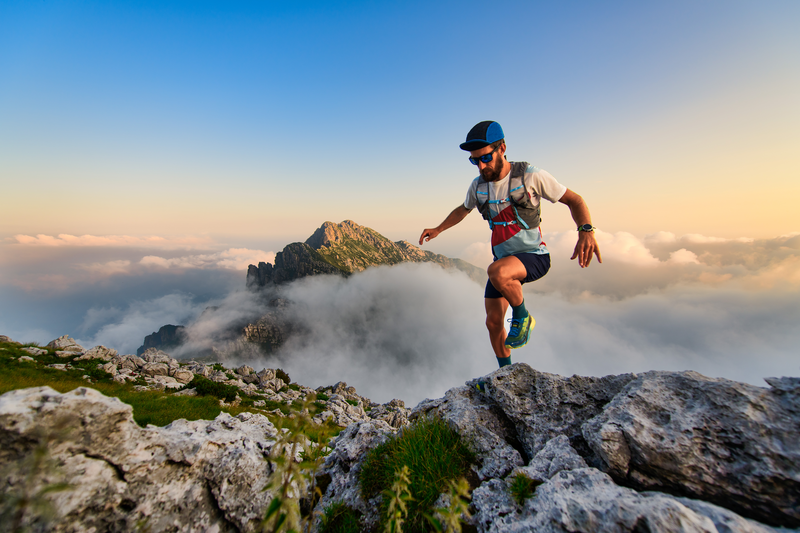
95% of researchers rate our articles as excellent or good
Learn more about the work of our research integrity team to safeguard the quality of each article we publish.
Find out more
ORIGINAL RESEARCH article
Front. Environ. Sci. , 27 November 2024
Sec. Soil Processes
Volume 12 - 2024 | https://doi.org/10.3389/fenvs.2024.1479712
This article is part of the Research Topic Reduction of Greenhouse Gas Emissions from Soil View all 7 articles
In rice paddies, which exhibit higher ammonia (NH₃) emission factors than upland soils, identifying key drivers of NH₃ flux intensity is crucial. Contrary to the commonly held view that NH₃ flux is primarily governed by soil ammonium (NH₄⁺) concentrations, we found no significant relationship between NH₃ flux and NH₄⁺ levels in the soil during rice cultivation. To pinpoint a primary factor influencing NH₃ flux intensity under conventional rice cropping practices, we conducted a 2-year field study applying four nitrogen (N) fertilization rates (0, 45, 90, and 180 kg N ha⁻¹) using urea [(NH₂)₂CO], the most common N fertilizer. NH₃ emissions were tracked using the ventilation method. Following N application, NH₃ flux sharply increased but rapidly returned to baseline. Half of the N applied as a basal fertilizer was incorporated within the soil, contributing only 10% of total NH₃ emissions. In contrast, top-dressed applications—20% of total N at the tillering stage and 30% at panicle initiation—accounted for approximately 90% of NH₃ loss. Seasonal NH₃ flux increased quadratically with rising N application rates, correlating strongly with NH₄⁺ concentrations in floodwater rather than soil. Grain yield responded quadratically to N levels, peaking at 120 kg N ha⁻¹ with a 37% increase over control yields. NH₃ flux intensity, defined as seasonal NH₃ flux per unit of grain yield, showed a quadratic response to N fertilization, decreasing with initial fertilizer additions (up to 38 kg N ha⁻¹) but then sharply increased with further N fertilization increase. Hence, reducing NH₄⁺ concentrations in floodwater through moderated N application and deeper fertilizer placement could be essential for minimizing NH₃ volatilization in rice systems.
The inevitable rise in global population necessitates a proportional increase in food production. The United Nations projects the population will reach 8.2 billion in 2025, increasing to 9.7 billion by 2050 (USDA, 2010). These trends indicate a continuous escalation in food demand. For instance, cereal production, needed for both human and animal consumption, is expected to reach 3 billion tons by 2050, up from the current 2.7 billion tons (Fao and AO, 2017). To meet these demands, crop production must intensify—an already challenging goal given the limited availability of arable land.
Among agricultural practices such as tillage, water management, and cultivar selection, fertilization remains the most effective for boosting crop productivity (Farooq F. et al., 2022). Of all nutrients applied, nitrogen (N) has the greatest impact on crop yields (Cagasan et al., 2020; He et al., 2024). For example, long-term studies in fertilized rice paddies in Korea showed that N fertilizer increased rice grain yields by an average of 45%, compared to yield increases of 9.8% and 5.1% with phosphorus (P) and potassium (K), respectively (Lee et al., 2008). However, this study also revealed that less than 30% of the applied N was absorbed by rice plants, leaving over 70% as a potential pollutant in the atmosphere, soil, and water (Cao et al., 2013). Soil N losses occur through leaching, runoff, denitrification, and volatilization (Cameron et al., 2013; Ma et al., 2019; Zeng et al., 2021). Notably, around 70% of the applied N is lost through NH₃ volatilization, with agriculture contributing approximately 80%–95% of atmospheric NH₃, stemming from N fertilization and livestock excretion (Sapek, 2013; Skorupka and Nosalewicz, 2021). Once emitted, NH₃ converts to ammonium (NH₄⁺) and forms fine particulate matter (PM₂.₅), which, when combined with anionic pollutants (SOx and NOx), can cause respiratory issues, including asthma and inflammation, impair lung function, and increase cancer risks (Xing et al., 2016; Yang L. et al., 2020).
In soils, NH₃ flux intensity is generally influenced by factors such as NH₄⁺ concentration, pH, and oxygen (O₂) levels (Chua et al., 2019; Merl and Koren, 2020; Kim et al., 2021). Higher NH₃ emissions are observed under conditions of elevated NH₄⁺ concentration, increased pH and temperature, and reduced O₂ (Rochette et al., 2013; Shen et al., 2020; Adegoke et al., 2022). Emissions are especially pronounced in flooded rice paddies compared to drier upland soils, due mainly to the lower O₂ levels in flooded soils (Li et al., 2017; Xu et al., 2020; Farooq F. et al., 2022). For example, in China, rice paddies exhibited a higher NH₃ emission factor (EF) of 0.179 kg NH₃-N per kg of applied N compared to 0.128 kg NH₃-N per kg in upland soils (Zhang et al., 2017; Farooq M. S. et al., 2022). Yet, many field studies have failed to establish clear relationships between NH₃ emission rates and these soil factors in flooded paddies (Wang et al., 2018), suggesting that additional factors may drive NH₃ flux intensity. While general factors affecting NH₃ emission are known, their specific impacts under varying N fertilization levels in rice paddies are less understood.
In this 2-year field study, we aimed to identify core factors affecting NH₃ flux intensity in flooded rice paddies. Using urea as the N source, we applied four different N levels across rice crops, monitoring NH₃ emissions alongside soil and water properties throughout the growing season to understand their role in NH₃ volatilization loss.
To identify key factors influencing NH₃ emission rates in a rice cropping system, a typical rice paddy was selected at the Gyeongsang National University experimental station in Jinju, Korea (35.15° N, 128.10° E) for a 2-year study. The site lies within a monsoon climate zone, with a 30-year average air temperature of 13.4°C and an annual precipitation of 1,518 mm (KMA, 2021). Mono-rice cultivation using conventional practices has been conducted on this field for over 50 years. The soil is a silt loam, classified as mixed, mesic Typic Endoaquepts (USDA, 2010), with an initial neutral pH of 6.0 (1:5 in H₂O) and low fertility, containing 19.4 g kg⁻1 organic C, 1.2 g kg⁻1 total N, and 31 mg kg⁻1 available P₂O₅.
Urea [(NH₂)₂CO] was chosen as the nitrogen (N) fertilizer. Based on the recommended fertilization rates for rice in Korea (N-P₂O₅-K₂O = 90–45–58 kg ha⁻1; RDA, 2019), four levels of N fertilization (0, 45, 90, and 180 kg N ha⁻1) were applied as treatments and maintained across both years of the study. The experimental design was a randomized complete block design (RCBD) with three replicates per treatment, resulting in 12 plots (10 m × 10 m each), separated by metal barriers. Nitrogen was applied in three split doses: 50% as a basal application, 20% at tillering, and 30% at panicle initiation. Basal fertilizers were incorporated mechanically into the surface layer (0–15 cm) 1 day before rice transplanting, while the tillering and panicle initiation applications were top-dressed at 15 and 45 days after transplanting, respectively. Phosphorus (P₂O₅, as superphosphate) and potassium (K₂O, as potassium chloride) were applied uniformly across all treatments.
Three-week-old rice seedlings (Sindongjin cultivar, Japonica type) were transplanted by hand at 15 cm × 30 cm spacing in late May, with harvest occurring in mid-October. The field was flooded to a depth of 5–7 cm, and water was drained 1 month before harvesting.
Ammonia (NH₃) emission rates were measured using a ventilation method (Nommik and Vahtras, 1982). A transparent acrylic chamber (12 cm in diameter, 25 cm in height) was utilized to hold a sponge that served as the primary NH₃ absorbent during each sampling period. This chamber was placed between rice plants, partially embedded into the soil. Each chamber contained two identical sponges: the lower sponge was positioned 8 cm above the soil surface to trap NH₃ emitted from the soil, while the upper sponge was placed 6 cm above the lower sponge to capture NH₃ from any external sources. All sponges were moistened with a 2 M H₃PO₄ and 4% glycerin solution (Wang et al., 2004), with H₃PO₄ acting as the main NH₃-trapping acid and glycerin reducing water evaporation from the sponge.
To minimize NH₃ loss during the study, NH₃ gas was collected systematically at controlled intervals. Sampling occurred daily for 1 week following nitrogen application, then every 2–3 days during the second and third weeks, and finally weekly, totaling 36 sampling sessions (Figure 1). Since the primary focus was on NH₃ emissions from the soil, only the lower sponges were collected for analysis, while the upper sponges, used solely to protect the lower sponges, were discarded. To extract trapped NH₃, the sponges were immediately soaked in a 2 M KCl solution, shaken at 120 rpm for 1 hour, then hand-squeezed, and filtered using a vacuum filter with Whatman 40 filter paper. The resulting NH₄⁺-N levels were measured by the indophenol blue method (Akao, 2023).
Figure 1. Changes in NH3 emission rates under different levels of N fertilization in rice paddy soil. Vertical bars indicate standard deviations (n = 3). Arrows indicate fertilization day.
The NH₃ emission rate was then calculated based on the NH₃ concentration trapped per unit surface area over each specified sampling interval.
where R: daily NH3 emission rate
The seasonal NH3 flux was computed by integrating the daily NH3 flux rate and sampling day interval:
where Ri: daily NH3 emission rate (kg N ha−1 d−1) of the ith sampling interval, Di: days between the ith and (i-1)th sampling interval, and n: number of samplings.
The NH3 emission factor (EF) was calculated by dividing the cumulative NH₃ emissions by the total nitrogen applied.
where EF: emission factor (%), Fluxes WNT: fluxes with N treatment (kg NH₃-N ha-1), Fluxes WoNT: fluxes without N treatment (kg NH₃-N ha-1) and total N applied (kg N ha−1).
The NH3 flux intensity was calculated by dividing the cumulative NH₃ emissions by the grain yield.
Soil temperature and redox potential were continuously monitored in the field throughout rice cultivation using automatic sensors and electrodes. These devices were installed at a soil depth of 5–10 cm and connected to a battery-powered data logger and an Eh meter, respectively. After harvesting, surface soil samples were collected for chemical analysis, including measurements of pH (1:5 with H₂O), organic carbon (using the Walkley-Black method), total N (via Kjeldahl digestion), available P₂O₅ (Lancaster method), and exchangeable cations—Ca2⁺, Mg2⁺, and K⁺—measured by ICP-OES following extraction with a 1 N ammonium acetate solution at pH 7.0.
Floodwater and soil samples were periodically collected during rice cultivation to monitor pH changes and NH₄⁺ concentrations. Floodwater samples were collected concurrently with NH₃ gas samples, while soil samples were taken at monthly intervals. To measure inorganic NH₄⁺ concentrations, floodwater was filtered through filter paper, and soils were extracted using a 2 M KCl solution. NH₄⁺-N concentrations were then quantified by the indophenol blue method (Tzollas et al., 2010).
At the rice maturation stage, ten representative plants were sampled manually from each plot. According to the Korean standards for rice yield assessment (RDA, 2019), grains and straw were separated using a thresher. Yield components, such as tiller number, panicles per tiller, 1000-grain weight, and ripening ratio, were measured. Finally, grain yield was standardized to a 14% moisture level, and the harvest index was calculated as the ratio of grain yield to total biomass.
Statistical analyses were carried out using the Statical Tool for Agricultural Research (STAR). The data were analyzed using analysis of variance (ANOVA) for RCBD. Separation of treatment effects was carried out using Tukey’s test at 5% level of significance. Regression analyses between N fertilizer level and seasonal NH3 flux, grain yield, and NH3 flux intensity were done using SigmaPlot10.
Soil temperature fluctuated similarly with changes in air temperature (Supplementary Figure S1), though no significant differences were observed among the various N fertilization treatments. Soil temperature gradually increased after rice transplanting, peaked at the reproductive stage (60–70 days post-transplanting), and then gradually declined. Both air and soil temperatures were slightly higher in the second year than in the first year of investigation, with cumulative air temperatures of 2,913°C and 2,942°C, respectively. The higher temperatures in the second year may have contributed to improved rice development and increased productivity.
Soil Eh values followed a typical pattern seen in rice cropping environments. Eh values dropped sharply from over 150 mV to between −150 and −260 mV within 2 weeks after flooding (Supplementary Figure S1). This highly reduced soil condition, with Eh values below −200 mV, was sustained until drainage in preparation for rice harvesting. Like soil temperature, soil Eh values showed no significant variation across different levels of N fertilization.
Similar NH₃ emission patterns were observed across both cropping years, regardless of N fertilization levels (Figure 1). Right after the application of chemical N fertilizer, NH₃ emission rates spiked sharply, peaking within 2–3 days, then rapidly declining to background levels. In this study, N fertilizer was applied in three stages: as a basal fertilizer (50% of total N) before transplanting, a tillering fertilizer (20%) on the 15th day after transplanting, and a panicle-initiating fertilizer (30%) on the 45th day after transplanting. Each N application triggered an increase in NH₃ emissions, with emission rates rising in proportion to the N fertilization level.
Seasonal NH₃ fluxes (Y, kg NH₃-N ha⁻1) increased significantly with higher N fertilization levels (X, kg N ha⁻1), following a quadratic relationship (Y = 46.4 + 0.028X + 0.0023X2, R2 = 0.816**) (Figure 2). In control plots (0 kg N ha⁻1), an average of 46.4 kg NH₃-N ha⁻1 was emitted, while adding 45, 90, and 180 kg N ha⁻1 of urea increased this flux by an average of 13%, 46%, and 171%, respectively. The ammonia emission factor (EF) (kg NH₃-N kg⁻1 N), which indicates net NH₃ emission losses from applied N fertilizer (Xu et al., 2019; Pan et al., 2022; Zhang et al., 2022), also showed a quadratic increase with higher N levels. For 45 kg N ha⁻1 of urea, the NH₃ EF averaged 0.13 kg NH₃-N kg⁻1 N, rising to approximately 0.23 and 0.44 kg NH₃-N kg⁻1 N for 90 and 180 kg N ha⁻1, respectively.
Figure 2. Cumulative NH3 emission, grain yield and NH3 flux intensity under different levels of N fertilization during rice cultivation. Vertical bars indicate standard deviations (n = 3).
The basal N fertilizer, incorporated into the surface soil layer 1 day before transplanting, accounted for only 8%–13% of total NH₃ emissions. In contrast, the tillering fertilizer (20% of total N), top-dressed at the tillering stage, contributed about 10%–15% of total NH₃ emissions. During the panicle initiation stage—typically the hottest period, around 45 days after transplanting—the remaining 30% of N fertilizer was broadcasted over the soil surface, accounting for 70%–80% of total NH₃ loss. This suggests that NH₃ flux intensity is strongly influenced by soil temperature and the method of fertilizer incorporation (Feng et al., 2017).
During rice cultivation, pH and NH₄⁺ concentrations, which directly influence NH₃ flux intensity (Rochette et al., 2013; Yu et al., 2020; Farooq M. S. et al., 2022), were monitored in both floodwater and soil. In floodwater, pH and NH₄⁺ concentrations fluctuated in parallel with NH₃ emission rates. While floodwater pH did not correlate precisely with urea application levels, it increased consistently following urea applications. In contrast, NH₄⁺ concentrations in floodwater showed a direct increase with higher urea application levels.
In comparison to the frequent floodwater monitoring, soil properties were analyzed only at monthly intervals rather than at each gas sampling. Due to this limited sampling frequency, no distinct response in soil pH and NH₄⁺ concentration to urea application levels was observed (Figure 3). Soil pH showed minimal sensitivity to urea-N fertilization levels, but NH₄⁺ concentrations increased proportionally with higher urea additions.
Figure 3. Changes in pH and NH4+ concentration in soil under different levels of N fertilization during rice cultivation. Vertical bars indicate standard deviations (n = 3).
Rice grain yield (Y, Mg ha⁻1) was significantly influenced by the level of N fertilization (X, kg N ha⁻1), following a negative-quadratic trend (Y = 5.36 + 0.033X - 1.37 × 10⁻⁴X2, R2 = 0.959**) (Figure 2). Grain productivity increased with higher N fertilization, reaching a maximum at approximately 120 kg N ha⁻1—about a 37% increase compared to the control (no N fertilization). Beyond this level, grain productivity decreased notably with further increases in N fertilization (Supplementary Table S1).
The NH₃ flux intensity, defined as seasonal NH₃ flux per unit of grain yield (Wang et al., 2018; Sun et al., 2019; Cai et al., 2023), was measured at 8.7 kg NH₃-N Mg⁻1 grain in the control treatment. This intensity (Y, kg NH₃-N Mg⁻1 grain) varied with N fertilization level (X, kg N ha⁻1) following a positive-quadratic response (Y = 8.7 - 0.039X + 0.0005X2, R2 = 0.749**) (Figure 2). While NH₃ flux intensity initially decreased slightly with N application up to approximately 38 kg N ha⁻1, it then increased sharply with higher levels of N fertilization.
Among the member countries of the Organization for Economic Cooperation and Development (OECD), Korea has the highest agricultural NH₃ flux intensity (Figure 4). In 2019, Korea emitted approximately 150 kg NH₃ ha⁻1 annually, which is 6.1 times higher than the OECD average of 24.7 kg ha⁻1 from the agricultural sector. Total NH₃ emissions in Korea increased by 37% over nearly 2 decades, from 231,000 tons in 2001 to 316,000 tons in 2019, primarily due to a significant rise in agricultural emissions (Figure 5). The agricultural sector contributed an average of 77% to total NH₃ emissions, closely aligned with the global agricultural contribution of 81% to NH₃ emissions (Van Damme et al., 2021). Agricultural NH₃ emissions in Korea rose by around 51% over the past 20 years, surpassing the 37% growth in overall NH₃ flux.
Figure 4. Comparison of agricultural NH3 emissions among the OECD countries in recent (2014–2019). The data of Columbia, Japan, and New Zealand were not available in the OECD statistics.
The main sources of agricultural NH₃ emissions are N-fertilized croplands and the livestock sector (Basosi et al., 2014; Zhang et al., 2018). Korea has the highest agricultural N balance among OECD countries, leading to considerable environmental impacts due to nitrogen release, including NH₃ emissions (Lim et al., 2021). This high N balance is attributed to intensive N input, primarily from chemical fertilizers and livestock manure. While chemical N fertilizer use decreased by 55% from 562,000 tons in 1990 to 251,000 tons in 2019, manure N production rose by 47%, from 206,000 tons in 1990 to 304,000 tons in 2019 (https://stats.oecd.org). Although chemical N fertilizer was the main source of NH₃ emissions in the 1990s, livestock manure has become the more significant contributor in recent years.
NH₃ emission losses are mainly influenced by NH₄⁺ concentration, soil pH, and oxygen levels (Wang et al., 2018; Yang L. et al., 2020). The rapid hydrolysis of urea fertilizer, driven by soil moisture, converts urea into NH₄⁺ and hydroxide ions (OH⁻), increasing NH₄⁺ concentrations in soil and thereby accelerating NH₃ volatilization (Yao et al., 2018; Li et al., 2023). Environmental factors, such as light intensity and air temperature, also affect NH₃ volatilization, as these conditions impact surface water temperature (Chen et al., 2015). Changes in solar radiation and temperature elevate NH₃ emission rates by increasing the surface compensation point and altering resistance dynamics (Loubet et al., 2018). Soil moisture further affects the NH₄⁺/NH₃ balance; higher soil water potential increases NH₄⁺ concentrations when NH₄⁺ supply exceeds NH₃ oxidation. Above field capacity, however, this balance shifts, impacting nitrification rates (Cameron et al., 2013; Yun and Ro, 2014).
The Intergovernmental Panel on Climate Change (IPCC) has proposed a global ammonia (NH₃) emission factor (EF) of 10% of nitrogen (N) applied to cropping lands, with an uncertainty range of 3%–30%. In 2019, the IPCC updated the NH₃ EF for various N fertilizer types, spanning from 0.2% to 14.2% (Hergoualc’h et al., 2019). A recent meta-analysis by Ma et al. (2021) estimated global NH₃ EF values of 12.56% for synthetic N fertilizers and 13.95% specifically for rice fields. However, in our study of the selected rice paddy, NH₃ EF increased exponentially with rising N fertilization levels, from approximately 13% at 45 kg N ha⁻1 of urea application to 23% and 44% at 90 and 180 kg N ha⁻1, respectively (Figure 2). This suggests that NH₃ EF should be adjusted based on N fertilizer input level (Ma et al., 2021; Wang et al., 2021), emphasizing the need for lower N fertilization to reduce NH₃ emissions in flooded rice paddies (Fan et al., 2011; Shan et al., 2015). In Korea, 90 kg ha⁻1 of N fertilization is recommended for rice, with urea as the primary N source, resulting in an estimated NH₃-N EF of around 23%.
Rice cropping is known to have a higher NH₃ EF than upland crops, largely due to lower oxygen (O₂) levels in paddy soils (Lee et al., 2024). The limited O₂ in waterlogged conditions inhibits nitrification, the process converting NH₄⁺-N to NO₃⁻-N, which leads to increased NH₃ emissions (Nurulhuda et al., 2018; Yang W. et al., 2020). In our mono-rice paddy field, which has a rice-growing period of 100–140 days followed by over 200 days of fallow period, soil Eh dropped from 150 mV to −200 mV shortly after flooding for rice cultivation (Supplementary Figure S1). This reduction process raised soil pH to between 7.5 and 9.0, further enhancing NH₃ emissions (Feng et al., 2017; Kim et al., 2021). Elevated pH levels were sustained throughout the flooded period (Figure 3) (Guo et al., 2018; Ding et al., 2019).
Among synthetic N fertilizers, urea leads to the highest NH₃ EF due to its rapid hydrolysis, which creates localized increases in soil pH that promote NH₃ volatilization (Bittman et al., 2014). Regardless of urea-N fertilization levels, NH₃ emissions spike shortly after N fertilizer application and quickly return to baseline (Figure 1). However, NH₃ EFs for N fertilizers can vary significantly depending on soil, climate, and site-specific management practices (Pan et al., 2016; Sommer et al., 2004). In this study, half of the urea was incorporated as basal fertilizer before rice transplanting, resulting in only 10% of the total NH₃ flux, whereas top-dressing 20% and 30% of urea-N at tillering and panicle initiation stages contributed 10%–15% and 70%–80% of total NH₃ fluxes, respectively. The basal fertilizer mixed into the plow layer likely led to lower NH₃ emissions due to NH₄⁺ adsorption onto clay particles (Xia et al., 2020; Mirkhani et al., 2023), in contrast to top-dressing at tillering and panicle initiation. Similar reductions in NH₃ volatilization and runoff losses were observed with mechanical side deep fertilization (MSDF) (Min et al., 2021). Moreover, a field investigation in China revealed that deep placement of urea-N fertilizer has indicated effective reduction in NH₃ volatilization to 1%–2% of the applied N, achieving a 91% reduction compared to surface broadcasting (Yao et al., 2018). Our data showed that NH₄⁺ concentration and pH in floodwater significantly increased after top-dressing at these stages (Figure 6), underscoring the importance of incorporating N fertilizer into the soil layer to reduce NH₃ emissions (Rochette et al., 2013; Huang et al., 2016).
Figure 6. Changes in pH and NH4+ concentration in floodwater under different levels of N fertilization during rice cultivation. Vertical bars indicate standard deviations (n = 3). Arrows indicate fertilization day.
In this study, NH₃ emissions correlated more strongly with NH₄⁺ concentrations in floodwater than in soil (Figure 7). While NH₃ flux is typically linked to soil NH₄⁺ levels, floodwater NH₄⁺ concentration was the primary determinant of NH₃ emissions in this rice paddy (Li et al., 2008; Yao et al., 2018; Canatoy et al., 2024). These findings suggest that incorporating chemical N fertilizers below the surface, rather than surface application, could effectively reduce NH₄⁺ concentration in floodwater (Li et al., 2014; Gaihre et al., 2015), thus limiting NH₃ volatilization during rice growth (Zhang et al., 2017; Yao et al., 2018). Moreover, the depth of N fertilizer placement should be selected with caution, as the upward movement of NH4+-N can result in its entry into floodwater. In the absence of site-specific data, a placement depth of 10 cm is recommended. However, for sandy loam soils, deeper placement may be required to mitigate potential nitrogen losses (Liu et al., 2015). Controlled-release N fertilizers have similarly been shown to decrease NH₃ losses by reducing NH₄⁺ levels in surface water (Xu et al., 2012).
Figure 7. Relationship between NH3 emission rates to NH4+ concentration in floodwater under different levels of N fertilization during rice cultivation. Vertical bars indicate standard deviations (n = 3).
NH₃ flux intensity, defined as total NH₃ flux per unit of grain yield (Wang et al., 2018), was 8.7 kg NH₃-N Mg⁻1 grain in the control treatment and showed a quadratic relationship with N fertilization level (Figure 2). While NH₃ flux intensity decreased slightly with a 38 kg N ha⁻1 urea application to 7.9 kg N Mg⁻1 grain, it increased sharply at higher N levels. At 120 kg N ha⁻1—the application rate for peak grain yield—the NH₃ flux intensity was 11.4 kg N Mg⁻1 grain, whereas the recommended 90 kg N ha⁻1 reduced this intensity by 2.1 kg N Mg⁻1 grain.
Rice grain yields differed by only 1.7% between the 120 and 90 kg N ha⁻1 applications, indicating that reduced N fertilization may be a viable strategy to mitigate NH₃ emissions without compromising yield (Subbarao and Searchinger, 2021). Additionally, alternative fertilizers like ammonium sulfate could further reduce NH₃ volatilization, though the specific reaction mechanisms warrant further investigation.
During rice cultivation, the majority of NH₃ emissions occurred shortly after the application of chemical N fertilizer. Total NH₃ flux exhibited a quadratic increase with rising N application levels. Basal N fertilization (50% of total N) was incorporated into the surface soil layer, slightly impacting total NH₃ flux. In contrast, the remaining urea-N was broadcasted on the soil surface during the tillering (20% of total N) and panicle initiation stages (30% of total N), contributing to 87%–92% of total NH₃ flux. NH₃ emission rates were strongly correlated with dissolved NH₄⁺-N concentration in floodwater rather than soil concentration. Incorporating N fertilizer into soil layers instead of broadcasting it on the surface effectively reduced NH₄⁺-N concentration in floodwater, thereby lowering NH₃ volatilization losses. While NH₃ flux intensity initially decreased with an additional 38 kg N ha⁻1 application, it increased significantly with higher N rates. In conclusion, to mitigate NH₃ volatilization losses in rice paddies, reducing N fertilization and incorporating fertilizer within the soil layer, rather than surface broadcasting, are essential strategies.
The original contributions presented in the study are included in the article/Supplementary Material, further inquiries can be directed to the corresponding authors.
RC: Conceptualization, Data curation, Formal Analysis, Investigation, Methodology, Project administration, Software, Writing–original draft, Writing–review and editing. SC: Investigation, Project administration, Resources, Writing–review and editing. SG: Formal Analysis, Investigation, Writing–review and editing. PK: Conceptualization, Funding acquisition, Project administration, Resources, Supervision, Writing–original draft, Writing–review and editing. GK: Project administration, Supervision, Writing–review and editing.
The author(s) declare that financial support was received for the research, authorship, and/or publication of this article. The author(s) declare that financial support was received for the research, authorship, and/or publication of this article. This work received funding from the Basic Science Research Program through the National Research Foundation of Korea, funded by the Ministry of Education (NRF-2023R1A2C3004842). Authors RC, SC, and SG were supported by scholarships from the BK21 Four program of the Ministry of Education and Human Resources Development, Republic of Korea. The funders were not involved in the study design, collection, analysis, or interpretation of data, the writing of this article, or the decision to submit it for publication.
The authors thankfully acknowledge the Gyeongsang National University for granting necessary permissions and providing the facilities to carry out the work.
The authors declare that the research was conducted in the absence of any commercial or financial relationships that could be construed as a potential conflict of interest.
All claims expressed in this article are solely those of the authors and do not necessarily represent those of their affiliated organizations, or those of the publisher, the editors and the reviewers. Any product that may be evaluated in this article, or claim that may be made by its manufacturer, is not guaranteed or endorsed by the publisher.
The Supplementary Material for this article can be found online at: https://www.frontiersin.org/articles/10.3389/fenvs.2024.1479712/full#supplementary-material
Adegoke, T. O., Moon, T. I., and Ku, H. H. (2022). Ammonia emission from sandy loam soil amended with manure compost and urea. Appl. Biol. Chem. 65 (1), 83. doi:10.1186/s13765-022-00752-4
Akao, S. (2023). Aliphatic amine interference in indophenol reaction for ammonia-nitrogen determination and its possibility as quantitative method for aliphatic amines. J. Water Environ. 46 (1), 1–9. doi:10.2965/jswe.46.1
Basosi, R., Spinelli, D., Fierro, A., and Jez, S. (2014). Mineral nitrogen fertilizers: environmental impact of production and use. Fertil. Compon. Uses Agric. Environ. Impacts 1, 3–43.
Bittman, S., Brook, J. R., Bleeker, A., and Bruulsema, T. W. (2014). Air quality, health effects and management of ammonia emissions from fertilizers. Air Qual. Manag. Can. Perspect. a Glob. Issue, 261–277. doi:10.1007/978-94-007-7557-2_12
Cagasan, U., Libre, M. J. P., Santiago, D. A., Cantoneros, J. A., Tumaca, C. M., Pablo, A., et al. (2020). Assessment on the nutrient status of lowland rice soil using minus one element technique (MOET). Eurasian J. Agric. Res. 4 (1), 56–63.
Cai, S., Zhao, X., Liu, X., and Yan, X. (2023). Nitrogen management to minimize yield-scaled ammonia emission from paddy rice in the Middle and Lower Yangtze River Basin: a meta-analysis. Environ. Pollut. 318, 120854. doi:10.1016/j.envpol.2022.120854
Cameron, K. C., Di, H. J., and Moir, J. L. (2013). Nitrogen losses from the soil/plant system: a review. Ann. Appl. Biol. 162 (2), 145–173. doi:10.1111/aab.12014
Canatoy, R. C., Cho, S. R., Galgo, S. J. C., Park, S. Y., and Kim, P. J. (2024). Biochar manure decreases ammonia volatilization loss and sustains crop productivity in rice paddy. Front. Environ. Sci. 12, 1421320. doi:10.3389/fenvs.2024.1421320
Cao, Y., Tian, Y., Yin, B., and Zhu, Z. (2013). Assessment of ammonia volatilization from paddy fields under crop management practices aimed to increase grain yield and N efficiency. Field Crops Res. 147, 23–31. doi:10.1016/j.fcr.2013.03.015
Chen, A., Lei, B., Hu, W., Lu, Y., Mao, Y., Duan, Z., et al. (2015). Characteristics of ammonia volatilization on rice grown under different nitrogen application rates and its quantitative predictions in Erhai Lake Watershed, China. Nutrient Cycl. Agroecosyst. 101, 139–152. doi:10.1007/s10705-014-9660-7
Chua, F. J. D., Sun, F., Mukherjee, M., and Zhou, Y. (2019). Comparison of nitrous oxide emission between a partial and full nitrification enriched ammonia-oxidising culture. Chemosphere 220, 974–982. doi:10.1016/j.chemosphere.2018.12.204
Ding, C., Du, S., Ma, Y., Li, X., Zhang, T., and Wang, X. (2019). Changes in the pH of paddy soils after flooding and drainage: modeling and validation. Geoderma 337, 511–513. doi:10.1016/j.geoderma.2018.10.012
Fan, X. H., Li, Y. C., and Alva, A. K. (2011). Effects of temperature and soil type on ammonia volatilization from slow-release nitrogen fertilizers. Commun. soil Sci. plant analysis 42 (10), 1111–1122. doi:10.1080/00103624.2011.566957
Fao, F.AO (2017). The future of food and agriculture–trends and challenges. future food Agric. trends challenges 4 (4).
Farooq, F., Choudhary, R. K., Kumawat, S. N., Gulshan, T., Singh, B., and Bhadu, A. (2022a). Customized fertilizer: a key for enhanced crop production. Int. J. Plant and Soil Sci., 954–964. doi:10.9734/ijpss/2022/v34i232505
Farooq, M. S., Uzair, M., Maqbool, Z., Fiaz, S., Yousuf, M., Yang, S. H., et al. (2022b). Improving nitrogen use efficiency in aerobic rice based on insights into the ecophysiology of archaeal and bacterial ammonia oxidizers. Front. Plant Sci. 13, 913204. doi:10.3389/fpls.2022.913204
Feng, Y., Guo, X. H., Sun, X. H., Ma, J. J., Lei, T., and Wang, H. Y. (2017). Research on the predication model of ammonia volatilization accumulation under moisture content and temperature. China Rural Water hydropower 8, 52–61.
Gaihre, Y. K., Singh, U., Islam, S. M., Huda, A., Islam, M. R., Satter, M. A., et al. (2015). Impacts of urea deep placement on nitrous oxide and nitric oxide emissions from rice fields in Bangladesh. Geoderma 259, 370–379. doi:10.1016/j.geoderma.2015.06.001
Guo, X., Li, H., Yu, H., Li, W., Ye, Y., and Biswas, A. (2018). Drivers of spatio-temporal changes in paddy soil pH in Jiangxi Province, China from 1980 to 2010. Sci. Rep. 8 (1), 2702. doi:10.1038/s41598-018-20873-5
He, X., Zhu, H., Shi, A., and Wang, X. (2024). Optimizing nitrogen fertilizer management enhances rice yield, dry matter, and nitrogen use efficiency. Agronomy 14 (5), 919. doi:10.3390/agronomy14050919
Hergoualc’h, K., Akiyama, H., Bernoux, M., Chirinda, N., Prado, A. D., Kasimir, Å., et al. 2019. N2O emissions from managed soils, and CO2 emissions from lime and urea application.
Huang, S., Lv, W., Bloszies, S., Shi, Q., Pan, X., and Zeng, Y. (2016). Effects of fertilizer management practices on yield-scaled ammonia emissions from croplands in China: a meta-analysis. Field crops Res. 192, 118–125. doi:10.1016/j.fcr.2016.04.023
Kim, M. S., Min, H. G., Koo, N., and Kim, J. G. (2021). Response to ammonia emission flux to different pH conditions under biochar and liquid fertilizer application. Agriculture 11 (2), 136. doi:10.3390/agriculture11020136
Lee, C. H., Kang, U. G., Park, K. D., Lee, D. K., and Kim, P. J. (2008). Long-term fertilization effects on rice productivity and nutrient efficiency in Korean paddy. J. plant Nutr. 31 (8), 1496–1506. doi:10.1080/01904160802208675
Lee, Y. J., Im, E. C., Lee, G., Hong, S. C., Lee, C. G., and Park, S. J. (2024). Comparison of ammonia volatilization in paddy and field soils fertilized with urea and ammonium sulfate during rice, potato, and Chinese cabbage cultivation. Atmos. Pollut. Res. 15 (4), 102049. doi:10.1016/j.apr.2024.102049
Li, H., Liang, X., Chen, Y., Tian, G., and Zhang, Z. (2008). Ammonia volatilization from urea in rice fields with zero-drainage water management. Agric. Water Manag. 95 (8), 887–894. doi:10.1016/j.agwat.2007.05.016
Li, T., Cui, J., Guo, W., She, Y., and Li, P. (2023). The influence of organic and inorganic fertilizer applications on nitrogen transformation and yield in greenhouse tomato cultivation with surface and drip irrigation techniques. Water 15 (20), 3546. doi:10.3390/w15203546
Li, X., Xia, L., and Yan, X. (2014). Application of membrane inlet mass spectrometry to directly quantify denitrification in flooded rice paddy soil. Biol. Fertil. soils 50, 891–900. doi:10.1007/s00374-014-0910-2
Li, Y., Huang, L., Zhang, H., Wang, M., and Liang, Z. (2017). Assessment of ammonia volatilization losses and nitrogen utilization during the rice growing season in alkaline salt-affected soils. Sustainability 9 (1), 132. doi:10.3390/su9010132
Lim, J. Y., Bhuiyan, M. S. I., Lee, S. B., Lee, J. G., and Kim, P. J. (2021). Agricultural nitrogen and phosphorus balances of Korea and Japan: highest nutrient surplus among OECD member countries. Environ. Pollut. 286, 117353. doi:10.1016/j.envpol.2021.117353
Liu, T. Q., Fan, D. J., Zhang, X. X., Chen, J., Li, C. F., and Cao, C. G. (2015). Deep placement of nitrogen fertilizers reduces ammonia volatilization and increases nitrogen utilization efficiency in no-tillage paddy fields in central China. Field Crops Res. 184, 80–90. doi:10.1016/j.fcr.2015.09.011
Loubet, B., Carozzi, M., Voylokov, P., Cohan, J. P., Trochard, R., and Génermont, S. (2018). Evaluation of a new inference method for estimating ammonia volatilisation from multiple agronomic plots. Biogeosciences 15 (11), 3439–3460. doi:10.5194/bg-15-3439-2018
Ma, R., Zou, J., Han, Z., Yu, K., Wu, S., Li, Z., et al. (2021). Global soil-derived ammonia emissions from agricultural nitrogen fertilizer application: a refinement based on regional and crop-specific emission factors. Glob. Change Biol. 27 (4), 855–867. doi:10.1111/gcb.15437
Ma, Z., Yue, Y., Feng, M., Li, Y., Ma, X., Zhao, X., et al. (2019). Mitigation of ammonia volatilization and nitrate leaching via loss control urea triggered H-bond forces. Sci. Rep. 9 (1), 15140. doi:10.1038/s41598-019-51566-2
Merl, T., and Koren, K. (2020). Visualizing NH3 emission and the local O2 and pH microenvironment of soil upon manure application using optical sensors. Environ. Int. 144, 106080. doi:10.1016/j.envint.2020.106080
Min, J., Sun, H., Wang, Y., Pan, Y., Kronzucker, H. J., Zhao, D., et al. (2021). Mechanical side-deep fertilization mitigates ammonia volatilization and nitrogen runoff and increases profitability in rice production independent of fertilizer type and split ratio. J. Clean. Prod. 316, 128370. doi:10.1016/j.jclepro.2021.128370
Mirkhani, R., Shorafa, M., Roozitalab, M. H., Heng, L. K., and Dercon, G. (2023). Ammonia emission and nitrogen use efficiency with application of nitrification inhibitor and plant growth regulator in a calcareous soil (Karaj, Iran). Geoderma Reg. 35, e00718. doi:10.1016/j.geodrs.2023.e00718
Nommik, H., Vahtras, K., and Huang, W. (1982). Retention and fixation of ammonium and ammonia in soils. Nitrogen in agricultural soils 22, 123–171.
Nurulhuda, K., Gaydon, D. S., Jing, Q., Zakaria, M. P., Struik, P. C., and Keesman, K. J. (2018). Nitrogen dynamics in flooded soil systems: an overview on concepts and performance of models. J. Sci. Food Agric. 98 (3), 865–871. doi:10.1002/jsfa.8683
Pan, S. Y., He, K. H., Lin, K. T., Fan, C., and Chang, C. T. (2022). Addressing nitrogenous gases from croplands toward low-emission agriculture. Npj Clim. Atmos. Sci. 5 (1), 43. doi:10.1038/s41612-022-00265-3
Pan, Y., Tian, S., Liu, D., Fang, Y., Zhu, X., Zhang, Q., et al. (2016). Fossil fuel combustion-related emissions dominate atmospheric ammonia sources during severe haze episodes: evidence from 15N-stable isotope in size-resolved aerosol ammonium. Environ. Sci. and Technol. 50 (15), 8049–8056. doi:10.1021/acs.est.6b00634
Rochette, P., Angers, D. A., Chantigny, M. H., Gasser, M. O., MacDonald, J. D., Pelster, D. E., et al. (2013). Ammonia volatilization and nitrogen retention: how deep to incorporate urea? J. Environ. Qual. 42 (6), 1635–1642. doi:10.2134/jeq2013.05.0192
Shan, L., He, Y., Chen, J., Huang, Q., and Wang, H. (2015). Ammonia volatilization from a Chinese cabbage field under different nitrogen treatments in the Taihu Lake Basin, China. J. Environ. Sci. 38, 14–23. doi:10.1016/j.jes.2015.04.028
Shen, H., Chen, Y., Hu, Y., Ran, L., Lam, S. K., Pavur, G. K., et al. (2020). Intense warming will significantly increase cropland ammonia volatilization threatening food security and ecosystem health. One Earth 3 (1), 126–134. doi:10.1016/j.oneear.2020.06.015
Skorupka, M., and Nosalewicz, A. (2021). Ammonia volatilization from fertilizer urea—a new challenge for agriculture and industry in view of growing global demand for food and energy crops. Agriculture 11 (9), 822. doi:10.3390/agriculture11090822
Sommer, S. G., Petersen, S. O., and Møller, H. B. (2004). Algorithms for calculating methane and nitrous oxide emissions from manure management. Nutrient Cycl. Agroecosyst. 69, 143–154. doi:10.1023/b:fres.0000029678.25083.fa
Subbarao, G. V., and Searchinger, T. D. (2021). Opinion: a “more ammonium solution” to mitigate nitrogen pollution and boost crop yields. Proc. Natl. Acad. Sci. 118 (22), e2107576118. doi:10.1073/pnas.2107576118
Sun, X., Zhong, T., Zhang, L., Zhang, K., and Wu, W. (2019). Reducing ammonia volatilization from paddy field with rice straw derived biochar. Sci. Total Environ. 660, 512–518. doi:10.1016/j.scitotenv.2018.12.450
Tzollas, N. M., Zachariadis, G. A., Anthemidis, A. N., and Stratis, J. A. (2010). A new approach to indophenol blue method for determination of ammonium in geothermal waters with high mineral content. Int. J. Environ. Anal. Chem. 90 (2), 115–126. doi:10.1080/03067310902962528
USDA (2010). Keys to soil taxonomy. 11th ed. Washington, DC: USDA-Natural Resources Conservation Service.
Van Damme, M., Clarisse, L., Franco, B., Sutton, M. A., Erisman, J. W., Kruit, R. W., et al. (2021). Global, regional and national trends of atmospheric ammonia derived from a decadal (2008–2018) satellite record. Environ. Res. Lett. 16 (5), 055017. doi:10.1088/1748-9326/abd5e0
Wang, C., Cheng, K., Ren, C., Liu, H., Sun, J., Reis, S., et al. (2021). An empirical model to estimate ammonia emission from cropland fertilization in China. Environ. Pollut. 288, 117982. doi:10.1016/j.envpol.2021.117982
Wang, H., Zhang, D., Zhang, Y., Zhai, L., Yin, B., Zhou, F., et al. (2018). Ammonia emissions from paddy fields are underestimated in China. Environ. Pollut. 235, 482–488. doi:10.1016/j.envpol.2017.12.103
Wang, Z. H., Liu, X. J., Ju, X. T., Zhang, F. S., and Malhi, S. S. (2004). Ammonia volatilization loss from surface-broadcast urea: comparison of vented-and closed-chamber methods and loss in winter wheat–summer maize rotation in North China Plain. Commun. Soil Sci. Plant Analysis 35 (19-20), 2917–2939. doi:10.1081/css-200036499
Xia, L., Li, X., Ma, Q., Lam, S. K., Wolf, B., Kiese, R., et al. (2020). Simultaneous quantification of N2, NH3 and N2O emissions from a flooded paddy field under different N fertilization regimes. Glob. Change Biol. 26 (4), 2292–2303. doi:10.1111/gcb.14958
Xing, Y. F., Xu, Y. H., Shi, M. H., and Lian, Y. X. (2016). The impact of PM2.5 on the human respiratory system. J. Thorac. Dis. 8 (1), E69–E74. doi:10.3978/j.issn.2072-1439.2016.01.19
Xu, C., Zhang, K., Zhu, W., Xiao, J., Zhu, C., Zhang, N., et al. (2020). Large losses of ammonium-nitrogen from a rice ecosystem under elevated CO2. Sci. Adv. 6 (42), eabb7433. doi:10.1126/sciadv.abb7433
Xu, J., Peng, S., Yang, S., and Wang, W. (2012). Ammonia volatilization losses from a rice paddy with different irrigation and nitrogen managements. Agric. Water Manag. 104, 184–192. doi:10.1016/j.agwat.2011.12.013
Xu, R., Tian, H., Pan, S., Prior, S. A., Feng, Y., Batchelor, W. D., et al. (2019). Global ammonia emissions from synthetic nitrogen fertilizer applications in agricultural systems: empirical and process-based estimates and uncertainty. Glob. change Biol. 25 (1), 314–326. doi:10.1111/gcb.14499
Yang, L., Li, C., and Tang, X. (2020a). The impact of PM2. 5 on the host defense of respiratory system. Front. Cell Dev. Biol. 8, 91. doi:10.3389/fcell.2020.00091
Yang, W., Que, H., Wang, S., Zhu, A., Zhang, Y., He, Y., et al. (2020b). High temporal resolution measurements of ammonia emissions following different nitrogen application rates from a rice field in the Taihu Lake Region of China. Environ. Pollut. 257, 113489. doi:10.1016/j.envpol.2019.113489
Yao, Y., Zhang, M., Tian, Y., Zhao, M., Zhang, B., Zhao, M., et al. (2018). Urea deep placement for minimizing NH3 loss in an intensive rice cropping system. Field Crops Res. 218, 254–266. doi:10.1016/j.fcr.2017.03.013
Yu, S., Xue, L., Feng, Y., Liu, Y., Song, Z., Mandal, S., et al. (2020). Hydrochar reduced NH3 volatilization from rice paddy soil: microbial-aging rather than water-washing is recommended before application. J. Clean. Prod. 268, 122233. doi:10.1016/j.jclepro.2020.122233
Yun, S. I., and Ro, H. M. (2014). Can nitrogen isotope fractionation reveal ammonia oxidation responses to varying soil moisture? Soil Biol. Biochem. 76, 136–139. doi:10.1016/j.soilbio.2014.04.032
Zeng, F., Zuo, Z., Mo, J., Chen, C., Yang, X., Wang, J., et al. (2021). Runoff losses in nitrogen and phosphorus from paddy and maize cropping systems: a field study in Dongjiang Basin, South China. Front. Plant Sci. 12, 675121. doi:10.3389/fpls.2021.675121
Zhang, L., Chen, Y., Zhao, Y., Henze, D. K., Zhu, L., Song, Y., et al. (2018). Agricultural ammonia emissions in China: reconciling bottom-up and top-down estimates. Atmos. Chem. Phys. 18 (1), 339–355. doi:10.5194/acp-18-339-2018
Zhang, M., Yao, Y., Zhao, M., Zhang, B., Tian, Y., Yin, B., et al. (2017). Integration of urea deep placement and organic addition for improving yield and soil properties and decreasing N loss in paddy field. Agric. Ecosyst. and Environ. 247, 236–245. doi:10.1016/j.agee.2017.07.001
Keywords: urea, ammonia emission intensity, ammonia emission factor, fertilizer incorporation, rice paddy
Citation: Canatoy RC, Cho SR, Galgo SJC, Kim PJ and Kim GW (2024) Reducing ammonia volatilization in rice paddy: the importance of lower fertilizer rates and soil incorporation. Front. Environ. Sci. 12:1479712. doi: 10.3389/fenvs.2024.1479712
Received: 17 August 2024; Accepted: 11 November 2024;
Published: 27 November 2024.
Edited by:
Yao Zhang, Colorado State University, United StatesReviewed by:
Muhammad Rahil Afzal, Jiangsu University, ChinaCopyright © 2024 Canatoy, Cho, Galgo, Kim and Kim. This is an open-access article distributed under the terms of the Creative Commons Attribution License (CC BY). The use, distribution or reproduction in other forums is permitted, provided the original author(s) and the copyright owner(s) are credited and that the original publication in this journal is cited, in accordance with accepted academic practice. No use, distribution or reproduction is permitted which does not comply with these terms.
*Correspondence: Pil Joo Kim, cGpraW1AZ251LmFjLmty; Gil Won Kim, Z2lsd29ua2ltNzRAZ21haWwuY29t
Disclaimer: All claims expressed in this article are solely those of the authors and do not necessarily represent those of their affiliated organizations, or those of the publisher, the editors and the reviewers. Any product that may be evaluated in this article or claim that may be made by its manufacturer is not guaranteed or endorsed by the publisher.
Research integrity at Frontiers
Learn more about the work of our research integrity team to safeguard the quality of each article we publish.