- 1Guizhou Provincial Key Laboratory for Protection of Ecological Environment, Guizhou Normal University, Guiyang, China
- 2School of Health Management, Guiyang Healthcare Vocational University, Guiyang, China
- 3Guizhou Provincial Engineering Research Center of Medical Resourceful Healthcare Products, Guiyang Healthcare Vocational University, Guiyang, China
Karst areas are often characterized by fragile ecological systems, and environmental pollution has increased the pressures on people living in such regions. This study aimed to investigate the status of pollution caused by heavy metals (Pb, Cd, Hg, As, and Cu) in soils based on different land uses in Changshun County, a karst area in southwestern China. Soil samples were collected from natural forestlands (NFLs), natural brush lands (NBLs), natural pasture lands (NPLs), artificial forestlands (AFLs), artificial brush lands (ABLs), and artificial pasture lands (APLs) for evaluation. The results suggest that the soil profile characteristics of the heavy metals studied herein vary significantly among different land uses. The heavy metal concentrations in all soil samples collected from NFLs were lower than those in samples from other land uses. Forest trees can protect soil from heavy metal pollution caused by atmospheric deposition; this is especially true for Hg. In cultivated forestlands and brush lands, special attention should be devoted to Cd pollution in the soil, which may be caused by the use of fertilizers. Changing both natural and artificial pastoral lands to forestlands could benefit the local ecosystems as it may reduce Hg contamination.
1 Introduction
Heavy metal pollution of soil is a significant environmental concern owing to its long-lasting impacts on the ecosystems and human health (Wei et al., 2023). The heavy metals present in soil affect plant growth, soil ecosystems, and human health through direct exposure as well as consumption of contaminated food (Shukla and Jain, 2022; Wen et al., 2022). Heavy metal pollution of soil has been a persistent global problem for over half a century and is an important threat to all life; for instance, some of the important causes underlying many diseases in humans, such as cancer, are attributable to heavy metal pollution (Milner and Kochian, 2008). This type of pollution arises from various human activities, such as industrial processes, mining, agriculture, and improper waste disposal (Shakoor et al., 2013; Huang et al., 2017). Industrial developments are profitable and have made life convenient; however, they also cause many environmental problems (Ma et al., 2024; Roy et al., 2014; Haddou et al., 2014; Rainio et al., 2015; Wang et al., 2015). When crops absorb and accumulate toxic metals from contaminated soils, they may harm animal and human health as these pollutants move up the food chain (Olawotin et al., 2012; Wang et al., 2020; Ahmed et al., 2013). Heavy metals like Cd can lead to cardiovascular diseases (Jin and Zhou, 2012); Pd can cause severe damage to the brain and central nervous system (Zhu et al., 2018); As can cause cancers (Yan and Zhang, 2021); Hg poses threats to the development of fetuses and young children (Clarkson et al., 2003); excess Cu in the environment may cause respiratory and digestive diseases (Geest et al., 2000).
The high geological background coupled with rocky desertification has led to prominent heavy metal soil pollution problems in the southwestern karst regions of China, which are important factors affecting the security of the ecological environments of these karst regions. There are large discrepancies in the soil heavy metal concentrations in karst mountain areas, and their impact factors are more complex than those in non-karst areas. Wang et al. (2021) noted that the levels of As, Cu, Pb, Cd, Cr, and Zn in the farmland soil around a Ni–Mo mining area in Guizhou were generally higher than the background values. Among these, Cd has been identified as a key factor contributing to soil heavy metal pollution. In addition, the Cd content in crops was between 0.02 and 1.28 mg kg-1, and Cd levels were highly enriched in chili peppers (Wang et al., 2020). Zhang et al. (2022a) indicated that the potential ecological hazards of heavy metals in farmland soil decreased in the order of Hg > Cd > As > Pb > Cr in central Guizhou Province. The areas in which high Hg values were observed were distributed in a planar pattern, while areas with high values of Cd, As, Pb, and Cr were mostly distributed in dotted patterns. Atmospheric deposition and industrial activities are the main causes of heavy metal pollution in cultivated soils. Zhang et al. (2022b) showed that the levels of Sb, As, Cd, Pb, and Cu in the soil along the banks of the Duliu River caused mild pollution; the human health risks from heavy metals in the soil in the research areas were generally low, with children having greater health risks than adults. Cd and Sb were the main elements influencing human health, and it was shown that the risks to human health based on soil Cd levels could be spatially differentiated in the order of soil type > altitude > distance from river > land use type > slope.
Over the past few decades, Guizhou has experienced serious environmental problems, such as desertification, deforestation, organic and inorganic pollution, and persistent water shortages (Wang et al., 2022; Chen et al., 2022). The local government and some researchers have attempted to formulate strategies to lead the local people out of poverty and develop ecofriendly lifestyles. Since the beginning of the 21st century, the provincial government has been trying to develop the local tourism industry, for which environmental quality improvement is a key step. Some of the croplands have been or are in the process of being rearranged as pasture lands or forestlands. The changes that have occurred to the soil pollutants during this period and considerations that require special attention need to be identified. The aims of the present study are as follows: (a) to comparatively study the heavy metals in the soils from both natural and artificial forestlands and pasture lands; (b) to analyze the incidental heavy metal pollution problems caused by human activities.
2 Materials and methods
2.1 Study region
Guizhou is a typical karst area located on the Yunnan–Guizhou Plateau and has a subtropical humid climate with annual average temperatures of approximately 12°C–25°C; the January temperatures range from −2°C to 12°C and July temperatures range from 20°C to 29°C. Changshun County (106°12′32″–106°38′48″E, 25°39′28″–26°17′17″N) is a typical karst region in Guizhou Province in southwestern China and covers an area of 1554.6 km2. The study area belongs to a humid zone in the mid-subtropical monsoon climate region and has obvious three-dimensional climatic characteristics. The mean annual temperature ranges from 13.4°C to 19.5°C and mean annual precipitation ranges from 1250 mm to 1400 mm, with four clearly evident seasons. The vegetation in this area includes broad-leaved mixed forests, evergreen broad-leaved forests, and montane elfin forests. Sufficient availability of water in this region promotes conditions for karst erosion and general erosion, and the desert area accounts for 77.9% of the total area. No obvious point pollution sources are noted in the study area.
2.2 Sampling
From March to June of 2022, eighteen soil profiles consisting of 120 soil samples were collected from natural forestlands (NFLs), natural brush lands (NBLs), natural pasture lands (NPLs), artificial forestlands (AFLs), artificial brush lands (ABLs), and artificial pasture lands (APLs) in Changshun County (Figure 1). These sampling areas have the same soil formation processes and similar geochemical backgrounds. All artificial areas (AFLs, ABLs, and APLs) were transferred from farmlands that were used for food production to the currently used types over a decade ago. The detailed information for each sampling site is listed in Table 1. Each soil profile was sampled at seven layers (0–10, 10–20, 20–30, 30–40, 40–50, 50–70, and 70–90 cm) if the soil depth was equal to or greater than 90 cm. Otherwise, sampling was carried out to the actual depth of the soil. For example, four samplings were performed if the soil depth was between 40 cm and 50 cm. The soil samples were transferred to the lab and dried at room temperature (ca. 28°C) before being ground and passed through a sieve (0.22 mm). The collected samples were stored in Ziploc bags for the analysis of heavy metals.
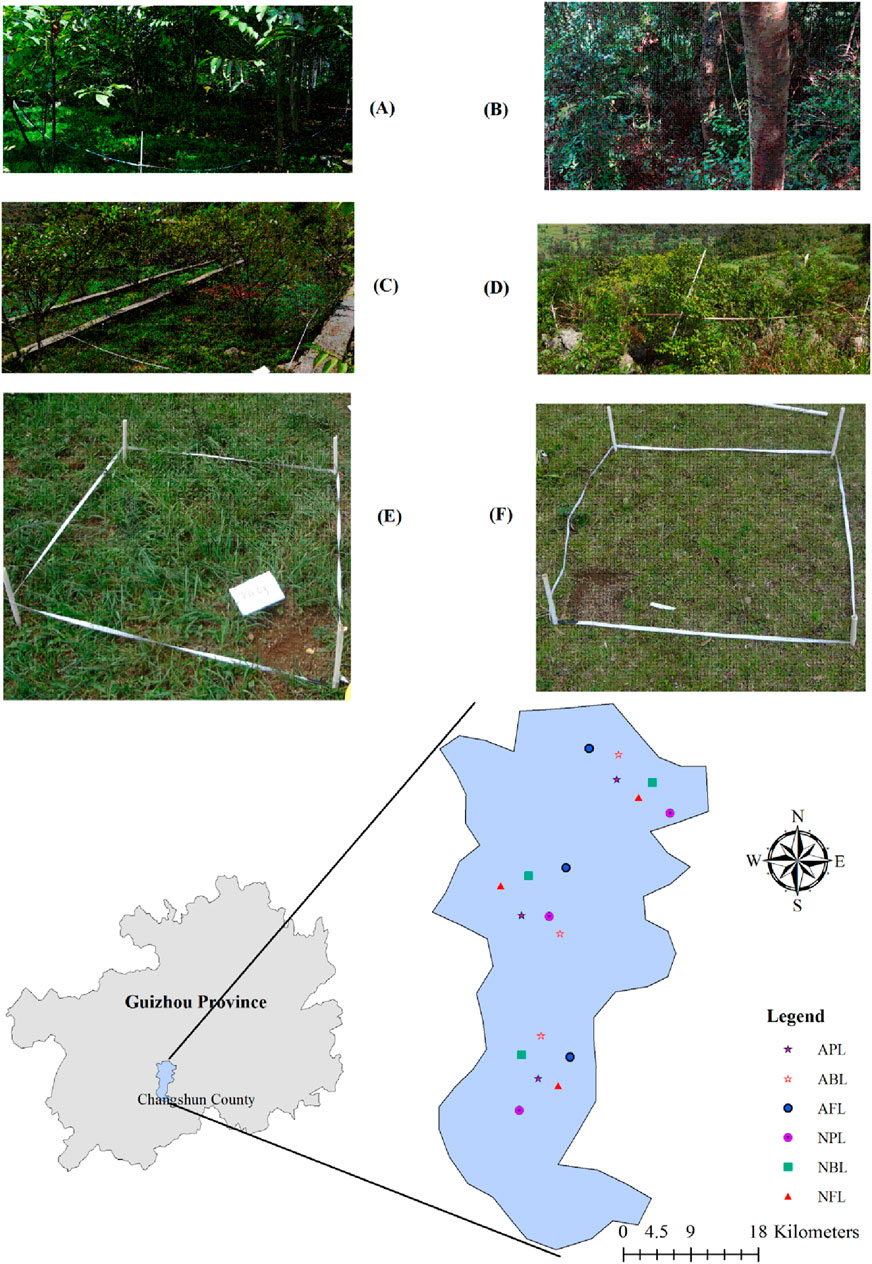
Figure 1. Study region and different types of land use: (A) artificial forest land; (B) natural forest land; (C) artificial brush land; (D) natural brush land; (E) artificial pasture land; (F) natural pasture land.
2.3 Analytical procedures
For analysis of Pb, Cd, and Cu, about 1 g (accurate to 0.0001 g) of each soil sample was weighed and digested with 30 mL of a digesting mixture composed of HNO3, HClO4, and HCl (HNO3:HClO4:HCl = 4:1:5 v/v/v). The Pb, Cd, and Cu contents were then determined with inductively coupled plasma atomic emission spectrometry (5300V, Perkin Elmer Corporation, Waltham, MA, USA) (Wang et al., 2020; Wang et al., 2021). For determination of As and Hg, about 0.3 g (accurate to 0.0001 g) of the soil sample was weighed and transferred to a 50-mL tube, followed by addition of 10 mL of a digesting solution composed of HCl, HNO3, and H2O (HCl:HNO3:H2O = 3:1:4 v/v/v) (Tokumoto et al., 2014). The tubes were then placed in a boiling water bath for 3 h and shaken at intervals of 30 min. The solutions were next transferred to 50-mL volumetric flasks and 5 mL of a reducing agent (10% thiourea solution) was added to each flask, followed by dilution to volume with 5% HCl (v/v). The As and Hg levels were determined using atomic fluorescence spectroscopy (AFS-933, Jitian Corporation, Shanghai, China). The quality of the analytical procedure was controlled using the certified reference material GBW 07403, which was analyzed in triplicate while the soil samples were replicated at a 20% level. The uncertainty of the analytical procedure was thus found to be within 10%. Analytical blanks were provided for all determinations (Huang et al., 2018; Huang et al., 2017). In addition, the other soil chemical indicators, such as total phosphorus (TP), total nitrogen (TN), and total potassium (TK), were tested simultaneously (Wang et al., 2022; Huang et al., 2018).
2.4 Assessment of heavy metals with gray clustering analysis
The heavy metal pollution at each study site was evaluated by gray clustering analysis, and the weight of each heavy metal in the clustering was determined. The basic theory of this analytical method can be found in literature (Pan et al., 2009; Fan et al., 2016). In the present study, five pollution indices (Pb, Cd, Hg, As, and Cu) were selected as the clustering indices to evaluate the pollution status at each studied site. The evaluation criteria were based on those recommended by the Ministry of Ecology and Environment of the People’s Republic of China (GB15618-2018) (Wang et al., 2021; Fan et al., 2016; Fan et al., 2012; Zhang et al., 2019) (Table 2).
2.4.1 Gray whitening function
In the present study, the whitening functions of the ith clustering object’s pollution index j are displayed as follows:
2.4.1.1 Class I
2.4.1.2 Class II
2.4.1.3 Class III
where xi is the ith concentration of the heavy metal in the soil sample; ai, bi, and ci are the criteria related to classes I, II, and III, respectively.
2.4.2 Clustering weight of each heavy metal in each classification
The measured content and biological toxicity index of each heavy metal were weighted, superimposed, and normalized. The biological toxicity indices of Pb, Cd, Hg, As, and Cu were found to be 4, 2, 1, 3, and 4, respectively (Fan et al., 2016; Fan et al., 2012). Then, the clustering weight of each heavy metal was calculated through the following formulas:
where Wi is the clustering weight of the ith heavy metal, n is the number of heavy metals studied, xi is the calculated concentration of the ith heavy metal in the soil sample, Si is the mean value of the ith heavy metal in the evaluation criteria, and Ki is the biological toxicity index of the ith heavy metal. The weighted values of each soil sample were calculated to obtain the corresponding weight value matrix αi.
2.4.3 Clustering coefficient and pollution degree
The clustering coefficients were calculated using the gray whitening functions and clustering weights, and these reflect the relationships between the clustering samples and gray classifications. The formula for calculation is as follows:
The value of δ determines the classification of the clustering sample site based on the clustering coefficient, where Rij is the gray whitening function, and αi is the weight of the ith indicator in the comprehensive clustering. The clustering sample site belongs to the classification with the maximum clustering coefficient.
2.5 Statistical analysis
Statistical analyses were conducted using Microsoft Excel 2003, SPSS 13.0 for Windows, and Origin 6.1 software.
3 Results and analyses
3.1 Characteristics of heavy metals under different land uses
The levels of heavy metals observed in the soil profiles from each land use are presented in Figure 2 and Table 3. The profile characteristics of these heavy metals clearly vary significantly among different land uses. As shown in Figure 2, the concentrations of Pb in the soil samples ranged from 2.99 to 84.44 mg kg-1, with a mean value of 38.73 mg kg-1. The concentrations of Cd in the soil samples ranged from 0.03 to 10.75 mg kg-1, with a mean value of 1.33 mg kg-1. The concentrations of Hg in the soil samples for different studied land uses ranged from ND (not detected given a detection limit of 0.005 mg kg-1) to 0.71 mg kg-1, with a mean value of 0.35 mg kg-1. The As concentrations in the soil samples from the NFLs showed a similar distribution as that of Pb for this type of land use; the mean concentration decreased from 31.42 mg kg-1 in the top 10 cm of the surface soil to 24.07 mg kg-1 in soil samples at 20–30 cm depth after a small increase (33.44 mg kg-1 in soil samples at 10–20 cm depth) and then increased gradually to 45.03 mg kg-1. In the soil samples from the NBLs, the As concentrations increased generally with depth, except for a weak decrease at a soil depth of 50–70 cm. For the NFLs and NPLs, the mean concentrations of As fluctuated irregularly, similar to those of Pb, with increase in soil depth. For the ABLs, AFLs, APLs, and NPLs, no obvious patterns of variation were found with changes in the soil depth. The concentrations of Cu in the soil samples ranged from 7.90 to 29.60 mg kg-1, with a mean value of 18.94 mg kg-1.
Overall, there were no regularity or significant distributions of all heavy metals from the surface to subsoil layers, and the distribution trends of each of the heavy elements in the same soil layer varied for different land uses (Table 3). The average values of Pb for different land uses decreased in the order of ABL > AFL > NBL > APL > NPL > NFL, among which the values for ABL and AFL were significant higher than the others. The distribution characteristics of Cd were in the order of ABL > AFL > NBL > NPL > NFL > APL, where the values in ABL and AFL were distinctly higher than in other land uses. There were minimal differences in the Cd content in NFL, NBL, NPL, and APL in the soil layers of depths 0–50 cm. The distribution of Hg decreased in the order of NPL > APL > ABL > AFL > NBL > NFL, and the discrepancies in the Hg values in each of the soil layers among the different land uses were large. The distribution of As decreased in the order of AFL > ABL > NFL > NPL > NBL > APL, among which the values in AFL and ABL were significantly higher than those in the NPL, NBL, and APL. The average values of Cu for different land use cased decreased in the order of ABL > APL > AFL > NFL > NBL > NPL, with only slight differences in soil layers at depths of 0–30 cm. However, there were large differences in Cu values in soil layers at depths of 30–90 cm. In summary, each of the heavy metals were present at higher concentrations in the artificial land use cases than under natural land use; this implies that human interference maybe an important factor in heavy metal pollution.
3.2 Heavy metal contamination under different land uses
According to the Environmental Quality of Soil Standards of China, the limits for the studied heavy metals are listed in Table 2 (Fan et al., 2016). There are three limiting values for each element based on soil acidity. In the present study, all soil samples had pH values less than 7.5. Therefore, two groups of the class II limit values were applied.
In the soil samples from the NFLs, all concentrations of Pb and Hg met the limits under class I. About 36% of the Cd concentrations met the limits under class I, 31.58% met the limits under class II, and the rest met the limits under class III. All of the Hg concentrations in the 0–40 cm depth range were very low and met the limits under class I. However, the Hg concentrations at depths greater than 40 cm exceeded 0.15 mg kg-1 and sometimes exceeded 0.30 mg kg-1. All of the As concentrations exceeded the limits under class I but were within the limits of class II, except the soil samples collected at depths of 40–90 cm, whose concentrations exceeded 25 mg kg-1. All concentrations of Cu met the limits under class I.
In the soil samples from NBLs, about 36.84% of the Pb concentrations met the limits under class I, and the rest were within the limits of class II. In both NPLs and APLs, most of the Pb concentrations were below 35 mg kg-1. It is worth noting that all concentrations of Hg exceeding 0.30 mg kg-1 (with some sample concentrations greater than 0.5 mg kg-1) were beyond the limits of class II. In the AFLs, all of the Pb concentrations exceeded the limits under class I, and all of the As concentrations exceeded the limits under class II. Most of the Hg concentrations exceed the limits under class II. In the soils from both AFLs and ABLs, all Cd concentrations exceeded 1.00 mg kg-1, which was beyond the limits of class III.
3.3 Clustering analysis of heavy metal pollution in soils under different land uses
According to the Equations 1–6, the heavy metals clustering weights under the different land use types was calculated. This results shown in Figure 3, Pb and Cu contributed little to the heavy metal concentrations under all kinds of land use based on the clustering weights. In the NBLs, AFLs, and ABLs, the clustering weights of Cd were greater than 50% of the total. In the NFLs and NPLs, the clustering weights of Cd were still greater than those of the other heavy metals. However, the clustering weights of Hg were largest among all the heavy metals studied in the APLs. Generally, the clustering weights of Hg in the pasture lands were greater than those in the brush lands, followed by forestlands. It was also found that the clustering weights of As accounted for a considerable portion of the heavy metal concentrations under all types of land use in this study and especially in the NFLs. The pollution degree of each sampling site is listed in Table 4. The soils from the NFLs, NPLs, and APLs belonged to class II, while those from NPL-3 belonged to class I. The soils from the NBLs, AFLs, and ABLs belonged to class III.
4 Discussion
4.1 Sources of heavy metals in the study area
The environmental fates of pollutants are determined by many factors. However, the physicochemical properties, geochemical baselines, anthropic activities, and microcircumstances are critical for determining the pollution levels and environmental behaviors of heavy metals (Teng et al., 2022). Based on field investigations and heavy metal distribution characteristics under different land uses, we hypothesized that the heavy metals in soils under different land uses would have different pollution sources. The baseline values (soil formation), application of fertilizers, and atmospheric deposition were considered as the critical sources in study area since there were no local mining or refining industries that would cause significant heavy metal pollution. Based on the Pearson correlation analysis, there were significant correlations between Pb and TK, Cd and TP, as well as As and TN; however, there were no significant correlations among Cu, Hg, and the soil nutrient elements (Table 5).
The Cu concentrations were very low in all soil samples; however, in the deeper layers, Cu concentrations in soils from APLs and ABLs were obviously higher than those from the other samples under study. In addition, the background value of Cu in the karst mountain area has been reported to be high (Wang et al., 2021). This finding indicates that Cu pollution is mainly from soil formation. The ABLs and AFLs were developed for growing different types of fruits; to promote good production, the land owners were reported to have applied different fertilizers that could cause heavy metal pollution. Previous studies have suggested that Cd, Pb, and As concentrations are higher in cultivated soils owing to fertilizer application (Atafar et al., 2010; Wang et al., 2012); these results indicate that fertilizer application is an important source of heavy metal pollution (Cd, Pb, and As) in ABLs and AFLs. According to the information listed in Tables 2, 3 and the GB15618-2018 standards, there were some samples in which the Hg contents were higher than the limiting value for paddy lands but lower than those for other land types. The production of Hg in Guizhou is known globally. Hg pollution in Guizhou Province has been a severe problem for a long time (Feng et al., 2008; Streets et al., 2005). It is well known that karst landforms have unique water ecological systems with dual above ground and underground structures; moreover, the underground water system is highly developed. Xu et al. (2020) noted that there was a significant positive correlation between Cd and Hg levels in underground water in karst mountain areas. In addition, the Cd content is relatively stable in the underground water in karst areas related to mining areas (Liao et al., 2023). Although there were no Hg mining or refining industries in the studied region, the groundwater could also be an important factor in the presence of heavy metals. Guo et al. (2024) showed that atmospheric deposition contributes to accumulation of heavy metals in soil. These results indicate that Hg pollution in this area may be caused by the underground water and atmospheric deposition (Feng et al., 2008).
4.2 Effects of land use changes on soil heavy metal pollution
Land use change is a significant factor affecting the sources and environmental behaviors of heavy metals. Atafar et al. (2010) reported that fertilizer application resulted in significantly higher concentrations of Cd, Pb, and As in cultivated soils (p-value <0.05). The clustering analysis results indicate that heavy metal pollution in the AFLs, ABLs, and NBLs are significantly higher than in other types of land use.
The Cd concentrations in the soil samples from the AFLs and ABLs were obviously higher than those from other types of land use in this study. This suggests that fertilizer application increased the concentrations of Cd, Pb, Cu, and As in the cultivated soils. The concentrations of Hg in the soil samples from NPLs were generally higher than those from the other types of land use. As these lands share the same soil formation processes and similar geochemical baselines, we believe that pollution caused by fertilizer application was the main reason for the increased Hg concentrations in soils from ABLs, which were higher than those from NBLs.
The results of this study show that there is a strong correlation between the soil Hg concentration and application of manure or compost. In forestlands, large tree canopies reduce Hg pollution caused by atmospheric deposition, which may be the main reason why Hg concentrations in the soil samples from NFLs were much lower than those from NBLs, APLs, and NPLs. Furthermore, Hg concentrations in soil samples from NBLs were slightly higher than those from APLs. We inferred that these were possibly the effects of management activities. The local farmers spread grass seeds to increase the grass quantity, and high levels of grazing also promote grass growth rates. Therefore, Hg concentrations in soils from APLs were generally lower than those in NPLs. This may be due to the fact that artificial grass planting has a certain alleviating effect on Hg pollution (Pacyna and Pacyna, 2001).
5 Conclusion
There were large variations in the concentrations of five types of heavy metals for different types of land use, and these concentrations were generally higher in artificial land uses than natural land use modes. NFLs can suppress Pb and Cu pollution to a certain degree, and the Cd and As levels in APLs are generally low. The lowest Cu concentrations were found in soils from NPLs. Exogenous introduction is an important method of heavy metal pollution, and land use change is a common practice in land resource management. Many positive and negative effects may arise during the process of land use change. In the study region, the soils were polluted by As and Cd to some extent, and fertilizer application was the main contributor in these two cases. Hg pollution is a serious issue in Guizhou Province, where soil concentrations often reach up to 0.59 mg kg-1 in NPLs, 0.47 mg kg-1 in APLs, 0.20 mg kg-1 in AFLs, and 0.36 mg kg-1 in ABLs. However, trees and brush plants play important roles in protecting soil from contamination by Hg through atmospheric deposition. Thus, changing both NPLs and APLs to forestlands could benefit the local ecosystems as these reduce Hg contamination. In addition, pastoral cultivation is a feasible method of alleviating soil Cd pollution. In the process of cultivating forestlands and brush lands, special attention should be devoted to Cd pollution of the soil.
Data availability statement
The original contributions presented in this study are included in the article/supplementary material, and any further inquiries may be directed to the corresponding author.
Author contributions
XJ: Conceptualization, Data curation, Formal analysis, Investigation, Methodology, Software, Writing–original draft. XW: Conceptualization, Data curation, Formal analysis, Funding acquisition, Investigation, Methodology, Project administration, Resources, Software, Supervision, Validation, Visualization, Writing–original draft, Writing–review and editing. YL: Investigation, Software, Writing–review and editing. YH: Investigation, Software, Writing–review and editing. XH: Conceptualization, Formal analysis, Funding acquisition, Investigation, Methodology, Project administration, Supervision, Writing–review and editing.
Funding
The authors declare that financial support was received for the research, authorship, and/or publication of this article. This research was funded by Guizhou Provincial Science and Technology Projects (nos. QKHZC[2023]YB215 and QKHZC[2023]YB141), Doctoral Research Fund of Guiyang Healthcare Vocational University (no. K2023-8), Department of Science and Technology of Guizhou Province (nos. LH[2017]7371, [2019]1217, [2016]2595-2, and [2019]2840), Department of Forestry of Guizhou Province (no. QLKH[2017]17), and Department of Education of Guizhou Province (no. KY[2021]302).
Conflict of interest
The authors declare that the research was conducted in the absence of any commercial or financial relationships that could be construed as a potential conflict of interest.
Publisher’s note
All claims expressed in this article are solely those of the authors and do not necessarily represent those of their affiliated organizations or those of the publisher, editors, and reviewers. Any product that may be evaluated in this article or claim that may be made by its manufacturer is not guaranteed or endorsed by the publisher.
References
Ahmed, M. K., Habibullah-Al-Mamun, M., Parvin, E., Akter, M. S., and Khan, M. S. (2013). Arsenic induced toxicity and histopathological changes in gill and liver tissue of freshwater fish, tilapia (Oreochromis mossambicus). Exp. Toxicol. Pathology 65, 903–909. doi:10.1016/j.etp.2013.01.003
Atafar, Z., Mesdaghinia, A., Nouri, J., Homaee, M., Yunesian, M., Ahmadimoghaddam, M., et al. (2010). Effect of fertilizer application on soil heavy metal concentration. Environ. Monit. Assess. 160, 83–89. doi:10.1007/s10661-008-0659-x
Chen, L. S., Xiong, K. N., Chen, Q. W., Shu, T., and Wu, J. (2022). Response mechanism of soil conservation function to rocky desertification under eco-environmental harness of karst areas. Resour. Environ. Yangtze Basin 29, 499–510. Available at: https://xueshu.baidu.com/usercenter/paper/show?paperid=1a3g0e602f4s0vu0qa6a00k087435300&sc_from=pingtai4&cmd=paper_forward&wise=0
Clarkson, T. W., Magos, L., and Myers, G. J. (2003). Human exposure to mercury: the three modern dilemmas. J. Trace Elem. Exp. Med. 16, 321–343. doi:10.1002/jtra.10050
Fan, L., Chen, F., and Fan, Y. (2012). Comprehensive assessment of soil environmental quality with improved grey clustering method: a case study of soil heavy metals pollution. J. Agric. Sci. Appl. 1, 67–73. doi:10.14511/jasa.2012.010302
Fan, M. Y., Yang, H., Huang, X. F., Cao, R. S., Zhang, Z. D., Hu, J. W., et al. (2016). Chemical forms and risk assessment of heavy metals in soils around a typical coal-fired power plant located in the mountainous area. China Environ. Sci. 36, 2425–2436. doi:10.3969/j.issn.1000-6923.2016.08.024
Feng, X. B., Li, P., Qiu, G. L., Wang, S. F., Li, G. H., Shang, L. H., et al. (2008). Human exposure to methylmercury through rice intake in mercury mining areas, Guizhou Province, China. Environ. Sci. and Technol. 42, 326–332. doi:10.1021/es071948x
Geest, H. G. V., Greve, G. D., Boivin, M. E., Kraak, M. H. S., and van Gestel, C. A. M. (2000). Mixture toxicity of copper and diazinon to larvae of the mayfly (ephoron virgo) judging additivity at different effect levels. Environ. Toxicol. Chem. 19, 2900–2905. doi:10.1002/etc.5620191208
Guo, B., Yang, G. F., and Liu, Q. (2024). Effect of atmospheric dry and wet deposition on heavy metal content in farmland in the Nanjing section of the Yangtze Rive. J. Geol. 48 (1), 85–91. Available at: https://kns.cnki.net/knavi/journals/JSDZ/detail?uniplatform=NZKPT.
Haddou, N., Ghezzar, M. R., Abdelmalek, F., Ognier, S., Martel, M., and Addou, A. (2014). Plasmacatalytic removal of lead acetate assisted by precipitation. Chemosphere 107, 304–310. doi:10.1016/j.chemosphere.2013.12.071
Huang, X. F., Hu, J. W., Qin, F. X., Quan, W. X., Cao, R. S., Fan, M. Y., et al. (2017). Heavy metal pollution and ecological assessment around the jinsha coal-fired power plant (China). Int. J. Environ. Res. Public Health 14, 1589. doi:10.3390/ijerph14121589
Huang, X. F., Zhou, Y. C., and Zhang, Z. M. (2018). Carbon sequestration anticipation response to land use change in a mountainous karst basin in China. Journal of Environmental Management. J. Environ. Manage. 228, 40–46. doi:10.1016/j.jenvman.2018.09.017
Jin, Z. G., and Zhou, M. Z. (2012). An assessment on contamination and potential ecological risk of cadmium and arsenic in the cultivated soils around the Ni-Mo mining area in Songli, Zunyi, China. J. Agro-Environment Sci. 31, 2367–2373. doi:10.1007/s11783-011-0280-z
Liao, H. W., Jiang, Z. C., Zhou, H., Qin, X. Q., Huang, Q. B., and Wu, H. Y. (2023). Heavy metal pollution and health risk assessment in karst basin around a lead-zinc mine. Environ. Sci. 44 (11), 6085–6094. doi:10.13227/j.hjkx.202210335
Ma, L., Zhang, L., Zhang, S., Zhou, M., Huang, W., Zou, X., et al. (2024). Soil protists are more resilient to the combined effect of microplastics and heavy metals than bacterial communities. Sci. total Environ. 906 (Jan.1), 167645. doi:10.1016/j.scitotenv.2023.167645
Milner, M. J., and Kochian, V. (2008). Investigating heavy-metal hyperaccumulation using Thlaspi caerulescens as a model system. Ann. Bot. 102, 3–13. doi:10.1093/aob/mcn063
Olawotin, R., Oyewole, S. A., and Grayson, R. L. (2012). Potential risk effect from elevated levels of soil heavy metals on human health in the Niger delta. Ecotoxicol. Environ. Saf. 85, 120–130. doi:10.1016/j.ecoenv.2012.08.004
Pacyna, J. M., and Pacyna, E. G. (2001). An assessment of global and regional emissions of trace metals to the atmosphere from anthropogenic sources worldwide. Environ. Rev. 9, 269–298. doi:10.1139/a01-012
Pan, A., Hu, L. H., Li, T. S., and Li, C. Z. (2009). Assessing the eutrophication of Shengzhong reservoir based on grey clustering method. Chinese Journal of Population. Resour. Environ. 7, 83–87. doi:10.1080/10042857.2009.10684929
Rainio, M. J., Eeva, T., Lilley, T., Stauffer, J., and Ruuskanen, S. (2015). Effects of early-life lead exposure on oxidative status and phagocytosis activity in great tits (Parus major). Comp. Biochem. Physiology C-Toxicology and Pharmacol. 167, 24–34. doi:10.1016/j.cbpc.2014.08.004
Roy, N. M., DeWolf, S., Schutt, A., Wright, A., and Steele, L. (2014). Neural alterations from lead exposure in zebrafish. Neurotoxicology Teratol. 46, 40–48. doi:10.1016/j.ntt.2014.08.008
Shakoor, M. B., Ali, S., Farid, M., Farooq, M. A., and Bharwana, S. A. (2013). Heavy metal pollution, a global problem and its remediation by chemically enhanced phytoremediation: a review. J. Biodivers. Environ. Sci. 3, 12–20. Available at: https://www.academia.edu/26062642/.
Shukla, L., and Jain, N. (2022). A review on soil heavy metals contamination: effects, sources and remedies. Appl. Ecol. Environ. Sci. 10, 15–18. doi:10.1080/15320383.2019.1592108
Streets, D. G., Hao, J. M., Wu, Y., Jiang, J. K., Chan, M., Tian, H. Z., et al. (2005). Anthropogenic mercury emissions in China. Atmos. Environ. 39, 7789–7806. doi:10.1016/j.atmosenv.2005.08.029
Teng, Y. G., Ni, S. J., Tuo, X. G., Zhang, C. J., and Ma, Y. X. (2022). Geochemical baseline and trace metal pollution of soil in Panzhihua mining area. Chin. J. Geochem. 21, 274–281. doi:10.1007/BF02831093
Tokumoto, M., Kutsukake, N., Yamanishi, E., Katsuta, D., Anan, Y., and Ogra, Y. (2014). Arsenic (+3 oxidation state) methyltransferase is a specific but replaceable factor against arsenic toxicity. Toxicol. Rep. 1, 589–595. doi:10.1016/j.toxrep.2014.08.011
Wang, F., Zhao, L., Shen, Y., Meng, H., Xiang, X., Cheng, H., et al. (2012). Analysis of heavy metal contents and source tracing in organic fertilizer from livestock manure in North China. Trans. Chin. Soc. Agric. Eng. 29, 202–208. doi:10.3969/j.issn.1002-6819.2013.19.025
Wang, X. F., Cao, R. S., Wu, X. L., Zhang, Z. M., and Huang, X. F. (2021). Study on heavy metals pollution assessment method of soil around a wasted mining in Karst mountain area. J. Guizhou Normal Univ. Nat. Sci. 39, 29–35. doi:10.16614/j.gznuj.zrb.2021.05.005
Wang, X. F., Huang, X. F., Hu, J. W., Wu, X. L., and Yao, S. M. (2020). Cadmium pollution and Bioconcentration characteristic in crops around the abandon Ni-Mo mining area in the karst mountainous. Environ. Chem. 39, 1872–1882. doi:10.7524/j.issn.0254-6108.2019051301
Wang, X. F., Huang, X. F., Xiong, K. N., Hu, J. W., Zhang, Z. M., and Zhang, J. C. (2022). Mechanism and evolution of soil organic carbon coupling with rocky desertification in south China karst. Forests 13, 28. doi:10.3390/f13010028
Wang, Y. Y., Wang, D. Z., Lin, L., and Wang, M. H. (2015). Quantitative proteomic analysis reveals proteins involved in the neurotoxicity of marine medaka Oryzias melastigma chronically exposed to inorganic mercury. Chemosphere 119, 1126–1133. doi:10.1016/j.chemosphere.2014.09.053
Wei, M., Pan, A., Ma, R., and Wang, H. (2023). Distribution characteristics, source analysis and health risk assessment of heavy metals in farmland soil in shiquan county, shaanxi province. Process Saf. Environ. Prot. 171, 225–237. doi:10.1016/j.psep.2022.12.089
Wen, M., Ma, Z., Bgingerich, D., Zhao, X., and Zhao, D. (2022). Heavy metals in agricultural soil in China: a systematic review and meta-analysis. Eco-environment and Health 1, 219–228. doi:10.1016/j.eehl.2022.10.004
Xu, C. X., Yan, H. L., Zhang, S. Q., Lei, L. S., and Hu, B. L. (2020). Heavy metal content and health risk assessment in water of karst cave in libo, Guizhou. Environ. Sci. and Technol. 43 (2), 204–212. doi:10.19672/j.cnki.1003-6504.2020.02.030
Yan, B., and Zhang, X. (2021). Current status, causes and harm of soil arsenic pollution. IOP Conf. Ser. Earth Environ. Sci. 769, 022034. doi:10.1088/1755-1315/769/2/022034
Zhang, D., Zhou, M. Z., Xiong, K. N., Gu, B. Q., and Yang, H. (2019). Risk assessment of nickel in soils and crops around the Ni-Mo polymetallic mining area in Song-lin, Zunyi, China. J. Agro-Environment Sci. 38, 356–365. doi:10.11654/jaes.2018-0746
Zhang, H. Z., Cui, W. G., Huang, Y. M., Li, Y. L., Zhong, X., and Wang, L. (2022a). Evaluation and source analysis of heavy metal pollution of farmland soil around the mining area of karst region of central Guizhou Province. Acta Sci. Circumstantiae 42, 412–421. doi:10.13671/j.hjkxxb.2021.0319
Zhang, S. Y., Wu, L. N., Zhang, G. Y., Wang, D., and Wu, P. (2022b). Soil heavy metal pollution analysis and health risk assessment in Karst areas along the upper reaches of the Du Liujiang River. Acta Sci. Circumstantiae 42, 421–433. doi:10.13671/j.hjkxxb.2021.0474
Keywords: land use, heavy metals, pollution, profile characteristics, karst area, southwestern China
Citation: Jiang X, Wang X, Liu Y, Huang Y and Huang X (2024) Heavy metal changes related to land use changes in a karst area: a case study in Changshun, Guizhou Province, China. Front. Environ. Sci. 12:1471160. doi: 10.3389/fenvs.2024.1471160
Received: 26 July 2024; Accepted: 28 August 2024;
Published: 18 September 2024.
Edited by:
Yaoguang Guo, Shanghai Polytechnic University, ChinaCopyright © 2024 Jiang, Wang, Liu, Huang and Huang. This is an open-access article distributed under the terms of the Creative Commons Attribution License (CC BY). The use, distribution or reproduction in other forums is permitted, provided the original author(s) and the copyright owner(s) are credited and that the original publication in this journal is cited, in accordance with accepted academic practice. No use, distribution or reproduction is permitted which does not comply with these terms.
*Correspondence: Xianfei Huang, aHhmc3dqc0Bnem51LmVkdS5jbg==
†These authors have contributed equally to this work