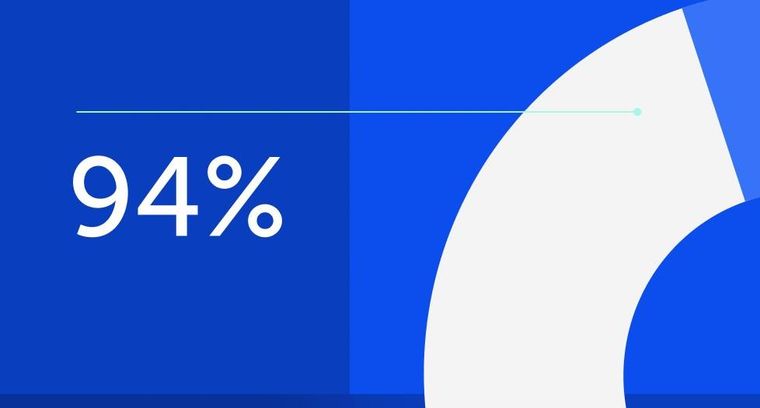
94% of researchers rate our articles as excellent or good
Learn more about the work of our research integrity team to safeguard the quality of each article we publish.
Find out more
ORIGINAL RESEARCH article
Front. Environ. Sci., 28 November 2024
Sec. Soil Processes
Volume 12 - 2024 | https://doi.org/10.3389/fenvs.2024.1502902
Phosphorus (P) is a key nutrient for crop growth and yield. The use of drip irrigation systems to apply phosphate fertilizer may enhance the migration distance and improve usage rates. However, emitter clogging poses a substantial challenge to phosphorus drip irrigation systems. In this study, we evaluated the influences of the phosphorus fertilizer type on soil-available phosphorus (P), plant growth, yield, and drip emitter clogging. Experiments were conducted in both seasons using different phosphorus fertilizers, including urea phosphate (UP, F1), monoammonium phosphate (MAP, F2), and a combination of urea phosphate and monoammonium phosphate (UP + MAP, F3). A treatment without phosphate fertigation (F0) was used as the control. Applying phosphorus fertilizers enhanced soil-available P content, increased plant height, and improved the leaf area index (LAI) to a larger extent than those without P fertilizer. This promoted photosynthesis and increased the dry matter mass, plant P and N uptake, and aboveground plant biomass. The maximum yield of 14,764.2 and 14,778.2 kg ha−1 was obtained under urea phosphate (F1) and monoammonium phosphate (F2) in 2022 and 2023 seasons, respectively. Phosphorus fertilization changes the composition and diversity of bacterial and fungal communities in the emitter biofilm. Urea phosphate and monoammonium phosphate increased the abundance of Proteobacteria and decreased the abundance of Acidobacteriota. Acidobacteriota was strongly positively correlated with extracellular protein, whereas Proteobacteria was negatively correlated with extracellular polysaccharides, extracellular protein, and extracellular polymer. The content of extracellular polymer and solid particles decreased by 30.1%–42.0% and 39.8%–79.7%, respectively, inducing a higher relative emitter discharge for the treatment with phosphorus fertilizers. Acidic phosphorus fertilizers, such as urea phosphate and monoammonium phosphate, can maintain the high performance of drip irrigation and produce high crop yield.
Phosphorus is a major nutrient-limiting factor for crop growth. Its availability affects the productivity of 30%–40% of the arable land in the world (Atere et al., 2019). A substantial amount of the phosphorus (P) fertilizer is added to agricultural soil to address soil phosphorus shortages and encourage high crop yields (Roy et al., 2017). Numerous studies have evaluated the influence of P fertilizer levels on crops and soil phosphorus; however, information on the effects of different types of P fertilizers on agricultural yields is limited (Sucunza et al., 2018; Liang et al., 2024). Fertilizers containing phosphorus can reduce the absorption and fixation capacity of soil for phosphorus, thereby improving the available phosphorus content in the soil (Vu et al., 2008). Different phosphorus fertilizers directly affect the migration, transformation, and distribution of phosphorus, owing to their composition and morphological differences, ultimately affecting the phosphorus absorption and yield of crops. The application of triple superphosphate (TSP) at an equivalent P rate considerably enhanced the number of leaves, leaf area, and plant height of different plants compared to monoammonium phosphate (MAP) (Chien et al., 1987). Zhang et al. (2019) investigated the effects of varying phosphorus types on soil-accessible phosphorus content, phosphorus accumulation in maize, phosphorus utilization, and yield; ammonium polyphosphate (APP) yielded better results than urea phosphate (UP), which yielded better than MAP. Liang et al. (2024) found that calcium superphosphate, ammonium polyphosphate, and MAP were more suitable for the alkaline soil environment.
The phosphorus utilization rate per season is only 10%–25% because the phosphate fertilizer is mainly applied via base application (Roberts and Johnston, 2015; Fan et al., 2020). Recent research has shown that using drip irrigation systems to apply phosphate fertilizers may increase the migration distance of phosphate fertilizers and improve the utilization rate (Liu et al., 2017; Chtouki et al., 2022). Drip irrigation provides remarkable benefits in terms of accuracy and effectiveness by delivering water-soluble P fertilizers to crop root zones through enclosed irrigation lines. The development of various water-soluble P fertilizers, including diammonium phosphate, MAP, and ammonium polyphosphate, coincides with the maturity of water fertilizer integration technologies. Cotton grown under film drip irrigation is frequently fertilized with water-soluble fertilizers, such as UP and ammonium polyphosphate, in Xinjiang. These fertilizers are superior to soluble ammonium phosphate in improving cotton quality and growth (Zhang et al., 2019; Ji et al., 2021).
However, the potential impact of emitter blocking on system performance continues to be a barrier to the wider application of P fertigation. Generally, potassium fertilizers have a lower risk of emitter clogging, whereas nitrogen and phosphate fertilizers present a higher risk. Phosphorous research has shown that previous fertilization considerably worsens the degree of clogging in drip emitters, including phosphate acid (Mikkelsen, 1989), diammonium phosphate, and ammonium dihydrogen phosphate (Li et al., 2015). However, when strongly acidic P fertilizers are delivered, H+ likely prevents carbonate and phosphate precipitation (Hao et al., 2018; Ma et al., 2020). Using acidic P fertilizers can mitigate emitter blocking, even in systems with saline water that have high concentrations of ions such as Ca2+, Mg2+, and HCO3− (Wang et al., 2020; Xiao et al., 2020).
Farmers frequently use compound fertilizers (Brownlie et al., 2021). A sensible fertilizer mix may fulfill the nutritional needs of various crops at different stages of growth, thereby reducing fertilizer costs and decreasing fertilization frequency (Chen et al., 2021). Fertilizer variety is one of the main variables for developing emitter plugging features under P fertigation (Mikkelsen, 1989; Xiao et al., 2020; Muhammad et al., 2021). According to Ma et al. (2020), UP and ammonium polyphosphate (APP) successfully reduced emitter blockage; however, monopotassium phosphate enhanced it. Similarly, it has been shown that phosphorus fertigation with acidic fertilizers such as UP and MAP can reduce emitter blockage (Wang et al., 2020). Nevertheless, according to certain research, applying acidic fertilizers, such as UP and APP, increases the risk of emitter clogging (Xiao et al., 2020; Shen et al., 2022). Currently, it is generally believed that acid–phosphate fertilizers prevent clogging; however, research on various water sources has shown that this is not always the case. Therefore, more research is required to understand the regulation and processes of emitter clogging using NP compound fertilizers. Furthermore, the prolonged use of extremely acidic P fertilizers may further lower soil pH, endangering crop development (Mikkelsen, 1989). It is possible to achieve a balance among crop safety, soil health, and drip irrigation systems using acidic P fertilizers with appropriate pH values based on the irrigation water quality. Further research is required on this as various areas and crop types have diverse phosphorus application strategies.
The selection of an appropriate phosphate fertilizer variety is an essential prerequisite for the use of phosphorus fertilizers in irrigation and fertilization. Soil-available phosphorus is closely related to phosphorus fertilizer varieties. Reasonable phosphorus application can optimize crop yield components, thereby increasing yield and phosphorus fertilizer utilization efficiency. Therefore, studying the effects of phosphorus fertilizer varieties on soil phosphorus availability, crop growth, and the drip irrigation system is a valuable endeavor that can contribute to the rational use of phosphorus fertilizer. The objectives of the present study were to 1) investigate the effects of phosphorus fertilizers on the change in the soil-available phosphorus content; 2) evaluate the implications of N-containing P fertilizers on maize growth, maize nitrogen/phosphorus uptake, and yield; and 3) clarify the effects of different P fertilizers on emitter blockage. A guide for effective phosphorus fertilizer selection for drip irrigation after the application of wastewater effluent is provided.
The experiments were conducted at Shanxi Agricultural University in Taigu, Shanxi Province, China (37°25′N, 112°36′E). The experimental region is semi-arid, with an annual mean precipitation of 440 mm. The experimental soil was classified as clay loam (Shirazi and Boersma, 1984), with a total nitrogen content of 0.730 g kg–1, an effective phosphorus content of 12.55 mg kg–1, and a pH of 8.19. The average bulk density was 1.39 g cm–3, and the field capacity was 0.30 cm3 cm–3.
Field experiments of drip-irrigated maize were conducted over two seasons, from May 1 to 17 September 2022 and from May 1 to 14 September 2023. Maize was seeded at a 50-cm row spacing and 30-cm planting spacing. Three different types of water-soluble phosphate fertilizers were used in both seasons: urea phosphate (F1), monoammonium phosphate (F2), and a combination of UP and monoammonium phosphate (F3). A treatment without phosphate fertigation (F0) was used as the control. Three replicates and a randomized whole-block layout were used. Consequently, a total of 12 experimental plots were constructed. Each plot had two driplines installed in the median between two neighboring rows of maize, with one dripline for irrigation in each row. Plain structure driplines with a nominal discharge rate of 2.0 L h–1 were used.
A similar irrigation schedule was used for all treatments. The amount of irrigation was determined based on the field moisture content and was applied when the average soil moisture in the root zone was reduced to 60%–70% of the field capacity (China Planning Press, 2020). The upper limit of irrigation water is the field capacity. Soil samples were collected weekly at depths of 0–60 cm using a 5-cm-diameter soil auger, with a sampling interval of 20 cm. Then, soil–water content was measured using the desiccation method. Additional measurements were conducted 1 day following an irrigation event. The total irrigation amounts in 2022 and 2023 were 90 mm and 120 mm, respectively. Before being delivered to the experimental plots for irrigation, the sewage effluent was held in a 40 m³ reservoir. The irrigation system for sewage effluent was outfitted using a submerged pump that had a lift of 36 m and a capacity of 15 m³ h–1. Disk filters (0.125 mm, 120 meshes) were installed ahead of the supply line and cleaned prior to each irrigation event. The system maintained an intake pressure of 0.1 MPa during irrigation.
Fertilization of 180 kg ha−1 N, 100 kg ha−1 P2O5, and 100 kg ha−1 K2O were used in the experiment throughout the irrigation season. Of these, basal fertilizers were applied at a rate of 70 kg ha−1 of N (urea), 40 kg ha−1 of P2O5 (superphosphate), and 20 kg ha−1 of K2O (K2SO4) before planting. The remaining fertilizers were delivered in three batches in both seasons for the phosphate fertilizer treatment (Table 1). For various treatments, an appropriate phosphate fertilizer was chosen based on the specific requirements of the experiment. To guarantee that the amount of nitrogen delivered was constant for each treatment, a certain amount of urea was injected (because UP and MAP are nitrogen-containing phosphate fertilizers). The fertilizers were fertigated using drip irrigation systems with a water-driven adjustable proportional pump (model 2504, TEFEN, Israel). Fertilization and irrigation in the control group (F0) were identical to those in the treatment group, except for the absence of phosphate fertilizers.
The wastewater used in the experiment was supplied by a sewage treatment plant that predominantly treated domestic sewage using an oxidation pond. To track the changes in water quality, water samples were obtained from the reservoir before each irrigation session (three and four sampling times in the 2022 and 2023 seasons, respectively). Several physical and biochemical parameters of the water were measured, and the averages and standard deviations are listed in Table 2.
Using a 5-cm-diameter auger, soil samples were taken around the center of each plot at 20-cm intervals from 0 to 40 cm in depth to ascertain the fluctuation in accessible phosphorus concentrations in the soil profile over the maize growing season. An emitter perpendicular to the dripline, where the average soil-accessible phosphorus concentration was anticipated, was approximately 10 cm from the sample location. Before analysis, the samples were air-dried, crushed, and passed through a 2-mm screen. For each sample, 5 g of air-dried soil was extracted with 50 mL of 0.5 mol L–1 NaHCO3, and the available phosphorus contents were measured using a SmartChem 450 (AMS, France).
Plant height and leaf area index (LAI) were measured at the seedling, joining, heading, filling, and maturity stages of three typical maize plants with good growth that were labeled in each experimental plot.
To examine the impact of different phosphate fertilizer types on maize development, the aboveground plant biomass was periodically evaluated. The aboveground plant biomass was calculated by weighing the plant samples after they were dried at 75°C to a consistent weight. Then, the dried plant samples were digested in a mixture of H2SO4 and H2O2 (Johnson and Ulrich, 1959). The total N (TN) and total P (TP) contents of plant samples were measured using a SmartChem 450 instrument (AMS, France). The products of the N and P concentrations and aboveground plant biomass were used to calculate the plant absorption of N and P (Wang et al., 2014). At harvest, maize was collected from three spots equidistant along the two middle rows in each plot. For each sample point, six plants in each row were used to calculate the yield and yield components. Yield components, including ear length, barren ear tip, kernel number per cob, and 100-seed weight, were measured.
Based on P uptake and maize yield, the crop P fertilizer utilization efficiency (%), P fertilizer partial factor productivity (kg kg−1), and P agronomic efficiency (kg kg−1) were calculated (Chtouki et al., 2024) according to Equations 1–3, respectively:
where P and P0 are the total amount of phosphorus absorbed by the plant (kg ha–1) at harvest with and without P fertilization, respectively; Y and Y0 are the maize yield (kg ha–1) with and without P fertilization, respectively; and PF is the amount of the phosphorus fertilizer input (kg ha–1).
The relative emitter discharge (Dra, %) in the lateral direction was measured at the end of the experiment in 2023. The test methods have been described in detail by Hao et al. (2017). Two emitter samples were obtained from the front, middle, and end of the capillaries. The average values of the six emitters were calculated to serve as the microbiological test samples for the experiment. The contents of solid particles (DW) and extracellular polymers (EPS) were measured. The EPS of biofilms contained predominantly extracellular polysaccharide (EPO) and extracellular protein (EPR) (Zhou et al., 2013).
Another part of the biofilm was frozen at −80° C to ascertain the changes in bacterial and fungal communities in the biofilm. The biofilm microbial DNA was extracted from the samples. Soil bacteria were analyzed by amplifying the V3–V4 region of the 16S rRNA, whereas fungi were analyzed by amplifying the ITS2 region. Majorbio Scientific Ltd. (Shanghai, China) performed bacterial and fungal biofilm polymerase chain reaction amplification, library creation, and Illumina MiSeq sequencing.
SPSS software (version 19.0; IBM, Armonk, NY, United States) was used to perform the statistical tests. One-way analysis of variance (ANOVA) with three replicates was used to test whether the phosphate fertilizer type had a significant effect on plant height, LAI, aboveground plant biomass, and maize yield, with a significance level set at 0.05. Spearman’s analysis was used to analyze the correlations among Dra, EPS, DW, and bacterial and fungal communities. Duncan’s multiple range test was performed on all treatments.
To investigate alpha diversity changes in soil bacteria and fungi, Chao 1, Ace, Shannon, and Simpson indices in each sample were calculated using MOTHUR software (version v.1.30.2) based on the abundance distribution of all amplicon sequence variant (ASV) in each sample.
To determine the dominant species and relative abundance of each dominant species in each sample at a certain taxonomic level, the R language (version 3.3.1) tool was used to create a community bar map.
The distribution of available phosphorus in the soil at different growth stages of maize treated with different phosphorus fertilizers is shown in Figure 1. In both seasons, the distribution of available phosphorus in the soil profile was layered. The available phosphorus content in the soil at different growth stages decreased as the soil depth increased. For example, the average available phosphorus content at maturity in 0–20 cm and 20–40 cm for the P fertilizer treatments was 13.55 mg kg–1 and 3.96 mg kg–1 in 2022 and 15.78 mg kg–1 and 5.78 mg kg–1 in 2023, respectively. This suggests that the distribution of available phosphorus remained concentrated in the 0–20 cm soil layer throughout the growth phase after the application of phosphate fertilizers and that it was difficult for it to migrate with irrigation water. Comparing the Olsen P (soil-accessible phosphorus) after harvest with that prior to planting, only treatment with mono-ammonium phosphate (F2) increased the available phosphorus content at 0–20 cm by 34.4% in 2022. However, the content of soil-available phosphorus was boosted by all P fertilization treatments, with values of 12.04–18.07 mg kg–1 at harvest, which was 10.94 mg kg–1 before sowing in 2023. The findings demonstrate that the soil-available phosphorus content in 2023 was higher than that in 2022. The mean value of soil-available phosphorus was 17.4–25.7 mg kg–1 for treatments with phosphate fertigation in 2023 and was 8.6–17.4 mg kg–1 in 2022. The retention of soil-available P increased with the number of fertigation events.
Figure 1. Soil-available phosphorus content at different growth stages in maize in (A, B) 2022 and (C, D) 2023.
Compared with the treatment without phosphate fertigation, phosphorus fertilizer increased the amount of phosphorus accessible in the soil from 0 to 40 cm, especially in the top layer. In comparison to the control group, the 0–20 cm soil accessible phosphorus concentration increased by 51.8%–179.2% and 78.9%–128.5% following the third fertilization treatment in 2022 and 2023 seasons, respectively. The content of Olsen P varied depending on the particular type of P fertilizer used. In both years, the available phosphorus content in the top layer treated with MAP (F2) was higher than that of the other treatments. Although the deep soil (20–40 cm) displayed distinct patterns, the available phosphorus content in the soil treated with UP (F1) was greater than that in the other treatments.
The plant height and LAI for the different treatments in 2022 and 2023 are compared in Figure 2. Plants treated with phosphorus usually grew taller than those not treated without the P fertilizer. For instance, the final plant height with the phosphorus fertilizer (247.3–255.1 cm) was 0.8%–3.99% higher than that for treatment without phosphorus fertilization in 2022. The ANOVA results indicated that the phosphorus type had no effect on plant height in both seasons. A similar result was found for LAI. For instance, the LAI for treatments with the P fertilizer at maturity was 3.30–3.62, which was 24.2%–36.5% higher than that for F0. According to the ANOVA results, phosphorus type had a substantial effect on the LAI on 14 August 2023.
Figure 2. Plant height and LAI of maize in (A, B) 2022 and (C, D) 2023 seasons, respectively. NS and * represent nonsignificant and significant differences, respectively, with a significance level set at 0.05.
The aboveground plant biomass during the two seasons is shown in Figure 3. Phosphorus fertilization increased the aboveground plant biomass; however, that P fertilizer type had no effect on the aboveground plant biomass in either year. For example, plant biomass for F1–F3 was 26,057.9–27,093.3 kg ha–1, which was 18.2%–22.9% higher than that for F0 at maturity in 2022. The aging of leaves treated with the phosphorus fertilizer was slow, and photosynthesis was enhanced, which promoted the growth of crops (Figure 2). In 2023, the plant biomass for treatments with P fertilization was 29,453.5–31,201.8 kg ha–1, whereas the plant biomass was 28,258.0 kg ha–1 for the control treatment. Compared to the different treatments, the maximum aboveground plant biomass was achieved using F2 treatment for both years.
Plant P and N uptake at maturity are shown in Tables 3, 4, respectively. Compared to the control (F0), P fertilization (UP, MAP, and UP + MAP) produced greater straw, grain, and total P uptake at maturity. For instance, the averaged P uptake in straw, grain, and the total for treatment with phosphorus fertilizer was 38.94, 80.71, and 119.66 kg ha–1, respectively, which was 5.34%–13.51% higher than that for F0 in 2023. The percentage of P absorbed by grains to the total phosphorus absorbed by maize for treatment F1, F2, F3 at maturity were 69.5%–75.2%, which was lower than that for F0 (76.7%) in 2022. A similar result was found in 2023, with 67.1%–67.7% for treatment with P fertilization and 69.1% for the control. Increasing P uptake reduced the percentage uptake in grain, indicating that more P was stored in the straw, especially in the leaves, which was useful for photosynthesis and accelerated crop growth. These results were consistent with those for LAI and aboveground plant biomass (Figure 2). Comparing the different fertilization treatments, the phosphorus absorption of maize treated with UP (F1) was the highest in both seasons. The increase in phosphorus absorption resulted in a decrease in available soil phosphorus to some extent in treatment F1 (Figure 1).
Compared to the control treatment, P fertilizer application generally increased the N content in the straw, grain, and total maize plant at maturity. For example, the average N uptake in straw, grain, and total maize plant for treatment with P fertigation was 53.65, 169.01, and 222.66 kg ha−1 in the 2022 season, respectively, which was higher than the control treatment. Similar results were observed in 2023 (Table 4).
The maize yield and yield components for the 2022 and 2023 experiments are reported in Tables 5, 6, respectively. The application of the phosphorus fertilizer substantially increased the year length, number of grains per year, and the 100–grain weight, and then increased the maize grain yield; however, there was no discernible difference between the phosphate fertilizer-treated and -untreated groups. The maximum yields of 14,764.2 and 14,778.2 kg ha−1 were obtained under treatments with UP (F1) and MAP (F2) in 2022 and 2023, respectively. Compared with F0, P fertilizers increased the maize yield by 7.4%–19.9% and 11.4%–19.0% in 2022 and 2023, respectively. The influence of phosphate fertilizers on maize yield was substantial in the 2023 growing season, with no effect on yield in 2022. This implies that the influence of P fertilizers increased as the number of fertigation events increased. This also indicated that the average yield (13,943.4 kg ha−1) for 2023 was 4.0% higher than that for 2022 (13,405.6 kg ha−1). The yield of maize showed no appreciable differences among the P fertilizer treatments.
Crop P fertilizer utilization efficiency (%), P fertilizer partial factor productivity (kg kg−1), and P agronomic efficiency (kg kg−1) are reported in Tables 5 and 6. The phosphorus fertilizer variety had no marked effect on the phosphorus fertilizer utilization efficiency, P fertilizer partial factor productivity, or P agronomic efficiency of maize in either season. In 2022, F1 treatment (UP) produced the highest phosphorus fertilizer utilization efficiency, P fertilizer partial factor productivity, and P agronomic efficiency, with values of 8.49%, 147.6 kg kg−1, and 24.57 kg kg−1, respectively. In 2023, the changes in the utilization efficiency of the three fertilizers were consistent: F2 (MAP) > F1 (UP) > F3 (UP + MAP).
The Dra, DW, and EPS contents of the emitters for different treatments are reported in Table 7. The EPS content in the emitter biofilm produced by the phosphorus fertilizers was substantially lower than that in the control treatment (F0). The content of EPO, EPR, and EPS decreased by 33.4%–58.5%, 17.4%–25.4%, and 30.1%–42.0%, respectively. The DW of the biofilm was reduced by 39.8%–79.7%. The lower DW for the phosphorus treatments demonstrated that the acid fertilizer could successfully reduce the blockage of the emitter. At the end of 2023, the Dra content for the different treatments was 94.1%–97.8%, in the following order: Dra (F1) > Dra (F3) > Dra (F2) > Dra (F0).
Variations in the richness and diversity indices of the bacterial and fungal communities in the various treatments are shown in Table 8. The ASV numbers were 692–790 and 447–476 for the bacterial and fungal communities, respectively, under different fertilization treatments. In general, bacterial and fungal alpha diversity in emitter biofilms increased with phosphorus fertilization. Compared to the control, phosphorus fertilizers increased the bacterial Ace, Chao, and Shannon indices by 9.7%–9.8%, 9.8%–10.2%, and 5.1%–7.3%, respectively. However, P fertilizer decreased the Simpson index by 42.6%–52.5%. The results indicate that phosphorus application increased the richness and uniformity of bacterial and fungal communities in the emitter biofilms.
Table 8. Variations in richness and diversity indices of bacterial and fungal microbial communities in various treatments.
The relative abundance of phyla in the bacterial groups with different clogging materials is shown in Figure 4. Compared to no P fertilization, phosphorus fertilization changed the composition of the microbial community structure in the emitter-clogging biofilm. For biofilm bacteria, the top five phyla included Actinobacteriota, Chloroflexi, Proteobacteria, Acidobacteriota, and Patescibacteria, accounting for almost 89% of the total bacterial abundance. UP (F1) increased Chloroflexi (15.0%) and Proteobacteria (11.1%) but decreased Actinobacteriota (3.5%), Acidobacteriota (25.0%), and Patescibacteria (20%). MAP (F2) increased Actinobacteriota and Proteobacteria by 13.8% and 27.8%, respectively, but decreased Chloroflexi, Acidobacteriota, and Patescibacteria by 17.6%, 33.3%, and 20%, respectively. For biofilm fungi, the dominant phylum was Ascomycota, accounting for over 68% of the total fungal abundance. Compared to non-fertigation treatment, phosphorus fertilizer application decreased Ascomycota by 1.7%–7.0% and increased Rozellomycota by 2.6%–27.6%.
Phosphorus fertilizers change bacterial and fungal community characteristics. Correlations among bacterial and fungal phyla, biofilm indices (EPO, EPR, EPS, and DW), and Dra are shown in Figure 5. Chloroflexi, Planctomycetota, Latescibacterota, NB1–j, and Sumerlaeota were negatively correlated with DW, whereas Patescibacteria and Deinococcota were positively correlated. Gemmatimonadota was negatively correlated with EPO and EPS. Actinobacteriota, Cyanobacteria, and SAR324_cladeMarine_group_B were positively correlated with EPR. Latescibacterota and Sumerlaeota were positively correlated with Dra. Bacteria may be more affected by phosphorus fertilizer than fungi. For the fungi, Monoblepharomycota was negatively correlated with DW, whereas Basidiomycota was positively correlated with EPR. The reduction in the EPS concentration suggests that the whole viscous extracellular polymer of the biofilm is released after fertilization, which diminishes the chance that nutrients and suspended particles would be adsorbed in the water and slows down clogging (Table 7).
Figure 5. Correlations among (A) bacterial and (B) fungi phyla and biofilm indices (EPO, EPR, EPS, and DW) and Dra. relative discharge (Dra), solid particles (DW), extracellular polysaccharide (EPO), extracellular protein (EPR), and extracellular polymer (EPS).
The distribution and absorption of phosphorus are critical for crop growth and development, and phosphorus movement and transformation in the soil have a direct impact on crop phosphorus absorption. The application of phosphorus fertilizers improved the labile P content in the soil (Chen et al., 2022). In the current study, phosphorus fertilizers increased the amount of phosphorus accessible in the soil from 0 to 40 cm, especially in the top layer (Figure 1). This is mainly because orthophosphate fertilizers can easily react with Ca2+, Fe3+, Al3+, and other clay minerals in calcareous soils, resulting in the formation of less soluble phosphorus, which prevent phosphorus from migrating through the soil (Li et al., 2002; Liu et al., 2022). The content of Olsen P (soil-accessible phosphorus) varied depending on the particular type of P fertilizer used. In both seasons, the available phosphorus content in the top layer treated with MAP (F2) was higher than that in the other treatments. Although the deep soil (20–40 cm) displayed distinct patterns, the available phosphorus content in the soil treated with UP (F1) was greater than that in the other treatments. All water-soluble phosphate fertilizers used were acid fertilizers in our experiment, which may account for the result. Phosphorus in calcareous soils can move freely as the pH decreases (Zhang et al., 2019). In the current study, UP was more potent in reducing soil pH than the other fertilizers and was less affected by soil fixation, inducing a strong migration distance. A similar result was reported by Ma et al. (2014); a key factor in increasing the availability of P is the efficient acidification of the rhizosphere environment, which depends on the characteristics of fertilizers (Ma et al., 2014).
The addition of phosphorus fertilizers can increase the availability of phosphorus in the soil to plants, successfully compensating for reduced plant production caused by P deficiency (Sahandi et al., 2019). In the current study, plants treated with phosphorus were usually taller, and LAI was greater than that in plants not treated with P fertilizers. A higher LAI and slower leaf aging rate promoted photosynthesis and further increased the dry matter mass (Figure 3). In 2023, the plant biomass for treatments with P fertilization was 29,453.5–31201.8 kg ha–1, whereas the plant biomass was 28,258.0 kg ha–1 for the control treatment. Similar results were reported by Wang et al. (2017), in which maize growth parameters, including plant height and LAI, were improved by treatment with P fertilizers.
The application of phosphorus may effectively encourage its accumulation in plants, increase the leaf area index, improve the efficiency of photosynthetic processes in maize, promote plant development and substance accumulation, and ultimately increase the maize production (Liang et al., 2024; Wang et al., 2024). The critical phases of requirements for phosphorus in maize are the middle and later stages of growth; maize dry matter and yield can be improved by increasing the amount of topdressing in later stages (Qi et al., 2024). Plants mostly utilize phosphorus as orthophosphate, of which H2PO4− is the most readily absorbed, followed by HPO42− and PO43−. The form of urea P is PO43−, which can only be partially changed into H2PO4− and HPO42− for crop absorption (Lambers, 2022; Chtouki et al., 2024). This conversion takes time, and the delayed improvement in fertilizer efficiency may help improve phosphorus absorption to some extent.
Our findings indicated that phosphorus fertilization promoted the absorption of nitrogen fertilizer. However, the yield of maize showed no appreciable differences among the P fertilizer treatments. The adequate quantity of P in the soil prior to the experiment (Olsen P concentration was 12.55 mg kg−1) may account for this result (Wang and Shen, 2019). The increase in phosphorus absorption resulted in a decrease in available soil phosphorus to some extent for treatment F1 (Figure 1). In addition to encouraging the development of maize plants, phosphorus fertilizer treatment also encouraged plant uptake of soil phosphorus, which led to a deficiency in the soil phosphorus concentration. The application of water-soluble phosphorus fertilizers can improve the activation rate of soil phosphorus and thus improve the utilization rate of phosphorus fertilizers. In the current study, F1 treatment (UP) produced the highest phosphorus fertilizer utilization efficiency, partial factor productivity, and agronomic efficiency in 2022. In 2023, the changes in the utilization efficiency of the three fertilizers were consistent, occurring in the following order: F2 (MAP) > F1 (UP) > F3 (UP + MAP). Salah et al. (2022) concluded that MAP fertilizers increase the crop output.
In this study, we demonstrated that the application of UP and MAP effectively reduced emitter clogging. The growth of microorganisms in the clogging biofilm inside the emitter can be effectively inhibited by acidic phosphorus fertilizers, which leads to a decrease in the number of microorganisms, modifications in the microbial community structure, and a reduction in the capacity to secrete viscous EPS. Compared to the control, the content of EPO, EPR, and EPS decreased by 33.4%–58.5%, 17.4%–25.4%, and 30.1%–42.0% in the phosphorus fertilizer treatments, respectively (Table 7). It is possible to successfully eradicate 16% of biofilms and 60% of bacteria by lowering the pH of irrigation water (Chen and Stewart, 2000). Studies have shown that microbial community activity is the starting factor of drip emitter clogging (Li et al., 2013; Lequette et al., 2021). The functional groups in EPO primarily serve as the “bone frame” of the biofilm, interacting with one another to create a cross network. The biofilm becomes less stable, and its structure is disrupted by the phosphorus fertilizer treatments. Some extracellular proteins are hydrophobic; they hinder the absorption of nutrients in the water to some degree, inhibiting the growth of biofilms and causing absorption to fall over an extended period of time (Song et al., 2021). The stability and shape of the biofilm are affected by this decrease, resulting in a loose structure and a propensity to separate (Zhou et al., 2013). Thus, the biofilms’ DW was lowered by 39.8%–79.7%. Generally, a greater DW represents more severe clogging in emitters (Zhou et al., 2013). The lower DW for the phosphorus treatments demonstrated that the application of MAP and UP successfully reduced the blockage of the emitter (Table 7). In contrast, the application of MAP and UP reduced the pH of the irrigation water, inhibiting chemical fouling. The results agreed well with those of studies that concluded that UP outperformed the anti-clogging abilities of weak acidic fertilizers (monopotassium phosphate) when using saline water (Muhammad et al., 2021; Wang et al., 2021).
The microbial community activity is the starting factor for drip emitter clogging (Li et al., 2013; Lequette et al., 2021). The results of the current study indicate that phosphorus application increases the richness and uniformity of bacterial and fungal communities in the emitter biofilms (Table 8). Given their important roles in microbial metabolism and bacterial multiplication, the high quantities of nitrogen and phosphorus in fertilizers may be related to an increase in variety (Lu et al., 2016). Both the UP and MAP increased the abundance of Proteobacteria and decreased the abundance of Acidobacteriota (Figure 4). Phosphorus fertilizers considerably changed bacterial and fungal community characteristics. To explore the key microorganisms in the biofilm formation process and how they interact with viscous extracellular polymers, blocking substances, and the degree of blockage, the correlations among bacterial and fungal phyla, biofilm indices (EPO, EPR, EPS, and DW), and Dra are studied, as shown in Figure 5. Acidobacteriota was strongly positively correlated with EPR, whereas Proteobacteria was negatively correlated with EPO, EPR, and EPS (Figure 5). Gemmatimonadota was negatively correlated with both EPO and EPS. The physiological characteristics of microorganisms were affected by EPS, which offers robust protection for biofilm stability (Hao et al., 2018; Song et al., 2021). The extracellular polymer secreted by microorganisms triggers substances in the water to aggregate, owing to its viscosity, creating a porous biofilm with a relatively stable structure and complex morphology. The reduction in EPS concentration suggests that the whole viscous extracellular polymer of the biofilm was released after fertilization, which diminishes the probability that nutrients and suspended particles would be adsorbed in the water and slows down clogging (Table 7).
Field studies lasting 2 years were conducted in a semi-humid area of the North China Plain to evaluate the effects of phosphorus fertilizer type on soil-available phosphorus, maize growth, and emitter clogging. Phosphorus fertilizer applications substantially increased the Olsen P content in the soil, especially in the 0–20 cm soil layer. This trend progressively strengthened as the number of fertigation events increased. Phosphorus fertilizers promoted the growth and yield of maize and the utilization rate of phosphorus fertilizers; however, the P fertilizer type had no statistical effect on height, LAI, plant P and N uptake, and plant biomass in either year. The effects of phosphate fertilizers on maize yield were more substantial during the 2023 growing season. Phosphorus fertilization changed the composition of the bacterial and fungal microbial community structures in the emitter-clogging biofilm. Both UP and MAP increased the abundance of Proteobacteria and decreased the abundance of Acidobacteriota. Compared with the control, the contents of EPO, EPR, EPS, and DW in the P fertilizer treatment were decreased by 33.4%–58.5%, 17.4%–25.4%, 30.1%–42.0%, and 39.8%–79.7%, respectively. Acidic phosphorus fertilizers, such as urea phosphorus and MAP, can maintain high drip irrigation performance and produce a high crop yield.
Fertilizers containing phosphorus typically exhibit noticeable residual effects. Additional research is needed to explore the long-term impact of applying various types of phosphorus fertilizers on the phosphorus forms and the rate of fertilizer use in the soil. Further research is warranted on the variations in phosphorus form transformation and the availability of different varieties of phosphate fertilizers in soils with different properties as the effectiveness of the same phosphate fertilizer can differ depending on soil properties.
The original contributions presented in the study are included in the article/supplementary material; further inquiries can be directed to the corresponding author.
FH: conceptualization, data curation, formal analysis, and writing–original draft. ZL: data curation, software, and writing–original draft. ZZ: funding acquisition, methodology, resources, and writing–review and editing. ZW: project administration, supervision, and writing–review and editing.
The author(s) declare that financial support was received for the research, authorship, and/or publication of this article. This study was financially supported by the Basic Research Project of Shanxi Province (grant no. 20210302124156), the Science and Technology Innovation Project of Colleges and Universities in Shanxi Province (grant no. 2021L126), the National Natural Science Foundation of China (grant no. 42207102), the Scientific Research Project of Shanxi Province Outstanding Doctor Work Award Fund (grant no. SXYBKY2018029), the Science and Technology Innovation Fund project of Shanxi Agricultural University (grant no. 2018YJ40), the Shanxi Water Conservancy Science and Technology Research and Promotion Project (grant no. 2023QT094), and the National Natural Science Foundation of China (grant no. 52279053).
The authors declare that this study was conducted in the absence of any commercial or financial relationships that could be construed as potential conflicts of interest.
The author(s) declare that no Generative AI was used in the creation of this manuscript.
All claims expressed in this article are solely those of the authors and do not necessarily represent those of their affiliated organizations, or those of the publisher, the editors, and the reviewers. Any product that may be evaluated in this article, or claim that may be made by its manufacturer, is not guaranteed or endorsed by the publisher.
Atere, C., Ge, T., Zhu, Z., Liu, S., Huang, X., Shibsitova, O., et al. (2019). Assimilate allocation by rice and carbon stabilisation in soil: effect of water management and phosphorus fertilisation. Plant Soil 445, 153–167. doi:10.1007/s11104-018-03905-x
Brownlie, W. J., Sutton, M. A., Reay, D. S., Heal, K. V., Hermann, L., Kabbe, C., et al. (2021). Global actions for a sustainable phosphorus future. Nat. Food 2, 71–74. doi:10.1038/s43016-021-00232-w
Chen, X., and Stewart, P. (2000). Biofilm removal caused by chemical treatments. Water Res. 34 (17), 4229–4233. doi:10.1016/S0043-1354(00)00187-1
Chen, X., Yan, X., Wang, M., Cai, Y., Weng, X., Su, D., et al. (2022). Long-term excessive phosphorus fertilization alters soil phosphorus fractions in the acidic soil of pomelo orchards. Soil Tillage Res. 215, 105214. doi:10.1016/j.still.2021.105214
Chen, Y., Hu, S., Guo, Z., Cui, T., Zhang, L., Lu, C., et al. (2021). Effect of balanced nutrient fertilizer: a case study in Pinggu District, Beijing, China. Sci. Total Environ. 754, 142069. doi:10.1016/j.scitotenv.2020.142069
Chien, S., Adams, F., Khasawneh, F., and Henao, J. (1987). Effects of combinations of triple superphosphate and a reactive phosphate rock on yield and phosphorus uptake by corn. SSSA 51, 1656–1658. doi:10.2136/sssaj1987.03615995005100060045x
China Planning Press (2020). Chinese National Standard GB/T 50485-2020: technical standard for microirrigation engineering. Beijing, China.
Chtouki, M., Naciri, R., Garr´e, S., Nguyen, F., Zeroual, Y., and Oukarroum, A. (2022). Phosphorus fertilizer form and application frequency affect soil P availability, chickpea yield, and P use efficiency under drip fertigation. J. Plant Nutr. Soil Sci. 185, 603–611. doi:10.1002/jpln.202100439
Chtouki, M., Naciri, R., and Oukarroum, A. (2024). A review on phosphorus drip fertigation in the Mediterranean region: fundamentals, current situation, challenges, and perspectives. Heliyon 10, e25543. doi:10.1016/j.heliyon.2024.e25543
Fan, J., Lu, X., Gu, S., and Guo, X. (2020). Improving nutrient and water use efficiencies using water-drip irrigation and fertilization technology in Northeast China. Agric. Water Manag. 241, 106352. doi:10.1016/j.agwat.2020.106352
Hao, F., Li, J., Wang, Z., and Li, Y. (2017). Effect of ions on clogging and biofilm formation in drip emitters applying secondary sewage effluent. Irrig. Drain. 66, 687–698. doi:10.1002/ird.2141
Hao, F. Z., Li, J. S., Wang, Z., and Li, Y. F. (2018). Effect of chlorination and acidification on clogging and biofilm formation in drip emitters applying secondary sewage effluent. Trans. ASABE 61, 1351–1363. doi:10.13031/trans.12764
Ji, B., Li, W., Xu, M., Niu, J., Zhang, S., and Yang, X. (2021). Varying synthetic phosphorus varieties lead to different fractions in calcareous soil. Sci. Agric. Sin. 54 (12), 2581–2594. doi:10.3864/j.issn.0578-1752.2021.12.009
Johnson, C. M., and Ulrich, A. (1959). “Analytical methods for use in plant analysis,” in Bulletin of the California agricultural experiment station. California agricultural experiment station, California, USA.
Lambers, H. (2022). Phosphorus acquisition and utilization in plants. Ann. Rev. Plant Biol. 73, 17–42. doi:10.1146/annurev-arplant-102720-125738
Lequette, K., Ait-Mouheb, N., Adam, N., Muffat-Jeandet, M., Bru-Adan, V., and Wéry, N. (2021). Effects of the chlorination and pressure flushing of drippers fed by reclaimed wastewater on biofouling. Sci. Total Environ. 758, 143598. doi:10.1016/j.scitotenv.2020.143598
Li, K. Y., Niu, W. Q., Zhang, R. C., and Liu, L. (2015). Accelerative effect of fertigation on emitter clogging by muddy water irrigation. TCSAE 31 (17), 81–90. doi:10.11975/j.issn.1002-6819.2015.17.011
Li, T., Wang, Y., Liu, F., Fan, X., and Gao, R. (2002). Study on phosphorus transport of different sectional levels soil. Soil Environ. Sci. 11 (3), 290–293. doi:10.16258/j.cnki.1674-5906.2002.03.017
Li, Y. K., Song, P., and Zhou, B. (2013). Microbiology mechanism and controlling methods for emitter clogging in the reclaimed water drip irrigation system. TCSAE 29 (15), 98–107. doi:10.3969/j.issn.1002-6819.2013.15.013
Liang, C., Liu, X., Lv, J., Zhao, F., and Yu, Q. (2024). The impact of different phosphorus fertilizers varieties on yield under wheat–maize rotation conditions. Agronomy 14, 1317. doi:10.3390/agronomy14061317
Liu, C., Dang, X., Mayes, M. A., Chen, L., and Zhang, Y. (2017). Effect of long-term irrigation patterns on phosphorus forms and distribution in the brown soil zone. Plos One 12 (11), e0188361. doi:10.1371/journal.pone.0188361
Liu, D., Liu, M., Liang, F., Li, Q., Tian, Y., and Jia, H. (2022). Effects of water-soluble phosphorus fertilizer on distribution of phosphorus in calcareous soil and utilization of phosphorus by maize. Plant Nutr. Fertil. 28 (9), 1720–1733. doi:10.11674/zwyf.2022029
Lu, H., Feng, Y., Wu, Y., Yang, L., and Shao, H. (2016). Phototrophic periphyton techniques combine phosphorous removal and recovery for sustainable salt-soil zone. Sci. Total Environ. 568, 838–844. doi:10.1016/j.scitotenv.2016.06.010
Ma, C., Xiao, Y., Puig-Bargués, J., Shukla, M., Tang, X., Hou, P., et al. (2020). Using phosphate fertilizer to reduce emitter clogging of drip fertigation systems with high salinity water. J. Environ. Manage. 263, 110366. doi:10.1016/j.jenvman.2020.110366
Ma, Q., Wang, X., Li, H. B., Li, H. G., Cheng, L. Y., Zhang, F. S., et al. (2014). Localized application of NH4+–N plus P enhances zinc and iron accumulation in maize via modifying root traits and rhizosphere processes. Field Crops Res. 164, 107–116. doi:10.1016/j.fcr.2014.05.017
Mikkelsen, R. L. (1989). Phosphorus fertilization through drip irrigation. J. Prod. Agric. 2 (3), 279–286. doi:10.2134/jpa1989.0279
Muhammad, T., Zhou, B., Liu, Z. Y., Chen, X. Z., and Li, Y. K. (2021). Effects of phosphorus-fertigation on emitter clogging in drip irrigation system with saline water. Agric. Water Manag. 243, 106392. doi:10.1016/j.agwat.2020.106392
Qi, X. Y., Cao, G. J., Geng, Y. H., and Zou, X. (2024). Effect of distribution ratio of phosphorus fertilizer in drip irrigation on absorption and utilization of phosphorus in maize. J. Jilin Agric. Uni. 46 (2), 205–210. doi:10.13327/j.jjlau.2020.5684
Roberts, T. L., and Johnston, A. E. (2015). Phosphorus use efficiency and management in agriculture. Resour. Conserv. Recy. 105, 275–281. doi:10.1016/j.resconrec.2015.09.013
Roy, E. D., Willig, E., Richards, P. D., Martinelli, L. A., Vazquez, F. F., Pegorini, L., et al. (2017). Soil phosphorus sorption capacity after three decades of intensive fertilization in Mato Grosso, Brazil. Agric. Ecosyst. Environ. 249, 206–214. doi:10.1016/j.agee.2017.08.004
Sahandi, M. S., Mehrafarin, A., Badi, H. N., Khalighi-Sigaroodi, F., and Sharifi, M. (2019). Improving growth, phytochemical, and antioxidant characteristics of peppermint by phosphate-solubilizing bacteria along with reducing phosphorus fertilizer use. Ind. Crops Prod. 141 (9), 111777. doi:10.1016/j.indcrop.2019.111777
Salah, Y. B., Oudadesse, H., Lefeuvre, B., Tounsi, S., and Feki, H. E. (2022). Purified monoammonium phosphate fertilizer promotes the yield and reduces heavy metals accumulation in tomato (Lycopersicon esculentum L.). Int. J. Environ. Sci. Technol. 19, 1753–1764. doi:10.1007/s13762-021-03223-3
Shen, Y., Puig-Bargués, J., Li, M. Y., Xiao, Y., and Li, Y. K. (2022). Physical, chemical and biological emitter clogging behaviors in drip irrigation systems using high-sediment loaded water. Agric. Water Mana. 270, 107738. doi:10.1016/j.agwat.2022.107738
Shirazi, M., and Boersma, L. (1984). A unifying quantitative analysis of soil texture. Soil Sci. Soc. Am. J. 48, 142–147. doi:10.2136/sssaj1984.03615995004800010026x
Song, P., Xiao, Y., Ren, Z. J., Brooks, J. P., Lu, L., Zhou, B., et al. (2021). Electrochemical biofilm control by reconstructing microbial community in agricultural water distribution systems. J. Haz. Mat. 403, 123616. doi:10.1016/j.jhazmat.2020.123616
Sucunza, F., Gutierrez Boem, F., Garcia, F., Boxler, M., and Rubio, G. (2018). Long-Term phosphorus fertilization of wheat, soybean and maize on mollisols: soil test trends, critical levels and balances. Eur. J. Agron. 96, 87–95. doi:10.1016/j.eja.2018.03.004
Vu, D., Tang, C., and Armstrong, R. (2008). Changes and availability of P fractions following 65 years of P application to a calcareous soil in a Mediterranean climate. Plant Soil 304 (1-2), 21–33. doi:10.1007/s11104-007-9516-x
Wang, L., Rengel, Z., Cheng, L. Y., and Shen, J. (2024). Coupling phosphate type and placement promotes maize growth and phosphorus uptake by altering root properties and rhizosphere processes. Field Crops Res. 306, 109225. doi:10.1016/j.fcr.2023.109225
Wang, L., and Shen, J. (2019). Root/Rhizosphere Management for improving phosphorus use efficiency and crop productivity. Better Crops Plant Food. 103, 36–39. doi:10.24047/BC103136
Wang, Z., Li, J. S., Hao, F. Z., and Li, Y. F. (2017). Effects of phosphorus fertigation and lateral depths on distribution of Olsen-P in soil and yield of maize under subsurface drip irrigation. ASABE Annu. Int. Meet. 2017. doi:10.13031/aim.201701105
Wang, Z., Li, J. S., and Li, Y. F. (2014). Effects of drip system uniformity and nitrogen application rate on yield and nitrogen balance of spring maize in the North China Plain. Field Crops Res. 159, 10–20. doi:10.1016/j.fcr.2014.01.006
Wang, Z., Li, J. S., and Yang, X. Q. (2021). Determining injection strategies of phosphorus-coupled nitrogen fertigation based on clogging control of drip emitters with saline water application. Irrig. Drain. 70, 1010–1026. doi:10.1002/ird.2626
Wang, Z., Yang, X. Q., and Li, J. S. (2020). Effect of phosphorus-coupled nitrogen fertigation on clogging in drip emitters when applying saline water. Irrig. Sci. 38, 337–351. doi:10.1007/s00271-020-00675-2
Xiao, Y., Puig-Bargués, J., Zhou, B., Li, Q., and Li, Y. K. (2020). Increasing phosphorus availability by reducing clogging in drip fertigation systems. J. Clean. Prod. 262, 121319. doi:10.1016/j.jclepro.2020.121319
Zhang, H., Zhang, J., Zhang, F., Liu, D., and Wei, C. (2019). Effects of different phosphorus fertilizerson soil phosphorus availability and maize yield under drip irrigation. J. Soil Water Conserv. 33 (2), 189–195. doi:10.13870/j.cnki.stbcxb.2019.02.030
Keywords: bacterial and fungal community, drip irrigation, phosphorus fertilizer, soil-available phosphorus, yield
Citation: Hao F, Liu Z, Zhen Z and Wang Z (2024) Impact of different types of nitrogen and phosphorus compound fertilizers on maize yield and drip emitter clogging using secondary sewage effluent. Front. Environ. Sci. 12:1502902. doi: 10.3389/fenvs.2024.1502902
Received: 27 September 2024; Accepted: 11 November 2024;
Published: 28 November 2024.
Edited by:
Christophe Darnault, Clemson University, United StatesReviewed by:
Bo Zhou, China Agricultural University, ChinaCopyright © 2024 Hao, Liu, Zhen and Wang. This is an open-access article distributed under the terms of the Creative Commons Attribution License (CC BY). The use, distribution or reproduction in other forums is permitted, provided the original author(s) and the copyright owner(s) are credited and that the original publication in this journal is cited, in accordance with accepted academic practice. No use, distribution or reproduction is permitted which does not comply with these terms.
*Correspondence: Zhilei Zhen, emhlbmNoZW5nQHN4YXUuZWR1LmNu
Disclaimer: All claims expressed in this article are solely those of the authors and do not necessarily represent those of their affiliated organizations, or those of the publisher, the editors and the reviewers. Any product that may be evaluated in this article or claim that may be made by its manufacturer is not guaranteed or endorsed by the publisher.
Research integrity at Frontiers
Learn more about the work of our research integrity team to safeguard the quality of each article we publish.