- 1Key Laboratory of Plant Hormones and Molecular Breeding of Chongqing, School of Life Sciences, Chongqing University, Chongqing, China
- 2Rice Research Institute, Nanchong Academy of Agricultural Sciences, Nanchong, China
- 3Department of Agriculture and Environmental Sciences and Cooperative Research, Lincoln University of Missouri, Jefferson City, MO, United States
- 4Laboratory of Legumes and Sustainable Agrosystems, Centre of Biotechnology of Borj-Cedria, Hammam-Lif, Tunisia
Cadmium (Cd) contamination of soil threatens human health, food security, and ecosystem sustainability. The in situ stabilization of Cd has been recognized as a potentially economical technology for the remediation of Cd-contaminated soil. Recently, biochar (BC) and activated carbon (AC) have received widespread attention as eco-friendly soil amendments that are more beneficial for plant growth, soil health, and remediation of contaminated soil. An experiment was performed in a paddy field to investigate the effects of two different types of BC (maize straw biochar and bamboo biochar) and AC (coconut shell activated carbon) in combination with rape organic fertilizer (R), calcium magnesium phosphate fertilizer (P), and fulvic acid (F), respectively, on soil Cd immobilization, Cd accumulation in rice, and yield. The results indicated that the BC/AC-based amendments reduced soil bioavailable Cd (DTPA-Cd) and brown rice Cd by 9.58%–27.06% and 19.30%–71.77%, respectively. The transformation of exchangeable Cd (Ex-Cd) to carbonate-bound Cd (Ca-Cd), Fe-Mn oxide bond (Ox-Cd), and residual (Re-Cd) in soil accounted for the mitigation of Cd uptake and enrichment by rice. Additionally, BC-/AC-based amendments altered soil physicochemical properties, which significantly increased the soil pH and cation exchange capacity (CEC), total nitrogen (TN), total phosphorus (TP), soil organic carbon (SOC), and dissolved organic carbon (DOC), directly promoting soil health. All BC-/AC-based amendments significantly increased FeIMP and MnIMP concentrations by 47.31%–160.34% and 25.72%–73.09% in the Fe/Mn plaque (IMP), respectively. Maize straw and bamboo biochar-based amendments significantly increased rice yield by 10.46%–20.41% and 9.94%–16.17%, respectively, while coconut shell-activated carbon severely reduced rice yield by 65.06%–77.14%. The correlation analysis revealed that leaf Cd and IMP primarily controlled Cd uptake by rice, and soil pH, Eh, CEC, SOC, IMP, and TP influenced DTPA-Cd in soil. This field study demonstrated that maize straw and bamboo biochar-based amendments not only reduced soil DTPA-Cd in paddy fields but also decreased the accumulation of Cd in brown rice, as well as improved rice yield, which has potential application in Cd-contaminated agriculture fields. Coconut shell-activated carbon severely decreased rice yields, which is not appropriate for rice production.
1 Introduction
As a highly hazardous heavy metal, cadmium (Cd) poses a serious threat to the health of ecosystems and growth of plants (Liang Q. et al., 2022a). Human health and global food security are significantly impacted by Cd due to its bioaccumulation in the food chain through the soil–plant–human channel (Li J. et al., 2022b; Liang T. et al., 2022b). Human consumption of Cd-enriched crops, such as rice, vegetables, and wheat, for long periods may lead to a series of health problems like cancer, neurological diseases, renal disease, and damage to the skeleton (Chaney, 2015; Awual et al., 2018). Large-scale release of Cd into the soil might result from human-related activities such as mining industries, manufacturing, disposal of wastewater, and sewage irrigation (Haris et al., 2021). The long-term improper application of chemical fertilizers and pesticides is also an important factor leading to increased bioavailable Cd (DTPA-Cd) in agricultural fields (Mahar et al., 2016; Rafique et al., 2020). Cd contamination accounts for the largest contaminated lands in China, especially in southern and southwestern China, accounting for approximately 52.6% of the metal-contaminated lands and 74.3% of the polluted lands (Lv et al., 2021). Remediation of Cd in agricultural soil is an economically challenging process due to the high costs of removing Cd from the soil. Currently, in situ immobilization technology using soil amendments is considered to be an effective method for the remediation of Cd-contaminated soils (Wu et al., 2019). Therefore, it is urgent to explore a sustainable and environmentally friendly soil amendment to effectively reduce the Cd bioavailability in agricultural soils, improve soil condition, and increase crop yield.
Recently, biochar has been increasingly being applied to mitigate heavy-metal pollution in agricultural fields (Břendová et al., 2016; Chen et al., 2021). Biochar is generally produced by pyrolysis of biomass (agricultural waste, wood, herbs, fruit shells, etc.) under oxygen-restricted or non-oxygenated environments at temperatures between 400°C and 700°C (Blanco-Canqui, 2017). Biochar has a relatively high speculative surface and a wide pore-size distribution, with the surface containing a considerable number of negatively charged chemical functional groups (-OH, -COOH, C=O, S2−, CO32−, PO43−, etc.), which can enhance the absorption, complexation, and precipitation of Cd in soil (He et al., 2019; Ibrahim et al., 2020; Nguyen et al., 2022). Biochar is mainly used for soil improvement, remediation of heavy metal-contaminated agricultural fields, and enhancement of soil fertility. Yuan et al. (2023) showed that rape straw biochar remarkably enhanced soil pH and promoted Cd adsorption in iron minerals through increased iron hydroxylation and recrystallization processes. Chen et al. (2022) researched the influence of rice biochar applied to acidic soils on Cd and Cu uptake and found the maximum reduction in brown rice Cd and Cu by 58.1% and 20.8%, respectively. Additionally, prepared fruit shell-based biochar is re-activated by pyrolysis with activators (steam, carbon dioxide, or chemical reagents) under non-oxygenated conditions to form activated carbon (Golia et al., 2022). Activated carbon has a higher porosity structure, including micropores, mesopores, and macropores, which provide a higher specific surface area in favor of enhanced adsorption performance, and those surface functional group categories are closely related to the types of activators (Aborisade et al., 2022). Currently, a few studies have shown that activated carbon has an excellent remediation effect on heavy metal-contaminated soils. Břendová et al. (2016) showed that applied coconut shell-activated carbon decreased the accumulation of Cd (by 57% on average), Pb (by 44% on average), and Zn (by 44% on average), as well as increased biomass in spinach (Spinacia oleracea L.) and mustard (Sinapis alba L.). Wei et al. (2022) showed that applied bacterium-loaded coconut shell-activated carbon reduced DTPA-Cd and Pb in soil by 25.1% and 42.65%, respectively, and also significantly reduced Cd and Pb content in the shoot and root of pak choi (Brassica chinensis L.).
Co-applications of biochar (BC)/activated carbon (AC) with other materials (such as lime, organic fertilizers, urea, calcium magnesium phosphate fertilizer, and fulvic acid) were reported not only to reduce Cd uptake by plants but also increase crop yield. Hong et al. (2022) reported that the combined application of seaweed-derived fertilizer with biochar and apatite reduced the concentration of Cd in maize kernels by 68.9%. Liu et al. (2022) showed that, in comparison with a single biochar or biochar–lime application, the biochar combined with compost was more highly efficient in reducing Cd transfer and bioaccumulation in vegetables. Liang T. et al. (2022b) revealed that the co-application of rape-derived biochar, rice-derived biochar, and Chinese milk vetch-derived biochar decreased the Cd concentration in rice grain by 65.38%. Liu et al. (2020) showed that applying biochar with a phosphate fertilizer markedly decreased Cd concentrations in alfalfas. Yin et al. (2022) showed that the combined application of spermidine and activated carbon removed the efficiency by 78.11%–120.86% of Cd and increased dry mass by 39.65%–92.95%.
The materials we selected to be incorporated into BC/AC were organic fertilizers, calcium magnesium phosphate fertilizer (P), and fulvic acid (F) to prepare BC/AC-based amendments. The BC/AC mixture not only provided chemical chelates for metals to reduce metal bioavailability in soil but also improved soil physicochemical properties and nutrient status. Based on previous reports, it was found that there are few studies reported on this topic. Therefore, it is critical to study the effective BC-/AC-based amendments by measuring the indicators of Cd absorption by rice and changes in soil Cd bioavailability. The targets of this research were to i) explore the effects of various ratios of composite BC-/AC-based amendments on soil physicochemical properties and DTPA-Cd; ii) examine the impacts of the composite BC-/AC-based amendments on rice performance, productivity, Cd uptake, and bioaccumulation; and iii) investigate the potential mechanisms to reduce Cd absorption and enrichment in rice. It was hypothesized that BC-/AC-based amendments would change the physicochemical properties and alter the Cd fractions of the soil, thereby reducing the Cd bioavailability in paddy soil and absorption by rice, leading to a higher yield.
2 Materials and methods
2.1 Field site
The rice field trial site was located in Pengan (31°07′54″N, 106°15′27″E) in Nanchong City, Sichuan Province, Southwest China (Figure 1). The topsoil of the field was purple sandy loam that has been used for the rape–rice rotation every year. Prior to the experiment, the physiochemical characteristics of the 0–20-cm topsoil were measured as follows: pH of 5.18 ± 0.12, soil redox potential (Eh) of 614.2 ± 26.4 mv, cation exchange capacity (CEC) of 13.19 ± 1.97 cmol/kg, total nitrogen (TN) of 0.96 ± 0.03 g/kg, total potassium (TK) of 6.25 ± 0.37 g/kg, total phosphorus (TP) of 0.24 ± 0.03 g/kg, soil organic carbon (SOC) of 8.84 ± 0.29 g/kg, soil dissolved organic carbon (DOC) of 60.90 ± 3.53 mg/kg, total soil Cd (TCd) of 0.32 ± 0.02 mg/kg, DTPA-Cd of 0.0741 ± 0.0057 mg/kg, and pollution index of 107. The TCd level of the paddy soil was over the standard value of 0.3 mg/kg (pH ≤ 5.5); thus, the soil was considered slightly Cd-contaminated.
2.2 Materials
The ingredients of BC-/AC-based amendments included biochar/activated carbon, rape organic fertilizer (R), calcium magnesium phosphate fertilizer (P), and fulvic acid (F), which were supplied by Henan Haopai Environmental Technology Co., Ltd., Shandong Ruifeng Trading., Ltd., and Jinan Hengyuan Chemical Co., Ltd., respectively. The rape organic fertilizer (R) was purchased from local agricultural markets. The rice cultivar tested was Fengyouxiangzhan (national authorized number rice 2003056) from Nanchong Academy of Agricultural Sciences. The maize straw and bamboo biochar were produced as follows: maize straw and bamboo were air-dried and crushed in a pulverizer and then fed into a carbonization furnace, which was heated at a rate of 10°C/min. Once the set conditions were reached, the temperature increase was stopped, and pyrolysis was carried out under anaerobic conditions at 500°C–600°C for 2.5 h. Coconut shell-activated carbon was prepared as follows: first, the coconut shell was dried at 70°C, then crushed into pulverized particles, and pyrolyzed at 500°C–600°C for 2.5 h to form coconut shell biochar. Second, the coconut shell biochar was mixed with phosphoric acid and then again pyrolyzed at 500°C–600°C for 2.5 to obtain coconut shell-activated carbon. Finally, it was washed and dried. All the prepared materials were passed through a 200-mesh sieve. The physicochemical properties of the materials are shown in Table 1.
2.3 Experimental design
The trial was performed in a rice field from March to September 2022, consisting of a fully randomized factorial design with three replications for each treatment. Two types of biochar were used, maize straw biochar (A) and bamboo biochar (B), and the activated carbon was coconut shell-activated carbon (AC). Three materials were mixed with rape organic fertilizer (R), calcium magnesium phosphate fertilizer (P), and fulvic acid (F), respectively, at various ratios. Ten treatments included control (CK); T1 = 1:3:0.5:0.5 (A1R3P0.5F0.5); T2 = 2:2:0.5:0.5 (A2R2P0.5F0.5); T3 = 3:1:0.5:0.5 (A3R1P0.5F0.5); T4 = 1:3:0.5:0.5 (B1R3P0.5F0.5); T5 = 2:2:0.5:0.5 (B2R2P0.5F0.5); T6 = 3:1:0.5:0.5 (B3R1P0.5F0.5); T7 = 1:3:0.5:0.5 (AC1R3P0.5F0.5); T8 = 2:2:0.5:0.5 (AC2R2P0.5F0.5); and T9 = 3:1:0.5:0.5 (AC3R1P0.5F0.5). There were 30 plots with a planting area of 20 m2 (6 m × 3.3 m) per plot. The amendment mixture was manually applied to each plot at a rate of 10 kg 5 days before rice transplanting, and simultaneously, the CK plot was supplemented with 1 kg calcium magnesium phosphate fertilizer and 1 kg fulvic acid. Each plot was separated by surrounding protective rows of 50 cm in width, with separate watering inlets and outlets for irrigation and drainage. The plot's edge was divided by a black plastic film to prevent water drainage. Selected rice seeds were sterilized with 2% sodium hypochlorite solution, washed with purified water, and then sown in the soil. Rice seedlings were transplanted to each plot having a population of 150,000 plants ha−1 on 1 May 2022, with rows and spacing of 33 cm and 20 cm, respectively. Before rice transplanting, each plot was applied with a fertilizer of N-P2O5-K2O (23-7-10, 810 kg ha−1) to provide sufficient nutrients required for rice growth. During the period of rice growth, local standard agronomic management practices for rice production were followed across plots, including irrigation, pesticide application, and fertilization.
2.4 Soil analysis
A sample of mixed soil was collected from the topsoil (20 cm) of each plot 3 days before harvest using the five-point sampling method. Furthermore, soil samples were taken on days 0, 30, 60, 90, and 135 for the determination of DTPA-Cd concentrations only. The soil samples collected were air-dried at 20°C–25°C, pulverized using a wooden hammer, and then sieved using a 2-mm sieve for soil testing. The pH of the soil was determined using a pH meter (PB-10, Sartorius, Germany) at a soil:water ratio (w/v) of 1:2.5; Eh was determined using an Eh meter (PRN-41, DKK-TOA Corporation, Japan); and CEC was determined by leaching the soil with ammonium acetate at pH 7, followed by Kjeltec auto-analyzer (forest soil method: LY/T 1243-1999). The other parameters were determined as follows: TN with the LY/T 1228-2015 method, TP by the LY/T 1232-2015 method, TK with the LY/T 1234-2015 method, SOC by digestion with potassium dichromate, DOC by ultra-pure water oscillation extraction, and TOC using an automatic organic carbon analyzer (vario TOC cube, Elementar, Germany). TCd in the soil was measured using an inductively coupled plasma emission spectrometer (ICP-OES; Thermo Fisher, United States) after digestion with HNO3-HF-HClO4. DTPA-Cd was extracted with diethylenetriaminepentaacetic acid (DTPA) (GB/T 23739-2009) and subsequently analyzed by ICP-OES. The fractions of Cd, including exchangeable Cd (Ex-Cd), carbonate-bound Cd (Ca-Cd), Fe-Mn oxide bond Cd (Ox-Cd), organic-bound Cd (Or-Cd), and residual Cd (Re-Cd), were measured following the methods proposed by Liu et al. (2022).
2.5 Plant analysis
At each stage of rice growth, rice basic seedling, highest seedling, tiller number, and plant height were recorded. Rice was harvested after 150 days, and the yield data were recorded. Then, 2 days before harvest, five rice plants with similar growth were collected from each plot; divided into roots, shoots, leaves, and panicles; and weighed for fresh biomass of each tissue. All tissue samples were then air-dried under sunlight and weighed for dry biomass. Effective panicles, filled grains, and empty grains of the five plants were counted, and effective panicles per hectare and filled-grain percentages were calculated. A total of 1,000 grains were weighed, and the panicle length was measured.
Before tissue Cd analysis, the plant samples collected were rinsed with deionized water, dried in an oven at 65°C for obtaining a consistent weight, then digested with HNO3-HClO4, and analyzed by ICP-OES. The dithionite–citrate–bicarbonate (DCB) method (Lin et al., 2017) was applied to extract the IMP on the fresh root surface. The bioconcentration factor (BCF) for rice root from the soil was computed using Equation 1 according to Liang T. et al. (2022b):
In addition, the translocation factor (TF), which is the capability of the crop to transfer Cd from the root to shoot, shoot to brown rice grain, or leaf to brown rice grain, was obtained using Equations 2–4, as reported by Liang T. et al. (2022b):
where Croot, Cshoot, Crice, and Cleaf are the Cd content in the root, shoot, rice grain, and leaf, respectively. Csoil was the total Cd concentrations in soil.
2.6 Statistical analysis
All data were calculated as an average of triplicate experiments and expressed as the mean ± standard error. The basic data were analyzed using Microsoft Excel 2016. One-way ANOVA was calculated using IBM SPSS Statistics version 25 (IBM Corp., Armonk, NY, United States). Significant differences among treatments were evaluated with the least significant difference (LSD) multiple comparisons test with a threshold value of p < 0.05. A correlation analysis was performed to evaluate the associations among soil physiochemical properties (pH, Eh, CEC, TN, TP, TK, SOC, and DOC) with DTPA-Cd and Cd concentrations in rice. Graphics were plotted using GraphPad Prism 9 (GraphPad Software, San Diego, United States), RStudio version 1.3.1093, and Adobe Illustrator 2022 (Adobe, San Jose, United States).
3 Results
3.1 Soil physiochemical properties
The changes in soil physiochemical properties by treatments, including pH, Eh, TN, TP, TK, CEC, SOC, DOC, and C/N ratio, are presented in Figure 2. Compared with CK, in all cases, the amendments increased the soil pH at a range of 0.03–0.39 units. T6, T3, and T5 showed a significant increase in soil pH by 0.39, 0.37, and 0.24 units (p < 0.05), respectively, while T9 and T8 resulted in the smallest increase by 0.03 and 0.06 units, respectively. Soil Eh was substantially decreased by treatments from 41.6 to 125.7 mV. T6, T5, T3, T2, and T9 significantly reduced soil Eh by 20.8%, 17.7%, 16.6%, 15.7%, and 13.8% (p < 0.05), respectively, while the increase by T7 and T8 was the least.
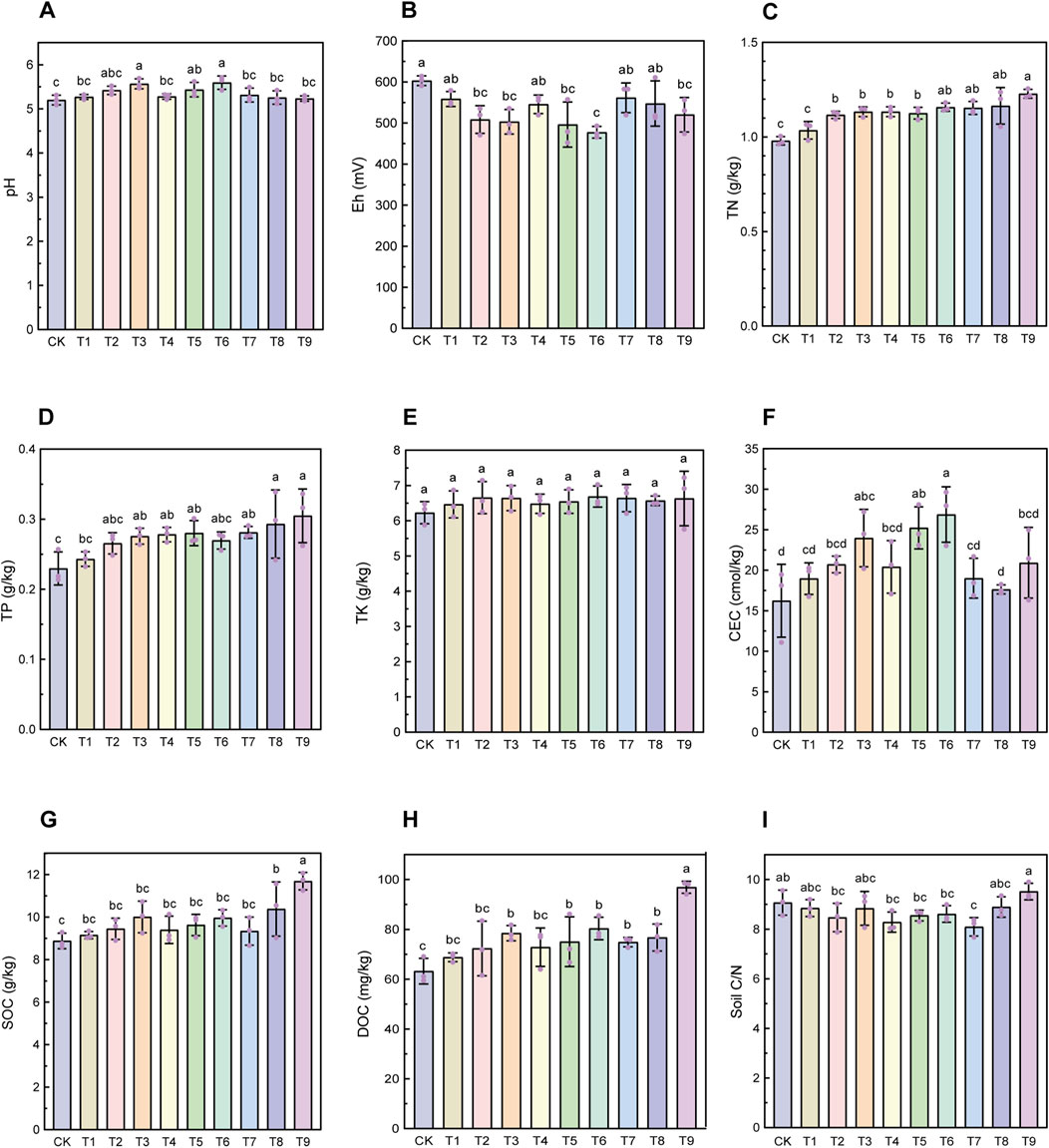
Figure 2. Effect of biochar-based amendments on soil physiochemical properties. Changes in soil pH (A), Eh (B), TN (C), TP (D), TK (E), CEC (F), SOC (G), DOC (H), and C/N ratio (I). Error bars represent the standard deviation of triplicates. Data are presented as the mean ± standard error (n = 3). The same lowercase letters mean no significant difference, while different lowercase letters mean a significant difference (ANOVA, LSD, p < 0.05).
The content of TN (Figure 2C) and TP (Figure 2D) showed a significant increase compared with CK, except for T1 (p < 0.05). T9 and T8 increased the soil TN by 25.3% and 18.8% and TP by 32.5% and 27.4%, respectively. There was no significant difference in TK (Figure 2E) among treatments (p > 0.05).
Treatments markedly improved soil CEC in the following order: T6 (98.3%) > T3 (80.6%) > T5 (73.2%) > T2 (62.9%) > T9 (43.7%) > T4 (40.2%) > T7 (30.5%) > T1 (30.2%) > T8 (21.1%) > CK. The treatments also substantially enhanced SOC (Figure 2G) and DOC (Figure 2H) (p < 0.05). The largest increases were caused by T9 , i.e., SOC from 8.88 to 11.69 g/kg. The soil C/N ratio (Figure 2I) was slightly decreased, except for T9 (9.52), compared with CK (9.07).
3.2 Fractionation of soil Cd
As shown in Figure 3, the treatments caused a significant reduction in soil Cd concentrations. The DTPA-Cd content of each treatment decreased rapidly in the first 60 days, increased slightly from 60 days to harvest, and then gradually stabilized.
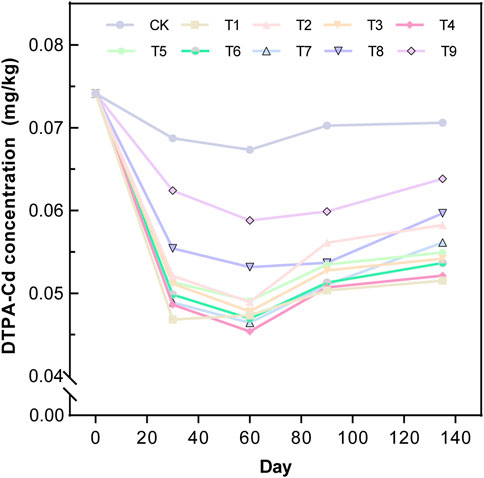
Figure 3. Effect of biochar-based amendments on dynamic changes in the soil DTPA-Cd concentration. Data are presented as the mean ± standard error (n = 3).
Compared to day 0, the reduction of BC-/AC-based amendments on day 60 ranged from 20.67% to 38.72%, with the CK also decreasing by 9.13%. After harvest, the highest DTPA-Cd content was 0.071 mg/kg in CK. DTPA-Cd in T1, T4, and T6 was significantly reduced by 27.06%, 26.19%, and 24.03% against CK (p < 0.05), respectively, whereas no significant difference was found between T3 (23.19%), T5 (22.15%), T7 (20.49%), T2 (17.54%), T8 (15.48%), and T9 (9.58%) (p > 0.05).
The sequential extraction of soil Cd indicated that, in all cases, Ex-Cd (Figure 4A) was significantly decreased by treatments in a range of 8.60%–46.90% (Figure 4). The reduction by T3, T6, and T2 was 46.90%, 45.45%, and 43.45% (p < 0.05), respectively. Ca-Cd (Figure 4B) was increased by the treatments in a range of 11.50%–44.90%, while the reduction by T7, T8, and T9 was observed. On the other hand, Ox-Cd (Figure 4C) was enhanced by treatments in a range of 27.52%–59.16%, which was significant among T2, T3, T5, and T6. Amendments resulted in the reduction in or-Cd (Figure 4D) by 6.41%–13.24%, but the differences were insignificant. Re-Cd (Figure 4D) in all treatments increased by 1.27%–11.59%, but T8 and T9 decreased by 5.60% and 2.09%, respectively.
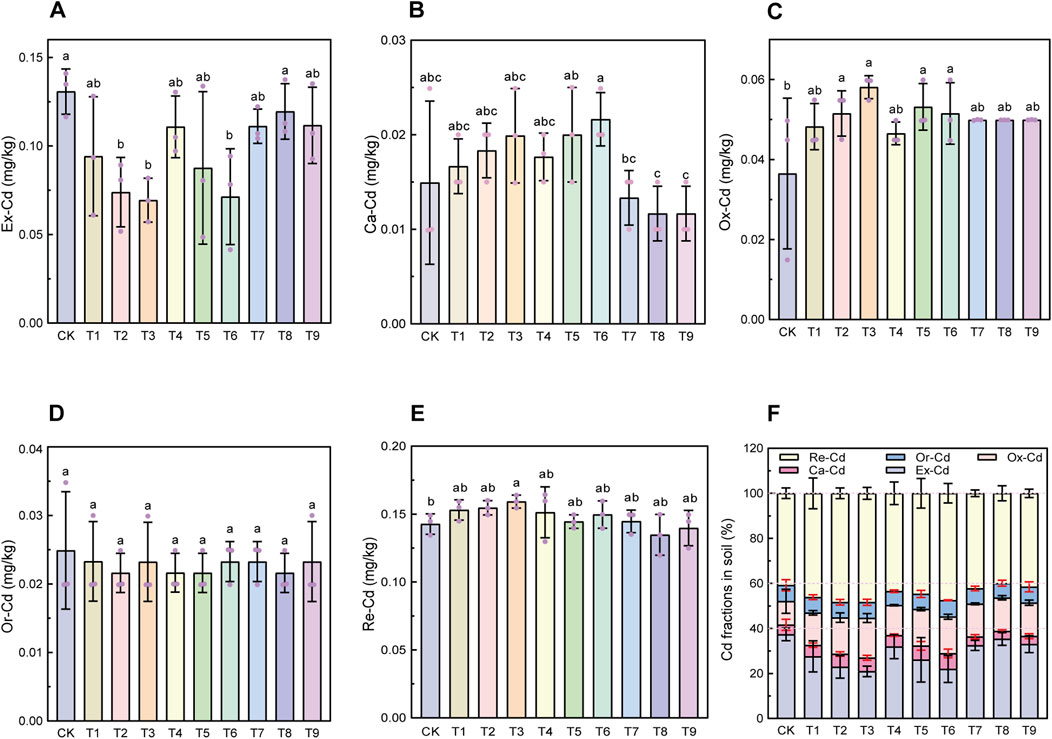
Figure 4. Effect of biochar-based amendments on soil Cd fractions. Changes in soil exchangeable Cd (Ex-Cd) (A), carbonate-bound Cd (Ca-Cd) (B), Fe-Mn oxide bond (Ox-Cd) (C), (d) organic-bound Cd (Or-Cd) (D), residual Cd (Re-Cd) (E), and distribution of cadmium fractions (F). Error bars represent the standard deviation of triplicates. Data are presented as the mean ± standard error (n = 3). Same lowercase letters mean no significant difference, while different lowercase letters mean a significant difference (ANOVA, LSD, p < 0.05).
The percentage of Cd fraction (Figure 4E) change by the amendments showed that, in all treatments, Ex-Cd, a bioavailable fraction, was transferred to more stable portions like Fe-Mn oxide bond, carbonate-bound, organic-bound, or residual Cd (Figure 4F). The concentrations of Cd fractions were as follows: Re-Cd > Ex-Cd > Ox-Cd > Or-Cd > Ca-Cd. The transformation of Ex-Cd in T3, T6, and T2 to other Cd fractions was 46.89%, 45.45%, and 43.45%, respectively, (P < 0.05). Furthermore, T3, T6, and T2 increased Ca-Cd, Ox-Cd, and Re-Cd fractions by 33.49%, 44.90%, and 22.60%; 59.15%, 41.22%, and 41.17%; and by 11.59%, 4.88%, and 8.35%, respectively.
3.3 Rice growth, biomass, and yield
Rice growth parameters such as basic seedlings, maximum seedlings, tiller number, plant height, effective panicles, tillering rate, and panicle rate are given in Table 2. T1–T6 demonstrated an increasing trend in comparison with CK, while T7–T9 displayed a decreasing trend. Yield component data given in Table 3, including 1,000-grain weight, filled grains, total grains, and panicles, showed that T6, T3, and T2 significantly enhanced filled-grain and 1,000-grain weight by 34.29% and 29.47%; 18.35% and 1.08%; and 2.41% and 2.26%, respectively (p < 0.05), while those of T9, T8, and T7 were significantly reduced by 60.80% and 57.07%; 52.95% and 8.12%; and 8.75% and 8.96%, respectively (p < 0.05). T7, T8, and T9 significantly decreased filled-grain percentage and panicle length (p < 0.05).
The changes in panicle and shoot weight are shown in Figure 5. Panicle fresh weight (Figure 5A) and dry weight (Figure 5B) had a similar trend in all treatments. The highest fresh panicle weight, dry panicle weight, fresh shoot weight (Figure 5C), and dry shoot weight (Figure 5D) were recorded in T3, which significantly increased by 47.85%, 35.77%, 26.68%, and 17.73%, respectively (p < 0.05). In contrast, T9 showed the least fresh and dry panicle weights, which significantly decreased by 57.67% and 55.47%, respectively (p < 0.05), while the T8 had the lowest fresh shoot weight and dry shoot weight, which decreased by 4.89% and 22.66%, respectively.
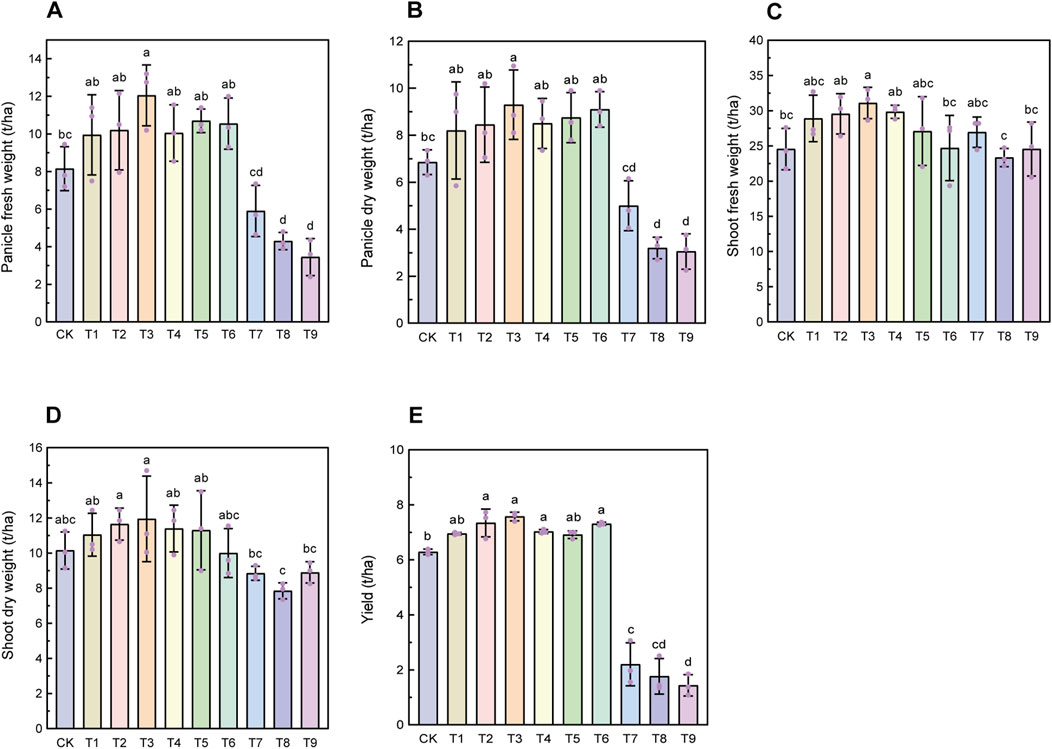
Figure 5. Effect of biochar-based amendments on the rice biomass and yield. Panicle fresh weight (A), panicle dry weight (B), shoot fresh weight (C), shoot dry weight (D), and rice yield (E). Error bars represent the standard deviation of triplicates. Data are presented as the mean ± standard error (n = 3). Same lowercase letters mean no significant difference, while different lowercase letters mean a significant difference (ANOVA, LSD, p < 0.05).
T1–T6 treatments enhanced the rice yield. The rice yield in all treatments was in the range of 1.44–7.58 t/ha (Table 3). T3, T2, T6, and T4 significantly increased the rice yield by 20.14%, 16.67%, 16.17%, and 11.64%, respectively (p < 0.05), while T9, T8, and T7 decreased the rice yield by 77.14%, 71.89%, and 65.06%, respectively (p < 0.05).
3.4 Cd content in rice tissues
As shown in Figure 6, the concentration of Cd in the tissues of rice was in the following order: root > shoot > leaf > brown rice. The root and shoot contained a much higher Cd content than the leaf and brown rice. The Cd content in rice tissues apart from the shoot of all amendments was lower than that in CK, while there was a slight increase in the shoot (Figure 6E). The Cd content in the root (Figure 6A) was the highest in CK and the lowest in T7, which significantly decreased by 33.13% compared with CK, and the reductions by T4, T5, T6, T8, T1, T3, and T2 were 32.03%, 29.52%, 21.01%, 20.33%, 19.81%, 16.54%, and 15.59%, respectively (p < 0.05). The maximum Cd concentration in the shoot was in T6, while the least Cd was in T9. As shown in Figure 6B, the decrease in Cd content caused by T9, T1, T2, and T8 in the shoot ranged from 1.47% to 9.28%, whereas the increase in Cd content caused by T3, T7, T4, T5, and T6 ranged from 2.82% to 87.41%. The highest Cd content in the leaf was in CK, while the lowest was in T8. As against CK, the percentage decrease in Cd in the leaf (Figure 6C) by amendments ranged from 17.01% to 53.47%. The effectiveness of Cd reduction in the leaf by treatments was in the following order: T8 > T2 > T9 > T7 > T3 > T6 > T5 > T1 > T4 > CK. The Cd concentration in brown rice grain (Figure 6D) was highest in CK. T2 showed the greatest reduction in Cd content in brown rice grain, which was 71.77% and significant compared with that of CK, followed by T8, T9, T3, T1, T7, and T6, which were 57.13%, 52.04%, 47.71%, 45.32%, 40.39%, and 38.16%, respectively. There were no statistically significant differences observed between T5 and T4, which was only 28.16% and 19.30% reduction, respectively (p > 0.05).
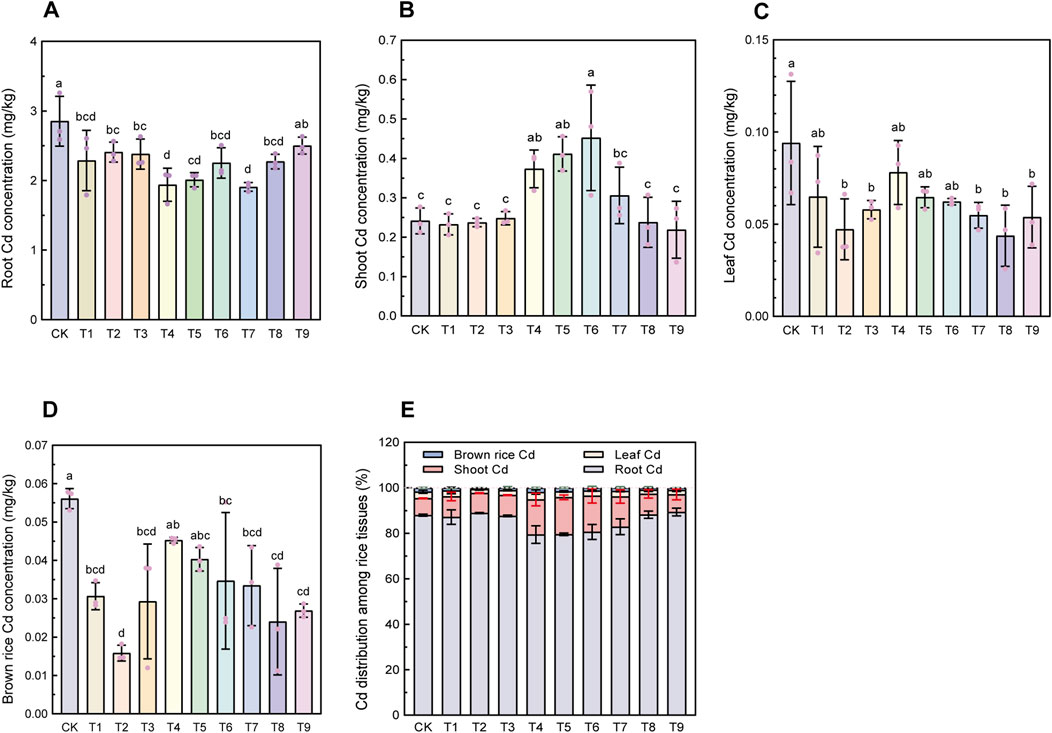
Figure 6. Effect of biochar-based amendments on the Cd concentration in rice tissues (LSD, p < 0.05). Cd concentration in the root (A), shoot (B), leaf (C), brown rice (D), and proportion of Cd in rice tissues (E). Error bars represent the standard deviation of triplicates. Data are presented as the mean ± standard error (n = 3). Same lowercase letters mean no significant difference, while different lowercase letters mean a significant difference (ANOVA, LSD, p < 0.05).
BCFsoil-root showed a decreasing trend among treatments, ranging from 7.94% to 31.90%. The decrease was significant in T4, T7, and T5 (Table 4; p < 0.05). TFroot-shoot of all treatments substantially increased from 0.08 to 0.20, while the increase was 141.76%, 135.31%, 131.76%, and 90.84% in T5, T6, T4, and T7, respectively (p < 0.05). TFshoot-rice of all amendments was significantly lower than that in CK (p < 0.05), with a reduction range of 41.63%–71.39%, whereas TFleaf-rice showed no significant change in all treatments.
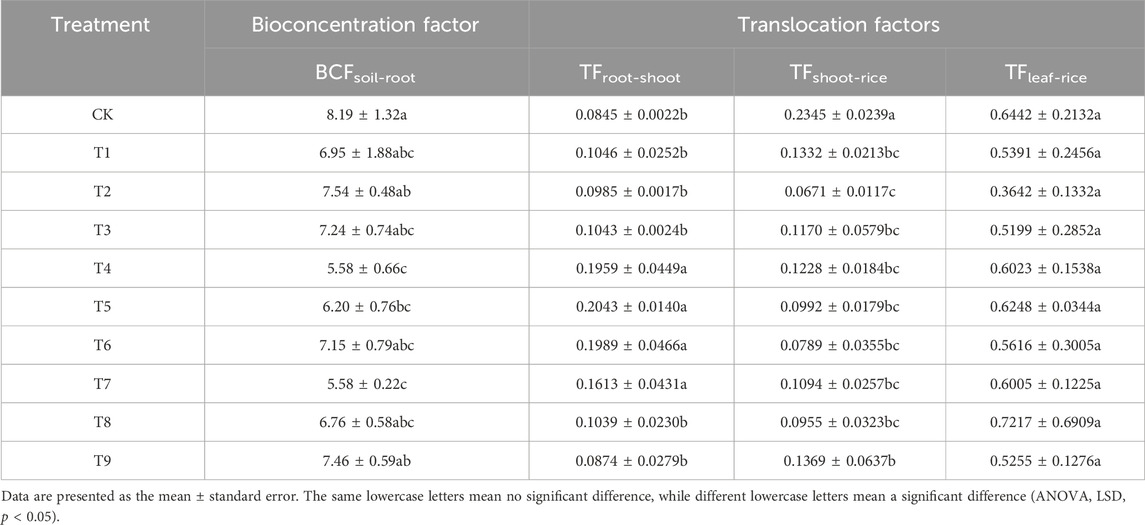
Table 4. Effect of biochar amendments on the bioconcentration factor (BCF) and translocation factor (TF).
3.5 IMP on the root surface
As shown in Figure 7, T3, T6, and T9 amendments induced a high level of IMP. IMP contained FeIMP (1.36–3.53 g/kg) and MnIMP (0.26–0.44 g/kg). T1, T4, and T7 caused no significant difference in the IMP content, whereas T2, T3, T5, T6, T8, and T9 significantly enhanced the formation of IMP by Fe, i.e., 92.42%, 160.34%, 84.49%, 113.67%, 94.59%, and 128.61% (Figure 7A), and Mn, i.e., 45.85%, 55.46%, 35.50%, 55.01%, 62.85%, and 73.09% (Figure 7B), respectively (p < 0.05).
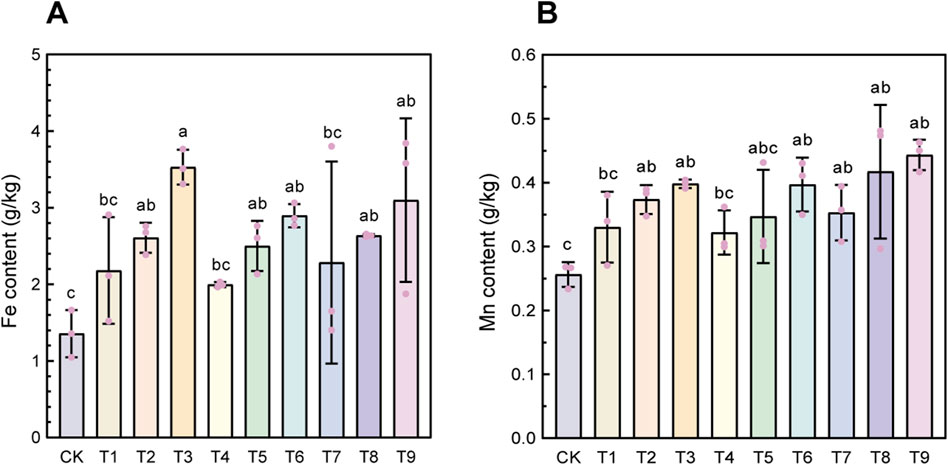
Figure 7. Content of FeIMP and MnIMP in IMP. Changes in FeIMP (A) and MnIMP (B) content of IMP on the rice root surface. Error bars represent the standard deviation of triplicates. Data are presented as the mean ± standard error (n = 3). Same lowercase letters mean no significant difference, while different lowercase letters mean a significant difference (ANOVA, LSD, p < 0.05).
3.6 Correlation among the soil properties, DTPA-Cd, and plant index
Principal component analysis (PCA) and correlation analysis of soil properties, DTPA-Cd, and plant indexes were performed to reveal the factors affecting soil Cd migration, plant Cd uptake, and accumulation. Data given in Figure 8 showed that PC1 and PC2 explained 51.88% of data variance, which suggested that the biochar-based amendments did cause a significant impact. Maize straw and bamboo biochar-based amendments had a greater effect on soil properties and rice growth, while coconut shell-activated carbon was less effective. Data given in Figure 9 showed that DTPA-Cd had a significantly positive correlation (p < 0.05) with soil IMP, TN, TP, and CEC, whereas there was also a strong correlation among Eh, pH, SOC, and DOC. In addition, decreased Cd content in rice grain primarily resulted from decreased uptake by rice plants. The Cd content in rice grain exhibited a high correlation with leaf Cd, root Cd, IMP, and Ex-Cd.
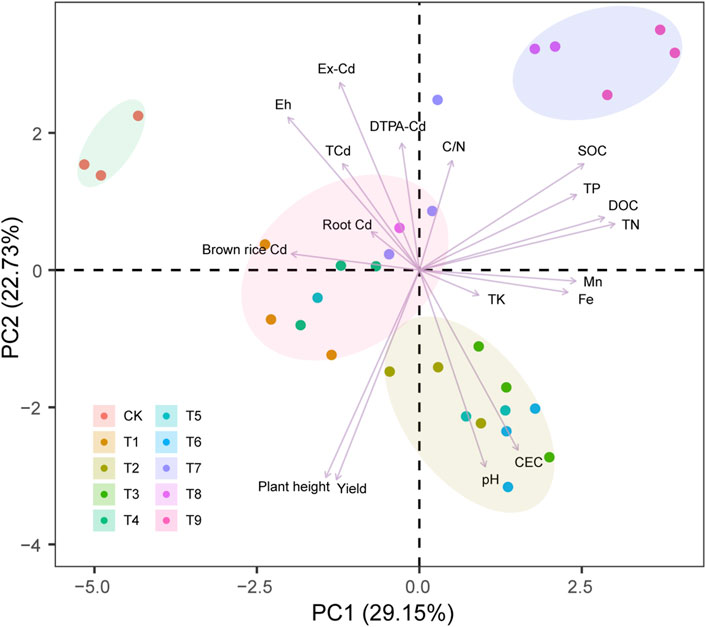
Figure 8. Principal component analysis (PCA) for soil properties, soil Cd fraction, tissue Cd content, rice yield, plant height, and plaque FeIMP and MnIMP content.
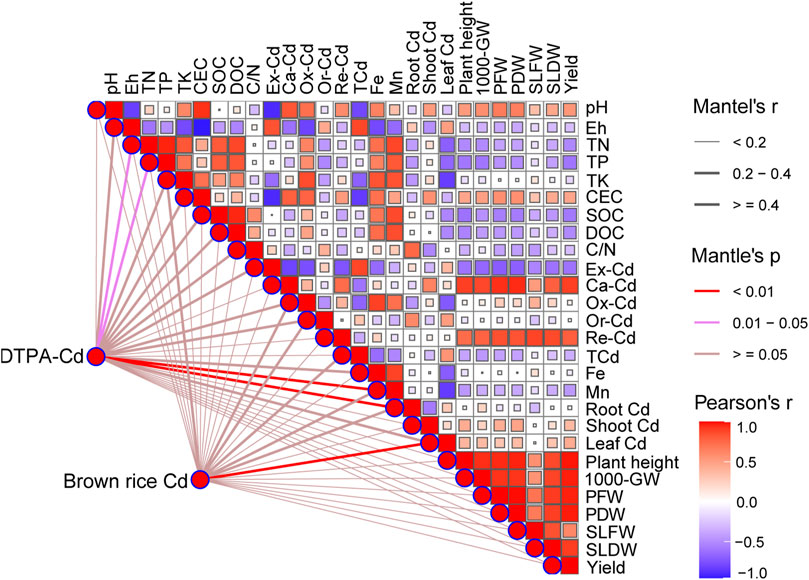
Figure 9. Correlation analysis of soil properties, soil Cd fraction, tissue Cd content, rice yield and components, plant height, and plaque FeIMP and MnIMP content. Here, 1000-GW represents 1000-grain weight, PFW represents fresh panicle weight, PDW represents dry panicle weight, SLFW represents fresh stem and leaf weight, and SLDW represents dry stem and leaf weight. Line width represents the distance-dependent Mantel r statistic. Rosy brown lines indicate non-significant difference, pink lines indicate significant difference (0.01 < p < 0.05), and red lines indicate extremely significant difference (p < 0.01).
4 Discussion
4.1 Effect on soil properties and Cd fraction
Prior research had indicated that the application of BC-/AC-based amendments had an impact on soil properties (Awasthi et al., 2019; Bagheri Novair et al., 2023), and the findings were in accordance with our research findings. Our results indicated that soil properties including pH, TN, TP, CEC, SOC, and DOC were improved by applying BC-/AC-based amendments.
Soil pH was recognized as a key determinant of metal solubility, which could directly influence the chemistry of soil Cd such as accumulation by plants and leachability (Lv et al., 2021). Leaching of heavy metals from soil is soil pH-dependent. Increasing the soil pH might lead to increased hydroxyl groups of metal cations and enhanced metal adsorption. Increased soil pH by treatments was due to the alkalinity of BC-/AC-based amendments (Zhang et al., 2017). Our study found that soil pH showed an increasing trend with the amounts of applied BC/AC (Figure 2A). The surface of BC/AC contained negatively charged functional groups, for instance, phenols, hydroxyl, amine, carboxyl, and mercaptan compounds, which were capable of binding hydrogen ions from the soil solution and increase the soil pH (Nguyen et al., 2017; Bagheri Novair et al., 2023). In addition, there was the possibility that BC-/AC-based amendments could stimulate or enrich the activity of soil microorganisms, thus increasing the soil pH (Lin et al., 2022). Increased soil pH might also provide active sites enhancing Cd adsorption or immobilization (Nguyen et al., 2023).
The value of Eh in the soil depended on the relative concentrations of oxidized and reduced substances in the soil solution (Chacón et al., 2020). The main factors affecting soil Eh were soil aeration, soil moisture, the metabolism of the plant root, and the proportion of decomposable soil organic matter (Yang et al., 2021). According to this research, soil Eh decreased with increasing biochar contents (Figure 2B). Xing et al. (2022) found that adding biochar to rice soil resulted in a broader range of soil Eh within 6 h (219 ∼ +334 mV) than control (−271 ∼ +391 mV), which had been confirmed by Yang et al. (2020). Klüpfel et al. (2014) and Rashid et al. (2022) indicated that biochar enhanced the redox reaction by providing electrons through a phenol group or accepting electrons through the quinone aromatic structure. These results showed the critical role of biochar in mediating the redox environment in soil. Much higher or lower soil Eh was not conducive to rice growth. Higher soil Eh presents a state of oxidation, faster organic matter decomposition, or nutrient leaching. Lower soil Eh could cause poor ventilation and restrict rice growth and development.
CEC was one of the critical soil properties strongly influenced by soil organic matter, which varied from <1 cmol/kg in sandy soil to >25 cmol/kg in clay soil (Guibert et al., 1999; Arabi et al., 2018). Our experiments revealed that applying BC-/AC-based amendments, especially those with high BC/AC content, significantly increased soil CEC (Figure 2F). These results were consistent with the findings found by Awasthi et al. (2019), Hussain Lahori et al. (2017), and Mohamed et al. (2015). Increasing soil CEC by BC/AC was a result of its highly porous characteristic and high specific area, which subsequently increased the adsorption capacity (Liu et al., 2012).
Our data indicated that amendments significantly increased TN, TP, SOC, and DOC contents in soil (Figure 2), consistent with the outcomes of published literature (Li Y. et al., 2022c; Jin et al., 2019). This might be because BC/AC, rape organic fertilizer, and calcium magnesium phosphate fertilizer were enriched in carbon, nitrogen, and phosphorus, which provided a source of organic material and increased the inputs of these compounds in soil (Arabi et al., 2018; Farhangi-Abriz et al., 2021). Previous research had also demonstrated that BC/AC could have a positive effect on crop growth and efficiency of nutrient availability (Backer et al., 2016; Azeem et al., 2023). Other surveys had demonstrated that biochar application could positively affect soil microorganisms and enzyme activity (Luis Moreno et al., 2022; Song et al., 2022). Moreover, TN, TP, SOC, and DOC contents increased with BC/AC content, and as a result, the rice yield increased (Figure 5E). Therefore, it was necessary to conduct long-term experiments in multiple geographical sites to validate the impacts of the application of BC/AC or derived materials on TN, TP, SOC, and DOC contents, in an effort to provide reliable evidence for the large-scale application of biochar-based materials.
DTPA-Cd was the bioavailable form that could be absorbed and utilized by plants. In comparison with total soil Cd, DTPA-Cd had a greater impact on metal toxicity and uptake by plants. DTPA-Cd played an essentially important role in managing Cd absorption by crops and forecasting the Cd toxicological effect in agroecosystems (Lahori et al., 2017; Wu et al., 2020). In our study, the DTPA-Cd content of each treatment was found to decrease rapidly in the early stage, increased slightly in the later stage, and finally stabilized. After harvest, the ultimate results showed that the treatments decreased soil DTPA-Cd, in which only the effects of T1, T4, and T6 were significant. Comparable findings were presented by Hong et al. (2022), who found that the co-application of biochar, apatite, and seaweed organic fertilizer at a ratio of 1.5:0.5:1 significantly reduced soil bioavailability of Cd, Cr, and Pb; enhanced soil conditions; and improved nutrient availability and yields. Liang T. et al. (2022b) revealed that the simultaneous application of rape straw biochar, rice straw, and Chinese milk vetch effectively reduced soil DTPA-Cd by 27.80%–46.62%. Furthermore, Xiong et al. (2019) revealed that rice straw, biochar, and engineered bacterium P. putida X4/pIME compost applied as a soil amendment significantly reduced Cd concentrations. This study also showed that BC-/AC-based amendments decreased Ex-Cd content in soil while increasing the content of other Cd fractions, including Ca-Cd, Ox-Cd, and Re-Cd (Figure 4). Similarly, Liu et al. (2022) and Xiong et al. (2019) revealed that biochar and biochar compost lowered the Ex-Cd content and increased that of other Cd fractions.
The reduction in DTPA-Cd in soil by BC-/AC-based amendments could be potentially because BC/AC-based amendments contained alkaline particles and produced OH− groups that could bind Cd ions from the soil solution to form insoluble complexes, thus reducing Cd bioavailability. Furthermore, the BC-/AC-based amendments contained high amounts of Ca2+ and Mg2+ ions and a large surface area that could interact with soluble Cd to enhance Cd adsorption or immobilization (Singh et al., 2023; Zanin Lima et al., 2023). pH was a prominent factor regulating the effectiveness of Cd, which showed a negative correlation to DTPA-Cd. It was reported that elevated soil pH caused an increased negative surface charge in soil, leading to facilitated surface precipitation, such as CdCO3 or Cd(OH)2, and decreased DTPA-Cd (Suzuki et al., 2020; Liang T. et al., 2022b). According to our research, soil pH was higher in BC/AC treatments than in CK, which was beneficial in reducing Cd mobilization and effectiveness. Furthermore, the addition of BC-/AC-based amendments could result in strengthening Cd complexation due to improved soil CEC and SOM, thereby contributing to the reduction in soil DTPA-Cd (Alaboudi et al., 2019). Additionally, studies had indicated that some soil microorganisms had the ability to absorb Cd. It might be possible that the addition of biochar-based amendments could enrich this group of soil microorganisms and, therefore, reduce the Cd content in soil (Qi et al., 2023; Haider et al., 2021; Mei et al., 2022).
4.2 Effect on rice yield and tissue Cd content
According to data on rice growth given in Table 2, yield components in Table 3, and biomass in Figure 5, BC-based amendments significantly increased the rice yield and yield components, as well as dry biomass. This result was in agreement with those reported by Mohamed et al. (2015), Arabi et al. (2018), and Li et al. (2019). On the other hand, AC-based amendments severely reduced the rice yield and caused the whitening of leaves during the seedling stage, which was contrary to the findings obtained by Břendová et al. (2016), who utilized biochar and coconut shell-activated carbon to reduce the phytoavailability and phytotoxicity of Cd, Pb, and Zn and reported that both carbon-based amendments increased spinach dry biomass by 114% and 55% in non-contaminated soil and 359% and 308% in contaminated soil, respectively. Therefore, the effectiveness of coconut shell-activated carbon needs to be further validated.
BC/AC exhibited strong adsorption of nutrients, such as ammonium, nitrate, phosphate, potassium, and trace elements, which could retain nutrients and reduce their loss from soil, enhancing nutrient uptake by plants and improving growth and yield (Lv et al., 2021). Furthermore, BC-based amendments improved soil properties by increasing soil pH and CEC and lowering soil Eh, which would enhance nutrient efficiency, especially of phosphorus and potassium (Alvarez et al., 2017), reduce nutrient leaching or loss from soil (Agegnehu et al., 2016), and slow down soil organic matter decomposition (Xing et al., 2022). Moreover, BC-based amendments would enrich soil bacterial communities, stimulate the activities of enzymes, and improve soil health and rice growth (Ibrahim et al., 2021; Hong et al., 2022).
This study demonstrated that Cd uptake and accumulation were dominated by roots, followed by shoots, leaves, and finally, brown rice grain (Figure 6). Maize straw biochar-based amendments were more effective than bamboo and coconut shell-activated carbon in reducing the Cd concentration in brown rice. T2 achieved the best reduction of Cd in brown rice by 71.77%. Liang T. et al. (2022b) revealed that the simultaneous application of Chinese vetch, rice straw, rape straw biochar, and iron-modified biochar remarkably reduced 65.38% and 62.65% of Cd concentration in rice grain, respectively. A comparable study was reported by Hong et al. (2022), who applied a combination of seaweed organic fertilizer, apatite, and biochar to maize grain, which reduced Cd, Pb, and Cr by 68.9%, 68.9%, and 65.7%, respectively. The analysis showed that the incorporation of BC/AC, rape organic fertilizer, calcium magnesium phosphate fertilizer, and fulvic acid significantly restrained Cd uptake by plants from the soil. Table 4 shows that BCFsoil-root was reduced by all treatments, illustrating that BC addition was more effective in decreasing Cd enrichment in brown rice as TFleaf-rice > TFroot-shoot > TFshoot-rice.
Cd transport from roots to brown rice grain was predominantly through shoots and leaves (Zhou et al., 2018). BC-/AC-based amendments inhibited Cd enrichment in various tissues of rice, which was most likely because of the reduction in DTPA-Cd in the soil. DTPA-Cd was found to be positively correlated with the Cd concentration in rice grain, and BC/AC-based amendments significantly lowered soil DTPA-Cd content and increased Re-Cd content. Thus, the BC/AC amendments reduced Cd uptake in rice by decreasing the bioavailability and transport of Cd in soil (Sun et al., 2016). Furthermore, the development of IMP was an effective barrier to prevent metal absorption by roots (Huang et al., 2022). Additionally, increased Fe2+ or Mn2+ might compete with Cd for the adsorption sites, thus being responsible for decreased Cd movement and storage in rice (Cai et al., 2020).
4.3 Effect on root IMP
IMP formed on the root surface was a kind of amphiphilic colloid, which could affect the chemical behavior and bioavailability of various elements in the soil through adsorption and co-precipitation. It effectively impeded Cd uptake by the root system and reduced Cd accumulation in rice tissues (Huang et al., 2022; Liang T. et al., 2022b). Previous studies had shown that the incorporation of organic materials, such as organic fertilizers, biochar, and livestock manure, could stimulate the generation of IMP (Li J. et al., 2020a; Liu et al., 2021). According to our research, applying BC-/AC-based amendments enhanced the IMP content, which simultaneously reduced the Cd concentration in the edible portion of rice (Figure 7; Figure 6D).
IMP formation was mainly influenced by the Fe2+ and Mn2+ concentrations, oxygen concentration, rhizosphere oxidase system, and soil microorganisms (Wu et al., 2016; Lin et al., 2017). In order to adapt to the flooded environment, rice morphological structures and physiological characteristics of the aboveground and root systems experienced specific changes, most prominently the formation of aerated tissues (Jiang et al., 2009). Aerated tissues transported oxygen through the leaves to the root system, which, in turn, released oxygen and other oxygenated substances into the rhizosphere, thereby oxidizing Fe2+ in the flooded soil to Fe3+ and Mn2+ to Mn (Ⅲ/Ⅳ). Fe3+ and Mn (Ⅲ/Ⅳ) formed insoluble oxides or hydroxides in the rhizosphere of rice, which were deposited on the surface of the roots, forming IMP (Machado et al., 2005; Kong et al., 2023; Zheng et al., 2024). Moreover, there existed oxidative enzyme systems (catalase, superoxidase, superoxide dismutase, etc.) in the rhizosphere of rice, and the application of BC-/AC-based amendments increased soil catalase and superoxidase enzyme activities, which could convert Fe2+ to Fe3+ and Mn2+ to Mn (Ⅲ/Ⅳ) (Chen H. et al., 2020a; Li Q. et al., 2020b; Zheng et al., 2023). Furthermore, iron-oxidizing and methane-oxidizing bacteria attached to the root surface of rice also played an active role in the formation of IMP (Yu et al., 2016; Danso et al., 2023). Fe2+ and Mn2+ in BC-/AC-based amendments were released into the soil solution, and soil redox conditions changed, which contributed to improved formation of IMP. The application of BC-/AC-based amendments modified the soil microenvironment, thereby promoting Fe2+ and Mn2+ release and contributing to IMP formation (Zhou et al., 2020).
4.4 Potential mechanism of Cd immobilization, Cd accumulation in rice grain, and rice yield
The distribution of DTPA-Cd in the soil directly affects the crop uptake and utilization of Cd. In our research, BC-/AC-based amendments reduced the DTPA-Cd content in soil. Therefore, it is important to explore its potential passivation mechanism. Figure 10 shows the potential mechanism of BC-/AC-based amendments on soil Cd immobilization, Cd accumulation in rice grain, and rice yield. The adsorption and immobilization of Cd by BC-/AC-based amendments was not a single process but rather a synergistic interaction of various reactions (Wu et al., 2020; Chen et al., 2021). The mechanisms mainly included physical adsorption, electrostatic action, ion exchange, complexation, and precipitation (Singh et al., 2023). BC/AC had a highly porous structure with a large specific surface area, and Cd could be adsorbed on its surface and also diffuse into the porous structure, which could be immobilized. In addition, the larger the specific surface area, the higher the physical adsorption capacity for Cd (Nguyen et al., 2023). The BC/AC surface contained alkali metals such as K, Na, Mg, and Ca, which could be exchanged with Cd, thereby reducing the concentration of Cd and increasing the nutrient concentration in the soil to promote the growth and development of crops (Lv et al., 2021). Additionally, the oxygen-containing functional groups (-OH, -COOH, and C=O) on the BC/AC surface performed ion-exchange reactions with Cd2+ [2 (R-OH) + Cd2+ → (R-O)2Cd + 2H+, 2 (R-OOH) + Cd2+ → (R-OO)2Cd + 2H+] (Zhou et al., 2018; Alaboudi et al., 2019). It also complexed with Cd on the surface of BC/AC, reducing the bioavailability and mobility of Cd (He et al., 2019). Electrostatic interaction was closely related to the valence state of Cd and CEC of the soil. A higher valence state of Cd causes a stronger electrostatic interaction, leading to Cd being more easily adsorbed. Increased CEC would make the soil negatively charged, which further enhanced the adsorption of Cd by soil (Zhang et al., 2020; Li H. et al., 2022a). Moreover, BC/AC contained soluble CO32- and PO43-, which could form a precipitation with Cd (Lahori et al., 2017). Additionally, our study showed that BC-/AC-based amendments decreased Ex-Cd content in soil and shifted it to a more stable morphology (Figure 4); as a result, DTPA-Cd was reduced. Therefore, the reduction in DPTA-Cd in the soil resulted in decreased Cd uptake by rice. Alternatively, the formation of IMP on the root surface reduced the accumulation of Cd in rice grains (Figure 7; Figure 6D).
The BC-based amendment material contained rich nutrients that contributed to enhanced rice yield. BC could enhance soil fertility and nutrient efficiency by improving soil physicochemical properties (SOM, CEC, and fertilizer holding capacity) and regulating soil pH to reduce nutrient losses (Chen et al., 2021). Additionally, the porous structure of BC contributed to reduced ammonia volatilization losses, improved fertilizer utilization, and facilitated nutrient cycling. Moreover, the functional groups (-OH, -COOH, C=O, S2-, CO32-, and PO43-) on the BC surface could enhance the rice yield through various mechanisms. First, the BC porous structure and large specific surface area could adsorb N, P, K, and other nutrients in the soil, reducing nutrient loss and promoting nutrient cycling by improving soil microbial activity, therefore enhancing the efficiency of fertilizer utilization (Agegnehu et al., 2016; Liu et al., 2022). Second, these functional groups could also form complexes or chelates with nutrient ions, resulting in the retention and slow release of nutrients, thus promoting nutrient uptake by rice (Farhangi-Abriz et al., 2021). Finally, BC and organic fertilizers could have a synergistic effect, further increasing rice yields and soil carbon storage.
Our research showed that AC-based amendments severely reduced rice yield. We reviewed a large amount of literature, with were fewer studies found in this area, and existing studies had contrary results to our study. Yin et al. (2022) showed that Salix integra dry mass increased more with the combination of spermidine and activated carbon treatment (39.65%–92.95%) than with the treatment alone (14.79%–62.80%). Wei et al. (2022) used a bacterial consortium (Enterobacter asburiae G3, Enterobacter tabaci I12, and Klebsiella variicola J2 in a 1:3:3 proportion) via physisorption, and sodium alginate encapsulation on coconut shell-activated carbon significantly increased the fresh and dry weights of pak choi biomass. These studies were conducted in dry fields; however, there were no studies in paddy fields. Therefore, the mechanism of rice yield reduction caused by AC-based amendments was difficult to elucidate. We hypothesized that AC-based amendments might have a high adsorption capacity for effective nutrients (N, P, and K) after rice transplantation, and rice was unable to absorb the nutrients, resulting in leaf yellowing (Figure 1). During the mid-growth period of rice, rice leaves showed white–green patches and reduced chlorophyll content, which may be one of the factors leading to the reduction in rice yield. At harvest, the plant height was significantly lower in the AC-based amendment application than in the other treatments. The yield component data illustrated that all the AC-based amendment application treatments severely reduced rice filled-grain percentage and 1,000-grain weight (Table 3). Additionally, AC-based amendments might cause stress to the roots of rice, resulting in the inability to complete normal growth and development. Furthermore, AC-based amendments might affect the activity of microorganisms in the soil, resulting in their inability to perform their normal functions. In conclusion, the mechanism of AC-based amendments that severely reduced rice yield needs further research.
4.5 Impact of BC-based amendments on environmental and economic benefits
BC-based amendments are an eco-friendly remediation material that had remarkable effects in the treatment of soil heavy metal pollution. Its effect on the environment was mainly reflected in the following aspects: (1) reduction in heavy-metal bioavailability. BC-based amendments reduced heavy-metal bioavailability and mobility in the soil through various mechanisms, such as physical adsorption, electrostatic action, ion exchange, complexation, and precipitation, thereby reducing their potential threat to ecosystems and human health (Xiong et al., 2019; Li J. et al., 2022b); (2) improvement in soil physicochemical properties. BC-based amendments could improve soil pH, increased soil cation exchange and organic matter content, improved soil structure, and thus enhanced the soil ability to immobilize heavy metals (Ibrahim et al., 2021; Lv et al., 2021); (3) promotion of soil microbial activity. BC-based amendments might alter the structure of soil microbial communities, increased the relative abundance of some beneficial microorganisms, promoted soil metabolic processes, and enhanced microbial activity in the soil (Chen S. et al., 2020b; Wang et al., 2021); (4) long-term immobilization of soil heavy metals. Research showed that BC-based amendments could effectively stabilize heavy metals in the soil for a long period of time, and during the immobilization process, the “aging effect” of biochar promotes a continuous increase in soil cation exchange and organic matter content, which, once again, reduces the bioavailability of heavy metals without potentially harming the soil (Ros et al., 2006; Herold et al., 2012).
Applying BC-based amendments improved the soil quality and increased the crop yield and quality, thereby increasing the economic benefits of agricultural outputs (Singh et al., 2023). Meanwhile, biochar production could reduce waste disposal costs by utilizing biomass resources such as agricultural waste, while providing a value-added product (Ndoung et al., 2021). However, whether the production and application of biochar were economically viable depended on a number of factors, including the cost of feedstock, scale of production, pyrolysis conditions, price of biochar, and type of crop (Nguyen et al., 2023). Although BC-based amendments had potential economic benefits, they needed to be assessed in detail on a case-by-case basis, as well as policy support and market development to realize their commercialization potential.
5 Conclusion
This study showed that BC-/AC-based amendments significantly enhanced soil pH, TN, TP, CEC, SOC, and DOC and decreased soil Eh and DTPA-Cd. Maize straw and bamboo biochar-based amendments significantly improved the rice yield and biomass, while coconut shell-activated carbon severely reduced the rice yield. This experiment showed that BC-/AC-based amendments effectively modified Cd fractionation in soil and induced the Cd transformation from bioavailable (Ex-Cd) to less bioavailable fractions (Ca-Cd, Ox-Cd, and Re-Cd). The alleviation of Cd absorption and enrichment in rice tissues by BC-/AC-based amendments were achieved through soil Cd fractional transformation or immobilization and IMP formation. The results demonstrated that BC-/AC-based amendments could be potentially used as eco-friendly and sustainable materials for improving soil health and remediating Cd-contaminated soil, which safeguards human health and the ecosystem from metal contamination. The results of the field trial showed that our material could immobilize soil Cd, but there are still limitations. We aimed for a long-term fixed trial, but due to the local government’s construction of high-standard farmland, which had already destroyed our experimental field, we only performed one season of rice experiments. In future, we will build a long-term fixed trial to achieve favorable results.
Data availability statement
The original contributions presented in the study are included in the article/Supplementary Material; further inquiries can be directed to the corresponding author.
Author contributions
JH: conceptualization, data curation, formal analysis, funding acquisition, investigation, methodology, software, writing–original draft, and writing–review and editing. DW: conceptualization, methodology, resources, and writing–review and editing. JY: conceptualization, visualization, and writing–review and editing. YS: data curation, formal analysis, investigation, software, visualization, and writing–review and editing. GA: writing–review and editing. LW: conceptualization, formal analysis, investigation, and writing–review and editing. ZL: conceptualization, funding acquisition, methodology, project administration, supervision, and writing–review and editing.
Funding
The author(s) declare that financial support was received for the research, authorship, and/or publication of this article. This work was supported by funding from the National Natural Science of China (grant no. 2017YFC0504405) and Nanchong Science and Technology Talent Innovation Project (no. 22KJCXRC0004).
Acknowledgments
The authors thank all the researchers in this study.
Conflict of interest
The authors declare that the research was conducted in the absence of any commercial or financial relationships that could be construed as a potential conflict of interest.
Publisher’s note
All claims expressed in this article are solely those of the authors and do not necessarily represent those of their affiliated organizations, or those of the publisher, the editors, and the reviewers. Any product that may be evaluated in this article, or claim that may be made by its manufacturer, is not guaranteed or endorsed by the publisher.
References
Aborisade, M. A., Feng, A., Zheng, X., Oba, B. T., Kumar, A., Battamo, A. Y., et al. (2022). Carbothermal reduction synthesis of eggshell-biochar modified with nanoscale zerovalent iron/activated carbon for remediation of soil polluted with lead and cadmium. Environ. Nanotechnol. Monit. and Manag. 18, 100726. doi:10.1016/j.enmm.2022.100726
Agegnehu, G., Bass, A. M., Nelson, P. N., and Bird, M. I. (2016). Benefits of biochar, compost and biochar–compost for soil quality, maize yield and greenhouse gas emissions in a tropical agricultural soil. Sci. Total Environ. 543, 295–306. doi:10.1016/j.scitotenv.2015.11.054
Alaboudi, K. A., Ahmed, B., and Brodie, G. (2019). Effect of biochar on Pb, Cd and Cr availability and maize growth in artificial contaminated soil. Ann. Agric. Sci. 64, 95–102. doi:10.1016/j.aoas.2019.04.002
Alvarez, J. M., Pasian, C., Lal, R., Lapez, R., and Ferna¡ndez, M. (2017). Vermicompost and biochar as substitutes of growing media in ornamental-plant production. JAH 19, 205–214. doi:10.37855/jah.2017.v19i03.37
Arabi, Z., Eghtedaey, H., Gharehchmaghloo, B., and Faraji, A. (2018). Effects of biochar and bio-fertilizer on yield and qualitative properties of soybean and some chemical properties of soil. Arab. J. Geosci. 11, 672. doi:10.1007/s12517-018-4041-1
Awasthi, M. K., Wang, Q., Chen, H., Liu, T., Awasthi, S. K., Duan, Y., et al. (2019). Role of compost biochar amendment on the (im)mobilization of cadmium and zinc for Chinese cabbage (Brassica rapa L.) from contaminated soil. J. Soils Sediments 19, 3883–3897. doi:10.1007/s11368-019-02277-8
Awual, Md. R., Khraisheh, M., Alharthi, N. H., Luqman, M., Islam, A., Rezaul Karim, M., et al. (2018). Efficient detection and adsorption of cadmium(II) ions using innovative nano-composite materials. Chem. Eng. J. 343, 118–127. doi:10.1016/j.cej.2018.02.116
Azeem, M., Sun, T.-R., Jeyasundar, P. G. S. A., Han, R.-X., Li, H., Abdelrahman, H., et al. (2023). Biochar-derived dissolved organic matter (BDOM) and its influence on soil microbial community composition, function, and activity: a review. Crit. Rev. Environ. Sci. Technol. 53, 1912–1934. doi:10.1080/10643389.2023.2190333
Backer, R. G. M., Schwinghamer, T. D., Whalen, J. K., Seguin, P., and Smith, D. L. (2016). Crop yield and SOC responses to biochar application were dependent on soil texture and crop type in southern Quebec, Canada. J. Plant Nutr. Soil Sci. 179, 399–408. doi:10.1002/jpln.201500520
Bagheri Novair, S., Cheraghi, M., Faramarzi, F., Asgari Lajayer, B., Senapathi, V., Astatkie, T., et al. (2023). Reviewing the role of biochar in paddy soils: an agricultural and environmental perspective. Ecotoxicol. Environ. Saf. 263, 115228. doi:10.1016/j.ecoenv.2023.115228
Blanco-Canqui, H. (2017). Biochar and soil physical properties. Soil Sci. Soc. Am. J. 81, 687–711. doi:10.2136/sssaj2017.01.0017
Břendová, K., Zemanová, V., Pavlíková, D., and Tlustoš, P. (2016). Utilization of biochar and activated carbon to reduce Cd, Pb and Zn phytoavailability and phytotoxicity for plants. J. Environ. Manag. 181, 637–645. doi:10.1016/j.jenvman.2016.06.042
Cai, Y., Wang, M., Chen, B., Chen, W., Xu, W., Xie, H., et al. (2020). Effects of external Mn2+ activities on OsNRAMP5 expression level and Cd accumulation in indica rice. Environ. Pollut. 260, 113941. doi:10.1016/j.envpol.2020.113941
Chacón, F. J., Sánchez-Monedero, M. A., Lezama, L., and Cayuela, M. L. (2020). Enhancing biochar redox properties through feedstock selection, metal preloading and post-pyrolysis treatments. Chem. Eng. J. 395, 125100. doi:10.1016/j.cej.2020.125100
Chaney, R. L. (2015). How does contamination of rice soils with Cd and Zn cause high incidence of human Cd disease in subsistence rice farmers. Curr. Pollut. Rep. 1, 13–22. doi:10.1007/s40726-015-0002-4
Chen, H., Yang, X., Wang, H., Sarkar, B., Shaheen, S. M., Gielen, G., et al. (2020a). Animal carcass- and wood-derived biochars improved nutrient bioavailability, enzyme activity, and plant growth in metal-phthalic acid ester co-contaminated soils: a trial for reclamation and improvement of degraded soils. J. Environ. Manag. 261, 110246. doi:10.1016/j.jenvman.2020.110246
Chen, L., Guo, L., Liao, P., Xiong, Q., Deng, X., Gao, H., et al. (2022). Effects of biochar on the dynamic immobilization of Cd and Cu and rice accumulation in soils with different acidity levels. J. Clean. Prod. 372, 133730. doi:10.1016/j.jclepro.2022.133730
Chen, S., Qi, G., Ma, G., and Zhao, X. (2020b). Biochar amendment controlled bacterial wilt through changing soil chemical properties and microbial community. Microbiol. Res. 231, 126373. doi:10.1016/j.micres.2019.126373
Chen, Z., Pei, J., Wei, Z., Ruan, X., Hua, Y., Xu, W., et al. (2021). A novel maize biochar-based compound fertilizer for immobilizing cadmium and improving soil quality and maize growth. Environ. Pollut. 277, 116455. doi:10.1016/j.envpol.2021.116455
Danso, O. P., Acheampong, A., Zhang, Z., Song, J., Wang, Z., Dai, J., et al. (2023). The management of Cd in rice with biochar and selenium: effects, efficiency, and practices. Carbon Res. 2, 41. doi:10.1007/s44246-023-00073-1
Farhangi-Abriz, S., Torabian, S., Qin, R., Noulas, C., Lu, Y., and Gao, S. (2021). Biochar effects on yield of cereal and legume crops using meta-analysis. Sci. Total Environ. 775, 145869. doi:10.1016/j.scitotenv.2021.145869
Golia, E. E., Aslanidis, P.-S. C., Papadimou, S. G., Kantzou, O.-D., Chartodiplomenou, M.-A., Lakiotis, K., et al. (2022). Assessment of remediation of soils, moderately contaminated by potentially toxic metals, using different forms of carbon (charcoal, biochar, activated carbon). Impacts on contamination, metals availability and soil indices. Sustain. Chem. Pharm. 28, 100724. doi:10.1016/j.scp.2022.100724
Guibert, H., Fallavier, P., and Roméro, J. (1999). Carbon content in soil particle size and consequence on cation exchange capacity of Alfisols. Commun. Soil Sci. Plant Analysis 30, 2521–2537. doi:10.1080/00103629909370392
Haider, F. U., Coulter, J. A., Cheema, S. A., Farooq, M., Wu, J., Zhang, R., et al. (2021). Co-application of biochar and microorganisms improves soybean performance and remediate cadmium-contaminated soil. Ecotoxicol. Environ. Saf. 214, 112112. doi:10.1016/j.ecoenv.2021.112112
Haris, M., Hamid, Y., Usman, M., Wang, L., Saleem, A., Su, F., et al. (2021). Crop-residues derived biochar: synthesis, properties, characterization and application for the removal of trace elements in soils. J. Hazard. Mater. 416, 126212. doi:10.1016/j.jhazmat.2021.126212
He, L., Zhong, H., Liu, G., Dai, Z., Brookes, P. C., and Xu, J. (2019). Remediation of heavy metal contaminated soils by biochar: mechanisms, potential risks and applications in China. Environ. Pollut. 252, 846–855. doi:10.1016/j.envpol.2019.05.151
Herold, M. B., Baggs, E. M., and Daniell, T. J. (2012). Fungal and bacterial denitrification are differently affected by long-term pH amendment and cultivation of arable soil. Soil Biol. Biochem. 54, 25–35. doi:10.1016/j.soilbio.2012.04.031
Hong, Y., Li, D., Xie, C., Zheng, X., Yin, J., Li, Z., et al. (2022). Combined apatite, biochar, and organic fertilizer application for heavy metal co-contaminated soil remediation reduces heavy metal transport and alters soil microbial community structure. Sci. Total Environ. 851, 158033. doi:10.1016/j.scitotenv.2022.158033
Huang, G., Ding, X., Liu, Y., Ding, M., Wang, P., Zhang, H., et al. (2022). Liming and tillering application of manganese alleviates iron manganese plaque reduction and cadmium accumulation in rice (Oryza sativa L.). J. Hazard. Mater. 427, 127897. doi:10.1016/j.jhazmat.2021.127897
Hussain Lahori, A., Zhang, Z., Guo, Z., Mahar, A., Li, R., Kumar Awasthi, M., et al. (2017). Potential use of lime combined with additives on (im)mobilization and phytoavailability of heavy metals from Pb/Zn smelter contaminated soils. Ecotoxicol. Environ. Saf. 145, 313–323. doi:10.1016/j.ecoenv.2017.07.049
Ibrahim, M. M., Tong, C., Hu, K., Zhou, B., Xing, S., and Mao, Y. (2020). Biochar-fertilizer interaction modifies N-sorption, enzyme activities and microbial functional abundance regulating nitrogen retention in rhizosphere soil. Sci. Total Environ. 739, 140065. doi:10.1016/j.scitotenv.2020.140065
Ibrahim, M. M., Zhang, H., Guo, L., Chen, Y., Heiling, M., Zhou, B., et al. (2021). Biochar interaction with chemical fertilizer regulates soil organic carbon mineralization and the abundance of key C-cycling-related bacteria in rhizosphere soil. Eur. J. Soil Biol. 106, 103350. doi:10.1016/j.ejsobi.2021.103350
Jiang, F. Y., Chen, X., and Luo, A. C. (2009). Iron plaque formation on wetland plants and its influence on phosphorus, calcium and metal uptake. Aquat. Ecol. 43, 879–890. doi:10.1007/s10452-009-9241-z
Jin, Z., Chen, C., Chen, X., Hopkins, I., Zhang, X., Han, Z., et al. (2019). The crucial factors of soil fertility and rapeseed yield - a five year field trial with biochar addition in upland red soil, China. Sci. Total Environ. 649, 1467–1480. doi:10.1016/j.scitotenv.2018.08.412
Klüpfel, L., Keiluweit, M., Kleber, M., and Sander, M. (2014). Redox properties of plant biomass-derived black carbon (biochar). Environ. Sci. Technol. 48, 5601–5611. doi:10.1021/es500906d
Kong, F., Zhou, J., Guan, D.-X., Wu, N., Lu, S., and Wang, H. (2023). Role of iron manganese plaque in the safe production of rice (Oryza sativa L.) grains: field evidence at plot and regional scales in cadmium-contaminated paddy soils. Sci. Total Environ. 903, 166183. doi:10.1016/j.scitotenv.2023.166183
Lahori, A. H., Guo, Z., Zhang, Z., Li, R., Mahar, A., Awasthi, M. K., et al. (2017). Use of biochar as an amendment for remediation of heavy metal-contaminated soils: prospects and challenges. Pedosphere 27, 991–1014. doi:10.1016/S1002-0160(17)60490-9
Li, H., Xiao, J., Zhao, Z., Zhong, D., Chen, J., Xiao, B., et al. (2022a). Reduction of cadmium bioavailability in paddy soil and its accumulation in brown rice by FeCl3 washing combined with biochar: a field study. Sci. Total Environ. 851, 158186. doi:10.1016/j.scitotenv.2022.158186
Li, J., Wang, S.-L., Zhang, J., Zheng, L., Chen, D., Wu, Z., et al. (2020a). Coconut-fiber biochar reduced the bioavailability of lead but increased its translocation rate in rice plants: elucidation of immobilization mechanisms and significance of iron plaque barrier on roots using spectroscopic techniques. J. Hazard. Mater. 389, 122117. doi:10.1016/j.jhazmat.2020.122117
Li, J., Zhang, P., Ye, J., Zhang, G., and Cai, Y. (2019). Simultaneous in-situ remediation and fertilization of Cd-contaminated weak-alkaline farmland for wheat production. J. Environ. Manag. 250, 109528. doi:10.1016/j.jenvman.2019.109528
Li, J., Zhang, S., and Ding, X. (2022b). Biochar combined with phosphate fertilizer application reduces soil cadmium availability and cadmium uptake of maize in Cd-contaminated soils. Environ. Sci. Pollut. Res. 29, 25925–25938. doi:10.1007/s11356-021-17833-4
Li, Q., Song, X., Yrjälä, K., Lv, J., Li, Y., Wu, J., et al. (2020b). Biochar mitigates the effect of nitrogen deposition on soil bacterial community composition and enzyme activities in a Torreya grandis orchard. For. Ecol. Manag. 457, 117717. doi:10.1016/j.foreco.2019.117717
Li, Y., Feng, H., Chen, J., Lu, J., Wu, W., Liu, X., et al. (2022c). Biochar incorporation increases winter wheat (Triticum aestivum L.) production with significantly improving soil enzyme activities at jointing stage. CATENA 211, 105979. doi:10.1016/j.catena.2021.105979
Liang, Q., Tian, K., Li, L., He, Y., Zhao, T., Liu, B., et al. (2022a). Ecological and human health risk assessment of heavy metals based on their source apportionment in cropland soils around an e-waste dismantling site, Southeast China. Ecotoxicol. Environ. Saf. 242, 113929. doi:10.1016/j.ecoenv.2022.113929
Liang, T., Zhou, G., Chang, D., Wang, Y., Gao, S., Nie, J., et al. (2022b). Co-incorporation of Chinese milk vetch (Astragalus sinicus L.), rice straw, and biochar strengthens the mitigation of Cd uptake by rice (Oryza sativa L.). Sci. Total Environ. 850, 158060. doi:10.1016/j.scitotenv.2022.158060
Lin, L., Gao, M., Qiu, W., Wang, D., Huang, Q., and Song, Z. (2017). Reduced arsenic accumulation in indica rice (Oryza sativa L.) cultivar with ferromanganese oxide impregnated biochar composites amendments. Environ. Pollut. 231, 479–486. doi:10.1016/j.envpol.2017.08.001
Lin, S., Wang, W., Sardans, J., Lan, X., Fang, Y., Singh, B. P., et al. (2022). Effects of slag and biochar amendments on microorganisms and fractions of soil organic carbon during flooding in a paddy field after two years in southeastern China. Sci. Total Environ. 824, 153783. doi:10.1016/j.scitotenv.2022.153783
Liu, J., Schulz, H., Brandl, S., Miehtke, H., Huwe, B., and Glaser, B. (2012). Short-term effect of biochar and compost on soil fertility and water status of a Dystric Cambisol in NE Germany under field conditions. Z. Pflanzenernähr. Bodenk. 175, 698–707. doi:10.1002/jpln.201100172
Liu, M., Zhao, Z., Chen, L., Wang, L., Ji, L., and Xiao, Y. (2020). Influences of arbuscular mycorrhizae, phosphorus fertiliser and biochar on alfalfa growth, nutrient status and cadmium uptake. Ecotoxicol. Environ. Saf. 196, 110537. doi:10.1016/j.ecoenv.2020.110537
Liu, N., Lou, X., Li, X., Shuai, Z., Liu, H., Jiang, Z., et al. (2021). Rhizosphere dissolved organic matter and iron plaque modified by organic amendments and its relations to cadmium bioavailability and accumulation in rice. Sci. Total Environ. 792, 148216. doi:10.1016/j.scitotenv.2021.148216
Liu, Q., Huang, L., Chen, Z., Wen, Z., Ma, L., Xu, S., et al. (2022). Biochar and its combination with inorganic or organic amendment on growth, uptake and accumulation of cadmium on lettuce. J. Clean. Prod. 370, 133610. doi:10.1016/j.jclepro.2022.133610
Luis Moreno, J., Bastida, F., Díaz-López, M., Li, Y., Zhou, Y., López-Mondéjar, R., et al. (2022). Response of soil chemical properties, enzyme activities and microbial communities to biochar application and climate change in a Mediterranean agroecosystem. Geoderma 407, 115536. doi:10.1016/j.geoderma.2021.115536
Lv, G., Yang, T., Chen, Y., Hou, H., Liu, X., Li, J., et al. (2021). Biochar-based fertilizer enhanced Cd immobilization and soil quality in soil-rice system. Ecol. Eng. 171, 106396. doi:10.1016/j.ecoleng.2021.106396
Machado, W., Gueiros, B. B., Lisboa-Filho, S. D., and Lacerda, L. D. (2005). Trace metals in mangrove seedlings: role of iron plaque formation. Wetl. Ecol. Manage 13, 199–206. doi:10.1007/s11273-004-9568-0
Mahar, A., Wang, P., Ali, A., Guo, Z., Awasthi, M. K., Lahori, A. H., et al. (2016). Impact of CaO, fly ash, sulfur and Na2S on the (im)mobilization and phytoavailability of Cd, Cu and Pb in contaminated soil. Ecotoxicol. Environ. Saf. 134, 116–123. doi:10.1016/j.ecoenv.2016.08.025
Mei, C., Wang, H., Cai, K., Xiao, R., Xu, M., Li, Z., et al. (2022). Characterization of soil microbial community activity and structure for reducing available Cd by rice straw biochar and Bacillus cereus RC-1. Sci. Total Environ. 839, 156202. doi:10.1016/j.scitotenv.2022.156202
Mohamed, I., Zhang, G., Li, Z., Liu, Y., Chen, F., and Dai, K. (2015). Ecological restoration of an acidic Cd contaminated soil using bamboo biochar application. Ecol. Eng. 84, 67–76. doi:10.1016/j.ecoleng.2015.07.009
Ndoung, O. C. N., Figueiredo, C. C. D., and Ramos, M. L. G. (2021). A scoping review on biochar-based fertilizers: enrichment techniques and agro-environmental application. Heliyon 7, e08473. doi:10.1016/j.heliyon.2021.e08473
Nguyen, T.-B., Nguyen, V.-T., Hoang, H.-G., Cao, N.-D.-T., Nguyen, T.-T., Vo, T.-D.-H., et al. (2022). Recent development of algal biochar for contaminant remediation and energy application: a state-of-the art review. Curr. Pollut. Rep. doi:10.1007/s40726-022-00243-6
Nguyen, T.-B., Sherpa, K., Bui, X.-T., Nguyen, V.-T., Vo, T.-D.-H., Ho, H.-T.-T., et al. (2023). Biochar for soil remediation: a comprehensive review of current research on pollutant removal. Environ. Pollut. 337, 122571. doi:10.1016/j.envpol.2023.122571
Nguyen, T. T. N., Xu, C.-Y., Tahmasbian, I., Che, R., Xu, Z., Zhou, X., et al. (2017). Effects of biochar on soil available inorganic nitrogen: a review and meta-analysis. Geoderma 288, 79–96. doi:10.1016/j.geoderma.2016.11.004
Qi, W.-Y., Chen, H., Wang, Z., Xing, S.-F., Song, C., Yan, Z., et al. (2023). Biochar-immobilized Bacillus megaterium enhances Cd immobilization in soil and promotes Brassica chinensis growth. J. Hazard. Mater. 458, 131921. doi:10.1016/j.jhazmat.2023.131921
Rafique, M., Ortas, I., Rizwan, M., Chaudhary, H. J., Gurmani, A. R., and Hussain Munis, M. F. (2020). Residual effects of biochar and phosphorus on growth and nutrient accumulation by maize (Zea mays L.) amended with microbes in texturally different soils. Chemosphere 238, 124710. doi:10.1016/j.chemosphere.2019.124710
Rashid, M. S., Liu, G., Yousaf, B., Hamid, Y., Rehman, A., Arif, M., et al. (2022). A critical review on biochar-assisted free radicals mediated redox reactions influencing transformation of potentially toxic metals: occurrence, formation, and environmental applications. Environ. Pollut. 315, 120335. doi:10.1016/j.envpol.2022.120335
Ros, M., Pascual, J. A., Garcia, C., Hernandez, M. T., and Insam, H. (2006). Hydrolase activities, microbial biomass and bacterial community in a soil after long-term amendment with different composts. Soil Biol. Biochem. 38, 3443–3452. doi:10.1016/j.soilbio.2006.05.017
Singh, P., Rawat, S., Jain, N., Bhatnagar, A., Bhattacharya, P., and Maiti, A. (2023). A review on biochar composites for soil remediation applications: comprehensive solution to contemporary challenges. J. Environ. Chem. Eng. 11, 110635. doi:10.1016/j.jece.2023.110635
Song, X., Li, H., Song, J., Chen, W., and Shi, L. (2022). Biochar/vermicompost promotes Hybrid Pennisetum plant growth and soil enzyme activity in saline soils. Plant Physiology Biochem. 183, 96–110. doi:10.1016/j.plaphy.2022.05.008
Sun, Y., Sun, G., Xu, Y., Liu, W., Liang, X., and Wang, L. (2016). Evaluation of the effectiveness of sepiolite, bentonite, and phosphate amendments on the stabilization remediation of cadmium-contaminated soils. J. Environ. Manag. 166, 204–210. doi:10.1016/j.jenvman.2015.10.017
Suzuki, T., Nakase, K., Tamenishi, T., and Niinae, M. (2020). Influence of pH and cations contained in rainwater on leaching of Cd(II) from artificially contaminated montmorillonite. J. Environ. Chem. Eng. 8, 104080. doi:10.1016/j.jece.2020.104080
Wang, C., Chen, D., Shen, J., Yuan, Q., Fan, F., Wei, W., et al. (2021). Biochar alters soil microbial communities and potential functions 3–4 years after amendment in a double rice cropping system. Agric. Ecosyst. and Environ. 311, 107291. doi:10.1016/j.agee.2020.107291
Wei, T., Li, X., Li, H., Gao, H., Guo, J., Li, Y., et al. (2022). The potential effectiveness of mixed bacteria-loaded biochar/activated carbon to remediate Cd, Pb co-contaminated soil and improve the performance of pakchoi plants. J. Hazard. Mater. 435, 129006. doi:10.1016/j.jhazmat.2022.129006
Wu, C., Shi, L., Xue, S., Li, W., Jiang, X., Rajendran, M., et al. (2019). Effect of sulfur-iron modified biochar on the available cadmium and bacterial community structure in contaminated soils. Sci. Total Environ. 647, 1158–1168. doi:10.1016/j.scitotenv.2018.08.087
Wu, C., Zou, Q., Xue, S.-G., Pan, W.-S., Huang, L., Hartley, W., et al. (2016). The effect of silicon on iron plaque formation and arsenic accumulation in rice genotypes with different radial oxygen loss (ROL). Environ. Pollut. 212, 27–33. doi:10.1016/j.envpol.2016.01.004
Wu, J., Li, Z., Huang, D., Liu, X., Tang, C., Parikh, S. J., et al. (2020). A novel calcium-based magnetic biochar is effective in stabilization of arsenic and cadmium co-contamination in aerobic soils. J. Hazard. Mater. 387, 122010. doi:10.1016/j.jhazmat.2019.122010
Xing, Y., Wang, J., Kinder, C. E. S., Yang, X., Slaný, M., Wang, B., et al. (2022). Rice hull biochar enhances the mobilization and methylation of mercury in a soil under changing redox conditions: implication for Hg risks management in paddy fields. Environ. Int. 168, 107484. doi:10.1016/j.envint.2022.107484
Xiong, Z., Zhang, J., Cai, P., Chen, W., and Huang, Q. (2019). Bio-organic stabilizing agent shows promising prospect for the stabilization of cadmium in contaminated farmland soil. Environ. Sci. Pollut. Res. 26, 23399–23406. doi:10.1007/s11356-019-05619-8
Yang, S., Chen, X., Jiang, Z., Ding, J., Sun, X., and Xu, J. (2020). Effects of biochar application on soil organic carbon composition and enzyme activity in paddy soil under water-saving irrigation. IJERPH 17, 333. doi:10.3390/ijerph17010333
Yang, X., Pan, H., Shaheen, S. M., Wang, H., and Rinklebe, J. (2021). Immobilization of cadmium and lead using phosphorus-rich animal-derived and iron-modified plant-derived biochars under dynamic redox conditions in a paddy soil. Environ. Int. 156, 106628. doi:10.1016/j.envint.2021.106628
Yin, Z., Yu, J., Han, X., Wang, H., Yang, Q., Pan, H., et al. (2022). A novel phytoremediation technology for polluted cadmium soil: Salix integra treated with spermidine and activated carbon. Chemosphere 306, 135582. doi:10.1016/j.chemosphere.2022.135582
Yu, H.-Y., Li, F.-B., Liu, C.-S., Huang, W., Liu, T.-X., and Yu, W.-M. (2016). “Iron redox cycling coupled to transformation and immobilization of heavy metals: implications for paddy rice safety in the red soil of south China,” in Advances in agronomy (Elsevier), 279–317. doi:10.1016/bs.agron.2015.12.006
Yuan, R., Si, T., Lu, Q., Bian, R., Wang, Y., Liu, X., et al. (2023). Rape straw biochar enhanced Cd immobilization in flooded paddy soil by promoting Fe and sulfur transformation. Chemosphere 339, 139652. doi:10.1016/j.chemosphere.2023.139652
Zanin Lima, J., Monici Raimondi Nauerth, I., Ferreira Da Silva, E., José Pejon, O., and Guimarães Silvestre Rodrigues, V. (2023). Competitive sorption and desorption of cadmium, lead, and zinc onto peat, compost, and biochar. J. Environ. Manag. 344, 118515. doi:10.1016/j.jenvman.2023.118515
Zhang, R.-H., Li, Z.-G., Liu, X.-D., Wang, B., Zhou, G.-L., Huang, X.-X., et al. (2017). Immobilization and bioavailability of heavy metals in greenhouse soils amended with rice straw-derived biochar. Ecol. Eng. 98, 183–188. doi:10.1016/j.ecoleng.2016.10.057
Zhang, S., Deng, Y., Fu, S., Xu, M., Zhu, P., Liang, Y., et al. (2020). Reduction mechanism of Cd accumulation in rice grain by Chinese milk vetch residue: insight into microbial community. Ecotoxicol. Environ. Saf. 202, 110908. doi:10.1016/j.ecoenv.2020.110908
Zheng, Q., Wang, W., Wen, J., Wu, R., Wu, J., Zhang, W., et al. (2023). Non-additive effects of bamboo-derived biochar and dicyandiamide on soil greenhouse gas emissions, enzyme activity and bacterial community. Industrial Crops Prod. 194, 116385. doi:10.1016/j.indcrop.2023.116385
Zheng, S., Xu, C., Luo, Z., Zhu, H., Wang, H., Zhang, Q., et al. (2024). Co-utilization of sepiolite and ferromanganese ore reduces rice Cd and as concentrations via soil immobilization and root Fe-Mn plaque resistance. Sci. Total Environ. 908, 168269. doi:10.1016/j.scitotenv.2023.168269
Zhou, G., Gao, S., Lu, Y., Liao, Y., Nie, J., and Cao, W. (2020). Co-incorporation of green manure and rice straw improves rice production, soil chemical, biochemical and microbiological properties in a typical paddy field in southern China. Soil Tillage Res. 197, 104499. doi:10.1016/j.still.2019.104499
Keywords: soil cadmium, rice, biochar, immobilization, food safety
Citation: Han J, Wu D, Yang J, Shi Y, Abid G, Wang L and Li Z (2024) A biochar-based amendment improved cadmium (Cd) immobilization, reduced its bioaccumulation, and increased rice yield. Front. Environ. Sci. 12:1487190. doi: 10.3389/fenvs.2024.1487190
Received: 27 August 2024; Accepted: 18 September 2024;
Published: 02 October 2024.
Edited by:
Babar Iqbal, Jiangsu University, ChinaReviewed by:
Irma Robles, Center of Research and Technologic Development in Electrochemistry, MexicoAsim Biswas, University of Guelph, Canada
Dongbo Lin, Guangdong Ocean University, China
Copyright © 2024 Han, Wu, Yang, Shi, Abid, Wang and Li. This is an open-access article distributed under the terms of the Creative Commons Attribution License (CC BY). The use, distribution or reproduction in other forums is permitted, provided the original author(s) and the copyright owner(s) are credited and that the original publication in this journal is cited, in accordance with accepted academic practice. No use, distribution or reproduction is permitted which does not comply with these terms.
*Correspondence: Zhengguo Li, emhlbmdndW9saUBjcXUuZWR1LmNu