- 1Centro Interdisciplinario para la Formación y Vinculación Social, Instituto Tecnológico y de Estudios Superiores de Occidente, Tlaquepaque, Jalisco, Mexico
- 2Instituto de Ecología, Universidad Nacional Autónoma de México, Mexico City, Mexico
- 3USDA Forest Service, Institute of Pacific Islands Forestry, Hilo, HI, United States
The tropical dry forest (TDF) biome has undergone a diversity of severe disturbances, with resulting transformations and continued pressures making this biome type one of the Earth’s most threatened. Supporting large numbers of native, often endemic species, fragments of TDF serve as important but precarious refugia. There are global efforts to restore this once extensive forest type, but the impact of TDF restoration on biodiversity and ecosystem function is poorly understood. Here, we present results from an analysis of 187 unique studies, published between January 1990 and February 2020, that examined a range of TDF restoration practices. We used a meta-analytical approach to compare survival, growth, and measures of ecosystem function in restored systems and either intact reference baselines or impacted controls. Understanding that social factors can represent over-riding constraints on restoration implementation or success, we also examined human dimensions variables, which were the least evaluated metrics in our review. We found that the survival of native species plantings showed high variability within and across regions and treatments – indicating a broad need for refined restoration prescriptions to better match practice to species and site. Synthesizing a global dataset provides insights needed to increase the success of TDF restoration.
Introduction
Ecological restoration is widely viewed as a critical tool for averting extinctions and recovering the functional capacity of ecosystems to provision goods and services while mitigating the effects of climate change. To this end, the International Union for the Conservation of Nature’s Bonn Challenge (2011), the New York Declaration on Forests (2014) and Initiative 20 × 20 (2014) all seek to establish forest restoration as a major instrument of global resource stewardship. These ambitious efforts have the potential to influence the ecological trajectory of 100s of millions of hectares of degraded land, but will require that countries and governments invest heavily into restoration planning. Such an investment requires a solid foundation of restoration knowledge.
The tropical dry forest (TDF) biome covers nearly half of all tropical land area in Africa, Central and South America, Asia and the Pacific (Murphy and Lugo, 1986). While there is variability across TDF with respect to edaphic and climatic conditions, biological diversity, ecosystem function, and provisioned services (Thakur et al., 2021), all TDF are defined by a severe (monthly rainfall of less than 100 mm) dry season that can last between 3–8 months. This marked seasonality distinguishes TDF from other tropical forest biomes, with TDF vegetation having evolved a diversity of mechanisms to endure long dry periods, including drought deciduous strategies (Banda et al., 2016; Pennington et al., 2009; Singh and Chaturvedi, 2017). Variability in TDF structure and function across the biome are also influenced by evapotranspiration (ET), mean annual temperature (MAT), and soil aridity (de la Peña-Domene et al., 2022), while variation in the normalized difference vegetation index (NDVI), a productivity indicator (Rivero-Villar et al., 2022), appears to reflect underlying variation in soil fertility.
Centuries of human disturbance also play an important role in shaping TDF, which have supported human communities throughout its distribution. The climates, vegetation types, and soils of this biome tend to be favorable to human settlement and agriculture, as has been demonstrated by the Maya of the Neotropical region, the Kānaka ʻŌiwi of Hawaiʻi, the Bamana of Mali, the Bemba of Zambia, and the Adivasi of India, among many others (Colfer et al., 2015; Omkar et al., 2012; Sato, 2020). In the past century, TDF has seen more intensive and larger scale conversions to rangeland for commercial livestock grazing, to intensive agriculture, tourism development, and mining extraction–all of which continue to degrade remaining TDF (Miles et al., 2006; Portillo-Quintero et al., 2015; Singh and Chaturvedi, 2017). The very high rates of historical and contemporary land-use change make TDF one of the Earthʻs most threatened biomes (Miles et al., 2006; Stoner and Sánchez-Azofeifa, 2009), with consequences that include: biomass loss during fire-based clearing and agricultural management (Ammondt et al., 2013; Corona-Núñez and Campo, 2023; Singh et al., 2004); lost soil function (Giardina et al., 2000; Gebremedihin et al., 2018; Gei and Powers, 2013); loss of tree biodiversity and associated fauna; lost capacity for regeration because of impacts to seed rain, seed bank, sprouting, and advanced regeneration (Chazdon, 2014; Chazdon et al., 2007); and reduced ecosystem services. Across the TDF biome, fragments of TDF serve as important refugia for biodiversity, supporting large numbers of endemic and threatened species (Sola, 2014), while highlighting the potential for restoration of adjacent land-uses to sequester carbon (Ahirwal et al., 2017a; Griscom, 2020; Mesa-Sierra et al., 2022a) and enhance resilience to natural disasters (Derroire et al., 2016; Goffner et al., 2019; Stan et al., 2021).
While the outcomes of successful TDF restoration include a variety of valued benefits, four knowledge, planning and implementation gaps currently limit the success of TDF restoration: (i) poorly quantified impacts of widely ranging land uses (e.g., slash and burn agriculture, mining) on soil structure and fertility including regeneration capacity, microclimate, and nutrient loss (Ahirwal et al., 2017b; Carrasco-Carballido et al., 2019; Chaturvedi et al., 2018); (ii) lack of information on controls over the avenues of regeneration available to a restoration practitioner (advanced regeneration, sprouting, seed bank, seed rain), which limits effective and efficient restoration planning (Leverkus et al., 2021; Martínez-Garza et al., 2016); (iii) poorly understood effects of climate change, variability and drought on restoration success (de la Peña-Domene et al., 2022; Dirzo et al., 2011; Singh and Chaturvedi, 2017); and (iv) a lack of studies focusing on local community interests in and perspectives on what defines successful restoration, especially with respect to how restoration meets the basic societal needs (Ceccon et al., 2020; Dimson and Gillespie, 2020; Tarrasón et al., 2010). Directly engaging these four gaps can help to define site specific restoration contexts and design robust but socially acceptable restoration projects that are resilient to climate change and context variability.
To assess how these four knowledge gaps constrain TDF restoration, we conducted a systematic literature review of 187 TDF restoration papers and meta-analysis to establish baseline information about enabling conditions for TDF restoration, with the goal of supporting decision makers and managers in their efforts to optimize restoration investments. We used our analysis to address the following four questions: 1) Where is TDF restoration research being conducted globally?; 2) Are collaborative networks being forged to support TDF restoration?; 3) What are the metrics for restoration success?; and 4) what do these metrics tell us about the success of TDF restoration? This work provides the first pantropical evaluation of TDF restoration.
Methods
Literature research
We used Web of Science (WoS) and Google Scholar (GS) databases to search for relevant studies published between January 1990 and February 2020, and that addressed TDF restoration anywhere in this biome. We queried WoS for the following terms within titles, abstracts, and keywords: [“Seasonally Dry Tropical Forest*” OR “Dryland*” OR “Tropical Dry Forest*”] AND [“Restoration*” OR “Reforestation*”]. We found that GS was not as systematized as WoS and so six searches were made in GS as follow: [“Seasonally Dry Tropical Forest” AND “Restoration”], [“Seasonally Dry Tropical Forest” AND “Reforestation”], [“Tropical Dry Forest” AND “Restoration”], [“Tropical Dry Forest” AND “Reforestation”], [“Dryland” AND “Restoration”], [“ Dryland” AND “Reforestation”]. Our queries returned 3,146 articles with English language titles, abstracts, and keywords. The body of some articles with English language abstracts were written in other languages, such as Portuguese and Spanish.
We removed articles from our analyses based on the following: i) duplication of a study (253 studies); ii) only marginally relevant to TDF or restoration (1,092 studies); iii) study relied only on remote sensing (703 studies) or exclusively on natural regeneration (748 studies). Details on these criteria and how they were implemented can be found in Supplementary Figure S1. For each of the 187 included articles, we determined whether a study compared restored sites against a reference site representing an intact ecological baseline or against a control plot representing the degraded initial condition and not receiving any restoration treatment or a secondary forest. For this latter comparison, while not receiving a treatment, control plots can support natural regeneration, which can mute contrasts with restored treatments. Conversely, continued degradation of controls over a study can increase apparent differences. Regardless, comparisons against both a reference site and against a control site are valuable for evaluating the changes that result from implementation of restoration prescriptions.
Classification
We generated a database with the following attributes for each of the 187 articles: (1) Study Type (field studies, greenhouse studies, or both); (2) Study Goals; (3) Funding; (4) Site Characteristics; (5) Management Prior to Restoration Actions; and (6) Restoration Strategy (Supplementary Table S1). Studies carried out in greenhouse studies were included exclusively for the analysis of general trends, but not in the further meta-analyses, recognizing their value to evaluate restoration approaches and the generation of basic knowledge that strengthens strategies in the field.
Characterization of the TDF ecoregions supporting restoration efforts
The TDF biome includes approximately 80 TDF ecotypes in five biogeographic regions (Olson et al., 2001): Afrotropical, Australasian, Indomalayan, Neotropical, and Oceanian. We did not include studies from Australasian TDF because the dry forests in this geography do not meet TDF criteria (de la Peña-Domene et al., 2022). Relying on the coordinates provided in each reviewed article we further characterize restoration sites using global geospatial data-bases for soils, climate, biogeography, and where possible history.
Data analysis
General trends
The general trends were described for field studies in terms of the reported previous land uses, the restoration actions, and the restored area. For all the studies, including field and greenhouse, we extracted information on the sources of financing, and the parameters to monitor restoration success (Table 1). We analyzed whether studies examined one or more of previously identified categories of restoration success (Mesa-Sierra et al., 2022b; see Supplementary Table S1 for details): 1) vegetation structure (e.g., survival, germination, canopy cover); 2) functional attributes of the vegetation (e.g., seed viability); 3) ecosystem function (e.g., carbon stores); 4) soil attributes (e.g., pH, moisture, nutrients); 5) biotic interactions (e.g., herbivory, pollination); 6) biotic composition and diversity (e.g., vegetation composition, arthropod community); and 7) services and social outcomes (e.g., social perception of environment). These align broadly with the nine parameters proposed by the Society of Ecological Restoration (SER) to evaluate restoration success: 1) diversity, richness, dominance, and physiognomy; 2) presence of native species; 3) diversity of functional groups; 4) viability of populations; 5) recovery of ecological processes; 6) landscape dynamics; 7) elimination of threats; 8) increased ecosystem resilience; and 9) capacity for self-maintenance (Balensiefer et al., 2004).
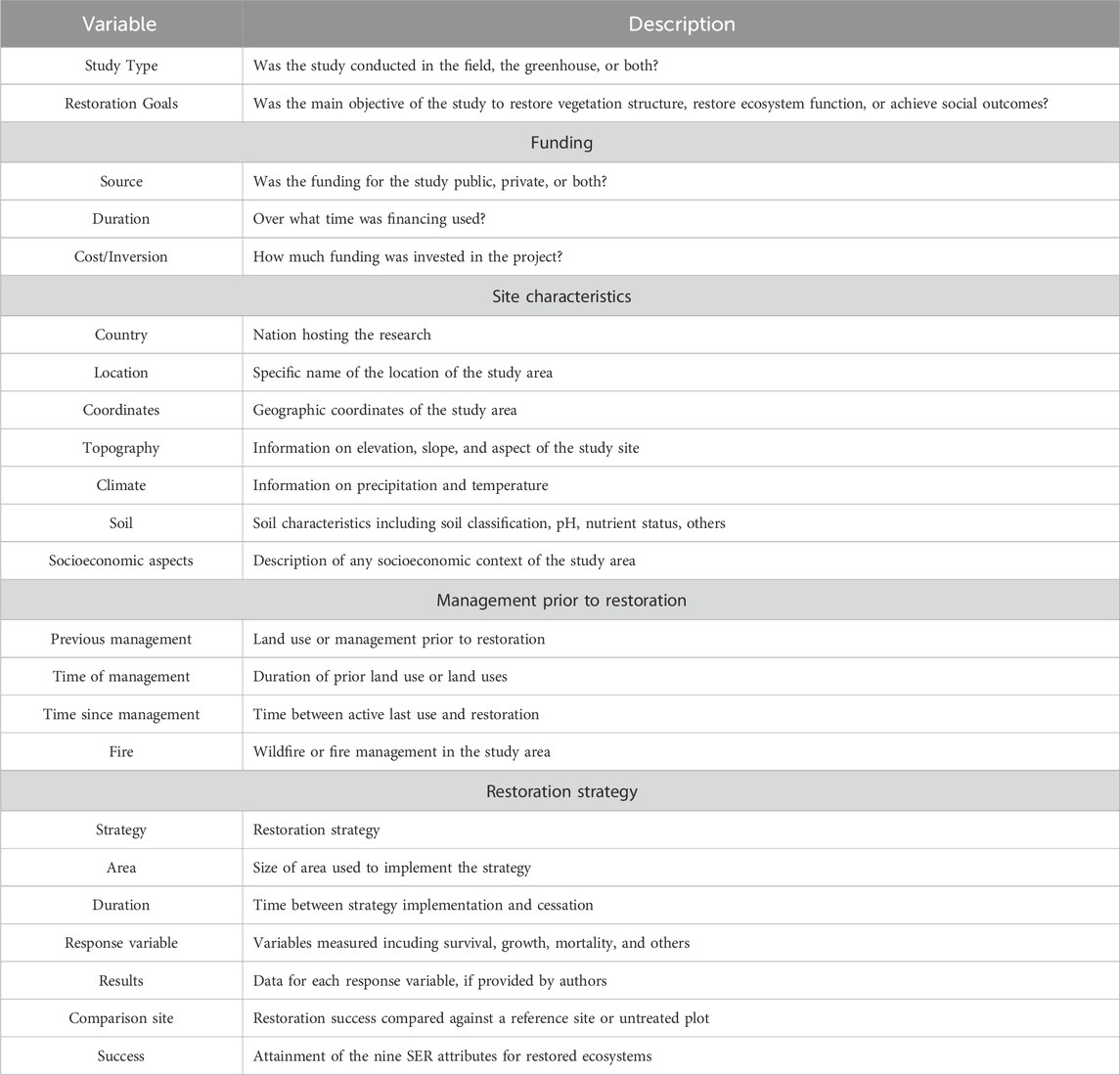
Table 1. Attributes recorded in literature review. The goals reported by the 187 studies were variable across and included: i) recovery of vegetation composition and structure (e.g., establishing populations of target species; achieving canopy cover, canopy height, stem density targets); ii) recovery of ecosystem processes (e.g., plant productivity, nutrient cycling, biological interactions); and iii) recovery of social benefits (e.g., provisioning of ecosystem services or creating a source of species with cultural or economic value). Funding information included source, duration, and amount, which we recorded to examine the economic dimensions of restoration initiatives, in addition to where the restoration was conducted, which consisted of the country, location and coordinates, topography, climate, soil conditions, and socioeconomic aspects of the site. Land use included the description of the previous management to restoration, duration of the previous land use, time elapsed between the suspension of management activity and the beginning of site restoration, and the presence of natural or human caused fires in the study area. We also examined the type of restoration strategy implemented, including the area restored and the time duration of the restoration effort, the response variables reported by the studies to measure the progress of the strategy, results, whether the results were compared against a reference site or control plot), and which of the Society of Ecological Restoration (SER) parameters were evaluated.
Assessment of the restoration drivers
Meta-analyses were conducted for field studies that compared a restoration treament agenst either a reference or a control site. We extracted means, statistical variation (i.e., standard errors, standard deviations), and sample sizes for restored, reference and control control groups for survival, height, above-ground carbon (AGC), and total soil nitrogen (N) and available soil phosphorus (P). For data provided only through figures, we used the software program DataThief III (Tummers, 2006) to extract values. We chose the log of response ratio (lnR) to measure the effect size of the restoration actions due to its suitability to compare disparate studies with small sample sizes (Hedges et al., 1999). We calculated lnR for proportional change in measures comparing restored and control or restored and reference sites, as a measure of restoration progress, to move away from a control site or to approach a reference system, respectively. Additionally, for those response variables that had data from at least two studies, we used random effect models using the “rma.uni” function in the metafor package in R (Viechtbauer, 2010) to calculate pooled summary effect size and significance of restoration effects on each variable. We used Cochran’s Q to test whether effect sizes were homogeneous across studies. All statistical analyzes were performed in R software (R Development Core Team, 2019).
Results
Literature review
The WoS and Google Scholar searches yielded 3,146 studies, of which 187 met the preliminary review criteria (Supplementary Table S2). Of these studies, 70% described field restoration projects, with an additional 11% involving both greenhouse and field work. The remaining 18% of the studies were review papers or greenhouse-based studies. The stated main objective of restoration studies was the recovery of vegetation structure (56%) and the recovery of vegetation structure and function (22%), with only 6% of the studies including social/human wellbeing objectives. During the 1994–2020 survey period, the Neotropical and Afrotropical biogeographic regions saw a peak in the number of publications in 2011 (Figure 1). For the four biogeographic regions, publication production prior 2010 did not surpass five published studies per year, but afterwards, the Neotropical region exceeded 10 published studies per year (Figure 1).
Pantropical distribution of the global restoration studies
The Neotropical biogeographic region produced the highest number of studies, followed by Afrotropical, Indomalayan, and Oceanian (Figures 2B, C). When looking at the trend by country, Mexico had the highest number of studies followed by India and then Ethiopia (Figure 2B). While Oceania was the region with the lowest number of studies, all studies were from the intensely studied Hawaiian Islands. When looking at the reviewed studies with field work, the Afrotropical region, despite having a low number of studies, the studies represented the largest areas being restored (Figure 2A). For the case of the Neotropical region, there are National examples of many studies with small, restored surfaces (e.g., Mexico) or few but large study areas (e.g., Ecuador) (Figure 2A). When comparing the restored area with the total extension of the TDF ecosystem in each region, it was observed that the highest proportion of forest restored was in Oceania with 46% of total TDF surface (based on de la Peña-Domene et al., 2022), while the Neotropics had only around 11% restored (Supplementary Table S3).
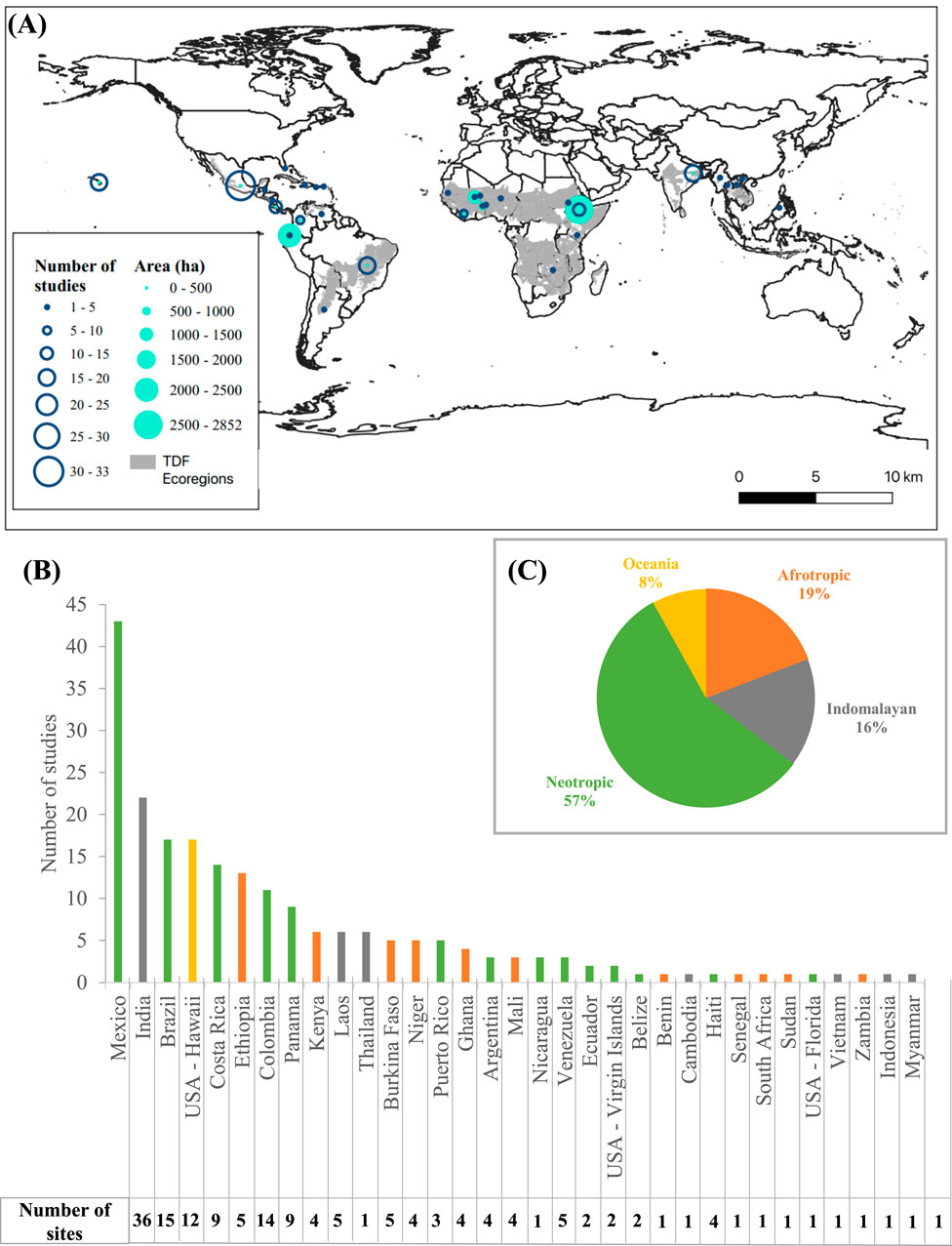
Figure 2. Total restored area represented by research plots (A). The location was placed in the center of the country and does not signify the actual in-country location of the study. Number of studies per country (B); distribution and number of restoration studies with fieldwork reviewed here (n = 153), and percentage of studies per biogeographic region (C).
General trends
The most commonly studied previous land-uses were cattle ranching (48% of total studies), cropping (25%), and mining (10%) but region to region variation was high; for example, in the Indomalayan region, the dominant studied land-use was mining (Figure 3A). We found that planting of desirable species and removal of non-desirable plants were the restoration actions most often implemented in the 187 studies (Figure 3B), but again, variation across regions was high. Invasive plant removal was the most commonly studied action in Oceania, planting in Indomalayan and Neotropical regions, and assisted natural regeneration in (exclusively) the Afrotropical region (Figure 3B). It is worth mentioning that among the five most reported actions we also find seed related, such as seeding, and enclosure, which corresponds to the exclusion of livestock to stop the disturbance. A total of 146 measures, grouped under seven variable sets (Supplementary Table S1), were identified across the four biogeographic regions, with variables related to vegetation structure being the most studied restoration action (47%) and those related to ecosystem dynamics (7.5%) and vegetation function (6%) being the least studied (Figure 4). Social measures appeared in 12.3% of all studies but were highly variable across regions. The following five measures were used to assess the vegetation structure variable set, in order of importance: Survival > Seedling Height > Germination > Seedling Growth > Seedling Diameter (Supplementary Table S1). Soil measures, the second most common variable set (Figure 4), included: soil nutrient availability > pH > soil moisture > litter attributes (Supplementary Table S1). In relation to the set of social variables, the most used measures were (Supplementary Table S1): Species values > Determinants of restoration program adoption > Economic benefits.
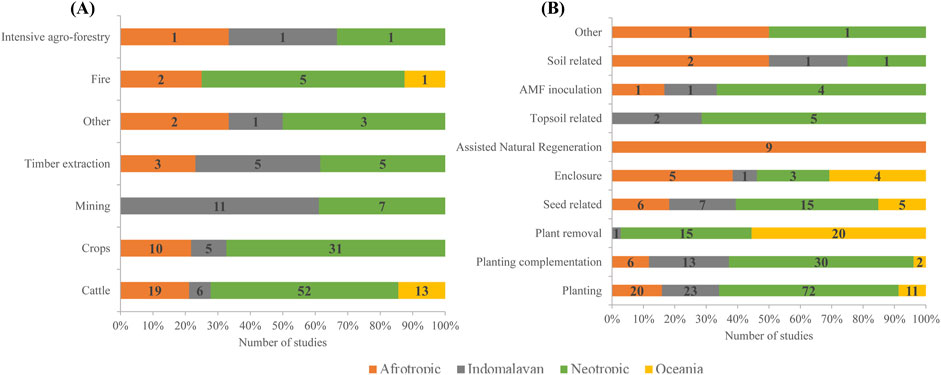
Figure 3. Disturbances prior to restoration activities identified by field studies (A), and restoration strategies or greenhouse treatments (B) reported by the studies per biogeographic regions. Number of studies are specified for each category. For details of the categories see Supplementary Table S1.
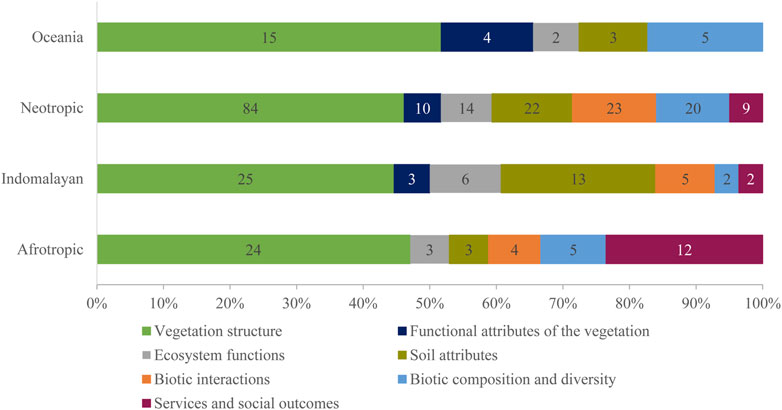
Figure 4. Variables used in the restoration studies per biogeographic regions. Number of studies are specified for each category. For details of the categories see Supplementary Table S1.
Restoration drivers
To further analyze restoration success and drivers, from the original 187 studies, we selected 64 studies, that include a control or reference site, a requirement established by the SER (Balensiefer et al., 2004) to evaluate restoration success. A higher proportion of comparisons in the studies were made against control plots (63%), rather than against reference sites (37%), yielding different insights into restoration success. Comparison of measures between a treated restoration site against an untreated control revealed how imposed treatments helped move a site away from a control site with the magnitude of the difference indicating level of success. However, this comparison cannot be used to assess how close a restored site is to a reference (or benchmark) site. Conversely, comparisons against a reference site cannot be used to evaluate improvements over an untreated control site. For the former, improvements over an untreated control can be quantified, but whether the treated site is approaching a reference site would be unknown. The converse is true for the latter.
These 64 studies directly addressed four of the nine criteria identified by the SER for evaluated restoration success, 1) diversity, richness and physiognomy, 2) presence of native species, 3) functional groups, and 4) the recovery of ecological processes. From the 64 studies, we were able to extract data for survival, height, above-ground carbon (AGC), and total soil nitrogen (N) and available soil phosphorus (P). Most of the studies that evaluated these five measures were conducted in the Neotropical and Indomalayan regions. The results of our meta-analysis identified variation across biogeographic region and restoration strategy. A significant effect of restoration compared with control plots was observed only for the structural variable measures of survival (LnR = 0.51, 95% CIs = 0.15 to 0.87) and height (LnR = −2.85, 95% CIs = −8.03 to 2.32) (Figure 5). The measurement of these structural variables in the control plots was made in self-recruited individuals. In the Indomalaya region, restoration had a positive but non-significant trend towards the recovery of survival of seedlings when compared to both a reference site (LnR = 0.95, 95% CIs = −0.87 to 2.77) and a control site (LnR = 1.39, 95% CIs = −0.45 to 3.24) (Figure 6). Although not significant, in the Neotropical region the survival (LnR = 0.492, 95% CIs = −0.06 to 1.4), and height (LnR = 0.48, 95% CIs = −0.55 to 1.52) of the restoration plots performed better than control plots (Figure 6). Oppositely, in the case of AGC, which depends on the woody growth, no positive trend in its recovery was observed when compared with a reference system, nor with a control site (Figure 6), in any of the biogeographic regions evaluated.
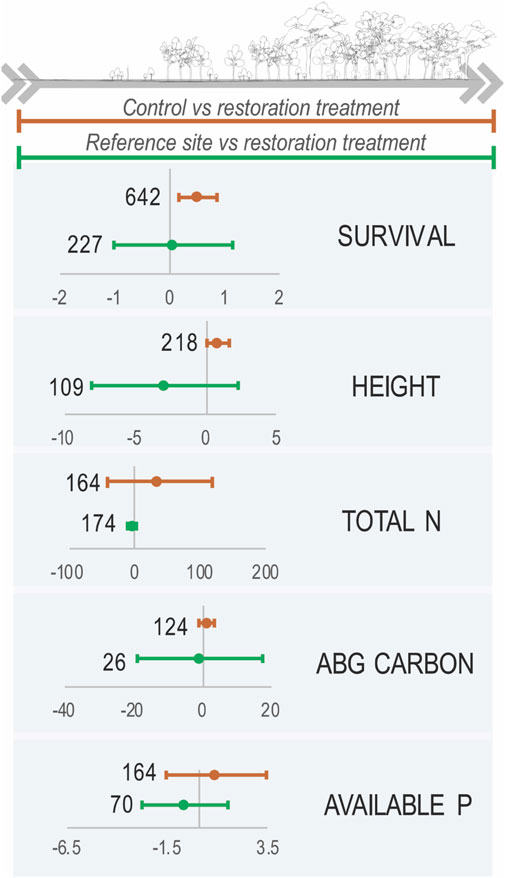
Figure 5. Mean effect size (LnR) of restoration on the survival and height of plantings, soil total N and available P concentrations, and above-ground carbon (AGC). Bars represent 95% confidence intervals (CI). Numbers to the left side of confidence intervals denote the number of observations. If the mean falls to the right of zero and CIs do not intersect with zero, we interpret that the restored plot had a higher level of the variable being measured than the compared reference site (green) or control plot(s) (brown); conversely if the mean falls to the left of zero and CIs do not intersect with zero, then the restored plot had a lower level of the variable being measured than the compared reference site or control plot. The X-axis represents level of degradation for the restored plot, with negative value means for reference site comparisons (mean falls on the left side of the figure with zero being the theoretical maximum) indicating the size of the gap with Reference sites; for comparisons with control sites, positive value means indicating the size of the gap with Control plots, with zero being the theoretical minimum. The drawing represents the gradient on the horizontal axis, going from the most degraded (negative side) to the values most similar to the reference site (positive side).
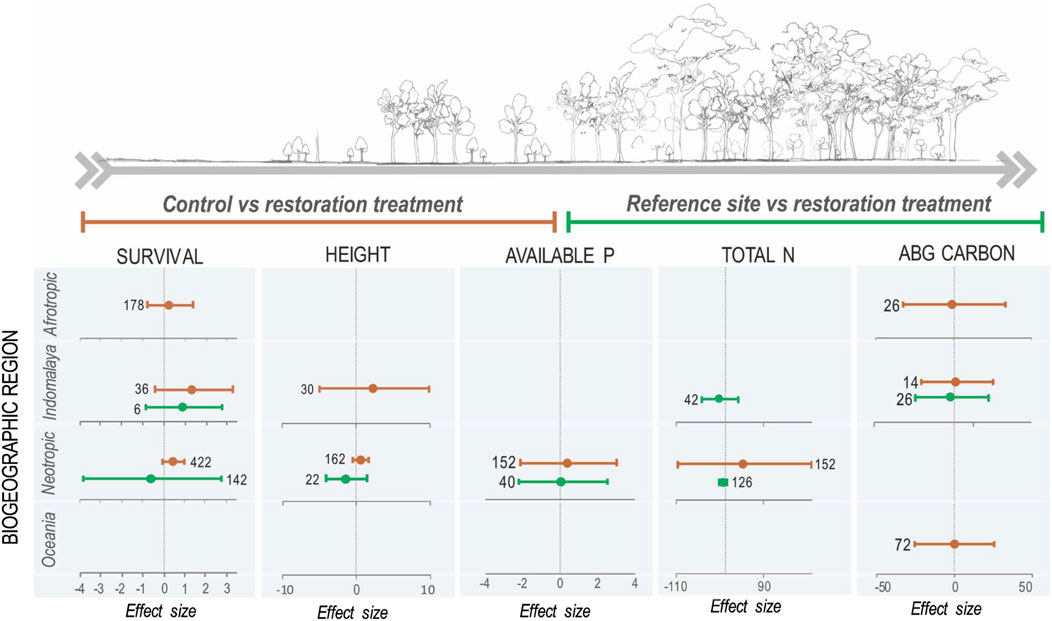
Figure 6. Mean effect size (LnR) of restoration on the survival and height of plantings, the available P and the total N of soil, and the above-ground (AGC) carbon, by biogeographic region as a subgroup analysis. Bars represent 95% confidence intervals (CI). The numbers at the left side of the confidence intervals denote the number of observations. If the bar falls in the positive side and does not intersect with zero, we interpret that the restored plot had a higher level than the control plot(s) (brown) or reference site (green), while if the bar falls in the negative side, then the restored plot had a lower level than the control plot(s) or reference site.
Finally, when evaluating the different restoration actions reported by the studies, in most of the reviewed cases, restoration was performed through plantings (43%). However, in this study we did not find a clear trend of the performance of this action when compared with a reference system, nor with a control site, reflecting highly variable values for planting success (Figure 7). When plantings were complemented with other management (e.g., fertilization, watering; see Supplementary Table S1 for details), restoration had a positive signficant effect on survival (LnR = 0.44, 95% CIs = 0.034 to 0.85) and non-significant effect on height (LnR = 0.92, 95% CIs = −1.05 to 2.89) of the planted seedlings. The different restoration actions did not have a positive effect on soil variables (Figure 7).
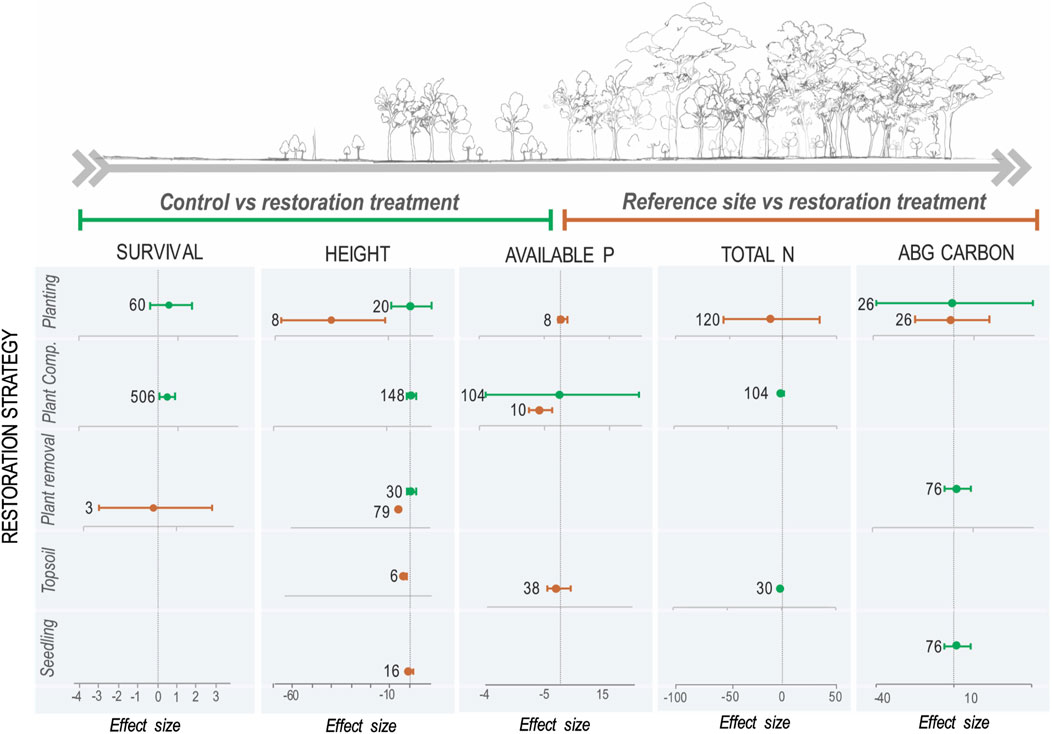
Figure 7. Mean effect size (LnR) of restoration on the survival and height of plantings, the available P and the total N of soil, and the above-ground (AGC) carbon, by restoration strategy as a subgroup analysis. Bars represent 95% confidence intervals (CI). The numbers at the left side of the confidence intervals denote the number of observations. If the bar falls in the positive side and does not intersect with zero, we interpret that the restored plot had a higher level than the comparative basis (control -brown- or reference -green-), while if the bar falls in the negative side, then the restored plot had a lower level than the comparative basis (control or reference).
Discussion
Globally, tropical dry forests (TDF) have been heavily degraded due to various land use and land cover changes, making them one of the most threatened ecosystems on Earth (Janzen, 1988; Miles et al., 2006). Given their extensive area and the unique flora and fauna they support, their restoration is an urgent conservation need. Robust recommendations are based on site-specific research that provides clear and conclusive insights into how interventions perform under various conditions. While research on TDF restoration is expanding, it lags behind that of more humid tropical systems (Dimson and Gillespie, 2020; Newton et al., 2012; Singh and Chaturvedi, 2017). In this paper, we sought to address four knowledge, planning, and implementation gaps: (i) poorly quantified impacts of diverse land uses on soil structure and fertility, including regeneration capacity, microclimate, and nutrient loss; (ii) lack of information on the controls over avenues of regeneration, limiting effective and efficient restoration planning; (iii) poorly understood effects of climate change, variability, and drought on restoration success; and (iv) a lack of studies focusing on local community interests and perspectives on what defines successful restoration, particularly regarding how restoration meets basic societal needs. We employed a detailed analysis of the restoration literature to directly engage these four gaps and found that: (i) the number of research studies on TDF increased dramatically between 1990 and 2020, although this increase varied significantly by geography, with substantial work on the role of soils in supporting restoration; (ii) there remain important knowledge gaps on the enabling conditions required to support TDF restoration (e.g., avenues of regeneration); (iii) constraints imposed by climate change; and (iv) efforts to understand the human dimensions of enabling conditions and constraints to TDF restoration are nascent, with work led in only a few regions.
The increase in research focused on tropical dry forests (TDF) reflects a growing global appreciation for and interest in tropical forest conservation, alongside an expanded understanding of the diverse goods and services TDF provide—services that are increasingly threatened by rapid conversion to non-forest uses. Additionally, there is a growing capacity among researchers, particularly those based in TDF regions, to study the threats facing these ecosystems (Singh and Chaturvedi, 2017). Despite this progress, our analysis indicates that core restoration questions for TDF still remain. Over 3 decades of research have produced significant insights into TDF restoration, including: (1) the success of plantings improves when complemented with maintenance actions such as irrigation or mulching; (2) soil variables serve as indicators of recovery, not only in terms of soil health but also regarding processes like nutrient fixation; (3) studies that incorporate social variables frequently adopt a transdisciplinary perspective; and (4) in areas where invasive species are the primary disturbance, successful initial strategies often involve their removal, setting the stage for effective planting efforts. However, the equivocal findings across the reviewed studies highlight critical gaps in knowledge. Many studies lack rigorous designs for evaluating success, failing to include comparisons with reference sites (ecological benchmarks necessary for assessing recovery) and controls (essential for measuring improvements over baseline degraded conditions). In our analysis, only 64 of the 187 TDF restoration papers included such comparisons. Without an expansion of robust research demonstrating to practitioners how specific strategies lead to desired outcomes—outcomes that can be evaluated against Society for Ecological Restoration (SER) success criteria—the potential for making rapid progress in TDF restoration will remain limited.
We included human dimensions of restoration success in our analysis because it has been clearly established that while there are biological and physical limitations to restoration, societal constraints can represent over-riding drivers of restoration failure. Few studies included here examined social aspects of TDF restoration, which is fundamentally a placed-based practice that is most likely to succeed when it relies on local knowledge with prescriptions that align with a locally-meaningful vision for desired outcomes, typically some mix of species biodiversity, vegetation structure, ecosystem function, or community dynamics–all contributing in some way to the recovery of ecosystem services (Arroyo-Rodríguez et al., 2020; Campo et al., 2023; Goffner et al., 2019). Increasingly, bringing local communities into restoration planning and implementation is seen as an effective way to integrate local values and perspectives, culturally-driven priorities, and local ecological knowledge into restoration planning, with the goal of co-production that enhances ecological sustainability and environmental justice (Kamelamela et al., 2022). Co-production can also: i) enhance recognition of the value of traditional knowledge in the selection of species for example, those with high cultural or economic value (e.g., firewood or timber species, or species that supply forage, traditional medicine, or other non-timber forest products); ii) improve identification of locally relevant species to augment ecosystem services; and iii) increase technical and labor capacities (Alvarez-Aquino and Williams-Linera, 2012; Burnett et al., 2019; Kamelamela et al., 2022). The spatial relationship between local communities and ecosystem services is crucial for effective restoration planning, with these communities serving as key beneficiaries of restored systems (Ceccon et al., 2020; Costa et al., 2021; Weston et al., 2015). The Afrotropic biogeographic region exhibits a relatively higher inclusion of social dimensions in restoration papers, likely due to its more arid climate, nutrient-poor soils, and the predominance of private funding for restoration initiatives. For instance, the Great Green Wall project has fostered resilient landscapes that yield significant ecological, social, and economic benefits on a large scale (Mirzabaev et al., 2022; Goffner et al., 2019; Wade et al., 2018). Research stemming from this ambitious project has underscored the importance of integrating scientific and traditional knowledge, along with the experiences of local communities, to develop optimal solutions for ecological, economic, and social recovery.
How does prior use influence restoration outcomes?
We found that deforestation for increasing livestock and crop production was a common disturbance across biogeographic regions, with both being widely observed drivers of global change in the tropics (Banda et al., 2016; Trejo and Dirzo, 2000). It is important to highlight that recent studies focused on the Neotropical region have found that, in Mexico, the area affected by burning exceeds the area that has been deforested (Montoya et al., 2023). This observation may indicate a common trend for tropical dry forests (TDF) worldwide, particularly in light of increasing drought periods and human interventions. It is also noteworthy that TDF are recognized for their fertile soils, which play a crucial role in contributing to the global food supply. However, research has shown that soil fertility values vary across biogeographic regions (Rivero-Villar et al., 2022), which is crucial for selecting the most appropriate strategies for ecosystem restoration. In three of the four regions included in our study (all except Oceania), planting seedlings was the most common restoration strategy. This approach is both costly and labor-intensive and carries a significant risk of failure (Alem, 2020; Brooks and Jordan, 2014; Martínez-Garza et al., 2016). Plantings can have unpredictable results and may require extensive management to ensure their success (Aerts et al., 2006; Griscom et al., 2005; Leverkus et al., 2021). However, in some cases this action is the last resort due to long periods of degradation and intensive land use. This is reflected in the high variability reported by studies in survival rates (Dimson and Gillespie, 2020; Ferreira Nunes et al., 2015; Sangsupan et al., 2018), which in some cases show values close to what registered in a preserved site and in others the values reflected a low success of the restoration practice.
Survival rates for outplanted seedlings have been reported to improve when the practice includes additional practices such as fertilization, mulching, and irrigation–all appearing to increase the probability of survival of the seedlings (Ceccon et al., 2004; Brooks and Jordan, 2014; Leverkus et al., 2021). In studies from the Indomalayan region, survival rates are similar to plots within a reference site, which may be evidence of the efficiency of having complementary actions with the plantings, especially related to soil recovery (Chaturvedi et al., 2018; Jaganathan and Liu, 2015; Kukrety et al., 2013). Similarly, in Hawaii, the combination of ungulate exclusion, invasive grass control, and active planting need to be combined as the three practiced separately are not effective (Brooks et al., 2009; Cabin et al., 2002; Libby et al., 2022). Plantings are often viewed as one of the primary goals of restoration, aimed at achieving large-scale restoration over the long term. This perspective stems from their high levels of acceptance and popularity, as well as social engagement and funding, often influenced by the collective perception of how restoration processes should be implemented (Chirwa et al., 2015; Silveira et al., 2021). However, many times, other less intensive restoration actions can be applied to accelerate natural succession if establishment limitation is not an impediment (de la Peña-Domene and Martínez-Garza, 2018). Therefore, it is essential to consider the importance of designing, implementing, and monitoring restoration efforts tailored to the specific needs and conditions of each region. The initial steps of restoration should be guided by the levels of disturbance, environmental conditions, and landscape context to facilitate the growth of mature trees, promoting an ecological and landscape-oriented vision that supports the recovery of ecosystem functions and secondary succession.
Our results show the importance of improving the planning of strategies in accordance with the disturbance driver. For example, mining was an important driver reported for only the Indomalayan and Neotropical regions. The country with the largest number of studies was India, where livestock is not the most important factor of disturbance of TDFs, and instead has extensive mining areas (Alem, 2020; Sheoran et al., 2010; Singh and Chaturvedi, 2017). One of the main consequences of this economic activity is the strong degradation of the soil. Soil fertility, mainly evaluated by the availability in limiting nutrients for plant growth, reflects not only the recovery of elements, but also of microorganisms, such as mycorrhizae, and the necessary interactions for soil biogeochemical processes (Ahirwal et al., 2017a; Hofhansl et al., 2020; Werden et al., 2018). Studies reviewed here showed that efficient practices following mining focus on the remediation of soil (Ahirwal et al., 2017b; Castellanos Barliza et al., 2018; Domínguez-Haydar et al., 2019), including removing the TDF topsoil prior to mining and replacing when mining is complete. The SER has proposed to use an intact ecosystem as a reference site to monitor and evaluate the progress of how well a degraded land is recovered (Balensiefer et al., 2004). However, most of the studies reviewed do not compare their results with a preserved ecosystem, mainly explained by two aspects. The first regarding the absence of a plan and appropriate indicators to evaluate the progress of a restoration project, which are usually missing because they are not part of the priorities or objectives (Tallis et al., 2021; Tobón et al., 2017). The second aspect is related to the state of conservation of the ecosystem, because it is one of the most threatened terrestrial ecosystems (Banda et al., 2016; Pennington et al., 2009; Singh and Chaturvedi, 2017), it is difficult for a restoration study to find a reference ecosystem within the same landscape or region against which to compare its restoration results. Rather, progress is evaluated against a similar disturbed site that does not receive treatment and serves as an experimental control. It is important to highlight that these comparisons have some limitations such as: i) not providing information to describe the successional trajectory of a retoration plot; ii) overstating that impact of the restoration effort, since comparing against a degraded site without a treatment may underestimate the real recovery time. However, they do allow to measure and evaluate the recovery of physiognomy, ecosystem functions, functional diversity, among others.
Limitations and conclusions
Our analyses reveal that the predominant strategy for restoring tropical dry forests (TDF) has been planting, a practice that consistently proves costly and yields uncertain results. This strategy often requires supplementary practices to enhance success, typically measured in terms of tree survivorship. This finding is not surprising, as planting desirable species in degraded TDF sites is frequently regarded as a last-resort method for re-establishing tree cover, despite the known impacts of microclimatic conditions and compromised soil health on survivorship. However, it is crucial to acknowledge that, despite ongoing efforts in TDF restoration, the areas covered by these projects are insufficient to mitigate the impacts of deforestation and fires. Our study highlights that, given the critical disturbances affecting the Neotropical region, which is also projected to face severe consequences from climate change (Miles et al., 2006), the average area restored in research studies is usually no larger than 500 ha. Although the Neotropical region boasts the highest number of TDF restoration studies, most of these efforts are academic, focusing on small-scale experimental plots that contribute to our understanding of restoration processes but fail to achieve significant large-scale impacts (Karam-Gemael et al., 2018; Martínez-Garza et al., 2021; Mesa-Sierra et al., 2022a). One of the primary challenges is funding restrictions, which hinder the development of restoration projects that adhere to ecological timelines, including long-term monitoring. Additionally, research plots often do not reflect the operational scale of restoration, as the goals differ markedly between academic studies and practical restoration initiatives. For example, the absence of gray literature or studies published in languages other than English or without an English abstract means that our conclusions do not reflect information on many restoration efforts that in some cases meet our experimental design criteria. Further, the sampling of literature captured in this study may not represent operational scale restoration efforts across TDF, and so should be interpreted cautiously when extrapolating to restoration practice broadly. The findings of this study aim reveal that restoration science currently underrepresents aspects related to social benefits in developing study objectives or metrics. Future TDF restoration projects should aim to integrate various stakeholders, fostering a balance between social and ecological factors. This integration is crucial for establishing a robust framework that facilitates the coordination of long-term landscape-scale projects.
Data availability statement
The original contributions presented in the study are included in the article/Supplementary Material, further inquiries can be directed to the corresponding author.
Author contributions
NM-S: Conceptualization, Data curation, Formal Analysis, Investigation, Methodology, Software, Validation, Visualization, Writing–original draft, Writing–review and editing. MdP-D: Conceptualization, Formal Analysis, Funding acquisition, Investigation, Methodology, Project administration, Resources, Supervision, Validation, Visualization, Writing–original draft, Writing–review and editing. JC: Conceptualization, Funding acquisition, Project administration, Resources, Supervision, Validation, Visualization, Writing–original draft, Writing–review and editing. CG: Conceptualization, Funding acquisition, Project administration, Resources, Supervision, Validation, Visualization, Writing–original draft, Writing–review and editing.
Funding
The author(s) declare that financial support was received for the research, authorship, and/or publication of this article. This study was funded by USDA Forest Service, Pacific Southwest Research Station, Grant number 17-IJ-11272136-045.
Acknowledgments
We are grateful to Ana Karen Pérez Nakashima, Brenda Itzel del Toro López, Samara Lizbet Ledesma Montes, Andrea de las Casas for their help during the revision phase. We also want to thank Anaitzi Rivero-Villar and Gerardo Rodríguez Tapia for their support during the development of this study and the brainstorming sessions. We used ChatGPT as an aid to translate parts of the article.
Conflict of interest
The authors declare that the research was conducted in the absence of any commercial or financial relationships that could be construed as a potential conflict of interest.
The author(s) declared that they were an editorial board member of Frontiers, at the time of submission. This had no impact on the peer review process and the final decision.
Publisher’s note
All claims expressed in this article are solely those of the authors and do not necessarily represent those of their affiliated organizations, or those of the publisher, the editors and the reviewers. Any product that may be evaluated in this article, or claim that may be made by its manufacturer, is not guaranteed or endorsed by the publisher.
Supplementary material
The Supplementary Material for this article can be found online at: https://www.frontiersin.org/articles/10.3389/fenvs.2024.1458613/full#supplementary-material
References
Aerts, R., Negussie, A., Maes, W., November, E., Hermy, M., and Muys, B. (2006). Survival of planted African wild olive seedlings in northern Ethiopian exclosures depends on planting season and shrub cover. J. Dryl. 1 (1), 64–71.
Ahirwal, J., Maiti, S. K., and Satyanarayana Reddy, M. (2017a). Development of carbon, nitrogen and phosphate stocks of reclaimed coal mine soil within 8 years after forestation with Prosopis juliflora (Sw.) Dc. Catena 156, 42–50. doi:10.1016/j.catena.2017.03.019
Ahirwal, J., Maiti, S. K., and Singh, A. K. (2017b). Changes in ecosystem carbon pool and soil CO 2 flux following post-mine reclamation in dry tropical environment, India. Sci. Total Environ. 583, 153–162. doi:10.1016/j.scitotenv.2017.01.043
Alem, S. (2020). Seed bury vs broadcast in direct seeding: their effects on the germination of different woody plant species, in a degraded semi-arid area, Southern Ethiopia. J. Degraded Min. Lands Manag. 7, 2041–2047. doi:10.15243/jdmlm.2020.072.2041
Alvarez-Aquino, C., and Williams-Linera, G. (2012). Seedling survival and growth of tree species: site condition and seasonality in tropical dry forest restoration. Bot. Sci. 90, 341–351. doi:10.17129/botsci.395
Ammondt, S. A., Litton, C. M., Ellsworth, L. M., and Leary, J. K. (2013). Restoration of native plant communities in a Hawaiian dry lowland ecosystem dominated by the invasive grass Megathyrsus maximus. Appl. Veg. Sci. 16, 29–39. doi:10.1111/j.1654-109x.2012.01208.x
Arroyo-Rodríguez, V., Fahrig, L., Tabarelli, M., Watling, J. I., Tischendorf, L., Benchimol, M., et al. (2020). Designing optimal human-modified landscapes for forest biodiversity conservation. Ecol. Lett. 23, 1404–1420. doi:10.1111/ele.13535
Balensiefer, M., Rossi, R., Ardinghi, N., Cenni, M., and Ugolini, M. (2004). SER international primer on ecological restoration.
Banda, K., Delgado-Salinas, A., Dexter, K. G., Linares-Palomino, R., Oliveira-Filho, A., Prado, D., et al. (2016). Plant diversity patterns in neotropical dry forests and their conservation implications. Science. 353, 1383–1387. doi:10.1126/science.aaf5080
Brooks, S., Cordell, S., and Perry, L. (2009). Broadcast seeding as a potential tool to reestablish native species in degraded dry forest ecosystems in Hawaii. Ecol. Restor. 27, 300–305. doi:10.3368/er.27.3.300
Burnett, K. M., Ticktin, T., Bremer, L. L., Quazi, S. A., Geslani, C., Wada, C. A., et al. (2019). Restoring to the future: Environmental, cultural, and management trade-offs in historical versus hybrid restoration of a highly modified ecosystem. Conserva. Letter. 12, e12606.
Brooks, W. R., and Jordan, R. C. (2014). Restoring tropical dry forest communities: effects of habitat management and outplantings on Composition and structure. Restor. Ecol. 22, 160–168. doi:10.1111/rec.12047
Cabin, R. J., Weller, S. G., Lorence, D. H., Cordell, S., Hadway, L. J., Montgomery, R., et al. (2002). Effects of light, alien grass, and native species additions on Hawaiian dry forest restoration. Ecol. Appl. 12, 1595. doi:10.2307/3099925
Campo, J., Giardina, C. P., and Dirzo, R. (2023). Tropical dry forest restoration in an era of global change: ecological and social dimensions. Sustainability 15, 3052. doi:10.3390/su15043052
Carrasco-Carballido, V., Martínez-Garza, C., Jiménez-Hernández, H., Márquez-Torres, F., and Campo, J. (2019). Effects of initial soil properties on three-year performance of six tree species in tropical dry forest restoration plantings. Forests 10, 428. doi:10.3390/f10050428
Castellanos Barliza, J., León Peláez, J. D., and Campo, J. (2018). Recovery of biogeochemical processes in restored tropical dry forest on a coal mine spoil in La Guajira, Colombia. Land Degrad. and Dev. 29, 3174–3183. doi:10.1002/ldr.3069
Ceccon, E., Méndez-Toribio, M., and Martínez-Garza, C. (2020). Social participation in forest restoration projects: insights from a national assessment in Mexico. Hum. Ecol. 48, 609–617. doi:10.1007/s10745-020-00178-w
Ceccon, E., Sánchez, S., and Campo, J. (2004). Tree seedling dynamics in two abandoned tropical dry forests of differing successional status in Yucatán, Mexico: a field experiment with N and P fertilization. Plant Ecol. 170, 277–285. doi:10.1023/B:VEGE.0000021699.63151.47
Chaturvedi, R. K., Pandey, S. K., Rahul, B., Shivam, S., and Raghubanshi, A. S. (2018). Woody species in tropical dry forest exhibit plasticity in physiological traits in response to variations in soil properties. MOJ Ecol. and Environ. Sci. 3, 364–367. doi:10.15406/mojes.2018.03.00114
Chazdon, R. L. (2014). Second growth: the promise of tropical forest regeneration in an age of deforestation. University of Chicago Press.
Chazdon, R. L., Letcher, S. G., van Breugel, M., Martínez-Ramos, M., Bongers, F., and Finegan, B. (2007). Rates of change in tree communities of secondary Neotropical forests following major disturbances. Philosophical Trans. R. Soc. B Biol. Sci. 362, 273–289. doi:10.1098/rstb.2006.1990
Chirwa, P. W., Larwanou, M., Syampungani, S., and Babalola, F. D. (2015). Management and restoration practices in degraded landscapes of Eastern Africa and requirements for up-scaling. Int. For. Rev. 17, 20–30. doi:10.1505/146554815816007009
Colfer, C. J. P., Elias, M., and Jamnadass, R. (2015). Women and men in tropical dry forests: a preliminary review. Int. For. Rev. 17, 70–90. doi:10.1505/146554815815834877
Corona-Núñez, R. O., and Campo, J. (2023). Climate and socioeconomic drivers of biomass burning and carbon emissions from fires in tropical dry forests: a Pantropical analysis. Glob. Change Biol. 29, 1062–1079. doi:10.1111/gcb.16516
Costa, TLSR, Mazzochini, G. G., Oliveira-Filho, A. T., Ganade, G., Carvalho, A. R., and Manhães, A. P. (2021). Priority areas for restoring ecosystem services to enhance human well-being in a dry forest. Restor. Ecol. 29 (7), e13426. doi:10.1111/rec.13426
de la Peña-Domene, M., and Martínez-Garza, C. (2018). Integrating density into dispersal and establishment limitation equations in tropical forests. Forests 9 (9), 570. doi:10.3390/f9090570
de la Peña-Domene, M., Rodríguez Tapia, G., Mesa-Sierra, N., Rivero-Villar, A., Giardina, C. P., Johnson, N. G., et al. (2022). Climatic and edaphic-based predictors of normalized difference vegetation index in tropical dry landscapes: a pantropical analysis. Glob. Ecol. Biogeogr. 31, 1850–1863. doi:10.1111/geb.13565
Derroire, G., Balvanera, P., Castellanos-Castro, C., Decocq, G., Kennard, D. K., Lebrija-Trejos, E., et al. (2016). Resilience of tropical dry forests - a meta-analysis of changes in species diversity and composition during secondary succession. Oikos 125, 1386–1397. doi:10.1111/oik.03229
Dimson, M., and Gillespie, T. W. (2020). Trends in active restoration of tropical dry forest: methods, metrics, and outcomes. For. Ecol. Manag. 467, 118150. doi:10.1016/j.foreco.2020.118150
Dirzo, R., Young, H. S., Mooney, H. A., and Ceballos, G. (2011). Seasonally dry tropical forests: ecology and conservation. Washington: Island Press.
Dominguez-Haydar, Y., Velásquez, E., Carmona, J., Lavelle, P., Chavez, L. F., and Jiménez, J. J. (2019). Evaluation of reclamation success in an open-pit coal mine using integrated soil physical, chemical and biological quality indicators. Ecolo. Indi. 103, 182–193.
Ferreira Nunes, Y. R., Fagundes, N. C. A., Veloso, M. D. M., Gonzaga, A. P. D., Domingues, E. B. S., Almeida, H. S., et al. (2015). Sobrevivência e crescimento de sete espécies arbóreas nativas em uma área degradada de floresta estacional decidual, norte de Minas Gerais. Rev. Árvore 39, 801–810. doi:10.1590/0100-67622015000500003
Gebremedihin, K. M., Birhane, E., Tadesse, T., and Gbrewahid, H. (2018). Restoration of degraded drylands through exclosures enhancing woody species diversity and soil nutrients in the highlands of Tigray, Northern Ethiopia. Nat. Conservation Res. 3 (1), 1–20. doi:10.24189/ncr.2018.001
Gei, M. G., and Powers, J. S. (2013). Do legumes and non-legumes tree species affect soil properties in unmanaged forests and plantations in Costa Rican dry forests? Soil Biol. Biochem. 57, 264–272. doi:10.1016/j.soilbio.2012.09.013
Giardina, C. P., Sanford, R. L., and Døckersmith, I. C. (2000). Changes in soil phosphorus and nitrogen during slash-and-burn clearing of a dry tropical forest. Soil Sci. Soc. Am. J. 64, 399–405. doi:10.2136/sssaj2000.641399x
Goffner, D., Sinare, H., and Gordon, L. J. (2019). The Great green Wall for the sahara and the sahel initiative as an opportunity to enhance resilience in sahelian landscapes and livelihoods. Reg. Environ. Change 19, 1417–1428. doi:10.1007/s10113-019-01481-z
Griscom, H. P. (2020). The long-term effects of active management and landscape characteristics on carbon accumulation and diversity within a seasonal dry tropical ecosystem. For. Ecol. Manag. 473, 118296. doi:10.1016/j.foreco.2020.118296
Griscom, H. P., Ashton, P. M. S., and Berlyn, G. P. (2005). Seedling survival and growth of native tree species in pastures: implications for dry tropical forest rehabilitation in central Panama. For. Ecol. Manag. 218, 306–318. doi:10.1016/j.foreco.2005.08.026
Hedges, L. V., Gurevitch, J., and Curtis, P. S. (1999). The meta-analysis of response ratios in experimental ecology. Ecology 80, 1150–1156. doi:10.2307/177062
Hofhansl, F., Chacón-Madrigal, E., Fuchslueger, L., Jenking, D., Morera-Beita, A., Plutzar, C., et al. (2020). Climatic and edaphic controls over tropical forest diversity and vegetation carbon storage. Sci. Rep. 10, 5066. doi:10.1038/s41598-020-61868-5
Jaganathan, G. K., and Liu, B. (2015). Role of seed sowing time and microclimate on germination and seedling establishment of Dodonaea viscosa (Sapindaceae) in a seasonal dry tropical environment — an insight into restoration efforts. Botany 93, 23–29. doi:10.1139/cjb-2014-0159
Janzen, D. H. (1988). Management of habitat fragments in a tropical dry forest: growth. Ann. Mo. Botanical Gard. 75, 105. doi:10.2307/2399468
Kamelamela, K. L., Springer, H. K., Keakealani, R. K., Ching, M. U., Ticktin, T., Ohara, R. D., et al. (2022). Kōkua aku, kōkua mai: an indigenous consensus-driven and place-based approach to community led dryland restoration and stewardship. For. Ecol. Manag. 506, 119949. doi:10.1016/j.foreco.2021.119949
Karam-Gemael, M., Loyola, R., Penha, J., and Izzo, T. (2018). Poor alignment of priorities between scientists and policymakers highlights the need for evidence-informed conservation in Brazil. Perspect. Ecol. Conservation 16, 125–132. doi:10.1016/j.pecon.2018.06.002
Kukrety, S., Gezan, S., Jose, S., and Alavalapati, J. R. R. (2013). Facilitating establishment of advance regeneration of Pterocarpus santalinus L.-An endangered tree species from India. Restor. Ecol. 21, 372–379. doi:10.1111/j.1526-100x.2012.00890.x
Leverkus, A. B., Lázaro González, A., Andivia, E., Castro, J., Jiménez, M. N., and Navarro, F. B. (2021). Seeding or planting to revegetate the world’s degraded land: systematic review and experimentation to address methodological issues. Restor. Ecol. 29. doi:10.1111/rec.13372
Libby, R., Sato, A. Y., Alapai, L., Brawner, W. P., Carter, Y. Y., Carter, K. A., et al. (2022). A Hawaiian tropical dry forest regenerates: natural regeneration of endangered species under biocultural restoration. Sustainability 14, 1159. doi:10.3390/su14031159
Martínez-Garza, C., Méndez-Toribio, M., Ceccon, E., and Guariguata, M. R. (2021). Ecosystem restoration in Mexico: insights on the project planning phase. Bot. Sci. 99, 242–256. doi:10.17129/botsci.2695
Martínez-Garza, C., Osorio-Beristain, M., Alcalá-Martínez, R. E., Valenzuela-Galván, D., and Mariano, N. (2016). “Eight years of experimental restoration in the seasonal forests of Mexico,” in Experiencias mexicanas en la restauración de los ecosistemas, 385–406.
Mesa-Sierra, N., de la Peña-Domene, M., Campo, J., and Giardina, C. P. (2022b). Restoring Mexican tropical dry forests: a national review. Sustainability 14, 3937. doi:10.3390/su14073937
Mesa-Sierra, N., Laborde, J., Chaplin-Kramer, R., and Escobar, F. (2022a). Carbon stocks in a highly fragmented landscape with seasonally dry tropical forest in the Neotropics. For. Ecosyst. 9, 100016. doi:10.1016/j.fecs.2022.100016
Miles, L., Newton, A. C., DeFries, R. S., Ravilious, C., May, I., Blyth, S., et al. (2006). A global overview of the conservation status of tropical dry forests. J. Biogeogr. 33, 491–505. doi:10.1111/j.1365-2699.2005.01424.x
Mirzabaev, A., Sacande, M., Motlagh, F., Shyrokaya, A., and Martucci, A. (2022). Economic efficiency and targeting of the african Great green Wall. Nat. Sustain. 5, 17–25. doi:10.1038/s41893-021-00801-8
Montoya, L. E., Corona-Núñez, R. O., and Campo, J. (2023). Fires and their key drivers in Mexico. Int. J. Wildland Fire 32 (5), 651–664. doi:10.1071/wf22154
Murphy, P. G., and Lugo, A. E. (1986). Ecology of tropical dry forest. Annu. Rev. Ecol. Syst. 17 (1), 67–88. doi:10.1146/annurev.es.17.110186.000435
Newton, A. C., del Castillo, R. F., Echeverría, C., Geneletti, D., González-Espinosa, M., Malizia, L. R., et al. (2012). Forest landscape restoration in the drylands of Latin America. Ecol. Soc. 17, art21. doi:10.5751/es-04572-170121
Olson, D. M., Dinerstein, E., Wikramanayake, E. D., Burgess, N. D., Powell, G. V. N., Underwood, E. C., et al. (2001). Terrestrial ecoregions of the world: a new map of life on Earth. BioScience 51, 933. doi:10.1641/0006-3568(2001)051[0933:teotwa]2.0.co;2
Omkar, K., Suthari, S., Alluri, S., Ragan, A., and Raju, V. S. (2012). Diversity of NTFPs and their utilization in adilabad district of Andhra Pradesh, India. J. Plant Stud. 1, 33. doi:10.5539/jps.v1n1p33
Pennington, R. T., Lavin, M., and Oliveira-Filho, A. (2009). Woody plant diversity, evolution, and ecology in the tropics: perspectives from seasonally dry tropical forests. Annu. Rev. Ecol. Evol. Syst. 40, 437–457. doi:10.1146/annurev.ecolsys.110308.120327
Portillo-Quintero, C., Sanchez-Azofeifa, A., Calvo-Alvarado, J., Quesada, M., and do Espirito Santo, M. M. (2015). The role of tropical dry forests for biodiversity, carbon and water conservation in the neotropics: lessons learned and opportunities for its sustainable management. Reg. Environ. Change 15, 1039–1049. doi:10.1007/s10113-014-0689-6
Rivero-Villar, A., de la Peña-Domene, M., Rodríguez Tapia, G., Giardina, C. P., and Campo, J. (2022). A pantropical overview of soils across tropical dry forest ecoregions. Sustainability 14, 6803. doi:10.3390/su.14116803
Sangsupan, H. A., Hibbs, D. E., Withrow-Robinson, B. A., and Elliott, S. (2018). Seed and microsite limitations of large-seeded, zoochorous trees in tropical forest restoration plantations in northern Thailand. For. Ecol. Manag. 419–420, 91–100. doi:10.1016/j.foreco.2018.03.021
Sheoran, V., Sheoran, A. S., and Poonia, P. (2010). Soil reclamation of abandoned mine land by revegetation: a review. Int. J. soil, sediment water 3 (2), 13. doi:10.3390/su11123393
Silveira, F. A. O., Ordóñez-Parra, C. A., Moura, L. C., Schmidt, I. B., Andersen, A. N., Bond, W., et al. (2021). Biome Awareness Disparity is BAD for tropical ecosystem conservation and restoration. J. Appl. Ecol. 59, 1967–1975. doi:10.1111/1365-2664.14060
Singh, A. N., Raghubanshi, A. S., and Singh, J. S. (2004). Impact of native tree plantations on mine spoil in a dry tropical environment. For. Ecol. Manag. 187, 49–60. doi:10.1016/s0378-1127(03)00309-8
Singh, J. S., and Chaturvedi, R. K. (2017). Tropical dry deciduous forest: research trends and emerging features. Springer.
Stan, K. D., Sanchez-Azofeifa, A., Duran, S. M., Guzmán Quesada, J. A., Hesketh, M., Laakso, K., et al. (2021). Tropical dry forest resilience and water use efficiency: an analysis of productivity under climate change. Environ. Res. Lett. 16 (5), 054027. doi:10.1088/1748-9326/abf6f3
Stoner, K. E., and Sánchez-Azofeifa, G. A. (2009). Ecology and regeneration of tropical dry forests in the Americas: implications for management. For. Ecol. Manag. 258, 903–906. doi:10.1016/j.foreco.2009.05.019
Tallis, H., Fargione, J., Game, E., McDonald, R., Baumgarten, L., Bhagabati, N., et al. (2021). Prioritizing actions: spatial action maps for conservation. Ann. N. Y. Acad. Sci. 1505 (1), 118–141. doi:10.1111/nyas.14651
Tarrasón, D., Urrutia, J. T., Ravera, F., Herrera, E., Andrés, P., and Espelta, J. M. (2010). Conservation status of tropical dry forest remnants in Nicaragua: do ecological indicators and social perception tally? Biodivers. Conservation 19, 813–827. doi:10.1007/s10531-009-9736-x
Thakur, T. K., Patel, D. K., Thakur, A., Kumar, A., Bijalwan, A., Bhat, J. A., et al. (2021). Biomass production assessment in a protected area of dry tropical forest ecosystem of India: a field to satellite observation approach. Front. Environ. Sci. 9, 757976. doi:10.3389/fenvs.2021.757976
Tobón, W., Urquiza-Haass, T., Koleff, P., Schroeter, M., Ortega-Álvarez, R., Campo, J., et al. (2017). Restoration planning to guide Aichi targets in a megadiverse country. Conserv. Biol. 31, 1086–1097. doi:10.1111/cobi.12918
Trejo, I., and Dirzo, R. (2000). Deforestation of seasonally dry tropical forest: a national and local analysis in Mexico. Biol. Conserv. 94 (2), 133–142. doi:10.1016/s0006-3207(99)00188-3
Tummers, B. (2006). DataThief III. Available at: https://datathief.org/.
Viechtbauer, W. (2010). Conducting meta-analyses in R with the metafor package. J. Stat. Softw. 36, 1–48. doi:10.18637/jss.v036.i03
Wade, T. I., Ndiaye, O., Mauclaire, M., Mbaye, B., Sagna, M., Guissé, A., et al. (2018). Biodiversity field trials to inform reforestation and natural resource management strategies along the African Great Green Wall in Senegal. New For. 49, 341–362. doi:10.1007/s11056-017-9623-3
Werden, L. K., Alvarado, J. P., Zarges, S., Calderón, M. E., Schilling, E. M., Gutiérrez, L. M., et al. (2018). Using soil amendments and plant functional traits to select native tropical dry forest species for the restoration of degraded Vertisols. J. Appl. Ecol. 55, 1019–1028. doi:10.1111/1365-2664.12998
Keywords: ecological restoration, meta-analysis, reference systems, systematic review, tropical dry forest
Citation: Mesa-Sierra N, de la Peña-Domene M, Campo J and Giardina CP (2025) Restoration of tropical dry forest: an analysis of constraints and successes across a highly threatened biome. Front. Environ. Sci. 12:1458613. doi: 10.3389/fenvs.2024.1458613
Received: 02 July 2024; Accepted: 09 December 2024;
Published: 07 January 2025.
Edited by:
Vinicius Londe, University of Georgia, United StatesReviewed by:
Abrham Abiyu, World Agroforestry, EthiopiaLeland Kendall Werden, ETH Zürich, Switzerland
Copyright © 2025 Mesa-Sierra, de la Peña-Domene, Campo and Giardina. This is an open-access article distributed under the terms of the Creative Commons Attribution License (CC BY). The use, distribution or reproduction in other forums is permitted, provided the original author(s) and the copyright owner(s) are credited and that the original publication in this journal is cited, in accordance with accepted academic practice. No use, distribution or reproduction is permitted which does not comply with these terms.
*Correspondence: Marinés de la Peña-Domene, bWFyaW5lc3BkQGl0ZXNvLm14