- 1Key Laboratory of Arid Climatic Change and Reducing Disaster of Gansu Province, Key Open Laboratory of Arid Climate Change and Disaster Reduction of CMA, Institute of Arid Meteorology, China Meteorological Administration, Lanzhou, China
- 2National Climate Centre, China Meteorological Administration, Beijing, China
- 3Lanzhou Regional Climate Center, Lanzhou, China
Drought, which is severe and recurring in Northern China, is the most significant meteorological disaster on a worldwide scale. However, a definitive conclusion has not yet been reached on the multi-scale drought features in Northern China under a warming context. Drought is affected by several circulation-related variables and a deeper scientific comprehension of their synergistic effects is required. This research addresses the spatiotemporal aspects of summer drought and its causes in the northern drought-prone belt of China (NDPB), based on meteorological observation and data reanalysis. The primary findings indicate that the NDPB is very vulnerable to drought, with the uniform mode being the region’s most significant spatial mode associated with summer droughts. Inter-annual variations are the primary cause of the summer drought in the NDPB when considering multi-scale changes. Regional drought has typically declined during this century because of the effect of multi-decadal scale changes, which cannot be disregarded. Considering the circulation background field, during summer drought years in the NDPB, the South Asian high is weaker and moves eastward, while the high-level westerly jet is weaker and more northerly. From Lake Baikal to Northern China, there is an anomalous anticyclonic circulation, and the mid-level subtropical high in the western Pacific is more southerly and easterly. As a result, the NDPB is governed by the unusual northerly circulation. The Mongolian and northeastern surface cyclone activity is decreasing, the low-level warm and humid airflow is feeble, and the NDPB is not experiencing anomalous water vapor transport. In the high-, middle-, and low-level arrangement, the NDPB circulations diverge at low levels and converge at high levels—unusual sinking in the vertical field results in regular droughts. Multiple circulation parameters on inter-annual, inter-decadal, and multi-decadal scales interact synergistically to impact summer droughts in NDPB. However, the synergistic elements that mainly impact various timescales differ. They are primarily influenced by northerly circulation, westerly winds, and the combined effect of the South Asian summer monsoon on inter-annual timescales. The combined impact of the upper westerly winds, northerly circulation, and East Asian, South Asian, and plateau summer monsoons significantly influences their inter-decadal timescales. The westerly winds, northerly circulation, and East Asian, plateau summer monsoons are the primary multi-decadal factors.
1 Introduction
Meteorological disasters account for about 70% of global natural disasters, and drought disasters account for more than one-third of meteorological disasters (Qin, 2015). During 1980–2009, global economic losses caused by drought averaged US$17,33 billion per year, while average annual losses during 2010–2017 increased to US$23.13 billion, far exceeding the growth rate of losses from other meteorological disasters (Wilhite, 2000; Su et al., 2018). In the context of climate warming, with the global water cycle further accelerating, the drought risk is increasing (Zhang et al., 2011; Zhang et al., 2015; Zhang et al., 2019a), and the frequency and intensity of droughts are increasing (IPCC, 2012).
Worldwide, China is among the nations that experience drought catastrophes the most frequently and with the worst effects. Large-scale drought catastrophes have impacted crops in recent years, with an average of 20.9 million hm2 affected annually and up to 40.54 million hm2 in specific years. Between several million to over 30 million tons of grain reduction occurs every year, and the annual direct economic cost of drought might reach 44 billion yuan (Su et al., 2018). Drought catastrophes limit sustainable economic and social growth and jeopardize ecological and food security. Since severe disasters and droughts are more common in China’s northern regions, studies have focused chiefly on droughts in the region. Since the 1970s, Northern China has experienced a severe drought trend (Wei and Cao, 1998; Huang et al., 1999; Ma and Fu, 2001; Zhou et al., 2020). In addition to decreasing precipitation, warming has an irreversible effect on droughts because it increases the severity and extent of droughts by 4%–7% (Ma and Fu, 2003), and in certain places has also caused the semi-arid zone to spread 300 km to the southeast (Ma, 2005; Ma and Fu, 2005; Qu et al., 2023). The dry and semi-arid climatic pattern of the northwest cannot be altered by a little increase in precipitation despite recent warming and humidifying trends in the area (Zhang et al., 2021; Zhang et al., 2022). Abnormal droughts have been regular throughout the last 40 years from the standpoint of all of Northern China (Li et al., 2023). There have been three summers since 2021 with significant regional droughts in the northwest.
Due to the unusual northerly airflow and the downdraft of the Lake Baikal high-pressure ridge, the formation mechanism of drought in Northern China indicates that the western Pacific subtropical high is oriented southerly and eastward in the middle and high latitudes, with a “high in the west and low in the east” circulation. It is easy to trigger drought events in Northern China (Shen et al., 2012). Drought is also more likely in the northwest summer when the upper and middle troposphere has an “upper high (western-type South Asia high)–mid-high (Xinjiang high-pressure ridge or Iranian high pressure eastward extending over the Tibetan Plateau)” structure (Qian et al., 2001). Northern China’s drought strongly correlates with warm, humid airflow, and monsoon activity. The western Pacific subtropical high is oriented southward, and it is difficult for warm, humid airflow from the ocean to move farther north in years when the East Asian summer monsoon is weak. According to Zhang et al. (2003), this is also the cause of drought in Northern China. Furthermore, because most of Northern China is located in the East Asian subtropical monsoon region, as well as being influenced by the westerly circulation and the Tibetan Plateau monsoon (Ding and Li, 2016; Zhang et al., 2019b), the monsoon and westerly winds—the combined influence of circulation and its synergistic effect—affect drought formation and development (Zhang et al., 2022).
Despite several studies on the evolving formation mechanism and changing features of drought in Northern China, little research has been done on the multi-scale features of drought against the backdrop of global warming. There is a severe paucity of knowledge on the effects of various circulation parameters on drought linkage. This study investigates the synergistic effects of several circulation factors on the northern summer drought, shows the multi-scale changing characteristics of the summer drought in Northern China, and reveals the circulation background field of typical drought modes. In light of this, we offer a reference for short-term climate prediction of summer drought in Northern China, and also scientific support for catastrophe mitigation and prevention, and sustainable economic development in Northern China.
2 Study area, data, and methods
2.1 Study area
In Northern China (includes Heilongjiang, Jilin, Liaoning, Inner Mongolia, Beijing, Tianjin, Hebei, Shandong, Henan, Shanxi, Shaanxi, Gansu, Qinghai, Ningxia, and Xinjiang Provinces), which is bordered by 105°E, the summer precipitation standard deviation (June–August) is low in the west and high in the east (Figure 1). Except for the Qinghai Plateau, the precipitation standard deviation is below 50 mm in the west and above 50 mm in the east. There were more than 150 mm precipitation variances in Henan, Shandong, and Liaoning. The area east of 105°E has a substantially higher standard deviation of summer precipitation than the area to the west, indicating that, although having significantly more summer precipitation, the area east of 105°E also has a higher risk of summer drought, summer drought disasters are prone to occur, it is an obvious area prone to drought disasters (Liao and Zhang, 2015; Wang and Sheng, 2015). Accordingly, the drought-prone zone of Northern China (NDPB) is the region in Northern China east of 105°E (Han et al., 2019) and is the focus of this research (Figure 2).
2.2 Data
This article makes use of both meteorological observations and National Centers for Environmental Prediction/National Center for Atmospheric Research (NCEP/NCAR) reanalysis. During 1961–2022, precipitation data for summer (June–August) came from 427 meteorological stations in Northern China, of which we chose 263 for the NDPB (Figure 2). We took the monthly average altitude, wind, sea-level pressure, surface pressure field, and specific humidity from the NCEP/NCAR reanalysis data, covering the years 1961–2022 with a resolution of 2.5° × 2.5°.
2.3 Methods
2.3.1 Circulation synergy index (Ics)
The westerly wind, northerly circulation, and the East Asian, South Asian, and plateau monsoons influence the NDPB’s summer climate because it is situated in the mid-latitudes of the northern hemisphere, and located in the northeast of the Qinghai Tibet Plateau. The text refers to the literature (Zhang et al., 2022) and the following circulation synergy index as Equation 1:
where WI, EMI, SMI, PMI, and NI are the westerly, East Asian summer monsoon, South Asian summer monsoon, plateau summer monsoon, and northerly circulation indices, respectively, and a, b, c, d, and e are weight coefficients, they are the correlation coefficient between various circulation indices and precipitation indices. The westerly index as Equation 2 (Li et al., 2008), East Asian summer monsoon index as Equation 3 (Wang and Fan, 1999), South Asian summer monsoon index as Equation 4 (Wang et al., 2001), plateau summer monsoon index as Equation 5 (Tang et al., 1979), and northerly index as Equation 6 (Bai and Xu, 1988) are often used in studies on droughts in the northwest region (Zhang et al., 2022):
where H (gpm) is the 500-hPa geopotential height field, λ is the number of longitudes taken along the latitude circle in the 70–110°E area, and
2.3.2 Ensemble empirical mode decomposition (EEMD)
To simulate many observations, the EEMD approach averages the measurement of several decompositions and adds white noise of the correct magnitude to the original data. Then, after multiple calculations, the set is averaged, which is an improvement of the Empirical Mode Decomposition (EMD) method. The EMD is modified by averaging the ensemble following computation. More information on the EEMD approach can be found in Yang et al. (2023). This method has been applied extensively (Wu and Huang, 2009; Huang and Shen, 2014; Bi et al., 2018).
2.3.3 Other methods
Because of its ability to extract meteorological field dominating signals and represent the peculiarities of their geographical distribution, the empirical orthogonal function (EOF) is unique in this regard. For thorough instructions, see Wei (2007).
3 Results and analysis
3.1 Abnormal characteristics of summer drought in NDPB
We performed an EOF decomposition of the summer precipitation of 263 stations for 1961–2022 to elucidate the anomalous features of the summer drought in the NDPB. The first four modes contributed 16.72%, 9.14%, 7.85%, and 6.23% of the variation, respectively. The North technique was used to perform the significance test, and the results indicate that the signals of the first three modes are relatively significant. The spatiotemporal properties of the first three modes are primarily analyzed in the following.
The EOF1 mode has constant regional distribution features, except for a small area in the south of the NDPB, which suggests that the dominant mode in the NDPB is the persistent change of summer drought (Figure 3A). With a variance contribution of up to 77.5%, inter-annual changes dominate this mode when considering multi-timescale changes of the EOF1 time coefficient (PC1). In contrast, inter-decadal changes are relatively weak, contributing only 8.3% to variance, while multi-decadal changes exhibit slightly greater potency than inter-decadal changes. Moreover, summer droughts in NDPB have declined since 2000 due to the effect of multi- and inter-decadal scales (Figure 3B).
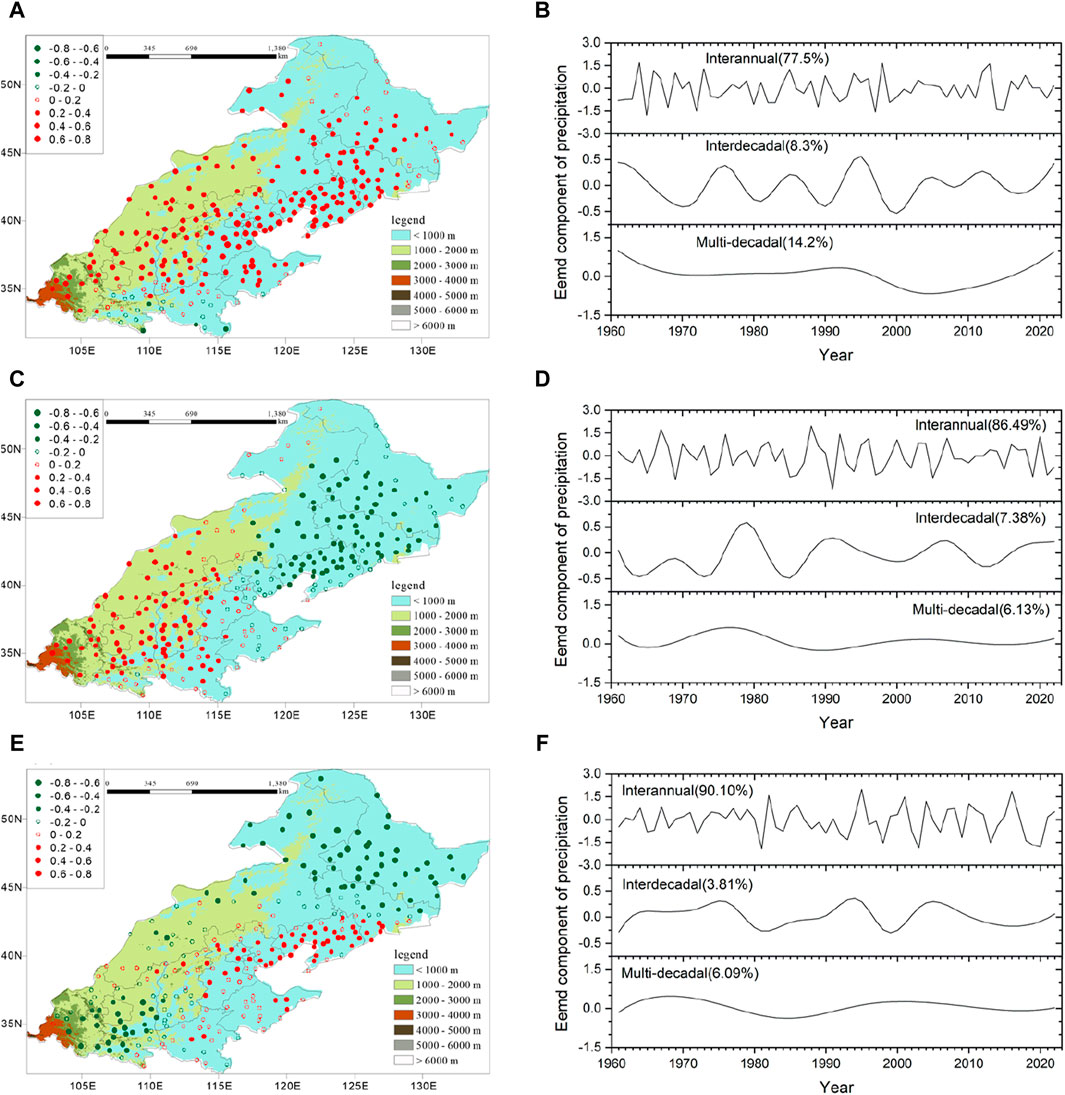
Figure 3. The modes of (A) EOF1, (C) EOF2, and (E) EOF3 and the corresponding time coefficients (B) PC1, (D) PC2, and (F) PC3 of summer precipitation in the NDPB.
The EOF2 mode displays a dipole distribution with reversal changes from southwest to northeast (Figure 3C). With a variance contribution as high as 86.49%, the time coefficient (PC2) shows that inter-annual variations are likewise the primary cause of this mode’s dominance. The multi- and inter-decadal changes are minimal in the interim. Furthermore, this mode has no discernible long-term changing trend when considering inter- and multi-decadal changes (Figure 3D). The EOF3 mode exhibits a “negative–positive–negative” tripolar distribution from southwest to northeast (Figure 3E). With a variance contribution as high as 90.1%, the time coefficient (PC3) shows that inter-annual variations are likewise the primary characteristic of this mode, which has no noticeable long-term change trend, and the inter- and multi-decadal changes are brittle (Figure 3F).
3.2 Main circulation characteristics of summer drought in the NDPB
The regional uniform mode is the most important when considering the anomalous mode of the summer drought in the NDPB. Thus, to comprehend the circulation features of the general summer drought in the NDPB, the background field of circulation corresponding to the regional uniform mode is adopted. First, we identify the abnormally dry years in the NDPB as 1972, 1999, 2015, 2000, and 1997 with PC1 standardized values less than −1.5. Next, we use a synthetic analysis to comprehend the circulation features of these years.
In summer drought years, the highest wind speed in the center of the high-altitude jet stream in the 200-hPa wind field is 28 m s−1 (Figure 4A), and the region in East Asia with wind speeds more than 24 m s−1 stretches eastward to 108°E. The jet center is situated in two latitudinal lengths north of the climatic state. The 200-hPa zonal wind anomaly in the 32–45°N latitudes of the Eurasian range is essentially consistent with negative values (Figure 4B), suggesting that one of the circulation features of the summer drought in the NDPB is the weak high-altitude jet stream and northerly.
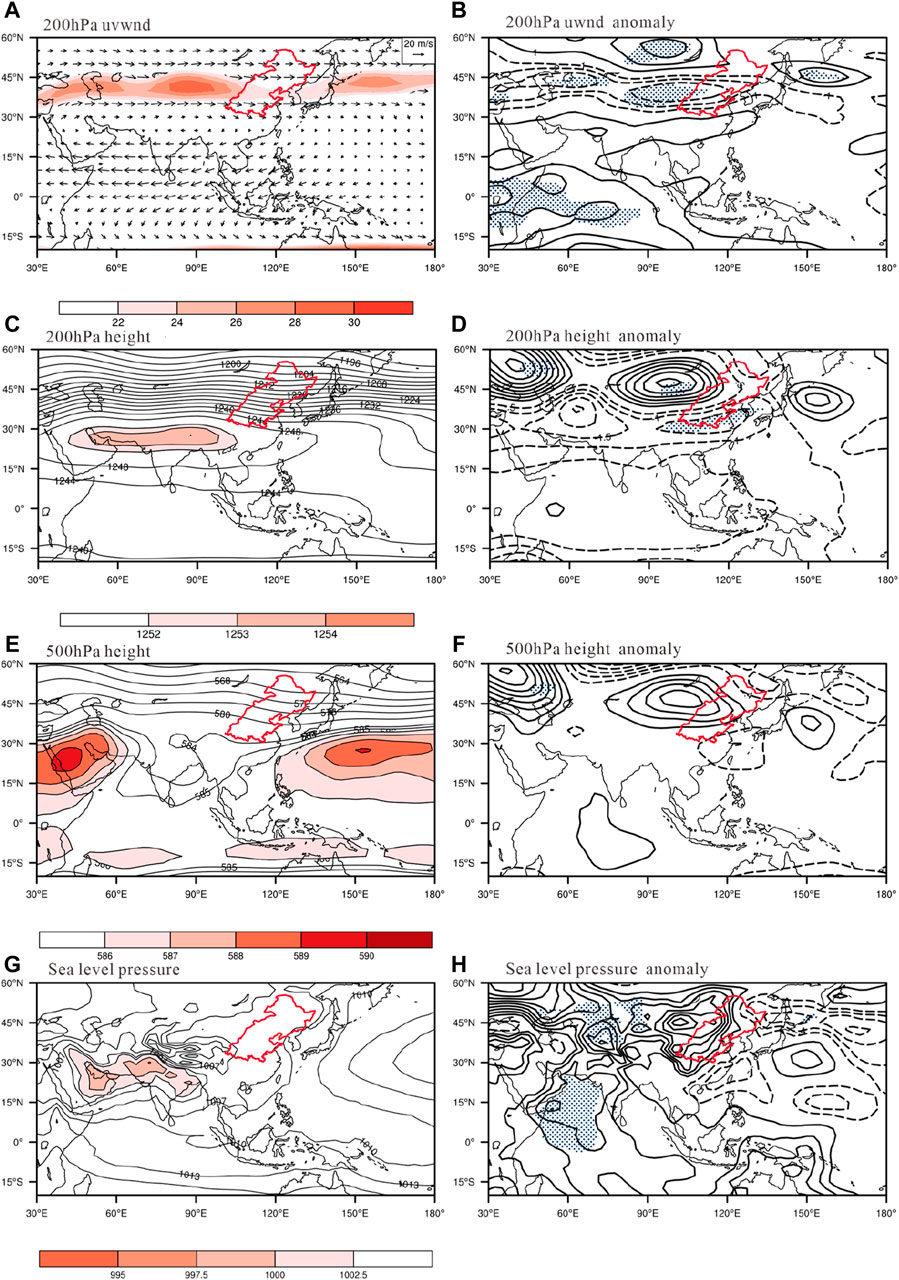
Figure 4. Fields of (A), unit: m/s) 200 hPa wind, (C), unit: dagpm) 200 hPa height, (E), unit: dagpm) 500 hPa height field, and (G), unit: hPa) sea-level pressure anomaly, and corresponding anomaly fields (B, D, F, H). The red enclosed area is the research area, the red shaded area in Figure (A) represents the region with zonal winds more than 22 m/s.
The maximum of the South Asian high-pressure center is only 1,253 dagpm (Figure 4C), which is 2 dagpm less than the climatic condition due to the effect of the high-altitude jet stream anomaly. In contrast, the 1,252 dagpm line only goes as far east as 105°E, departing from the climatic condition by six degrees of longitudes. In the 200-hPa height anomaly field, the Eurasian range consistently exhibits negative values for latitudes 10–38°N (Figure 4D). As a result, in summer drought years in the NDPB, the intensity of the South Asian high is weak and to the west.
The strength of the western Pacific subtropical high in summer drought years in the NDPB is not significantly different from the climatic state in the 500-hPa height field (Figure 4E). Three latitudinal distances south and five longitude distances east of the relative climatic state(Figure omit) correspond to the northern limit and west boundary, respectively, of the 586 dagpm line, at 31°N and 120°E. Furthermore, the NDPB is governed by an anomalous northerly airflow, and the ridge around Lake Baikal is stronger. In the 500-hPa height anomaly area, there are positive anomalies from the south of Lake Baikal to northeastern North China and negative anomalies from the western Pacific to southern China (Figure 4F).
As a result, during the NDPB’s summer drought years, the subtropical high in the western Pacific on an eastward or southward position, the Lake Baikal ridge gets more robust, and unusually north winds blow from the south of Lake Baikal to northeastern North China.
During summer drought in the NDPB, the 1005-hPa low-pressure closed contour range in Mongolia and northeastern China is much narrower than in the climatic state in the sea-level pressure field (Figure 4G). In the sea-level pressure anomaly field, consistently positive anomalies extend from Mongolia to northeastern China (Figure 4H). Therefore, during summer drought years in the NDPB, there are also abnormally few cyclones in northeast China and Mongolia.
There are two anomalous cyclonic circulations in Heilongjiang and the region extending from southern China to the west coast of Japan in the 850-hPa anomaly wind field in the summer drought years in the NDPB (Figure 5A). The area of China east of 100°E is dominated by extreme northerly winds. The whole water vapor flux and its divergence field in summer drought years (Figure 5B) reveal that the NDPB is a divergence area of water vapor transport flux with no southwesterly water vapor from the ocean.
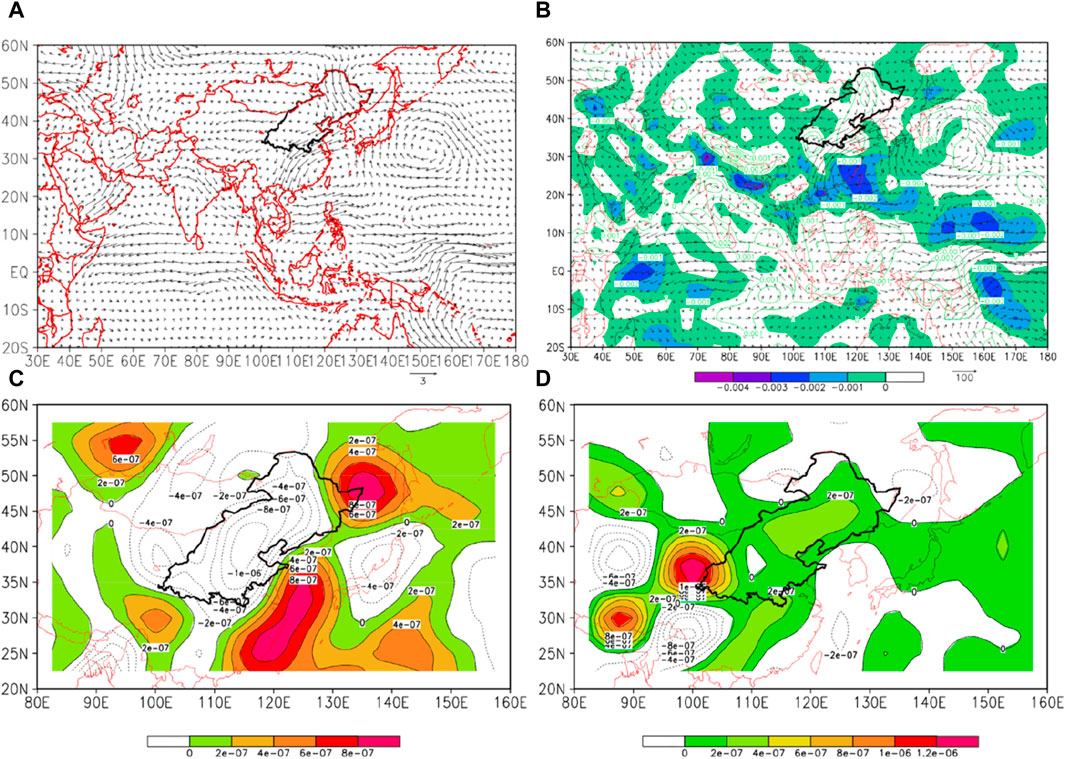
Figure 5. The 850-hPa anomaly wind field (A), unit: m/s), whole-layer water vapor flux and divergence (B), unit: kg/(ms), 200-hPa divergence field (C), unit: 10−1 s−1), and 700-hPa divergence field (D), unit: 10−1 s−1) in summer drought years in the NDPB. The black enclosed area is the research area.
Therefore, during summer drought years in the NDPB, there is no anomalous water vapor transport pathway from the ocean. Within the research region, the 200-hPa divergence field (Figure 5C) is negative, indicating convergence due to the effect of high-, medium-, and low-altitude circulation. There is divergence because the 700-hPa divergence field is positive (Figure 5D). In summer drought years in the NDPB, there is less precipitation due to atypical subsidence in the vertical field, as shown by the high- and low-level divergence.
3.3 Influence of multiple circulation factors on summer drought in the NDPB
The westerly wind, northerly circulation, and the East Asian, South Asian, and plateau monsoons influence the NDPB’s summer climate because it is situated in the mid-latitudes of the northern hemisphere, and numerous elements typically work in concert to impact circulation. We examine it using the previously developed Ics to comprehend the synergistic effect of multiple circulation parameters on summer drought in the NDPB. First, we compute the correlation coefficients between the westerly, East Asian, South Asian, plateau summer monsoon, and northerly indexes and the time coefficient (PC1) of the EOF1 mode of summer precipitation in the NDPB (Table 1).

Table 1. The correlation coefficients between the time coefficient (PC1) of EOF1 mode and circulation index.
The summer monsoon in East Asia and PC1 have a poor relationship; however, there is a substantial link with the other four indices: PC1 has a negative correlation with the northerly circulation (p < 0.001) and the plateau summer monsoon (p < 0.01), and a positive correlation with the westerly wind (p < 0.001) and the South Asian summer monsoon (p < 0.05).
Then, using circulation parameters that are substantially linked with PC1, we construct the following synergy index based on the Ics described in this paper, where each circulation index’s weight coefficient corresponds to its corresponding correlation coefficient:
It is evident that the NDPB’s dryness results from the combined impacts of the westerly wind, plateau summer monsoon, South Asian summer monsoon, and northerly circulation. Furthermore, the stronger the north wind and plateau summer monsoon, the less rainfall there is throughout summer in the NDPB. Also, the weaker the westerly and South Asian summer monsoon, the dryer is the NDPB.
We examine the regression fields of Ics with 200-hPa zonal wind and height, 500-hPa height, sea-level pressure, and 850-hPa wind to understand better the synergistic impacts of several circulation parameters on summer drought in the NDPB (Figures 6A–E). The regression coefficient is determined by taking the synergy index’s inverse. The 200-hPa zonal wind field and height field, as well as the 500-hPa height field, sea-level pressure field, and 850-hPa wind field, are quite comparable by comparing the anomaly fields in Figures 4, 5. So there is a synergistic effect of the westerly wind, South Asian monsoon, plateau monsoon, and northerly circulation on the summer dryness in the NDPB.
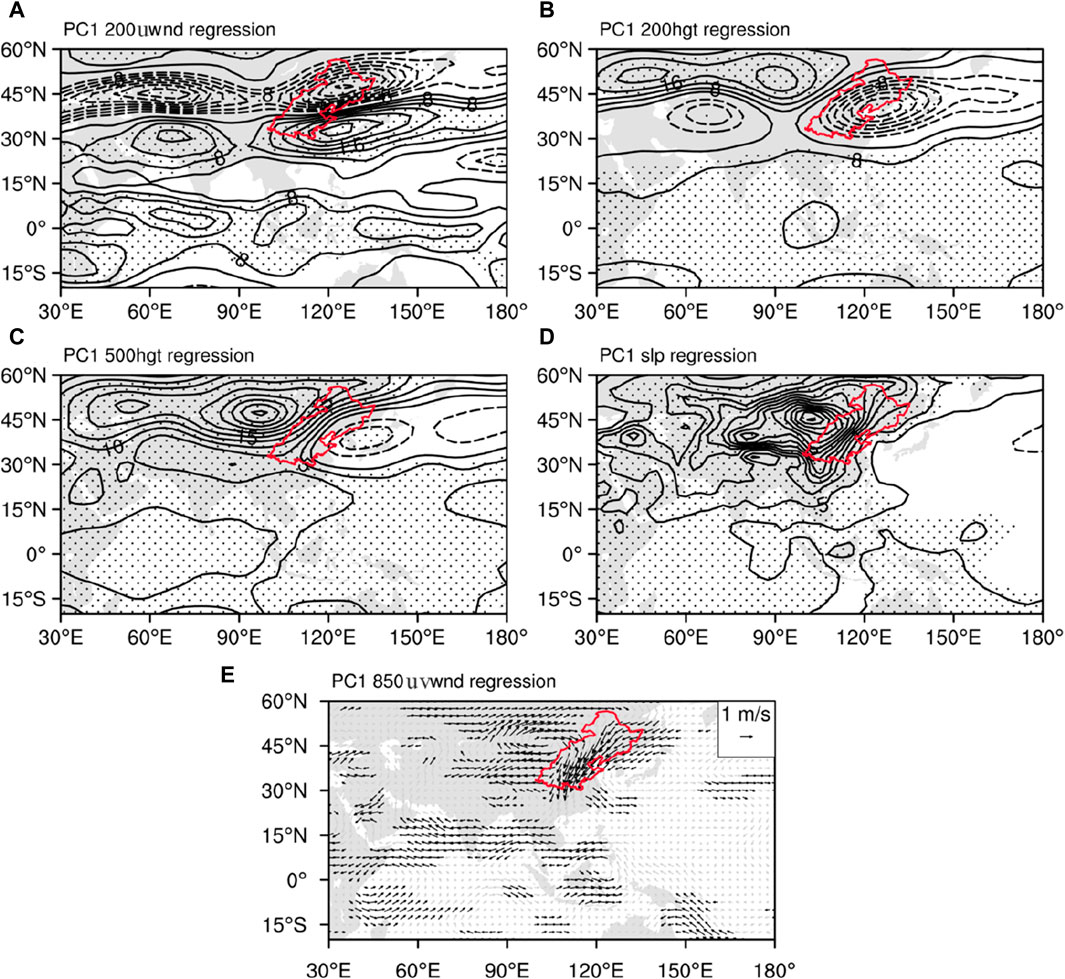
Figure 6. Regression fields of multi-circulation synergy index and (A) 200-hPa zonal wind, (B) height, (C) 500-hPa height, (D) sea-level pressure, and (E) 850-hPa wind. The red enclosed area is the study area.
While inter-annual variations dominate the constant pattern of summer precipitation in the NDPB, changes at the inter- and multi-decadal scales are also significant (Figure 3B). Stated differently, inter-annual, inter-decadal, and multi-decadal multi-timescale fluctuations are present in the summer drought.
We have already shown that the westerly wind, South Asian summer monsoon, plateau summer monsoon, and northerly circulation are the primary causes of summer dryness in the NDPB before multi-timescale decomposition. Do contributing elements for circulation vary depending on the timescale? The correlation coefficients between PC1 and the various timescale components of each circulation index are displayed in Table 2. At various timescales, the circulation elements that substantially impact the summer drought in the NDPB are not precisely the same.
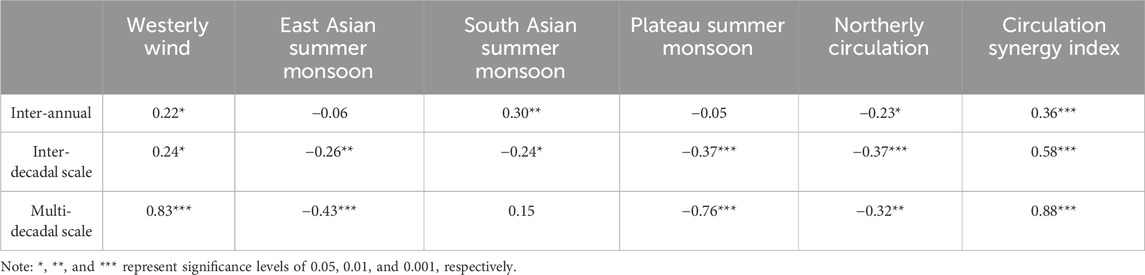
Table 2. The correlation coefficients between the time coefficient (PC1) of the EOF1 mode and the different timescale components of the circulation index.
On the inter-annual scale, westerly winds, South Asian summer monsoon, and the northerly circulation are significant. On the inter-decadal scale, westerly winds, East Asian summer monsoon, South Asian summer monsoon, plateau summer monsoon, and the northerly circulation all have substantial impacts. Except for the South Asian summer monsoon, all four circulations have notable impacts on a multi-decadal period. Furthermore, throughout a range of periods, the NDPB’s summer drought is substantially impacted differently by the same circulation system. For instance, there is a large positive correlation between the summer monsoon in South Asia and PC1 on an inter-annual basis. On the inter-decadal scale, there is a strong negative association between the two; however, on the multi-decadal range, the correlation is not significant. In the inter- and multi-decadal timeframes, there is a noteworthy inverse relationship between the East Asian summer monsoon and PC1, but this relationship is not as strong in the inter-annual range.
We establish the inter-annual, inter-decadal, and multi-decadal Ics that affect summer drought in the NDPB:
At various timescales, the correlations between PC1 and Ics are stronger than those of any one circulation component (Table 2). There may be a synergistic effect between the many circulation elements acting at different timescales and drought in the NDPB.
We examine the inter-annual, inter-decadal, and multi-decadal Ics, as well as the 200-hPa zonal wind and height, 500-hPa height, and the regression fields of sea-level pressure and 850-hPa wind, to better understand the synergy of the influence of multiple circulation factors on summer drought in the NDPB at different timescales (Figure 7). The inverse of the synergy index is also used to calculate the regression coefficient here to facilitate the comparison between the regression field and the circulation background field in drought years.
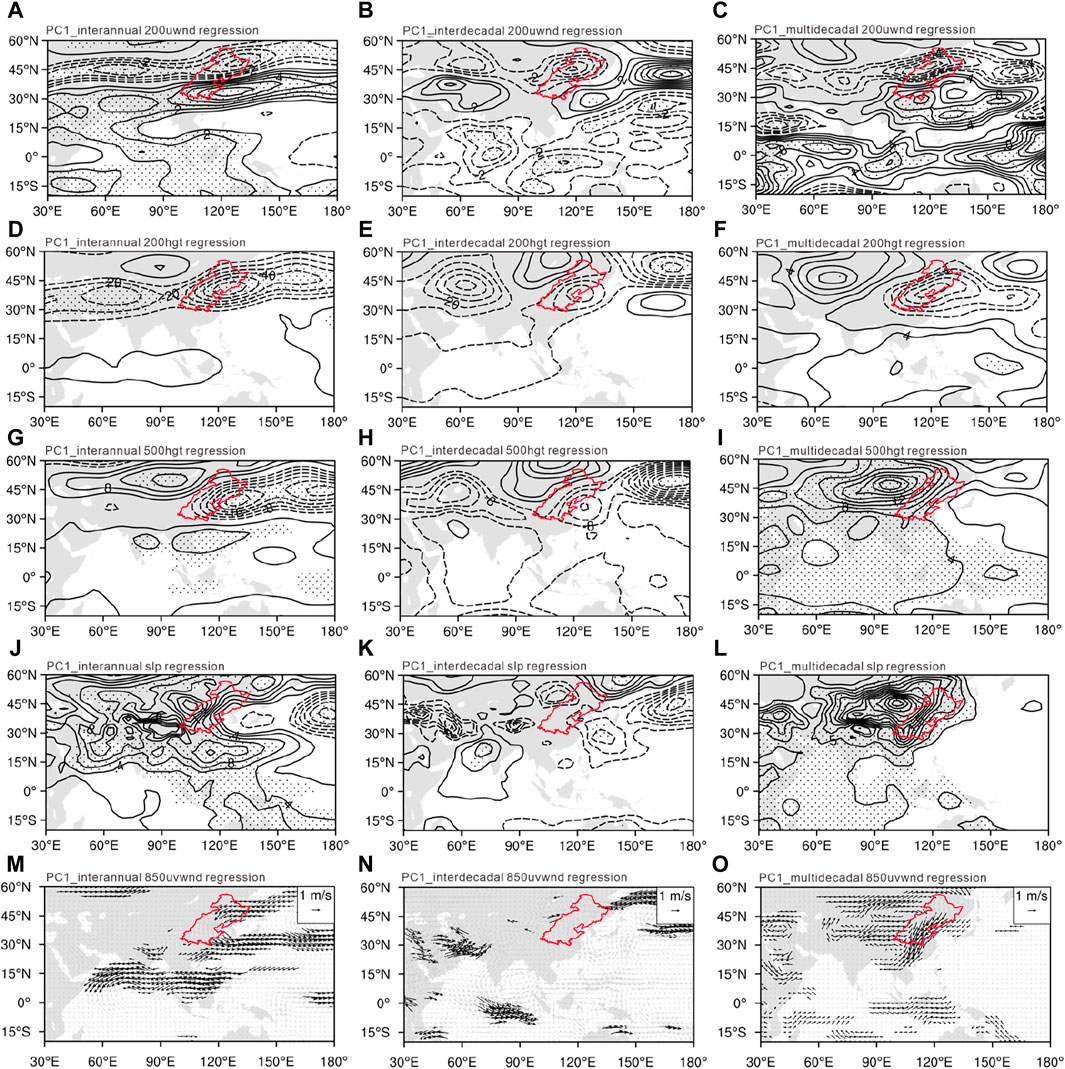
Figure 7. Regression fields of multi-circulation synergy index at different timescales and 200-hPa zonal wind (A–C), 200-hPa height (D–F), 500-hPa height (G–I), sea-level pressure (J–L), and 850-hPa wind (M–O). The red enclosed area is the study area.
From the 200-hPa zonal wind regression field to see (Figures 7A–C), at inter-annual, inter-decadal, and multi-decadal scales, it is essentially consistent with negative values in the 32–45°N latitudes of the Eurasian range. From the 200-hPa height regression field to see (Figures 7D–F), at inter-annual and inter-decadal scales, it is essentially consistent with negative values in the 32–45°N latitudes of the Eurasian range. From the 500-hPa height regression field to see (Figures 7G–I), at inter-annual and inter-decadal scales, it is essentially consistent with negative values in the 32–45°N latitudes of the Eurasian range. From the sea-level pressure regression field to see (Figures 7J–L), at inter-annual and multi-decadal scales, it is consistently positive anomalies extend from Mongolia to northeastern China. From the 850-hPa wind regression field to see (Figures 7M–O), at inter-annual, inter-decadal, and multi-decadal scales, there are two anomalous cyclonic circulations in Heilongjiang and the region extending from southern China to the west coast of Japan.
In summary, the regression field and the circulation field in drought years are quite similar. Compared to inter-decadal and inter-decadal scale, they are more similar on an inter-annual scale. Therefore, the NDPB experiences summer drought due to the synergistic impacts of several circulation components operating on distinct periods, with the inter-annual scale contributing the most.
To further clarify the main circulation impact systems of the NDPB during drought years, Figure 8 provides schematic diagrams of the main impact systems at high, medium, ground, and surface levels, as well as their contributions at different time scales.
4 Discussion and conclusion
This research examines the spatiotemporal patterns and causes of summer dryness in the NDPB for 1961–2022 based on meteorological observations and NCAR/NCEP reanalysis data. The primary findings follow.
The most critical geographical mode of the summer drought in the NDPB is the spatially consistent mode. The dipole mode with reversal change from southwest to northeast and the “negative–positive–negative” tripole mode from southwest to the northeast are its two principal spatial modes. When considering modifications across several timescales, inter-annual variations often account for most summer droughts in the NDPB; nonetheless, changes in many timescales are noticeable. Over the past century, regional droughts have diminished due to changes at multi-decadal scales.
From circulation background field to see, in summer drought years in the NDPB, the high-level westerly jet is feeble, with a northward-oriented jet axis and a westward-oriented jet center, under its influence, the intensity of the South Asia high pressure is relatively weak and its location is biased towards the east. The mid-level Pacific subtropical high is oriented southward and eastward, while the weak South Asia high is positioned eastward under its influence. From Lake Baikal to Northern China, an anomalous anticyclonic circulation occurs. The anomalous northerly airflow governs the NDPB. The NDPB is a region of divergence of water vapor transport flux without anomalous water vapor transport from the ocean because of the abnormal northerly airflow at the lower levels and the apparent decrease in surface Mongolian and northeastern cyclone activity. The upper-level circulation in the NDPB and the low-level circulation diverge under the arrangement of high-, medium-, and low-level circulation, manifesting as anomaly subsidence in the vertical field and leading to less precipitation and more droughts.
The NDPB is situated in the mid-latitudes of the northern hemisphere, where many circulations simultaneously impact the summer droughts. Several circulation parameters related to inter-annual, inter-decadal, and multi-decadal summer droughts are also affected. However, the circulation elements that substantially impact the summer droughts on various timescales are not precisely the same. The coordinated impact of the westerly, South Asian summer monsoon, and northerly circulation primarily affects them inter-annually. They are mainly impacted by the westerly winds and East Asian, South Asian, and plateau summer monsoons on an inter-decadal scale. On a multi-decadal scale, the combined impact of the westerly winds, East Asian summer monsoon, plateau summer monsoon, and northerly circulation primarily influences the collaborative influence of the northerly circulation.
It is worth noting that the same circulation system has significant differences in its impact on summer drought in the NDPB at different time scales. For instance, the summer monsoon in South Asia has a negligible impact on the multi-decadal scale, a positive contribution on the inter-annual scale, and a negative impact on the inter-decadal scale. The inter- and multi-decadal scales benefit significantly from the summer monsoon in East Asia, with a negative although not large contribution on an inter-annual scale.
The examination of the spatiotemporal features of the summer drought in the NDPB in this study is only diagnostic, and the statistical discussion of the reasons for spatially uniform circulation leaves much to be understood in terms of numerical simulation. More information is also required to understand the potential formation processes of the tripolar and polar modes. Furthermore, trustworthy forecast data must also make clear how the NDPB’s summer drought will evolve in the future.
Data availability statement
The original contributions presented in the study are included in the article/supplementary material, further inquiries can be directed to the corresponding authors.
Author contributions
JY: Data curation, Formal Analysis, Funding acquisition, Visualization, Writing–original draft, Writing–review and editing. QZ: Conceptualization, Funding acquisition, Supervision, Writing–review and editing. PW: Resources, Supervision, Writing–review and editing. PY: Conceptualization, Funding acquisition, Supervision, Validation, Writing–review and editing. YL: Data curation, Resources, Software, Writing–review and editing. ZL: Formal Analysis, Validation, Writing–review and editing. XL: Data curation, Writing–review and editing.
Funding
The author(s) declare that financial support was received for the research, authorship, and/or publication of this article. This research was supported by the National Natural Science Foundation of China (42375039, 42230611, and 41975016), The Second Comprehensive Scientific Investigation and Research Project on the Qinghai-Tibet Plateau (2019QZKK0105), Key Innovation Team of China Meteorological Administration “Climate Change Detection and Response” (CMA2022ZD03).
Acknowledgments
We thank International Science Editing (http://www.internationalscienceediting. com) for editing this manuscript.
Conflict of interest
The authors declare that the research was conducted in the absence of any commercial or financial relationships that could be construed as a potential conflict of interest.
The reviewer ZY declared a past co-authorship with the author YP to the handling editor.
Publisher’s note
All claims expressed in this article are solely those of the authors and do not necessarily represent those of their affiliated organizations, or those of the publisher, the editors and the reviewers. Any product that may be evaluated in this article, or claim that may be made by its manufacturer, is not guaranteed or endorsed by the publisher.
References
Bi, S., Sun, L., Li, X., Chen, C., and Lu, Y. (2018). Characteristics of drought and flood disasters in the middle and lower reaches of the Yellow River from 1470 to 1911 based on EEMD method. J. Nat. Disasters 27, 137–147. doi:10.13577/j.jnd.2018.0117
Ding, Y., and Li, Y. (2016). A review on climatology of afro-asian summer monsoon and its long-term variability. J. Trop. Meteorol. 32, 786–796. doi:10.16032/j.issn.1004-4965.2016.06.002
Han, L., Zhang, Q., Jia, J., Wang, Y., and Huang, T. (2019). Drought severity, frequency, duration and regional differences in China. J. Desert Res. 39, 1–10. doi:10.7522/j.issn.1000-694X.2018.00112
Huang, N. E., and Shen, S. S. (2014). Hilbert-Huang transform and its applications, Interdisciplinary mathematical sciences. 2nd edition (Hackensack, New Jersey: World Scientific). doi:10.1142/8804
Huang, R., Xu, Y., and Zhou, L. (1999). The interdecadal variation of summer precipitations in China and the drought trend in north China. Plateau Meteorol., 465–476. doi:10.3321/j.issn:1000-0534.1999.04.001
IPCC (2012). “Summary for policymakers,” in Managing the risks of extreme events and disasters to advance climate change adaptation: special report of the intergovernmental panel on climate change. Editors C. B. Field, Q. Dahe, T. F. Stocker, and V. Barros (Cambridge, UK, and New York, NY, USA: Cambridge University Press), 3–21.
Li, S., Ge, W., and Wang, F. (2023). Characteristics of drought events and their impacts on vegetation in northern China from 1982 to 2019. Res. Soil Water Conserv. 30, 251–259. doi:10.13869/j.cnki.rswc.2023.03.017
Li, W., Wang, K., Fu, S., and Jiang, H. (2008). The interrelationship between regional westerly index and the water vapor budget in northwest China. J. Glaciol. Geocryol. 30, 28–34. doi:10.3321/j.issn:1000-6923.2007.04.026
Liao, Y., and Zhang, C. (2015). Spatio-temporal distribution characteristics and disaster change of drought in China based on meteorological drought composite index. Meteorol. Mon. 43 (11), 1402–1409. doi:10.7519/j.issn.1000-0526.2017.11.009
Ma, Z. (2005). Dry/wet variation and its relationship with regional warming in arid-regions of northern China. Chin. J. Geophys., 1011–1018. doi:10.3321/j.issn:0001-5733.2005.05.006
Ma, Z., and Fu, C. (2001). Trend of surface humid index in the arid area of northern China. Acta Meteorol. Sin., 737–746. doi:10.3321/j.issn:0577-6619.2001.06.010
Ma, Z., and Fu, C. (2003). Interannual characteristics of the surface hydrological variables over the arid and semi-arid areas of northern China. Glob. Planet. Change, Asia Monsoon Environ. Syst. Glob. Change 37, 189–200. doi:10.1016/S0921-8181(02)00203-5
Ma, Z., and Fu, C. (2005). Decadal variations of arid and semi-arid boundary in China. Chin. J. Geophys., 519–525. doi:10.3321/j.issn:0001-5733.2005.03.008
Qian, Z., Wu, T., Song, M., Ma, X., Cai, Y., and Liang, X. (2001). Arid disaster and advances in arid climate researches over northwest China. Adv. Earth Sci., 28–38. doi:10.3321/j.issn:1001-8166.2001.01.007
Qin, D. (2015). National assessment report on risk management and adaptation of climate extremes and disasters released. Beijing: China Science Press.
Qu, L., Zhao, y., Zhou, Y., and Meng, L. (2023). Why has the summer rainfall increased prominently in the west Tarim Basin of northwest China since 2010? Atmos. Res. 284, 106620. doi:10.1016/j.atmosres.2023.106620
Shen, X., Zhu, C., and Li, M. (2012). Possible causes of persistent drought event in north China during the cold season of 2010. Chin. J. Atmos. Sci. 36, 1123–1134. doi:10.3878/j.issn.1006-9895.2012.11192
Su, B., Huang, J., Fischer, T., Wang, Y., Kundzewicz, Z. W., Zhai, J., et al. (2018). Drought losses in China might double between the 1.5 °C and 2.0 °C warming. Proc. Natl. Acad. Sci. 115, 10600–10605. doi:10.1073/pnas.1802129115
Tang, M., Shen, Z., and Chen, Y. (1979). On climatic characteristics of the Xizang Plateau monsoon. Acta Geogr. Sin., 33–42. doi:10.11821/xb197901004
Wang, B., and Fan, Z. (1999). Choice of South Asian summer monsoon indices. Bull. Am. Meteorol. Soc. 80, 629–638. doi:10.1175/1520-0477(1999)080<0629:COSASM>2.0.CO;2
Wang, H., and Sheng, P. (2015). The North China/northeastern Asia severe summer drought in 2014. J. Clim. 28, 6667–6681. doi:10.1175/jcli-d-15-0202.1
Wang, K., Jiang, H., and Wu, H. (2001). Analysis on characteristics of interannual variability of the South asian summer monsoon. Plateau Meteorol., 318–324. doi:10.3321/j.issn:1000-0534.2001.03.015
Wei, F. (2007). Modern climatic statistical diagnosis and prediction technology. Beijing: China Meteorological Press.
Wei, F., and Cao, H. (1998). Regional characteristics of drought anomalies in north China. J. Appl. Meteorol. Sci., 78–85.
Wilhite, D. A. (2000). Chapter 1 drought as a natural hazard: concepts and definitions. Drought Mitig. Cent. Fac. Publ.
Wu, Z., and Huang, N. E. (2009). Ensemble empirical mode decomposition: a noise-assisted data analysis method. Adv. Adapt. Data Anal. 01, 1–41. doi:10.1142/S1793536909000047
Yang, J., Zhang, Q., Yue, P., Wang, P., Duan, X., Zhang, J., et al. (2023). Characteristics of warming and humidification in the Yellow River's upper reaches and their impact on surface water resources. Int. J. Climatol. 43, 7667–7681. doi:10.1002/joc.8286
Zhang, Q., Han, L., Hao, X., Han, T., Jia, J., and Lin, J. (2015). On the impact of the climate change on the agricultural disaster loss caused by drought in China and the regional differences between the North and the South. Acta Meteorol. Sin. 73, 1092–1103. doi:10.11676/qxxb2015.083
Zhang, Q., Li, Y., and Chen, L. (2011). Major Characteristics,Key issues and coping strategiesof climate change. J. Desert Res. 31, 492–499. doi:10.3724/SP.J.1146.2006.01085
Zhang, Q., Lin, J., Liu, W., and Han, L. (2019a). Precipitation seesaw phenomenon and its formation mechanism in the eastern and western parts of Northwest China during the flood season. Sci. China Earth Sci. 62, 2083–2098. doi:10.1007/s11430-018-9357-y
Zhang, Q., Tao, S., and Chen, L. (2003). The inter-annual variability of East Asian summer monsoon indices and its association with the pattern of general circulation over East Asia. Acta Meteorol. Sin., 559–568. doi:10.3321/j.issn:0577-6619.2003.05.005
Zhang, Q., Yang, J., Duan, X., Ma, P., Lu, G., Zhu, B., et al. (2022). The eastward expansion of the climate humidification trend in northwest China and the synergistic influences on the circulation mechanism. Clim. Dyn. 59, 2481–2497. doi:10.1007/s00382-022-06221-4
Zhang, Q., Yang, J., Wang, W., Ma, P., Lu, G., Liu, X., et al. (2021). Climatic warming and humidification in the arid region of northwest China: multiscale characteristics and impacts on ecological vegetation. J. Meteorol. Res. 35, 113–127. doi:10.1007/s13351-021-0105-3
Zhang, Q., Yao, Y., Wang, Y., Wang, S., Wang, J., Yang, J., et al. (2019b). Characteristics of drought in Southern China under climatic warming, the risk, and countermeasures for prevention and control. Theor. Appl. Climatol. 136, 1157–1173. doi:10.1007/s00704-018-2541-2
Keywords: Northern China, summer drought, circulation, multiple factors, synergistic influence
Citation: Yang J, Zhang Q, Wang P, Yue P, Li Y, Liang Z and Liu X (2024) Characteristics of summer drought circulation and the synergistic effect of multiple factors in the Northern drought-prone belt of China. Front. Environ. Sci. 12:1464917. doi: 10.3389/fenvs.2024.1464917
Received: 15 July 2024; Accepted: 06 August 2024;
Published: 21 August 2024.
Edited by:
Haikun Zhao, Nanjing University of Information Science and Technology, ChinaReviewed by:
Zesu Yang, Chengdu University of Information Technology, ChinaJianling Yang, Earthquake Agency of Ningxia Hui Autonomous Region, China
Copyright © 2024 Yang, Zhang, Wang, Yue, Li, Liang and Liu. This is an open-access article distributed under the terms of the Creative Commons Attribution License (CC BY). The use, distribution or reproduction in other forums is permitted, provided the original author(s) and the copyright owner(s) are credited and that the original publication in this journal is cited, in accordance with accepted academic practice. No use, distribution or reproduction is permitted which does not comply with these terms.
*Correspondence: Qiang Zhang, zhangqiang@cma.gov.cn; Pengling Wang, wangpl@cma.gov.cn