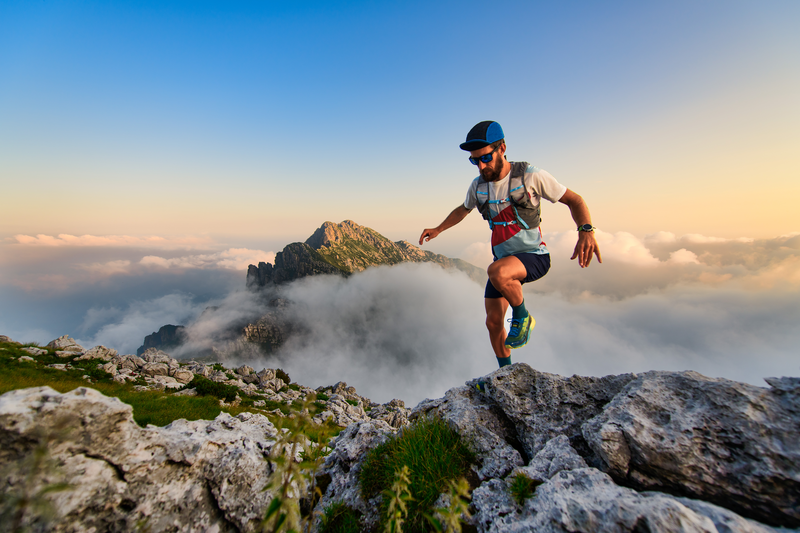
95% of researchers rate our articles as excellent or good
Learn more about the work of our research integrity team to safeguard the quality of each article we publish.
Find out more
ORIGINAL RESEARCH article
Front. Environ. Sci. , 09 January 2025
Sec. Environmental Informatics and Remote Sensing
Volume 12 - 2024 | https://doi.org/10.3389/fenvs.2024.1476761
Flood risk assessment is crucial for effective disaster risk management and community resilience. However, the current research lacks strength in identifying high-risk areas, implementing flood early warning systems, prioritising risk reduction measures, and allocating resources for emergency response planning and management. This study aims to assess flood hazard in Mirzadhare, Charsadda a highly flood-prone area in Khyber Pakhtunkhwa province of Pakistan. The study used an integrated approach by employing geographical information system (GIS) and multi-criteria decision analysis (MCDA) techniques. Further, the study used multiple datasets, including rainfall, stream density, and village points to map out flood susceptibility in the study region. Data was collected from field surveys, questionnaires, and interviews, allowing for a detailed analysis of flood hazards. Selecting average precipitation, peak river flow, and historical flood frequency as indicators, the weights of the three are 0.4, 0.3, and 0.3 respectively, an indicator system for predicting flood disasters was constructed. The results categorised the study area into four hazard zones: very high, high, medium, and low, based on their susceptibility to flood hazards. The study findings reveal that more than 65% of the area, including agricultural land with other livelihood settlements, is at a very high risk of flood hazard. Over 50% of the population lives in floodplains and faces an extremely high risk of future flood events. The precision of the results may have been affected by the accuracy and completeness of the data sources utilised, such as historical flood records, precipitation data, stream network data, and stream density. This combination of methods enabled the creation of accurate, data-driven flood risk maps. The hazard map of the area serves as a valuable tool for decision-making, resource allocation, and the development of flood risk management strategies. Based on the study findings, regular updates and continuous monitoring are recommended to ensure the accuracy and relevance of the flood hazard information over time.
Flood is a hydro-metrological event that poses a significant risk to communities residing in flood-prone areas. A flood hazard refers to the potential occurrence of a water overflow event, while a flood risk represents the probability and potential consequences (Ali, 2007). Flood hazards result from a combination of factors, including intense rainfall, topography, soil characteristics, land use patterns, and the presence of rivers or other bodies of water. Areas located in floodplains or low-lying regions are particularly vulnerable to flood hazards due to their proximity to water sources, limited natural drainage capabilities, and additional factors such as land use practices, deforestation, and climate variability, all of which further exacerbate flood risks (Fida et al., 2022). The limited drainage network, particularly in floodplain and low-lying areas, exacerbates flood risks by reducing the capacity for effective water flow and drainage. This phenomenon is compounded by factors such as poor maintenance, increased urbanization, and changes in land use. Studies conducted by Valjarević (2024) have highlighted the critical role of river properties and drainage networks in flood management, emphasizing their influence on flood capacity and hazard mitigation. The severity of flood hazards can vary, ranging from localized flash floods to widespread riverine flooding, depending on the scale and intensity of the precipitation event (Rehman et al., 2022). The probability of flooding is determined by considering historical data, climate patterns, and hydrological modelling (Hamidi et al., 2022). Flood return periods, such as the 10-year, 50-year, and 100-year floods, were estimated using probability-based statistical methods, specifically Extreme Value Theory (EVT) and the Gumbel Distribution. These approaches are commonly used in flood frequency analysis to model the likelihood of extreme flood events. The Gumbel Distribution is particularly suitable for modeling the distribution of maximum annual flood data, such as peak discharge or flood levels, as it captures the behavior of extreme events (Al Khoury et al., 2023). The return period is calculated by determining the exceedance probability of a given flood magnitude, where a 100-year flood represents an event with a 1% chance of occurring in any given year. This method allows for the estimation of the likelihood of floods of varying magnitudes over the long term and is integral to our hazard risk assessment (Alexander et al., 2011; Bibi et al., 2018). The magnitude of potential impacts depends on various factors, including the volume and velocity of floodwater, the duration of the event, the vulnerability of exposed elements (such as infrastructure and populations), and the effectiveness of existing flood mitigation measures (Huo et al., 2023; Yamaguchi et al., 2007).
Understanding and assessing flood hazards are crucial for effective disaster management, mitigation planning, and community resilience. Assessments of flood hazard aim to identify the potential consequences of flooding, including damage to buildings, infrastructure, and critical facilities and the potential loss of life and livelihoods (Huo et al., 2023; Pelling, 2003). Flood susceptibility assessments consider the exposure of elements at risk, considering factors such as population density, land use, building standards, and emergency preparedness. Such analysis helps identify high-risk areas and prioritize resources for flood risk reduction measures, early warning systems, and emergency response planning (Farish et al., 2017; Huo et al., 2023). Managing flood risk requires a multi-faceted approach that encompasses various strategies, including land-use planning, floodplain zoning, structural measures, non-structural measures, community awareness and preparedness initiatives (Huo et al., 2023; Meyer et al., 2012). Effective flood risk management involves a combination of preventive measures (reducing the likelihood of flooding through infrastructure and land-use planning), protective measures (safeguarding critical assets), and responsive measures (such as early warning systems and evacuation plans) (Kafle and Murshed, 2006). However, the ongoing climate change phenomenon further complicates flood risk management efforts, as it can influence precipitation patterns, sea levels, and extreme weather events (Jeffers, 2011; Shen et al., 2022; Zhao et al., 2023). The increasing frequency and intensity of rainfall events, coupled with rising sea levels, may intensify flood hazards and increase the susceptibility of coastal and low-lying areas (Huo et al., 2019; Ullah et al., 2023). Adapting to climate change and incorporating climate projections into flood risk assessments are crucial for ensuring the long-term effectiveness of flood management strategies (Hussain et al., 2023; Li et al., 2013).
Pakistan is exposed to natural disasters, including floods, which affect millions of people annually (Rana et al., 2020; Shen et al., 2022). Pakistan is located in a geographically sensitive region that is very prone to natural disasters such as earthquakes and floods. Within the past 10 years, natural disasters have impacted 124 out of 158 districts in the country, affecting over 33 million people. In 2010, the country faced a catastrophic flood caused by the yearly monsoon rains, submerging one-fifth of the country in water. Pakistan is one of the five South Asian countries with the highest population density that is exposed to floods due to its geographic location and climate change-induced heavy rainfall (Abbas et al., 2022; Khan et al., 2021). The lowland of the Indus River system was twisted as a flooded and flood-exposed area (Abbas et al., 2023; Tayyab et al., 2021). River floods occur during the summer and affect most low-lying areas due to their high exposure levels (Abbas et al., 2023; Rafiq and Blaschke, 2012; Rebi et al., 2023). The downstream region of the Indus River system has been officially acknowledged as a flood-prone area with a high susceptibility to flooding and associated hazards (Abbas et al., 2022; Tayyab et al., 2021). During the summer months, floods are common and affect most low-lying communities because of the high amounts of exposure they experience (Ahmad and Afzal, 2020; Rafiq and Blaschke, 2012). There is a high risk of flooding in the Khyber Pakhtunkhwa province of Pakistan, particularly in the Swat and Kabul Rivers (Qamer et al., 2023). Over the past 10 years, the province has been subjected to many devastating floods (Khan et al., 2022), the most notable of which occurred in the years 2007, 2008, 2010, 2012, 2014, 2018, and 2020, with the most recent disaster occurring in 2022 (Nanditha et al., 2023). The higher susceptibility to riverine floods in Khyber Pakhtunkhwa results from the presence of rivers and their tributaries that are making the region susceptible to hazards (Ahmad et al., 2022). Annually, district Charsadda experiences catastrophic floods due to its substantial proximity to the Swat and Kabul Rivers and their tributaries (Ahmad et al., 2022; Ali et al., 2022). According to the Report on Disaster Risk Management in Asia by Asian Disaster Preparedness Center, Bangkok, Thailand ADPC (2014), the 2010 mega flood was the most destructive flood due to its record-breaking inundation and extensive coverage, resulting in a significant loss of human lives and the displacement of millions of people. A mega flood is defined as an extreme flood event with a magnitude and impact far exceeding typical flood occurrences.
This study employed a multi-criteria decision analysis (MCDA) methodology combined with geographical information system (GIS) techniques to assess flood hazards in the Mizadhare Union Council (UC), a highly flood-prone area in Charsadda. The GIS platform, specifically ArcGIS, was used for spatial analysis, integrating key flood-related variables such as past flood history, average rainfall, stream discharge, and river stream density to create flood hazard maps. The MCDA process followed a step-by-step approach: first, the problem of flood hazard assessment was defined. Next, relevant criteria influencing flood risk were selected, and weights were assigned using the Analytic Hierarchy Process (AHP), based on expert judgment and community input. Data for each criterion were collected and normalized to ensure comparability. The flood risk of each area was then assessed and scored according to these weighted criteria. Finally, the scores were aggregated to generate a comprehensive flood hazard risk map, which categorized the region into high, medium, and low-risk zones. This approach allowed for a robust evaluation of flood hazards in the region, providing valuable insights for risk management and mitigation efforts (Ibrahim et al., 2024a; Khan et al., 2013). The assessment focused on the villages within Mirzadhare, aiming to understand the flood risk and analyze susceptibility levels in this area. By analyzing three key parameters, namely, average rainfall over the past 20 years, stream frequency, and stream density, a comprehensive flood hazard assessment was conducted to determine the risk levels of each village. Some important data regarding the peak discharge, past flood history, and flood risk based on average perception was also collected and analyzed. The significance of flood hazard assessment lies in its potential to inform decision-making processes related to disaster preparedness, response, and mitigation measures. Local authorities and stakeholders can prioritize resource allocation and develop appropriate strategies to minimize the impact of potential flooding events by identifying the villages that are most prone to flood hazards and those at high, medium, and low risk (Ibrahim et al., 2024b; Nasiri and Shahmohammadi-Kalalagh, 2013; Ouma and Tateishi, 2014). The combination of AHP-GIS analysis enables the effective analysis and prioritization of various flood risk indicators. Moreover, the methodology extends beyond conventional post-flood analysis and proactively utilizes a multifaceted data collection approach, including statistical methods such as questionnaires and field interviews, to gather ground data and validate findings. By integrating diverse data sources, we ensure the reliability and accuracy of our assessments, thereby enhancing the scientific validity of our research. This comprehensive framework, validated through iterative refinement and calibration, offers crucial insights and tools for effective flood management and risk reduction.
Previous studies, such as Shahid et al. (2021), have analyzed flood risks using climate and hydrological data, focusing on land use and climate change impacts on runoff in the Gilgit watershed. However, these studies often lack the integration of local knowledge through community engagement and overlook critical variables like stream density and past flood history. Similarly, Bibi et al., 2018, Flood risk assessment of river Kabul and Swat catchment area, District Charsadda, emphasizes land use and runoff dynamics but skips multivariable analyses. This study addresses these gaps by incorporating flood-specific variables past flood history, stream discharge and frequency, and river stream density while integrating AHP with GIS tools and local community input for a comprehensive flood hazard assessment.
Based on the published research, key parameters such as average rainfall patterns over the past 2 decades, stream frequency, and stream density were analyzed. These parameters were weighted and integrated into a comprehensive analysis to generate a flood hazard map. This map will serve as a valuable tool for understanding the flood risk for different areas within Mirzadhare and will add to formulating effective mitigation and preparedness strategies. The subsequent sections of this report provide a detailed account of the methodology employed in the flood hazard assessment, present the results and findings, and discuss their implications for disaster management, urban planning, and infrastructure development in Mirzadhare, Charsadda, Pakistan. While the findings and recommendations of this assessment are context-specific to Mirzadhare, they can serve as a valuable reference for other flood-prone regions having the same topography and facing similar challenges.
Mirzadhare is situated in a flood-prone region due to its proximity to a nearby river stream (Figure 1). Mirzadhare encompasses a diverse landscape with agricultural fields, residential areas, and infrastructure. It is located between 34°14′21″N to 34°18′10″N latitudes and 71°38′22E to 71°42′15″E longitudes and is 316–360 m above sea level (Chitrali, 2010). The Mizadhare Union Council (UC) is located in the arid region of Charsadda District, Khyber Pakhtunkhwa (KP), Pakistan. The climate of this area is characterized by hot summers and mild winters, with annual precipitation being relatively low, typically ranging between 250–500 mm. The region experiences most of its rainfall during the monsoon season, from June to September, which is often concentrated in short, intense bursts, leading to flash floods (Nadir and Ahmed, 2022). A climatological analysis over the past 30 years has shown variations in rainfall patterns, with some years experiencing more extreme precipitation events. The hydrological characteristics of the region are influenced by the nearby Swat River and its tributaries, with river discharge showing significant seasonal variation, particularly during the monsoon period (Hamidi et al., 2022). The topography of the area is dominated by low-lying floodplains and gently sloping land, which exacerbate flood risks due to limited natural drainage capacity. These combined climatic, hydrological, and topographical features contribute to the area’s vulnerability to recurrent flooding, particularly in flood-prone zones near the riverbanks (Chitrali, 2010).
Figure 1. Location map of the study area; (A) shows the map of Pakistan, (B) shows the district Charsadda map, and (C) shows the study area Mirzadhare.
The region experiences a typical cold winter and hot summer. The winter starts in mid-November and lasts till March, whereas summer starts in mid-May and lasts till September. The average maximum temperature in summer is 44°C, while the minimum temperature is 25°C. The minimum temperature usually recorded during winter is 4°C, and the maximum is 18°C (GoP, 2021). Based on the Provincial Irrigation Department report, the maximum average annual rainfall in the area is 278 mm, recorded in 2022, (Nadir and Ahmed, 2022). According to the District Census report, Mirzadhare’s population was 27,058 in 2021 (Hamidi et al., 2022). Based on its flood history and background, Mirzadhare needs to be the focal point for the flood hazard assessment (Bibi et al., 2018). The assessment aimed to evaluate the flood hazards in the villages within Mirzadhare and provide insights into the susceptibility level of each village. Its unique topography, hydrological features, and land use patterns characterise the region. Understanding the dynamics of these factors is essential for assessing flood vulnerability accurately and formulating appropriate disaster management strategies (Bibi et al., 2018). The area comprises 17 villages, each with distinct characteristics and exposure to flood hazards. These villages are inhabited by local communities who depend on the surrounding arable land for their livelihoods (Fida et al., 2020). It is crucial to assess these villages’ flood susceptibility to safeguard the residents’ lives and properties and promote sustainable development in the area (Rehman et al., 2016).
The study adopted an integrated approach to assess the flood hazard and construct a flood hazard distribution map for UC Mirzadhare, district Charsadda, Khyber Pakhtunkhwa, Pakistan. The flood susceptibility map was developed through ArcGIS by integrating remote sensing and field data on flood-causing factors. The overall methodology consists of five main steps. These include, 2) data collection and analysis, 3) flood hazard indicator inventory maps, 4) flood hazard indicators and causing factors, and 5) flood susceptibility mapping.
The flood hazard assessment conducted at Mirzadhare utilized a variety of datasets to ensure an inclusive analysis of flood hazards. Hazard indicators were selected based on the authentication of previous studies, as shown in Table 1. Under the framework shown in Figure 2, the selected indicators were executed and analyzed. The framework explains the roadmap of how the indicators were selected based on the published research. The indicators were also weighted as per their importance given by the experts of the Provincial Disaster Management Authority Khyber Pakhtunkhwa (PDMA-KP), Pakistan. The weighted results were normalized and then applied to the ArcGIS platform to develop a flood risk map overlay. The following data sources were employed as discussed:
Thieken et al. (2023) explain flood risk as the probability of experiencing an unusually high water level, leading to increased vulnerability. Flood risk is further defined by the Office of the Public Works and Local Government Dublin (UNESCO, 2009) as the multiplication of a flood-prone area’s hazard level, vulnerable circumstances, and exposure conditions to that hazard. This research integrates the data about hazard assessment as part of overall flood risk assessment by analysing the selected indicators, i.e., rainfall data, and peak discharge river stream density. For this study, the AHP model was employed to determine the relative importance of each flood hazard component, based on expert judgment and local community input. The process involved creating a hierarchy structure, where flood risk criteria (e.g., past flood history, rainfall, stream discharge, etc.) were evaluated through pairwise comparisons. These comparisons were carried out using a scale from one to 9, where one represents equal importance and nine represents extreme importance of one criterion over another. The resulting pairwise comparison matrix was then used to calculate the normalized weights for each criterion. The weights were determined based on their perceived significance in contributing to flood risk, with higher weights assigned to more influential variables such as past flood history and stream discharge. These weights were then applied to each criterion in the hazard risk equation, allowing for a quantitative assessment of flood hazard likelihood in the study area. The AHP model is designed to combine the components’ weight to assess mainly the hazard part of the above equation for this specific study. Each component involves a couple of criteria, and each contains multiple sub-criteria. The criteria of hazard risk components are adopted from the study outline. A hierarchy structure is used to evaluate flood risk criteria, ranked based on judgments of decision makers through AHP pairwise comparisons (Kobayashi and Porter, 2012). The selection of the above variables shown in Table 1 is based on their situational importance in the selected part. As cited above, previous researchers also selected these variables to assess flood hazard risk. Each variable has its specific weightage for assessing hazard likelihood.
To incorporate the rainfall pattern into the flood hazard assessment, historical precipitation data were obtained from the official website of NASA (https://giovanni.gsfc.nasa.gov/giovanni/accessed on 15 March 2023) data portal. This data source provided comprehensive and reliable information on rainfall patterns over the past 20 years. By incorporating this dataset into the analysis, we gained insights into the average rainfall distribution within the study area. Understanding rainfall’s spatial variability helped identify areas with higher rainfall intensity and assess their correlation with flood hazards. The integration of rainfall data enhanced the accuracy and reliability of the flood hazard assessment. The average rainfall map development involved obtaining the rainfall data from the official website of NASA for the past 20 years saved in a suitable format and imported into ArcMap (GIS) software. The data were converted into a raster format to facilitate spatial analysis and manipulation. The coordinates of the study area were converted to the appropriate Universal Transverse Mercator (UTM) coordinate system to ensure accurate spatial analysis. Using the extract by mask function in ArcMap, the rainfall data was clipped to the boundary of the study area. This step ensured that only the rainfall values within the region were considered in the subsequent analysis, providing a focused assessment specific to the study area. The Inverse Distance Weighting (IDW) interpolation method was employed to create the average rainfall map. The IDW method assigns weights to nearby rainfall measurements to estimate values at unsampled locations within the study area. This interpolation process generated a continuous surface representing the annual average rainfall distribution across the area, shown in Figure 3A.
Figure 3. (A) Shows average rainfall map of the study area in millimetres (mm), (B) Shows river stream frequency map in
The Arc-Hydro tool was used to generate stream orders based on the river network data obtained from the earth data source to calculate the stream frequency in cubic meters per second. Stream orders represent the hierarchy of streams within the study area, with higher orders indicating larger streams. After obtaining the stream orders, the results were converted into a feature dataset using the Arc Toolbox in ArcMap. This conversion allowed for easier data manipulation and analysis, as analyzed for the GIS-based flood hazard assessment and mapping in the case study of the Panjkora river basin, eastern Hindukush region of Pakistan by Ullah and Zhang (2020). The grid code column was examined, and similar numbers indicating stream orders were merged. The count of merged stream orders was calculated to determine how often each stream order occurred. A new field named “frequency” was added to the attribute table to record the sum of stream orders for each merged value. The Line Density tool in ArcMap was utilized to calculate the stream frequency. The stream order frequency column was added to the population field, and the Line Density tool was applied. This process generated a continuous density surface indicating the frequency of streams within the study area. It was evaluated based on the results studied by Luu and Von Meding, 2018 and generated the same type of stream density map for the flood risk assessment of Quang Nam, Vietnam, by using a special multi-criteria analysis (Luu and Von Meding, 2018). The density values (number of streams per square meter) were reclassified into four scales: low, medium, high, and very high to highlight the stream frequency zones. The resulting stream frequency map indicated the areas with varying degrees of stream density and potential flood risk in the selected area shown below (Figure 3B). This map identifies the risk zones with different levels in the range of low which is less than 25,000
The river stream lines dataset is instrumental in understanding the hydrological network within the study area. By mapping the stream density that flows through Mirzadhare, we gained insights into watercourses’ spatial distribution and connectivity. This information was vital for identifying potential flood pathways and assessing the vulnerability of villages located close to these streams. Analyzing the river stream data allowed us to identify areas at higher risk and prioritize resources accordingly. Village points were utilized to locate and analyze the individual villages within Mirzadhare. These points provided precise geographic coordinates for each village, facilitating a detailed assessment of flood hazards at the village level. By considering the proximity of villages to river streams, we could better understand which villages were more prone to flood hazards. This information was crucial for resource allocation, mitigation planning, and implementing targeted measures to reduce vulnerability and enhance resilience.
The initial calculation determined the direction of water flow within the study area, indicating how streams connect and flow into one another. The same method was used for flood risk analysis using a GIS-based AHP: a case study of Bitlis Province of Turkey by Aydin and Sevgi Birincioğlu (2022). Once the flow direction was obtained, the flow accumulation was calculated which represents the total upstream flow contributing to each cell within the river network. The same methodology was applied during the GIS-based flood hazard mapping study using the relative frequency ratio method: A case study of the Panjkora River Basin, eastern Hindu Kush region, Pakistan by Ullah and Zhang (2020). The Arc-Hydro tool was used to calculate the flow accumulation values, which indicate the potential stream density in different areas. The stream order was calculated based on the flow accumulation values in Cusec (cfs) to determine the stream discharge. Stream order represents the hierarchical organization of streams based on their size and position within the river network. Higher stream order values correspond to denser stream networks. The stream order values obtained were used to generate a stream discharge map. In this map, areas with higher stream order values indicated denser stream networks, while areas with lower stream order values represented regions with lower stream density.
The stream discharge map provided a visual representation of the varying levels of stream density across the study area. By following these steps, the stream density parameter was calculated using the Arc-Hydro tool. The flow direction, flow accumulation, and stream order allowed for the identification of areas with higher stream density, indicating potential flood-prone areas from low to high shown (Figure 3C). High stream density induces a quicker and more direct reaction to precipitation, it can affect stream discharge. In the event of severe and prolonged rainfall, high stream density can lead to greater stream discharge and a higher risk of flooding when accompanied by other variables including limited storage capacity, decreased infiltration, and human alterations to the terrain. In conclusion, excessive stream discharge is a major element raising the risk of flooding during periods of heavy rain. Increased precipitation, less infiltration, and rivers’ quick reaction to flow variations can all combine to cause stream discharge to rise quickly, exceeding both natural and man-made defences and raising the probability of flooding in nearby areas.
The flood hazard assessment in Mirzadhare employed GIS and MCDA techniques to analyze and evaluate flood hazards within the study area. The following are the details of these techniques.
MCDA assessed flood hazards by integrating and weighing multiple parameters. The three key parameters used in this assessment, namely, past flood history, average rainfall over the past 20 years, stream frequency, and stream density, were assigned relative weights based on their importance. MCDA facilitated the combination of these parameters into a comprehensive flood hazard index, allowing for the classification of villages into different risk categories (de Brito and Evers, 2016). Among the MCDA approaches, we used the family, the analytic hierarchy process (AHP), which was initially presented by Thomas L. Saaty as a beneficial tool for prioritizing and deciding (Saaty, 1990). This highly modifiable technique has been applied in multiple domains, such as economics, transport, planning, resource distribution, and, more recently, flood risk management for decision-making (de Brito and Evers, 2016). One of the advantages of the AHP is that it allows decision-makers or experts to contribute their thoughts, incorporate GIS, arrange criteria and sub-criteria, and secure uniformity in judgment (Ishizaka and Labib, 2009). The AHP pairwise comparison is demonstrated in three steps as; the first step is the division of the goal into related sectors, the second step is the comparing of conditions through two-way assessments to create a matrix satisfying the below Equation 1;
Where A is the weighting indicator and
The bigger the value of the risk factor index
The initial step in the project involved acquiring the necessary data for the flood hazard assessment. This included obtaining the shapefile of Khyber Pakhtunkhwa (KPK) and Charsadda district to establish the study area’s geographic boundaries. Additionally, the main shapefile of Mirzadhare was obtained to define the specific area of focus within the study region. River streamlines and village points were also collected to assess the hydrological network and village locations within Mirzadhare. Furthermore, rainfall data for the past 20 years was obtained from the official website of NASA (https://search.earthdata.nasa.gov/accessed on 15 March 2023). The obtained historical rainfall data was processed and analyzed to create the rainfall parameter. Statistical techniques, such as questionairs, interviews with relevant departments, field surveys and FGD,s with locals, were employed to calculate the average rainfall and its flooding situations over the past 20 years. The stream frequency parameter was derived by analyzing the river streamlines data. GIS tools were used to calculate the frequency of streams within the study area. The streamlines were analyzed, and the number of streams intersecting each cell or segment was determined. This analysis indicated the density of the river network. It helped identify areas with a higher concentration of streams, which could potentially contribute to increased flood hazards. The stream density parameter was derived by evaluating the river streamline data. GIS techniques were used for developing the map of the stream density within the study area. The length of streams within each cell or segment was measured, and the density was determined by dividing the total length of streams by the corresponding area. This analysis provided insights into the distribution of streams across the study area, helping identify areas with higher stream density, which could indicate an increased likelihood of flooding. By completing these steps, the three main parameters, namely, rainfall, stream frequency, and stream density, were derived to create a comprehensive flood hazard map. Some of the statistical data were collected through interviews and questionnaires from relevant stakeholders for constructing Tables and Figures.
The flood hazard assessment in Mirzadhare utilized a combination of four key parameters: flood historical profile, average rainfall, stream frequency, and stream density, which the last three were integrated through a weighted overlay analysis. The assignment of weights to each parameter was based on expert opinion, with average rainfall carrying a weight of 40%, stream frequency at 30%, and stream density at 30%, resulting in a total weight of 100%. After weighting, the results and final normalized scores as 0.4, 0.3, and 0.3 are (Table 2) below under the defined score for each rank of indicator (Table 3) and their threshold range as well. The weighted overlay analysis processed the integrated parameters to generate a comprehensive flood hazard map, visually representing the relative susceptibility of the villages within the selected area. The flood hazard map (Figure 4) revealed the presence of four distinct hazard zones: extremely or very high, high, medium, and low under the threshold for the hazard ranking (Table 3). All these resulting values are coming in the range of greater values than 0.2, which shows a high risk of flooding the selected area. The results present a new method for the local administration and community to highlight the high-risk areas for flood mitigation as the same method analyzed by Chinh Luu and Jason Von Meding for the hazard assessment in Quang Nam, Vietnam (Luu and Von Meding, 2018). The hazard map of the Mirzadhare also highlights the areas having the flood plain with high rank values greater than 0.2 as per the weighted values which attracts the special attention and the implementation of targeted risk mitigation measures to minimize potential damage and ensure the safety of the inhabitants. Furthermore, seven villages (Ghulam Qadir Killy, Taulandi, Asugai, Dang Qila, Nama, Duba, Totakai, and Ghazo) were identified as falling within the high-hazard zone, as presented in below Figure 4. The comparison allows for adjustments based on new data, changes in climatic conditions, or modifications in the landscape, enabling effective and up-to-date flood risk management.
Figure 4, was obtained to clarify the process for determining the areas during the MCDA procedures. The areas shown in the figure were identified through a combination of spatial analysis using GIS and overlaying flood hazard criteria. Each area was evaluated based on the weighted criteria derived through AHP, and flood hazard risk was assessed by comparing the various variables against pre-determined thresholds. The MCDA process allowed us to rank these areas according to their relative flood risk, and Figure 4 illustrates the resulting hazard classification.
The nature of the flood risk depends on the rate of precipitation, river water intensity, and river capacity. A survey was conducted in the study area, where it was found that previous flood marks on the walls of the exposed settlements and trees were almost from four to eight feet in height. These marks showed a high level of flood in Mirzadhare which indicates the high level of likelihood in the future flooding. Somewhere in those villages of the area, which are more distant, show less than four feet of water marks on trees and walls, showing medium levels of flood in the past. Those villages at a distance of more than 2 km from the river, less than 2 feet height of past flood water marks on the exposed settlements were found as shown in (Figure 5) which shows the low risk of flooding in the future. However, some of the land degradation and damages were observed in agricultural land, showing realistic signs of flood there. Some of the villages of the Mirzadhare, which are kilometres away from the river, had a low level of flood experience in the past.
Figure 5. Past flood marks on the exposed settlements of Mirzadhare (Source; field survey, July 2022).
Questionnaire data was collected from the relevant authority for more exposed villages to riverine floods in the selected area. In response shown in Table 3, it was found that the maximum number of respondents, about 60% and 65%, scored seven to nine respectively, that in the past, they experienced high levels of flood in the nearby villages. Some respondents, about 30%, scored five that there had been a medium flood in every monsoon season. About 20% of responses received to score of three for the community living a distance of more than 3 km away from the river, had experienced a low level of flooding in their area. Due to this inundation situation, they agreed on a high-level flood risk. This assessment also reveals that the risk of flooding in the whole Mirzadhare area is positive and acceptable for the people living there in the range from low to high based on the characteristics of area exposure.
Annual temperature changes and rise have profound effects on various aspects of the environment and climate, including the frequency and intensity of flooding. As global temperatures rise, the atmosphere’s capacity to hold moisture increases (Abbas et al., 2023). For every 1°C rise in temperature, the atmosphere can hold approximately 7% more moisture. This enhanced moisture capacity leads to more intense and prolonged precipitation events especially in Monsoon period, which can overwhelm natural and man-made drainage systems, resulting in increased flooding (Ahmad and Afzal, 2020). Rising temperatures are causing glaciers and ice caps to melt at an accelerated rate. This melting contributes to higher sea levels, which can exacerbate riverine flooding (Abbas et al., 2023; Ahmad et al., 2015). The flood occurs mainly in the monsoon season (Figure 6) based on the data collected from the irrigation department, which starts in the middle of May and ends in the middle of September. In these months due to daily rainfall in the upper region, the amount of water exceeds the normal level, coming out of the banks of rivers and causing floods (Ali et al., 2022). To find out the time of the flood in the study area, questionnaire data was collected randomly inside the community which is further verified by the data provided by the irrigation department, (Figure 6), for the last 22 years’ maximum monthly rainfall. Most respondents about 50% say that, in this area, floods mostly occur in the monsoon period from July to September. Also, 20% of the respondents say that sometimes the chances of flooding in the area also exceed in May due to high precipitation.
The data collected from the Provincial Irrigation Department shows annual discharge of the river Swat increases in Charsadda, especially at Munda Headwork close to the Mirzadhare. Also, an interview was conducted about the below shown (Figure 7), in which the discharge rate of water at Munda Headwork is high in months of monsoon season (June, July, August, and September). So, in these months the likelihood of flood is higher as compared to other months of the year. When the discharge rate becomes high, the water also brings some sedimentation and load from the upper areas, which gives more chances to stop water and inundate the areas near the bank of the river in Mirzadhare.
Figure 7. Last 22 years monthly average peak discharge in Monsoon at Munda Headwork (Source; Irrigation dpt).
Seasonal trends analyzed historical data to identify seasonal patterns of flood incidence. Highlighted periods with high risk, such as monsoon seasons prone to heavy rainfall. Inspected long-term trends in flood risk over the years and identified any increasing or decreasing trends in flood risk based on historical rainfall data. In conclusion, the study reveals an understanding of the temporal and spatial distribution of flood risk in Mirzadhare as shown in Table 4. The results outcome provide a foundation to inform decision-making in land use planning, infrastructure development, and emergency management to mitigate the impact of floods in the study region, especially during high and prolonged rainy seasons. The study showed that heavy rainfall which mostly occurs in the Monsoon season is the main driving factor of flooding in the region and the other indicators are the triggering base for the severity of the hazard. During the interview, some of the important spatial points were discussed. The local people said that most of the flooding situations in the Swat River occur in the monsoon season, which starts in May and ends in August but when there is high rainfall, the water level in the river rises causing floods (Table 4). They also said that they had experienced flooding in the river in May, June, July, and August. One of the reasons also is the melting snow in those areas where the river starts. Some of the respondents claimed that change in weather is also a contributory cause and that change mostly occurs in these monsoon months. A low-level flooding occurs whenever there is a high precipitation in any other month, even in March or April.
This research study analyses the flood hazard by probing different indicators to find the weights for the main variables, such as average rainfall data, river stream density, river stream discharge rate, and the last 20 years of flood history. Furthermore, some interviews and questionnaire data were collected to investigate the temporal dynamics of flooding in the selected area. The weights assigned to the parameters were based on expert opinion, which introduces a degree of subjectivity (Luu and Von Meding, 2018; Smith, 2013). Alternative weightings of additional parameters that were not considered in this study could potentially yield slightly different results. The results of this research study show a positive correlation between the past flooding history and the risk of future flooding, which is consistent with the findings of Ullah and Zhang (2020) and Sarkar and Mondal (2020). By analyzing the average rainfall and village points indicator, the results revealed that the maximum area near the river and its tributaries show high susceptibility to flood hazards. It is worth mentioning that the stated exposed area has flat topography that further exacerbates its susceptibility to flood hazards. Among the 17 villages assessed within the study area, five villages (i.e., Sarasang, Mian Sahib Killy, Garhi Dildar, Dagai, and Mirzadhare) were classified under the very high hazard zone. These villages demonstrated a high likelihood of flood hazards and posed a significant risk in the event of a flood occurrence. For instance, Ibrahim et al. (2024a) and Ibrahim et al. (2024b) calculated the exposure levels towards flooding in similar topographical regions which shows a high level of susceptibility. Furthermore, Wang et al. (2022) for the assessment of urban flooding in Wuhan China and Vignesh et al. (2021) for flood risk assessment utilised the same integrated approach of multi-criteria decision-making model and geospatial techniques. Based on the consideration of the village points, it also shows a direct link with flood hazard assurance as studied by Ahmad and Afzal (2020), Alexander et al. (2011), and Ullah and Zhang (2020) for assessing flood hazard.
Overall the area shows a high risk of flooding, while their susceptibility may be slightly lower for those villages, which are more than 3 km away or at higher altitudes compared to the very high-hazard zone, as shown in Figure 6. These villages still face a considerable level of hazard risk and demand appropriate measures to mitigate flood-related damages effectively. Four villages (i.e., Duaba Dang Qila, Totakai, Ghazo and Qadar Kaly) were categorized under the medium hazard zone, indicating a moderate risk of flood hazards. Some of the villages were determined to be within the low-hazard zone, suggesting a relatively lower susceptibility to flood hazards. However, it is crucial to note that even though these villages fall under the low-hazard zone, it is still susceptible to any high possible flooding in the future. Therefore, precautionary measures should be in place to safeguard the community during flood events to strengthen the DRR strategy as suggested by different studies conducted by Ibrahim et al. (2024a); Luu and Von Meding, 2018), Shahid et al. (2021), and Valjarević (2024) and Shen et al. (2022) for different regions around the globe. The hazard map shown in Figure 6, derived from the integrated analysis of average rainfall, stream frequency, and stream density, provides valuable insights into the flood risk across the study area. The results also show that the maximum precipitation is recorded in the monsoon season due to climate change and also backed by the peak discharge data that the likelihood of flooding is high in this season starting from the mid of May to mid-September. The precipitation shows a direct relation with flood hazard occurrence because when there is heavy rainfall in the upper region, it accumulates huge amounts of water into the river and raises the water level. The same correlation and trends were also studied by Rebi et al. (2023), Ibrahim et al. (2024b) and Hussain et al. (2023) in different studies and phenomena about extreme flooding events. Using the same analysis method which was applied by Ullah and Zhang (2020) and also generated maps showing direct relation for assessing the flood risk at the Panjkora River Basin of the Hindukush region, Pakistan.
The stream frequency and flood hazard have a direct relation that arises from several factors; areas with higher stream frequency tend to have a higher drainage density, meaning there are more channels available to carry water away from the landscape. During periods of heavy rainfall or snowmelt, this dense network of streams quickly collects and conveys water downstream, potentially leading to higher flood risk. Streams with higher frequencies often have smaller channel capacities, especially in regions with narrow river channels as analyses by Ullah et al. (2023). When faced with a sudden influx of water from heavy precipitation, these smaller channels may reach their capacity more rapidly, causing water to overflow onto adjacent floodplains. In areas with a high stream frequency, runoff from precipitation events can quickly accumulate and flow downstream at faster velocities as comparatively examined by Rebi et al. (2023) in the Spatiotemporal precipitation trends and associated large-scale teleconnections in Northern Pakistan. This rapid runoff can increase the likelihood of flooding, particularly in areas with impermeable surfaces or limited natural vegetation to absorb and slow the flow of water as studied by Ali et al. (2023). The relationship between the frequency of streams and the risk of floods is directly influenced by factors, such as the density of drainage, the capacity of the channels, the velocity of runoff, and the topography effects. Areas with higher stream frequencies are generally more susceptible to flooding because they are better connected by a dense network of channels, which can quickly collect, convey, and potentially overwhelm water during extreme weather events. The same relation was studied by Rahman et al. (2023) and Rahman et al. (2019) for assessing flood hazards with their impacts in the Hindulush region of Pakistan.
The flood hazard assessment relies on historical data, such as average rainfall patterns over the past 22 years with the average peak discharge at the Munda Headwork. While historical data provides valuable insights into flood patterns, it may not fully capture the potential impact of future climate change but also ensure the probability of future flooding. Based on the scale of the impacts, the area has experienced many catastrophic floods, like 2004, 2006, 2008, 2009, 2010, 2012, and 2014 flood, which brought much disruption to the living community and their settlements (Farish et al. (2017); Nanditha et al. (2023) recently studied that the northern areas of the Khyber Pakhtunkhwa province of Pakistan have seen a series of catastrophic floods in the last decade, most notably in 2010, which was declared a super flood in 2014, 2018, and 2020, and the most recent catastrophes in 2022. The increased susceptibility to riverine floods in the areas is due to the existence of rivers and their tributaries that are prone to hazards as analysed by Ahmad et al. (2022); Rahman et al. (2022). Climate change and variability can alter precipitation patterns, intensify storms, and lead to more frequent or severe flooding in the downstream area of the Khyber Pakhtunkhwa province, including all other floodplains (Abbas et al., 2022). To effectively address this issue, an inclusive flood risk assessment is conducted to analyze and evaluate the flood hazards in the study area. Based on the historical profile of average precipitation, there is a direct relation between rainfall and flooding, indicating that precipitation is the driving factor of flood hazard in the region. Therefore, it is important to supplement the historical data with projections and scenarios that incorporate potential future climate conditions as analysed by Ahmad and Afzal (2020); Ahmad et al. (2015); Abbas et al. (2022); and Abbas et al. (2023).
Furthermore, the evaluation of flood hazards primarily focused on the immediate threat posed by river streams. It is crucial to acknowledge that other sources of flooding, such as urban drainage systems and flash floods may also contribute to overall flood risk in the study area as indicated by Ali et al. (2023). These additional sources of flooding should be evaluated and incorporated into future assessments to provide a more comprehensive understanding of flood hazards. Furthermore, the flood hazard assessment focused on the physical vulnerability of villages but did not explicitly assess the exposure and susceptibility of critical infrastructure, economic assets, and ecological systems. While the flood hazard map generated through the Weighted Overlay analysis, provides a useful visual representation of flood hazard likelihood, it should not be the sole basis for decision-making. Local knowledge, community engagement, and stakeholder input are effective in ensuring flood risk management strategies’ relevance, effectiveness, and acceptance. It is essential to acknowledge that flood hazard assessment based on the available data, expert opinion and assigned weight the efforts were made to ensure data accuracy, limitations may exist as compared by Uddin et al. (2019) for the mapping of climate vulnerability of the coastal region of Bangladesh using principal component analysis. The accuracy and applicability of future flood hazard assessments can be enhanced by recognizing the limitations and addressing them through ongoing data collection, analysis, and stakeholder engagement. Moreover, the quality and completeness of the data sources used, including past flood history, rainfall data, stream network data, and stream density, may have influenced the precision of the results. The results of this study can be used to create customized flood risk contingency plans for the neighbourhood areas and for other districts having the same topography. Decision makers can use the map of flood risk and exposure for future flood mitigation and preparedness plans. The accuracy and applicability of future flood hazard assessments can be enhanced by recognising the limitations and addressing them through ongoing data collection, analysis, and stakeholder engagement. The outcomes might also be included in a map to assist in regarding land use planning and zonation. Despite the thorough methodology and comprehensive analysis in our flood hazard assessment, it is crucial to recognize inherent limitations. Despite efforts to obtain reliable and current data, inconsistencies and gaps exist within rainfall, stream networks, and village locations. To ensure the effectiveness of flood hazard assessments, it is recommended the establish a comprehensive monitoring system that integrates remote sensing technologies, GIS tools, and real-time data collection from hydrological stations to track changes in rainfall, stream discharge, and flood frequency. A structured data collection framework, incorporating regular field surveys, community feedback, and satellite imagery analysis, will help keep flood hazard maps up-to-date. Periodic reassessments, conducted annually or biennially, using the latest hydrological, meteorological, and land use data are essential to reflect changes in flood risks due to climate variability or infrastructural developments. Active community involvement through awareness programs and capacity-building initiatives will enhance data accuracy and preparedness, while collaboration with government agencies will ensure the integration of updated maps into land use planning, disaster management policies, and risk mitigation strategies. Additionally, adopting advanced technological tools such as IoT-based sensors and UAVs (drones) can enable continuous monitoring of vulnerable zones and river systems, ensuring a proactive approach to flood management.
The present study has effectively identified villages at varying risk levels (i.e., low to very high-risk levels) by analysing multiple indicators, including the flood historical profile, average rainfall patterns, stream frequency, and stream density. The results reveal that more than 65% of the area is highly susceptible to flood hazards, with over 50% of the total population and 55% of the agriculture setup located in flood hazard zones. In the context of flood management, the focus should be on tackling heavy precipitation and unplanned human activities, which are the driving factors of flooding in the Swat River Basin, including the study area. To mitigate flood hazard risks and enhance community resilience, it is recommended to implement modern meteorological monitoring and early warning systems, flood hazard zonation, land-use planning, develop adaptive plans with flood-resilient infrastructure, and integrate climate change projection and flood hazard information into future disaster preparedness plans. While the findings are robust, there are some limitations to the study. The reliance on historical flood data may not fully capture the recent shifts in flood dynamics due to climate change and urbanization. Additionally, the study could benefit from more detailed, high-resolution meteorological data and real-time flood monitoring to better predict future flood events. Moreover, the spatial extent of the analysis, although comprehensive, is limited by available data on land use and floodplain management, which could be improved in future studies.
The raw data supporting the conclusions of this article will be made available by the authors, without undue reservation.
MI: Conceptualization, Data curation, Formal Analysis, Investigation, Methodology, Resources, Software, Visualization, Writing–original draft, Writing–review and editing. AH: Conceptualization, Investigation, Methodology, Project administration, Software, Supervision, Validation, Writing–review and editing. WU: Funding acquisition, Investigation, Supervision, Validation, Writing–review and editing. SU: Data curation, Investigation, Methodology, Project administration, Resources, Supervision, Validation, Writing–review and editing. ZX: Data curation, Formal Analysis, Validation, Writing–review and editing.
The author(s) declare that financial support was received for the research, authorship, and/or publication of this article. This research was funded by the National Natural Science Foundation of China (Grant Nos 42261144749 and 42377158), National Foreign Expert Individual Human Project (Category H) (H20240400); Shaanxi Province Agricultural science and technology 114 public welfare platform to serve rural revitalization practical technical training (Grant No. 2024NC-XCZX-06) and the International Science and Technology Cooperation Program of Shaanxi Province (Grant No. 2024GH-ZDXM-24).
The authors acknowledge the Irrigation Department, Khyber Pakhtunkhwa, and the Provincial Disaster Management Authority (PDMA), Khyber Pakhtunkhwa, Pakistan for providing historical data on peak discharge with stream discharge and flood damages, respectively. The authors are thankful to the Environmental Systems Research Institute (ESRI) for land use land/cover data and the National Aeronautics and Space Administration (NASA) for providing the precipitation data. The authors are grateful to the reviewers for their insightful remarks for enlightening the manuscript. The authors acknowledge Rabdan Academy, United Arab Emirates (UAE) for supporting the Article Processing Charges (APC) of the research manuscript.
The authors declare that the research was conducted without any commercial or financial relationships that could be interpreted as a potential conflict of interest.
All claims expressed in this article are solely those of the authors and do not necessarily represent those of their affiliated organizations, or those of the publisher, the editors and the reviewers. Any product that may be evaluated in this article, or claim that may be made by its manufacturer, is not guaranteed or endorsed by the publisher.
Abbas, A., Bhatti, A. S., Ullah, S., Ullah, W., Waseem, M., Zhao, C., et al. (2023). Projection of precipitation extremes over South Asia from CMIP6 GCMs. J. Arid Land 15 (3), 274–296. doi:10.1007/s40333-023-0050-3
Abbas, A., Ullah, S., Ullah, W., Waseem, M., Dou, X., Zhao, C., et al. (2022). Evaluation and projection of precipitation in Pakistan using the coupled model intercomparison project phase 6 model simulations. Int. J. Climatol. 42 (13), 6665–6684. doi:10.1002/joc.7602
Ahmad, D., and Afzal, M. (2020). Flood hazards and factors influencing household flood perception and mitigation strategies in Pakistan. Environ. Sci. Pollut. Res. 27 (13), 15375–15387. doi:10.1007/s11356-020-08057-z
Ahmad, I., Tang, D., Wang, T., Wang, M., and Wagan, B. (2015). Precipitation trends over time using Mann-Kendall and spearman’s rho tests in swat river basin, Pakistan. Adv. Meteorology 2015, 1–15. doi:10.1155/2015/431860
Ahmad, N., Khan, S., Ehsan, M., Rehman, F. U., and Al-Shuhail, A. (2022). Estimating the total volume of running water bodies using geographic information system (GIS): a case study of Peshawar Basin (Pakistan). Sustainability 14 (7), 3754. doi:10.3390/su14073754
Alexander, M., Viavattene, C., Faulkner, H., and Priest, S. (2011). “A GIS-based flood risk assessment tool: supporting flood incident management at the local scale,” in Flood hazard research centre. London: Middlesex University.
Ali, A., Ullah, W., Khan, U. A., Ullah, S., Ali, A., Jan, M. A., et al. (2023). Assessment of multi-components and sectoral vulnerability to urban floods in Peshawar–Pakistan. Nat. Hazards Res. 4 (3), 507–519. doi:10.1016/j.nhres.2023.12.012
Ali, A. M. S. (2007). September 2004 flood event in southwestern Bangladesh: a study of its nature, causes, and human perception and adjustments to a new hazard. Nat. Hazards 40 (1), 89–111. doi:10.1007/s11069-006-0006-x
Ali, W., Hashmi, M. Z., Jamil, A., Rasheed, S., Akbar, S., and Iqbal, H. (2022). Mid-century change analysis of temperature and precipitation maxima in the Swat River Basin, Pakistan. Front. Environ. Sci. 10, 973759. doi:10.3389/fenvs.2022.973759
Al Khoury, I., Boithias, L., and Labat, D. (2023). A review of the application of the soil and water assessment tool (SWAT) in karst watersheds. Water 15 (5), 954. doi:10.3390/w15050954
Aydin, M. C., and Sevgi Birincioğlu, E. (2022). Flood risk analysis using gis-based analytical hierarchy process: a case study of Bitlis Province. Appl. Water Sci. 12 (6), 122. doi:10.1007/s13201-022-01655-x
Babcicky, P., and Seebauer, S. (2021). People, not just places: expanding physical and social vulnerability indices by psychological indicators. J. Flood Risk Manag. 14 (4), e12752. doi:10.1111/jfr3.12752
Balica, S. F., Wright, N. G., and van der Meulen, F. (2012). A flood vulnerability index for coastal cities and its use in assessing climate change impacts. Nat. Hazards 64 (1), 73–105. doi:10.1007/s11069-012-0234-1
Bibi, T., Nawaz, F., Abdul Rahman, A., Azahari Razak, K., and Latif, A. (2018). Flood risk assessment of river Kabul and Swat catchment area: district Charsadda, Pakistan. Int. Archives Photogrammetry, Remote Sens. Spatial Inf. Sci. 42, 105–113. doi:10.5194/isprs-archives-xlii-4-w9-105-2018
Chen, K., Blong, R. J., and Jacobson, C. (2001). MCE-RISK: integrating multicriteria evaluation and GIS for risk decision-making in natural hazards. Environ. Model. Softw. 16, 387–397. doi:10.1016/s1364-8152(01)00006-8
Chitrali, S. Z. (2010). Rapid assessment of flood effected areas in Charsadda. Islamabad, Pakistan: NRSP-KPK. Available at: https://nrsp.org.pk/publications/Annual-Reports/NRSP-Annual-Report-2010-11.pdf.
de Brito, M. M., and Evers, M. (2016). Multi-criteria decision-making for flood risk management: a survey of the current state of the art. Nat. Hazards Earth Syst. Sci. 16 (4), 1019–1033. doi:10.5194/nhess-16-1019-2016
Dewan, A. (2013). Floods in a megacity: geospatial techniques in assessing hazards, Risk and vulnerability (1 ed.). Springer Dordrecht. doi:10.1007/978-94-007-5875-9
Farish, S., Munawar, S., Siddiqua, A., Alam, N., and Alam, M. (2017). Flood risk zonation using gis techniques: district Charsadda, 2010 floods Pakistan. Environ. Risk Assess. Remediat. 1 (2). doi:10.4066/2529-8046.100014
Fida, M., Hussain, I., Tao, W., Rashid, A., and Ali Shah, S. A. (2020). Land use and land cover change analysis of District Charsadda, Pakistan along Kabul River in 2010 flood: using an advance geographic information system and remote sensing techniques. Nat. Hazards Earth Syst. Sci. Discuss., 1–16. doi:10.5194/nhess-2020-255
Fida, M., Li, P., Wang, Y., Alam, S. K., and Nsabimana, A. (2022). Water contamination and human health risks in Pakistan: a review. Expo. Health 15, 619–639. doi:10.1007/s12403-022-00512-1
Gupta, A. K., and Nair, S. (2010). Flood risk and context of land-uses: Chennai city case. J. Geogr. Regional Plan. 3, 365–372.
Hamidi, A. R., Jing, L., Shahab, M., Azam, K., Atiq Ur Rehman Tariq, M., and Ng, A. W. (2022). Flood exposure and social vulnerability analysis in rural areas of developing countries: an empirical study of Charsadda District, Pakistan. Water 14 (7), 1176. doi:10.3390/w14071176
Huo, A., Liu, Q., Zhao, Z., Elbeltagi, A., Abuarab, M. E.-S., and Ganjidoust, H. (2023). Habitat quality assessment and driving factor analysis of xiangyu in feng River Basin based on InVEST model. Water 15 (23), 4046. doi:10.3390/w15234046
Huo, A., Peng, J., Cheng, Y., Zheng, X., and Wen, Y. (2019). Temporal characteristics of the rainfall induced landslides in the Chinese loess plateau (China). Water 14 (22), 3674. doi:10.1007/978-3-030-01665-4_98
Huo, A.-D., Dang, J., Song, J.-X., Chen, X. H., and Mao, H.-R. (2016). Simulation modeling for water governance in basins based on surface water and groundwater. Agric. Water Manag. 174, 22–29. doi:10.1016/j.agwat.2016.02.027
Hussain, A., Hussain, I., Ali, S., Ullah, W., Khan, F., Rezaei, A., et al. (2023). Assessment of precipitation extremes and their association with NDVI, monsoon and oceanic indices over Pakistan. Atmos. Res. 292, 106873. doi:10.1016/j.atmosres.2023.106873
Ibrahim, M., Huo, A., Ahmed, A., Zhao, Z., and Zhong, F. (2024a). Comprehensive assessment of flood exposure in arid regions: integrating GIS techniques and multi-method approaches–A case study of downstream Swat River, Pakistan. Int. J. Disaster Risk Reduct. 109, 104515. doi:10.1016/j.ijdrr.2024.104515
Ibrahim, M., Huo, A., Ullah, W., Ullah, S., Ahmad, A., and Zhong, F. (2024b). Flood vulnerability assessment in the flood prone area of Khyber Pakhtunkhwa, Pakistan. Front. Environ. Sci. 12, 1303976. doi:10.3389/fenvs.2024.1303976
Ishizaka, A., and Labib, A. (2009). Analytic hierarchy process and expert choice: benefits and limitations. OR Insight 22 (4), 201–220. doi:10.1057/ori.2009.10
Jeffers, J. M. (2011). The Cork City flood of November 2009: lessons for flood risk management and climate change adaptation at the urban scale. Ir. Geogr. 44 (1), 61–80. doi:10.1080/00750778.2011.615283
Kafle, S. K., and Murshed, Z. (2006). Community-based disaster risk management for local authorities. Bangkok, Thailand: Asian Disaster Preparedness Center (ADPC), 19–27.
Khan, A. N., Khan, B., Qasim, S., and Khan, S. N. (2013). Causes, effects and remedies: a case study of rural flooding in district Charsadda, Pakistan. J. Manag. Sci. 7 (1).
Khan, I., Ali, A., Waqas, T., Ullah, S., Ullah, S., Shah, A. A., et al. (2022). Investing in disaster relief and recovery: a reactive approach of disaster management in Pakistan. Int. J. Disaster Risk Reduct. 75, 102975. doi:10.1016/j.ijdrr.2022.102975
Khan, I., Lei, H., Shah, A. A., Khan, I., and Muhammad, I. (2021). Climate change impact assessment, flood management, and mitigation strategies in Pakistan for sustainable future. Environ. Sci. Pollut. Res. 28, 29720–29731. doi:10.1007/s11356-021-12801-4
Kobayashi, Y., and Porter, J. W. (2012). Flood risk management in the people's Republic of China: learning to live with flood risk. Asian Development Bank. Available at: https://books.google.com/books?id=GxI_CQAAQBAJ.
Li, G.-F., Xiang, X.-Y., Tong, Y.-Y., and Wang, H.-M. (2013). Impact assessment of urbanization on flood risk in the Yangtze River Delta. Stoch. Environ. Res. Risk Assess. 27 (7), 1683–1693. doi:10.1007/s00477-013-0706-1
Luu, C., and Von Meding, J. (2018). A flood risk assessment of Quang Nam, Vietnam using spatial multicriteria decision analysis. Water 10 (4), 461. doi:10.3390/w10040461
Meyer, V., Priest, S., and Kuhlicke, C. (2012). Economic evaluation of structural and non-structural flood risk management measures: examples from the Mulde River. Nat. Hazards 62, 301–324. doi:10.1007/s11069-011-9997-z
Moreira, L. L., de Brito, M. M., and Kobiyama, M. (2021). Review article: a systematic review and future prospects of flood vulnerability indices. Nat. Hazards Earth Syst. Sci. 21 (5), 1513–1530. doi:10.5194/nhess-21-1513-2021
Nadir, H., and Ahmed, A. (2022). Hydrological analysis and statistical modelling of swat River Basin for flood risk assessment. Adv Earth and Env Sci 3 (4), 1–13. doi:10.47485/2766-2624.1028
Nanditha, J., Kushwaha, A. P., Singh, R., Malik, I., Solanki, H., Chuphal, D. S., et al. (2023). The Pakistan flood of August 2022: causes and implications. Earth's Future 11 (3), e2022EF003230. doi:10.1029/2022ef003230
Nasiri, H., and Shahmohammadi-Kalalagh, S. (2013). Flood vulnerability index as a knowledge base for flood risk assessment in urban area. J. Nov. Appl. Sci. 2 (8), 269–272.
Nasiri, H., Yusof, M. J. M., Ali, T. A. M., and Hussein, M. K. B. (2019). District flood vulnerability index: urban decision-making tool. Int. J. Environ. Sci. Technol. 16 (5), 2249–2258. doi:10.1007/s13762-018-1797-5
Ouma, Y. O., and Tateishi, R. (2014). Urban flood vulnerability and risk mapping using integrated multi-parametric AHP and GIS: methodological overview and case study assessment. Water 6 (6), 1515–1545. doi:10.3390/w6061515
Pelling, M. (2003). Natural disaster and development in a globalizing world. 1st Edn. Routledge, Tylor & Francis Group. Available at: https://www.routledge.com/Natural-Disaster-and-Development-in-a-Globalizing-World/Pelling/p/book/9780415279581?srsltid=AfmBOooeG_JD4SYlaNqLZh1OzhnaaJs7TWXtudytAk7HjQ8RBjkHDO5w.
Penning-Rowsell, E., Floyd, P., Ramsbottom, D., and Surendran, S. (2005). Estimating injury and loss of life in floods: a deterministic framework. Nat. Hazards 36 (1), 43–64. doi:10.1007/s11069-004-4538-7
Qamer, F. M., Abbas, S., Ahmad, B., Hussain, A., Salman, A., Muhammad, S., et al. (2023). A framework for multi-sensor satellite data to evaluate crop production losses: the case study of 2022 Pakistan floods. Sci. Rep. 13 (1), 4240. doi:10.1038/s41598-023-30347-y
Qin, Y., Xu, X., Xu, K., Li, A., and Yang, D. (2013). Fuzzy evaluation system of agriculture drought disaster risk and its application. Trans. Chin. Soc. Agric. Eng. 29 (10), 83–91.
Rafiq, L., and Blaschke, T. (2012). Disaster risk and vulnerability in Pakistan at a district level. Geomatics, Nat. Hazards Risk 3 (4), 324–341. doi:10.1080/19475705.2011.626083
Rahman, A.-u., Mahmood, S., Dawood, M., Rahman, G., and Chen, F. (2019). “Impact of climate change on flood factors and extent of damages in the Hindu Kush Region,” in Oxford research encyclopedia of natural hazard science.
Rahman, G., Rahman, A.-u., Anwar, M. M., Dawood, M., and Miandad, M. (2022). Spatio-temporal analysis of climatic variability, trend detection, and drought assessment in Khyber Pakhtunkhwa, Pakistan. Arabian J. Geosciences 15 (1), 81. doi:10.1007/s12517-021-09382-4
Rahman, Z. U., Ullah, W., Bai, S., Ullah, S., Jan, M. A., Khan, M., et al. (2023). GIS-based flood susceptibility mapping using bivariate statistical model in Swat River Basin, Eastern Hindukush region, Pakistan. Front. Environ. Sci. 11. doi:10.3389/fenvs.2023.1178540
Rana, I. A., Jamshed, A., Younas, Z. I., and Bhatti, S. S. (2020). Characterizing flood risk perception in urban communities of Pakistan. Int. J. Disaster Risk Reduct. 46, 101624. doi:10.1016/j.ijdrr.2020.101624
Rebi, A., Hussain, A., Hussain, I., Cao, J., Ullah, W., Abbas, H., et al. (2023). Spatiotemporal precipitation trends and associated large-scale teleconnections in Northern Pakistan. Atmosphere 14 (5), 871. doi:10.3390/atmos14050871
Rehman, A., Jingdong, L., Du, Y., Khatoon, R., Wagan, S. A., and Nisar, S. K. (2016). Flood disaster in Pakistan and its impact on agriculture growth (a review). Environ. Dev. Econ. 6 (23), 39–42.
Rehman, A., Song, J., Haq, F., Mahmood, S., Ahamad, M. I., Basharat, M., et al. (2022). Multi-hazard susceptibility assessment using the analytical hierarchy process and frequency ratio techniques in the Northwest Himalayas, Pakistan. Remote Sens. 14 (3), 554. doi:10.3390/rs14030554
Saaty, T. L. (1990). How to make a decision: the analytic hierarchy process. Eur. J. operational Res. 48 (1), 9–26. doi:10.1016/0377-2217(90)90057-i
Saaty, T. L., and Vargas, L. G. (2006). The Analytic Hierarchy Process: wash criteria should not be ignored. Int. J. Manag. Decis. Mak. 7 (2-3), 180–188. doi:10.1504/ijmdm.2006.009142
Sarkar, D., and Mondal, P. (2020). Flood vulnerability mapping using frequency ratio (FR) model: a case study on Kulik river basin, Indo-Bangladesh Barind region. Appl. Water Sci. 10 (1), 17–13. doi:10.1007/s13201-019-1102-x
Scheuer, S., Haase, D., and Meyer, V. (2011). Exploring multicriteria flood vulnerability by integrating economic, social and ecological dimensions of flood risk and coping capacity: from a starting point view towards an end point view of vulnerability. Nat. Hazards 58 (2), 731–751. doi:10.1007/s11069-010-9666-7
Shen, L., Wen, J., Zhang, Y., Ullah, S., Cheng, J., and Meng, X. (2022). Changes in population exposure to extreme precipitation in the Yangtze River Delta, China. Clim. Serv. 27, 100317. doi:10.1016/j.cliser.2022.100317
Smith, K. (2013). Environmental hazards: assessing risk and reducing disaster. Routledge, Tylor & Francis Group. doi:10.4324/9780203805305
Tayyab, M., Zhang, J., Hussain, M., Ullah, S., Liu, X., Khan, S. N., et al. (2021). Gis-based urban flood resilience assessment using urban flood resilience model: a case study of peshawar city, khyber pakhtunkhwa, Pakistan. Remote Sens. 13 (10), 1864. doi:10.3390/rs13101864
Thieken, A., Merz, B., Kreibich, H., and Apel, H. (2023). Methods for flood risk assessment: concepts and challenges.
Uddin, M. N., Islam, A. S., Bala, S. K., Islam, G. T., Adhikary, S., Saha, D., et al. (2019). Mapping of climate vulnerability of the coastal region of Bangladesh using principal component analysis. Appl. Geogr. 102, 47–57. doi:10.1016/j.apgeog.2018.12.011
Ullah, K., and Zhang, J. (2020). GIS-based flood hazard mapping using relative frequency ratio method: a case study of Panjkora River Basin, eastern Hindu Kush, Pakistan. Plos one 15 (3), e0229153. doi:10.1371/journal.pone.0229153
Ullah, W., Karim, A., Ullah, S., Rehman, A.-U., Bibi, T., Wang, G., et al. (2023). An increasing trend in daily monsoon precipitation extreme indices over Pakistan and its relationship with atmospheric circulations. Front. Environ. Sci. 11, 1228817. doi:10.3389/fenvs.2023.1228817
UNESCO (2009). Global trends in water-related disasters: an insight for policymakers. UNESCO. Available at: https://unesdoc.unesco.org/ark:/48223/pf0000184137_eng.
Velasquez, M., and Hester, P. (2013). An analysis of multi-criteria decision making methods. Int. J. Operations Res. 10.
Vignesh, K. S., Anandakumar, I., Ranjan, R., and Borah, D. (2021). Flood vulnerability assessment using an integrated approach of multi-criteria decision-making model and geospatial techniques. Model. Earth Syst. Environ. 7 (2), 767–781. doi:10.1007/s40808-020-00997-2
Wang, X., Xia, J., Zhou, M., Deng, S., and Li, Q. (2022). Assessment of the joint impact of rainfall and river water level on urban flooding in Wuhan City, China. J. Hydrology 613, 128419. doi:10.1016/j.jhydrol.2022.128419
Wang, Y., Li, Z., Tang, Z., and Zeng, G. (2011). A GIS-based spatial multi-criteria approach for flood risk assessment in the dongting lake region, hunan, Central China. Water Resour. Manag. 25 (13), 3465–3484. doi:10.1007/s11269-011-9866-2
Yamaguchi, S., Ikeda, T., Iwamura, K., Naono, K., Ninomiya, A., Tanaka, K., et al. (2007). Development of GIS-based flood-simulation software and application to flood-risk assessment. 2nd IMA international conference on flood risk assessment.
Keywords: flood hazard, GIS, MCDA, risk mapping, Charsadda, Pakistan
Citation: Ibrahim M, Huo A, Ullah W, Ullah S and Xuantao Z (2025) An integrated approach to flood risk assessment using multi-criteria decision analysis and geographic information system. A case study from a flood-prone region of Pakistan. Front. Environ. Sci. 12:1476761. doi: 10.3389/fenvs.2024.1476761
Received: 06 August 2024; Accepted: 23 December 2024;
Published: 09 January 2025.
Edited by:
Dmitry Efremenko, German Aerospace Center (DLR), GermanyReviewed by:
Muhammad Shahid, Brunel University London, United KingdomCopyright © 2025 Ibrahim, Huo, Ullah, Ullah and Xuantao. This is an open-access article distributed under the terms of the Creative Commons Attribution License (CC BY). The use, distribution or reproduction in other forums is permitted, provided the original author(s) and the copyright owner(s) are credited and that the original publication in this journal is cited, in accordance with accepted academic practice. No use, distribution or reproduction is permitted which does not comply with these terms.
*Correspondence: Aidi Huo, aHVvYWlkaUBjaGQuZWR1LmNu; Waheed Ullah, d3VsbGFoQHJhLmFjLmFl
Disclaimer: All claims expressed in this article are solely those of the authors and do not necessarily represent those of their affiliated organizations, or those of the publisher, the editors and the reviewers. Any product that may be evaluated in this article or claim that may be made by its manufacturer is not guaranteed or endorsed by the publisher.
Research integrity at Frontiers
Learn more about the work of our research integrity team to safeguard the quality of each article we publish.