- 1PowerChina HuaDong Engineering Corporation Limited, Hangzhou, China
- 2PowerChina ChongQing Engineering Corporation Limited, Chongqing, China
With the impact of climate change in recent years, the instability of precipitation and the increase of evaporation have made water resources in Shaanxi Province more and more stressed. How to maintain stable economic development while ensuring the sustainable development of agriculture within the limited water resources has become an important issue facing Shaanxi Province. This paper analyzes the temporal and spatial distribution characteristics of maize irrigation water requirement in Shaanxi Province based on meteorological data and obtains the ideal irrigation water requirement. However, considering the agricultural water scarcity issue in this region, this paper establishes different water scarcity scenarios, adopts the Jensen model to construct an objective function aimed at minimizing crop yield reduction, and solves it using a genetic algorithm to obtain the optimized maize irrigation scheduling under different water scarcity scenarios. The results analysis indicates the following: (1) The effective rainfall in Shaanxi Province from 1960 to 2019 shows a slight upward trend, while the maize water requirement and maize irrigation water requirement shows a downward trend. (2) The spatial distribution of maize irrigation water requirement in Shaanxi Province decreases gradually from north to south. High-value areas are mainly distributed in the northern regions of Yulin City and Yan’an City in Shanbei. Low-value areas are distributed in Ankang City in Shannan. (3) In the face of water scarcity, spring maize should ensure water use during the middle growth period, fast development period, and initial growth periods. Under the same water scarcity conditions, the yield reduction rate is the highest in Guanzhong, followed by the Shannan, and the lowest in Shanbei. This paper aims to provide a scientific and reasonable optimization plan for maize irrigation in Shaanxi Province by calculating and analyzing the maize irrigation water requirement in Shaanxi Province, combined with optimization tools such as genetic algorithms, to address the challenges brought by water resource constraints.
1 Introduction
Facing the dual pressures of global climate change and population growth, water scarcity has emerged as a global challenge. As the most populous country globally, China boasts a large total water resource volume. However, its per capita water resource availability significantly falls below the global average, highlighting a pronounced issue of water scarcity. In 2020, China’s total water resources amounted to 31,605.2 billion cubic meters, accounting for only 6% of the world’s water resources, indicating a relative scarcity of water resources in the country. Meanwhile, as an agricultural powerhouse, China’s agricultural water consumption has remained persistently high. Agriculture, as the foundation of the national economy, is one of the primary sectors consuming water resources. In 2020, agricultural water use in China accounted for 61.5% of total water use, a proportion significantly higher than in other countries. This high proportion of agricultural water use not only exacerbates the stress on China’s water resources but also poses a serious threat to the sustainable development of agricultural production. In agricultural production, traditional irrigation methods often lead to substantial waste of water resources, exacerbating the already strained water resources. Furthermore, rapid industrialization and urbanization have led to an increasing requirement for water in industrial and urban sectors, further exacerbating the supply-demand imbalance of water resources.
As a crucial province in the northwest plains of China, Shaanxi Province serves as both a significant agricultural production base and faces the dilemma of water scarcity. With the influence of climate change in recent years, the instability of precipitation and increased evaporation have intensified the strain on water resources in Shaanxi Province. Agriculture, as the primary sector consuming water resources, requires crucial control and management of water usage. Balancing economic stability within limited water resources while ensuring the sustainability of agriculture has become a critical issue for Shaanxi Province. In recent years, with the continuous advancement of technology, significant improvements have been made in agricultural water use efficiency. Improvements in agricultural irrigation techniques, the development of water-saving agriculture, and the construction of agricultural water conservancy projects have all provided strong support for enhancing agricultural water use efficiency and reducing the proportion of agricultural water consumption. However, despite some achievements, water scarcity remains one of the crises faced by Shaanxi Province. To address this challenge, continuous improvement of irrigation schedulings and reduction of agricultural water usage have become focal points of research for many scholars.
Research progress on crop irrigation schedulings can be analyzed from multiple perspectives. In terms of water-saving irrigation technology application and development, China’s agricultural water-saving irrigation technology is also constantly improving, and micro irrigation technology has been proven to be the most effective water-saving technology, saving 50%–70% of water compared to surface flood irrigation; Compared to sprinkler irrigation, it saves 15%–20% of water. (Zhang and Hu, 2021). Additionally, the impact and mechanisms of water-saving irrigation technologies such as controlled irrigation, alternate wetting and drying irrigation, and dry seeding cover on rice yield have been extensively studied (Wang et al., 2022; Gu et al., 2022). Regarding the optimization of irrigation schedulings, researchers have proposed various models and methods to optimize the allocation of crop water resources. These include multi-objective planning models considering economic and ecological benefits (Li et al., 2005), as well as single-crop models and multi-crop water resource optimization models based on SPAC theory (Wang et al., 2014). The aim of these studies is to achieve efficient utilization of water resources and maximize crop yields through scientifically designed irrigation schedulings. The selection of efficient water-saving irrigation models is also a current research focus. Studies indicate that selecting efficient water-saving irrigation models adapted to the needs of sustainable development in the region is crucial for addressing agricultural water scarcity issues (Zhang et al., 2019).
Moreover, research on non-adequate irrigation decision indicators provides a scientific basis for the rational use of limited water resources (Guo et al., 2007). The impact of changes in irrigation management practices on crop water use is also an important aspect of research. For example, the accelerated pace of irrigation management reform in the Yellow River Basin irrigation areas, where the transformation from water user associations exceeds contracted management, has become the leading mode of reform (Wang et al., 2011). This underscores the significant role of innovation and reform in irrigation management practices in improving irrigation efficiency and promoting agricultural sustainable development.
The main focus of this article is on researching the optimization of irrigation schedulings. For instance, Reta et al. (2024) considered the impact of climate change on future crop water demand and irrigation systems, and used a generalized algebraic modeling system with non negative constraints and non negative constraints based on farmer adaptation to program and optimize farmland allocation. Ashkan et al. (2022) combined the Taguchi optimization method with the HYDRUS-1D model, and its successful application provides a promising method for optimizing the triggered irrigation scheduling of walnut seedlings. Martínez-Romero et al. (2021) used AquaCrop and MOPECO models to calculate and compare the crop water yield and irrigation water productivity functions of typical irrigated crops in the region under several irrigation strategies, and evaluated the performance of the models through experiments. Gao et al. (2023) conducted field experiments to analyze cotton yield and water-salt distribution characteristics in southern Xinjiang under two irrigation modes and various irrigation quotas. Ma et al. (2024) determined the irrigation scheduling for rice in the Hohai University water-saving garden by constructing multi-objective functions. Guo et al. (2017) analyzed the irrigation scheduling for alfalfa based on the Jensen model. Wang et al. (2007) used the Jensen model and aimed to maximize relative yield to determine the optimized irrigation scheduling for rice in the semi-arid areas of western Heilongjiang Province. While Shaanxi Province serves as a significant agricultural production base in China, with maize being one of its primary crops, there is a lack of research on optimal irrigation systems for corn in Shaanxi Province.
Therefore, based on meteorological data from Shaanxi Province, this article conducts in-depth calculations and analyses to determine the water requirement and irrigation water requirement for maize, considering various water scarcity scenarios. By adopting a strategy with minimizing grain yield reduction as the objective function and utilizing genetic algorithms for optimization, different water allocation schemes for maize under different water scarcity scenarios are obtained. These schemes provide important scientific basis and guidance for Shaanxi Province to achieve stable and sustainable agricultural production under limited water resources conditions.
2 Materials
2.1 Geographical characteristics
Shaanxi Province, located in the inland of China and situated in the middle reaches of the Yellow River, belongs to the relatively water-scarce northwest plain region. Climatically, Shaanxi Province transitions gradually from temperate to warm-temperate and further to subtropical climates from north to south. This climatic diversity provides rich conditions for agricultural production and ecological diversity in Shaanxi Province, but it also brings about uneven distribution of water resources. During the warm and humid seasons, the southern region is susceptible to extreme weather events like heavy rainstorms, while the northern region tends to be relatively dry, exacerbating water scarcity issues. In terms of topography, Shaanxi Province generally features higher terrain in the north and south with lower elevation in the central region. The two major mountain ranges, the Beishan and Qinling Mountains, naturally divide Shaanxi Province into three main regions: the northern part is the Loess Plateau, the central part is the Guanzhong Plain, and the southern part is the Qinba Mountainous region. This topographical variation significantly impacts the distribution of water resources in Shaanxi Province. The northern Loess Plateau has relatively scarce water resources due to its higher elevation, while the Guanzhong Plain, despite its flat terrain, faces prominent water supply-demand contradictions due to dense population and frequent agricultural activities. The Qinba Mountainous region possesses relatively abundant water resources, but due to its complex terrain, the difficulty in development and utilization is higher. From the perspective of water resources, Shaanxi Province faces severe spatial and temporal disparities in water resource distribution. On one hand, influenced by climate and topography, water resources in Shaanxi Province exhibit significant differences in both time and space. On the other hand, with rapid economic development and continuous population growth, the requirement for water resources in Shaanxi Province is increasing, while the available water resources are relatively limited, leading to an increasingly prominent water supply-demand contradiction in the province.
The topographic map of Shaanxi Province is shown in Figure 1.
2.2 Status of water use
From the perspective of agricultural water usage, it constitutes a substantial portion of the total water consumption in Shaanxi Province. In 2004, agricultural water usage accounted for 65.83% of the total, decreasing to 59.50% by 2019. The decreasing trend in the proportion of agricultural water usage over time indicates significant improvements in farmland irrigation efficiency due to advancements in agricultural technology and the widespread adoption of water-saving irrigation measures, leading to a substantial reduction in water usage per unit of land area. Simultaneously, the optimization of agricultural structure has also contributed to reducing agricultural water requirement, with a decrease in the cultivation area of high-water-consuming crops and a gradual increase in the cultivation area of water-saving crops. These factors collectively contribute to the decline in the proportion of agricultural water usage.
Similar to agricultural water usage, industrial water usage also demonstrates a declining trend. With the upgrading of industrial technology and increasing environmental awareness, industrial enterprises in Shaanxi Province have actively implemented water-saving measures to enhance water resource utilization efficiency. Additionally, some high-water-consuming and highly polluting industrial projects have been gradually phased out or relocated, thereby reducing the requirement for industrial water usage. The proportions of domestic and ecological water usage have been continuously increasing. With population growth and the accelerated urbanization process, the domestic water usage of residents has steadily increased. As people’s demands for living standards continue to rise, there is also a growing requirement for ecological water usage, such as urban greening and landscape construction.
The schematic diagram of the urban water use structure in Shaanxi Province is shown in Figure 2.
2.3 Crop yields
From Figure 3, it is apparent to observe the proportion of wheat and maize as the two major staple crops in Shaanxi Province, and based on this, analyze the changing trend in the structure of grain production. Wheat, as a traditional staple crop, once held a significant position in the grain production structure of Shaanxi Province. However, over time, the proportion of wheat production has shown a noticeable decline. This change may be influenced by various factors, including a reduction in cultivation area, limitations in cultivation techniques, and changes in market demand. With its long growth cycle and high demands on soil and water resources, amidst increasingly scarce resources, the decreasing proportion of wheat production also reflects the adjustment and optimization of agricultural production structure.
The proportion of maize production, on the other hand, shows an increasing trend. Maize, as a highly adaptable crop with a short growth cycle, high yield, and strong market demand, has seen a rapid growth in production. With advancements in agricultural technology and adjustments in planting structures, more and more farmers are choosing to cultivate maize, thereby driving the rapid increase in maize production. The increase in maize production not only meets market demand but also brings new growth points to grain production in Shaanxi Province. Apart from wheat and maize, the changes in production of other crops are relatively less pronounced. These crops may exhibit relatively stable production changes due to factors such as smaller cultivation scale and relatively stable market demand. Nevertheless, they still hold a certain proportion in the grain production structure of Shaanxi Province, playing an important role in enriching grain varieties and meeting diversified demands.
The changing trend in grain production structure aligns with the variation in the cultivated area of grain crops in Shaanxi Province. The fluctuation in cultivated area directly impacts the production of grain crops, while the changes in production structure reflect the adjustment and optimization of agricultural production structure. Therefore, by analyzing the changes in cultivated area and production structure, we can gain a deeper understanding of the development trends and challenges faced by grain production in Shaanxi Province.
2.4 Data sources
The water usage data for Shaanxi Province is sourced from the Shaanxi Provincial Water Resources Bulletin, while the cultivation production data are obtained from the Shaanxi Provincial Statistical Yearbook. The meteorological station data is sourced from the National Meteorological Information Center of the China Meteorological Administration, comprising 34 meteorological stations with daily meteorological data spanning 60 years from 1960 to 2019.
Based on the changes in agricultural water usage, cultivation area, and production of grain crops in Shaanxi Province as described earlier, this study focuses on analyzing the irrigation water requirements and optimizing irrigation schedulings for maize cultivation based on meteorological station data. Shaanxi Province is divided into three climatic regions, namely Shanbei, Guanzhong Plain, and Shannan, based on geographical location and climate differences. Shanbei mainly refers to the cities of Yan’an and Yulin in Shaanxi Province, including the counties and districts under the jurisdiction of these two cities. Guanzhong is located north of the Qinling Mountains, south of the Beishan Mountains, east of the Longshan Mountains, and west of the Yellow River. It is a river valley plain basin surrounded by mountains on three sides: south, north, and west, and open to the east, extending for more than 300 km from east to west. Shannan includes three prefecture level cities, Hanzhong, Ankang, and Shangluo. Shanbei and Shannan mainly cultivate spring maize, while Guanzhong Plain, due to its geographical and climatic characteristics, practices double cropping with mainly summer maize cultivation. Therefore, this study primarily investigates spring maize in Shanbei and Shannan, and summer maize in the Guanzhong Plain region. The distribution of meteorological stations can be observed in Figure 4.
3 Methods
3.1 Effective rainfall
The effectiveness of precipitation is related to factors such as rainfall characteristics, groundwater depth, crop growth conditions, soil texture, and structure (Wang et al., 2013; Yao et al., 2018). Due to the numerous factors influencing effective rainfall, in practical agricultural production, the calculation of effective rainfall often employs the method of effective utilization coefficient, which is based on empirical formulas (Allen et al., 1998). The calculation methods of effective rainfall and effective rainfall coefficient are shown in Equations 1, 2.
In the formula,
3.2 Maize water requirement
The water requirement of maize refers to the amount of water consumed during the growth period of maize, which is relatively stable under certain yield conditions and in specific regions (Guo et al., 2017; Xiao et al., 2008). The water requirement of maize is calculated using the single crop coefficient method recommended by the FAO, it is expressed by Equation 3 (Zhang et al., 2020).
In the equation,
In the equation,
The crop coefficient is calculated using the segmented single-value average method recommended by FAO-56 in 1998. When using it, it needs to be revised according to local conditions. According to the growth and development characteristics of crops, the crop growth stages are divided into the initial growth stage, rapid development stage, mid-growth stage, and maturity stage. In this study, the entire growth stages of maize are divided into four stages: initial growth period, fast development period, middle growth period, and maturity stage. The division of time nodes for each growth stage is shown in Table 1 (Qin et al., 2016; Li and Fan, 2015).
*In the following paragraphs, ① is used to represent the Initial growth period; ② is used to represent the Fast development period; ③ is used to represent the Middle growth period; ④ is used to represent the Maturity.
The values of crop coefficients need to consider local conditions, and the revised values of crop coefficients refer to research by Liang et al. (2011) and Kang et al. (1990) as shown in Table 2.
3.3 Maize irrigation water requirement
The irrigation water requirement refers to the amount of irrigation water needed to supplement crop water needs when the rainfall during the growth stage is insufficient. The irrigation water requirement is calculated by Equation 5.
In the formula,
3.4 Optimization model of irrigation scheduling
3.4.1 Objective function
where,
The process of solving Ya is described in Equations 6–13. In the formula,
3.4.2 Constraint condition
a. Available irrigation water
In the process of optimizing agricultural irrigation water and adjusting water resources allocation, the available irrigation water was determined according to statistical yearbook with the constraints are shown in Equation 14.
where
b. Maximum irrigation quota constraint
If the irrigation water amount is less than the calculated maximum irrigation quota, the irrigation depth is not enough and irrigation water is insufficient for crop growth, which will increase the irrigation times. If the irrigation amount is greater than this calculated quota, deep percolation or surface runoff loss will occur. The constraint equation for irrigation water demand is given in Equation 15.
where
3.4.3 Situation setting
In this study, six scenarios were set up to study the optimal allocation of irrigation water under different water shortage conditions, including agricultural full irrigation (AI), irrigation water reduced by 10% (IWS10), irrigation water reduced by 20% (IWS20), irrigation water reduced by 30% (IWS30), irrigation water reduced by40% (IWS40), and irrigation water reduced by 50% (IWS50).
3.4.4 Model solving principle
The objective function being single-target, the model primarily employs a Genetic Algorithm with enhanced elitism preservation strategy for optimization. Genetic Algorithm is an optimization algorithm that simulates principles from natural selection and genetics. It explores the solution space efficiently by mimicking operations like selection, crossover, and mutation, akin to biological evolution, to find the optimal solution to a problem. When solving single-target problems, Genetic Algorithm efficiently explores the solution space and gradually converges to the optimal solution through iterations. However, standard Genetic Algorithms may encounter slow convergence or get trapped in local optima when solving complex problems. To overcome these issues, this paper adopts the enhanced elitism preservation strategy in the Genetic Algorithm. Elitism preservation strategy is an effective optimization technique that preserves a portion of excellent individuals in each generation, directly passing them to the next-generation, thereby avoiding the loss of excellent genes and accelerating the convergence speed of the algorithm.
By combining the global search capability of Genetic Algorithm with the rapid convergence characteristic of the elitism preservation strategy, it becomes more efficient in solving single-target problems. This algorithm not only finds the global optimal solution in large solution spaces but also completes the solution process in a shorter time, providing strong support for practical problem-solving.
In terms of the preset parameters of the NSGA-algorithm. The preset population number
4 Results
4.1 Temporal and spatial analysis of maize effective rainfall, water requirement and irrigation water requirement
As the main maize planting region, the water resources situation during the growth process of maize in Shaanxi Province is of significant importance for the stability and sustainability of agricultural production. Based on the time distribution of effective rainfall, water requirement, and irrigation water requirement during the maize growing period in Shaanxi Province from 1960 to 2019 (as shown in Figure 5), this paper provides a detailed analysis of the trends of these indicators.
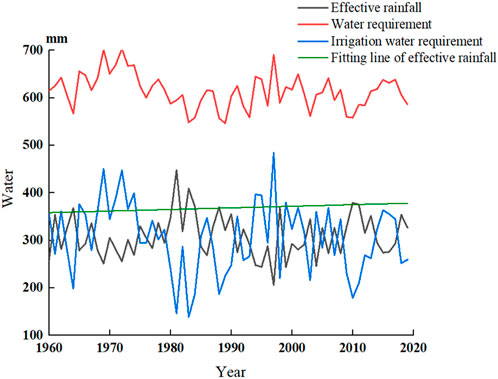
Figure 5. The change process of effective rainfall, water requirement and irrigation water requirement during the corn fertility period in Shaanxi Province.
Effective rainfall has shown a slight increase trend over the past 60 years. Although this trend is not significant, it reflects to some extent the subtle changes in the climate conditions of Shaanxi Province. The increase in effective rainfall helps meet the water requirement during the maize growth process, but due to the small magnitude of the growth, its impact on maize growth is relatively limited.
From the perspective of water requirement, there has been a significant downward trend from 1960 to 2019. This trend may be related to the progress of agricultural technology and adjustments in planting structure. With the continuous development of agricultural technology, the optimization of maize varieties and improved drought resistance have gradually reduced the water requirement during maize growth. In addition, adjustments in planting structure may also affect water requirement, such as increasing the proportion of water-saving crops.
The trend of irrigation water requirement is similar to that of water requirement, showing a downward trend as well. This indicates that irrigation water has been more effectively utilized in the maize planting process in Shaanxi Province. This may be related to advancements in irrigation technology, improvements in water resource management strategies, and increased awareness of water conservation among farmers. It is worth noting that the maximum value of irrigation water requirement occurred in 1997, reaching 476.45 mm. This may be due to exceptionally dry weather that year, leading to a significant increase in irrigation water requirement for maize growth. The minimum value occurred in 1983, only 139.02 mm, which may be related to abundant rainfall and sufficient soil moisture that year.
According to Figure 6, there are significant differences in the irrigation water requirements during various stages of the growth period between spring maize and summer maize. These differences not only manifest in different growth stages, but also vary with different sowing times. For spring maize, the distribution of its irrigation water requirements shows a certain regularity. During the maturity stage, as the growth of maize plants tends to be perfect, the requirement for water reaches its peak, resulting in the largest irrigation water requirement. In the rapid growth stage, maize plants are in a phase of rapid growth and also have a relatively strong requirement for water, with the irrigation water requirement following closely behind. In contrast, the irrigation water requirements in the middle and initial growth stages are relatively small. Unlike spring maize, the distribution of irrigation water requirements for summer maize exhibits a different pattern. During the rapid growth stage, the irrigation water requirement for summer maize is the largest, as the high temperature and frequent rainfall in summer promote rapid maize growth and high water requirement. The irrigation water requirements in the middle and initial growth stages are relatively small, while the irrigation water requirement in the maturity stage drops to the lowest level. There are also significant differences in the irrigation water requirements of maize in different regions. Due to the dry climate and relatively scarce water resources in Shanbei, the irrigation water requirement of maize during the middle growth stage accounts for a large proportion to compensate for the lack of soil moisture. In the Guanzhong region, the climate is relatively humid, and water resources are relatively abundant, so the irrigation water requirement of maize during the rapid growth stage accounts for a higher proportion. In Shannan, due to the complex terrain and diverse climate conditions, the distribution of maize irrigation water requirements shows certain regional characteristics.
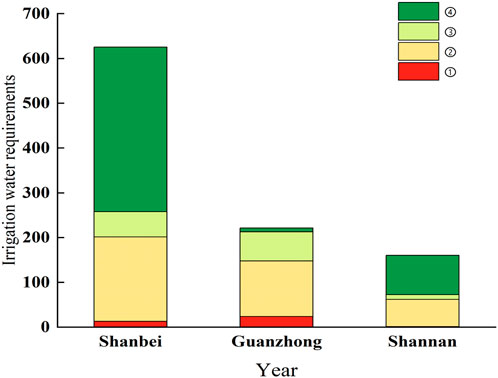
Figure 6. Water needs for irrigation in the stage of corn fertility in various regions of Shaanxi Province (①Initial growth period; ②Fast development period; ③Middle growth period; ④Maturity).
The proportions of the initial growth stage, rapid development stage, middle growth stage, and maturity stage vary among different regions during the total growth period. In Shanbei, the proportions are 2.08%, 30.08%, 9.02%, and 58.81%, respectively. In the Guanzhong region, the proportions are 10.66%, 56.09%, 29.32%, and 3.93%, respectively. In Shannan, the proportions are 0.74%, 37.98%, 6.60%, and 3.93%, respectively. These variations in proportions reflect the differences in climate conditions in different regions.
The irrigation water requirement for the entire growth period and each growth stage of maize is illustrated in Figure 7. As shown in Figure 7A, there are significant spatial differences in the average irrigation water requirement for maize crops in Shaanxi Province over multiple years throughout the entire growth period. Overall, high-value areas are mainly concentrated in the northern regions of Yulin and northern Yan’an cities. These areas are typically located in the Loess Plateau, characterized by arid climate and scarce rainfall, leading to a higher reliance on irrigation during the maize growth process. Therefore, irrigation becomes a critical factor in ensuring normal maize growth and yield in these regions. In contrast, low-value areas are distributed in Ankang city in Shannan. The climate in Shannan is humid with abundant rainfall, resulting in relatively sufficient natural water supply during maize growth. Consequently, irrigation water requirement is relatively low in these areas. The spatial distribution of irrigation water requirement for maize in Shaanxi Province aligns with the trend of maize water requirement, both showing a decreasing trend from north to south. This distribution pattern is closely related to the climatic conditions, topography, and maize planting structure in Shaanxi Province.
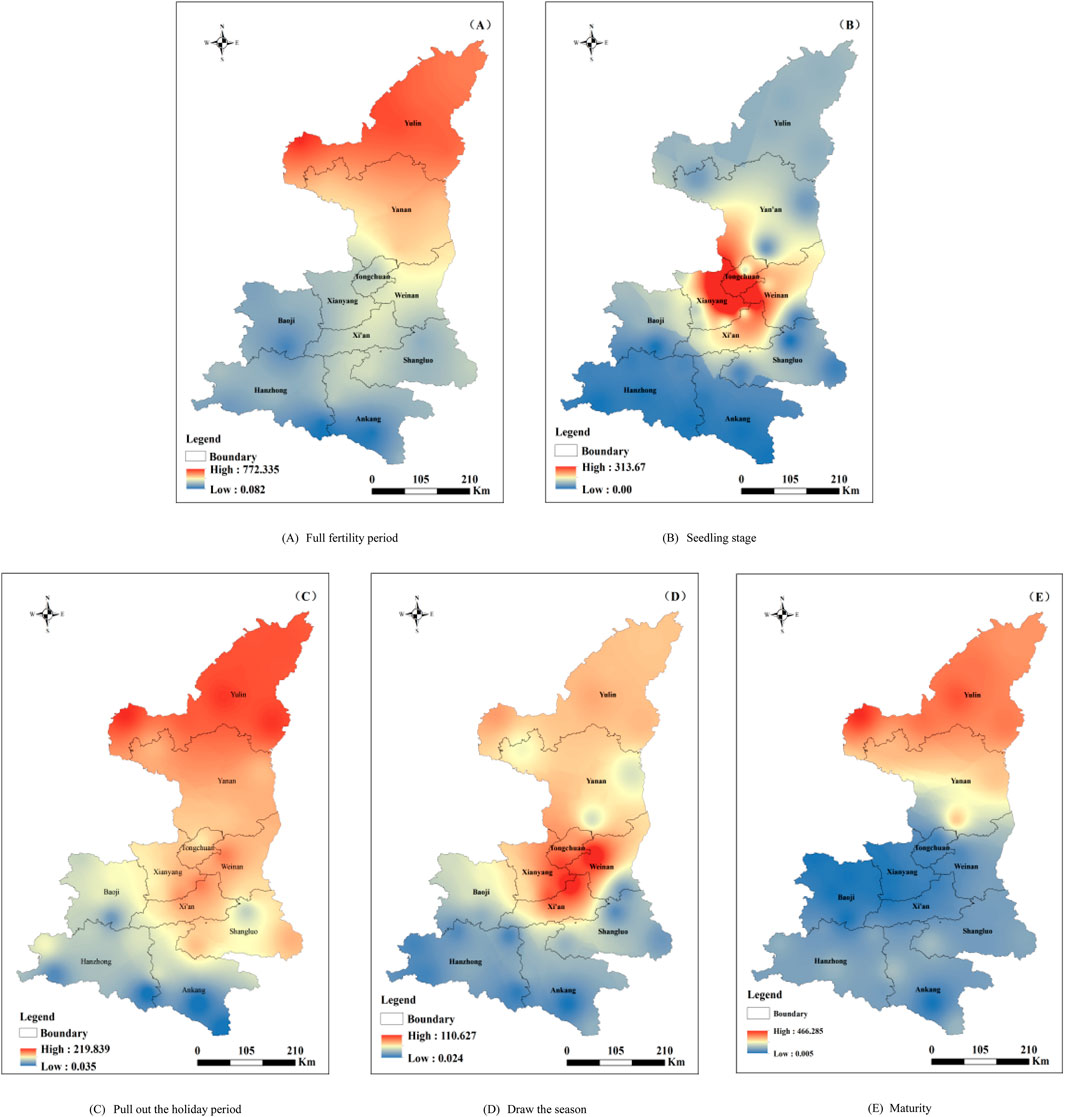
Figure 7. Spatial distribution map of the amount of water required for corn irrigation in Shaanxi Province. (A) Full fertility period (B) Seedling stage (C) Pull out the holiday period (D) Draw the season (E) Maturity.
Furthermore, there is a close relationship between the irrigation water requirement of crops in planting areas and their effective rainfall and crop water requirement. Effective rainfall is one of the important factors influencing irrigation water requirement. In areas with abundant rainfall, most of the water needed for maize growth comes from natural rainfall, resulting in relatively low irrigation water requirement. In arid areas where rainfall is insufficient, crop water requirement is high, and irrigation becomes a necessary means of supplementing water. Crop water requirement reflects the water needs of maize at different growth stages. During periods of vigorous growth, such as rapid development stages, maize requires a large amount of water, leading to increased irrigation water requirement. Conversely, during the early growth or maturation stages, crop water requirement is relatively low, and irrigation water requirement decreases accordingly.
Based on Figures 7B–E, significant spatial distribution characteristics of irrigation water requirement for different growth stages of maize in Shaanxi Province can be observed. When formulating irrigation strategies, factors such as climate, soil, and planting structure in various regions should be fully considered to ensure adequate water supply for maize at each growth stage. Additionally, efforts should be made to strengthen the research and application of water resource management and water-saving technologies to improve water resource utilization efficiency and promote the sustainable development of agricultural production.
4.2 Irrigation scheduling optimization results
The article has calculated the optimal irrigation water allocation for various growth stages of spring maize in Shanbei under different water shortage conditions. The specific optimal water allocation for each growth stage is shown in Figure 8.
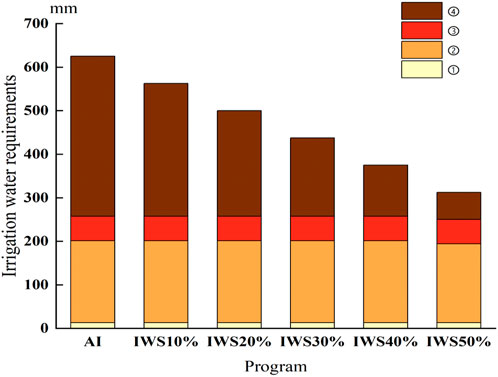
Figure 8. Optimization plan for irrigation water in Shanbei (①Initial growth period; ②Fast development period; ③Middle growth period; ④Maturity).
Under the IWS10% water shortage scenario, adjustments have been made to the irrigation strategy for spring maize. During the initial growth period, since the plants are still small and have low water requirements, irrigation water accounts for only 2.32% of the total irrigation volume. As the maize enters the fast development period and experiences rapid growth, the water requirement increases, with irrigation water accounting for 33.42% of the total. The middle growth period, crucial for reproductive growth, requires some water support, with irrigation water accounting for 10.03%. By the time maize reaches maturity and starts fruit formation and biomass accumulation, irrigation water requirement peaks at 54.23%. Compared to full irrigation (AI scenario), irrigation water volume is reduced by 62.52 mm during the maturity stage, while remaining unchanged in other stages.
As water shortage intensifies to IWS20%, further adjustments occur in irrigation water allocation for each growth stage. The initial growth period irrigation water percentage slightly increases to 2.89%, while the fast development period rises to 41.78%, and the middle growth period to 12.53%. However, irrigation water percentage during the maturity stage decreases to 42.79%. Compared to the AI scenario, irrigation water volume during the maturity stage reduces by 175.05 mm, indicating stricter water management during this critical growth phase under water shortage conditions.
At IWS30%, irrigation water allocation continues to adjust for each growth stage. The initial growth period irrigation water percentage rises to 3.31%, fast development period to 47.75%, and middle growth period to 14.33%, while decreasing to 34.62% during the maturity stage. Compared to the AI scenario, irrigation water volume during the maturity stage decreases by 231.31 mm, highlighting greater restrictions on water supply during the most severe water shortage conditions.
Under the water deficit scenario of IWS40%, the allocation of irrigation water becomes even more strained. The proportion of irrigation water used during the initial growth period reaches 3.86%, increases to 55.70% during the fast development period, and further increases to 16.71% during the middle growth period, while it is only 23.72% during the maturity stage. Compared to the AI scenario, the irrigation water volume during the maturity stage decreases by 287.58 mm, indicating that under extreme water scarcity conditions, to ensure the basic growth of maize, a significant reduction in irrigation water during the maturity stage is necessary.
Under the most severe IWS50% scenario, irrigation water allocation becomes extremely stringent. The initial growth period irrigation water percentage reaches 4.63%, fast development period 56.05%, and middle growth periodsurges to 20.06%, while dropping to 19.26% during the maturity stage. Compared to the AI scenario, not only does irrigation water volume during the maturity stage significantly decrease by 313.49 mm, but there’s also a noticeable decrease in irrigation water volume during the middle growth period. This suggests that under extreme water shortage conditions, significant reductions in irrigation water volume during both the maturity and middle growth period are necessary to ensure basic reproductive growth of maize.
From the above data, it is evident that as irrigation water shortage increases, significant changes occur in the irrigation water allocation for various growth stages of spring maize in Shanbei. With milder water shortages, reductions in irrigation water volume mainly focus on the maturity stage. However, as water shortage becomes more severe, not only does irrigation water volume during the maturity stage decrease significantly, but irrigation water volume during the middle growth periodalso starts to be affected. This underscores the need for more precise irrigation water allocation and management to ensure the basic growth and yield of spring maize under water shortage conditions.
The optimal water allocation for different growth stages of summer maize in the Guanzhong region under various water shortage conditions is depicted in Figure 9.
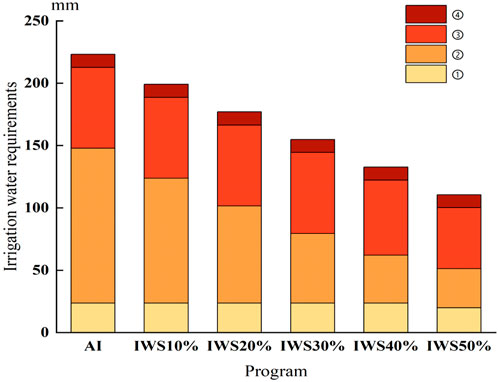
Figure 9. Optimization plan for irrigation water in Guanzhong (①Initial growth period; ②Fast development period; ③Middle growth period; ④Maturity).
Under the IWS10% scenario, irrigation management for summer maize is relatively lenient. The irrigation water percentages during the initial growth period, fast development period, middle growth period, and maturity stages respectively account for 11.91%, 50.22%, 32.57%, and 5.30% of the total irrigation volume. Compared to the AI scenario, the irrigation water volume remains unchanged for the initial growth period but decreases by 24.1 mm for the fast development period, with no change for the middle growth period and maturity stages. Summer maize needs a lot of water during its fast development period, which is very important for its growth. The irrigation percentages during the middle growth period and maturity stages are 32.57% and 5.30%, respectively. While irrigation water volume decreases for each growth stage compared to the AI scenario, overall, normal growth of summer maize can still be ensured.
As the degree of water shortage increases, significant changes occur in irrigation water allocation. Under the IWS20% scenario, the irrigation water percentages during the initial growth period, fast development period, middle growth period, and maturity stages respectively become 14.89%, 37.77%, 40.72%, and 6.63% of the total irrigation volume. Compared to the AI scenario, irrigation water volume decreases by 63.94 mm for the fast development period, with no change for other stages. Under the IWS30% scenario, the irrigation water percentages during the initial growth period, fast development period, middle growth period, and maturity stages respectively become 17.01%, 29.42%, 46.53%, and 7.57% of the total irrigation volume. Compared to the AI scenario, irrigation water volume decreases by 83.11 mm for the fast development period, with no change for other stages. The irrigation volume gradually decreases during the fast development period while increasing during the middle growth period, as summer maize becomes more reliant on irrigation during the middle growth periodto ensure normal development of the ears under reduced water availability.
When the water shortage scenario reaches IWS40%, the irrigation water percentages during the initial growth period, fast development period, middle growth period, and maturity stages respectively become 18.10%, 28.51%, 44.56%, and 8.83% of the total irrigation volume. Compared to the AI scenario, irrigation water volume decreases by 90.06 mm for the fast development period and shows noticeable decreases for the initial growth period and middle growth period. This indicates severe restrictions on summer maize growth under severe water shortage conditions, necessitating strict control over irrigation water volume during each growth stage.
In the extreme water shortage scenario of IWS50%, the irrigation water percentages during the initial growth period, fast development period, middle growth period, and maturity stages respectively become 17.75%, 27.96%, 43.70%, and 10.60% of the total irrigation volume. Compared to the AI scenario, irrigation water volume decreases by 96.29 mm for the fast development period and shows significant decreases for the middle growth period. This demonstrates the need for stringent control over irrigation water volume during each growth stage to ensure the basic growth requirements of summer maize under extreme water shortage conditions.
Under different water shortage conditions, the optimal water allocation for each growth stage of spring maize in the Shannan region is illustrated in Figure 10.
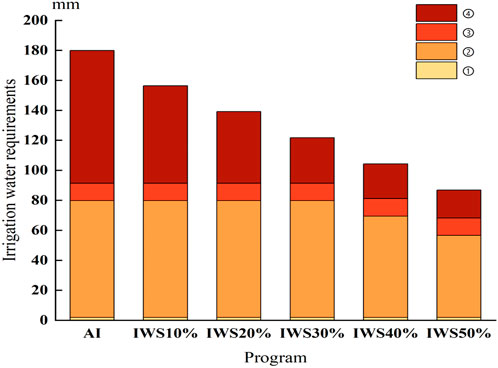
Figure 10. Optimization plan for irrigation water in Shannan (①Initial growth period; ②Fast development period; ③Middle growth period; ④Maturity).
Under the IWS10% scenario, irrigation water for the initial growth period, fast development period, middle growth period, and maturity stages respectively accounts for 1.24%, 49.76%, 7.44%, and 41.56% of the total irrigation volume. Compared to full irrigation, there is no reduction in irrigation water for the initial growth period, fast development period, and middle growth period, while irrigation water during the maturity stage decreases by 23.41 mm. As the water shortage intensifies to IWS20%, irrigation water for the initial growth period, fast development period, middle growth period, maturity respectively becomes 1.55%, 62.20%, 9.31%, and 26.95% of the total irrigation volume. Compared to full irrigation, irrigation water during the maturity stage decreases by 54.71 mm, while irrigation water for other stages remains unchanged. With further increase in water shortage to IWS30%, irrigation water for the initial growth period, fast development period, middle growth period, maturity respectively becomes 1.77%, 65.20%, 10.63%, and 22.40% of the total irrigation volume. Compared to full irrigation, irrigation water for the fast development period decreases by 6.45 mm, and for the maturity stage by 63.91 mm. When the water shortage reaches IWS40%, irrigation water for the initial growth period, fast development period, middle growth period, maturity respectively becomes 2.06%, 63.66%, 12.41%, and 21.87% of the total irrigation volume. Compared to full irrigation, irrigation water for the fast development period decreases by 18.10 mm, and for the maturity stage by 67.91 mm. Under the IWS50% scenario, irrigation water for the initial growth period, fast development period, middle growth period, maturity respectively becomes 2.48%, 61.51%, 14.89%, and 21.13% of the total irrigation volume. Compared to full irrigation, irrigation water for the fast development period decreases by 29.75 mm, and for the maturity stage by 71.91 mm.
In summary, under mild water shortage conditions, irrigation strategies mainly focus on meeting the water requirement during the maturity stage. However, as the water shortage worsens, irrigation strategies adjust by reducing water usage during other growth stages, prioritizing the water requirement during the fast development period and maturity stages.
When analyzing the impact of different irrigation water shortages on the yield of spring maize in the Shaanxi region, significant differences in yield reduction rates were observed among Shanbei, Guanzhong, and Shannan, as shown in Table 5. These differences are not only related to the extent of reduction in irrigation water but also closely related to the crop growth characteristics, sensitive periods to water, and adjustments in irrigation strategies in each region.
Under the IWS10% scenario, the reduction rates in the Shanbei, Guanzhong, and Shannan are relatively low, at 1.92%, 3.54%, and 3.14%, respectively. This indicates that under mild water shortages, the impact on spring maize yield is minimal. However, as the irrigation water shortage increases, the reduction rates gradually rise. When the irrigation water shortage reaches IWS20%, the reduction rates in the Shanbei, Guanzhong, and Shannan increase to 4.22%, 7.49%, and 6.22%, respectively. It can be observed that the increase in reduction rate in the Guanzhong region is significant, which may be due to the higher sensitivity of summer maize in this region to water. With further exacerbation of the water shortage to the IWS30% scenario, the reduction rates in Shanbei, Guanzhong, and Shannan reach 7.14%, 12.51%, and 10.54%, respectively. At this point, the reduction rate in the Guanzhong region is significantly higher than that in Shanbei and Shannan, indicating a stronger sensitivity of spring maize to water shortage in this region. Under even more severe water shortage conditions, namely IWS40% and IWS50%, the reduction rates in the Shanbei, Guanzhong, and Shannan continue to increase. Particularly in the Guanzhong region, under the IWS50% scenario, the reduction rate reaches 27.57%, becoming the most severely affected area among the three regions.
As shown in Figure 11, “I” represents the optimized model, while “II” represents the unprocessed model. The maize reduction rates after model processing are much lower than those without model processing, where irrigation water is proportionally allocated. This indicates that through scientific adjustments and optimization of irrigation strategies, it is possible to effectively reduce maize reduction rates under the same reduced irrigation water conditions. The optimal solutions obtained after model processing show that, under water shortage conditions, the Guanzhong region suffers the most severe reduction in yield. This is mainly because the summer maize in the Guanzhong region has a high sensitivity index to water, especially during the fast development period, where the requirement for water and sensitivity are significant. Therefore, under water shortage conditions, the yield of summer maize in the Guanzhong region is more easily affected. In comparison, spring maize in Shanbei and Shannan can adjust irrigation strategies to prioritize the water supply for growth stages with higher water requirement, thereby mitigating the impact of water shortage on yield to some extent.
In summary, the application of irrigation optimization models can effectively reduce reduction rates while also allowing for the development of more scientifically reasonable irrigation strategies tailored to the characteristics of different regions and maizes to address the challenge of water scarcity. This is of great significance for ensuring food security and improving the efficiency of agricultural water resource utilization.
5 Discussions
5.1 Feasibility analysis
Factors affecting maize water requirements in the field include meteorological conditions (Msowoya et al., 2016; Zakaria, 2017), soil properties (Silva et al., 2020; Balnco-Canqui et al., 2012), maize cultivation structure (Zhang et al., 2020; Huang et al., 2017), field management measures (Shinde et al., 2020), and so on. The water requirements of maizes vary greatly at different growth stages. In Shaanxi Province, spring maize and summer maize planting face significant seasonal climate differences due to different sowing times. Spring maize is planted at the end of March or early April, when precipitation is generally low in spring, resulting in a greater requirement for water during the initial growth period. Summer maize is planted in early June, when summer precipitation is abundant, which to some extent reduces the water requirement of maizes. Therefore, in the initial growth period, the irrigation water requirement of summer maize planted in the Guanzhong region is significantly lower than that of spring maize planted in the northern and southern regions of Shaanxi due to more precipitation. This difference is not only reflected in the irrigation water requirements but also in the overall growth condition of maizes. In Shanbei and Shannan, due to insufficient spring precipitation, spring maize often requires more irrigation during the initial growth period to ensure normal growth. In the Guanzhong region, however, summer maize benefits from abundant summer precipitation and relatively higher soil moisture content, resulting in better growth conditions.
The difference in sowing time leads to differences in the number of days, rainfall, evapotranspiration, and other factors during the growth stages. This, in turn, results in significant differences in the proportion of irrigation water in the entire growth stage for the same growth stage. Taking summer maize in the Guanzhong region and spring maize in Shanbei and Shannan as examples, although they all go through the maturity stage, the irrigation requirement during this stage varies significantly due to different sowing times. During the maturity stage, summer maize in the Guanzhong region requires only 4.8% of the total irrigation water due to abundant summer rainfall and relatively high soil moisture content. In contrast, spring maize in Shanbei and Shannan requires 58.8% and 50.9% of the total irrigation water, respectively, due to less spring precipitation and lower soil moisture content. This difference in irrigation requirement should take into account the difference in water sensitivity index and sowing time when allocating irrigation water. The water sensitivity index reflects the sensitivity of maizes to water at different growth stages, while sowing time differences determine the rainfall, evapotranspiration, and other conditions at different growth stages. Only by considering these two factors comprehensively can we formulate more scientifically sound irrigation plans to ensure that maizes receive adequate water supply at all growth stages, thereby achieving high yields and efficiency.
According to the “Water Consumption Quota for Industrial Water Use in Shaanxi Province” data, the calculated irrigation water requirement in this paper is close to the data in the quota, particularly in the 75% hydrological year. However, there is a significant difference in the 25% hydrological year. This is mainly because some areas in the quota have zero irrigation quotas, while actual investigations have found that these areas still require partial supplementary irrigation. Therefore, the calculated results in this paper are relatively larger than those in the quota. Other research results (Shang et al., 2022; Liu et al., 2021) also show that the calculated irrigation water requirement in this paper is similar to the results of existing studies, indicating that the research results in this paper are relatively reliable and can provide basic support for the formulation and management of irrigation quotas in maize planting areas in Shaanxi Province.
5.2 Policy recommendations
(1) In Shaanxi Province, maize production accounts for 49.51% of agricultural grain production. When facing a shortage of irrigation water and aiming to minimize the reduction in yield, the optimal allocation of irrigation water should prioritize ensuring water supply during the middle growth period, fast development period, and initial growth period for spring maize in Shanbei and Shannan, while for summer maize in the Guanzhong region, irrigation water should be ensured during the initial growth period, middle growth period, and maturity stages.
(2) The maize planting area in Shaanxi Province accounts for 24.30% of the sown area of grain maizes, with the majority of suitable planting areas located in the Guanzhong region. Under the same water shortage conditions, the Guanzhong region has the highest reduction rate in yield, followed by Shannan, and the lowest in Shanbei. To ensure the maize yield in Shaanxi Province, irrigation water for maize in the Guanzhong region must be prioritized.
(3) The advancement of science and technology drives the continuous improvement of water-saving irrigation techniques. Combining the climatic characteristics, geological conditions, and hydrological conditions of different regions in Shaanxi Province, suitable irrigation techniques should be selected to promote the increase in maize yield and quality, thus achieving efficient water-saving in the region and high yield and production of maizes.
5.3 Outlook
The article analyzes the spatial and temporal distribution of effective rainfall, water requirement, and irrigation water requirement in Shaanxi Province. It also explores the optimal allocation of irrigation water under conditions of insufficient water supply, aiming to minimize grain yield reduction. This analysis provides some theoretical support for maize cultivation in Shaanxi Province to cope with drought to a certain extent. However, in calculating the overall water requirement for maize cultivation in the province, the impact of terrain and topography in different regions of Shaanxi Province has not been considered, nor have factors such as cropping structure, crop rotation techniques, and the use of agrochemicals on soil water structure, soil nutrient content, and their influence on crop growth trends. Further research is needed to delve into these aspects in future studies.
6 Conclusion
The study analyzed the spatiotemporal distribution characteristics of effective rainfall, water requirement, and irrigation water requirement for maize in Shaanxi Province, based on meteorological data from 34 weather stations in the province combined with the crop coefficient method. The ideal water quantity under full irrigation in Shaanxi Province was obtained from the basic data. However, considering the scarcity of water resources in the region, agricultural water shortages are a challenge. Therefore, this study, based on the ideal irrigation water quantity, set different water scarcity scenarios. It employed the Jensen model to construct an optimization model with the objective of minimizing grain yield reduction, and solved it using a genetic algorithm to obtain optimized irrigation regimes for maize under different water scarcity scenarios. The main conclusions are as follows:
(1) The effective rainfall during the maize growing seasons in Shaanxi Province from 1960 to 2019 ranged from 206.05 to 447.93 mm, showing a slight increasing trend. The water requirement for maize ranged from 546.46 to 703.42 mm, showing a decreasing trend, similar to the trend observed for irrigation water requirement, which also decreased over time. The maximum irrigation water requirement occurred in 1997, reaching 476.45 mm, while the minimum was in 1983, at only 139.02 mm. This variation may be attributed to abundant rainfall and sufficient soil moisture in 1983.
The spatial distribution of irrigation water requirement for maize in Shaanxi Province showed a decreasing trend from north to south. High-demand areas were mainly located in the northern parts of Yulin and Yan’an cities in northern Shaanxi. These areas are typically located on the Loess Plateau, characterized by arid climates and scarce rainfall, leading to a high dependency on irrigation for maize growth. Low-demand areas were distributed in Ankang City in Shannan. The humid climate and abundant rainfall in Shannan provide relatively sufficient natural water supply for maize growth, resulting in lower irrigation water requirement in these areas. This distribution pattern is closely related to the climate conditions, topography, and maize planting structure in Shaanxi Province.
(2) For spring maize in Shanbei and Shannan, it is crucial to ensure water supply during the middle growth period, fast development period, and initial growth periods, while irrigation water during the maturation stage can be moderately reduced. When irrigation water is reduced by 30%, Shannan needs to reduce irrigation water during the fast development period and maturation stages, while for summer maize in the Guanzhong region, irrigation during the fast development period is reduced first under water shortage conditions. When facing severe water shortages, irrigation during the initial growth period, fast development period, and middle growth period is successively reduced. Under the same water scarcity conditions, the reduction in yield in the Guanzhong region is much greater than in Shanbei and Shannan.
(3) From the analysis of yield reduction rates, under the same water scarcity conditions, the Guanzhong region had the highest reduction rate, followed by Shannan, and Shanbei had the lowest reduction rate. This is because when facing water shortages, spring maize in Shanbei and Shannan first reduces irrigation during the maturation stage with a water sensitivity index of 0.10, while summer maize in the Guanzhong region reduces irrigation during the fast development period with a water sensitivity index of 0.17. The higher the water sensitivity index, the higher the reduction in yield under water scarcity conditions. Therefore, under the conditions of IWS30% (30% irrigation water shortage), IWS40% (40% irrigation water shortage), and IWS50% (50% irrigation water shortage), the reduction rate in Shannan is significantly higher than in Shanbei, even though both regions plant spring maize.
Data availability statement
The original contributions presented in the study are included in the article/supplementary material, further inquiries can be directed to the corresponding author.
Author contributions
KC: Conceptualization, Data curation, Methodology, Resources, Validation, Visualization, Writing–original draft. KL: Software, Supervision, Validation, Writing–review and editing. XF: Conceptualization, Formal Analysis, Writing–review and editing.
Funding
The author(s) declare financial support was received for the research, authorship, and/or publication of this article. This work was supported by Scientific Research Project of the PowerChina ChongQing Engineering Corporation Limited [QKY2023-CQ-02-03].
Acknowledgments
We sincerely thank all individuals and institutions that have contributed to this research. Special thanks go to PowerChina ChongQing Engineering Corporation Limited for providing the (QKY2023-CQ-02-03) project funding, which is crucial to our research. We are deeply grateful for the use of NSGA-II in our article; it has made us more efficient in solving single-objective problems and has provided strong support for exploring the optimization of corn irrigation scheduling in Shaanxi Province. We appreciate the technical support throughout the research process, which played a key role in the successful completion of the article. Finally, we express our sincere gratitude to the editors and reviewers of Frontiers for their valuable comments and suggestions, which greatly improved the quality of our paper.
Conflict of interest
Authors KC, KL, and XF were employed by PowerChina HuaDong Engineering Corporation Limited. Authors KC, KL, and XF were employed by PowerChina ChongQing Engineering Corporation Limited.
Publisher’s note
All claims expressed in this article are solely those of the authors and do not necessarily represent those of their affiliated organizations, or those of the publisher, the editors and the reviewers. Any product that may be evaluated in this article, or claim that may be made by its manufacturer, is not guaranteed or endorsed by the publisher.
References
Allen, R. G., Pereira, L. S., Raes, D., and Smith, M. (1998). Crop evapotranspiration-guidelines for computing crop water requirements-FAO irrigation and drainage paper 56. Rome: Fao.
Ashkan, T., Naghi, A. Z., and Mohammadreza, S. N. (2023). Irrigation scheduling of walnut seedlings using HYDRUS-1D and Taguchi optimization approach. J. Irrigation Drainage Eng. 149 (1). doi:10.1061/(ASCE)IR.1943-4774.0001735
Blanco-Canqui, H., Claassen, M. M., and Presley, D. R. (2012). Summer cover crops fix nitrogen, increase crop yield, and improve soil–crop relationships. Agron. J. 104 (1), 137–147. doi:10.2134/agronj2011.0240
Gao, F. K., Wang, L., Li, X. G., Ning, H. F., Han, Q. S., Liu, H., et al. (2023). Effects of spring lrrigation on water and salt distribution in soil and cotton growth in southern Xinjiang. J. Irrigation Drainage 42 (01), 54–63. doi:10.13522/j.cnki.ggps.2022404
Gu, X., Liu, K., Gao, J., and Liu, L. J. (2022). Research advances of water-saving lrrigation methods and their influences on rice yield. Hybrid. Rice 37 (02), 7–13. doi:10.16267/j.cnki.1005-3956.20210715.256
Guo, Y. B., Feng, H., and Wu, P. T. (2007). Research progress on deficit irrigation diagnosis index of crop. Chin. Agric. Sci. Bull. (08), 520–525. doi:10.3969/j.issn.1000-6850.2007.08.114
Guo, Y. F., Huo, Y. Z., and Wang, W. D. (2017). A study on water consumption law and lrrigation regime for alfalfa. Water Sav. Irrig. (03), 8–10+13. doi:10.3969/j.issn.1007-4929.2017.03.003
Huang, S. B., Gao, Y. B., Li, Y. B., Xu, L. N., Tao, H. B., Wang, P., et al. (2017). Influence of plant architecture on maize physiology and yield in the Heilonggang River valley. Crop J. 5 (1), 52–62. doi:10.1016/j.cj.2016.06.018
Kang, S. Z., Zhang, X. L., He, Z. Z., and Zhang, X. (1990). Research on the isoline map of corn water requirement and division of irrigation zone in shaanxi povince. J. Water Resour. Water Eng. (03), 19–34.
Li, H. M., and Fan, J. Z. (2015). Analysis on meteorological drought characteristics of corn growing period in Guanzhong Summer. J. Shaanxi Meteorology (04), 1–5.
Li, T., Kang, S. Z., and Su, X. L. (2005). Advances in research on the crop optimal irrigation scheduleand water allocating models. J. Northwest A and F Univ. Sci. Ed. (12), 148–152+158. doi:10.13207/j.cnki.jnwafu.2005.12.031
Liang, W. Q., Cai, H. J., and Wang, J. (2011). Experimental study on crop coefficient of maize in Guanzhong region of shaanxi province. Water Sav. Irrig. (12), 1–4.
Liu, H., Zhang, X. L., Wang, Y. X., Guo, Y., Luo, J. M., and Shen, Y. J. (2021). Spatio-temporal characteristics of the hydrothermal conditions in the growth period andvarious gro wth stages of maize in China from 1960 to 2018. Chin. J. Eco-Agriculture 29 (08), 1417–1429. doi:10.13930/j.cnki.cjea.201009
Ma, C., Wu, T. A., Zhang, W. Z., Li, J., and Jiao, X. Y. (2024). Optimization of multi-objective irrigation schedule for rice based on AquaCrop model. J. Irrigation Drainage 43 (01), 9–16. doi:10.13522/j.cnki.ggps.2023279
Martínez-Romero, A., López-Urrea, R., Montoya, F., Pardo, J. J., and Domínguez, A. (2021). Optimization of irrigation scheduling for barley crop, combining AquaCrop and MOPECO models to simulate various water-deficit regimes. Agric. Water Manag. 258, 107219. doi:10.1016/J.AGWAT.2021.107219
Msowoya, K., Madani, K., Davtalab, R., Mirchi, A., and Lund, J. R. (2016). Climate change impacts on maize production in the warm heart of africa. Water Resour. Manag. 30 (14), 5299–5312. doi:10.1007/s11269-016-1487-3
Qin, X. J., Wang, Y., and HangFu, H. L. (2016). Summary of comparative experiment of spring maize with different sowing dates in Weibei upland of Shaanxi province. Agric. Technol. 36 (02), 77. doi:10.11974/nyyjs.20160133062
Reta, B. G., Hatiye, S. D., and Finsa, M. M. (2024). Crop water requirement and irrigation scheduling under climate change scenario, and optimal cropland allocation in lower kulfo catchment. Heliyon 10 (10), e31332. doi:10.1016/J.HELIYON.2024.E31332
Shang, M. F., Zhao, J. C., Han, T., Li, S., Wang, K. C., Gao, Z. Z., et al. (2022). Temporal and spatial variation of maize water requirement and water surplus and deficitin China from 1961 to 2020. J. China Agric. Univ. 27 (04), 22–30. doi:10.11841/j.issn.1007-4333.2022.04.02
Shinde, M. G., Pawar, D. D., Kale, K. D., and Dingre, S. K. (2020). Performance of cabbage at different irrigation levels under drip and microsprinkler irrigation systems. Irrigation Drainage 70 (4), 581–592. doi:10.1002/IRD.2557
Silva, P. C. G., Tiritan, C. S., Echer, F. R., Cordeiro, C. F. S., Rebonatti, M. D., and Santos, C. H. (2020). No-tillage and crop rotation increase crop yields and nitrogen stocks in sandy soils under agroclimatic risk. Field Crops Res. 258, 107947. doi:10.1016/j.fcr.2020.107947
Wang, B., Fan, C., Bai, L. Q., Wang, J., and Wu, C. L. (2022). Precise management mode of controlled rice irrigation in Changgang lrrigationDistrict of Heilongiiang Province. Water Resour. Dev. Res. 22 (09), 35–40. doi:10.13928/j.cnki.wrdr.2022.09.008
Wang, H. B., Tian, J. C., Song, L. L., and Wang, C. J. (2014). Research on the theories and process of crop optimal lrrigation schedules. China Rural Water Hydropower (06), 21–25. doi:10.3969/j.issn.1007-2284.2014.06.006
Wang, J. X., Xing, X. J., Zhang, L. J., and Liu, Y. K. (2011). Empirical research on the change of irrigation management patterns and its impacts on crop water use. Geogr. Res. 30 (09), 1683–1692. doi:10.11821/yj2011090012
Wang, K. Q., Fu, Q., Ji, F., and Xu, S. Q. (2007). Study on rice water production function and optimization of lrrigation schedule of semiarid area of western Heilongjiang province. Water Sav. Irrig. (08), 48–51. doi:10.3969/j.issn.1007-4929.2007.08.017
Wang, X. D., Ma, X. Q., Xu, Y., and Chen, C. (2013). Temporal analysis of the crop water surplus deficit indexfor the whole growth period in the huaihe basin. Resour. Sci. 35 (03), 665–672.
Xiao, J. F., Liu, Z. D., and Chen, Y. M. (2008). Study on the water requirement and water requirement regulation of maize in China. J. Maize Sci. (04), 21–25.
Yao, D. L., Gao, F., Li, Z. J., and Hu, T. T. (2018). Water supply and requirement of winter wheat and summer maize in Yangling. Agric. Res. Arid Areas 36 (01), 115–120+163. doi:10.7606/j.issn.1000-7601.2018.01.18
Zakaria, M. S. (2017). Climatic variables:Evaporation, sunshine, relative humidity,soil and air temperature and its adverse effects on cotton production. Inf. Process. Agric. 5 (1). doi:10.1016/j.inpa.2017.09.006
Zhang, J., Zhang, H., Chen, D. D., Li, G. L., Zhang, J. T., Zang, H. Z., et al. (2020). Spatial and temporal distribution characteristics of effective rainfall and water requirement of summer maize growth period in henan province. J. Henan Agric. Sci. 49 (09), 173–180. doi:10.15933/j.cnki.1004-3268.2020.09.022
Zhang, L., Chen, F., and Lei, Y. (2020). Climate change and shifts in cropping systems together exacerbate China’s water scarcity. Environ. Res. Lett. 15 (10), 104060. doi:10.1088/1748-9326/abb1f2
Zhang, L., Zhou, W., and Li, D. X. (2019). Research progress in irrigation mode selection of high-efficiency water-saving agriculture. J. Drainage Irrigation Mach. Eng. 37 (05), 447–453. doi:10.3969/j.issn.1674-8530.18.0135
Keywords: water resources, irrigation water requirement, Jensen model, irrigation scheduling optimization, Shaanxi province
Citation: Chen K, Liu K and Fang X (2024) Study on optimization of maize irrigation scheduling in Shaanxi Province. Front. Environ. Sci. 12:1466691. doi: 10.3389/fenvs.2024.1466691
Received: 18 July 2024; Accepted: 25 October 2024;
Published: 06 November 2024.
Edited by:
Xudong Huang, North China University of Water Conservancy and Electric Power, ChinaCopyright © 2024 Chen, Liu and Fang. This is an open-access article distributed under the terms of the Creative Commons Attribution License (CC BY). The use, distribution or reproduction in other forums is permitted, provided the original author(s) and the copyright owner(s) are credited and that the original publication in this journal is cited, in accordance with accepted academic practice. No use, distribution or reproduction is permitted which does not comply with these terms.
*Correspondence: Xudong Fang, ZmFuZ194ZEBoZGVjLmNvbQ==