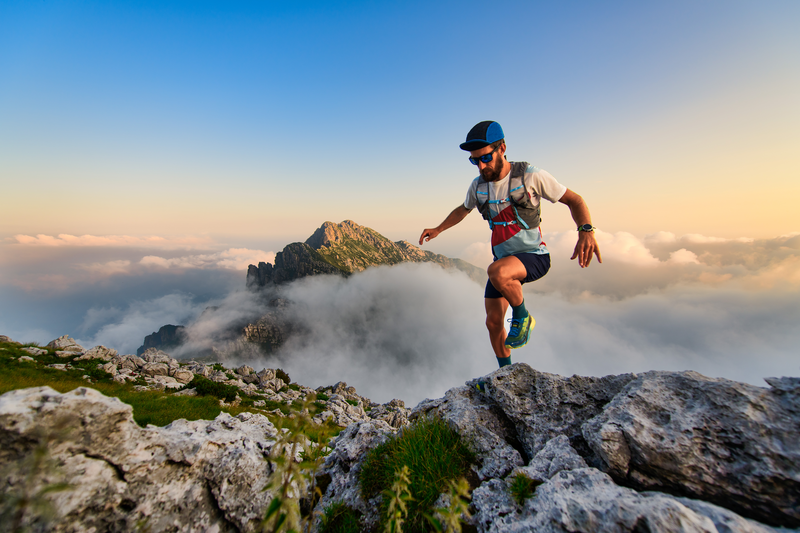
94% of researchers rate our articles as excellent or good
Learn more about the work of our research integrity team to safeguard the quality of each article we publish.
Find out more
ORIGINAL RESEARCH article
Front. Environ. Sci. , 03 January 2025
Sec. Soil Processes
Volume 12 - 2024 | https://doi.org/10.3389/fenvs.2024.1511449
This article is part of the Research Topic Soil and Water Loss and Environmental Effects View all 8 articles
Understanding the characteristics of the soil water content and preferential flow is critical for a thorough comprehension of soil nutrient loss in Karst slopes/ecosystems. We monitored the soil water content and soil temperature at 0–20, 20–40, and 40–60 cm depths on a typical Karst dolomite slope at a high frequency to determine the water distribution characteristics and confirm the occurrence of preferential flow from 2018 to 2021. The soil properties and nutrients in different soil layers during the rainy and dry seasons were determined along the slope (from upper to lower slope positions, with a total of 9 sampling sites). The results revealed that the saturated hydraulic conductivity of the soil at the upper slope position was significantly (p < 0.05) greater than that at the middle and lower slope positions. The soil water content at the down slope position was greater than that at the middle and upper slope positions, further more, coupling monitoring of the soil water content and temperature revealed obvious preferential flow in the Karst dolomite slope. In addition to the spatial variability in the water content, the soil nutrients exhibited regular spatial variations. The dissolved organic carbon (DOC), dissolved organic nitrogen (DON), total nitrogen (TN), total phosphorus (TP) and total potassium (TK) contents were the lowest at the upper slope position and the highest at the down slope position, whereas the difference in nutrients between the rainy and dry seasons was the greatest at the upper slope position. Our results demonstrated that the patterns of the soil water content and surface nutrient loss are consistent along the Karst dolomite slope and are related to the occurrence of preferential flow. Furthermore, the results suggested that, compared with those in previous studies, which focused only on soil properties in the Karst regions of Southwest China, the variation in the soil water content and occurrence of preferential flow may be more important than previously assumed.
Karst landforms constitute one of the main types of landforms worldwide, accounting for approximately 12% of the Earth’s land area, and occur primarily in the Mediterranean Sea area, Eastern Europe, the Middle East, Southeast Asia, Southeast America and the Caribbean region (Peng and Wang, 2012; Lu et al., 2014; Hartmann et al., 2015). Owing to notable development and severe soil erosion, the soil in Karst regions is thin and scattered (Herman et al., 2012; Li et al., 2019; Liu et al., 2020; Zhang et al., 2021).
Notably, total nitrogen (TN) and total phosphorus (TP) in soil are the major limiting nutrients of ecosystem productivity and play key roles in ecosystem restoration or succession (Zhang et al., 2015; Wen et al., 2016). The ecological problem of soil nutrient degradation in Karst regions has attracted the attention of many researchers (Turrión et al., 2009; Albaladejo et al., 2012). Li et al. (2017) studied the impacts of agricultural abandonment on soil C and N contents and reported that the soil C and N contents increased following agricultural abandonment. Moreover, the significant effects of the lithology and land cover type on soil nutrients have been reported in a few studies (Kooijman et al., 2005; Karchegani et al., 2012; Hoffmann et al., 2014; Rossel et al., 2014). In addition, relevant studies have noted that water is the main driving force of soil erosion, as well as the carrier and driving force of nutrient loss (Peng and Wang, 2012). In the absence of significant differences in the lithology, human factors, vegetation coverage, and other conditions, soil erosion may be the main factor leading to nutrient depletion in Karst regions.
At present, studies on the impact of soil erosion on nutrient migration on Karst slopes have focused mostly on surface water movement. Song et al. (2017) reported that runoff erosion directly affects the process of soil nutrient depletion, especially under heavy rainfall conditions, and that an excessive water input can cause a reduction in the soil nutrient content. Wang et al. (2016) analyzed the impact of runoff on soil nutrients on Karst slopes. Yao et al. (2021) studied the effects of rainfall intensity and underground fracture gaps on nutrient loss in karstic sloping farmland and reported that the effect of underground cavities on the loss of sediment nutrients was greater than that of runoff. Owing to the chemical and physical weathering on Karst slopes, the weathering degree of bedrock is high, macropores are obviously developed, the water holding capacity of the soil layer is low, and the permeability is high. Previous research has shown that the surface runoff coefficient on Karst slopes is less than 5% and that almost all of the rainfall infiltrates ( Chen et al., 2012; Fu et al., 2015). Fissures and sinkholes distributed on Karst slopes are important water and nutrient leakage channels, and surface water and soil easily seep into the ground along holes (cracks), resulting in notable nutrient loss (Jiang et al., 2014). In contrast to surface runoff, subsurface flow is the primary mechanism of soil nutrient loss in Karst areas. Subsurface flow determines the infiltration and redistribution of precipitation in soil and affects the soil water content in different soil layers. Further more, due to the presence of cracks, they affect soil water distribution, facilitate rapid infiltration of water, and may lead to more frequent occurrence of preferential flow during water infiltration during rainfall (Sheng et al., 2009; Hardie et al., 2011). The subsurface flow that bypasses part of the soil matrix through macropores and does not follow Darcy’s law is referred to as preferential flow (Clothier et al., 2008). Soil preferential flow is not an individual phenomenon. Moreover, this process is common at various spatial scales (from pores to watersheds) and time scales (from 10 s to 10 years) and can occur in soils with different textures and structures (Nimmo, 2012; Liu et al., 2005). The existence of preferential flow enhances the infiltration depth and infiltration area, accelerates the downward movement of water, and provides a preferential flow path for water infiltration. Water is the main carrier of soil nutrients, and the content of soil nutrients is affected by water content changes (Korkanc and Dorum, 2019), preferential flow may accelerate the loss of surface soil nutrients during rainfall (Hopp and McDonnell, 2009). In summary, preferential flow significantly affects soil nutrient levels, which can promote or inhibit the ability of soil to filter nutrients, thereby enhancing or harming the functionality of ecosystems (Clothier et al., 2008; Wei et al., 2024). Therefore, it is essential to account for the characteristics of preferential flow in Karst soil and understand the relationship between preferential flow and slope water distribution characteristics in slopes, which is conducive to elucidating the impact of preferential flow on soil nutrient loss.
The geological structure and soil characteristics in Karst areas are unique, leading to differences in the water infiltration process compared with those in other regions, and preferential flow may occur more frequently. Although the importance of preferential flow in the process of soil nutrient loss in Karst areas has been recognized, research on the development status of preferential flow in Karst slope soils is lacking, whereas research on the impact of preferential flow on soil nutrients has also been relatively slow. Notably, methodological and technical difficulties are encountered in studying the developmental characteristics of preferential flow on Karst slopes and its impact on nutrient distribution. Previous studies on preferential flow changes have usually been performed via tracer and staining methods. Different methods present distinct advantages and focuses, and it is currently difficult to unify them. In addition, onsite measurement methods are destructive, with limited experimental samples and a lack of monitoring and analysis of preferential flow under natural rainfall conditions (Gerke et al., 2015; Hencher, 2010; Allaire et al., 2009). Therefore, evaluating the impact of preferential flow in soil on nutrient loss within those soils is difficult. Obviously, studying preferential flow on Karst slopes via appropriate methods is important for distinguishing whether preferential flow in soil on Karst slopes has developed and for determining its impact on the distribution of soil nutrients within those slopes. With improvements in experimental and numerical research methods, preferential flow has been analyzed through synergistic observations of the soil water content and temperature (Li et al., 2014; Gharabaghi et al., 2015; An et al., 2017). In alignment with previous studies, this study aimed to analyze preferential flow on Karst slopes through long–term high–frequency monitoring of the soil water content and temperature at different slope positions.
The Karst region in southwestern China is one of the three major areas with concentrated Karst distributions in the world (Jiang et al., 2014; Hu et al., 2021). Dolomite [CaMg(CO3)2] is a widely distributed carbonate rock in this region with favorable porosity and permeability. Therefore, it is realistic to choose a Karst dolomite slope in this region to analyze the impact of preferential flow on soil nutrient loss. The main objectives of this study were to (1) explore the characteristics of preferential flow on Karst slopes under natural rainfall conditions and its relationship with water distribution on those slopes and (2) analyze the characteristics of nutrient loss on Karst slopes and its potential relationship with preferential flow. The results could provide useful information on the conditions under which the spatial characteristics of nutrient loss on Karst slopes are beneficial for the restoration and management of degraded slopes. This study offers a theoretical basis for the prevention and control of rocky desertification and for ecological governance.
The experimental site is located in the Mulian Comprehensive Experimental Demonstration Zone of Ecosystem Observation of the Chinese Academy of Sciences, Huanjiang County, Guangxi Autonomous Region (24°43′59″–24°44′49″N, 108°18′57″–108°19′58″E). It is a typical Karst landscape unit (Figure A1). This county features a subtropical monsoon climate, with an average annual temperature of 19.9°C. The annual precipitation reaches 1,389.1 mm, with abundant rainfall but an uneven seasonal distribution. The rainy season (April to September) accounts for more than 70% of the annual rainfall, and the greatest amount occurs from June to July. The dry season lasts from October to March of the following year.
The soil in the study area is derived from dolomite residues, the main types of clay minerals are illite (2:1 non expansive clay mineral), montmorillonite (2:1 expansive clay mineral), and kaolinite (1:1non expansive clay mineral), with montmorillonite having a higher content. Except for the mountaintop, the research area is almost continuously covered by shallow soil. The thickness of the soil layer gradually increases downwards along the slope, and the degree of soil development gradually increases. Along the slope direction, clay particles mainly gather at the down slope, while gravel is concentrated on the upper slope. Meanwhile, the soil organic matter content gradually increases from the upper to the down of slope, the soil organic matter content was significantly higher at the down slope (26.96 ± 1.53b) than for the upper slope (22.06 ± 1.84a) and middle-slopes (23.58 ± 1.23a). To eliminate the impact of vegetation on the erosion process, we conducted experiments on slopes where the vegetation and species diversity levels were not significant, and where the main vegetation types included herbs and shrubs. To clarify the physical properties of different soil layers in the vertical profile of the study slope, soil samples were collected in the exact same location from the surface to the deep soil layer in the vertical profile according to the geomorphological characteristics of the dolomite slope. A total of nine sampling points were established along the slope direction in the upper slope (sites 1, 2, and 3), middle slope (sites 4, 5, and 6), and down slope (sites 7, 8, and 9) sections, with two profiles arranged for each sampling point as duplicates. The soil layers on the dolomite slope at the test site were further divided into three layers according to their color and texture (from top to bottom): the A layer, AC layer and C layer. The “A” layer is black surface layer. “AC” is a yellow illuvial horizon and “C” is a white dolomite strongly weathered layer. To clarify the physical properties and nutrient contents in the different soil layers within the vertical profile of the Karst dolomite slope, soil samples were collected from the surface layer to the deep soil layer along the vertical profile according to the abovementioned soil layer division. We collected undisturbed soil samples from these layers with a ring knife to quantify the soil bulk density (BD) and saturated hydraulic conductivity (Ks). Disturbed soil samples were used to determine the soil mechanical composition and soil nutrients, with samples for soil nutrient measurement collected at the same sites in March 2022 and October 2022.
The soil bulk density (BD) and porosity were determined via the ring knife method (Cerda and Doerr, 2010), The soil mechanical composition was analyzed by the pipette method, and the samples were classified in terms of their sand (0.05–2 mm), silt (0.002–0.05 mm) and clay (<0.002 mm) compositions on the basis of the American standard classification system (Duan et al., 2021). The basic conditions of the soil samples are shown in Figure 1.
Figure 1. The soil physical properties of various layers in different sites: (A) Bulk density (BD) and total porosity (TPOR), capillary porosity (CP) and non-capillary porosity (AP); (B) Soil mechanical composition.
The total nitrogen (TN) content was analyzed via the semimicro-Kjeldahl-flow injection method (HGCF-200, China), the total phosphorus (TP) content was determined via NaOH fusion-molybdenum-antimony anticolor development-UV spectrophotometry (UV-2600, China), and the total potassium (TK) content was obtained via the NaOH melting atomic absorption flame photometry method (AA320CRT, China) (Bao, 2000). Dissolved organic carbon and dissolved organic nitrogen (DOC and DON, respectively) levels were analyzed via extraction with 0.5 M K2SO4 (Xiao et al., 2020). The constant-head method was used to measure the soil saturation hydraulic conductivity, Ks (mm/min), which can be calculated as follows:
where Ks (mm/min) is the saturated hydraulic conductivity, Q (mL) is the outflow within time t, L (cm) is the linear distance of the water flow path, A (cm2) is the water flow cross-sectional area, ΔH (cm) is the total head difference between the beginning and end of the seepage path, and T (min) is the outflow time.
To obtain the change in the soil water content on the test Karst slope, three soil water monitoring sites were established at three slope sites (upper, middle, and down slope sites). One soil moisture sensor (Hydra II) was installed horizontally to obtain the soil profiles at depths of 0–20 cm on the upper slope, and three soil moisture sensors were installed horizontally to obtain the soil profiles at depths of 0–20 cm, 20–40 cm, and 40–60 cm at the middle and down slope sites. The sensors were calibrated before installation. Rainfall and air temperature data were obtained from meteorological stations within the Mulian catchment. The soil water contents at all the sites were recorded at 30–min intervals beginning in January 2018.
The statistical analyses were performed via the SPSS 21.0 for Windows statistical software package. Additionally, the tests included the normality test, homogeneity test of variance, t-test (significance: 0.05), and one-way analysis of variance (ANOVA) of the soil properties among the different soil layers and sites. Correlation analysis was performed, and related charts were constructed via Origin 21 software.
As shown in Figure 1, the clay content (particle size<0.002 mm) among the slope positions (sites 1–9) gradually increased from the upper to down slope positions, with the lowest clay content at site 1 and the highest at site 9 for the same soil layer. In addition, the change in the soil BD gradually decreased from the upper to lower slope positions with increasing slope. The lowest BD at site 9 was 1.10 g/cm3, and the maximum BD at site 1 was 1.26 g/cm3. This difference may be due to the higher content of coarse particles at the upper slope position than at the down slope position. The aeration porosity (>0.015 mm) at the upper slope position was obviously greater than those at the middle and down slope positions, and the value ranged from 3.59% to 13.23% from sites 1 to 9. Although the total porosity at the down slope position was high, the aeration porosity was lower than that at the upper slope position. A comparison of the same slope sites in the different soil layers revealed that the sand content (particle size range 0.05–2 mm) and BD significantly increased and that the soil aeration porosity gradually decreased from the A layer to the C layer.
Figure 2 shows that the Ks of the dolomite slope exhibited significant spatial heterogeneity. The Ks decreased gradually from sites 1 to 9, and the Ks of the soil at the down slope position was significantly lower than that at the upper and middle slope positions (p < 0.05). Moreover, the difference in the Ks between the different test sites at the same slope position was not significant. The difference in Ks among the different slope positions was greatest in layer A. For layer A, the average Ks at site 1 was 52.34 mm/min, whereas that at site 9 was 25.24 mm/min. At the same slope position, the Ks values of the different soil layers also significantly differed, and the Ks values of the A layer were significantly higher than those of the AC and C layers. As indicated in Table 1, the differences in Ks among the down, middle and upper slope positions in the A, AC and C layers were significant (p < 0.05). In summary, the Ks values of Karst dolomite slopes exhibit consistent spatial variability.
Figure 2. Vertical variation of saturated hydraulic conductivity of soil profiles at different slope positions. Different uppercase and lowercase indicated significant differences at 0.05 level between different slope positions.
Table 1. Test for saturated hydraulic conductivity of different slope sites (upper slope, middle slope and lower slope) in the test Karst slope different soil layer (A layer, AC layer and C layer).
As shown in Figure 3, the DOC, DON, TN, TP and TK contents along the slope during the rainy and dry seasons were the lowest at the upper slope position and the highest at the down slope position. In addition, the difference in soil nutrients between the rainy and dry seasons gradually decreased from the upper slope position to down slope position. The soil nutrient levels at each site were lower during the rainy season than during the dry season. Notably, soluble nutrients in the soil were lost after the rainy season. This phenomenon was most evident at the upper slope position, and the decrease in soil nutrients at the upper slope position was greater than that at the middle and down slope positions after the rainy season. In the vertical profile, the soil DOC, DON, TN, TP, and TK contents gradually decreased with increasing soil layer depth. Notably, these values were highest in the A layer and lowest in the C layer. In addition, the analysis of nutrient changes at different soil depths during the rainy and dry seasons revealed that the loss of soil nutrients after the rainy season mainly occurred in the A layer. The loss of nutrients in the A layer was greater than that in the AC and C layers after the rainy season, and the DOC, DON, TN, TP, and TK contents in the A layer at the upper slope position significantly differed between the rainy and dry seasons (p < 0.05). Compared with those before the rainy season, the soil nutrient levels in each layer basically decreased, but the nutrient levels in the deep layers (AC and C layers) at the down slope position slightly increased. Thus, after the rainy season, the DOC, DON, TN, TP, and TK migrated under the influence of water infiltration, resulting in a significant decrease in nutrients in the topsoil layer.
Figure 3. Rainy and dry season variations of soil nutrient content in different slopes and soil horizons on karst dolomite slopes.
The correlations between the changes in soil nutrients and the basic properties during the rainy and dry seasons are shown in Figure 4. The results revealed that the correlations between the soil nutrient changes and soil properties were consistent across the upper, middle and down slope positions, indicating that the losses of soil DOC, DON, TN, TP, and TK were consistent. Further analysis of the correlations between the changes in soil nutrients and soil properties revealed a significant positive correlation between the changes in soil nutrients and Ks at the three slope positions (p < 0.01).
Figure 4. Correlation analysis between soil nutrient content variation and soil properties at different slope locations on karst dolomite slopes.
Further analysis of the correlation between the soil nutrient change rate and basic soil properties revealed that the change in soil nutrients at the three slope positions was significantly positively correlated with Ks (p < 0.01) and significantly positively correlated (p < 0.05 or p < 0.01) with the noncapillary porosity (NCP). In addition, there was a significant negative correlation (p < 0.01) between the changes in soil nutrients and BD at the upper and middle slope positions. Therefore, soil nutrient loss is related to soil properties, especially NCP and Ks.
As indicated in Table 3, the rainy and dry seasons are very distinct in the Karst dolomite area. The rainfall amount in December 2018 was only 7.0 mm, but in September, it reached 228.0 mm. Similar variation patterns were also observed in 2019, 2020, and 2021. The minimum rainfall during the monitoring period was 0.4 mm (January 2019), and the maximum rainfall was 740.4 mm (September 2020). Due to the significant difference in nutrients between the rainy and dry seasons, mainly concentrated in the surface layer, the dynamic trend of soil moisture content in the surface layer (0–20 cm) of different slope positions was analyzed (Figures 5, 6). As shown in Figure 5, during the monitoring period from 2018 to 2021, the average soil water contents at the upper, middle and down slope positions were 0.14, 0.09 and 0.39 cm3/cm3, respectively. Notably, the soil water content gradually increased from the upper to down slope positions in general, and the down slope position presented a higher soil water content than that at the upper and middle slope positions. Additionally, the coefficient of variation (CV) of the soil water content at the different slope positions was analyzed during the monitoring period. The CV is the ratio of the standard deviation to the average value, which reflects the degree of statistical dispersion of the sample values. The CV values of the soil water content at the upper, middle and down slope positions were 50.23%, 36.76% and 17.49%, respectively. The CV of the soil water content was greater at the upper slope position than at the middle and down slope positions, indicating that the fluctuation in the soil water content at the upper slope position was the greatest.
Figure 5. Descriptive statistics of soil water content on the different slope positions during the observation period.
Figure 6. Temporal variation of daily average soil water content on the different slope positions during the observation period.
Furthermore, as shown in Figure 6, the variation characteristics of the soil water content and the response to rainfall at the three slope positions were basically consistent. The soil water content is generally high during rainfall, and the maximum soil water contents at the upper, middle and down slope positions are 0.38, 0.43 and 0.57 cm3/cm3, respectively. The maximum soil water content occurs during rainfall. Moreover, the difference in the soil water content between the upper and middle slope positions was small, whereas the difference between the upper and down slope positions was notable. In general, the characteristics of the soil water content on the Karst dolomite slope during the monitoring period were as follows: down slope > middle slope > upper slope. In addition, the variation trends in the soil water content and CV were opposite. That is, the soil water content at the upper slope was low, with a relatively large variation range and a relatively dispersed distribution. In contrast, the soil water content at the down slope position was high, with a small variation range and a relatively concentrated distribution, and the response to rainfall was not as obvious as that at the upper slope position.
The changes in soil water and temperature during the monitoring period when typical preferential and matrix flow processes occurred in the slope are shown in Figures 7, 8, respectively. Figure 7 shows that the soil water content gradually increased from depths of 0–20 cm, 40–60 cm and 20–40 cm. During preferential flow, water directly infiltrated from the surface to depths of 40–60 cm, and the deep soil water content changed rapidly. Moreover, temperature analysis revealed that because the air temperature (20.9°C) was lower than the topsoil temperature (25.8°C and 24.7°C) and heat exchange between rainwater and soil was greater, resulting in the topsoil temperature gradually decreasing with increasing rainfall. The 20–40 cm layer exhibited soil matrix flow, and because the soil temperature at 0–20 cm was greater than that at 20–40 cm and 40–60 cm, the soil temperature at 20–40 cm first increased but then decreased. The preferential flow caused the soil temperature at depths of 40–60 cm to decrease prior to the other layers. Moreover, during the infiltration process, the rainwater temperature increased due to the heat exchange with the high-temperature soil, resulting in the soil temperature at depths of 40–60 cm gradually rising later.
The changes in the soil water content and temperature during matrix flow differed from those during preferential flow (Figure 8). In particular, with rainfall infiltration, the soil water content at depths of 0–20 cm, 20–40 cm and 40–60 cm increased in turn. During rainfall, the temperature within the 0–20 cm soil layer gradually decreased because the rain temperature was lower than the surface soil temperature. However, due to the difference in temperature among the three soil layers, the soil temperature at 0–20 cm was greater than that of the deeper soil, so water replacement occurred with rainfall infiltration. That is, before the rainfall arrives, the water in the surface soil infiltrates into the deeper soil, causing the soil temperature to rise at the depth at 40–60 cm, however, when the rainfall arrives, the temperature begins to decrease.
During the monitoring period, the soil water content in the Karst slope varied among different slope positions under the same climatic conditions, as shown in Figures 5, 6. The soil water content at the down slope position was significantly greater than that at the middle and upper slope positions. The CV of the soil water content was the lowest at the down slope position, which was the opposite of the trend in the soil water content. Cui and Zhi (2018) noted that the main controlling factors affecting soil hydrological processes and water distributions differ at different regional scales. On a small scale, when there are no significant differences in the slope topography or vegetation, factors including the soil BD, texture, and cracks, more greatly impact soil hydrological processes.
We consider that the low soil water content at the upper slope position during the monitoring period may be related to the large number of aeration pores and the high Ks of the soil at that position. The distribution of pores in the Karst slope is evident, and the content of aeration pores is high at the upper slope position, which notably affects the process of soil water infiltration (Hincapie and Germann, 2009). Zhang et al. (2014) noted that soil with more aeration pores and a denser macropore distribution exhibited greater soil connectivity and greater Ks. This may be the main reason why the Ks at the upper slope position is significantly (p < 0.05) greater than that at the middle and down slope positions (Figure 2; Table 2). In addition, soil with more aeration pores can provide favorable infiltration channels for surface water (Sheng et al., 2009; Krisnanto et al., 2016). Therefore, preferential flow occurs easily in the surface soil later because soil water content is less concentrated in that soil layer, the depth of the wetting front is significantly greater than that in soil with fewer aeration pores, and the subsurface flow process is fast, so preferential flow easily occurs (Liu et al., 2005; Abaci and Papanicolaou, 2009).
An analysis of the monitoring results of the soil water content revealed that preferential flow occurs on Karst slopes during rainfall (Figure 7). We speculate that the frequency of soil preferential flow varies among the different slope positions and that the frequency of soil preferential flow at the down slope position is lower than that at the middle and upper slope positions. This may be due to the significant difference in the soil Ks among the different slope positions, as shown in Figure 2 and Table 2.
The soil at the upper slope position exhibits a higher water permeability than that at the down slope position, and the Ks is significantly greater than that at the down slope position. Therefore, the resistance of the soil water content on the down slope in the process of infiltration is high, and resulting in a lower possibility of preferential flow at the down slope position. In addition, when the soil water content at the lower slope position is the highest, but the CV is the lowest, as shown in Figures 5, 6. Bodner et al. (2013) pointed out that the frequency of preferential flow is lower for soils with high water content and low CV values, and the transport capacity of soil water is lower, which is consistent with our view. Moreover, the close relationship between the occurrence frequency of preferential flow and the soil water content has also been widely recognized. The main principle is that water easily infiltrates soil with a high frequency of preferential flow, resulting in a low soil water content in the surface layer (Greve et al., 2010; Hardie et al., 2011; Tao et al., 2017; Wei et al., 2024).
In addition to the porosity and Ks, the difference in the soil mechanical composition affects the frequency of preferential flow (Hincapie and Germann, 2009; Beven and Germann, 2013). As shown in Figure 1, the soil mechanical composition at the different slope positions on the Karst slope exhibits obvious spatial variability, and the soil sand content at the upper slope position is significantly greater than that at the down slope position. Tao et al. (2020) noted that on granite Benggang slopes, an increase in the sand content promotes the formation and development of preferential soil flow, and the amount of preferential flow in the sand layer is three times greater than that of matrix flow. This is consistent with the analysis of preferential flow and texture in this study. The occurrence frequency of preferential flow at the upper slope position with a higher sand content is greater than that at the down slope position with a lower sand content. The reason for this phenomenon may be that the specific surface area of sand particles is smaller than that of clay particles, and the adsorption capacity for soil water content is low. Therefore, under the same porosity, water is more likely to be discharged from pores in soils with higher sand contents (Carbajal-Morón et al., 2017), which improves the soil water infiltration capacity, and thus the degree of preferential flow is greater.
We found that the soil DOC, DON, TN, TP, and TK contents in the dolomite Karst slope exhibited obvious spatial heterogeneity. Along the slope direction, the soil nutrient content gradually increased from the upper to down slope positions. In addition, the degree of nutrient loss at the different slope positions varied, and the difference in soil nutrients between the rainy and dry seasons gradually decreased at the nine test points from the upper to down slope positions. Along the vertical profile direction, the DOC, DON, TN, TP, and TK contents in the A layer during the rainy and dry seasons significantly differed, whereas the nutrient contents in the AC and C layers slightly changed (the difference was not significant). As shown in Figure 4, the soil aeration porosity and Ks significantly affected the changes in soil nutrients during the rainy and dry seasons. From the upper to down slope positions, the soil sand content, aeration pore content and Ks gradually decreased, and the loss of soil nutrients between the rainy and dry seasons was low. The significant effects of the lithology and soil properties on the loss of soil nutrients have been reported in a few studies (Albaladejo et al., 2012; Hoffmann et al., 2014; Rossel et al., 2014; Turrión et al., 2009). Teixeira and Misra (2005) noted that the magnitude of the soil nutrient loss was affected by the distribution of macropores. In addition to the soil properties, this difference may be related to subsurface flow. Soil physical properties and lithology affect the soil nutrient loss by influencing the distribution and development of subsurface flow.
Soil water–nutrient seepage is the primary mechanism of soil and water loss in Karst areas (Perakis and Hedin, 2002), and soil nutrients migrate mainly through hydrodynamic forces, which are closely related to water infiltration (Fu et al., 2016; Dai et al., 2017). Combined with Figures 3, 5 the trends in nutrient loss are consistent with the variation characteristics of the CV of the soil water content, with a high CV of the soil water content associated with an increase in the migration rate of soil nutrients. These findings indicate that soil water infiltration due to rainfall is the most direct factor influencing soil nutrient loss on Karst slopes.
Moreover, owing to the pronounced development of surface Karst features and the dense distribution of underground fissures in the Karst area of Southwest China, there is obvious underground seepage in this area (Zhang et al., 2011; Yuan et al., 2012). The results of this study also revealed a preferential flow phenomenon on the Karst slope (Figure 7). We considered that the existence of preferential flow further caused differences in nutrient loss along the slope direction. Preferential flow is an important component connecting the aboveground and underground systems in Karst areas and provides seepage and storage spaces for soil, water, and nutrients. Preferential flow can lead to water displacement, and some of the collected upper soil water moves to the deeper soil to replace the original water (Ma et al., 2016). Soil nutrients, especially DOC and DON, are active components of soil nitrogen and exhibit notable mobility, which can be lost by runoff or leaching with water transport (Perakis and Hedin, 2002; Teixeira and Misra, 2005). Combined with the theory of water replacement, these findings indicate that soluble nutrients may directly reach the deep layer via preferential flow channels. Soil water infiltration at the upper slope position progressed rapidly, and preferential flow frequently occurred, resulting in underdeveloped slope surface runoff, which could easily result in a reduction in the nutrient content in the soil through subsurface flow during rainfall. The rapid infiltration of soil water at the upper slope position and frequent preferential flow contribute to the rapid infiltration of surface water into deep layers, resulting in underdeveloped slope surface runoff and soil nutrient loss during rainfall. This finding is similar to the results of Yan et al. (2019), who reported that fissures with poor connectivity have better soil nutrient and fertility conditions. With decreasing slope position, the soil type on Karst dolomite slopes transitions from lithologic and shallow soils to Alfisols (Meng et al., 2022). The high clay content of Alfisols at the down slope position and the low frequency of preferential flow are helpful for maintaining the relative stability of nutrient levels. The existence of preferential flow easily leads to the direct downward migration of nutrients without interactions with upper soil organisms, which notably impacts the regional nutrient distribution (Allaire et al., 2009). The rate of nutrient loss at the upper slope position was greater than that at the down slope position, indicating that the preferential flow area at the upper slope position was the main zone of rapid soil nutrient loss.
In addition, the variation in TP between the rainy and dry seasons was the lowest among all soil nutrients. This may be due to the intense transformation reaction of phosphorus in soil and the high ability of the soil to adsorb and retain phosphorus, which makes it difficult to transport phosphorus in the soil. In conclusion, preferential flow in Karst dolomite rock leads to nutrient migration, resulting in significant differences in soil surface nutrient changes between the rainy and dry seasons at different slope positions.
The Ks of the Karst dolomite slope exhibited clear heterogeneity along the slope, the Ks at the upper slope position is significantly (p < 0.05) greater than that at the middle and down slope positions. Owing to the low hydraulic conductivity at the down slope position, the soil water content was greater than that at the middle and upper slope positions. Coupling monitoring of the soil water content and temperature revealed obvious preferential flow on the Karst dolomite slope, and the water content and temperature changes in the soil layer with preferential flow occurred earlier than those in the other soil layers without preferential flow. The patterns of the soil water content and surface nutrient loss on the Karst dolomite slope are consistent across the various positions and are related to the occurrence of preferential flow. There are significant differences in the changes between the different slope positions during the rainy and dry seasons. The average water content at the lower slope position is the highest, and the CV values of the soil water content and nutrient loss are the lowest. Our results demonstrate that the preferential flow area at the upper slope position is the main zone of soil nutrient loss. Therefore, in Karst areas with rocky desertification and ecological management, we should first analyze the occurrence of preferential flow in surface Karst zones and then explore nutrient migration mechanism. Moreover, soil and water conservation measures should be implemented based on the preferential flow and nutrient characteristics of different slope positions. Seal off on the upper and middle slopes to avoid human disturbance, while coverage and no tillage measures should be carried out on the down slope to reduce nutrient loss.
The original contributions presented in the study are included in the article/supplementary material, further inquiries can be directed to the corresponding authors.
XD: Conceptualization, Data curation, Formal Analysis, Investigation, Methodology, Validation, Visualization, Writing–original draft, Writing–review and editing. ZF: Data curation, Methodology, Project administration, Software, Writing–review and editing. YD: Conceptualization, Investigation, Project administration, Software, Supervision, Writing–original draft. HC: Conceptualization, Data curation, Funding acquisition, Project administration, Resources, Supervision, Validation, Writing–review and editing.
The author(s) declare that financial support was received for the research, authorship, and/or publication of this article. We thank the financial support for the research provided by the National Natural Science Foundation of China (No. 41930866), the Guangxi Key Research and Development Program, China (No. AB22035058) and the National Key Research and Development Program of China (No. 2023YFD1902801).
The authors declare that the research was conducted in the absence of any commercial or financial relationships that could be construed as a potential conflict of interest.
The author(s) declare that no Generative AI was used in the creation of this manuscript.
All claims expressed in this article are solely those of the authors and do not necessarily represent those of their affiliated organizations, or those of the publisher, the editors and the reviewers. Any product that may be evaluated in this article, or claim that may be made by its manufacturer, is not guaranteed or endorsed by the publisher.
Abaci, O., and Papanicolaou, A. T. (2009). Long-term effects of management practices on water-driven soil erosion in an intense agricultural sub-watershed: monitoring and modelling. Hydrol. Process. 23 (19), 2818–2837. doi:10.1002/hyp.7380
Albaladejo, J., Ortiz, R., Garcia-Franco, N., Navarro, A. R., Almagro, M., Pintado, J. G., et al. (2012). Land use and climate change impacts on soil organic carbon stocks in semi-arid Spain. J. Soils Sediments 13 (2), 265–277. doi:10.1007/s11368-012-0617-7
Allaire, S. E., Roulier, S., and Cessna, A. j. (2009). Quantifying preferential flow in soils: a review of different techniques. J. Hydrology 378 (1–2), 179–204. doi:10.1016/j.jhydrol.2009.08.013
An, N., Hemmati, S., and Cui, Y. J. (2017). Numerical analysis of soil volumetric water content and temperature variations in an embankment due to soil-atmosphere interaction. Comput. Geotechnics 83, 40–51. doi:10.1016/j.compgeo.2016.10.010
Bao, S. D. (2000). Soil and agricultural chemistry analysis. 3rd ed. Beijing: China Agriculture Publishing House, 263–270.
Beven, K., and Germann, P. (2013). Macropores and water flow in soils revisited. Water Resour. Res. 49 (6), 3071–3092. doi:10.1002/wrcr.20156
Bodner, G., Scholl, P., and Kaul, H. P. (2013). Field quantification of wetting-drying cycles to predict temporal changes of soil pore size distribution. Soil Tillage Res. 133, 1–9. doi:10.1016/j.still.2013.05.006
Carbajal-Morón, N. A., Manzano, M. G., and Mata-González, R. (2017). Soil hydrology and vegetation as impacted by goat grazing in Vertisols and Regosols in semi-arid shrublands of northern Mexico. Rangel. J. 39 (4), 363–373. doi:10.1071/RJ17061
Cerda, A., and Doerr, S. H. (2010). The effect of ant mounds on overland flow and soilerodibility following a wildfire in eastern Spain. Ecohydrology 3 (4), 392–401. doi:10.1002/eco.147
Chen, H. S., Liu, J. W., Zhang, W., and Wang, K. L. (2012). Soil hydraulic properties on the steep karst hillslopes in northwest guangxi, China. Environ. Earth Sci. 66 (1), 371–379. doi:10.1007/s12665-011-1246-y
Clothier, B. E., Green, S. R., and Deurer, M. (2008). Preferential flow and transport in soil: progress and prognosis. Eur. J. Soil Sci. 59, 2–13. doi:10.1111/j.1365-2389.2007.00991.x
Cui, G., and Zhu, J. (2018). Prediction of unsaturated flow and water backfill during infiltration in layered soils. J. Hydrology 557, 509–521. doi:10.1016/j.jhydrol.2017.12.050
Dai, Q. H., Peng, X. D., Yang, Z., and Zhao, L. S. (2017). Runoff and erosion processes on bare slopes in the karst rocky desertification area. CATENA 152, 218–226. doi:10.1016/j.catena.2017.01.013
Duan, X. Q., Deng, Y. S., Tao, Y., He, Y. B., Lin, L. R., and Chen, J. Z. (2021). The soil configuration on granite residuals affects benggang erosion by altering the soil water regime on the slope. Int. Soil Water Conservation Res. 9 (3), 419–432. doi:10.1016/j.iswcr.2021.03.003
Fu, Z. Y., Chen, H. S., Xu, Q. X., Jia, J., Wang, S., and Wang, K. L. (2016). Role of epikarst in near-surface hydrological processes in a soil mantled subtropical dolomite karst slope: implications of field rainfall simulation experiments. Hydrol. Process. 30 (5), 795–811. doi:10.1002/hyp.10650
Fu, Z. Y., Chen, H. S., Zhang, W., Xu, Q. X., Wang, S., and Wang, K. L. (2015). Subsurface flow in a soil-mantled subtropical dolomite karst slope: a field rainfall simulation study. Geomorphology 250, 1–14. doi:10.1016/j.geomorph.2015.08.012
Gerke, K. M., Sidle, R. C., and Mallants, D. (2015). Preferential flow mechanisms identified from staining experiments in forested hillslopes. Hydrol. Process. 29 (21), 4562–4578. doi:10.1002/hyp.10468
Gharabaghi, B., Safadoust, A., Mahboubi, A. A., Mosaddeghi, M. R., Unc, A., Ahrens, B., et al. (2015). Temperature effect on the transport of bromide and E. coli NAR in saturated soils. J. Hydrology 522, 418–427. doi:10.1016/j.jhydrol.2015.01.003
Greve, A., Andersen, M. S., and Acworth, R. I. (2010). Investigations of soil cracking and preferential flow in a weighing lysimeter filled with cracking clay soil. J. Hydrology 393 (1–2), 105–113. doi:10.1016/j.jhydrol.2010.03.007
Hardie, M. A., Cotching, W. E., Doyle, R. B., Holz, G., Lisson, S., and Mattern, K. (2011). Effect of antecedent soil moisture on preferential flow in a texture-contrast soil. J. Hydrology 398 (3–4), 191–201. doi:10.1016/j.jhydrol.2010.12.008
Hartmann, A., Goldscheider, N., Wagener, T., Lange, M., and Weiler, M. (2015). Karst water resources in a changing world: review of hydrological modeling approaches. Rev. Geophys. 52 (3), 218–242. doi:10.1002/2013RG000443
Hencher, S. R. (2010). Preferential flow paths through soil and rock and their association with landslides. Hydrol. Process. 24 (12), 1610–1630. doi:10.1002/hyp.7721
Herman, E. K., Toran, L., and William, W. B. (2012). Clastic sediment transport and storage in fluviokarst aquifers: an essential component of karst hydrogeology. Carbonates Evaporites 27 (3–4), 211–241. doi:10.1007/s13146-012-0112-7
Hincapie, I., and Germann, P. F. (2009). Impact of initial and boundary conditions on preferential flow. J. Contam. Hydrology 104 (1–4), 67–73. doi:10.1016/j.jconhyd.2008.10.001
Hoffmann, U., Hoffmann, T., Jurasinski, G., Glatzel, S., and Kuhn, N. J. (2014). Assessing the spatial variability of soil organic carbon stocks in an alpine setting (Grindelwald, Swiss Alps). Geoderma 232–234, 270–283. doi:10.1016/j.geoderma.2014.04.038
Hopp, L., and McDonnell, J. J. (2009). Connectivity at the hillslope scale: identifying interactions between storm size, bedrock permeability, slope angle and soil depth. J. Hydrology 376 (3–4), 378–391. doi:10.1016/j.jhydrol.2009.07.047
Hu, P. L., Zhao, Y., Xiao, D., Xu, Z. H., Zhang, W., Xiao, J., et al. (2021). Dynamics of soil nitrogen availability following vegetation restoration along a climatic gradient of a subtropical karst region in China. J. Soils Sediments 21, 2167–2178. doi:10.1007/s11368-021-02915-0
Jiang, Z. C., Lian, Y. Q., and Qin, X. Q. (2014). Rocky desertification in Southwest China:impacts, causes, and restoration. Earth-Science Rev. 132, 1–12. doi:10.1016/j.earscirev.2014.01.005
Karchegani, P. M., Ayoubi, S., Mosaddeghi, M. R., and Honarjoo, N. (2012). Soil organic carbon pools in particle-size fractions as affected by slope gradient and land use change in hilly regions, western Iran. J. Mt. Sci. 9, 87–95. doi:10.1007/s11629-012-2211-2
Kooijman, A. M., Jongejans, J., and Sevink, J. (2005). Parent material effects on Mediterranean woodland ecosystems in NE Spain. Catena 59 (1), 55–68. doi:10.1016/j.catena.2004.05.004
Korkanc, S. Y., and Dorum, G. C. (2019). The nutrient and carbon losses of soils from different land cover systems under simulated rainfall conditions. CATENA 172, 203–211. doi:10.1016/j.catena.2018.08.033
Krisnanto, S., Rahardjo, H., Fredlund, D. G., and Leong, E. C. (2016). Water content of soil matrix during lateral water flow through cracked soil. Eng. Geol. 210, 168–179. doi:10.1016/j.enggeo.2016.06.012
Li, D. J., Wen, L., Yang, L. Q., Luo, P., Xiao, K. C., Chen, H., et al. (2017). Dynamics of soil organic carbon and nitrogen following agricultural abandonment in a karst region. J. Geophys. Res. Biogeosciences 122 (1), 230–242. doi:10.1002/2016JG003683
Li, X. W., Jin, M. G., Huang, J. O., and Yuan, J. J. (2014). The soil–water flow system beneath a cotton field in arid North-west China, serviced by mulched drip irrigation using brackish water. Hydrogeology J. 23, 35–46. doi:10.1007/s10040-014-1210-5
Li, Z. W., Xu, X. L., Zhu, J. X., Xu, C. H., and Wang, K. L. (2019). Effects of lithology and geomorphology on sediment yield in karst mountainous catchments. Geomorphology 343, 119–128. doi:10.1016/j.geomorph.2019.07.001
Liu, H. H., Zhang, R., and Bodvarsson, G. S. (2005). An active region model for capturing fractal flow patterns in unsaturated soils: model development. J. Contam. Hydrology 80, 18–30. doi:10.1016/j.jconhyd.2005.07.002
Liu, Y., Chen, J., Zhang, X. J., Li, Q. Y., Du, Y., Liu, J. C., et al. (2020). Herbaceous community species composition and productivity are affected by soil depth and funnel effect in a simulated karst experiment. Glob. Ecol. Conservation 22, e01033. doi:10.1016/j.gecco.2020.e01033
Lu, X. Q., Toda, H., Ding, F. J., Fang, S. Z., Yang, W. X., and Xu, H. G. (2014). Effect of vegetation types on chemical and biological properties of soils of karst ecosystems. Eur. J. Soil Biol. 61, 49–57. doi:10.1016/j.ejsobi.2013.12.007
Ma, Y. Y., Qu, L. Q., Wang, W., Yang, X. S., and Lei, T. W. (2016). Measuring soil water content through volume/mass replacement using a constant volume container. Geoderma 271, 42–49. doi:10.1016/j.geoderma.2016.02.003
Meng, Q. M., Wang, S., Fu, Z. Y., Deng, Y. S., and Chen, H. S. (2022). Soil types determine vegetation communities along a toposequence in a dolomite peak-cluster depression catchment. Plant Soil 475, 5–22. doi:10.1007/s11104-022-05308-5
Nimmo, J. R. (2012). Preferential flow occurs in unsaturated conditions. Hydrol. Process. 26, 786–789. doi:10.1002/hyp.8380
Peng, T., and Wang, S. J. (2012). Effects of land use, land cover and rainfall regimes on the surface runoff and soil loss on karst slopes in southwest China. CATENA 90, 53–62. doi:10.1016/j.catena.2011.11.001
Perakis, S. S., and Hedin, L. O. (2002). Nitrogen loss from unpolluted South American forests mainly via dissolved organic compounds. Nature 415, 416–419. doi:10.1038/415416a
Rossel, R. A. V., Webster, R., Bui, E. N., and Baldock, J. A. (2014). Baseline map of organic carbon in Australian soil to support national carbon accounting and monitoring under climate change. Glob. Change Biol. 20 (9), 2953–2970. doi:10.1111/gcb.12569
Sheng, F., Wang, K., Zhang, R. D., and Liu, H. H. (2009). Characterizing soil preferential flow using iodine-starch staining experiments and the active region model. J. Hydrology 367 (1–2), 115–124. doi:10.1016/j.jhydrol.2009.01.003
Song, X. W., Gao, Y., Green, S. M., Dungait, J. A. J., Peng, T., Quine, T. A., et al. (2017). Nitrogen loss from Karst area in China in recent 50 years: an in-situ simulated rainfall experiment’s assessment. Ecol. Evol. 7 (23), 10131–10142. doi:10.1002/ece3.3502
Tao, Y., He, Y. B., Duan, X. Q., Zou, Z. Q., Lin, L. R., and Chen, J. Z. (2017). Preferential flows and soil moistures on a Benggang slope: determined by the water and temperature co-monitoring. J. Hydrology 553, 678–690. doi:10.1016/j.jhydrol.2017.08.029
Tao, Y., Zou, Z. Q., Guo, L. H., Yang, B. L., Lin, L., Lin, H., et al. (2020). Linking soil macropores, subsurface flow and its hydrodynamic characteristics to the development of benggang erosion. J. Hydrology 586, 124829. doi:10.1016/j.jhydrol.2020.124829
Teixeira, P. C., and Misra, R. K. (2005). Measurement and prediction of nitrogen loss by simulated erosion events on cultivated forest soils of contrasting structure. Soil Tillage Res. 83 (2), 204–217. doi:10.1016/j.still.2004.07.014
Turrión, M.-B., Schneider, K., and Gallardo, J. F. (2009). Carbon accumulation in umbrisols under Quercus pyrenaica forests: effects of bedrock and annual precipitation. CATENA 79 (1), 1–8. doi:10.1016/j.catena.2009.04.004
Wang, D. J., Shen, Y. X., Huang, J., and Li, Y. H. (2016). Rock outcrops redistribute water to nearby soil patches in karst landscapes. Environ. Sci. Pollut. Res. 23 (9), 8610–8616. doi:10.1007/s11356-016-6091-9
Wei, H., Yang, Y. F., Wang, J. Y., Meng, Q. M., and Deng, Y. S. (2024). A comparison of preferential flow characteristics and influencing factors between two soils developed in the karst region of Southwest China. Soil Tillage Res. 241, 106132. doi:10.1016/j.still.2024.106132
Wen, L., Li, D. J., Yang, L. Q., Luo, P., Chen, H., Xiao, K. C., et al. (2016). Rapid recuperation of soil nitrogen following agricultural abandonment in a karst area, southwest China. Biogeochemistry 129, 341–354. doi:10.1007/s10533-016-0235-3
Xiao, D., Tan, Y. J., Liu, X., Yang, R., Zhang, W., He, X. Y., et al. (2020). Responses of soil diazotrophs to legume species and density in a karst grassland, southwest China. Agric. Ecosyst. and Environ. 288, 106707. doi:10.1016/j.agee.2019.106707
Yan, Y. J., Dai, Q. H., Jin, L., and Wang, X. D. (2019). Geometric morphology and soil properties of shallow karst fissures in an area of karst rocky desertification in SW China. CATENA 174, 48–58. doi:10.1016/j.catena.2018.10.042
Yao, Y. W., Dai, Q. H., Gan, Y. X., Gao, R. X., Yan, Y. J., and Wang, Y. H. (2021). Effects of rainfall intensity and underground hole (fracture) gap on nutrient loss in karst sloping farmland. Sci. Agric. Sin. 54 (1), 140–151. doi:10.3864/j.issn.0578-1752.2021.01.010
Yuan, H., Hu, N., Huang, Y. X., Zhang, Y. Z., He, X. Y., and Xie, H. X. (2012). Study on the response of soil nutrient content to soil loss in karst sloping land of southwest China. Meteorological Environ. Res. 3 (3–4), 46–52. doi:10.13989/j.cnki.0517-6611.2012.12.150
Zhang, J., Chen, H. S., Fu, Z. Y., and Wang, K. L. (2021). Effects of vegetation restoration on soil properties along an elevation gradient in the karst region of southwest China. Agric. Ecosyst. and Environ. 320, 107572. doi:10.1016/j.agee.2021.107572
Zhang, W., Zhao, J., Pan, F., Li, D., Chen, H., and Wang, K. (2015). Changes in nitrogen and phosphorus limitation during secondary succession in a karst region in southwest China. Plant Soil 391, 77–91. doi:10.1007/s11104-015-2406-8
Zhang, X. B., Bai, X. Y., and He, X. B. (2011). Soil creeping in the weathering crust of carbonate rocks and underground soil losses in the karst mountain areas of southwest China. Carbonates Evaporites 26 (2), 149–153. doi:10.1007/s13146-011-0043-8
Zhang, Z. B., Zhou, H., Zhao, Q. G., Lin, H., and Peng, X. (2014). Characteristics of cracks in two paddy soils and their impacts on preferential flow. Geoderma 228–229, 114–121. doi:10.1016/j.geoderma.2013.07.026
To better describe and more clearly show the sampling sites for karst slope, we added the photographs of actual peak-cluster depression karst landscape, and marked the sampling sites of slope shown in Figure A1.
Keywords: water distribution, preferential flow, nutrient loss, Karst region, dynamic monitoring
Citation: Duan X, Fu Z, Deng Y and Chen H (2025) Characteristics of water distribution and preferential flow processes and nutrient response on dolomite slopes in the southwestern karst region. Front. Environ. Sci. 12:1511449. doi: 10.3389/fenvs.2024.1511449
Received: 15 October 2024; Accepted: 19 November 2024;
Published: 03 January 2025.
Edited by:
Xudong Peng, Guizhou University, ChinaReviewed by:
Mehmet Ali Çullu, Harran University, TürkiyeCopyright © 2025 Duan, Fu, Deng and Chen. This is an open-access article distributed under the terms of the Creative Commons Attribution License (CC BY). The use, distribution or reproduction in other forums is permitted, provided the original author(s) and the copyright owner(s) are credited and that the original publication in this journal is cited, in accordance with accepted academic practice. No use, distribution or reproduction is permitted which does not comply with these terms.
*Correspondence: Hongsong Chen, aGJjaHNAaXNhLmFjLmNu
Disclaimer: All claims expressed in this article are solely those of the authors and do not necessarily represent those of their affiliated organizations, or those of the publisher, the editors and the reviewers. Any product that may be evaluated in this article or claim that may be made by its manufacturer is not guaranteed or endorsed by the publisher.
Research integrity at Frontiers
Learn more about the work of our research integrity team to safeguard the quality of each article we publish.