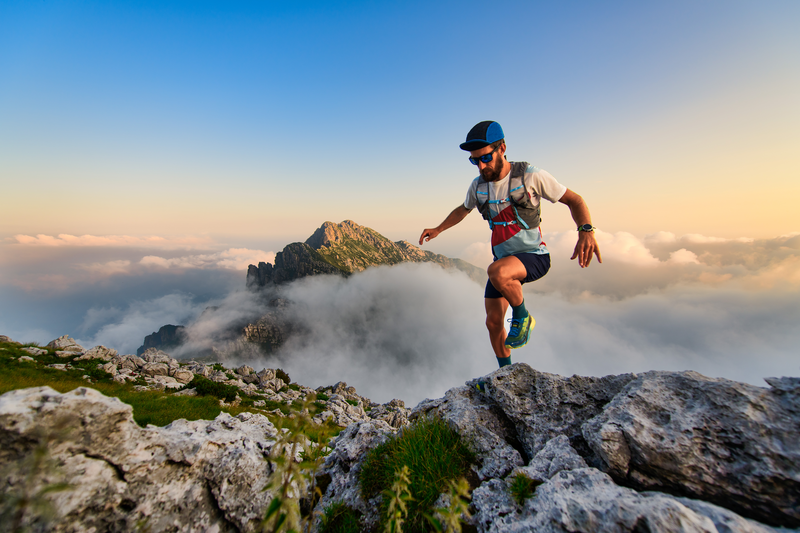
94% of researchers rate our articles as excellent or good
Learn more about the work of our research integrity team to safeguard the quality of each article we publish.
Find out more
ORIGINAL RESEARCH article
Front. Environ. Sci. , 03 January 2025
Sec. Environmental Informatics and Remote Sensing
Volume 12 - 2024 | https://doi.org/10.3389/fenvs.2024.1506701
This article is part of the Research Topic Restoring Our Blue Planet: Advances in Marine and Coastal Restoration View all 3 articles
Coastal basins stand out for their continent-estuary interface and connection as corridors of mangrove forests. The Maracanã River Basin (MRB) represents this environment, holding various ecosystem services for the component municipalities, protected areas with highly sensitive environments and water demand, and potential for multiple water uses. The proposed aim was to identify areas of degradation and environmental conservation in the MRB using the Blueprint model as support for water resource management. The methodology involved the application of the Blueprint model in the MRB, based on land use and cover information, rainfall, and characterization of the ecological units. The results showed that the MRB basin predominantly exhibits the degradation and restoration/connectivity classes in the Annual Blueprint (ABP) and Dry Blueprint (DBP), respectively. On the other hand, the Rainy Blueprint (RBP) predominantly exhibits Environmental Integrity. Statistical tests showed significant differences between ABP-RBP and DBP-RBP, which can be explained by the fact that on an annual scale of analysis, Blueprint classes are more heterogeneous, with a tendency toward environmental integrity, and intermediate classes in the rainy period; in the dry period, restoration and connectivity and degradation classes predominate. The correlation analysis indicates that natural vegetation cover shows a significant correlation with annual precipitation, rainy and dry quarters. These results provide significant insight into understanding the dynamics of degradation and conservation areas, assisting decision-makers in the environmental planning of the basin. In addition, the climatic component showed a differential response on annual and seasonal scales, acting as a modulating agent of the indicators.
A watershed is a topographically delineated area defined by an open and dynamic drainage system, interacting with elements such as rocks, sediments, climate, geomorphology, hydrology, vegetation, soil, and social variables (Gomes et al., 2021). Therefore, the watershed is also used as a unit for physical, socioeconomic, and policy analyses suitable for its planning and management, given its importance for various living organisms and activities such as agriculture and industry (Wang et al., 2016). Consequently, watershed management contributes to better utilization of water resources through instruments and decision-making related to ecological, land, and water processes (Katusiime and Schütt, 2020).
Worldwide, demographic, economic, and technological trends have accelerated the human capacity to modify the environment, impacting the global climate (Fassoni-Andrade et al., 2021). The potential observed consequences are associated with variations in the amount, spatial, and temporal distribution of rainfall, as well as landscape changes resulting from the growth of food and energy production in urban centers (Cosgrove and Loucks, 2015; Mello et al., 2020).
Water is considered an economic and finite resource. It depends on supply conditions linked to the climatic regime, which ensures the main form of entry into environmental systems through rainfall. Population growth increases demand and leads to conflicts due to reduced availability, whether through decreased quantity or deteriorated water quality, posing a threat to regional sustainable development (Bu et al., 2020; Meng and Wu, 2021; Wang et al., 2022).
Hence, one aspect of understanding the balance of a basin’s components is analyzing the synergy between land use and climate change effects. Combined, these can cause more pronounced effects, such as soil degradation, decreased water recharge, and increased surface runoff (Marhaento et al., 2018). Implementing a systemic approach that combines land use, water, biodiversity, and climate is essential to understanding a basin’s integrated functioning and its synergy with ecosystem services (Bao et al., 2024; Saik et al., 2024).
In Brazil, conflicts over multiple water uses intensified in the 1980s due to the land use and land cover changes, hydroelectric constructions, water pollution caused by the lack of domestic and industrial sewage treatment, and the irrigation demands of extensive agriculture and livestock farming (Schussel and Neto, 2015; Velastegui-Montoya et al., 2020). For those reasons, the National Water Resources Policy (PNRH - Law 9433/97) became necessary to regulate water resource management. It advocates integrated management considering landscape occupation, promotion of multiple water uses, encouragement of decentralized management, the necessity of planning at different levels, management instruments, and the creation of the National Water Resources Management System (SINGREH) (Leandro da Silva et al., 2021).
The unregulated use of water impacts soil degradation and the water recharge of river basins. In this regard, indices that involve the mediation of the basin’s environmental quality essential for evaluating water resource management, the state of degradation, and water governance. They also support policies aimed at environmental and water resource management (Ferreira S. C. G. et al., 2020). Thus, in a world where unsustainable water consumption is on the rise, tools that assist in decision-making and mitigation measures are necessary to ensure the rational use of water (Ferreira S. C. G. et al., 2020).
In this context, various indices have been created to aid the sustainability of water resources concerning soil degradation, priority areas for water recharge, and ecological indicators in river basins. Examples include the Water Poverty Index (WPI), the Canadian Water Sustainability Index (CWSI), the West Java Water Sustainability Index (WJWSI), and the Watershed Sustainability Index (WSI) developed by (Chaves and Alipaz, 2007), the latter being the most widely used and comprehensive for evaluating water sustainability (Silva et al., 2020; Da Silva et al., 2021; Branchi, 2022; Zare Bidaki et al., 2023).
The river basins in the Amazon region exhibit unique characteristics due to the complexity of the biome and the activities carried out within them, influenced by various historical contexts and marked by processes and conflicts. This has resulted in extensive areas experiencing soil degradation (Lapola et al., 2023). The northeastern mesoregion of Pará has a history of occupation initially characterized by vegetal extractivism at the onset of European colonization. However, the need to occupy the Amazon in response to other nations led to the development of agricultural production at a national significance level, as well as the construction of roads, which resulted in the emergence of urban centers (Cordeiro et al., 2017).
In this sense, the Maracanã River Basin (MRB), located in northeastern Pará, hosts various activities across the municipalities it encompasses. These include agriculture, mining, and oil palm cultivation, which can impact its function as a hydrological and geo-environmental unit, given its importance for biodiversity and the conservation of fluvial-marine ecosystems (FAPESPA, 2023). Therefore, integrated analysis can assist in evaluating the impacts of human activities carried out throughout the MRB, understanding the current state of conservation, and identifying essential areas for maintaining environmental quality.
The integrated analysis of basins allows for a systemic view of ecological processes. One study that employed a similar application was the Blueprint model in the Tapajós River basin, where it was found that the regions of greatest conservation were those within protected areas (PAs), as well as in the identification of priority areas for restoration (Petry et al., 2019). However, climatic variables such as rainfall distribution over the years were not used in the analysis, therefore representing a gap to be explored, aiming at an integrated and systemic approach (Petry et al., 2019).
Regarding the application of integrated analysis in the MRB, the closest was developed for the Marapanim River basin, where the WSI was applied (Da Silva et al., 2021). Other studies conducted in the Maracanã, Caripi, and Igarapé-Açú river basins analyzed the influence of land use on surface runoff, sustainability, and availability of water. These studies observed intermediate water sustainability, the threat to water availability, soil degradation, and strong surface runoff in the middle and upper course regions (Tamasauskas and Tamasauskas, 2016; Tamasauskas et al., 2016; Raiol et al., 2024). Therefore, there is a lack of studies that address an integrated and systemic analysis in MRB, and studies that can help understand the synergy of hydrology and environmental management of land use are important.
Given what has been said, it is essential to map the regions that present areas of degradation and higher conservation along the headwaters, main rivers, and tributaries, aiming to contribute to the sustainable planning of natural resources in the MRB. Therefore, the proposal was to assess the areas of environmental degradation and conservation in the MRB using the Blueprint model to assist in water resource management and environmental planning of the basin.
The MRB (Figure 1) is located in the northeast mesoregion of the state of Pará, in the coastal zone of the Amazon, with a total area of 3.093.26 km2. According to the State Water Resources Policy (Law No. 6.381/2001), it falls within the Northeast Atlantic Coastal Hydrographic Region (Pará, 2001; Pará, 2008), covering 14 municipalities: Maracanã, Igarapé-Açú, Santarém-Novo, Nova Timboteua, São Francisco do Pará, Castanhal, Santa Maria do Pará, São Miguel do Guamá, Bonito, Capanema, Peixe-Boi, Primavera, São João de Pirabas, and Salinópolis. Its main headwaters are in the municipalities of Castanhal, São Miguel do Guamá, and Santa Maria do Pará, and its mouth is in the bay of the municipality of Maracanã. In the lower course of the MRB, there are two protected areas (PAs), namely, the Extractive Reserve of Maracanã (Resex Maracanã), covering approximately 20.38% of its territory, and the Chocoaré - Mato Grosso RESEX, covering 60.34%.
The basin is characterized as fluvial-marine and has a climate in the megathermal humid category, type Am, according to the Köppen classification (Alvares et al., 2013). The average annual temperature ranges from 25°C to 27°C, and the average annual precipitation varies from 2,500 to 2,800 mm, with high intensity between the months of February to April (rainy season), and drought from September to November (dry period) (Alvares et al., 2013).
The methodology framework (Figure 2) consisted of using the Blueprint model, which acts as a structural and systematic planning tool developed by (Petry et al., 2019) for the Tapajós River basin (Brazil). This tool is supported by defining scenarios and indicators regarding the conservation status of natural resources, based on biological, hydrological, land use, and geomorphological information, through a perspective of aquatic and terrestrial environments (Petry et al., 2019). In this application of the Blueprint model, we consider rainfall data as an indicator to assist land use in the hydrological response of the MRB. Furthermore, we propose a new scale for the selection criteria of ecological units (EUs) and Blueprint classes.
The application of the Blueprint model to the MRB was based on the use of the drainage network obtained from (IBGE, 2021) through continuous cartographic databases at a scale of 1:250,000 (derived from the update of original digital databases with the Google Earth system and the Shuttle Radar Topography Mission - SRTM digital elevation model). The hydrographic basins were defined according to the Ottocodificadas basin system (ANA, 2017), adopting level 4 for the MRB. The analytical procedures were conducted using QGIS 3.34 software.
The land use and land cover (LULC) data from the TerraClass for 2020 (INPE, 2023) were used to define the predominant land occupation forms within the basin. The original classes (12) were reclassified (4) for the study area, aiming to meet the method’s application (Table 1), with the evaluation of the ecological units (EUs) according to their integrity, using spatial attributes of the activities carried out along the basin that affect the flow and connectivity of water bodies (Petry et al., 2019).
In the classification of EU, the systematization proposed by (Linke et al., 2019) was used, as defined in the digital database of HydroAtlas (level 12) (Available at Google Earth Engine: ee.FeatureCollection(“WWF/HydroATLAS/v1/Basins/level12”)), which generated 23 EUs. These units adhere to criteria of representativeness, uniqueness, functionality, and resilience, varying in size according to drainage areas (Petry et al., 2019).
The precipitation data were derived from WorldClim v1.4 for the period of 1950–2000, with a resolution of 30 arc-seconds (Linke et al., 2019). This long-term historical series enabled the definition of relative values for the basin, including annual and quarterly averages for both rainy and less rainy periods.
Monthly average rainfall data from ERA5-Land Monthly Aggregated - ECMWF Climate Reanalysis for the years 1950–2000 were used to understand the behavior of rainfall influence over the years on the basin (Muñoz Sabater, 2019). ERA5-Land Monthly Aggregated - ECMWF Climate Reanalysis data were converted within the GEE platform from meters to millimeters for each year’s accumulated sum. This dataset was chosen because the precipitation data from HydroAtlas derived from WorldClim v1.4 between 1950 and 2000 did not present a time series for the accumulated sum of each year.
Table 2 summarizes the criteria adopted to identify the relative conservation status according to the Blueprint method, with simulation under three rainfall volume conditions: annual average, average of the rainiest quarter, and average of the least rainy quarter. The units were reclassified according to the criteria and grouped by degree of similarity of response.
The calculation of the Blueprint (BP) (Equation 1) considered the reclassification criteria (Figure 3) presented in Table 2. Where BP corresponds to the Blueprint; and Xi are the variables used in the multiplication (Petry et al., 2019).
The final classification of the Blueprint (Table 3), based on the Natural Breaks (Jenks) classification method, defined 5 intervals resulting in the scale of environmental integrity, intermediate integrity, restoration and connectivity, degradation, and intense human impact.
Statistical analysis of the results was performed in the R environment (R Core Team, 2023), using the Shapiro-Wilk test (Table 4) to check the normality of the data (p-value < 0.05). Next, the Kruskal–Wallis test, as a non-parametric test, was employed to assess if there is a significant difference in the mean of at least one Blueprint (Rosier et al., 2023). Finally, the post hoc Dunn test was conducted to allow multiple comparisons to verify existing differences in each Blueprint (García-Galar et al., 2023).
In defining the clusters, the Ward method was adopted, which is based on a classic criterion of sum of squares, producing groups that minimize dispersion within groups in each binary fusion, while also seeking clusters in the multivariate Euclidean space (Murtagh and Legendre, 2014). The hierarchical cluster analysis was conducted in the Minitab 17 software, adopting the Euclidean squared distance as the linkage measure between the Annual Blueprint with the rainy and less rainy periods (Almeida et al., 2023). Spearman’s correlation (ggplot2 package) concluded the statistical validation. This ranges from −1 to 1, with values close to 1 indicating a strong positive correlation between the variables under analysis (de Queiroz et al., 2020; van den Heuvel and Zhan, 2022).
The precipitation in the MRB basin (Figure 4) showed an annual average of 2.517.13 ± 154.17 mm, with the highest values occurring from February to April (rainy quarter). The highest monthly averages were recorded in March with 482.10 ± 33.77 mm, April with 427.80 ± 62.07 mm, and February with 373.00 ± 25.47 mm, respectively. The lowest values in the basin were found from September to November (dry quarter), with the lowest monthly averages being 23.48 ± 5.98 mm in November, 29.39 ± 5.98 mm in October, and 45.57 ± 12.82 mm in September, respectively.
The results of the precipitation time series (Figure 5) indicated a negative trend in annual precipitation, with an average reduction of 7.6 mm/year (m = −7.6, p-value = 0.2, R2 = 0.03). However, this trend was not statistically significant, and only 3% of the precipitation variation could be explained by temporal variation, which indicates a strong influence of other factors, such as climate and environment.
The results of the Blueprints (Figure 6) showed that in the ABP, the degradation class predominates (34.51%), covering 106,740.97 ha, followed by the Intermediate Integrity class (27.73%) with 85,785.25 ha, Restoration and Connectivity (22.69%) with 70,182.35 ha, and Environmental Integrity (15.07%) with 46,617.41 ha (Table 5). In the RBP, the majority of the area is classified as Environmental Integrity (61.64%) with 190,675.32 ha and Intermediate Integrity (38.36%) with 118,650.66 ha. However, in the DBP, there was an increase in values, resulting in the majority of the classes being: Restoration and Connectivity (39.98%) with 123,671.55 ha, Degradation (34.51%) with 106,740.97 ha, and Intermediate Integrity (25.51%) with 78,913.46 ha.
Figure 6. Blueprints Classes for MRB. (A) Annual Blueprint (ABP). (B) Rainy Blueprint (RBP). (C) Dry Blueprint (DBP).
In this context, the analysis of the Blueprint during the annual average period and the average periods of the wet and dry quarters (Figure 7) showed significant differences in how the trends of conservation and degradation can vary at different times of the year, influenced by the rainfall regime.
Figure 7. Boxplot graph of the: Annual Blueprint (ABP), Rainy Blueprint (RBP) and Dry Blueprint (DBP).
The Kruskal–Wallis test results showed significant differences between the groups of ABP, RBP, and DBP (chi-squared = 29.395, df = 2, p-value < 0.05). The Dunn test indicated significant differences between ABP-RBP and DBP-RBP (p-adj < 0.05). These results suggest that the ABP provides a better distribution of values for understanding environmental conditions over a specific period of time (annual average) rather than on a seasonal (quarterly) scale.
The cluster analysis through the resulting dendrogram (Figures 8, 9) indicated a strong similarity between the two groups (1–9/7–16). This is due to the proximity of responses in most parts of the basin to the analyzed criteria, showing that the basin tends to respond similarly under climatic pressure, across the upper, middle, and lower courses of the basin. This similarity is especially evident in the behavior of the annual average precipitation and during the less rainy period.
The scenario observed in the middle-lower course of the basin is mainly influenced by the presence of primary vegetation and mangroves. The upper course of the basin differs, with a tendency toward degradation, due to the dominant forms of land use.
Figures 10–12 indicate a significant correlation of the Annual Blueprint (ABP) with the average annual precipitation (r = 0.98), ABP with natural vegetation cover (r = 0.90), and average annual precipitation with natural vegetation cover (r = 0.88) (p < 0.001). Other significant relationships were found between the ABP and agriculture (r = 0.59) and average annual precipitation with agriculture (r = 0.60) (p < 0.01), as well as natural vegetation cover with agriculture (r = 0.50; p < 0.05).
Figure 10. Correlation between land use variables, average annual precipitation, and ABP. Abbreviations: Natural Vegetation Cover (VC), Bare Soil (BS), Crop-Livestock (CL), Average Annual Rainfall (AAR), Annual BluePrint (ABP) (p-value: * significance at the 0.05 level, ** significance at the 0.01 level, ***significance at the 0.001 level).
Figure 11. Correlation between land use variables, average precipitation for the rainy trimester, and RBP. Abbreviations: Natural Vegetation Cover (VC), Bare Soil (BS), Crop-Livestock (CL), Average Trimester Rainfall Rainy (ARR), Rainy BluePrint (RBP) (p-value: * significance at the 0.05 level, ** significance at the 0.01 level, ***significance at the 0.001 level).
Figure 12. Correlation between land use variables, average precipitation for the dry trimester, and RBP. Abbreviations: Natural Vegetation Cover (VC), Bare Soil (BS), Crop-Livestock (CL), Average Trimester Rainfall Dry (ADR), Dry BluePrint (DBP) (p-value: * significance at the 0.05 level, ** significance at the 0.01 level, ***significance at the 0.001 level, NA no variation).
The relationship between land use and cover during the rainy season showed a significant correlation between RBP and the average rainfall for the rainy quarter (r = 0.82), RBP and natural vegetation cover (r = 0.89), and the average rainfall for the rainy quarter with natural vegetation cover (r = 0.73) (p < 0.001). Another significant correlation, albeit slightly less strong, was found between RBP and agriculture (r = 0.60; p < 0.01).
In the dry season, the most significant correlation was between DBP and natural vegetation cover (r = 0.90; p < 0.001), and DBP and agriculture (r = 0.61; p < 0.01). The correlation present in all three correlation matrices was between agriculture and natural vegetation cover (r = 0.50; p < 0.05). Although this correlation is lower compared to the others, it is still significant and can be explained by the spatial distribution, where areas closer to the mouth of the basin have higher natural vegetation cover and lower agricultural activity.
The trend in the seasonal variation of rainfall in the eastern Amazon highlights an increase in the frequency of dry days during the months of September to November (defined as the number of days per year with rainfall below 1 mm/day), particularly further south in the Amazon (p > 0.01). This increase is attributed to the weakening of Atlantic Ocean moisture, influenced by the South Atlantic Convergence Zone (SACZ), and the increase in subsidence (descent) of wind over the region between 10°S and 20°S (5°S–5°N). Additionally, there is a deficit of specific humidity in the 1,000–3,000 hPa range south of 10°S (Espinoza et al., 2019).
Several studies have identified negative trends in annual precipitation in various regions of the Amazon Basin, including the coastal basins in the eastern Amazon as found in this research. Haghtalab et al. (2020) found a significant drying trend in the eastern and southern regions of the Amazon Basin between 1982 and 2018, specifically, the Tocantins region in the eastern Amazon experienced an increase in the number of dry days during both wet and dry seasons, increasing by about 1 day per year. An analysis of long-term rainfall patterns from 1929 to 1998 identified a negative trend in annual precipitation for the entire Amazon Basin, with a more pronounced negative trend in northern Amazonia, linked to changes in sea surface temperatures (SST) and more frequent El Niño events (Marengo, 2004). This decrease in precipitation is highlighted in climate scenarios through the interpretation of the observed anomalies, being that the scenario estimates of Representative Concentration Pathway (RCP) 4.5 and 8.5, they point to a trend of increasing temperature (between 1°C and three°C) and reducing precipitation (between 5% and 20%) for the coastal municipalities of the state of Pará, which consequently affects the coastal basins in the region (Chou et al., 2014; Santos et al., 2021).
Another relationship also in the decrease in rainfall trends in the Amazon basin was observed from 1998 to 2015 and found that while most of the region showed no significant annual rainfall trends, 4.2% of the area exhibited significant negative trends, specifically, September, which marks the peak of the dry season (Silva Junior et al., 2018). This reduction in rainfall during the dry season could negatively impact water-dependent ecological processes and increase the risk of drought-related events such as wildfires (Silva Junior et al., 2018). A meta-analysis of regional and global climate model simulations indicated that deforestation in the Amazon basin has led to a reduction in annual mean rainfall and projected that continued deforestation could lead to an 8.1% reduction in annual mean rainfall by 2050 (Spracklen and Garcia-Carreras, 2015). This trend suggests a significant negative impact on rainfall due to deforestation (Spracklen and Garcia-Carreras, 2015).
The rainy season manifests with greater intensity in the northeastern region of the Amazon, a trend influenced by the stationary patterns of the South Atlantic Convergence Zone and the Intertropical Convergence Zone (ITCZ), peaking in the month of March (Nobre and Shukla, 1996; Cavalcante et al., 2020). It is also observed that rainy periods are associated with La Niña in the Pacific Ocean, as well as with the gradient mode of Sea Surface Temperature (SST) pointing southward in the Atlantic Ocean (anomalously warm SST in the southern basin and cold in the northern basin), configurations that intensify ITCZ events in the region and give rise to heavy rainfall extremes in the state of Pará (Souza et al., 2000; Ferreira D. B. et al., 2020).
Large-scale meteorological systems (ITCZ and SACZ) and smaller-scale systems (instability lines, convective clusters, maritime and river breezes) can act in a specific region in the state of Pará, resulting in rainy climatic extremes in any quarter of the year (Cohen et al., 1995; Fisch et al., 1998; Ferreira D. B. et al., 2020). The interrelationship between variability modes and atmospheric systems is direct, as systems such as the ITCZ and SACZ are weakened or intensified due to the manifestation of the El Niño-Southern Oscillation (ENSO) and/or the meridional gradient of SST anomalies (Ferreira D. B. et al., 2020).
Precipitation plays a crucial role in the classes found in the Blueprints, where it was observed that only during the rainy season does it predominantly exhibit low values, indicating classes of environmental and intermediate integrity. In the remaining annual and less rainy periods, precipitation values are higher, denoting more vulnerable hydrological units. During the rainy season, annual precipitation has a positive relationship with plant growth and above-ground biomass, while the dry season influences this dynamic, affecting the carbon cycle and forest regeneration, especially in tropical forests (Becknell et al., 2021).
The reduction in precipitation, particularly in a scenario of more intense and prolonged droughts over time, can influence forest regeneration and biomass formation, affecting biodiversity. This decrease may occur in both pessimistic and optimistic scenarios, being more significant in the pessimistic scenario, with a reduction of 160 mm/year every 20 years until 2,100, on the Amazonian Atlantic coast, which could alter the structures and functioning of ecosystems (Anjos et al., 2021).
The results obtained from the classes of environmental integrity and intermediate integrity in the annual, rainy, and less rainy periods highlight the large portion of vegetative cover in the lower course region. The PAs, represented by mangrove forests along the Maracanã River, are relevant for gene flow in species, serving as ecological corridors (Torres-Amaral et al., 2023).
The influence of vegetative cover on agriculture, with the incorporation of legumes and agroforestry systems, plays an important role in improving soil biological health, increasing microbial community abundance, biomass, and ecological structure, compared to no vegetative cover cropping in agricultural activities (Rego and Kato, 2018; Muhammad et al., 2021).
Vegetative cover has been shown to be a determining factor in environmental conservation conditions in the Blueprint model, which, according to the method proposed by (Petry et al., 2019), aims at ecological maintenance, with the preservation of forest species and combating biodiversity loss (Brandão et al., 2022). The results in both periods (rainy and less rainy) are essential for understanding these changes and dynamics in the behavior of land use and land cover classes in the dry and rainy seasons, emphasizing the importance of maintaining vegetative cover for the hydrological cycle at these seasonal scales, as these are periods that highlight vegetation resilience, especially in the less rainy period.
Precipitation in all three periods showed a significant correlation with land use and land cover classes, especially with natural vegetative cover, emphasizing the importance of rainfall in these classes, as it is an essential component of the hydrological cycle, aiding in recharge and water balance (Serrão et al., 2022). Natural vegetative cover also plays a crucial role in reducing the loss of fine soil particles and increasing the content of silt, clay, and organic matter, thus preventing soil erosion (Abolmaali et al., 2024; Zhu et al., 2024).
The obtained results can aid in the spatial understanding of areas experiencing water deficit and degradation, particularly in the southern region of the basin, which exhibits greater heterogeneity in land use classes, impacting the hydrological cycle (water recharge, runoff, and evapotranspiration) more intensely on an annual and seasonal scale. Therefore, there is a need for more significant maintenance of vegetative cover areas, as well as the implementation and strengthening of existing environmental and water management policies.
This application of the Blueprint model in MRB is an adaptation of the original methodology proposed by Petry et al. (2019), considering the average distribution of precipitation on an annual and seasonal scale, as the representation of land use and rainfall are indicators of hydrological response. Therefore, this adapted methodology presents this gap of having considered only two variables: land use and land cover and precipitation, reinforcing above all the development of exploratory and analytical work of the data in situ in applications of this methodology in the future. Another factor that compromised the results is the lack of data from hydrometeorological stations in the MRB.
Other applications of this methodology adapted from the Blueprint model are suggested, considering other hydrometeorological and geomorphological variables, as well as in situ data, making the model more robust and improving the accuracy. The Imagery use of high resolution can improve the results by the classification more detailed. Another approach is to compare results applied in other basins worldwide, also comparing the temporal applicability of land use changes, since over the years these scenarios of soil conservation and degradation can alter the EUs.
The MRB exhibited a rainfall distribution with a less rainy period from September to November and a rainy period between February and April. The basin predominantly showed the “Degradation” class in ABP and the “Restoration and Connectivity” class in DBP. On the other hand, in RBP, there was a predominance of “Environmental Integrity”, emphasizing the importance of rainfall in the Blueprint model as an ecological indicator and for ecosystem restoration in the basin. In the correlation analyses, it was possible to verify that in the Blueprint model, the natural vegetation cover class showed a significant correlation with all variables, playing a fundamental role in the ecosystem services of the MRB.
The main contribution of the research was to discuss a methodology applied to the decision-making process aimed at conservation practices in the watershed environment. The influence of rainfall precipitation was tested on both annual and seasonal scales, highlighting the importance of climate monitoring coupled with hydrological monitoring as indicators of watershed hydrodynamics and its capacity for recovery in extreme weather events.
In the MRB, as in other coastal basins, areas of degradation occur in regions most sensitive to water recharge, indicating the need for actions to conserve areas prioritized for environmental quality. It is important to note the limitation of land use and lack of available precipitation data from hydrometeorological stations in the basin. Therefore, there is a need to enhance the Blueprint model using other variables that may better respond to a more in-depth integrated analysis, suggesting more robust results.
The original contributions presented in the study are included in the article/supplementary material, further inquiries can be directed to the corresponding authors.
LR: Conceptualization, Data curation, Formal Analysis, Investigation, Methodology, Software, Validation, Visualization, Writing–original draft, Writing–review and editing. YR: Data curation, Formal Analysis, Software, Validation, Writing–review and editing. AL: Conceptualization, Data curation, Formal Analysis, Investigation, Methodology, Resources, Software, Supervision, Validation, Visualization, Writing–original draft, Writing–review and editing. AV-M: Conceptualization, Data curation, Formal Analysis, Investigation, Methodology, Software, Supervision, Validation, Visualization, Writing–review and editing.
The author(s) declare that financial support was received for the research, authorship, and/or publication of this article. This contribution is part of the master thesis of the Environmental Sciences Program (PPGCA) from the Federal University of Pará, with a scholarship from Coordenação de Aperfeiçoamento de Pessoal de Nível Superior - Brazil (CAPES), which financed this study under Finance Code 001. This study was supported by the research project of the ESPOL University “ILCA: Impact of Land Cover change in the Amazon” with the code no. FICT-7-2023.
The authors declare that the research was conducted in the absence of any commercial or financial relationships that could be construed as a potential conflict of interest.
The author(s) declare that no Generative AI was used in the creation of this manuscript.
All claims expressed in this article are solely those of the authors and do not necessarily represent those of their affiliated organizations, or those of the publisher, the editors and the reviewers. Any product that may be evaluated in this article, or claim that may be made by its manufacturer, is not guaranteed or endorsed by the publisher.
Abolmaali, S. M., Tarkesh, M., Mousavi, S. A., Karimzadeh, H., Pourmanafi, S., and Fakheran, S. (2024). Identifying priority areas for conservation: using ecosystem services hotspot mapping for land-use/land-cover planning in central of Iran. Environ. Manage 73, 1016–1031. doi:10.1007/s00267-024-01944-y
Almeida, A. K., de Almeida, I. K., Guarienti, J. A., Finck, L. F., and Gabas, S. G. (2023). Time of concentration model for non-urban tropical basins based on physiographic characteristics and observed rainfall responses. Water Resour. Manag. 37, 5493–5534. doi:10.1007/s11269-023-03616-8
Alvares, C. A., Stape, J. L., Sentelhas, P. C., de Moraes Gonalves, J. L., and Sparovek, G. (2013). Köppen’s climate classification map for Brazil. Meteorol. Z. 22, 711–728. doi:10.1127/0941?2948/2013/0507
ANA (2017). Agência Nacional de Águas. Base Hidrográfica Ottocodificada Multiescalas 2017. Available at: https://metadados.snirh.gov.br/geonetwork/srv/por/catalog.search#/metadata/0c698205-6b59-48dc-8b5e-a58a5dfcc989 (Accessed August 7, 2022).
Anjos, L. J. S., Barreiros de Souza, E., Amaral, C. T., Igawa, T. K., and Mann de Toledo, P. (2021). Future projections for terrestrial biomes indicate widespread warming and moisture reduction in forests up to 2100 in South America. Glob. Ecol. Conserv. 25, e01441. doi:10.1016/j.gecco.2020.e01441
Bao, J., Li, W., Zhu, J., Fan, S., and Mao, L. (2024). Impacts of land use structures on ecosystem services relationships within specialized tea planting regions: a case study of Anxi County. Front. Environ. Sci. 12. doi:10.3389/fenvs.2024.1464490
Becknell, J. M., Vargas, G. G., Pérez-Aviles, D., Medvigy, D., and Powers, J. S. (2021). Above-ground net primary productivity in regenerating seasonally dry tropical forest: contributions of rainfall, forest age and soil. J. Ecol. 109, 3903–3915. doi:10.1111/1365-2745.13767
Branchi, B. A. (2022). Sustentabilidade de Bacias Hidrográficas e Índices Compostos: Aplicação e Desafios. Sociedade and Natureza 34. doi:10.14393/sn-v34-2022-63868
Brandão, D. O., Barata, L. E. S., and Nobre, C. A. (2022). The effects of environmental changes on plant species and forest dependent communities in the Amazon region. Forests 13, 466. doi:10.3390/f13030466
Bu, J., Li, C., Wang, X., Zhang, Y., and Yang, Z. (2020). Assessment and prediction of the water ecological carrying capacity in Changzhou city, China. J. Clean. Prod. 277, 123988. doi:10.1016/j.jclepro.2020.123988
Cavalcante, R. B. L., Ferreira, D. B., Pontes, P. R. M., Tedeschi, R. G., da Costa, C. P. W., and de Souza, E. B. (2020). Evaluation of extreme rainfall indices from CHIRPS precipitation estimates over the Brazilian Amazonia. Atmos. Res. 238, 104879. doi:10.1016/j.atmosres.2020.104879
Chaves, H. M. L., and Alipaz, S. (2007). An integrated indicator based on basin hydrology, environment, life, and policy: the watershed sustainability index. Water Resour. Manag. 21, 883–895. doi:10.1007/s11269-006-9107-2
Chou, S. C., Lyra, A., Mourão, C., Dereczynski, C., Pilotto, I., Gomes, J., et al. (2014). Assessment of climate change over south America under RCP 4.5 and 8.5 downscaling scenarios. Am. J. Clim. Change 03, 512–527. doi:10.4236/ajcc.2014.35043
Cohen, J. C. P., Silva Dias, M. A. F., and Nobre, C. A. (1995). Environmental conditions associated with amazonian squall lines: a case study. Mon. Weather Rev. 123, 3163–3174. doi:10.1175/1520-0493(1995)123<3163:ECAWAS>2.0.CO;2
Cordeiro, I., Arbage, M., and Schwartz, G. (2017). “Nordeste Paraense: Configuração Atual e Aspectos Identitários,” in Nordeste Paraense: Panorama geral e uso sustentável das florestas secundárias (Belém, Pará), 19–58.
Cosgrove, W. J., and Loucks, D. P. (2015). Water management: current and future challenges and research directions. Water Resour. Res. 51, 4823–4839. doi:10.1002/2014WR016869
Da Silva, J. C. C., De Lima, A. M. M., De Holanda, B. S., Da Silva De Andrade Moreira, F., and Da Costa Cavalcante, J. (2021). Índice de sustentabilidade nas sedes municipais da bacia hidrográfica do rio Marapanim (Pará/Brasil). Rev. Gestao Ambient. Sustentabilidade 10, e18300. doi:10.5585/geas.v10i1.18300
de Queiroz, M. G., da Silva, T. G. F., Zolnier, S., Jardim, A. M. da R. F., de Souza, C. A. A., Araújo Júnior, G. do N., et al. (2020). Spatial and temporal dynamics of soil moisture for surfaces with a change in land use in the semi-arid region of Brazil. Catena Amst 188, 104457. doi:10.1016/j.catena.2020.104457
Espinoza, J. C., Ronchail, J., Marengo, J. A., and Segura, H. (2019). Contrasting North–South changes in Amazon wet-day and dry-day frequency and related atmospheric features (1981–2017). Clim. Dyn. 52, 5413–5430. doi:10.1007/s00382-018-4462-2
FAPESPA (2023). Fundação Amazônia de Amparo a Estudos e Pesquisas. Anuário estatístico do Pará 2023. Available at: https://fapespa.pa.gov.br/sistemas/anuario2023/(Accessed January 12, 2024).
Fassoni-Andrade, A. C., Fleischmann, A. S., Papa, F., Paiva, R. C. D., Wongchuig, S., Melack, J. M., et al. (2021). Amazon hydrology from space: scientific advances and future challenges. Rev. Geophys. 59. doi:10.1029/2020RG000728
Ferreira, D. B., Souza, E. B., and Oliveira, J. V. de (2020a). Identificação de extremos de precipitação em municípios do Estado do Pará e sua relação com os modos climáticos atuantes nos oceanos Pacífico e Atlântico. Rev. Bras. Climatol. 27, 197–222. doi:10.5380/abclima.v27i0.64630
Ferreira, S. C. G., de Lima, A. M. M., and Corrêa, J. A. M. (2020b). Indicators of hydrological sustainability, governance and water resource regulation in the Moju river basin (PA) – eastern Amazonia. J. Environ. Manage 263, 110354. doi:10.1016/j.jenvman.2020.110354
Fisch, G., Marengo, J. A., and Nobre, C. A. (1998). Uma revisão geral sobre o clima da Amazônia. Acta Amaz. 28, 101. doi:10.1590/1809-43921998282126
García-Galar, A., Lamelas, M. T., and Domingo, D. (2023). Assessment of oak groves conservation statuses in natura 2000 sacs with single photon lidar and sentinel-2 data. Remote Sens. Basel 15, 710. doi:10.3390/rs15030710
Gomes, R. C., Bianchi, C., and Oliveira, V. P. V. (2021). Análise da multidimensionalidade dos conceitos de bacia hidrográfica. GEOgraphia 23. doi:10.22409/geographia2021.v23i51.a27667
Haghtalab, N., Moore, N., Heerspink, B. P., and Hyndman, D. W. (2020). Evaluating spatial patterns in precipitation trends across the Amazon basin driven by land cover and global scale forcings. Theor. Appl. Climatol. 140, 411–427. doi:10.1007/s00704-019-03085-3
IBGE (2021). Instituto Brasileiro de Geografia e Estatística. Geociências. IBGE. Bases Cartográficas continuas. Available at: https://www.ibge.gov.br/geociencias/downloads-geociencias.html?caminho=cartas_e_mapas/bases_cartograficas_continuas/bc250/versao2021/ (Accessed August 7, 2022).
INPE (2023). Instituto Nacional de Pesquisas Espaciais. Bioma Amazônia-TerraClass. TerraBrasilis catalogue. Available at: http://terrabrasilis.dpi.inpe.br/geonetwork/srv/eng/catalog.search#/metadata/f49a47f8-12ae-4052-8e0d-2e581458dc69/ (Accessed June 30, 2023).
Katusiime, J., and Schütt, B. (2020). Linking land tenure and integrated watershed management-A review. Sustain. Switz. 12, 1667. doi:10.3390/su12041667
Lapola, D. M., Pinho, P., Barlow, J., Aragão, L. E. O. C., Berenguer, E., Carmenta, R., et al. (2023). The drivers and impacts of Amazon forest degradation. Science 1979, eabp8622. doi:10.1126/science.abp8622
Leandro da Silva, F., Terumi Fushita, Â., Bianchessi da Cunha -Santino, M., Bianchini Júnior, I., and Carlos Toledo Veneziani Júnior, J. (2021). Revista Brasileira de Geografia Física Gestão de recursos hídricos e manejo de bacias hidrográficas no Brasil: elementos básicos, histórico e estratégias, 1626–1653.
Linke, S., Lehner, B., Ouellet Dallaire, C., Ariwi, J., Grill, G., Anand, M., et al. (2019). Global hydro-environmental sub-basin and river reach characteristics at high spatial resolution. Sci. Data 6, 283. doi:10.1038/s41597-019-0300-6
Marengo, J. A. (2004). Interdecadal variability and trends of rainfall across the Amazon basin. Theor. Appl. Climatol. 78. doi:10.1007/s00704-004-0045-8
Marhaento, H., Booij, M. J., and Hoekstra, A. Y. (2018). Hydrological response to future land-use change and climate change in a tropical catchment. Hydrological Sci. J. 63, 1368–1385. doi:10.1080/02626667.2018.1511054
Mello, K. de, Taniwaki, R. H., Paula, F. R. de, Valente, R. A., Randhir, T. O., Macedo, D. R., et al. (2020). Multiscale land use impacts on water quality: assessment, planning, and future perspectives in Brazil. J. Environ. Manage 270, 110879. doi:10.1016/j.jenvman.2020.110879
Meng, X., and Wu, L. (2021). Prediction of per capita water consumption for 31 regions in China. Environ. Sci. Pollut. Res. 28, 29253–29264. doi:10.1007/s11356-021-12368-0
Muhammad, I., Wang, J., Sainju, U. M., Zhang, S., Zhao, F., and Khan, A. (2021). Cover cropping enhances soil microbial biomass and affects microbial community structure: a meta-analysis. Geoderma 381, 114696. doi:10.1016/j.geoderma.2020.114696
Muñoz Sabater, J. (2019). ERA5-Land monthly averaged data from 1981 to present. Copernic. Clim. Change Serv. C3S Clim. Data Store CDS. doi:10.24381/cds.68d2bb30
Murtagh, F., and Legendre, P. (2014). Ward’s hierarchical agglomerative clustering method: which algorithms implement ward’s criterion? J. Classif. 31, 274–295. doi:10.1007/s00357-014-9161-z
Nobre, P., and Shukla, J. (1996). Variations of Sea Surface temperature, wind stress, and rainfall over the tropical atlantic and south America. J. Clim. 9, 2464–2479. doi:10.1175/1520-0442(1996)009<2464:VOSSTW>2.0.CO;2
Pará (2001). Lei Ordinária no 6.381, de 25 de julho de 2001. Dispõe sobre política estadual Recur. hídricos, institui Sist. gerenciamento Recur. hídricos dá outras Provid. Available at: https://www.semas.pa.gov.br/legislacao/files/pdf/517.pdf (Accessed January 31, 2024).
Pará (2008). Resolução Conselho Estadual de Recursos Hídricos (CERH) No 004, de 03 de Setembro de 2008. Dispõe sobre Div. do estado em regiões hidrográficas dá outras Provid. Available at: https://www.semas.pa.gov.br/wp-content/uploads/2012/09/Resolu%C3%A7%C3%A3o-CERH-n%C2%BA-04-Disp%C3%B5e-sobre-a-divis%C3%A3o-do-estado-em-regi%C3%B5es-hidrogr%C3%A1ficas.pdf (Accessed March 31, 2024).
Petry, P., Higgins, J., Carneiro, A., Rodrigues, S., Harrison, D., Bara, P., et al. (2019) “Conservação da Bacia do Tapajós: Uma Visão de Sustentabilidade,” São Paulo/SP: The Nature Conservancy.
Raiol, L. L., Velastegui-Montoya, A., and Lima, A. M. M. (2024). “Water availability of the Maracanã River watershed, eastern Amazon,” in IGARSS 2024 - 2024 IEEE International Geoscience and Remote Sensing Symposium, Athens, Greece, 07-12 July 2024 (IEEE), 2090–2093. doi:10.1109/IGARSS53475.2024.10641539
R Core Team (2023). R: a language and environment for statistical computing. R Foundation for Statistical Computing, Vienna, Austria. Available at: https://www.R-project.org/) (Accessed ) (Accessed June 5, 2024).
Rego, A. K. C., and Kato, O. R. (2018). Agricultura de corte e queima e alternativas agroecológicas na Amazônia. Novos Cad. NAEA 20. doi:10.5801/ncn.v20i3.3482
Rosier, I., Diels, J., Somers, B., and Van Orshoven, J. (2023). The impact of vegetated landscape elements on runoff in a small agricultural watershed: a modelling study. J. Hydrol. Amst 617, 129144. doi:10.1016/j.jhydrol.2023.129144
Saik, P., Koshkalda, I., Bezuhla, L., Stoiko, N., and Riasnianska, A. (2024). Achieving land degradation neutrality: land-use planning and ecosystem approach. Front. Environ. Sci. 12. doi:10.3389/fenvs.2024.1446056
Santos, M. R. da S., Vitorino, M. I., Pereira, L. C. C., Pimentel, M. A. da S., and Quintão, A. F. (2021). Socioenvironmental vulnerability to climate change: conditions of coastal municipalities in Pará state. Ambiente and Sociedade 24. doi:10.1590/1809-4422asoc20200167r1vu2021l3ao
Schussel, Z., and Neto, P. N. (2015). Gestão por bacias hidrográficas: do debate teórico à gestão municipal. Ambiente and Sociedade 18, 137–152. doi:10.1590/1809-4422asoc838v1832015
Serrão, E. A. O., Silva, M. T., Ferreira, T. R., Paiva de Ataide, L. C., Assis dos Santos, C., Lima, A. M. M., et al. (2022). Impacts of land use and land cover changes on hydrological processes and sediment yield determined using the SWAT model. Int. J. Sediment Res. 37, 54–69. doi:10.1016/j.ijsrc.2021.04.002
Silva, J., Fernandes, V., Limont, M., Dziedzic, M., Andreoli, C. V., and Rauen, W. B. (2020). Water sustainability assessment from the perspective of sustainable development capitals: conceptual model and index based on literature review. J. Environ. Manage 254, 109750. doi:10.1016/j.jenvman.2019.109750
Silva Junior, C. H. L., Almeida, C. T., Santos, J. R. N., Anderson, L. O., Aragão, L. E. O. C., and Silva, F. B. (2018). Spatiotemporal rainfall trends in the Brazilian legal Amazon between the years 1998 and 2015. Water (Basel) 10, 1220. doi:10.3390/w10091220
Souza, E. B. de, Kayano, M. T., Tota, J., Pezzi, L., Fisch, G., and Nobre, C. (2000). On the influences of the El Niño, La niña and Atlantic Dipole Paterni on the Amazonian rainfall during 1960-1998. Acta Amaz. 30, 305–318. doi:10.1590/1809-43922000302318
Spracklen, D. V., and Garcia-Carreras, L. (2015). The impact of Amazonian deforestation on Amazon basin rainfall. Geophys Res. Lett. 42, 9546–9552. doi:10.1002/2015GL066063
Tamasauskas, C. E., and Tamasauskas, P. (2016). Mudanças de uso e cobertura da terra e escoamento superficial na bacia hidrográfica do rio caripi-pa: uma análise a partir das geotecnologias/changes in land use and cover and runoff in the caripi-pa river basin: an analysis based on geotechnologies. Geoamazônia 4, 153–173. doi:10.18542/geo.v4i08.12489
Tamasauskas, P., Souza, L., Lima, A., Pimentel, M., and Rocha, E. (2016). Métodos de avaliação da influência das áreas ripárias na sustentabilidade hidrológica em bacias hidrográficas no nordeste do estado do Pará. Cad. Geogr. 45. doi:10.5752/p.2318-2962.2016v26n.45p.172
Torres-Amaral, C., Anjos, L. J. S., Vieira, I. C. G., and de Souza, E. B. (2023). The climatic risk of Amazonian protected areas is driven by climate velocity until 2050. PLoS One 18, e0286457. doi:10.1371/journal.pone.0286457
van den Heuvel, E., and Zhan, Z. (2022). Myths about linear and Monotonic Associations: Pearson’s r, Spearman’s ρ, and Kendall’s τ. Am. Stat. 76, 44–52. doi:10.1080/00031305.2021.2004922
Velastegui-Montoya, A., De Lima, A., and Adami, M. (2020). Multitemporal analysis of deforestation in response to the construction of the tucuruí dam. ISPRS Int. J. Geoinf 9, 583. doi:10.3390/ijgi9100583
Wang, G., Mang, S., Cai, H., Liu, S., Zhang, Z., Wang, L., et al. (2016). Integrated watershed management: evolution, development and emerging trends. J Res (Harbin) 27, 967–994. doi:10.1007/s11676-016-0293-3
Wang, X., Zhang, S., Tang, X., and Gao, C. (2022). Research on water resources environmental carrying capacity (WRECC) based on support-pressure coupling theory: a case study of the Guangdong-Hong Kong-Macao Greater Bay Area. J. Environ. Manage 320, 115805. doi:10.1016/j.jenvman.2022.115805
Zare Bidaki, R., Esmaeilzadeh, M., and Chaves, H. M. L. (2023). Assessing watershed sustainability with automatic expert-based methods and managers’ preferences. Sustain Water Resour. Manag. 9, 70. doi:10.1007/s40899-023-00847-w
Keywords: land use, rainfall, blueprint model, ecological indicator, Amazon
Citation: Raiol LL, Rocha YAdS, Lima AMMd and Velastegui-Montoya A (2025) Assessment of environmental degradation and conservation in the Maracanã River Basin, eastern amazon. Front. Environ. Sci. 12:1506701. doi: 10.3389/fenvs.2024.1506701
Received: 05 October 2024; Accepted: 09 December 2024;
Published: 03 January 2025.
Edited by:
Shuisen Chen, Guangzhou Institute of Geography, ChinaReviewed by:
Rina Kumari, Central University of Gujarat, IndiaCopyright © 2025 Raiol, Rocha, Lima and Velastegui-Montoya. This is an open-access article distributed under the terms of the Creative Commons Attribution License (CC BY). The use, distribution or reproduction in other forums is permitted, provided the original author(s) and the copyright owner(s) are credited and that the original publication in this journal is cited, in accordance with accepted academic practice. No use, distribution or reproduction is permitted which does not comply with these terms.
*Correspondence: Lucas Lima Raiol, bHVjYXMucmFpb2xAaWcudWZwYS5icg==; Andrés Velastegui-Montoya, ZHZlbGFzdGVAZXNwb2wuZWR1LmVj
Disclaimer: All claims expressed in this article are solely those of the authors and do not necessarily represent those of their affiliated organizations, or those of the publisher, the editors and the reviewers. Any product that may be evaluated in this article or claim that may be made by its manufacturer is not guaranteed or endorsed by the publisher.
Research integrity at Frontiers
Learn more about the work of our research integrity team to safeguard the quality of each article we publish.