- 1Department of Forest Ecosystems and Society, Oregon State University, Corvallis, OR, United States
- 2Department of Land, Air, and Water Resources, University of California Davis, Davis, CA, United States
- 3ORISE Fellow, USDA Forest Service Pacific Northwest Research Station, Corvallis, OR, United States
- 4College of Earth, Ocean, and Atmospheric Sciences, Oregon State University, Corvallis, OR, United States
- 5USDA Forest Service Western Wildland Environmental Threat Assessment Center, Corvallis, OR, United States
- 6College of Liberal Arts, Oregon State University, Corvallis, OR, United States
Coastal fog occurs along many of the world’s west coast continental environments. It is particularly consequential during summer when an increased frequency of fog co-occurs with the seasonal dryness characteristic of most west coast climate systems, for example, in the Pacific coast of North and South America, the southwestern African coast, and southern coastal Europe. Understanding coastal fog formation and effects has consequences for many disciplines, including the physical (e.g., atmospheric science, oceanography), biological (e.g., biogeography, ecophysiology), and socio-ecological realms (e.g., Indigenous cultural knowledge, public safety, economics). Although research practices differ across disciplines, they share many of the challenges needed to advance fog science. For example, coastal fog remains difficult to reliably monitor when, where, and why it occurs, which adds difficulty to understanding fog’s effects on all facets of the integrated coastal system. These shared challenges provide ripe opportunities for interdisciplinary collaboration, a template with past success in advancing fog-related science that can continue to have success in the future. In this perspectives review, we summarize the current status and frontiers of fog-related science from multiple disciplines, leveraging examples primarily drawn from the Pacific Northwest coastal region of the United States to show how interdisciplinary collaboration is needed to continue to advance our collective understanding of coastal fog formation and effects on west coast environments.
1 Introduction
Fog is a defining feature of western coastal (ocean eastern boundary) environments and is a critical source of moisture in the seasonally-dry mid-latitude regions of the Americas, Africa, and Europe, with widespread and complex effects (Figure 1). Globally, these coasts house endemic species (e.g., Dawson, 1998; Gonzales et al., 2023), productive ecosystems (e.g., Alaback, 1996), and industries that benefit from wetting and cooling effects of fog. Fog also vectorizes fungal pathogens (Shaw et al., 2021), hinders visibility (e.g., Abdel-Aty et al., 2011), contributes to aviation accidents (Gultepe, 2023), and impacts tourism and public health (Hackney et al., 1985). However, fog remains difficult to reliably predict, observe, and model on operational forecast and climate-dynamical time scales (Koračin et al., 2014; Torregrosa et al., 2014).
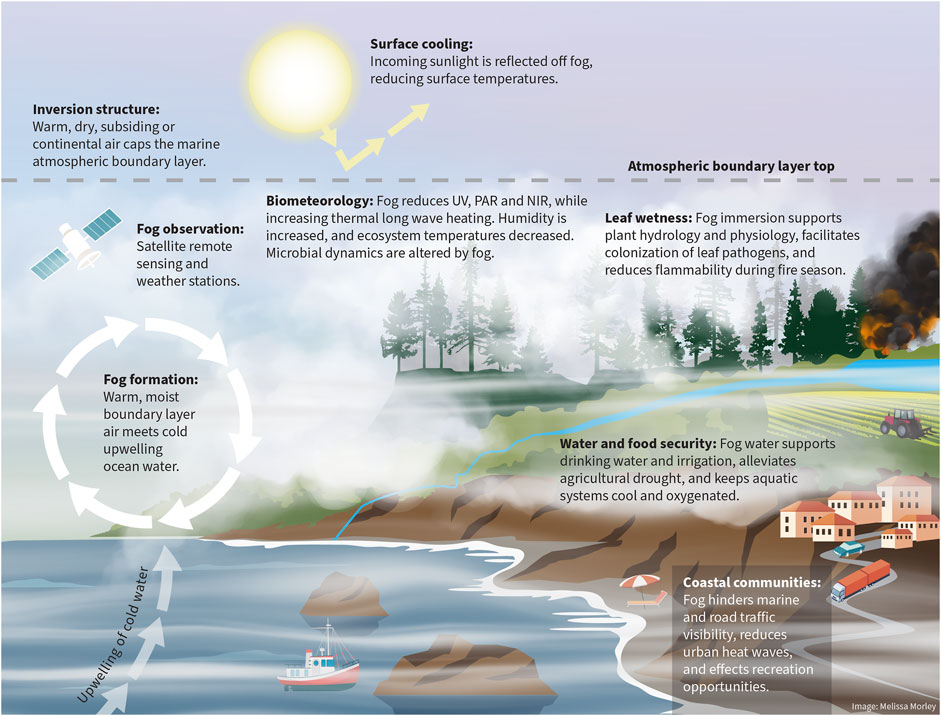
Figure 1. Coastal fog processes and impacts. Fog forms over the ocean and intersects with the coast as it moves landward, impacting overlapping aspects of the natural and built environment.
Multiple scientific disciplines have vested interest in building understanding of the where’s, when’s, and why’s of coastal fog occurrence and effects. We focus on the physical sciences (forecasting, observation, measurement, and coastal meteorology and climatology), biological sciences (ecophysiology, old-growth forest conservation, biometeorology), and socio-ecological sciences (public health, indigenous perspectives, forestry, agriculture, fisheries, and forest pathogens) (Figure 2). Despite not sharing common research languages, many challenges are universal across disciplines. For example, fog is geographically variable, making it difficult to reliably monitor when and where it occurs, and, by extension, to study and predict fog formation, movement, and its socio-environmental effects. These shared challenges provide ripe opportunities for interdisciplinary collaboration, a template with past success in fog science. For example, physical controls on coastal fog formation and movement draw on explanations from both coastal meteorology and oceanography (Leipper, 1948; 1994; Samelson et al., 2021), connections that have been extended to better understand diverse phenomena including species’ distributions (Weathers et al., 2020), plant water budgets (Fischer et al., 2009), and fire season fuel moisture (Emery et al., 2018). Reinforcing this collaborative approach can help answer some of the most pressing research questions surrounding coastal fog: Can we predict when and where fog occurs?; Will climate warming change fog frequency?; Does fog influence conservation of coastal biota?; How do fog and climate shape cultural knowledge systems?
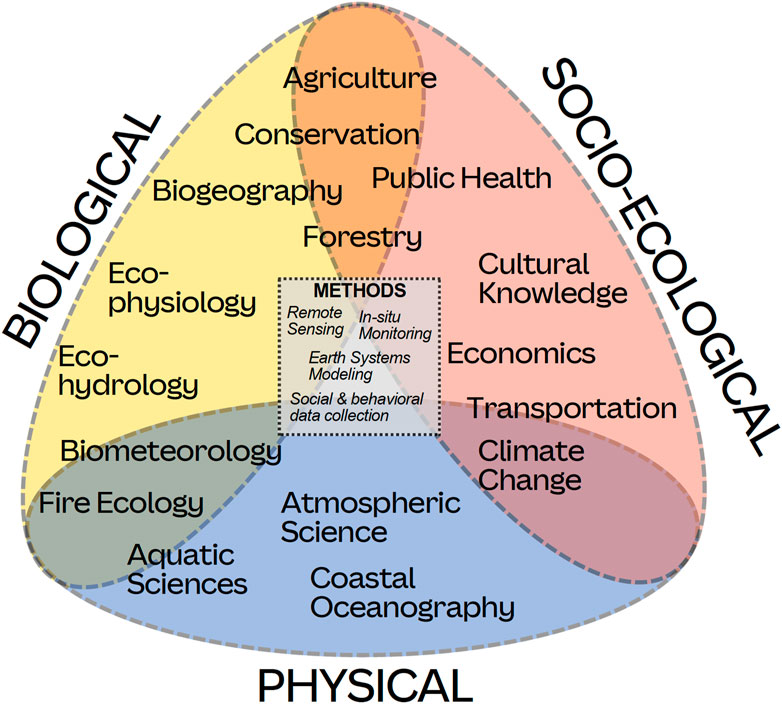
Figure 2. Many different disciplines are involved in fog-related research, spanning the realms of biological, socio-ecological, and physical knowledge systems. These divisions are not absolute, but interact in an often diffuse manner, mirroring the widespread impacts of fog on multiple aspects of the environment and often using common technologies and methods to monitor and study coastal fog. Some Indigenous science aspects encompass the entire figure holistically. The diversity of relevant fields creates special challenges but also exciting opportunities for interdisciplinary collaboration in fog science.
Our goal is to highlight current progress, frontiers, and challenges from selected disciplines as a framework to spur interdisciplinary collaboration in global and regional fog science, supported with illustrative examples drawn from our collective research experience in the coastal Pacific Northwest (PNW), United States.
2 Uniting the interdisciplinary nature of coastal fog research and monitoring
2.1 Fog observation, measurement, and forecasting
Historically, fog detection relied heavily on naked-eye assessments, e.g., using fixed visibility markers at known distances. More quantitative methods deploy Transmissometers or Forward Scatter Sensors on weather stations, providing accurate continuous measurements of near-surface visibility and therefore fog. These can be costly and their location is biased towards urban centers that are usually warmer than surrounding areas (Baldocchi and Waller, 2014). Alternatively, satellite imagery provides large spatial coverage and repeated observations used to continuously map fog for large regions (e.g., Bendix et al., 2006; Clemesha et al., 2016; Torregrossa et al., 2016; Ran et al., 2002). However, most satellite products struggle to distinguish fog from similar atmospheric features like low-lying stratus clouds and have relatively coarse spatial resolution (often 1–4 km), making it difficult to detect geographic variability of small patches of coastal fog. Spatial gaps can be partially filled using on or near-surface cameras (e.g., Bassiouni et al., 2017; del Río et al., 2021) and supported by observational records of cloud base height and visibility (e.g., Johnstone and Dawson, 2010; Dye et al., 2020), but require extensive networks over consecutive years to draw regionally meaningful conclusions.
Fog detection alone is insufficient for characterizing fog. One common measurement is fog water deposition to the surface, which is measured by instruments such as the Standard Fog Collector (Fernandez et al., 2018) and has important applications for water resources, particularly in arid regions (Azeem et al., 2020). Research across the globe in water-scarce western coastal environments have assessed water collection rates and the feasibility of fog water for community and ecosystem consumption (Correggiari et al., 2017). Other properties of fog that can be measured include the liquid water content, droplet concentration, droplet size distribution, and fog chemistry such as concentrations of solutes like sulfur dioxide or hydrogen chloride. Such measurements are critical to understand processes such as fog water deposition rates and pollution levels. Future advances in fog observation will draw from the growing realm of machine learning, linking multi-scale and multi-source monitoring systems (e.g., satellites, cameras, and weather stations) to better predict timing and geography of fog, which can advance fog forecasting as well as research on the utilization for fog as a water resource. Cross-disciplinary collaboration with qualitative social and behavioral data collection methods can assess the outcomes of fog water collection projects, as has been done in Morocco (Ait El Kadi et al., 2024), Bolivia (Castelli et al., 2023) and Peru (Ojani, 2024) and additionally can aid in understanding cultural histories and meanings of fog.
More and better measurements will hopefully lead to a better ability to forecast fog. Fog formation and life cycles are dominated by processes at many scales, from synoptic weather patterns to local aerosol species and concentrations, making its prediction exceptionally difficult (Koračin, 2017). A lack of high-resolution fog measurements limits improvements in subgrid physics parameterizations, though recent field campaigns across the globe have made progress in extensive measurement collection of the physical and chemical properties of fog (Fernando et al., 2021).
2.2 Coastal oceanography and climatology
Fog appears along western coasts at the intersection of land, ocean, and atmosphere, driven by regional and seasonal climate norms. The driving physical processes include the trapping of moist marine air under warm, dry subsiding or continental air and above cool, upwelled coastal ocean water, with warm offshore, upstream sea-surface temperature controlling the properties of the moist trapped air (Leipper, 1948; 1994; Koračin et al., 2005, 2014; Clemesha et al., 2017; Samelson et al., 2021). Fog along western coasts is typically formed through one of two mechanisms, though other mechanisms are possible. In the first, air is advected over a cool ocean surface, typically associated with eastern ocean boundary currents, and cooled to its dewpoint. Such fog forms at the surface and grows upward. In the second, the cloud base of pre-existing low stratus descends until it reaches the surface. Samelson et al. (2021) suggest that the first mechanism is more important for coastal fog in the Pacific Northwest. Globally, it is not known which mechanism is dominant.
The characteristics of these driving elements are likely to shift under climate change, with potential consequences for the coastal fog environment that are still poorly understood. With its dependence on regional physical processes that are sensitive to global climate change, fog plays a critical intermediary role that links global change to a broad range of local impacts, from ecosystem health to public safety. Some climate-model predictions of future fog have been conducted along the PNW coast (O’Brien et al., 2013) and over the North Pacific Ocean (Kawai et al., 2018). However most widely published climate models cannot resolve coastal fog dynamics, and further developments and evaluations are nascent due to sparse long-term empirical records for validating models and the general challenge of developing reliable model predictions of regional climate change (O’Brien et al., 2013). Along the Pacific Northwest coast, Dye et al. (2020) found that coastal fog and low-level clouds over land appear to have declined since 1996 but that coastal fog and low-level clouds over the water have held steady. Such a finding highlights the need for the careful consideration of the spatial variability of fog in coastal regions. Changes in the strength of upwelling winds (Sydeman et al., 2014) or in the source waters for coastal upwelling (Rivas and Samelson, 2011) are two possible future climate evolutions that would likely affect fog.
2.3 Plant ecophysiology and ecohydrology
During fog immersion, the tall canopies of fog-adapted forests enable interception and direct absorption of water droplets deposited on leaves through foliar transport (Limm et al., 2009), and plants with an abundance of root structure at surface soil layers can draw additional moisture from fog drip (Fischer et al., 2009; Carbone et al., 2013; Baguskas et al., 2016). Northern California coastal redwoods (Sequoia sempervirens) attain up to two-thirds of their water from fog, while 80% of co-occurring species in redwood forests are capable of absorbing fog water (e.g., Burgess and Dawson, 2004). Coastal Oregon Douglas-fir (Pseudotsuga menziesii) also gain significant portions of their water budget through fog drip (Harr, 1982). By eliminating vapor pressure deficit within the upper canopy, fog modulates tree water supply and negates vertical water movement to leaves, promoting moisture retention in soil (Burgess and Dawson, 2004). Fog reduces photosynthetically active radiation by reflecting sunlight, however there is an increase in light-use efficiency as high relative humidity allows stoma to remain open for gas exchange without threatening water supply (Still et al., 2009). Potential photosynthetic gains can be counteracted by reduced leaf temperatures from fog shading, but in most cases fog remains a net benefit to photosynthesis (Oliphant et al., 2011; Berry and Goldsmith, 2020; Baguskas et al., 2021). Additionally, in Peru, coastal advection fog has supported reforestation projects that have restored soil and above-ground biomass in areas that had previously been desertified (Certini et al., 2019). Advances in understanding how fog impacts regional net carbon assimilation and water budget can come from linking satellite measurements of photosynthesis activity and evapotranspiration to high resolution fog datasets, though there is considerable uncertainty in quantifying the amount of water intercepted by the canopy or absorbed through leaves outside of the site scale.
2.4 Biometeorology
Biometeorological aspects of coastal fog integrate cell biology, physics, and atmospheric science, ultimately affecting many interacting features of the coastal system including conservation, forest health, agriculture, and public health–future collaborative research can enhance these linkages. Fog interacts with forest canopies through multiple biotic and abiotic processes linking the plants, microbes, invertebrates, and vertebrates coexisting in these systems. Canopy radiative processes are an intricate balance of the four main wavebands—ultraviolet (UV), visible/PAR, near infrared (NIR) and longwave (TIR). Turbulent processes transport trace gasses (water vapor, CO2 and methane) and biotic aerosols (spores, pollens, bacteria, small invertebrates, other microbes). In addition to ecohydrological effects (2.3), increased TIR from coastal fog can protect species sensitive to frost (Snyder and de Melo-Abreu, 2005).
Fog-related reductions in incoming UV positively affect biology, including photomorphogenesis, phototropism, and flavonoid accumulation, but overexposure can cause DNA damage, reactive oxygen species production, cell death, wilting, yellowing, and decreased photosynthesis. Plant pathogens can be negatively affected by UV-B reductions, but Section 2.6 discusses increased susceptibility of trees and plants to pathogens related to the increased leaf wetness and humidity from fog. A similar issue can be seen in insects and amphibians and their sensitivity to increased UV (e.g., Lundsgaard et al., 2020; Shi and Liu, 2021; Dong et al., 2024). Fog increases deposition of microbes in land ecosystems, and fog itself modifies the microbial floral composition (Evans et al., 2019). Fog deposition, while providing water and additional nutrients to biota, also potentially increases toxic chemicals in the food web, including transmission to humans (Weiss-Penzias et al., 2019), and reduced visibility impedes bird migration (Becciu et al., 2021). Forests can also affect the distribution of fog by fostering cooler, humid environments and emitting organic aerosols that may become condensation nuclei (Hunova et al., 2021).
2.5 Forest dynamics and conservation of old-growth forests ecosystems
Coastal PNW forest ecosystems support iconic old-growth stands, and the moist-cool productive environment of the fog zone is tightly tied to their biogeography and fire ecology. Historically, coastal Sitka spruce (Picea sitchensis), Douglas-fir, and shoreline lodgepole pine (Pinus contorta var. contorta), all contributed to large patches of old-growth forest interspersed with younger stands, non-forest openings, and watershed interconnectivity (Wimberley, 2002; Spies et al., 2018). Extensive logging through the 1900s liquidated much of the old forest, resulting in fragmented remnant mature and old-growth forests now highly valued for conservation and ecosystem services (Johnson, Franklin, Reeves, 2023). Fog, and the moist environment it facilitates, contributes to the productive growing conditions driving these old forests (e.g., 2.3 and 2.9) as well as the fire regimes (Agee, 1993; Spies et al., 2018) influencing their persistence. Coastal old-growth forests are regional fire refugia (Meddens et al., 2018; Krawchuk et al., 2020; Naficy et al., 2021) – locations that experience less severe or frequent fire than the surrounding landscape or region–and more broadly as disturbance refugia, including from drought stress and wind. Summer fog is intuitively an ameliorating factor of fuel moisture and flammability in these systems (Emery et al., 2018; Williams et al., 2018) though no focused research has linked coastal fog, fire, and old forests explicitly for the PNW. Coastal PNW fire regimes are typically characterized by infrequent fire with large patches of high severity fire effects (Spies et al., 2018), however finer scaled (trees and stands) fire refugia within these fires play critical roles in the persistence of older forest (Naficy et al., 2021). A more nuanced pyrogeography, including mixed severity fire regimes and Indigenous cultural burning, is increasingly being recognized as having contributed to the socio-ecological dynamics of these coastal forest ecosystems in the past, and will be important to building our understanding of socially and ecologically sustainable management of coastal forests and old growth into the future. Climate change is projected to increase fire activity (Dye et al., 2024) and shift vegetation types (Reilly et al., 2024) in coastal PNW forests, and recent heat waves exposed vulnerabilities to heat scorch and mortality where fog was not present to buffer near surface temperatures (Still et al., 2023). Future studies at the intersection of fire ecology, old forests, fog, and ecological disturbances are important investments for supporting the conservation and adaptation of old-growth forest ecosystems of the coastal fog region.
2.6 Fog and forest pathogens
Spatiotemporal patterns of fog influence plant moisture and temperature, especially foliage. Forest pathogens (microbes that cause disease in forest plants) that colonize foliage require particular environmental parameters for spore release, transport, deposition, and colonization and infection of leaves. The PNW native fungus Nothophaeocryptopus gaeumannii infects Douglas-fir needles, causing Swiss needle cast disease. Its distribution is controlled by fog, leaf wetness, and seasonal temperature dynamics. An active outbreak has been in effect since the late 1990s along coastal Oregon, and subsequently Washington and SW British Columbia, but is uncommon further south (Shaw et al., 2021). Winter temperature and summer leaf wetness during the spore dispersal period (May–August, especially June-July), correlate with infection (Manter et al., 2005). Mild winter temperatures allow rapid colonization of internal portions of the leaf, initiating sporophore development. The fungal development causes early leaf casting, reducing tree productivity. Fog and drizzle create extended leaf wetness periods, facilitating leaf colonization by spores, which are otherwise susceptible to dry-season desiccation. Aerial landscape disease surveys indicate that 75% of areas with visible disease occur within 18 km of the coastline (Shaw et al., 2021), where most coastal fog occurs. Given the conservation, commercial, and recreational role of Douglas-fir in the PNW, reliable spatiotemporal mapping of fog is critical to consider pathogen effects now and in the future.
2.7 Public health and safety
Climate change impacts various aspects of human wellbeing, but health impacts of fog in the context of changing climates have not received extensive attention from researchers. Both mental and physical health may be impacted by fog or its absence. For instance, fog can be hypothesized to contribute to depression. Yet while seasonal affective disorder has been extensively studied, the specific impacts of fog have not. Fog reduces transportation visibility (road, water, rail, and air), leading to injurious or fatal accidents (Abdel-Aty et al., 2011; Fultz and Ashley, 2016). Many coastal communities have poor road networks for evacuation during natural disasters, which may be particularly difficult to navigate in foggy conditions (Dye et al., 2021). It’s plausible that fog has negative respiratory health impacts, especially when fog is contaminated by smoke or other pollutants (Hackney et al., 1985). Fog may also alleviate heat-related illness, especially in elderly people during hot summers (Gershunov et al., 2011, cited in Torregrosa et al., 2014).
Accessing drinking and irrigation water through fog “harvesting” raises innovative research questions involving household water and food insecurity and their impacts on multiple aspects of human health. Survey tools for measuring household food and water insecurity make it possible to study potential relationships between fog and both of these constructs, which are fundamental to human wellbeing (Young et al., 2019; Brewis et al., 2020). Thus the potential links between fog and public health are multiple, under-studied, and ripe for advancement.
2.8 Indigenous perspectives on and responses to fog
Across cultures, people see fog as an important factor impacting their wellbeing, navigational abilities, and environments. Environmental anthropologists focus on how humans experience climate change and respond to changing environments, particularly in ways that impact their wellbeing, local ecosystem processes, and climate change itself. Traditional ecological knowledge (TEK), also known as Indigenous local knowledge, is of particular interest because it is based on thousands of years of close co-existence with local ecologies.
In a recent collaborative project, local indicators of climate change were collected by anthropologists through qualitative interviews and focus groups at 48 sites globally, across different climate zones and livelihood strategies, with clouds and fog identified as climate-impacted elements in 15 sites (Reyes-García et al., 2023, 2024a, b). This collaboration is a model for future studies that aim to collect fog-related TEK in western coastal settings.
Integrating TEK into fog research can be accomplished through forms of community-based participatory research that are co-led by Indigenous councils, elders, and researchers, and by ensuring that non-indigenous researchers involved in such partnerships are sensitive to the multiple meanings that fog can carry. Human experiences and responses are mediated by meanings, and entities carry multiple meanings, adding complexity to social-environmental systems. Fog has been an important entity for Indigenous North Americans since time immemorial, with roles in stories about the sea, salmon, and human gender relations. Indigenous Canadians tell a story of a woman whose brothers did not allow her to “be who she truly is,” a sea lover and swimmer. Raven made fog so she could disappear into the sea and be left alone (King, 2021). Tlingit peoples maintain a story in which Raven’s wife ran away, escaping because “she was the fog.” She ran into the water, and all the salmon she had dried followed her (Alaska Native Knowledge Network, 2006).
Fog is known by Indigenous peoples to keep waters cool and better oxygenated for salmon, supplemented by controlled fires to create smoke, which also blocks sun from overheating waters and maintains oak savanna (National Park Service, 2023). Indigenous Californians and Nevadans recognized that long-lasting winter fog impacts respiratory health, referring to it as pogonip or “white death” (Alonzo, 2019). Fog is also a metaphor for forgetting trauma, as in the documentary “The Thick Dark Fog” (Vasquez, 2011) examining Indigenous North Americans’ memories of abuse at childhood boarding schools, and as a metaphorical barrier created by settler colonialism preventing Indigenous peoples from learning and knowing the orature of their ancestors (Park, 2023).
To further illuminate perspectives on fog among Indigenous peoples, research should combine archival, ethnohistorical, and ethnographic research in close collaboration with Tribes, First Nations and other Indigenous groups. Recent scholarship offers guidelines for inclusive science that contributes to climate change adaptation efforts in ways that recognize histories of settler colonialism and reduce negative impacts of climate change on vulnerable peoples (Hernandez, 2022).
2.9 Forestry, agriculture, and fisheries
Agriculture is strongly tied to the coastal fog belt’s influence on species distributions (Franklin and Dyness, 1988). In the PNW coastal fog zone, Sitka spruce, western hemlock (Tsuga heterophylla) and western redcedar (Thuja plicata) dominate, transitioning to Douglas-fir further inland. However, higher-value Douglas-fir is the preferred forest plantation species, even in the fog zone, where it overlaps with prevalence of Swiss needle cast infestations that reduce the tree’s productivity and economic value (2.6). In California, coastal redwood is the preferred plantation species in the fog belt because of its high timber value as a timber species and natural association with fog. Food crop agriculture benefits from dry-season fog immersion, increasing water (+50%) and light (+70%) use efficiency in commercial farms (Baguskas et al., 2018). By increasing stream base flow and reducing water temperatures in the warm, dry season, fog cover positively impacts salmon productivity (Sawaske and Freyburg, 2015; Torregrosa et al., 2019). Salmon support a robust fisheries, culinary, and tourism industry across the region and have since time immemorial been a keystone species for North America’s west coast Indigenous peoples and ecosystems (Colombi and Brooks, 2012) but face multiple population pressures, including increased water temperatures (Wainwright and Weitkamp, 2013), that could be further affected by changing fog cover.
3 Conclusion and future collaborative perspective
Nearly all aspects of the coastal socio-environmental system are linked to fog. Its initial physical formation over the ocean and movement landward leads to fog as an integral part in the ecohydrological cycle of coastal ecosystems, with heightened importance during dry seasons, maintains a prominent role in Indigenous experience, and impacts agriculture, health, and conservation. A widespread, collaborative approach is needed to build these socio-environmental linkages across disciplines and peoples, many of which are discussed here, combining disciplinary knowledge and experience bases to tackle the shared challenges that will push fog science forward.
Data availability statement
The original contributions presented in the study are included in the article/supplementary material, further inquiries can be directed to the corresponding authors.
Author contributions
AD: Conceptualization, Supervision, Visualization, Writing–original draft, Writing–review and editing. SR: Conceptualization, Visualization, Writing–original draft, Writing–review and editing, Supervision. Sd: Conceptualization, Writing–original draft, Writing–review and editing. AI: Conceptualization, Writing–original draft, Writing–review and editing. YJ: Conceptualization, Writing–original draft, Writing–review and editing. JK: Writing–original draft, Writing–review and editing, Conceptualization. MK: Writing–original draft, Writing–review and editing, Conceptualization. KM: Writing–original draft, Writing–review and editing, Conceptualization. LO: Writing–original draft, Writing–review and editing, Conceptualization. KP: Writing–original draft, Writing–review and editing, Conceptualization. RS: Writing–original draft, Writing–review and editing, Conceptualization. DS: Writing–original draft, Writing–review and editing, Conceptualization. CS: Writing–original draft, Writing–review and editing, Conceptualization.
Funding
The author(s) declare that financial support was received for the research, authorship, and/or publication of this article. This research was supported jointly by Oregon Sea Grant, Earth Science Information Partners Water and Fire Program, USDA Forest Service Western Wildland Environmental Threat Assessment Center, Oregon Climate Service, and a USDA Forest Service Research Participation Program administered by the Oak Ridge Institute for Science and Education (ORISE) through an interagency agreement between the U.S. Department of Energy and the U.S. Department of Agriculture.
Conflict of interest
The authors declare that the research was conducted in the absence of any commercial or financial relationships that could be construed as a potential conflict of interest.
Publisher’s note
All claims expressed in this article are solely those of the authors and do not necessarily represent those of their affiliated organizations, or those of the publisher, the editors and the reviewers. Any product that may be evaluated in this article, or claim that may be made by its manufacturer, is not guaranteed or endorsed by the publisher.
References
Abdel-Aty, M., Ekram, A. A., Huang, H., and Choi, K. (2011). A study on crashes related to visibility obstruction due to fog and smoke. Accid. Analysis and Prev. 43, 1730–1737. doi:10.1016/j.aap.2011.04.003
Ait El Kadi, M., Bouchaou, L., Castelli, G., Re, V., Çakmakçı, Y., Bresci, E., et al. (2024). Multi-aspect assessment of operational fog collection systems: a rural development perspective, insights from the Sidi Ifni project in Morocco. J. Arid Environ. 222, 105174. doi:10.1016/j.jaridenv.2024.105174
Alaback, P. B. (1996). “Biodiversity patterns in relation to climate: the coastal temperate rainforests of North America,” in High-latitude rainforests and associated ecosystems of the west coast of the Americas. Editors R. G. Lawford, E. Fuentes, and P. B. Alaback (New York, NY: Springer), 116, 105–133. doi:10.1007/978-1-4612-3970-3_7
Alaska Native Knowledge Network (2006). Tlingit Indians of southeastern Alaska: raven and the fog woman. Available at: http://www.ankn.uaf.edu/iks/subsistence/tlingit/fogwoman.html (Accessed August 21, 2006).
Alonzo, A. (2019). Native Americans feared pogonip, the ‘white death. Reno Gazette-Journal. https://www.rgj.com/story/news/local/leader-courier/2019/12/06/native-americans-feared-pogonip-white-death/4363158002/.
Azeem, M., Noman, M. T., Wiener, J., Petru, M., and Louda, P. (2020). Structural design of efficient fog collectors: a review. Environ. Technol. and Innovation 20, 101169. doi:10.1016/j.eti.2020.101169
Baguskas, S. A., Clemesha, R. E. S., and Loik, M. E. (2018). Coastal low cloudiness and fog enhance crop water use efficiency in a California agricultural system. Agric. For. Meteorology 252, 109–120. doi:10.1016/j.agrformet.2018.01.015
Baguskas, S. A., King, J. Y., Fischer, D. T., D’Antonio, C. M., and Still, C. J. (2016). Impact of fog drip versus fog immersion on the physiology of Bishop Pine saplings. Funct. Plant Biol. 44, 339–350. doi:10.1071/FP16234
Baguskas, S. A., Oliphant, A. J., Clemesha, R. E. S., and Loik, M. E. (2021). Water and light-use efficiency are enhanced under summer coastal fog in a California agricultural system. J. Geophys. Res. Biogeosciences 126, e2020JG006193. doi:10.1029/2020JG006193
Baldocchi, D., and Waller, E. (2014). Winter fog is decreasing in the fruit growing region of the Central Valley of California. Geophys. Res. Lett. 41, 3251–3256. doi:10.1002/2014gl060018
Bassiouni, M., Scholl, M., Torres-Sanchez, A., and Murphy, S. F. (2017). A method for quantifying cloud immersion in a tropical mountain forest using time-lapse photography. Agric. For. Meteorology 243, 100–112. doi:10.1016/j.agrformet.2017.04.010
Becciu, P., Panuccio, M., Dell’Omo, G., and Sapir, N. (2021). Groping in the fog: soaring migrants exhibit wider scatter in flight directions and respond differently to wind under low visibility conditions. Front. Ecol. Evol. 9, 745002. doi:10.3389/fevo.2021.745002
Bendix, J., Thies, B., Nauss, T., and Cermak, J. (2006). A feasibility study of daytime fog and low stratus detection with TERRA/AQUA-MODIS over land. Meteorol. Appl. 13, 111–125. doi:10.1017/S1350482706002180
Berry, Z. C., and Goldsmith, G. R. (2020). Diffuse light and wetting differentially affect tropical tree leaf photosynthesis. New Phytol. 225, 143–153. doi:10.1111/nph.16121
Brewis, A., Workman, C., Wutich, A., Jepson, W., and Young, S.the Household Water Insecurity Experiences Research Consortium Network (HWISE-RCN) (2020). Household water insecurity is strongly associated with food insecurity: evidence from 27 sites in low- and middle-income countries. Am. J. Hum. Biol. 32, e23309. doi:10.1002/ajhb.23309
Burgess, S. S. O., and Dawson, T. E. (2004). The contribution of fog to the water relations of Sequoia sempervirens (D. Don): foliar uptake and prevention of dehydration. Plant, Cell and Environ. 27, 1023–1034. doi:10.1111/j.1365-3040.2004.01207.x
Carbone, M. S., Williams, A. P., Ambrose, A. R., Boot, C. M., Bradley, E. S., Dawson, T. E., et al. (2013). Cloud shading and fog drip influence the metabolism of a coastal pine ecosystem. Glob. Change Biol. 19, 484–497. doi:10.1111/gcb.12054
Castelli, G., Cuni Sanchez, A., Mestrallet, A., Montaño, L. C., López de Armentia, T., Salbitano, F., et al. (2023). Fog as unconventional water resource: mapping fog occurrence and fog collection potential for food security in Southern Bolivia. J. Arid Environ. 208, 104884. doi:10.1016/j.jaridenv.2022.104884
Certini, G., Castelli, G., Bresci, E., Calamini, G., Pierguidi, A., Paredes, L. N. V., et al. (2019). Fog collection as a strategy to sequester carbon in drylands. Sci. Total Environ. 657, 391–400. doi:10.1016/j.scitotenv.2018.12.038
Clemesha, R. E. S., Gershunov, A., Iacobellis, S. F., and Cayan, D. R. (2017). Daily variability of California coastal low cloudiness: a balancing act between stability and subsidence. Geophys. Res. Lett. 44, 3330–3338. doi:10.1002/2017GL073075
Clemesha, R. E. S., Gershunov, A., Iacobellis, S. F., Williams, A. P., and Cayan, D. R. (2016). The northward march of summer low cloudiness along the California Coast. Geophys. Res. Lett. 43, 1287–1295. doi:10.1002/2015GL067081
Colombi, B. J., and Brooks, J. F. (2012). Keystone Nations: indigenous peoples and salmon across the North Pacific. Santa Fe, NM: School for Advanced Research Press, 336.
Correggiari, M., Castelli, G., Bresci, E., and Salbitano, F. (2017). Fog collection and participatory approach for water management and local development: practical reflections from case studies in the atacama drylands BT – water and land security in drylands: response to climate change. In M. Ouessar, D. Gabriels, A. Tsunekawa, and S. Evett (Eds.) (pp. 141–158). Cham: Springer International Publishing.
Dawson, T. (1998). Fog in the California redwood forest: ecosystem inputs and use by plants. Oecologia 117, 476–485. doi:10.1007/s004420050683
del Río, C., Lobos, F., Siegmund, A., Tejos, C., Osses, P., Huaman, Z., et al. (2021). GOFOS, ground optical fog observation system for monitoring the vertical stratocumulus-fog cloud distribution in the coast of the Atacama Desert, Chile. J. Hydrology 597, 126190. doi:10.1016/j.jhydrol.2021.126190
Dong, W., Hou, D., Hou, Q., Jin, H., Li, F., and Wu, S. (2024). Effects of ultraviolet light stress on protective and detoxification enzymes in insects. Trop. Plants 3, 0. doi:10.48130/tp-0024-0008
Dye, A. W., Kim, J. B., McEvoy, A., Fang, F., and Riley, K. L. (2021). Evaluating rural Pacific Northwest towns for wildfire evacuation vulnerability. Nat. Hazards 107, 911–935. doi:10.1007/s11069-021-04615-x
Dye, A. W., Rastogi, B., Clemesha, R. E. S., Kim, J. B., Samelson, R. M., Still, C. J., et al. (2020). Spatial patterns and trends of summertime low cloudiness for the Pacific Northwest, 1996–2017. Geophys. Res. Lett. 47, e2020GL088121. doi:10.1029/2020GL088121
Dye, A. W., Reilly, M. J., McEvoy, A., Lemons, R., Riley, K. L., Kim, J. B., et al. (2024). Simulated future shifts in wildfire regimes in moist forests of Pacific Northwest, USA. JGR Biogeosciences 129, e2023JG007722. doi:10.1029/2023JG007722
Emery, N. C., D'Antonio, C. M., and Still, C. J. (2018). Fog and live fuel moisture in coastal California shrublands. Ecosphere 9, e02167. doi:10.1002/ecs2.2167
Evans, S. E., Dueker, M. E., Logan, J. R., and Weathers, K. C. (2019). The biology of fog: results from coastal Maine and Namib Desert reveal common drivers of fog microbial composition. Sci. Total Environ. 647, 1547–1556. doi:10.1016/j.scitotenv.2018.08.045
Fernandez, D. M., Torregrosa, A., Weiss-Penzias, P. S., Zhang, B. J., Sorensen, D., Cohen, R. E., et al. (2018). Fog water collection effectiveness: mesh intercomparisons. Aerosol Air Qual. Res. 18 (1), 270–283. doi:10.4209/aaqr.2017.01.0040
Fernando, H. J. S., Gultepe, I., Dorman, C., Pardyjak, E., Wang, Q., Hoch, S. W., et al. (2021). C-Fog: life of coastal fog. Bull. Am. Meteorological Soc. 102, E244–E272. doi:10.1175/BAMS-D-19-0070.1
Fischer, D. T., Still, C. J., and Williams, A. P. (2009). Significance of summer fog and overcast for drought stress and ecological functioning of Coastal California endemic plant species. J. Biogeogr. 36, 783–799. doi:10.1111/j.1365-2699.2008.02025.x
Franklin, J. F., and Dyness, C. T. (1988). Natural vegetation of Oregon and Washington. Corvallis, Oregon: Oregon State University Press.
Fultz, A. J., and Ashley, W. S. (2016). Fatal weather-related general aviation accidents in the United States. Phys. Geogr. 37, 291–312. doi:10.1080/02723646.2016.1211854
Gershunov, A., Johnson, Z., Margolis, H. G., and Guirguis, K. (2011). The California heat wave 2006 with impacts on statewide medical emergency: a space–time analysis. Geogr. Res. Forum 31, 53–69. https://grf.bgu.ac.il/index.php/GRF/article/view/381.
Gonzales, F., Craven, D., and Armesto, J. (2023). Islands in the mist: a systematic review of the coastal lomas of South America. J. Arid Environ. 104942, 104942. doi:10.1016/j.jaridenv.2023.104942
Gultepe, I. (2023). A review on weather impact on aviation operations: visibility, wind, precipitation, icing. J. Airl. Operations Aviat. Manag. 2 (1), 1–44. doi:10.56801/jaoam.v2i1.1
Hackney, J. D., Linn, W. S., and Avol, E. L. (1985). Potential risks to human respiratory health from ‘acid fog’: evidence from experimental studies of volunteers. Environ. Health Perspect. 63, 57–61. doi:10.1289/ehp.856357
Harr, R. D. (1982). Fog drip in the bull run municipal watershed, Oregon. JAWRA J. Am. Water Resour. Assoc. 18, 785–789. doi:10.1111/j.1752-1688.1982.tb00073.x
Hernandez, J. (2022). Fresh banana leaves: healing indigenous landscapes through indigenous science. Berkeley, CA: North Atlantic Books.
Hunova, I., Brabc, M., Geletic, J., Maly, M., and Dumitrescu, A. (2021). Statistical analysis of the effects of forests on fog. Sci. Total Environ. 781, 146675. doi:10.1016/j.scitotenv.2021.146675
Johnson, K. N., Franklin, J. F., and Reeves, G. F. (2023). The making of the northwest forest plan: the wild science of saving old growth ecosystems. Corvallis, OR: Oregon State University Press, 472.
Johnstone, J. A., and Dawson, T. E. (2010). Climatic context and ecological implications of summer fog decline in the Coast Redwood region. Proc. Natl. Acad. Sci. 107, 4533–4538. doi:10.1073/pnas.0915062107
Kawai, H., Koshiro, T., Endo, H., and Arakawa, O. (2018). Changes in marine fog over the North Pacific under different climates in CMIP5 multimodel simulations. J. Geophys. Res. Atmos. 123, 10911–10924. doi:10.1029/2018JD028899
King, N. (2021). The coming of fog (A raven tale from indigenous peoples of northwestern Canada). Available at: https://www.nancykingstories.com/world-tales/the-coming-of-fog-a-raven-tale-from-indigenous-peoples-of-northwestern-canada.
Koračin, D. (2017). “Modeling and forecasting marine fog,” in Marine fog: challenges and advancements in observations, modeling, and forecasting. Editors D. Koračin, and C. E. Dorman (Springer), 477–509.
Koračin, D., Dorman, C. E., Lewis, J. M., Hudson, J. G., Wilcox, E. M., and Torregrosa, A. (2014). Marine fog: a review. Atmos. Res. 143, 142–175. doi:10.1016/j.atmosres.2013.12.012
Koračin, D., Leipper, D. F., and Lewis, J. M. (2005). Modeling sea fog on the U.S. California coast during a hot spell event. Geofizika 22, 59–82. https://hrcak.srce.hr/111.
Krawchuk, M. A., Meigs, G. W., Cartwright, J. M., Coop, J. D., Davis, R., Holz, A., et al. (2020). Disturbance refugia within mosaics of forest fire, drought, and insect outbreaks. Front. Ecol. Environ. 18, 235–244. doi:10.1002/fee.2190
Leipper, D. F. (1948). Fog development at San Diego, California. J. Mar. Res. 7, 337–346. https://elischolar.library.yale.edu/journal_of_marine_research/673.
Leipper, D. F. (1994). Fog on the US west coast: a review. Bull. Am. Meteorological Soc. 75, 229–240. doi:10.1175/1520-0477(1994)075<0229:fotuwc>2.0.co;2
Limm, E. B., Simonin, K. A., Bothman, A. G., and Dawson, T. E. (2009). Foliar water uptake: a common water acquisition strategy for plants of the redwood forest. Oecologia 161, 449–459. doi:10.1007/s00442-009-1400-3
Lundsgaard, N. U., Cramp, R. L., Franklin, C. E., and Martin, L. (2020). Effects of ultraviolet-B radiation on physiology, immune function and survival is dependent on temperature: implications for amphibian declines. Conserv. Physiol. 8, coaa002. doi:10.1093/conphys/coaa002
Manter, D. K., Reeser, P. W., and Stone, J. K. (2005). A climate-based model for predicting geographic variation in Swiss Needle Cast severity in the Oregon Coast Range. Phytopathology 95, 1256–1265. doi:10.1094/PHYTO-95-1256
Meddens, A. J., Kolden, C. A., Lutz, J. A., Smith, A. M., Cansler, C. A., Abatzoglou, J. T., et al. (2018). Fire refugia: what are they, and why do they matter for global change? BioScience 68, 944–954. doi:10.1093/biosci/biy103
Naficy, C. E., Meigs, G. W., Gregory, M. J., Davis, R., Bell, D. M., Dugger, K., et al. (2021). Fire refugia in old-growth forests—final report to the USGS northwest climate adaptation center. Corvallis, OR: Oregon State University, 39.
National Park Service (2023). Fog, redwoods, and a changing climate. Available at: https://www.nps.gov/articles/000/fog-redwoods-and-a-changing-climate.htm (Accessed January 23, 2023).
O’Brien, T. A., Sloan, L. C., Chuang, P. Y., Faloona, I. C., and Johnstone, J. A. (2013). Multidecadal simulation of coastal fog with a regional climate model. Clim. Dyn. 40, 2801–2812. doi:10.1007/s00382-012-1486-x
Ojani, C. (2024). Ecology of capture: creating land titles out of thin air in coastal Peru. Ethnos 89 (1), 80–99. doi:10.1080/00141844.2021.1965643
Oliphant, A. J., Dragoni, D., Deng, B., Grimmond, C. S. B., Schmid, H.-P., and Scott, S. L. (2011). The role of sky conditions on gross primary production in a mixed deciduous forest. Agric. For. Meteorology 151, 781–791. doi:10.1016/j.agrformet.2011.01.005
Park, E. Y. (2023). “Review: in prophecy fog, jani lauzon reclaims the giant rock.” Intermission Mag. Available at: https://www.intermissionmagazine.ca/reviews/prophecy-fog-coal-mine/ (Accessed November 18, 2023).
Ran, Y., Ma, H., Liu, Z., Wu, X., Li, Y., and Feng, H. (2002). Satellite fog detection at dawn and dusk based on the deep learning algorithm under terrain-restriction. Remote Sens. 14, 4328. doi:10.3390/rs14174328
Reilly, M. J., Glavich, D. A., Acker, S. A., Kearns, H. S. J., Willhite, E. A., Kerns, B. K., et al. (2024). “Climate, disturbance, and vegetation change in the Oregon Coast,” in Climate change vulnerability and adaptation in coastal Oregon. Gen. Tech. Rep. PNW-GTR-xxx. Editor J. Halofsky, and et al. (Portland, OR: U.S. Department of Agriculture, Forest Service, Pacific Northwest Research Station).
Reyes-García, V., Álvarez-Fernandez, S., Benyei, P., García-del-Amo, D., Junqueira, A., Labeyrie, V., et al. (2023). Local indicators of climate change impacts described by Indigenous Peoples and local communities: study protocol. PLOS One 18, e0279847. doi:10.1371/journal.pone.0279847
Reyes-García, V., García-del-Amo, D., Álvarez-Fernández, S., Benyei, P., Calvet-Mir, L., Junqueira, A. B., et al. (2024a). Indigenous Peoples and local communities report ongoing and widespread climate change impacts on local social-ecological systems. Commun. Earth and Environ. 5, 29–10. doi:10.1038/s43247-023-01164.y
Reyes-García, V., García-Del-Amo, D., Porcuna-Ferrer, A., Schlingmann, A., Abazeri, M., Attoh, E. M. N. A. N., et al. (2024b). Local studies provide a global perspective of the impacts of climate change on Indigenous Peoples and local communities. Sustain. Earth Rev. 7, 1–11. doi:10.1186/S42055-023-00063-6
Rivas, D., and Samelson, R. M. (2011). A numerical modeling study of the upwelling source waters along the Oregon coast during 2005. J. Phys. Oceanogr. 41, 88–112. doi:10.1175/2010JPO4327.1
Samelson, R. M., de Szoeke, S. P., Skyllingstad, E. D., Barbour, P. L., and Durski, S. M. (2021). Fog and low-level stratus in coupled ocean-atmosphere simulations of the northern California Current System upwelling season. Mon. Weather Rev. 149, 1593–1617. doi:10.1175/MWR-D-20-0169.1
Sawaske, S. R., and Freyberg, D. L. (2015). Fog, fog drip, and streamflow in the Santa cruz mountains of the California coast range. Ecohydrology 8, 695–713. doi:10.1002/eco.1537
Shaw, D. C., Ritókova, G., Lan, Y.-H., Mainwaring, D. B., Russo, A., Comeleo, R., et al. (2021). Persistence of the Swiss Needle Cast outbreak in Oregon coastal Douglas-Fir and new insights from research and monitoring. J. For. 119, 407–421. doi:10.1093/jofore/fvab011
Shi, C., and Liu, H. (2021). How plants protect themselves from ultraviolet-B radiation stress. Plant Physiol. 187, 1096–1103. doi:10.1093/plphys/kiab245
Snyder, R. L., and de Melo-Abreu, J. P. (2005). “Frost protection,” in Fundamentals, practice, and economics, Vols. 1 and 2. Rome: UN FAO.
Spies, T. A., Hessburg, P. F., Skinner, C. N., Puettmann, K. J., Reilly, M. J., Davis, R. J., et al. (2018). “Chapter 3: old growth, disturbance, forest succession, and management in the area of the Northwest Forest Plan,” in Synthesis of science to inform land management within the northwest forest plan area. Gen. Tech. Rep. PNW-GTR-966. Editors T. Spies, and et al. (Portland, OR: U.S. Department of Agriculture, Forest Service, Pacific Northwest Research Station), 95–243.
Still, C. J., Riley, W. J., Biraud, S. C., Noone, D. C., Buenning, N. H., Randerson, J. T., et al. (2009). Influence of clouds and diffuse radiation on ecosystem-atmosphere CO2 and CO 18O exchanges. J. Geophys. Res. 114, G01018. doi:10.1029/2007JG000675
Still, C. J., Sibley, A., DePinte, D., Busby, P. E., Harrington, C. A., Schulze, M., et al. (2023). Causes of widespread foliar damage from the June 2021 Pacific Northwest Heat Dome: more heat than drought. Tree Physiol. 43, 203–209. doi:10.1093/treephys/tpac143
Sydeman, W. J., García-Reyes, M., Schoeman, D. S., Rykaczewski, R. R., Thompson, S. A., Black, B. A., et al. (2014). Climate change and wind intensification in coastal upwelling ecosystems. Science 345, 77–80. doi:10.1126/science.1251635
Torregrosa, A., Combs, C., and Peters, J. (2016). GOES-derived fog and low cloud indices for coastal North and Central California ecological analyses. Earth Space Sci. 3, 46–67. doi:10.1002/2015EA000119
Torregrosa, A., Flint, L. E., and Flint, A. L. (2019). Hydrologic resilience from summertime fog and recharge: a case study for coho salmon recovery planning. J. Am. Water Resour. Assoc. 56, 134–160. doi:10.1111/1752-1688.12811
Torregrosa, A., O’Brien, T. A., and Faloona, I. C. (2014). Coastal fog, climate change, and the environment. Eos 95, 473–474. doi:10.1002/2014EO500001
Wainwright, T. C., and Weitkamp, L. A. (2013). Effects of climate change on Oregon Coast coho salmon: habitat and life-cycle interactions. Northwest Sci. 87, 219–242. doi:10.3955/046.087.0305
Weathers, K. C., Ponette-González, A. G., and Dawson, T. (2020). Medium, vector, and connector: fog and the maintenance of ecosystems. Ecosystems 23, 217–229. doi:10.1007/s10021-019-00388-4
Weiss-Penzias, P. S., Bank, M. S., Clifford, D. L., Torregrosa, A., Zheng, B., Lin, W., et al. (2019). Marine fog inputs appear to increase methylmercury bioaccumulation in a coastal terrestrial food web. Sci. Rep. 9, 17611. doi:10.1038/s41598-019-54056-7
Williams, A. P., Gentine, P., Moritz, M. A., Roberts, D. A., and Abatzoglou, J. T. (2018). Effect of reduced summer cloud shading on evaporative demand and wildfire in Coastal Southern California. Geophys. Res. Lett. 45, 5653–5662. doi:10.1029/2018GL077319
Wimberley, M. C. (2002). Spatial simulation of historical landscape patterns in coastal forests of the Pacific Northwest. Can. J. For. Res. 32, 1316–1328. doi:10.1139/x02-054
Young, S., Boateng, G., Jamaluddine, Z., Miller, J., Frongillo, E., Neilands, T., et al. (2019). The Household Water InSecurity Experiences (HWISE) Scale: development and validation of a household water insecurity measure for low-income and middle-income countries. BMJ Glob. Health 4, e001750. doi:10.1136/bmjgh-2019-001750
Keywords: atmosphere, biogeography, collaboration, FOG, interdisciplinary science, Pacific Northwest, socio-ecological systems, west coast
Citation: Dye A, Rauschenbach S, de Szoeke S, Igel AL, Jin Y, Kim JB, Krawchuk MA, Maes K, O’Neill L, Paw U KT, Samelson R, Shaw DC and Still C (2024) Fog in western coastal ecosystems: inter-disciplinary challenges and opportunities with example concepts from the Pacific Northwest, USA. Front. Environ. Sci. 12:1488401. doi: 10.3389/fenvs.2024.1488401
Received: 29 August 2024; Accepted: 24 October 2024;
Published: 06 November 2024.
Edited by:
Folco Giomi, Independent Researcher, Padova, ItalyReviewed by:
Charles Jones, Department of Geography, University of California, Santa Barbara, United StatesGiulio Castelli, University of Florence, Italy
Musaddaq Azeem, University of Leeds, United Kingdom
Copyright © 2024 Dye, Rauschenbach, de Szoeke, Igel, Jin, Kim, Krawchuk, Maes, O’Neill, Paw U, Samelson, Shaw and Still. This is an open-access article distributed under the terms of the Creative Commons Attribution License (CC BY). The use, distribution or reproduction in other forums is permitted, provided the original author(s) and the copyright owner(s) are credited and that the original publication in this journal is cited, in accordance with accepted academic practice. No use, distribution or reproduction is permitted which does not comply with these terms.
*Correspondence: Alex Dye, YWxleC5keWVAb3JlZ29uc3RhdGUuZWR1; Sonya Rauschenbach, c3JhdXNjaGVuYmFjaEB1Y2RhdmlzLmVkdQ==