- 1Geology Department, Faculty of Science, Tanta University, Tanta, Egypt
- 2Central Laboratory for Elemental and Isotopic Analysis (CLEIA), Nuclear Research Center (NRC), Atomic Energy Authority, Cairo, Egypt
- 3Arctic Sustainability Lab, Department of Arctic and Marine Biology, Faculty of Biosciences, Fisheries and Economics, UiT The Arctic University of Norway, Tromsø, Norway
- 4Research Department, CloudEARTHi AS, Tromsø, Norway
- 5OSEAN-Outermost Regions Sustainable Ecosystem for Entrepreneurship and Innovation, University of Madeira Colégio dos Jesuítas, Funchal, Portugal
Surface freshwater systems globally face severe stresses due to overpopulation and associated waste. The Ismailia Canal, a crucial freshwater source in the eastern Nile Delta, Egypt, serves multiple purposes and is endangered by various environmental activities. This study characterizes the canal’s water using physicochemical parameters to evaluate its suitability for different uses. Water samples were collected twice in winter and summer seasons of the year 2018 from eight sites distributed along the course of Ismailia Canal. A comprehensive chemical analysis of the samples was carried out. Water chemistry was graphically and statistically assessed. Water qualities were evaluated using WHO guidelines, water quality index (WQI), Pollution indices of metals (PIm) and long- and short-term effect of trace elements on irrigation. Results show that the water is slightly alkaline and moderately hard, with higher salinity in winter than summer. Major cations and anions are higher in winter, whereas NO₃ is higher in summer. The canal water is primarily of the Ca(Mg)-HCO₃ type, influenced mainly by rock-water interactions. While most physicochemical parameters meet drinking water standards, Al, Sb, As, Cd, Fe, Pb, and Tl exceed limits, with significant impacts from Al and Tl year-round, and seasonal impacts from As, Pb, Cd, and Fe. For irrigation, water quality is generally unaffected in winter, but Mo and Se have slight impacts in summer for long-term use. This research is vital for informing sustainable water management practices, which are crucial for Egypt’s research initiatives, economic stability, and environmental sustainability.
1 Introduction
Since the 1990s, there has been a global worsening of water degradation, and this trend is predicted to continue, posing more risks and having detrimental effects on both the environment and human health. It is well recognized that human survival and social development depend critically on the quality of the water (Fu et al., 2023; Yan et al., 2023). However, the quality of natural water is declining due to the quick development of industry and population growth, endangering not only human health but also ecological services (Wang et al., 2022; Fu et al., 2023). Eutrophication and heavy metal contamination are two pressing environmental issues affecting water resources (Cheng et al., 2021; Wang et al., 2022). To prevent a worldwide water crisis in the near future, concerns about water quality require immediate attention and well-coordinated, well-informed action.
Fresh surface water, though representing a small percentage of Earth’s water, plays a crucial role in human life and the environment. It supports various human activities, including drinking, irrigation, livestock, industrial uses, fishing, recreational activities, hydropower plants, water treatment plants, and wastewater treatment (Dieter et al., 2017; NISC, 2005; Jaskuła et al., 2021; Ghobara and Salem, 2017; Salem et al., 2019). Additionally, fresh surface water sustains ecological systems, providing habitats for numerous plants and animals (NISC, 2005; Elshobary et al., 2020; Salem et al., 2017a). It also influences groundwater resources (NISC, 2005).
The state of fresh surface water can be assessed in terms of quantity and quality. Quantity is influenced by direct withdrawals for human uses, such as drinking, irrigation, and industrial processes, as well as by the withdrawal of groundwater that recharges surface water. Human activities and climate change significantly impact surface water availability, with climate fluctuations further affecting water use (Dieter et al., 2017; Jaskuła et al., 2021; Salem et al., 2017b; Salem et al., 2017c). Quality reflects a range of physical, chemical, and biological characteristics that affect water suitability for various purposes. Exceeding permissible limits for these characteristics can negatively impact human health, especially through drinking, irrigation, or recreational activities (NISC, 2005; Salem et al., 2021). Growing populations and intensified agricultural and industrial activities have turned surface water canals and agricultural drains into dumping sites for industrial wastewater, untreated urban and rural waste, and irrigation runoff (Stahl et al., 2009).
Water contamination, particularly with metals, is a pressing environmental issue. Surface freshwater systems globally face severe stress from overpopulation and associated waste (Ghodeif et al., 2011; UNESCO-MERIC, 2003). Metal pollution poses significant hazards to human health and ecosystems due to its chronic nature, stability, toxicity, and bioaccumulation (Jaskuła et al., 2021; He et al., 2019; Stamatis et al., 2019; Doležalová et al., 2019; Sojka et al., 2019). Consequently, the concentrations, distribution, and sources of metal pollutants are of global concern (Jaskuła et al., 2021; Nawrot et al., 2020; Mandeng et al., 2019; Sun et al., 2018).
In Egypt, freshwater is vital for supporting a growing population and various economic activities. The Ismailia Canal, an important freshwater source, serves areas from Greater Cairo to Ismailia, Sharkia, Port Said, and Suez governorates, providing water for drinking, irrigation, domestic, and industrial purposes (Geriesh et al., 2008). However, the canal is at risk from unwise direct and indirect activities in its surrounding environments (Geriesh et al., 2008). This study uses the Ismailia Canal as a case study to characterize its water and evaluate its suitability for different human uses based on its physicochemical parameters.
2 Ismailia canal
The Ismailia Canal (Figure 1) is a man-made waterway constructed between 1858 and 1863 to supply water to villages along the Suez Canal and to workers during its excavation. It extends from its inlet at the River Nile north of Cairo, near Shubra, passing through the El-Qalioubeya and El-Sharkeya governorates, and flows through Wadi El-Tumulat to its outlet at El-Temsah Lake in the Ismailia governorate. Before reaching Ismailia town, the canal bifurcates: one branch flows north to Port Said governorate, and the other flows south to supply Suez governorate (Geriesh et al., 2008; Goher et al., 2014; Geriesh, 1994; Geriesh and El-Rayes, 2001; Ghodeif et al., 2011; Stahl and Ramadan, 2008).
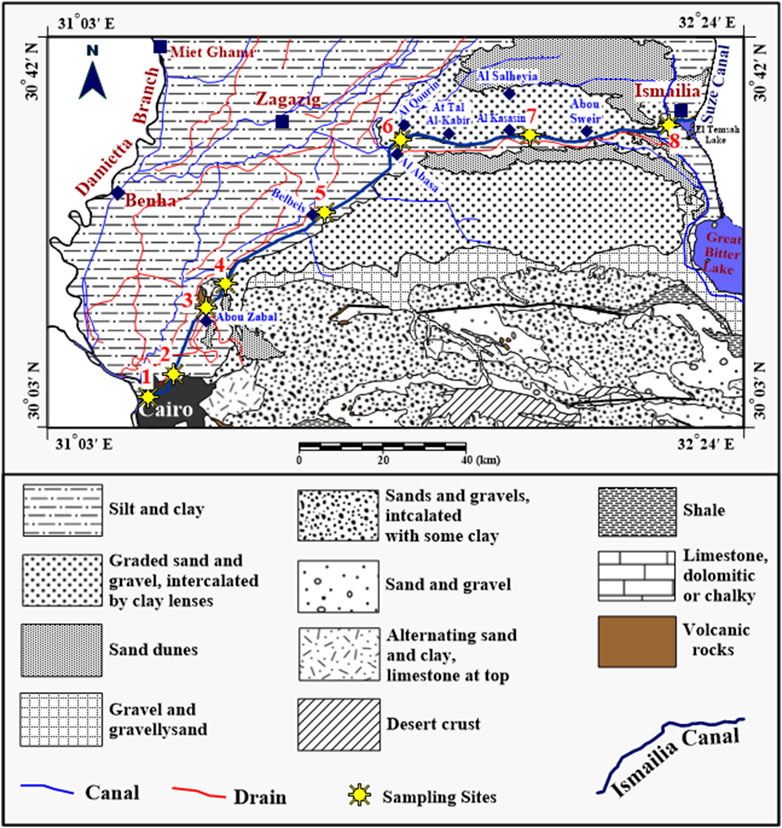
Figure 1. Locations of sampling sites along Ismailia Canal superimposed on the geologic map of the study area (Source: The Geologic Map of Egypt 1981. Modified after Salem et al., 2020.
The Ismailia Canal, being the most distal downstream segment of the Nile River, contains pollutants discharged into the Nile, as large amounts of untreated urban municipal, industrial, and rural domestic waste enter the river. Additionally, it is susceptible to direct pollution from human activities (Geriesh et al., 2008; Ghodeif et al., 2011; Stahl and Ramadan, 2008). Potential contamination sources vary with human activities and can directly or indirectly affect water quality (UNESCO-MERIC, 2003; Ghodeif et al., 2011). Figure 2 identifies large-scale activities and facilities that may contaminate the canal, including agricultural practices, urbanization, industrial development, and transportation (Ghodeif et al., 2011). The lack of vegetative buffer filtration increases contaminant percolation into the canal water. Roads flanking both sides of the canal and rainwater drainage contribute to metal and organic material accumulation in the water (Geriesh, 1994; Geriesh and El-Rayes, 2001; Ghodeif et al., 2011).
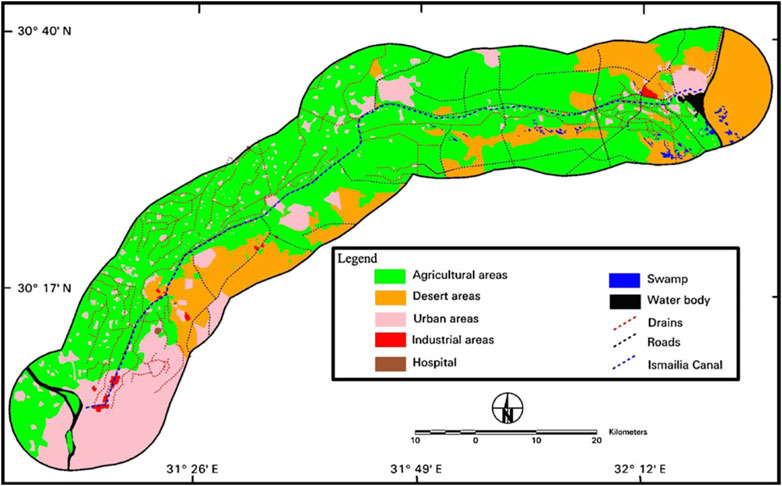
Figure 2. Land uses in the environs of Ismailia Canal that may represent potential sources of contamination (modified after Ghodeif et al., 2011).
The upstream portion of the Ismailia Canal from Cairo to Abu Zaabal includes several industrial activities such as petroleum, petro-gases, iron and steel, fertilizers, aluminum sulfate, and detergent production (Figure 2), which are major pollution sources. Additionally, water treatment plants discharge wastewater rich in aluminum, iron, and manganese into the canal. Common pollution sources from urban areas include chemical leaks, industrial runoff, and liquid waste, which can pollute canal water and subsequently contaminate the soil through irrigation (Geriesh et al., 2008; Stahl and Ramadan, 2008). In rural areas, potential pollution sources include irrigation runoff, agricultural effluents, excessive use of heavy metal-containing fertilizers and pesticides, unsafe sewerage systems, waste disposal seepage, and septic tanks near the canal (Geriesh et al., 2008; Ghodeif et al., 2011; Stahl and Ramadan, 2008). Municipal effluents, sewage runoff, and industrial waste pumped into drains and surface water canals cause water resource degradation with severe health concerns (Herzog et al., 2012). Downstream reaches of the canal face high pollution risks, acting as an effluent stream during winter, especially during droughts (Geriesh et al., 2004).
The canal flows through diverse geological environments (Figure 1), including Nile silts and muds, Miocene limestone, marls and sandstone, Oligocene gravels, and basaltic sheets upstream, and Wadi El-Tumilat sands and muds downstream (Geriesh et al., 2008). Groundwater and surface water systems are usually connected and can impact each other (Ghodeif et al., 2011). The hydrologic relationship between the canal and groundwater varies significantly. The canal is recharged by the surrounding shallow aquifer during the High Dam gates’ closure, while it acts as an influent stream, losing water, for the rest of the year. The canal acts as an effluent stream in most parts except from Abu-Zaabal to Inshas and from At-Tal Al Kabir to Al Kasasin, where it acts as an influent stream (Geriesh, 1994; Ghodeif et al., 2011).
3 Methodology
Eight sites were selected for sampling of Ismailia canal water as shown in Figure 1. Stream water samples were collected from the upstream at Cairo city to the downstream at Ismailia City.
Water samples were collected twice in the winter (in January) and the summer (in August) of the year 2018. Samples were collected from depths of 0.5 m below the water surface in the middle width of the stream and filtered to removes the suspended material from water. The sampling process was conducted following the standard methods set by APHA/AWWA/WEF (2017) for the examination of water. New, pre-cleaned, acid-rinsed, high-density polyethylene bottles with polyethylene caps were used. In each site, samples were collected in two bottles one is unacidified and the other is pre-acidified using HNO3 to pH < 2. Samples were stored in cold storage at temperatures below 4oC. A Hach portable EC/TDS meter and portable pH meter (Model P 314) were used to measure EC, TDS and pH in site after calibration. Ion Chromatography (GBC, Timerline Instrument, Boulder, Colorado) was employed to determine the concentrations of K, Na, Mg, Ca, Cl, SO₄, HCO₃, CO₃, and NO₃ ions. Total hardness (TH) was calculated using Equation 1. An Inductively Coupled Plasma-Optical Emission Spectrometer (ICP-OES, Prodigy HDICP, Teledyne, Leeman Labs) was used to analyze trace elements, including Al, Sb, As, Ba, Be, Cd, Cr, Co, Cu, Fe, Pb, Li, Mn, Mo, Ni, Se, Tl, and V. Preservation, preparation, and chemical analyses of the water samples were performed in the Central Laboratory for Elemental and Isotopic Analysis (CLEIA), Nuclear Research Center (NRC), Atomic Energy Authority, and the Geochemistry Lab., Nuclear Materials Authority, Egypt. The obtained results were tabulated, statistically analyzed, and graphically presented. The Surfer program (version 11.1.719—Surface Mapping System—Golden Software, Inc.) was used for mapping purposes. Statistical analyses, including correlation and cluster analysis, were performed using PAST version 2.17c (Hammer et al., 2001).
Drinking water quality index (WQI) were calculated based on WHO (2017) guidelines and the relative importance of each parameter for drinking suitability (for calculation procedure see Abbasi and Abbasi, 2012; Salem et al., 2017b; Xiao et al., 2021). According to Gao et al. (2020), WQI values are classified into five categories can be assigned: undrinkable (WQI ≥300), very poor (200:300), poor (100:200), good (50:100), and excellent (WQI<50). Only the good and Excellent classes were recognized in the studied water. Pollution indices of metals (PIm) were calculated, using Equation 2 (Goher et al., 2014), for the individual elements to evaluate the pollution effect of each element on the quality of Ismailia canal water for drinking and irrigation purposes in both winter and summer seasons.
where:
Ci is the concentration of each element; Si is the standard limit of each element based on the concerned quality criteria, and the subscript suffixes, max. and min, refer to the maximum and minimum values. Effects of elements are classified based on the PIm values into five classes, which are: No Effect (PIm <1), Slight Effect (PIm = 1–2), Moderate Effect (PIm = 2–3), Strong Effect (PIm = 3–5) and Serious Effect (PIm >5).
4 Results
4.1 Statistical evaluation and spatial variation
Thirty physicochemical parameters: pH, EC, TDS, K, Na, Mg, Ca, Cl, SO4, HCO3, CO3, NO3, Al, Sb, As, Ba, Be, Cd, Cr, Co, Cu, Fe, Pb, Li, Mn, Mo, Ni, Se, Tl and V were determined in the collected samples. Table 1 shows the descriptive statistical summary of the obtained results.
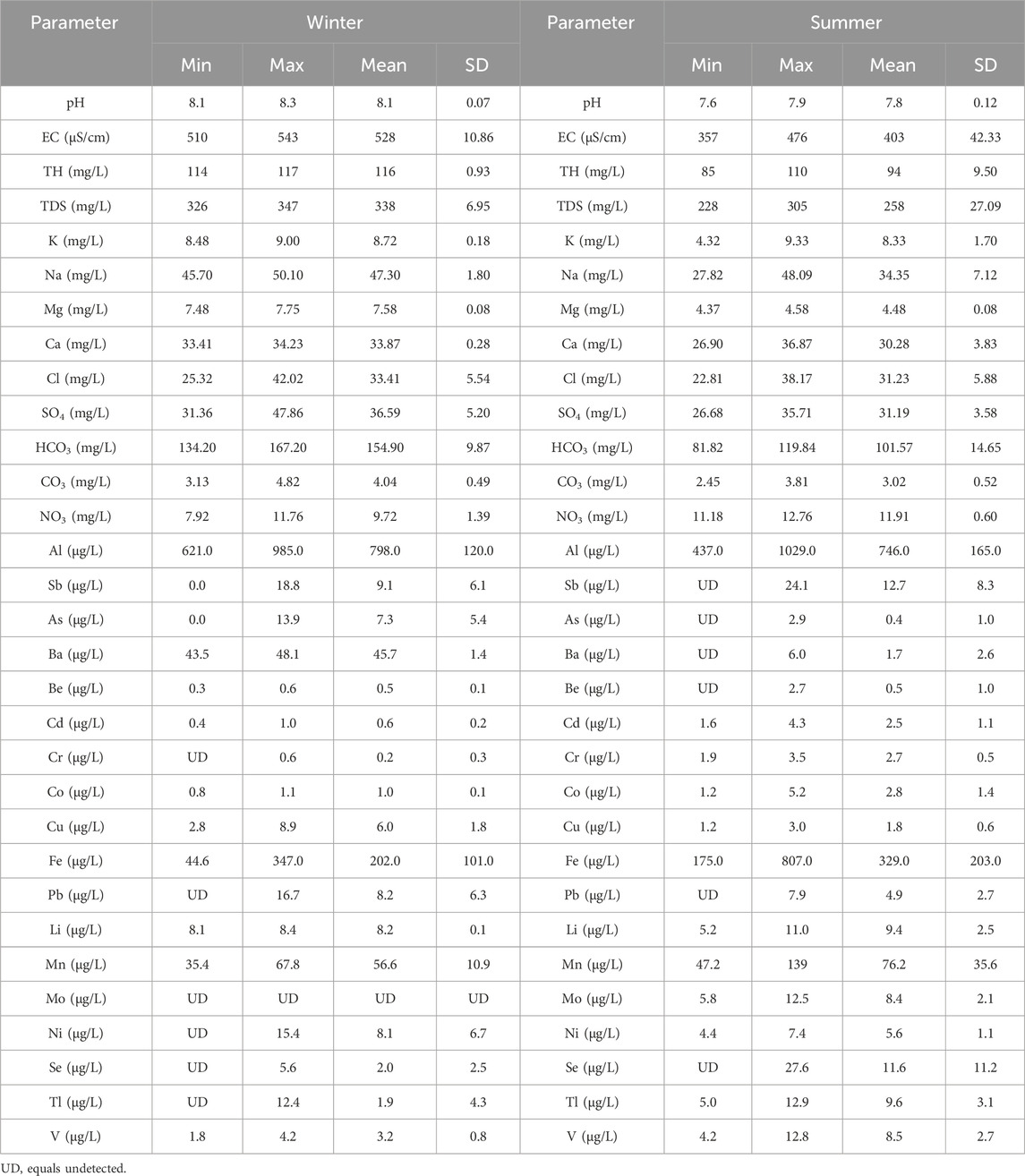
Table 1. Summary of the descriptive statistics of the determined physicochemical parameters in the water samples of Ismailia Canal.
4.1.1 The pH, EC, TH and TDS
The pH of water samples ranges from 8.08 to 8.29 with a mean value of 8.14 in winter (Figure 3A). In summer, the pH values range from 7.64 to 7.98 with a mean value of 7.83 (Figure 3B), indicating that Ismailia Canal water tends to be slightly alkaline. The pH values of Ismailia Canal water are within the range of 6.5–9.0 set by USEPA (2008) for freshwater. Regarding seasonal variation (Figure 4A), the highest pH value, 8.29, is recorded in winter at sampling site 1, while the lowest value, 7.64, is recorded in summer at sampling site 2. However, the variation of pH along the canal shows an increasing trend in summer and a decreasing trend in winter. As shown in Figure 4A, the pH values do not show significant seasonal or spatial variations along the canal. Several studies mention the slightly alkaline nature of Ismailia Canal water (Goher et al., 2014; Abdo and El-Nasharty, 2010; Elhaddad, 2014; Khalil et al., 2012; Yusuf et al., 2018) and attribute this alkalinity to increased photosynthetic activity, which raises the pH. Conversely, the decomposition of organic matter releases acidic gases, lowering the pH of the water (Yusuf et al., 2018).
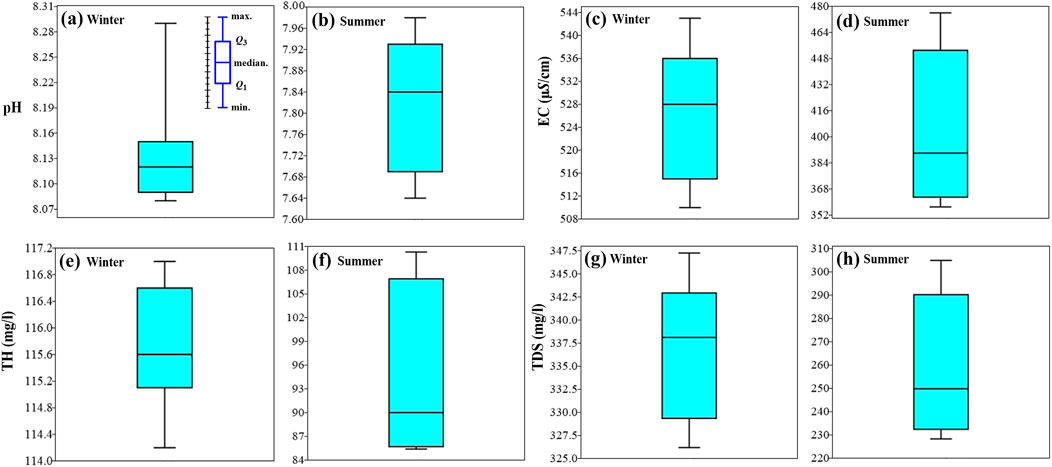
Figure 3. Box plots of pH (A, B), EC (C, D), TH (E, F) and TDS (G, H) in winter and summer seasons for Ismailia Canal water samples.
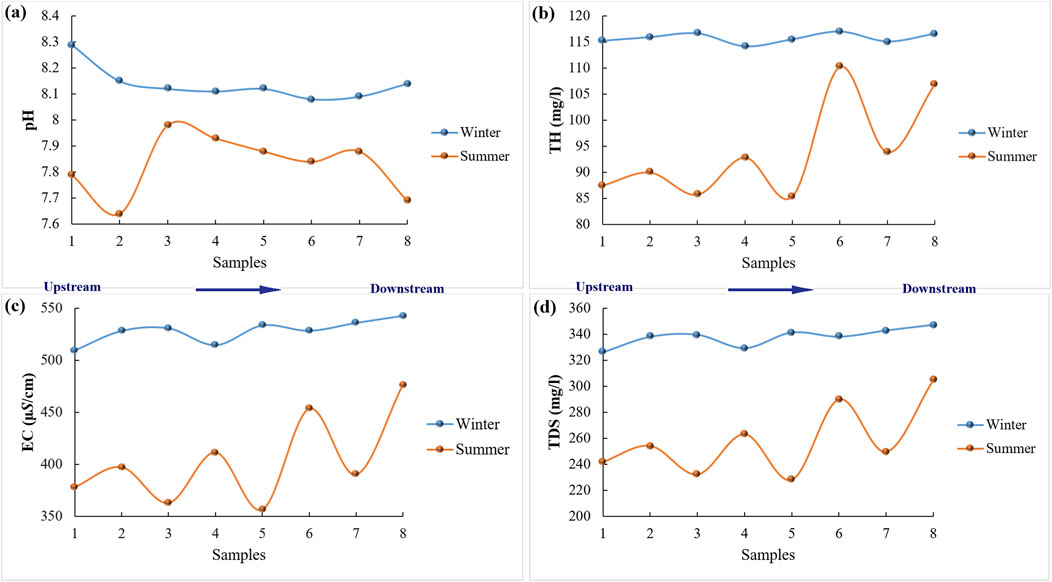
Figure 4. Spatial variation of pH (A), TH (B), EC (C) and TDS (D), TH in winter and summer seasons along the watercourse of Ismailia canal.
The total hardness (TH as CaCO₃ in mg/L) of the water samples shows an increasing trend in both winter and summer (Figure 4B). It ranges from 114 to 117 mg/L with a mean value of 116 mg/L in winter (Figure 3E), while in summer, it ranges from 85 to 110 mg/L with a mean value of 94 mg/L (Figure 3F). According to Dhok et al. (2013), Gebresilasie et al. (2021), McGowan (2000), Ramya et al. (2015), Rout and Sharma (2011), and Singh et al. (2021), the water of Ismailia Canal is classified as moderately hard in both winter and summer. As shown in Figure 4B, TH shows significant spatial variation in summer along the canal, while it shows insignificant changes between sampling sites in winter.
The EC of the water samples ranges from 510 to 543 μS/cm with a mean value of 528 μS/cm in winter (Figure 3C). In summer, it fluctuates along the canal (Figure 4C) and ranges from 357 to 476 μS/cm with a mean value of 403 μS/cm (Figure 3D). As shown in Figure 4C, the EC reaches its highest value in winter at sampling site eight and its lowest value in summer at sampling site 5. The TDS ranges from 326 to 347 mg/L with a mean value of 338 mg/L in winter (Figure 3G), while in summer, TDS ranges from 228 to 305 mg/L with a mean value of 258 mg/L (Figure 3H). A similar variation is observed for TDS, with general increasing trends from upstream to downstream, where the highest values are recorded in the downstream reaches of the canal (Figures 4C,D). TDS and EC are relatively higher in winter than in summer, which could be attributed to the lowering of the Ismailia Canal water level during winter, concentrating the dissolved ions. Additionally, the death and decay of microorganisms release different ions into the water. In summer, the water level increases, leading to high dilution and a drop in EC and TDS values (Salem et al., 2019; Abdo and El-Nasharty, 2010; Yusuf et al., 2018; Abdel-Satar et al., 2017; Islam et al., 2015). The average TDS of Ismailia Canal water recorded a little higher values to Damietta Nile Branch (266.25 mg/L, El-Battrawy et al., 2022), lower values in contrast to El-Salam Canal in the Northern East Nile Delta region (852.60 mg/L, El-Amier, et al., 2021) and lower concentrations relative to surface water of Obuasi mining district in Ghana (669 mg/L, Armah et al., 2010).
4.1.2 Major and minor ions
In winter, the cations K, Na, Mg, and Ca range from 8.48 to 9.0 mg/L, 45.66–50.09 mg/L, 7.48–7.75 mg/L, and 33.41–34.23 mg/L, respectively, with mean values of 8.72, 47.34, 7.58, and 33.87 mg/L, respectively (Figures 5A, C, E, G). In summer, these cations range from 4.32 to 9.33 mg/L, 27.82–48.09 mg/L, 4.37–4.58 mg/L, and 26.9–36.87 mg/L, respectively, with mean values of 8.33, 34.35, 4.48, and 30.28 mg/L, respectively (Figures 5B, D, F, H).
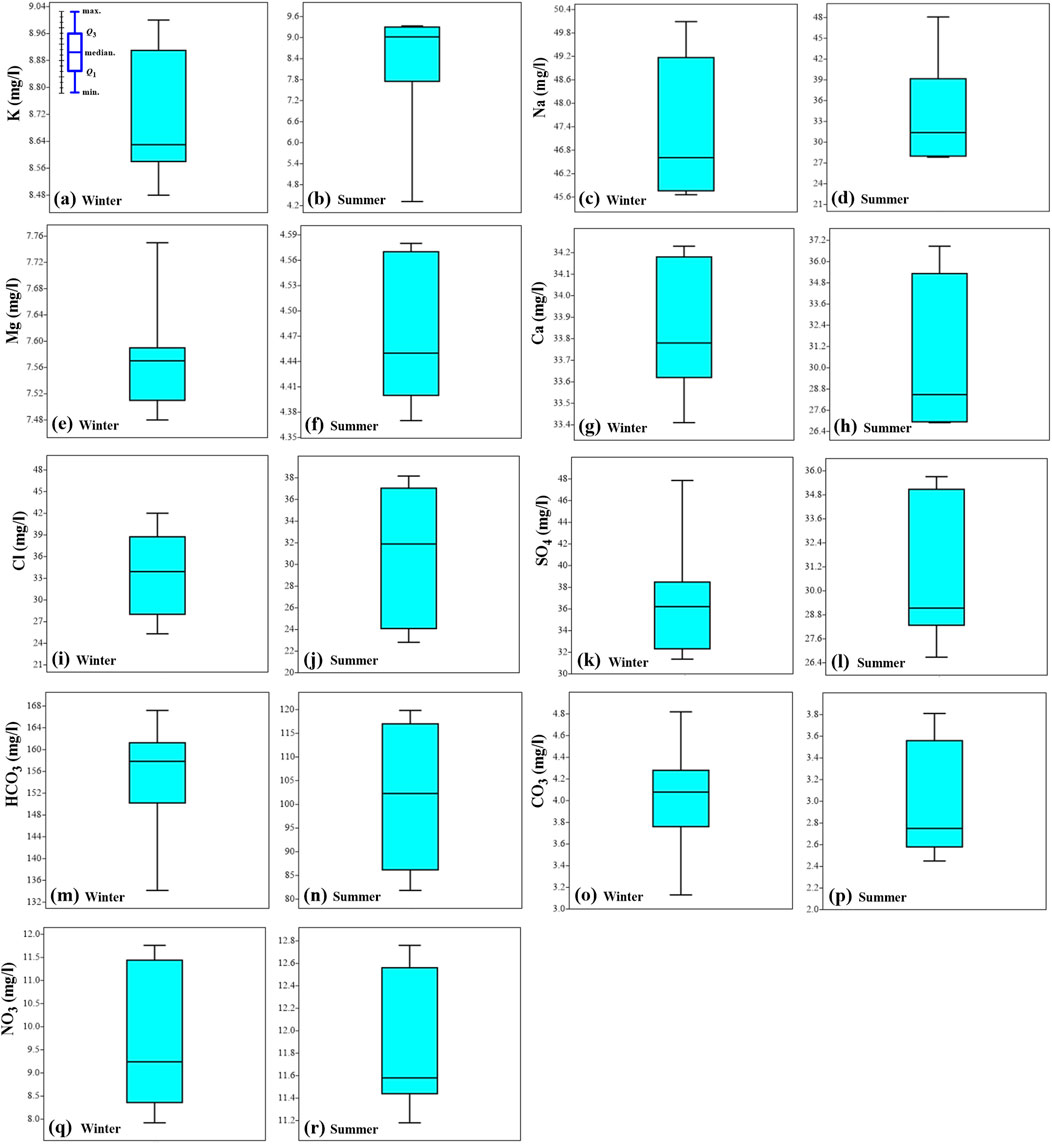
Figure 5. Box plots of ions concentrations K (A, B), Na (C, D), Mg (E, F), Ca (G, H), Cl (I, J), SO4 (K, L), HCO3 (M, N) CO3 (O, P) and NO3 (Q, R) in winter and summer seasons for Ismailia canal water samples.
K shows insignificant seasonal changes except at sampling site 4, where its summer value is much lower than its winter value (Figure 6). Na, Ca, and Mg exhibit seasonal variations, with Na and Ca fluctuating along the canal in summer and showing insignificant changes between sites in winter. Mg displays insignificant variation between sites in both seasons. Generally, all cations show increasing trends from upstream to downstream in both winter and summer, except Mg, which slightly decreases in summer (Figure 6B).
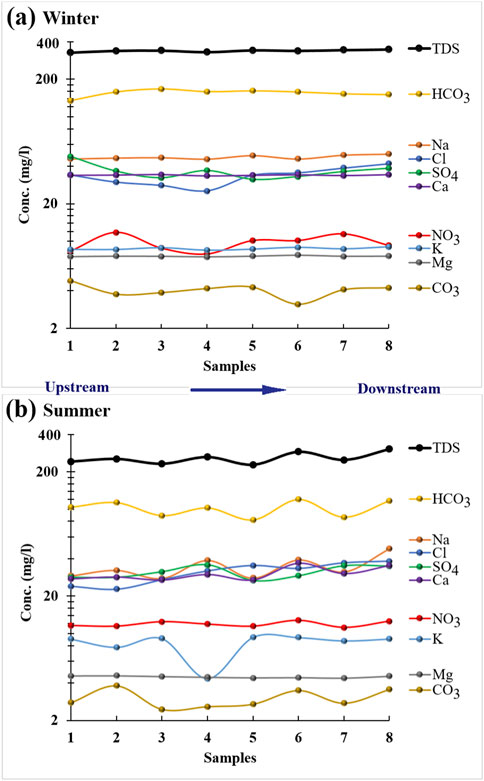
Figure 6. Spatial variation of TDS, TH and ions concentrations in winter (A) and summer (B) seasons along the watercourse of Ismailia canal.
The anions Cl, SO₄, HCO₃, CO₃, and NO₃ range in winter from 25.32 to 42.02 mg/L, 31.36–47.86 mg/L, 134.15–167.19 mg/L, 3.13–4.82 mg/L, and 7.92–11.76 mg/L, respectively, with mean values of 33.41, 36.59, 154.95, 4.04, and 9.72 mg/L, respectively (Figures 5I, K, M, O, Q). In summer, these anions range from 22.81 to 38.17 mg/L, 26.68–35.71 mg/L, 81.82–119.84 mg/L, 2.45–3.81 mg/L, and 11.18–12.76 mg/L, respectively, with mean values of 31.23, 31.19, 101.57, 3.02, and 11.91 mg/L, respectively (Figures 5J, L, N, P, R).
All anions show significant seasonal variations except Cl and SO₄, which display similar distribution patterns in both seasons (Figure 6). Generally, all anions increase from upstream to downstream in both winter and summer, except SO₄ and CO₃, which decrease in winter (Figure 6A). HCO₃ fluctuates in summer and shows insignificant spatial changes in winter along the canal. HCO₃ is relatively higher in winter than in summer, possibly due to bacterial decomposition and fermentation of organic matter, which increases during drought periods, producing HCO₃ as a final product, along with the high evaporation rate in summer (Salem et al., 2019; Abdo and El-Nasharty, 2010; Yusuf et al., 2018).
CO₃ and NO₃ display opposite distribution patterns in winter and summer across all sampling sites except sites two and 6. All anions are relatively higher in winter than in summer, except NO₃, which is higher in summer than in winter.
Based on the mean values of cations and anions, cations follow the dominance order Na > Ca > K > Mg in both winter and summer. Anions follow the order HCO₃ > SO₄ > Cl > NO₃ > CO₃ in winter and HCO₃ > Cl > SO₄ > NO₃ > CO₃ in summer.
4.1.3 Trace elements
In the winter season, trace elements such as Al, Ba, Be, Cd, Co, Cu, Fe, Li, Mn, and V are recorded in all samples. Meanwhile, Sb, As, Cr, Pb, Ni, Se, and Tl are recorded in 87.5%, 75%, 50%, 75%, 75%, 50%, and 37.5% of the samples, respectively, and Mo is not detected at all (Figure 7A). In the summer season, trace elements including Al, Cd, Cr, Co, Cu, Fe, Li, Mn, Mo, Ni, Tl, and V are present in all samples. Sb, As, Ba, Be, Pb, and Se are recorded in 87.5%, 12.5%, 25%, 25%, 87.5%, and 75% of the samples, respectively (Figure 7B).
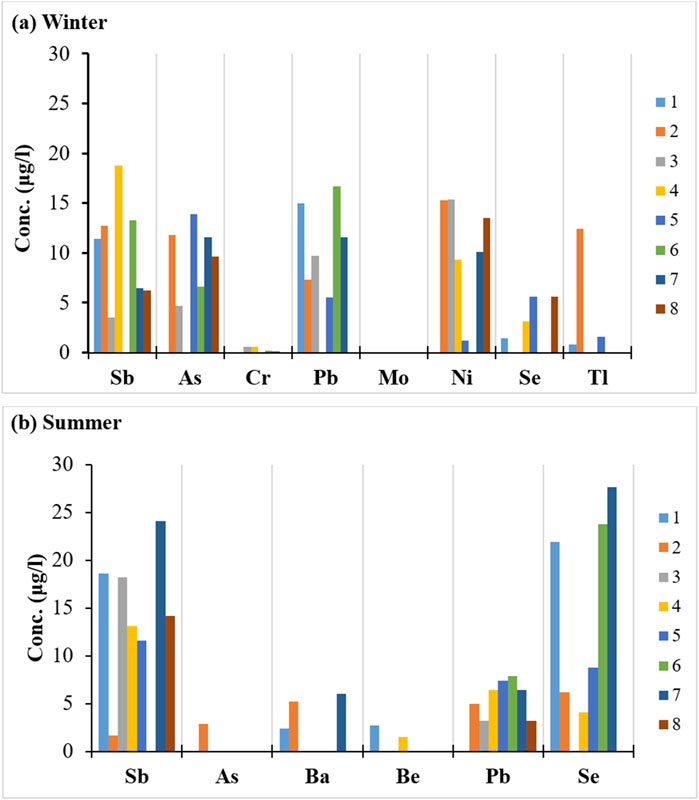
Figure 7. Histograms show the recorded element concentrations in the collected water samples: (A) in winter and (B) in summer.
As shown in Figure 8, the trace element concentrations vary along the course of the Ismailia Canal in both winter and summer. Elements such as As (Figures 7A, B), Ba (Figure 8A; Figure 7B), and Cu (Figures 7C, D) have higher concentrations in winter than in summer. Conversely, elements like Cd (Figures 8D, E), Cr (Figure 7A; Figure 8D), Co (Figures 8D, E), Mo (Figure 8D), and V (Figures 8C, D) have higher concentrations in summer than in winter. In most samples, Al, Be, Pb, and Ni have higher concentrations in winter compared to summer. In contrast, Sb, Fe, Li, Mn, Se, and Tl concentrations are higher in summer than in winter (Figures 7, 8).
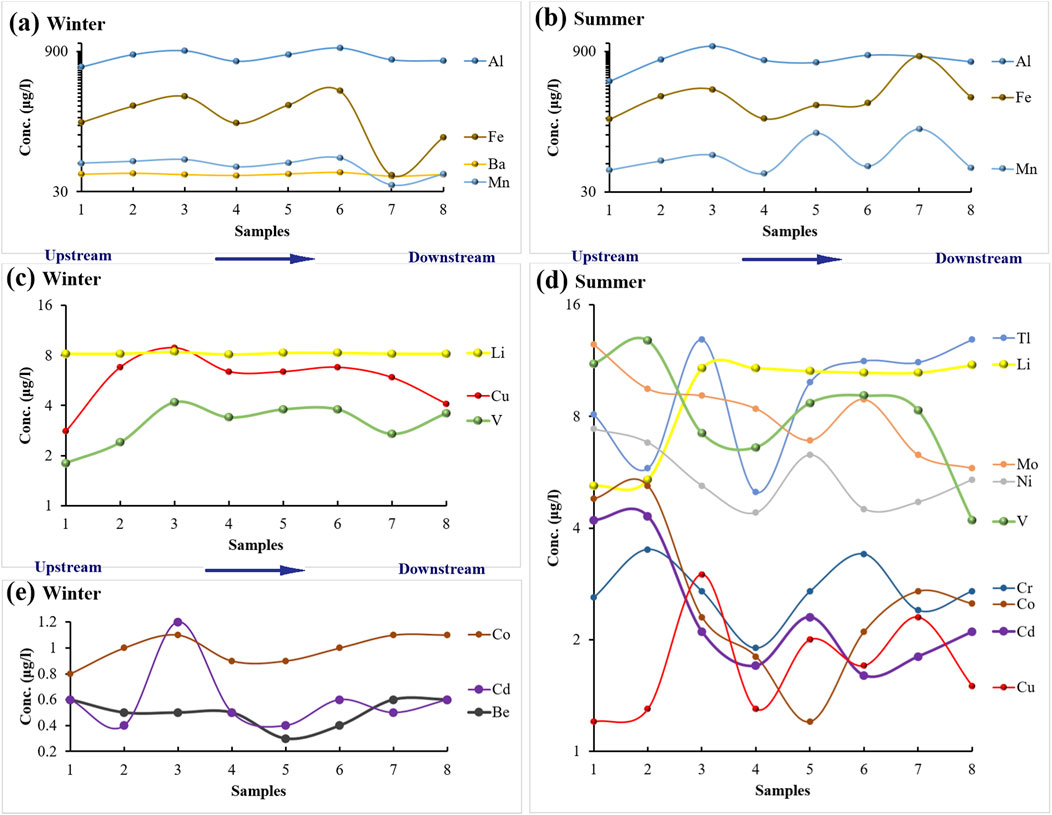
Figure 8. Spatial variation of the trace metals’ concentrations along the watercourse of Ismailia Canal in winter (A, C, E) and summer (B, D) seasons.
Seasonal variations in metal concentrations may be attributed to changes in pH, where the canal water has relatively higher pH values in winter than in summer. pH changes can alter the chemical state of many pollutants, affecting their solubility and transport between canal water and bottom sediments (USEPA, 2021). The geology and lithology (Figure 1), as well as human activities such as agricultural practices, urbanization, and industrial activities (Figure 2), influence the inputs of H and OH ions in the water and the level of chemical pollution through rock-water interactions, leakage, leachates, and direct effluent discharges (USEPA, 2021; Li, et al., 2023). Additionally, rainwater and road maintenance works are potential sources of pollution that may result in the accumulation of metals and organic material in the canal water (Geriesh, 1994; Geriesh and El-Rayes, 2001; Ghodeif et al., 2011; Stahl and Ramadan, 2008; Geriesh et al., 2008).
Armah et al. (2010) found that the Obuasi mining area in Ghana contains surface water with elevated concentrations of heavy metals, particularly Hg, Pb, As, Cu, and Cd, exceeding the permissible levels set by the WHO and clearly indicating human impact. El-Amier et al. (2021) indicated that the levels of Fe and Zn in the El-Salam Canal in the Northern East Nile Delta are within acceptable limits for both drinking and irrigation purposes. However, they found that Mn, Pb, Cu, Co, Ni, Cr, and Cd were present at higher concentrations than recommended. The heavy metals in the raw water samples from the Nile River were analyzed by El-Battrawy et al. (2022). Their findings revealed that Copper, Cobalt, and Zinc were not detected in the raw water samples. However, the concentration of Cadmium was measured at 0.021 ± 0.0008 mg/L, Nickel at ±0.047 0.0002 mg/L, and Lead at ± 0.067 0.0005 mg/L, all of which exceeded the permissible limits as per the guidelines provided by WHO (2017). The difference between the water of the Al-Salam Canal and the water of the Nile River, on the one hand, and the water of the Ismailia Canal, on the other hand, in the content and type of pollutants leads to the conclusion that the local environment around the Ismailia Canal, including its industrial, agricultural, urban, and other activity, played a major role in canal water pollution.
4.1.4 Correlation analysis
Pearson’s correlation analysis (Table 2) is used to determine the relationships between the physicochemical parameters of Ismailia Canal water, with correlation strengths estimated based on Evans (1996) guidelines (McSeveny et al., 2009; Beldjazia and Alatou, 2016).
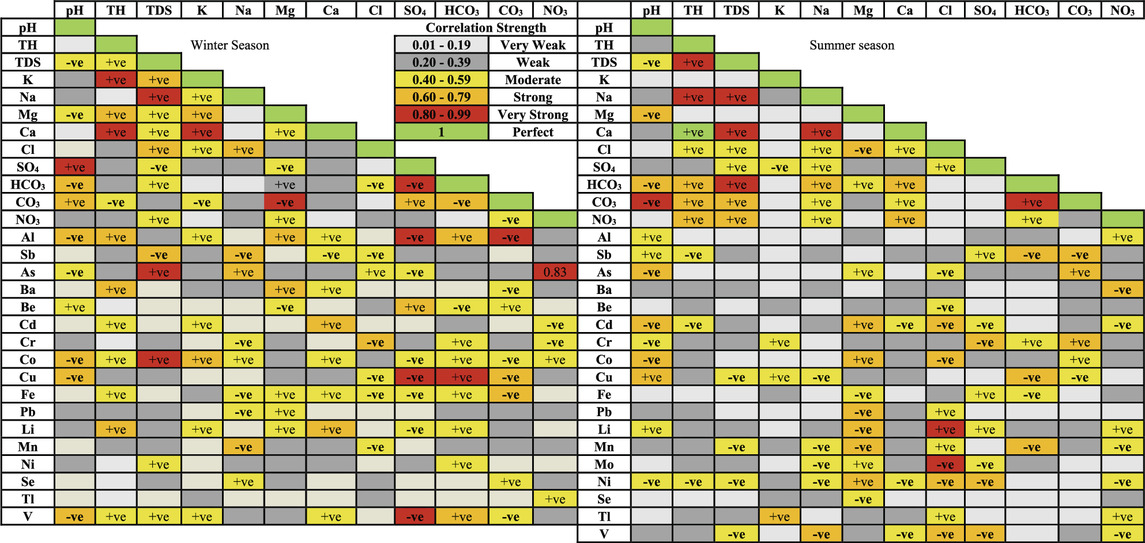
Table 2. Pearson’s correlation analysis of the physicochemical parameters of the collected water samples.
In the winter season, pH shows a very strong positive correlation with SO₄, a strong positive correlation with CO₃, and strong negative correlations with HCO₃, Al, Co, Cu, and V (Table 2). In the summer, the correlation with SO₄ becomes weak, while its correlation with CO₃ remains very strong but negative. It also retains a strong negative correlation with HCO₃ and shows strong negative correlations with Mg, Al, Cd, and Co, and a strong positive correlation with Cu (Table 2).
Total hardness (TH) in winter shows very strong correlations with K and Ca, and strong correlations with Mg, Al, Ba, and Li (Table 2). In summer, TH correlates very strongly with TDS and Na, and strongly with HCO₃, CO₃, and NO₃, with a perfect correlation with Ca (Table 2).
TDS in winter has very strong correlations with Na, As, and Co, and strong correlations with K and Cl, as well as a strong negative correlation with Sb (Table 2). In summer, TDS shows very strong correlations with Na, Ca, and HCO₃, and strong correlations with CO₃ and NO₃, while the correlation with Cl weakens, and other parameters show insignificant correlations (Table 2).
In winter, K is very strongly correlated with Ca and strongly with Mg. Na shows a strong correlation with Cl, and Mg has a very strong negative correlation with CO₃. Other correlations between cations are less significant (Table 2). In summer, important correlations include very strong and strong correlations between Na and Ca and HCO₃, respectively, a strong negative correlation between Mg and Cl, and strong correlations between Ca and HCO₃ and NO₃ (Table 2).
Among anions in winter, there is a very strong negative correlation between SO₄ and HCO₃, and strong positive and negative correlations between CO₃ and SO₄ and HCO₃, respectively (Table 2). In summer, the only significant correlation is a very strong correlation between HCO₃ and CO₃ (Table 2).
In winter, important correlations between trace elements and cations include K and Co, Na and Sb, As, and Mn (negative for Sb and Mn), Mg and Al and Ba, and Ca and Cd and Li (Table 2). In summer, significant correlations include K and Tl, Na and V (negative), and Mg with Cd, Co, Pb, Li, Mn, and Ni (negative for Pb, Li, and Mn) (Table 2).
For trace elements and anions in winter, significant correlations include a strong negative correlation between Cl and Cr, very strong negative correlations between SO₄ and Al, Cu, and V, a strong correlation between SO₄ and Be, very strong correlation between HCO₃ and Cu, strong correlations between HCO₃ and Al and V, a very strong negative correlation between CO₃ and Al, strong negative correlations between CO₃ and Cu and Fe, and a very strong correlation between NO₃ and As (Table 2). In summer, significant correlations include very strong positive and negative correlations between Cl and Li and Mo, respectively, strong negative correlations between Cl and Cd, Co, Ni, and V, strong negative correlations between SO₄ and Cr, Ni, and V, strong negative correlations between HCO₃ and Sb, Cu, and Mn, strong correlations between CO₃ and As and Cr, a strong negative correlation between CO₃ and Sb, and a strong negative correlation between NO₃ and Ba (Table 2).
Tables 3 shows that correlation relationships among trace elements vary more in summer than in winter. In winter, Al is very strongly correlated with Cu and Fe and strongly with Be, Li, and V. Sb is strongly correlated with As and Li, Ba with Fe and Mn, and Fe with Li and Mn. Other strong correlations include Be-Fe, Cd-Li, Cr-Cu, Co-Ni, Cu-V, and Pb-Se (Table 3). In summer, Al is strongly correlated with Be, Cu, and Ni, As with Cd, Cr, Co, Li, and V, Ba with Co and Fe, Cd with Co, Li, Ni, Pb, Mo, and V, Co with Pb, Mo, Ni, and V, and Li with Ni, V, and Mo. Other strong correlations include Sb-Cr, Be-Mo, Cu-Tl, Fe-Mn, Mo-V, and Ni-V (Table 3).
4.1.5 Cluster analysis
Cluster analysis is performed on the Ismailia Canal water samples, and Figure 9 shows the dendrograms of the chemical data for winter and summer seasons. Four clusters are obtained in both seasons with similar associations. As shown in Figure 9, TDS forms cluster 1, while NO₃, K, Mg, CO₃, and all the measured trace elements form cluster 2. Cluster three includes Ca, Na, Cl, and SO₄, and HCO₃ forms cluster 4.
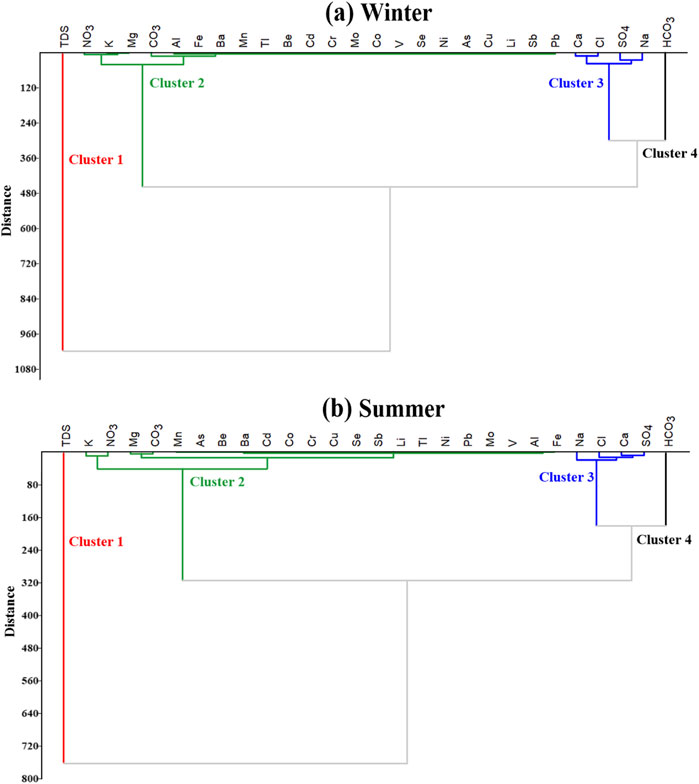
Figure 9. Dendrograms show the ions associations in Ismailia canal water samples in winter (A) and summer (B) seasons.
In both winter and summer seasons, the major ions Na, Ca, Cl, and SO₄ cluster together, indicating the chemical nature of the canal water (Figures 9A, B). NO₃, K, Mg, CO₃, and all the measured trace elements group together, suggesting water pollution is influenced by human activities and rock weathering processes. HCO₃ is isolated in a separate cluster, highlighting its role in the bacterial decomposition and fermentation of organic matter, with HCO₃ being the final product of these processes, along with the impact of high evaporation rates in summer (Abdo and El-Nasharty, 2010; Salem et al., 2019; Yusuf et al., 2018).
4.2 Hydrochemical evaluation
4.2.1 Water types
The Spreadsheet for Water Analyses™ (RockWare Aq•QA), is used to construct the Piper trilinear diagram, where ion concentrations are expressed as meq/l, to characterize the water types in the Ismailia Canal (Piper, 1994). As shown in Figure 10A, for the winter season, samples fall in the field of ‘no dominant type’ on the cationic triangle, close to the field of ‘Na(K)-type’. On the anionic triangle, the samples fall in the ‘HCO₃-type’. For the summer season (Figure 10B), most samples fall in the field of ‘no dominant type’ on the cationic triangle, with one sample in the ‘Na(K)-type’ field. On the anionic triangle, most samples fall in the ‘HCO₃-type’ field, with some in the ‘no dominant type’ field, showing a linear trend extending from ‘HCO₃-type’ to ‘no dominant type’.
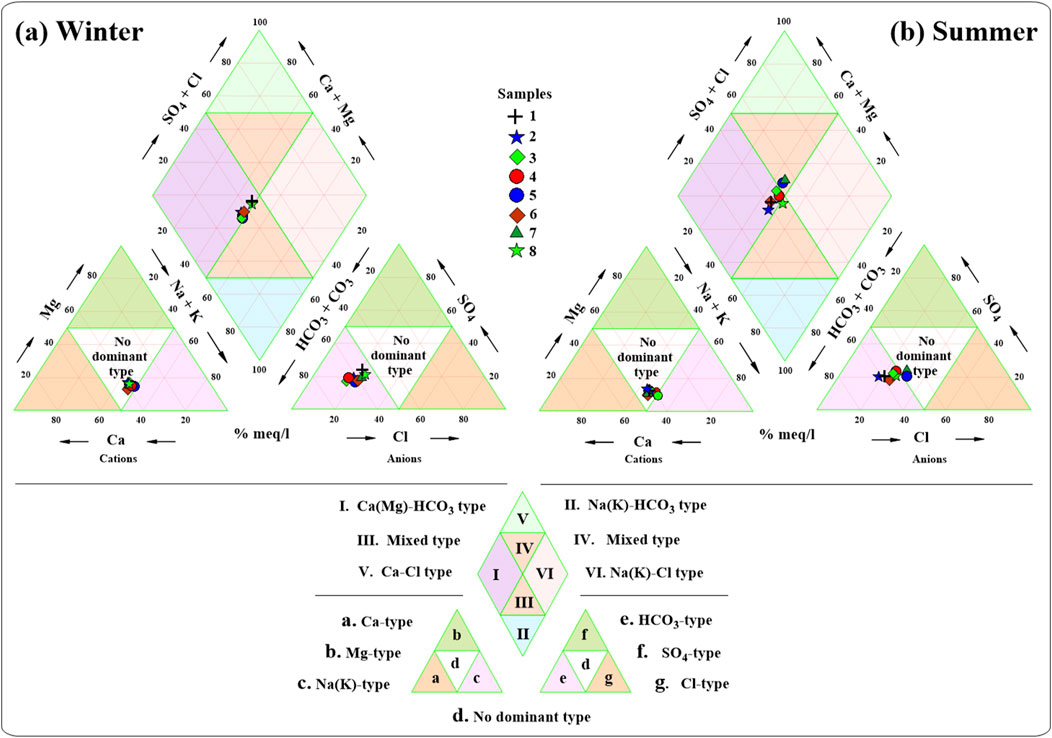
Figure 10. Piper diagrams show the hydrochemical types of the water samples of Ismailia canal in (A) winter and (B) summer.
On the diamond plot for the winter season, samples fall in the field characterized by the Ca(Mg)-HCO₃ water type (Figure 10A). In the summer season, most samples also fall in the Ca(Mg)-HCO₃ water type field, with some samples falling into the mixed water type fields (Figure 10B).
4.2.2 Major processes
The Gibbs diagram is used to estimate the main processes controlling the surface water chemistry in the Ismailia Canal (Gibbs, 1970). As shown in Figure 11 for both winter and summer seasons, the surface water samples of the Ismailia Canal fall in the area dominated by rock dominance. However, the ratio Na/(Na + Ca) is greater than 0.5 in both winter and summer seasons (Figure 11A), while the ratio Cl/(Cl + HCO₃) is less than 0.5 in both seasons (Figure 11B). This suggests that the chemistry of the Ismailia Canal water might not be solely controlled by rock weathering in its anion composition and/or evaporation in its cation composition (Lu et al., 2015; Chai et al., 2024).
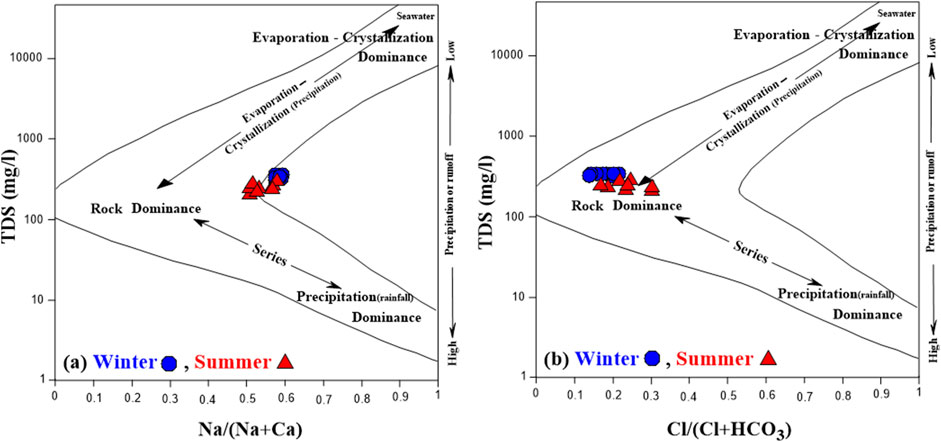
Figure 11. (A, B) Gibbs diagrams of the surface water of Ismailia canal in winter and summer seasons.
5 Discussion
5.1 Water quality
The water quality of the Ismailia Canal and the distribution of metals have been studied by several authors (Elshobary et al., 2020; Geriesh et al., 2008; Goher et al., 2014; Stahl and Ramadan, 2008; Geriesh et al., 2004; Abdo and El-Nasharty, 2010; Abd El-Hady and Hussian, 2012; Abdo, 2013; Abdo et al., 2012; Abdo et al., 2010; El-Sayed, 2008; Ibrahim et al., 2009; Khalifa, 2014; Tarek and Ali, 2007; Youssef et al., 2010). By comparing the obtained results of the physicochemical parameters of the water samples with the standard limits for drinking water according to WHO (2017) (Table 4), we find that the concentrations of ions K, Na, Mg, Ca, Cl, SO₄, HCO₃, CO₃, and NO₃ do not exceed the standard limits for drinking water. However, concentrations of Al, Sb, As, Cd, Fe, Pb, and Tl exceed the respective limits set by WHO (2017).
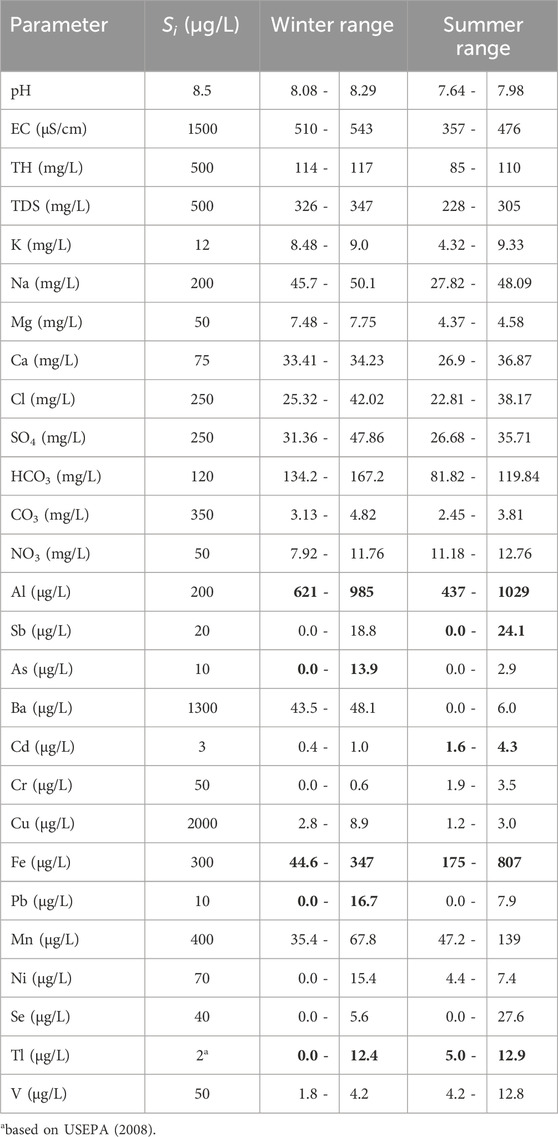
Table 4. Drinking water standard limits (Si) according to WHO (2017) guidelines and the concentration ranges of the physicochemical parameters of the canal water in winter and summer seasons. The bold parameters ranges exceeded their equivalent standard limits.
Based on the drinking water suitability limits for trace elements, the following elements exceeded the allowable limits: Al (all samples), Fe (3 and 6), pb (1, six and 7) and Ti (2). While in the summer, the following elements exceeded the limits of suitability: Al (all samples), Sb (7), Cd (1 and 2), Fe (2, three and 7) and Ti (all samples). The diversity of pollution in one season is attributed to the difference in pollution sources along the canal path, and the difference between the two seasons is due to the difference in the interaction between the canal water and the groundwater (Salem et al., 2019; Geriesh et al., 2008).
The general assessment of the drinking water quality of the Ismailia Canal was calculated by WQI, taking into account the chemically measured elements and their relative weight in water quality. The samples under study showed quality from excellent to good (Figure 12). It was also noted that in the winter the upstream samples towards Cairo are of lower quality than those of the downstream towards Ismailia. As for the winter, there is no specific trend where the highest value of WQI is noticed in sample six and the lowest value is in sample 4.
National Academy of Sciences and the National Academy of Engineering (1972) recommended maximum concentrations of trace elements for irrigation purposes (Table 5). The current results revealed that the water of the Ismailia Canal is safe for both long- and short-term irrigation uses in the two seasons except Mo and Se in summer season. Mo exceeds the recommended limit for long-term use but is safe for short-term use, while Se exceeds the recommended limits for both long- and short-term uses (NAS/NAE, 1972; Pratt, 1972; Ayers and Westcot, 1985; Davis and Dewiest, 1966; Puntamkar et al., 1988; Yasmin et al., 2019; Rowe and Abdel-Magid, 1995; Fipps, 2015; Gupta and Gupta, 2003; 2015).
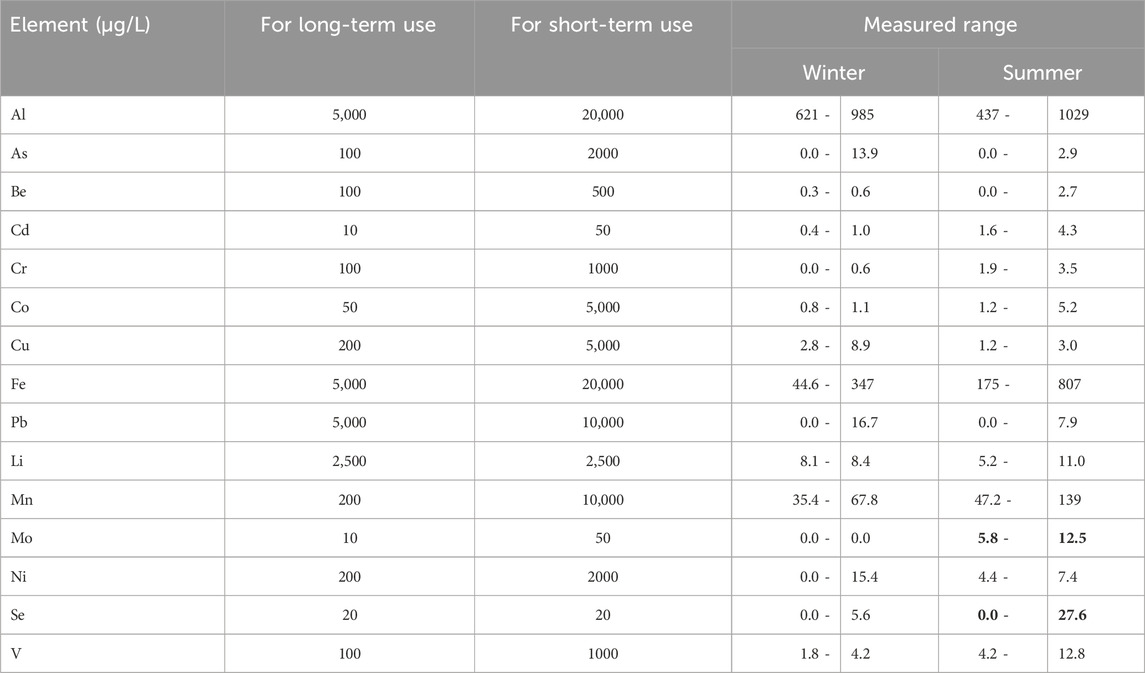
Table 5. Recommended maximum concentrations of trace elements in irrigation water. The bold parameters’ ranges exceeded their equivalent Recommended maximum concentrations of trace elements in irrigation water for long-term use.
5.2 Pollution index of metals (PIm)
Pollution indices of metals (PIm) are calculated to estimate the pollution effect of each metal on the Ismailia Canal water quality for drinking and irrigation purposes in both winter and summer seasons. Table 6 presents the results of the PIm. Based on WHO (2017) guidelines for trace elements in drinking water (Table 4), the canal water is strongly affected by Al and Tl in both winter and summer seasons. Additionally, it is slightly affected by As and Pb in the winter season, and by Cd and Fe in the summer season.
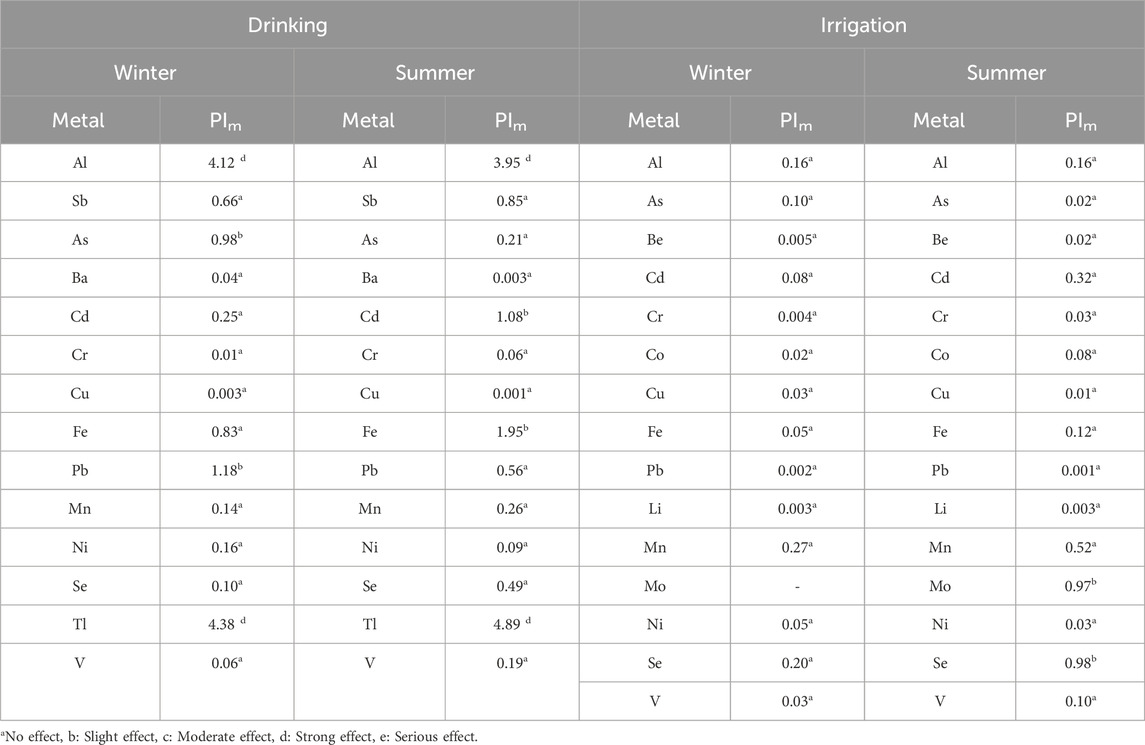
Table 6. Pollution indices of metals in the cluster groups of groundwater samples according to the WHO (2017) for drinking water.
For irrigation purposes, based on the recommended maximum concentrations of trace elements for long-term use in irrigation water (Table 5) adapted from the National Academy of Sciences and the National Academy of Engineering (NAS/NAE, 1972) and Pratt (1972), the elements have no significant effect on the canal water quality in the winter season. However, in the summer season, the canal water is slightly affected by Mo and Se.
5.3 Seasonal variation in water chemistry
The presented results reveal that the canal water is slightly alkaline, with pH values within the standard limits for freshwater (WHO, 1993). Based on the obtained TH values, the canal water has moderate hardness (Dhok et al., 2013; Gebresilasie et al., 2021; McGowan, 2000; Ramya et al., 2015; Rout and Sharma, 2011; Singh et al., 2021; Kothari et al., 2021). Salinity is low in both seasons but higher in winter than in summer. All major ions showed relatively higher concentrations in winter compered to summer. This is could be attributed to the interaction between the low level canal water and groundwater during winter especially during the drought period from January to February. During summer, canal water level increased due to receiving large amount of Nile water which leads to an inverse relationship with groundwater, which results in reducing the salinity of the canal water (Salem et al., 2019; Abdo and El-Nasharty, 2010; Elhaddad, 2014; Yusuf et al., 2018; Abdel-Satar et al., 2017; Islam et al., 2015). Unlike other ions, NO₃ has higher concentrations in summer, likely due to increased irrigation practices and the canal receives more irrigation waste water (Geriesh et al., 2004).
Seasonal variations in trace element concentrations might be due to natural processes like water-rock interactions and human activities such as agriculture, industrial activities, urbanization, and road maintenance (Geriesh, 1994; Geriesh and El-Rayes, 2001; Ghodeif et al., 2011; Geriesh et al., 2008; Stahl et al., 2009). Fluctuating pH might alter the chemical state of many pollutants, changing their solubility and transport between canal water and sediments (USEPA, 2021).
Correlation analyses (Tables 2 and 3) show varying correlation strengths and directions between the physicochemical parameters of the canal water in winter and summer. In winter, pH strongly correlates with SO₄ and CO, in contrast, pH weakly correlates with SO₄ and very strongly negatively with CO. The relationship between pH and HCO₃ remains strong and negative in both seasons. Variations in Al, As, Cd, Co, Cu, and V concentrations might be associated with the changes in pH, which revealed from the strong negative correlations between pH and these elements (Algül and Beyhan, 2020). K, Na, and Cl ions are the most effective in determining canal water salinity in winter, as shown by strong correlations with TDS. However, Na, Ca, HCO₃, CO₃, and NO₃ have the major effect on water salinity during summer as indicated by strong correlations with TDS.
Piper and Gibbs diagrams (Figure 10; Figure 11) indicated that the canal water is mainly of the Ca(Mg)-HCO₃ type in both seasons, with mixed types in some summer samples. Water-rock interaction is the major process affecting canal water chemistry.
5.4 Anthropogenic contamination sources
The Ismailia Canal passes through heavily populated areas, making it susceptible to domestic and industrial discharges. Rainwater drainage can also contribute to metal and organic material accumulation in the canal water. Roads surrounding the canal represent another potential contamination source. Natural sources of metals in water primarily include mineral weathering (Klavinš et al., 2000). Industrial effluents, non-point pollution sources, and changes in atmospheric precipitation can locally increase metal concentrations. Airborne contaminants may deposit directly into the canal water or wash into it after deposition on land (Abdo and El-Nasharty, 2010; NISC, 2005). The most abundant sources of metals are anthropogenic, related to agricultural and industrial activities (Jaskuła et al., 2021; Cymes et al., 2017; Nguyen et al., 2020).
Cluster analysis confirms that TDS, ions, and trace elements form the same four clusters in both winter and summer (Figure 9). HCO₃, representing a separate cluster, is mainly produced by organic matter decomposition. The grouping of NO₃ and trace elements in one cluster indicates the impact of human activities (Salem et al., 2019; Abdo and El-Nasharty, 2010; Yusuf et al., 2018).
5.5 Water quality assessment for drinking and irrigation
Based on WHO (2017) drinking water standard and WQI, chemically Ismailia Canal water is suitable for drinking and most measured parameters are within limits in both seasons, except for Al, As, Fe, Pb, and Tl, which exceed limits in winter, and Al, Sb, Cd, Fe, and Tl, which exceed limits in summer (Table 4). The canal water is strongly affected by Al and Tl in both seasons and slightly affected by As and Pb in winter, and by Cd and Fe in summer, as indicated by the pollution indices of metals (Table 6; Figure 13).
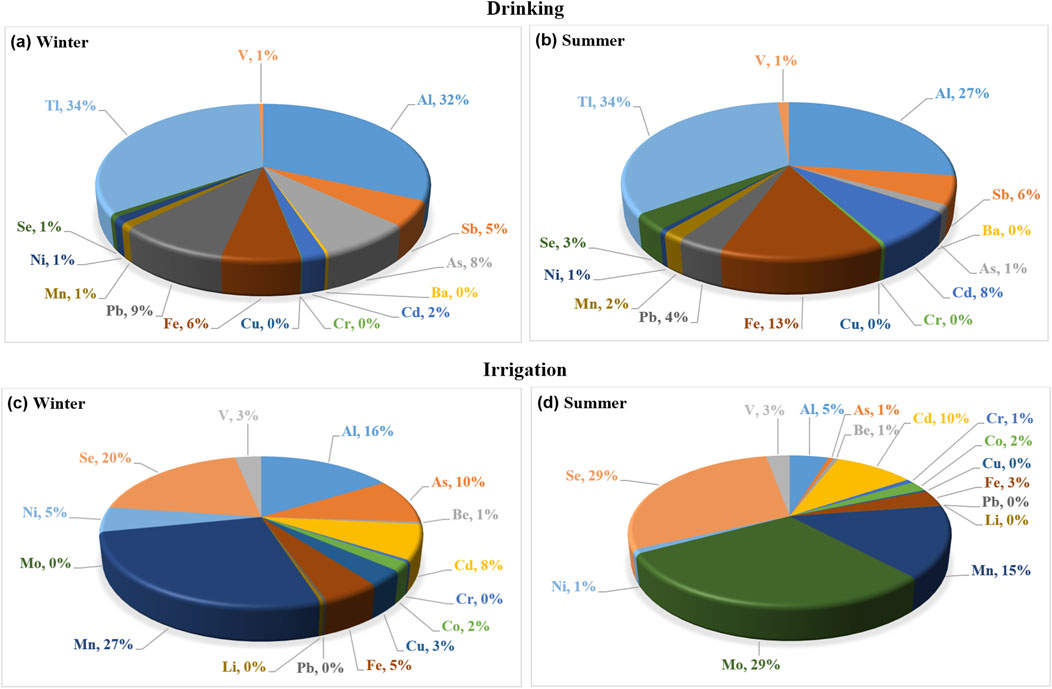
Figure 13. The relative ratios of PIm of the trace elements in Ismailia canal water, in both winter (A, C) and summer (B, D) seasons, based on the guidelines of trace elements for drinking and irrigation uses.
Heavy Metals are defined as metal and metalloid elements and they are one of the main environmental hazards to be concerned about. According to WHO, the majority of heavy metals are hazardous and pose a risk to public health (Csata et al., 1968). Cr, Cd, Ni, and As are classified as carcinogens among the hazardous heavy metals found in surface water (Aendo et al., 2022). TL can cause both acute and chronic poisoning (Liu et al., 2018). The potential hazard risk of Cu to aquatic creatures is the highest ranking (Donnachie et al., 2014). Zn presents more health concerns through the water-food chain and has a large capacity for bioaccumulation (Cui et al., 2021). Co is considered potentially harmful metal because of their toxicity and effects on the ecosystem (Cui et al., 2021). Fe is necessary for humans’ biological systems to function normally (Gülay et al., 2018). Fe deficiency is the root cause of anemia. Conversely, too much iron is bad for people. Haemochromatosis, which results from excessive Fe consumption, damages tissue because of Fe accumulation. Fe poisoning in humans initially manifests as intestinal damage, diarrhea, and vomiting (Smith et al., 2017). Al is also a suspected causative agent of neurological disorders such as Alzheimer’s disease and presenile dementia.
For irrigation purposes, all trace elements are within the recommended maximum concentrations for long- and short-term irrigation, except Mo and Se, which exceed recommended concentrations for long-term use (Table 5). The concerned elements have no effect on canal water quality for irrigation in winter, but in summer, the water is slightly affected by Mo and Se (Table 6; Figure 13).
5.6 Sustainability and environmental impact
The findings from the Ismailia Canal study have significant implications for sustainability and environmental management, both locally and globally. Water quality is a critical factor in maintaining sustainable ecosystems and ensuring the health and wellbeing of human populations (e.g., Keeler et al., 2012; Bunch et al., 2011). This study’s insights into the seasonal variations in water chemistry and the identification of pollution sources are crucial for developing effective water management strategies.
The Ismailia Canal serves as a vital freshwater resource for the eastern Nile Delta, supporting agricultural, industrial, and domestic needs (Geriesh et al., 2008; Goher et al., 2014; Geriesh, 1994; Geriesh and El-Rayes, 2001; Ghodeif et al., 2011; Stahl and Ramadan, 2008). Ensuring the canal’s water quality is essential for sustainable water management. By identifying key pollutants such as Al, Sb, As, Cd, Fe, Pb, and Tl, and understanding their seasonal variations, this study provides a foundation for targeted mitigation efforts. Implementing measures to reduce these contaminants can improve water quality and ensure the long-term availability of clean water for various uses.
The presence of heavy metals and other pollutants in the canal poses significant risks to both aquatic ecosystems and human health. Metals like Al, Pb, and Cd are known to have toxic effects on aquatic life (e.g., Okereafor et al., 2020; Baby et al., 2010), which can disrupt ecosystems and reduce biodiversity. By addressing the sources of these pollutants, such as industrial discharges and agricultural runoff, we can protect the health of aquatic ecosystems. This is particularly important in regions like the Nile Delta, where water bodies are integral to local biodiversity (e.g., Abdel-Raheem et al., 2024; Redeker and Kantoush, 2014). Water pollution is a global issue, and the challenges faced by the Ismailia Canal are reflective of broader trends seen in many parts of the world. Rapid industrialization, urbanization, and agricultural expansion contribute to the degradation of water quality in numerous regions (e.g., Akhtar et al., 2021; Zhang et al., 2019; Ren et al., 2014). Studies like this one provide valuable data that can be used to inform global water management policies. By sharing knowledge and strategies, regions facing similar challenges can develop more effective solutions to combat water pollution and promote sustainable practices.
Climate change exacerbates the challenges of maintaining water quality by altering precipitation patterns (e.g., Murdoch et al., 2000; Whitehead et al., 2009), increasing the frequency of extreme weather events, and affecting water availability. The seasonal data from the Ismailia Canal highlight the importance of adaptive management strategies that can respond to these changes. Understanding how water chemistry varies with seasons allows for the development of flexible water management plans that can better cope with the impacts of climate change.
Establishing a continuous monitoring program for the Ismailia Canal will help track changes in water quality over time and assess the effectiveness of implemented mitigation measures. This will also provide data to anticipate and respond to emerging threats. Efforts should be made to control pollution at its source, particularly targeting industrial effluents and agricultural runoff. Implementing stricter regulations and promoting best practices in agriculture and industry can significantly reduce the input of harmful contaminants into the canal.
This study of the Ismailia Canal underscores the importance of monitoring and managing water quality to ensure sustainability and protect environmental health. By addressing the challenges identified and implementing recommended actions, we can contribute to a healthier, more sustainable future for the Nile Delta region and beyond.
6 Conclusion
The Ismailia Canal is a crucial surface freshwater source for various purposes in the eastern Nile Delta region. However, the canal is at risk from unwise direct and indirect activities in the surrounding environments. The canal water is slightly alkaline and moderately hard, with higher salinity in winter than in summer. Major cations and anions have relatively higher concentrations in winter, while NO₃ is higher in summer. The canal water is primarily of the Ca(Mg)-HCO₃ type, mainly influenced by rock-water interactions. The water chemistry suggests that the canal is not solely controlled by rock weathering or evaporation.
Based on WHO (2017) drinking water quality standards, most physicochemical parameters are within the standard limits, except for Al, Sb, As, Cd, Fe, Pb, and Tl. The water is strongly affected by Al and Tl in both seasons, slightly affected by As and Pb in winter, and slightly affected by Cd and Fe in summer. For irrigation purposes, all trace elements are within the recommended maximum concentrations for long- and short-term use in winter. However, in summer, Mo and Se slightly affect the water quality for long-term use.
To reduce the serious surface water pollution status and maintain environmental sustainability, it is crucial to replan the waste water discharge system of the residential, industrial, and agricultural activities along the canal banks. In order to safeguard the environment and the health of the many populations in the area, it is advised that they receive further education and communication training. In addition, the government must keep a close eye on the canal’s water pollution. Encouraging the local people, industrial owner as well as others, to apply sustainable wastewater treatment systems to reduce canal water pollution and raising awareness of local stakeholders of the potential of nature-based water treatment systems to contribute to addressing critical sustainability challenges is essential.
Data availability statement
The datasets presented in this study can be found in online repository. Readers can access the repository from: http://abualam.info/salem_etal_2024.
Author contributions
ZE-SS: Conceptualization, Data curation, Formal Analysis, Investigation, Methodology, Project administration, Resources, Software, Supervision, Validation, Visualization, Writing–original draft, Writing–review and editing. AA: Data curation, Formal Analysis, Investigation, Methodology, Project administration, Resources, Software, Validation, Visualization, Writing–original draft. TA-A: Funding acquisition, Validation, Visualization, Writing–review and editing.
Funding
The author(s) declare that financial support was received for the research, authorship, and/or publication of this article. This paper received funding from the Open Access publishing fund from UiT the Arctic University of Norway.
Acknowledgments
We would like to extend our sincere gratitude to Paweł Tomczyk for his invaluable editorial guidance, as well as to our reviewers, Venkatramanan Senapathi, Jun Xiao, and Predrag Ilić, for their insightful comments and constructive feedback. Their expertise and attention to detail greatly contributed to improving the quality of this work.
Conflict of interest
The authors declare that the research was conducted in the absence of any commercial or financial relationships that could be construed as a potential conflict of interest.
Publisher’s note
All claims expressed in this article are solely those of the authors and do not necessarily represent those of their affiliated organizations, or those of the publisher, the editors and the reviewers. Any product that may be evaluated in this article, or claim that may be made by its manufacturer, is not guaranteed or endorsed by the publisher.
References
Abd El-Hady, H. H., and Hussian, A. M. (2012). Regional and seasonal variation of phytoplankton assemblages and its biochemical analysis in Ismailia canal, River Nile, Egypt. J. Appl. Sci. Res. 8 (7), 3433–3447.
Abdel-Raheem, K. H., Khalil, M. M., Abdelhady, A. A., and Tan, L. (2024). Anthropogenic–induced environmental and ecological changes in the Nile Delta over the past half-century. Sci. Total Environ. 926, 171941. doi:10.1016/j.scitotenv.2024.171941
Abdel-Satar, A. M., Ali, M. H., and Goher, M. E. (2017). Indices of water quality and metal pollution of Nile River, Egypt. The Egyptian Journal of aquatic research. Egypt. J. Aquat. Res. 43 (1), 21–29. doi:10.1016/j.ejar.2016.12.006
Abdo, M. H. (2013). Impact of drought period on water quality and trace metals distributions in water and sediment of Ismailia canal, River Nile, Egypt. Life Sci. J. 10 (4), 485–492.
Abdo, M. H., and El-Nasharty, S. M. (2010). Physico-chemical evaluations and trace metals distribution in water-surficial sediment of Ismailia Canal, Egypt. J. Nat. Sci. 8 (5), 198–206.
Abdo, M. H., Goher, M. E., and Sayed, M. F. (2012). Environmental evaluation of Ismailia Canal water and sediment, Egypt. J. Egypt. Acad. Soc. Environ. Dev. 13 (2), 61–78.
Abdo, M. H., Sabae, S. Z., Haroon, B. M., Refaat, B. M., and Mohammed, A. S. (2010). Physico-chemical characteristics, microbial assessment and antibiotic susceptibility of pathogenic bacteria of Ismailia Canal Water, River Nile, Egypt. J. Am. Sci. 6 (5), 234–250.
Aendo, P., De Garine-Wichatitsky, M., Mingkhwan, R., Senachai, K., Santativongchai, P., Krajanglikit, P., et al. (2022). Potential health effects of heavy metals and carcinogenic health risk estimation of Pb and Cd contaminated eggs from a closed gold mine area in Northern Thailand. Foods (Basel, Switzerland) 11 (18), 2791. doi:10.3390/foods11182791
Akhtar, N., Syakir Ishak, M. I., Bhawani, S. A., and Umar, K. (2021). Various natural and anthropogenic factors responsible for water quality degradation: a review. Water 13 (19), 2660. doi:10.3390/w13192660
Algül, F., and Beyhan, M. (2020). Concentrations and sources of heavy metals in shallow sediments in Lake Bafa, Turkey. Sci. Rep. 10, 11782. doi:10.1038/s41598-020-68833-2
APHA/AWWA/WEF (2017). Standard methods for the examination of water and wastewater. 23nd Edition. Washington DC. USA: American Public Health Association/American Water Works Association/Water Environment Federation. doi:10.2105/SMWW.2882.219
Armah, F. A., Obiri, S., Yawson, D. O., Onumah, E. E., Yengoh, G. T., Afrifa, E. K., et al. (2010). Anthropogenic sources and environmentally relevant concentrations of heavy metals in surface water of a mining district in Ghana: a multivariate statistical approach. J. Environ. Sci. Health Part A 45, 1804–1813. doi:10.1080/10934529.2010.513296
Ayers, R. S., and Westcot, D. W. (1985). “Water quality for agriculture,” in FAO irrigation and drainage paper 29 rev. 1. Rome: FAO.
Baby, J., Raj, J. S., Biby, E. T., Sankarganesh, P., Jeevitha, M. V., Ajisha, S. U., et al. (2010). Toxic effect of heavy metals on aquatic environment. Int. J. Biol. Chem. Sci. 4 (4), 939–952. doi:10.4314/ijbcs.v4i4.62976
Beldjazia, A., and Alatou, D. (2016). Precipitation variability on the massif forest of mahouna (north eastern-Algeria) from 1986 to 2010. Int. J. Manag. Sci. Bus. Res. 5 (3), 21–28.
Bunch, M. J., Morrison, K. E., Parkes, M. W., and Venema, H. D. (2011). Promoting health and well-being by managing for social–ecological resilience: the potential of integrating ecohealth and water resources management approaches. Ecol. Soc. 16 (1), 6. doi:10.5751/es-03803-160106
Chai, N., Zhao, Z., Li, X., Xiao, J., and Jin, Z. (2024). Chemical weathering processes in the Chinese Loess Plateau. Geosci. Front. 15, 101842. doi:10.1016/j.gsf.2024.101842
Cheng, B. F., Zhang, Y., Xia, R., Wang, L., Zhang, N., and Zhang, X. F. (2021). Spatiotemporal analysis and prediction of water quality in the Han River by an integrated nonparametric diagnosis approach. J. Clean. Prod. 328, 129583. doi:10.1016/j.jclepro.2021.129583
Csata, S., Gallay, S. F., and Toth, M. (1968). Akute niereninsuffizienz als folge einer zinkchlorid ver giftung. Z. Urol. 61, 327–330.
Cui, L., Wang, X., Li, J., Gao, X., Zhang, J., and Liu, Z. (2021). Ecological and health risk assessments and water quality criteria of heavy metals in the Haihe River. Environ. Poll. 290, 117971. doi:10.1016/j.envpol.2021.117971
Cymes, I., Glińska-Lewczuk, K., Szymczyk, S., Sidoruk, M., and Kuriata-Potasznik, A. (2017). Distribution and potential risk assessment of heavy metals and arsenic in sediments of a dam reservoir: a case study of the Łoje retention reservoir, NE Poland. J. Elem. 22 (3), 843–856. doi:10.5601/jelem.2017.22.1.1253
Dhok, R. P., Patil, A. S., and Ghole, V. S. (2013). Hardness of groundwater resources and its suitability for drinking purpose. Int. J. Pharm. Chem. Sci. 2 (1), 169–172.
Dieter, C. A., Maupin, M. A., Caldwell, R. R., Harris, M. A., Ivahnenko, T. I., Lovelace, J. K., et al. (2017). Estimated use of water in the United States in 2015. U.S. Geol. Surv. Circ. 1441, 65p. doi:10.3133/cir1441
Doležalová, W. H., Mihočová, S., Chovanec, P., and Pavlovský, J. (2019). Potential ecological risk and human health risk assessment of heavy metal pollution in industrial affected soils by coal mining and metallurgy in Ostrava, Czech Republic. Int. J. Environ. Res. Public Health. 16 (22), 4495. doi:10.3390/ijerph16224495
Donnachie, R. L., Johnson, A. C., Moeckel, C., Pereira, M. G., and Sumpter, J. P. (2014). Using risk-ranking of metals to identify which poses the greatest threat to freshwater organisms in the UK. Environ. Pollut. 194, 17–23. doi:10.1016/j.envpol.2014.07.008
El-Amier, Y. A., Kotb, W. K., Bonanomi, G., Fakhry, H., Marraiki, N. A., and Abd-ElGawad, A. M. (2021). Hydrochemical assessment of the irrigation water quality of the El-Salam Canal, Egypt. Water 13, 2428. doi:10.3390/w13172428
El-Battrawy, O. A., Hasballah, A. F., and El-Gohary, H. A. (2022). Assessment of surface water quality of the Nile River at Damietta branch, Egypt. Sci. J. Damietta Fac. Sci. 13 (2), 1–9.
Elhaddad, E. (2014). Effects of pollution on hydro-chemical and water quality assessment of the Ismailia Canal water, Egypt. Int. J. Pure App. Biosci. 2 (5), 124–131.
El-Sayed, S. A. (2008). Microbiological studies on Ismailia canal, River Nile, Egypt (M.Sci.Thesis). Egypt: Fac. of Sci., Al-Azhar Univ.
Elshobary, M. E., Essa, D. I., Attiah, A. M., Salem, Z. E., and Qi, X. (2020). Algal community and pollution indicators for the assessment of water quality of Ismailia canal, Egypt. Stoch. Environ. Res. Risk Assess. 34 (7), 1089–1103. doi:10.1007/s00477-020-01809-w
Evans, J. D. (1996). Straightforward statistics for the behavioral sciences. Pacific Grove, Calif: Brooks/Cole Publishing, 600.
Fipps, G. (2015). Irrigation water quality standards and salinity management strategies. Agri life extension Texas A&M system. B- 1667, 4–03.
Fu, X. W., Wu, R. B., Qi, H. Y., and Yin, H. L. (2023). Long-term trends in surface water quality of China’s seven major basins based on water quality identification index and big data analysis. Environ. Impact Asses. 100, 107090. doi:10.1016/j.eiar.2023.107090
Gao, Y., Qian, H., Ren, W., Wang, H., Liu, F., and Yang, F. (2020). Hydrogeochemical characterization and quality assessment of groundwater based on integrated-weight water quality index in a concentrated urban area. J. Clean. Prod. 260, 121006. doi:10.1016/j.jclepro.2020.121006
Gebresilasie, K. G., Berhe, G. G., Tesfay, A. H., and Gebre, S. E. (2021). Assessment of some physicochemical parameters and heavy metals in hand-dug well water samples of kafta humera woreda, tigray, Ethiopia. Int. J. Anal. Chem. 8867507, 1–9. doi:10.1155/2021/8867507
Geriesh, M. H. (1994). Hydrogeological and hydrogeochemical evaluation for the groundwater resources in the Suez Canal region, Egypt. Ismailia, Egypt: Suez Canal University, Faculty of Science. Ph.D. Thesis.
Geriesh, M. H., Balke, K. D., and El-Rayes, A. E. (2008). Problems of drinking water treatment along Ismailia canal province, Egypt. J. Zhejiang Univ. Sci. B 9 (3), 232–242. doi:10.1631/jzus.b0710634
Geriesh, M. H., and El-Rayes, A. E. (2001). “Municipal contamination of shallow groundwater beneath south Ismailia villages,” in 5Th Inter. Conf. On geochemistry. Alex. Univ. (Egypt), 241–253. doi:10.6084/m9.figshare.16732300
Geriesh, M. H., Stueben, D., Berner, Z., and Ibraheim, M. (2004). “Deficiencies of simple technologies in surface water purification: a case study of surface water treatment for drinking purposes at Suez city, Egypt,” in The 7thinternational Conference of Geology of arab world (GAW 7). Cairo, Egypt, 429–437.
Ghobara, M., and Salem, Z. E. (2017). “Spatiotemporal fluctuations in phytoplankton communities and their potential indications for the pollution status of the irrigation and drainage water in the Middle Nile Delta Area, Egypt,” in The handbook of environmental chemistry (conventional water resources and agricultural in Egypt, 2019) (Berlin, Heidelberg: Springer).
Ghodeif, K. O., Arnous, M. O., and Geriesh, M. H. (2011). Define a protected buffer zone for Ismailia canal, Egypt using geographic information systems. Arab. J. Geosci. 6 (1), 43–53. doi:10.1007/s12517-011-0326-3
Gibbs, R. J. (1970). Mechanisms controlling world water chemistry. Science 17, 1088–1090. doi:10.1126/science.170.3962.1088
Goher, M. E., Hassan, A. M., Abdel-Moniem, I. A., Fahmy, A. H., and El-sayed, S. M. (2014). Evaluation of surface water quality and heavy metal indices of Ismailia Canal, Nile River, Egypt. Egypt. Egypt. J. Aquatic Res. 40 (3), 225–233. doi:10.1016/j.ejar.2014.09.001
Gülay, A., Çekiç, Y., Musovic, S., Albrechtsen, H. J., and Smets, B. F. (2018). Diversity of iron oxidizers in groundwater-fed rapid sand filters: evidence of Fe(II)-Dependent growth by curvibacter and undibacterium spp. Front. Microbiol. 9, 2808. doi:10.3389/fmicb.2018.02808
Gupta, I. C., and Gupta, S. K. (2003). Use of Saline Water in Agriculture. A study of arid and semi-arid zones of India. 3rd edition. Jodhpur, India: Scientific Publishers.
Gupta, S. K., and Gupta, I. C. (2015). Management of saline and waste water in agriculture. Jodhpur, India: Scientific Publishers.
Hammer, Ø., Harper, D. A. T., and Ryan, P. D. (2001). Past: paleontological statistics software package for education and data analysis. Palaeontol. Electron. 4 (1), 9pp.
He, Z., Li, F., Dominech, S., Wen, X., and Yang, S. (2019). Heavy metals of surface sediments in the Changjiang (Yangtze River) Estuary: distribution, speciation and environmental risks. J. Geochem. Explor. 198, 18–28. doi:10.1016/j.gexplo.2018.12.015
Herzog, C. M., Dey, S., Hablas, A., Khaled, H. M., Seifeldin, I. A., Ramadan, M., et al. (2012). Geographic distribution of hematopoietic cancers in the Nile delta of Egypt. Ann. Oncol. Official J. Eur. Soc. Med. Oncol./ESMO 23 (10), 2748–2755. doi:10.1093/annonc/mds079
Ibrahim, H. S., Ibrahim, M. A., and Samhan, F. A. (2009). Distribution and bacterial bioavailability of selected metals in sediments of Ismailia Canal, Egypt. J. Hazard. Mater. 168 (2-3), 1012–1016. doi:10.1016/j.jhazmat.2009.02.132
Islam, A., Rakib, M. A., Islam, M. S., Jahan, K., and Patwary, M. A. (2015). Assessment of health hazard of metal concentration in groundwater of Bangladesh. Am. Chem. Sci. J. 5 (1), 41–49. doi:10.9734/ACSj/2015/13175
Jaskuła, J., Sojka, M., Fiedler, M., and Wróżyński, R. (2021). Analysis of spatial variability of river bottom sediment pollution with heavy metals and assessment of potential ecological hazard for the warta river, Poland. Minerals 11 (3), 327. doi:10.3390/min11030327
Keeler, B. L., Polasky, S., Brauman, K. A., Johnson, K. A., Finlay, J. C., O’Neill, A., et al. (2012). Linking water quality and well-being for improved assessment and valuation of ecosystem services. Proc. Natl. Acad. Sci. 109 (45), 18619–18624. doi:10.1073/pnas.1215991109
Khalifa, N. (2014). Population dynamics of rotifera in Ismailia canal, Egypt. J. Biodivers. Environ. Sci. (JBES). 4 (2), 58–67.
Khalil, M., Sayed, M., Amer, A., and Nassif, M. (2012). Impact of pollution on macroinvertebrates biodiversity in Ismailia Canal, Egypt. Egypt. J. Aquatic Biol. Fish. 16 (4), 69–89. doi:10.21608/ejabf.2012.2143
Klavinš, M., Briede, A., Rodinov, V., Kokorite, I., Parele, E., and Klavina, I. (2000). Heavy metals in rivers of Latvia. Sci. Total Environ. 262 (2), 175–183. doi:10.1016/s0048-9697(00)00597-0
Kothari, V., Vij, S., Sharma, S., and Gupta, N. (2021). Correlation of various water quality parameters and water quality index of districts of Uttarakhand. Environ. Sustain. Indic. 9, 100093. doi:10.1016/j.indic.2020.100093
Li, X., Xiao, J., Chai, N., and Jin, Z. (2023). Controlling mechanism and water quality assessment of arsenic in China’s Yellow River Basin. J. Clean. Prod. 418, 137953. doi:10.1016/j.jclepro.2023.137953
Liu, L., Zhang, L., Pinghao, Y. E., and Liu, Q. (2018). Influencing factors of university students’ use of social network sites: an empirical analysis in China. IJET 13 (3), 71–86. doi:10.3991/ijet.v13i03.8380
Lu, Y., Tang, C., Chen, J., and Chen, J. (2015). Groundwater recharge and hydrogeochemical evolution in leizhou peninsula, China. J. Chem. 2015, 1–12. doi:10.1155/2015/427579
Mandeng, E. P. B., Bidjeck, L. M. B., Bessa, A. Z. E., Ntomb, Y. D., Wadjou, J. W., Doumo, E. P. E., et al. (2019). Contamination and risk assessment of heavy metals, and uranium of sediments in two watersheds in Abiete-Toko gold district, Southern Cameroon. Heliyon 5 (10), 02591. doi:10.1016/j.heliyon.2019.e02591
McGowan, W. (2000). Water processing: residential, commercial, light-industrial. 3rd Edn. Lisle, IL: Water Quality Association.
McSeveny, A., Conway, R., Wilkes, S., and Smith, M. (2009). Guideline for interpreting correlation coefficient by Ith phanny to accompany: international mathematics for the middle year 5. Pearson Australia ITH PHANNY.
Murdoch, P. S., Baron, J. S., and Miller, T. L. (2000). Potential effects of climate change on surface-water quality in North America 1. JAWRA J. Am. Water Resour. Assoc. 36 (2), 347–366. doi:10.1111/j.1752-1688.2000.tb04273.x
NAS/NAE (1972). “Water quality criteria,” in A report of the committee on water quality criteria. Environmental studies board, national Academy of sciences, national Academy of engineering. Washington DC: The Environmental Protection Agency. Report No. EPA-R3-73-033. APA 2170.
Nawrot, N., Wojciechowska, E., Rezania, S., Walkusz-Miotk, J., and Pazdro, K. (2020). The effects of urban vehicle traffic on heavy metal contamination in road sweeping waste and bottom sediments of retention tanks. Sci. Total Environ. 749, 141511. doi:10.1016/j.scitotenv.2020.141511
Nguyen, B. T., Do, D. D., Nguyen, T. X., Nguyen, V. N., Phuc Nguyen, D. T., Nguyen, M. H., et al. (2020). Seasonal, spatial variation, and pollution sources of heavy metals in the sediment of the Saigon River, Vietnam. Environ. Pollut. 256, 113412. doi:10.1016/j.envpol.2019.113412
NISC (2005). “Five year review of Executive Order 13112 on invasive species,” in National invasive species council. Washington, DC: U.S. Department of the Interior.
Okereafor, U., Makhatha, M., Mekuto, L., Uche-Okereafor, N., Sebola, T., and Mavumengwana, V. (2020). Toxic metal implications on agricultural soils, plants, animals, aquatic life and human health. Int. J. Environ. Res. public health 17 (7), 2204. doi:10.3390/ijerph17072204
Piper, A. M. (1994). Graphical interpretation of water analysis. Trans. Am. Geophys. Union 25, 914–928.
Pratt, P. F. (1972). Quality criteria for trace elements in irrigation waters. Calif. Agric. Exp. Stn., 46.
Puntamkar, S., Kant, K., and Mathur, S. (1988). The effect of saline water irrigation on soil properties. Trans- action Indian Soc. Dexter Technol. Krishi Bhawan, Bikaner, Rajasthan, India 15, 69–72.
Ramya, P., Babu, A. J., Reddy, E. T., and Rao, L. V. (2015). A study on the estimation of hardness in ground water samples by edta tritrimetric method. Int. J. Recent Sci. Res. 6 (6), 4505–4507.
Redeker, C., and Kantoush, S. A. (2014). The Nile Delta: urbanizing on diminishing resources. Built Environ. 40 (2), 201–212. doi:10.2148/benv.40.2.201
Ren, L., Cui, E., and Sun, H. (2014). Temporal and spatial variations in the relationship between urbanization and water quality. Environ. Sci. Pollut. Res. 21, 13646–13655. doi:10.1007/s11356-014-3242-8
Rout, C., and Sharma, A. (2011). Assessment of drinking water quality: a case study of ambala cantonment area, Haryana, India. Int. J. Environ. Sci. 2 (2), 933–945.
Rowe, D. R., and Abdel-Magid, I. M. (1995). Handbook of wastewater reclamation and reuse. Boca Raton, FL: CRC Press, 550pp.
Salem, Z. E., El Nahrawy, A., and Ghobara, M. (2019). Spatiotemporal hydrochemical evaluation and quality assessment of drainage water compared to canal surface water in the middle nile delta, Egypt. Hdb. Env. Chem. 75, 123–162. doi:10.1007/698_2018_296
Salem, Z. E., Elsaiedy, G., and El Nahrawy, A. (2017b). “Assessment of the groundwater quality for drinking and irrigation purposes in the central Nile Delta Region, Egypt,” in The handbook of environmental chemistry (groundwater in the nile Delta, 2019) (Berlin, Heidelberg: Springer).
Salem, Z. E., Elsaiedy, G., and El Nahrawy, A. (2017c). “Hydrogeochemistry and quality assessment of groundwater under some central Nile Delta villages, Egypt,” in The handbook of environmental chemistr, springer (groundwater in the nile Delta, 2019) (Berlin, Heidelberg).
Salem, Z. E., Fathy, M. S., Helal, A.-F. I., Afifi, S. Y., and Attiah, A. M. (2020). Use of subsurface temperature as a groundwater flow tracer in the environs of Ismailia Canal, Eastern Nile Delta, Egypt. Arabian J. Geosciences 13 (13), 503. doi:10.1007/s12517-020-05518-0
Salem, Z. E., Fathy, M. S., Helal, A. I., Afifi, S. Y., and Attiah, A. M. (2021). “Groundwater quality for irrigation as an aspect of sustainable development approaches: a case study of semi-arid area around Ismailia canal, eastern nile delta, Egypt,” in Groundwater in Egypt’s deserts. Editors A. Negm, and A. Elkhouly (Springer Water). doi:10.1007/978-3-030-77622-0_12
Salem, Z. E., Ghobara, M., and El Nahrawy, A. (2017a). Spatio-temporal evaluation of the surface water quality in the middle Nile Delta using Palmer’s algal pollution index. Egypt. J. Basic Appl. Sci. 4, 219–226. doi:10.1016/j.ejbas.2017.05.003
Singh, S., Parashar, V., Kalyanasundaram, M., Khare, S. R., Purohit, M., Stålsby Lundborg, C., et al. (2021). Physicochemical quality monitoring of groundwater for drinking purposes in rural Ujjain, Central India: findings of a 2-year longitudinal study. SN Appl. Sci. 3, 240. doi:10.1007/s42452-020-04125-8
Smith, M. R., Golden, C. D., and Myers, S. S. (2017). Potential rise in iron deficiency due to future anthropogenic carbon dioxide emissions. GeoHealth 1, 248–257. doi:10.1002/2016GH000018
Sojka, M., Jaskuła, J., and Siepak, M. (2019). Heavy metals in bottom sediments of reservoirs in the lowland area of western Poland: concentrations, distribution, sources and ecological risk. Water 11 (1), 56. doi:10.3390/w11010056
Stahl, R., and Ramadan, A. B. (2008). Environmental studies on water quality of the Ismailia canal, Egypt. Sci. Rep. Forschungszentrum Karlsruhe der Helmholtz-Gemeinschaft Wissenschaftliche Berichte FZKA 7427, 58.
Stahl, R., Ramadan, A. B., and Pimpl, M. (2009). Bahr el-baqar drain system/Egypt environmental studies on water quality Part I: bilbeis drain/bahr el-baqar drain. Forschungszentrum Karlsruhein Helmholtz-Gemeinschaft Wiss. Berichte FZKA7505, 72.
Stamatis, N., Kamidis, N., Pigada, P., Sylaios, G., and Koutrakis, E. (2019). Quality indicators and possible ecological risks of heavy metals in the sediments of three semi-closed East mediterranean gulfs. Toxics 7 (2), 30. doi:10.3390/toxics7020030
Sun, X., Fan, D., Liu, M., Tian, Y., Pang, Y., and Liao, H. (2018). Source identification, geochemical normalization and influence factors of heavy metals in Yangtze River Estuary sediment. Environ. Pollut. 241, 938–949. doi:10.1016/j.envpol.2018.05.050
Tarek, S. J., and Ali, A. O. (2007). The effect of industrial waste of oil companies on the distribution of organic pollution in Ismailia Canal water. Bull. Natl. Res. Cent. Egypt. 32 (6), 647–659.
UNESCO-MERIC (2003). Water for people, water for life. Executive summary of the world water development report. Arabic edition. Paris, France: UNESCO, 1–36.
USEPA (2008). EPA's report on the environment (roe), final report. Washington, D.C.: United States Environmental Protection Agency. EPA/600/R-07/045F (NTIS PB2008-112484).
USEPA (2021). Causal analysis/diagnosis decision information system (CADDIS). Sources, Stressors Responses 2.
Wang, Y. P., Wang, S., Zhao, W. W., and Liu, Y. X. (2022). The increasing contribution of potential evapotranspiration to severe droughts in the Yellow River basin. J. Hydrol. 605, 127310. doi:10.1016/j.jhydrol.2021.127310
Whitehead, P. G., Wilby, R. L., Battarbee, R. W., Kernan, M., and Wade, A. J. (2009). A review of the potential impacts of climate change on surface water quality. Hydrological Sci. J. 54 (1), 101–123. doi:10.1623/hysj.54.1.101
WHO (1993). Guidelines for drinking-water quality: volume 1: recommendations. 2nd ed. Geneva: World Health Organization.
WHO (2017). Guidelines for drinking-water quality: fourth edition incorporating the first addendum. Geneva: World Health Organization.
Xiao, J., Wang, L., Chai, N., Liu, T., Jin, Z., and Rinklebe, J. (2021). Groundwater hydrochemistry, source identification and pollution assessment in intensive industrial areas, eastern Chinese loess plateau. Environ. Poll. 278, 116930. doi:10.1016/j.envpol.2021.116930
Yan, Y. N., Wang, R., Chen, S., Zhang, Y., and Sun, Q. L. (2023). Three-dimensional agricultural water scarcity assessment based on water footprint: a study from a humid agricultural area in China. Sci. Total Environ. 857, 159407. doi:10.1016/.j.scitotenv.2022.159407
Yasmin, G., Islam, D., Islam, M., ShariotUllah, M., and Adham, A. (2019). Evaluation of groundwater quality for irrigation and drinking purposes in Barishal district of Bangladesh. Fundam. Appl. Agric. 4 (1), 632. doi:10.5455/faa.301258
Youssef, M., El-Taweel, G. E., El-Naggar, M., El-Hawary, S. E., El- Meleigy, M. A., and Ahmed, S. A. (2010). Hydrocarbon degrading bacteria as indicator of petroleum pollution in Ismailia Canal, Egypt. World Appl. Sci. J. 8 (10), 1226–1233.
Yusuf, A., Olasehinde, A., Mboringong, M. N., Tabale, R. P., and Daniel, E. P. (2018). Evaluation of heavy metals concentration in groundwater around Kashere and its environs, upper Benue trough, Northeastern Nigeria. Glob. J. Geol. Sci. 16, 25–36. doi:10.4314/gjgs.v16i1.4
Zhang, F., Huang, G., Hou, Q., Liu, C., Zhang, Y., and Zhang, Q. (2019). Groundwater quality in the Pearl River Delta after the rapid expansion of industrialization and urbanization: distributions, main impact indicators, and driving forces. J. Hydrology 577, 124004. doi:10.1016/j.jhydrol.2019.124004
Keywords: Nile Delta, water quality, Ismailia canal, environmental sustainability, trace elements, seasonal variation
Citation: El-Said Salem Z, Attiah AM and Abu-Alam T (2024) Hydrochemical characterization and sustainability assessment of Ismailia canal water, Eastern Nile Delta, Egypt: implications for human health and environmental safety. Front. Environ. Sci. 12:1475082. doi: 10.3389/fenvs.2024.1475082
Received: 02 August 2024; Accepted: 24 October 2024;
Published: 11 November 2024.
Edited by:
Paweł Tomczyk, Wroclaw University of Environmental and Life Sciences, PolandReviewed by:
Venkatramanan Senapathi, National College, Tiruchirappalli, IndiaJun Xiao, Chinese Academy of Sciences (CAS), China
Predrag Ilić, PSRI Institute for Protection and Ecology of the Republic of Srpska, Banja Luka, Bosnia and Herzegovina
Copyright © 2024 El-Said Salem, Attiah and Abu-Alam. This is an open-access article distributed under the terms of the Creative Commons Attribution License (CC BY). The use, distribution or reproduction in other forums is permitted, provided the original author(s) and the copyright owner(s) are credited and that the original publication in this journal is cited, in accordance with accepted academic practice. No use, distribution or reproduction is permitted which does not comply with these terms.
*Correspondence: Tamer Abu-Alam, tamer.abu-alam@uit.no