by trace elements in barapukuria coal mine region, Bangladesh. Environ. and Earth Sci. Res. J. 8, 1–10. doi:10.18280/eesrj.080101
Schot, P. P., and Van der Wal, J. (1992). Human impact on regional groundwater composition through intervention in natural flow patterns and changes in land use. J. Hydrol. (Amst) 134, 297–313. doi:10.1016/0022-1694(92)90040-3
Singh, K., Singh, R., Malyan, S. K., Rawat, M., Kumar, P., Kumar, S., et al. (2018). Health risk assessment of drinking water in Bathinda District, Punjab, India. J. Indian Water Resour. Soc. 38, 34–41.
Tajwar, M., and Uddin, A. (2021). “Heavy mineral distribution in arsenic-contaminated coastal sediments,” in The southeastern part of Bangladesh. doi:10.1130/abs/2021AM-370919
Tajwar, M., and Uddin, A. (2022). Hydrochemical characteristics and quality assessment of groundwater in hatiya island, southern coastal region of bangladesh. GSA Connects 2022 Meet. Denve, 382667. doi:10.1130/abs/2022AM-382667
Tajwar, M., Uddin, A., Lee, M.-K., Nelson, J., Zahid, A., and Sakib, N. (2023). Hydrochemical characterization and quality assessment of groundwater in hatiya island, southeastern coastal region of Bangladesh. Water (Basel) 15, 905. doi:10.3390/w15050905
Tirkey, P., Bhattacharya, T., Chakraborty, S., and Baraik, S. (2017). Assessment of groundwater quality and associated health risks: a case study of Ranchi city, Jharkhand, India. Groundw. Sustain Dev. 5, 85–100. doi:10.1016/j.gsd.2017.05.002
Triki, I., Trabelsi, N., Zairi, M., and Dhia, H. B. (2014). Multivariate statistical and geostatistical techniques for assessing groundwater salinization in Sfax, a coastal region of eastern Tunisia. Desalination Water Treat. 52, 1980–1989. doi:10.1080/19443994.2013.803937
U.S. Environmental Protection Agency U.S. EPA (2001). Baseline human health risk assessment Vasquez Boulevard and I-70 superfund site.
U.S. Environmental Protection Agency U.S. EPA (2004). Risk assessment guidance for superfund volume I: human health evaluation manual (Part E).
Wongsasuluk, P., Chotpantarat, S., Siriwong, W., and Robson, M. (2014). Heavy metal contamination and human health risk assessment in drinking water from shallow groundwater wells in an agricultural area in Ubon Ratchathani province, Thailand. Environ. Geochem Health 36, 169–182. doi:10.1007/s10653-013-9537-8
World Health Organization (2021). Manganese in drinking-water: background document for development of WHO Guidelines for drinking-water quality.
Keywords: groundwater, toxic elements, manganese, health hazards, pollution evaluation indices
Citation: Tajwar M, Rahman M, Shreya SS, Sakib N, Gazi MY, Hasan M, Samm-A A and Zahid A (2025) Is the groundwater of Dhaka city, Bangladesh contaminated with naturally occurring potential toxic elements?. Front. Environ. Sci. 12:1514154. doi: 10.3389/fenvs.2024.1514154
Received: 20 October 2024; Accepted: 13 December 2024;
Published: 06 January 2025.
Edited by:
Yalçın Tepe, Giresun University, TürkiyeReviewed by:
Venkatramanan Senapathi, National College, Tiruchirappalli, IndiaSyed Hafizur Rahman, Jahangirnagar University, Bangladesh
Copyright © 2025 Tajwar, Rahman, Shreya, Sakib, Gazi, Hasan, Samm-A and Zahid. This is an open-access article distributed under the terms of the Creative Commons Attribution License (CC BY). The use, distribution or reproduction in other forums is permitted, provided the original author(s) and the copyright owner(s) are credited and that the original publication in this journal is cited, in accordance with accepted academic practice. No use, distribution or reproduction is permitted which does not comply with these terms.
*Correspondence: Shamiha Shafinaz Shreya, c2hyZXlhQGR1LmFjLmJk