- 1Instituto Politécnico Nacional, Escuela Nacional de Ciencias Biológicas, Laboratorio de Evaluación de la Salud de los Ecosistemas Acuáticos, Ciudad de México, México
- 2Instituto Politécnico Nacional, Coordinación Politécnica para la Sustentabilidad, Ciudad de México, México
Microplastics (MPs) are considered pollutants of emerging concern. In rivers, MPs (≤5 mm) are transported by currents and deposited in lakes, reservoirs, estuaries, and oceans. In Mexico, MPs in freshwater ecosystems have been scarcely studied. This work aimed to determine the presence of MPs in rivers of the Sierra Gorda Biosphere Reserve (SGBR). Samples of surface water and sediment collected from the Escanela, Jalpan, Ayutla, and Santa María rivers were processed. MPs in water were extracted by direct filtration using nitrocellulose filters with a 1.2 μm pore size; MPs in sediments were extracted by density suspension using a ZnCl2 solution (ρ ≈ 1.5 g/cm3). The surface of MPs was examined using SEM-EDX, and spectra of trace elements deposited on the MPs were obtained; additionally, MP polymers were characterized with FTIR. Fibers were the most abundant MP forms in both matrices. Up to 110.87 items L−1 were detected in water and 175 items kg−1 (dry weight) in sediments. Surface wear of MPs was observed, and trace elements were detected, including Al, Fe, Cr, Si, and Zn, among others. The polymers identified in MP samples were polyethylene terephthalate, polyester, polypropylene, high-density polyethylene, polyamide, and polystyrene. The test results revealed the presence of MPs with trace metals on their surface in the freshwater ecosystems of the SGBR, which may be related to mining operations in the study area, changes in land use, wastewater discharges, atmospheric transport, and dry and wet deposition. These findings demonstrated the ubiquity of these pollutants and their presence even in areas intended for conservation.
1 Introduction
Today, synthetic polymers commonly known as plastics are indispensable in human life due to their low cost, resistance to corrosion, and light weight, among other characteristics (Brandsch and Piringer, 2007; Jambeck et al., 2015). Since the 1950s, these materials have been produced uninterruptedly. The global annual production of plastics has exceeded 390.7 million tons (Mt), 4% more than in 2020, when the COVID-19 pandemic was declared (Plastics Europe, 2022). In addition, large amounts of plastic waste are produced each year. It is estimated that nearly 12 thousand Mt of plastics will be disposed of in the environment by the year 2050 (Geyer et al., 2017). Only a small amount of plastics is being recycled; the rest end up in landfills, wastewater, and aquatic ecosystems (Tofa et al., 2019). Globally, rivers transport between 1.15 and 2.41 Mt of plastics to the oceans each year (Lebreton et al., 2017).
Small plastic particles, known as microplastics (MPs), are emitted and deposited in the environment from plastic production and pollution. Sources of MPs include sewage, agriculture, fisheries, and tourism, to name a few (Allen et al., 2022). According to their origin, MPs can be classified as primary and secondary. Primary MPs are manufactured in sizes <5 mm, while secondary MPs attain that size due to the physical, chemical, and biological degradation and fragmentation of larger plastic waste items (Shahnawaz et al., 2019; Badea et al., 2023). Polymers such as polypropylene, polyethylene, polystyrene, polyethylene terephthalate, polyvinyl chloride, and others have been frequently found in MPs (Burns and Boxall, 2018; Schell et al., 2020). MPs have different shapes, including fibers, fragments, spheres, foams, and films, as well as various colors (Rochman et al., 2019; Kutralam-Muniasamy et al., 2020; Badea et al., 2023).
The input and presence of MPs in rivers have been associated with several factors. MPs can be discharged into wastewater from the use of cleaning and personal care products and as industrial waste (Reddy and Nair, 2022). The smallest and lightest particles are suspended in the atmosphere, carried by the wind, and deposited in areas far from the place of emission; atmospheric transport is a route for the transfer and subsequent deposition of MPs in remote and pristine areas (Evangeliou et al., 2020; Su et al., 2022). Rainfall plays a central role; on the one hand, it contributes to the moist deposition of MPs from the atmosphere on soils, and on the other, surface runoff is a carrier transporting MPs from soil to water bodies (Wei et al., 2023). Within rivers, MPs can also emerge from fragmentation, wear, and tear of plastic waste, including bottles, containers, bags, textiles, and face masks, among others (Badea et al., 2023; Kaur et al., 2023). Eventually, these particles are carried by currents and deposited in lakes, reservoirs, estuaries, and oceans.
In recent years, MPs have been considered pollutants of emerging concern. These can be ingested, bioaccumulated, and biomagnified through food webs, thereby posing risks to ecosystems and human health (Eerkes-Medrano et al., 2015; Rochman et al., 2019; Kutralam-Muniasamy et al., 2020; Nantege et al., 2023). MP intake has been reported in fish (Sulaiman et al., 2023), birds (Hoang and Mitten, 2022), aquatic macroinvertebrates (Nantege et al., 2023), and even humans (Malafaia and Barceló, 2023). The different polymers and additives (plasticizers, flame retardants, and antioxidants, among others) used to improve the physical properties of plastics can be toxic to organisms (Lithner et al., 2011; Hahladakis et al., 2018; Sridharan et al., 2022). Furthermore, MPs can be vectors of microorganisms (biofilm) and organic matter and carriers of traces of pollutants such as pesticides, drugs, personal care products, and metals that increase the potential hazards of MPs (Atugoda et al., 2021; Cao et al., 2021; Cormier et al., 2021; Kinigopoulou et al., 2022).
Most studies worldwide have focused on marine environments and, to a lesser extent, on epicontinental systems (D’Avignon et al., 2022). Studies in Latin America and the Caribbean represent only 4.8% of global scientific production, with Mexico contributing approximately 0.63% (Orona-Návar et al., 2022). On the other hand, the ubiquity of MPs in remote areas has been demonstrated, having been found in freshwater, ice, and snow in the Arctic and Antarctic (Citterich et al., 2023), even in places like the Byers Peninsula, which has been designated Antarctic Specially Protected Area No. 126 (González-Pleiter et al., 2020; ATCM, 2022). MPs have been recorded in sediment, water, and biota in several protected areas around the world, being an issue that should be addressed (Kutralam-Muniasamy et al., 2021). In Mexico, only one of the country’s most polluted rivers, the Atoyac River, has been examined for the presence of MPs in sediments (Shruti et al., 2019); therefore, the presence of MPs in freshwater bodies within conservation areas such as Protected Natural Areas (PNAs) remains unknown. This work aimed to determine the presence and degree of MP pollution in aquatic ecosystems flowing through the Sierra Gorda Biosphere Reserve (SGBR) and to evaluate the potential sources of MP pollution and transport routes based on the analysis of multiple variables and environmental traits. To our knowledge, this comprehensive study investigates MP pollution and sources in surface water and sediments of freshwater ecosystems in a poorly addressed region of the world. Specifically, this is the first study focused on freshwater bodies within a Protected Natural Area (PNA) in Mexico.
2 Materials and methods
2.1 Study area
The SGBR is one of the most valuable PNAs in Mexico. This Reserve covers 3,836 km2 and is located in the Central Mexican Highlands. The altitudinal range is 300–3,160 m a.s.l. It hosts a diverse vegetation (pine forest, oak forest, deciduous forest, and xeric scrub). The local climate varies from semi-warm to warm and sub-humid to semi-dry, with a mean annual temperature mostly above 18°C and precipitation above 80 mm in the rainy season (Supplementary Figure S1). Escanela, Jalpan, Ayutla, and Santa María are tributaries of the Tamuín River, which flows to the northeast of the PNA to join the Pánuco River, in turn reaching the Gulf of Mexico (Carabias et al., 1999). The Jalpan Reservoir is located at the confluence of the Escanela and Jalpan rivers; it was declared a Ramsar site (designation number 1352) as a wetland of global importance as it meets conservation criteria 2 and 4 (Pedraza-Ruiz, 2004). The SGBR comprises 11 core areas where activities are mainly limited to conservation and research (Ejecutivo, 1997). Two main towns, Ahuacatlán and Jalpan, are located adjacent to the Escanela and Jalpan rivers, as well as several small towns in the SGBR, which are home to approximately 95,000 inhabitants (INEGI, 2021). Tourism, sport fishing, mining, and farming are some of the activities in the Reserve. Mining is common in the region, with over 100 mines, mostly artisanal, located within the reserve polygon (SGM, 2024).
2.2 Field sampling and environmental variables
Ten sampling sites were selected in the Reserve rivers and reservoir: Escanela River, four sites; Jalpan River, two sites; Jalpan Reservoir, one site on its tributary and one on its effluent; Ayutla River, one site; Santa Maria River, one site (Figure 1). Environmental matrices were sampled, and environmental variables were monitored in October 2022, which corresponds to the rainy season (Supplementary Figure S1). Physicochemical parameters such as temperature (°C), dissolved oxygen (mg L−1), oxygen saturation (%), conductivity (mS cm−1), turbidity (NTU), and salinity (UPS) were recorded in situ at each study site using a multiparameter probe (Hydrolab®, Quanta). In addition, 500 mL of water was collected in duplicate polyethylene containers for physicochemical testing, plus 100 mL in sterile bags (Whirl-Pak®) for laboratory microbiological tests. The following parameters were determined in the laboratory: total nitrogen (NT, mg L−1), nitrites (NO2, mg L−1), nitrates (NO3, mg L−1), ammonia nitrogen (NH3, mg L−1), sulfates (SO4, mg L−1), orthophosphates (PO4, mg L−1), total phosphorus (PT, mg L−1), color (Pt-Co), and total suspended solids (TSS, mg L−1), according to the techniques of the HACH DR3900 spectrophotometer; hardness (CaCO3, mg L−1), alkalinity (CaCO3, mg L−1), chlorides (Cl, mg L−1), 5-day biochemical oxygen demand (BOD5, mg L−1), and fecal and total coliforms (MPN 100 mL−1) were determined following American Public Health Association techniques (Baird et al., 2017).
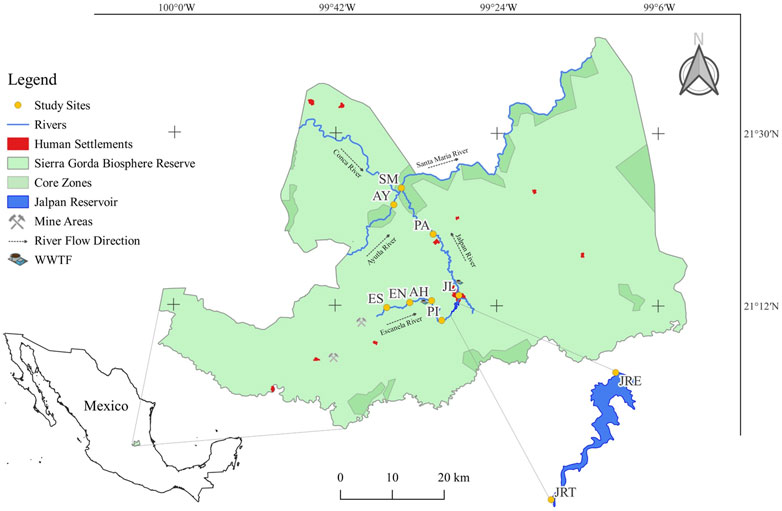
Figure 1. Sierra Gorda biosphere reserve, Queretaro. Escanela River: PI, Pizquintla; AH, Ahuacatlán; EN, Escanelilla; ES, Escanela; Jalpan Reservoir: JRT, Jalpan Reservoir Tributary; JRE, Jalpan Reservoir Effluent. Jalpan River: PA, Purísima; JL, Jalpan. Santa Maria River: SM, Santa María. Ayutla River: AY, Ayutla. WWTF, Wastewater Treatment Facility.
2.3 Characterization of study sites: land use, NDVI, and WQI
The percentage of land use, the Normalized Difference Vegetation Index (NDVI), and the Water Quality Index (WQI) were calculated to characterize the study sites. The land uses considered were natural vegetation, secondary vegetation, induced pastures, agriculture, and human settlements. The percentage of each land use was estimated considering a polygon comprising 2 km upstream of each study site and 0.5 km on both sides (Rodríguez-Romero et al., 2021). Polygons were established from a Land Use and Vegetation map issued by Mexico’s National Institute of Statistics, Geography, and Informatics (INEGI, in Spanish). Moreover, NDVI was calculated with Landsat 9 OLI TIRS imagery from the USGS viewer for the study period and with the following equation:
where B5 is the reflectance value in the near-infrared light band, and B4 is the reflection value of the visible red band (Tarpley et al., 1984). This index ranges from −1 to +1, where values close to one indicate that the vegetation is more vigorous. This work established four categories based on Hartoyo et al. (2022) to grade the vegetation condition (Table 1).
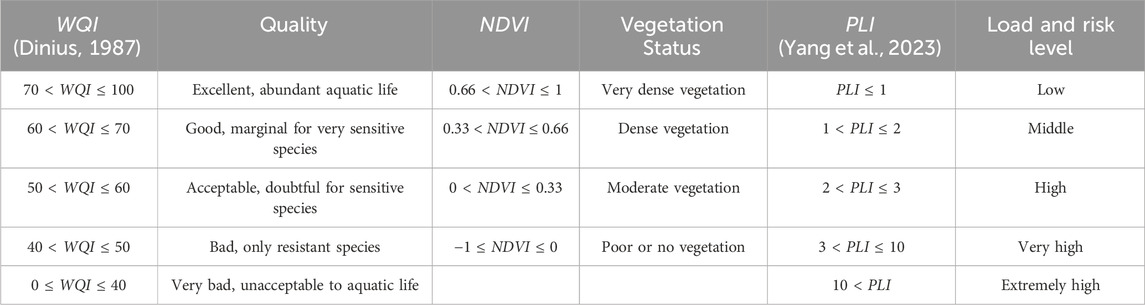
Table 1. Categories used for the Water Quality Index (WQI), the Normalized Difference Vegetation Index (NDVI), and the Pollution Load Index (PLI).
On the other hand, the WQI proposed by Dinius (1987) was calculated with the following equation:
where i indicates a parameter, Ii is the subscript in parameter i, Wi is the weighted value of parameter i, and n is the number of parameters. This index ranges from 0 to 100, where values close to 100 indicate better water quality (Table 1). The parameters considered to calculate the index were dissolved oxygen, biochemical oxygen demand, nitrates, hardness, color, fecal and total coliforms, alkalinity, chlorides, conductivity, air and water temperature, and pH.
2.4 Extraction and characterization of microplastics
Surface water and sediment samples were collected from each site and placed in 500 mL glass containers with metal caps. Sediment composite samples were collected with a metallic shovel, taking into account three sections of the streambed, the central and both littoral zones of rivers. Water surface samples were collected in the same sections of the river as those of sediment samples. MPs in water were extracted by direct filtration using nitrocellulose filters (1.2 μm pore size and 47 mm in diameter; MF-Millipore™) and a glass filtration system (WHEATON®). MPs in the sediments were extracted using the density suspension method (Imhof et al., 2012; Li et al., 2018; Debraj and Lavanya, 2023). Samples were oven-dried at 40°C ± 1°C and then sieved through a 4.75 mm mesh size. From the resulting sediment, four 20 g subsamples were individually placed in a glass flask. As a pretreatment, 25 mL of 30% H2O2 was added to remove organic waste. Then, 50 mL of a ZnCl2 solution (ρ ≈ 1.5 g/cm3) was added to each flask, stirred, and left to stand for 48 h. Subsequently, the solution was filtered using the method for water samples. MPs concentrations in water and sediment were expressed as the number of items per liter and the number of items per kilogram (dry weight), respectively. Additionally, for sediments, the mean grain size was determined by a granulometric analysis as per the D422-63 methods of the American Society for Testing and Materials (ASTM, 2007).
MPs were characterized in terms of shape and color under a stereo microscope (Leica®, DMS1000). Subsequently, the morphology and elemental composition of the surface were analyzed using scanning electron microscopy (SEM; FEI®, Quanta FEG 250) equipped with an X-ray energy dispersed spectroscopy (EDX) system (Bruker®, XFlash 6160) (Li et al., 2018). The identified polymer type with FTIR was a percentage (12%) randomly selected from all particles extracted on the filters, following European Commission (2013) that suggests subsamples of 10%. The polymeric composition of MPs was determined with Fourier-transform infrared spectroscopy (FTIR; SHIMADZU®, IRAffinity-1S) (Debraj and Lavanya, 2023); polymers were characterized by comparing the resulting spectra with the “Open Specy” open-access database (Cowger et al., 2021). The MP concentrations were proportionally adjusted according to FTIR results. About 10% of analyzed particles were no-plastic. Microstructure tests were carried at the Centro de Desarrollo de Productos Bióticos (Center for the Development of Biotic Products; CEPROBI, in Spanish) and the Centro de Nanociencias y Micro y Nanotecnologías (Center for Nanosciences and Micro and Nanotechnologies; CNMN, in Spanish) of the Instituto Politécnico Nacional (National Polytechnic Institute).
2.4.1 Control measures
Several control procedures were applied to avoid external contamination by MPs during sample processing. Glass and metal were the only materials allowed during sample processing; aluminum foil was used to cover the containers. Before use, collection containers and laboratory instruments were washed and then rinsed with filtered deionized water. All the solutions used were filtered. Only cotton laboratory coats and polymer-free gloves were used during sample processing. Samples were processed under a laminar-flow hood, and the windows were kept closed to prevent air currents. The stereo microscope and work area were thoroughly cleaned before each use. Each process included the collected samples and two control samples.
2.5 Microplastic pollution
The Pollution Load Index (PLI) was calculated to assess the degree of MP pollution (Tomlinson et al., 1980; Huang et al., 2023; Yang et al., 2023; Yin and Zhao, 2023). PLI was calculated with the following equations:
where i represents a study site and n is the number of sites located in rivers. The PLI integrates a concentration factor (CFi), which is the concentration of MPs at each site (Ci) divided by the minimum concentration reported in the literature (Co). Given the lack of previous data for the study area, the lowest MPs concentration recorded in this study was used as Co, as suggested by other studies (Kabir et al., 2021; Neelavannan et al., 2022). This index considers five categories of pollution load and its risk level (Table 1).
2.6 Data analysis
The statistical processing and graphical representation of the data were performed using the XLSTAT 2023.1.2 and R 4.3.2 software. Geospatial data management was performed with QGIS 3.22.13. The Mann-Whitney U test was used to detect differences between MPs in surface water and MPs in sediment. Pearson’s multiple correlation was calculated to explore potential relationships between environmental variables and MP abundance. A principal component analysis (PCA) using Pearson’s correlation index was used to explore the relationship between environmental variables, spatial characteristics, and study sites. Thus, we included the environmental variables: land use (%), altitude, indices (PLI-water and PLI-sediments, NDVI, WQI) current velocity, mean grain size, and depth to assess the significance of parameters that explain the patterns of microplastics detected on the study sites. PCA is an effective method to analyze multi-attribute data because reduces the number of dimensions (variables) in large datasets to principal components that retain most of the original information. The PCA transforms potentially correlated variables into a smaller set of uncorrelated variables called principal components. The so-called “principal components” can be displayed in a new coordinate system where the projected data in the first coordinate represents the highest variance followed by the projected data in the second coordinate representing the second highest variance and so on. To compute the PCA, the data were first log-transformed [ln (x+1)] and a factor analysis was carried out to exclude redundant variables (Romía and Bernàrdez, 2009; Cao et al., 2020; Ibrahim et al., 2023). We use the eigenvalue-one criterion of Kowalkowski et al. (2006) to select the number of components to be described. The thresholds for significant differences were p < 0.05.
3 Results
3.1 Environmental characteristics
The physicochemical parameters (Supplementary Table S1) were included in a multiple correlation analysis. There were significant positive and negative relationships between the different variables. Some variables were also correlated with MP concentration and PLI in water and sediment (Supplementary Figure S3). On the other hand, the analysis of land uses showed that native vegetation predominated in sites SM and AY (79.90% and 69.17%, respectively), followed by induced pastures and agriculture. In the remaining sites, secondary vegetation predominated, and human settlements were present. In JL, human settlements accounted for 55.55% of land use, while in PA, agriculture accounted for 80.07%. From ES to JRE, the predominant land use was secondary vegetation (Figure 2A). The NDVI showed that vegetation was vigorous in most study sites, except for JRE, JL, and PA, where vegetation quality was moderate; some areas in JRE and JL showed values very close to zero, indicating poor vegetation quality or no vegetation (bare or impermeable soil) (Figure 2B). According to the WQI scores, sites EN, PI, JRE, JL, and SM achieved Excellent quality, while ES, AH, PA, and AY had Good quality; in contrast, JRT had Acceptable quality (Figure 2C). Finally, the grain size analysis showed that PA had the largest mean grain size (3,400 μm) and PI had the smallest (890 μm); the mean size was 2033 μm (Supplementary Figure S2).
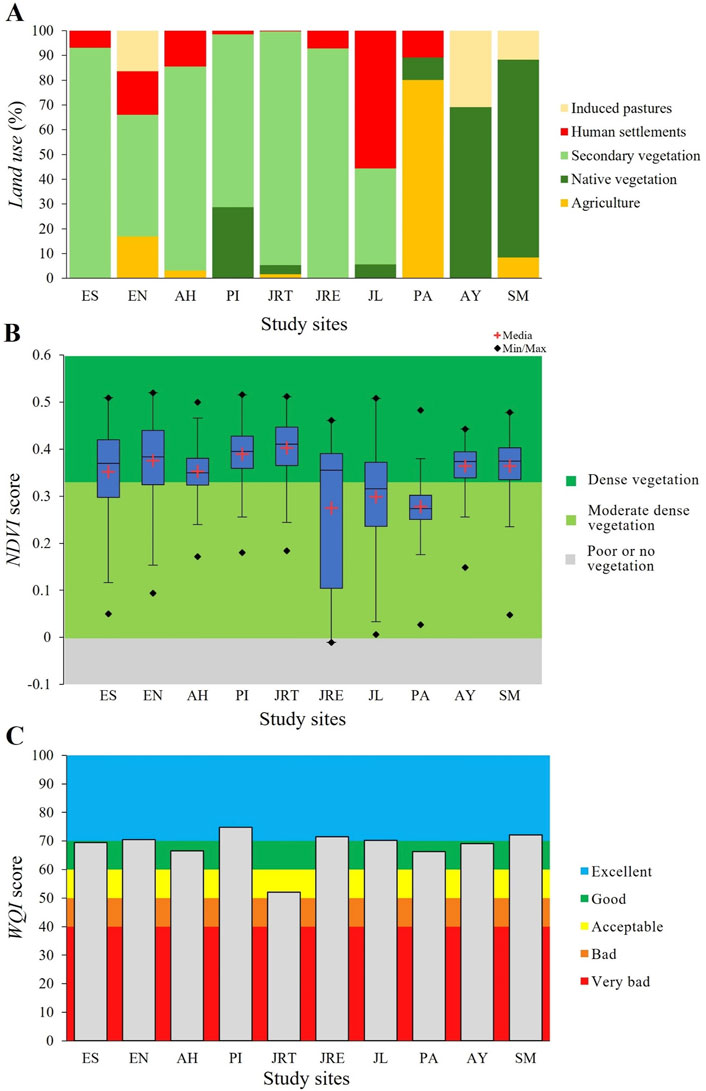
Figure 2. Environmental attributes at the study sites. (A) Percentage of land use; (B) Normalized Difference Vegetation Index (NDVI); (C) Water Quality Index (WQI). PI, Pizquintla; AH, Ahuacatlán; EN, Escanelilla; ES, Escanela; JRT: Jalpan Reservoir Tributary; JRE: Jalpan Reservoir Effluent; PA, Purísima; JL, Jalpan; SM, Santa María; AY, Ayutla.
3.2 Microplastics in SGBR freshwater ecosystems
Plastic waste, including bags, bottles, sandals, tires, fishing nets, and others, was detected on the riverbed and river slopes (Supplementary Figure S4). MPs were also found; the highest amount of MPs in water was found at site ES (110.87 items L−1), and a lower number was observed at sites JRE, JL, and SM (4.76 items L−1, 4.49 items L−1, and 4.35 items L−1, respectively) (Figure 3). In sediments, up to 175 items kg−1 were found at site ES, while the lowest concentration was 12.5 items kg−1 at sites PI and AY (Figure 3). The mean MP levels were 28.8 items L−1 in water and 55 items kg−1 in sediments. These results revealed the presence of MPs in rivers located within the SGBR. Fibers were the predominant MP forms in water and sediments; however, fragments, films, and spheres were also found (Figures 4A, 5). In addition, MPs of various colors were identified: in water, blue (32%), orange (11%), green (10%) and others; in sediments, blue (29%), black (29%), gray (8%) and others; in both environments, colorless MPs were observed (37% and 21%, respectively) (Figures 4B, 5).
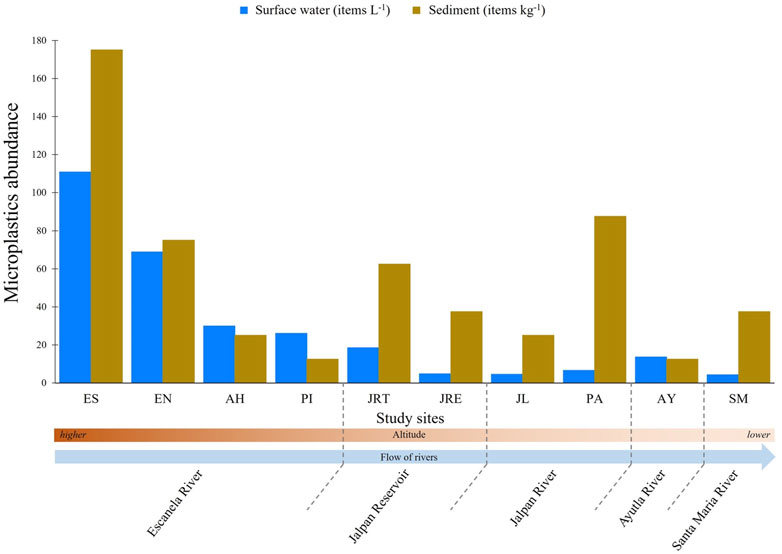
Figure 3. Abundance of MPs in surface water and sediment at study sites across the SGBR freshwater systems. PI, Pizquintla; AH, Ahuacatlán; EN, Escanelilla; ES, Escanela; JRT, Jalpan Reservoir Tributary; JRE, Jalpan Reservoir Effluent; PA, Purísima; JL, Jalpan; SM, Santa María; AY, Ayutla.
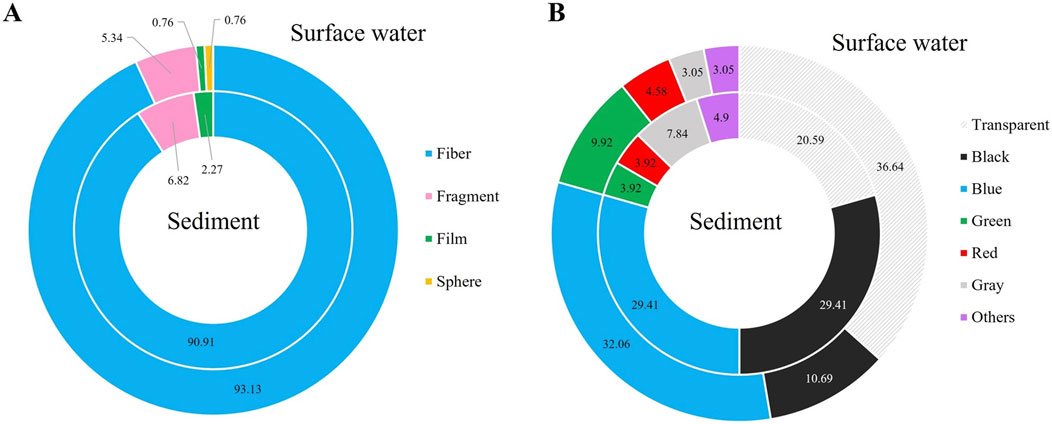
Figure 4. Shapes and colors of MPs extracted from surface water and sediment. (A) Percentage of different forms of MPs; (B) Percentage of MP colors. Outer circle: surface water; inner circle: sediment.
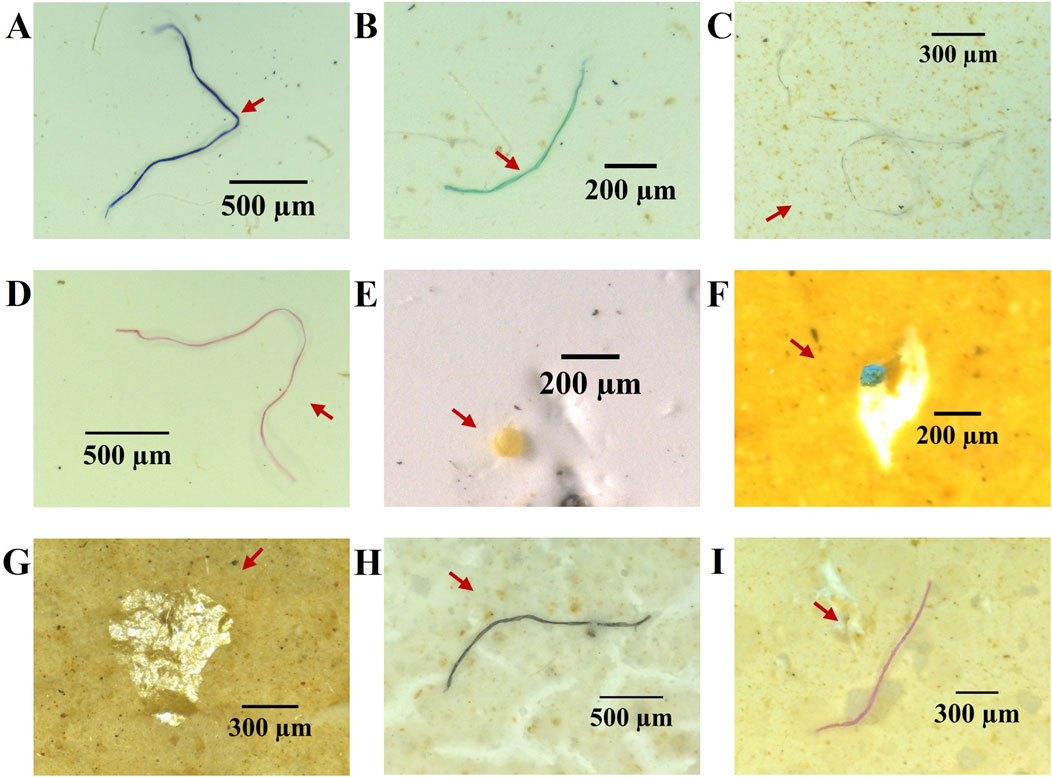
Figure 5. Images of some MPs found in the rivers of the SGBR. (A–E) MPs extracted from surface water samples; (F–I) MPs extracted from sediment samples.
MPs showed an irregular surface and aggregations of various elements. EDX confirmed the presence of several elements such as C, O, S, Cl, K, Ca, Si and traces of metallic elements such as Al, Fe, Zn, Cr, Au, Ti, and V on the surface of the MPs (Figure 6). The polymers identified with FTIR were polyethylene terephthalate, polyester, polypropylene, polystyrene, high-density polyethylene, and polyamide (Figure 7). In addition to detecting MPs, the degree of MP pollution was estimated using the Pollution Load Index (PLI). The pollution loads in the freshwater ecosystems studied were PLI values of 1.90 in water and 1.78 in sediment, corresponding to the “medium” category. A “high” MP load was calculated for surface water at sites ES in the Escanela River and JRT at the entrance of the reservoir, and for sediments at sites ES and EN (Figure 8).
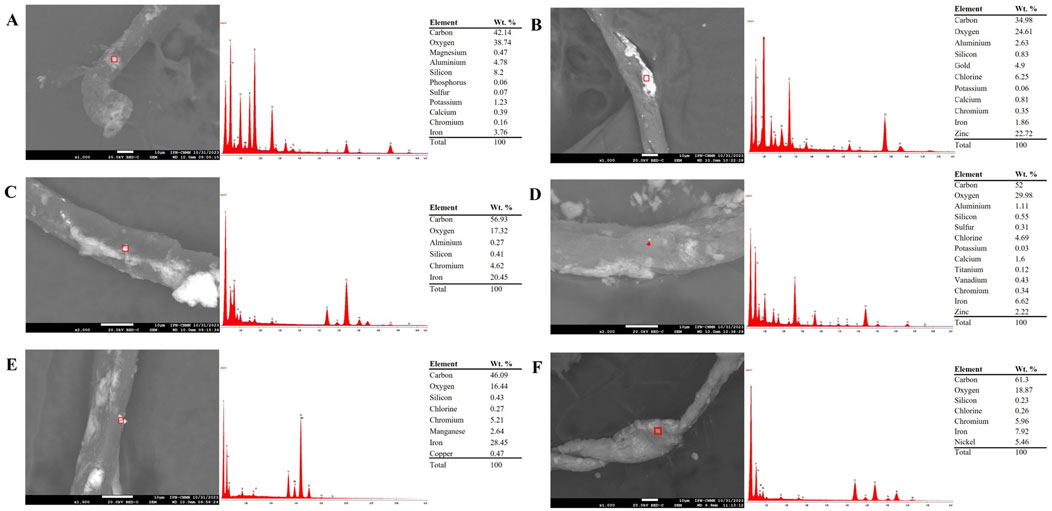
Figure 6. SEM-EDX microscopy with a semi-quantitative analysis of mass percentages of trace elements found on MP surfaces. (A–F) Images and elemental analysis of some microplastics found in rivers of the SGBR.
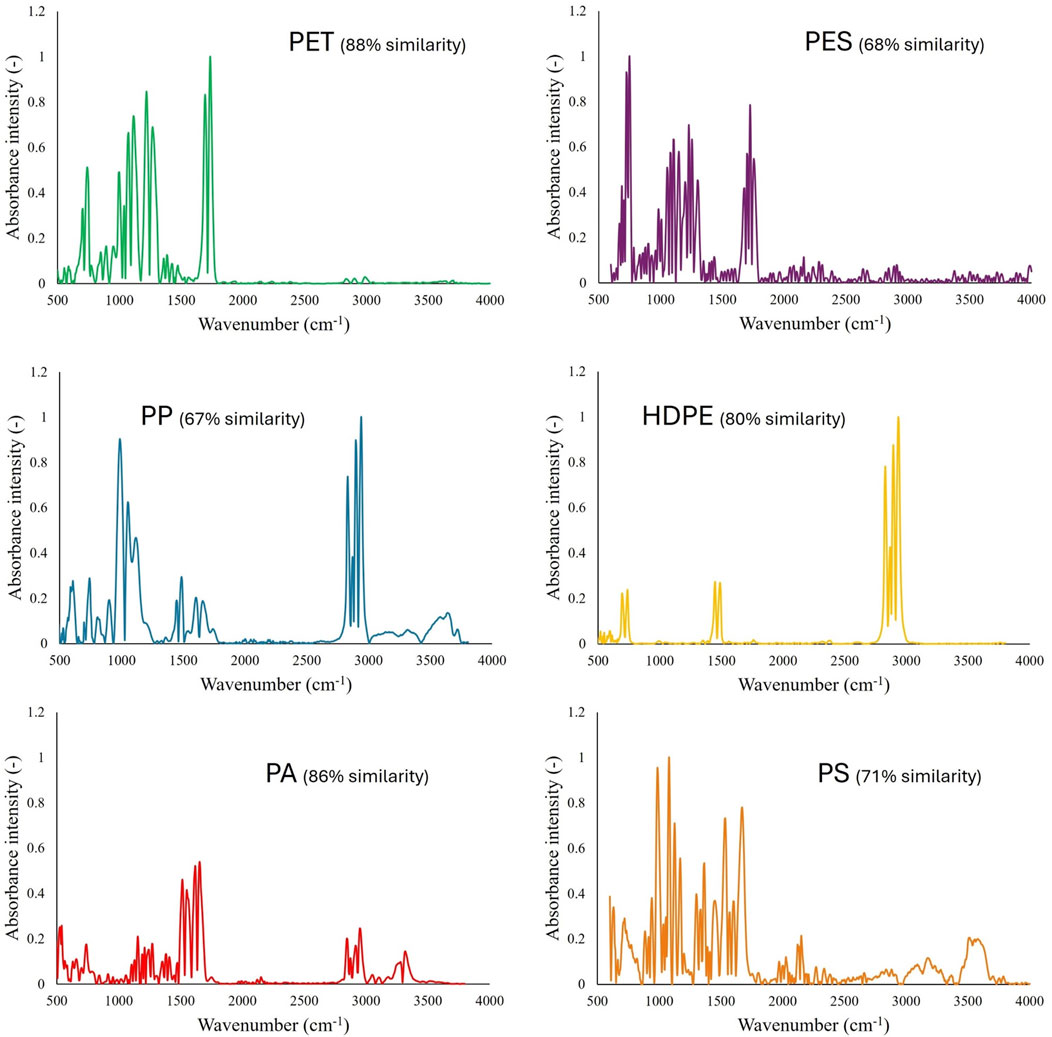
Figure 7. Spectra of the polymers identified in some MPs extracted from water and sediments from the rivers of the SGBR. PET, polyethylene terephthalate; PES, polyester; PP, polypropylene; PS, polystyrene; HDPE, high-density polyethylene; PA, polyamide.
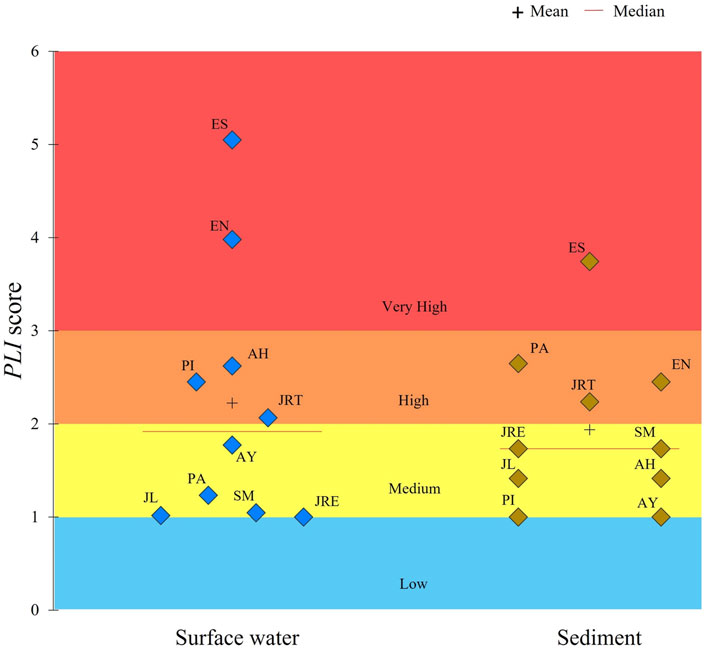
Figure 8. Pollution Load Index (PLI) in surface water and sediments. PLI values are represented for each study site. Mann-Whitney U test, p = 0.781. PI, Pizquintla; AH, Ahuacatlán; EN, Escanelilla; ES, Escanela; JRT, Jalpan Reservoir Tributary; JRE, Jalpan Reservoir Effluent; PA, Purísima; JL, Jalpan; SM, Santa María; AY, Ayutla.
3.3 Relationship between environmental variables, NDVI, WQI, land use, and microplastic pollution in the SGBR
According to the scree-plot (Figure 9) and considering the eigenvalue-one criterion, the four first components were taken into account (eigenvalues >1). A varimax rotation was applied to PCA in order to make interpretation easier by maximizing the variance of the squared factors loadings by column. The cumulative variance for these components is 80.6% of the total variance of the original dataset, with 52.91% in the first two components (Supplementary Table S2). The values of communalities showed that all variables have been described to an acceptable level (above 0.6), with exception of WQI and induced pasture. In this sense, PCA showed the influence of environmental variables, indices, and microplastic pollution on the study sites (Figure 10). The first component of the PCA (PC1) revealed a gradient of MP pollution load along the rivers; upstream areas (sites ES and EN) and areas with anthropic land uses (from JRT to PA) showed a higher MP load (extreme and mid right of PC1 in Figure 10, respectively) than downstream and better-conserved areas (extreme left of PC1 in Figure 10). The headwater areas of the Escanela River (ES) and the tributary of the Jalpan Reservoir (JRT) are characterized by secondary vegetation and slower currents. In contrast, sites SM, AY, and PI (extreme left of PC1 in Figure 10), characterized mainly by native vegetation, were negatively correlated with MP concentration and PLI in surface water and sediment (Supplementary Figure S3). These sites also include induced pastures, higher current velocity, greater depths, and some of the highest WQI and NDVI scores. Moreover, sites with larger mean grain sizes and faster currents were found to contain lower MP levels in water (negative correlation) (Supplementary Figure S3).
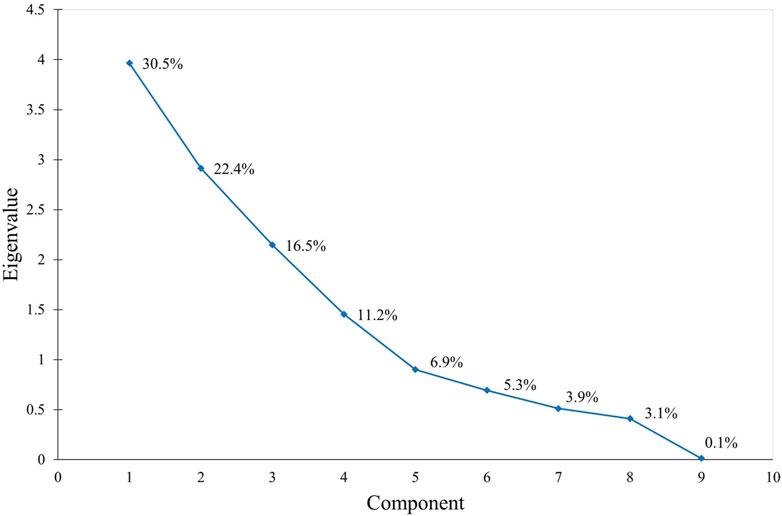
Figure 9. Scree-plot for the principal component analysis of the environmental factors and indices assessed in the rivers of the SGBR.
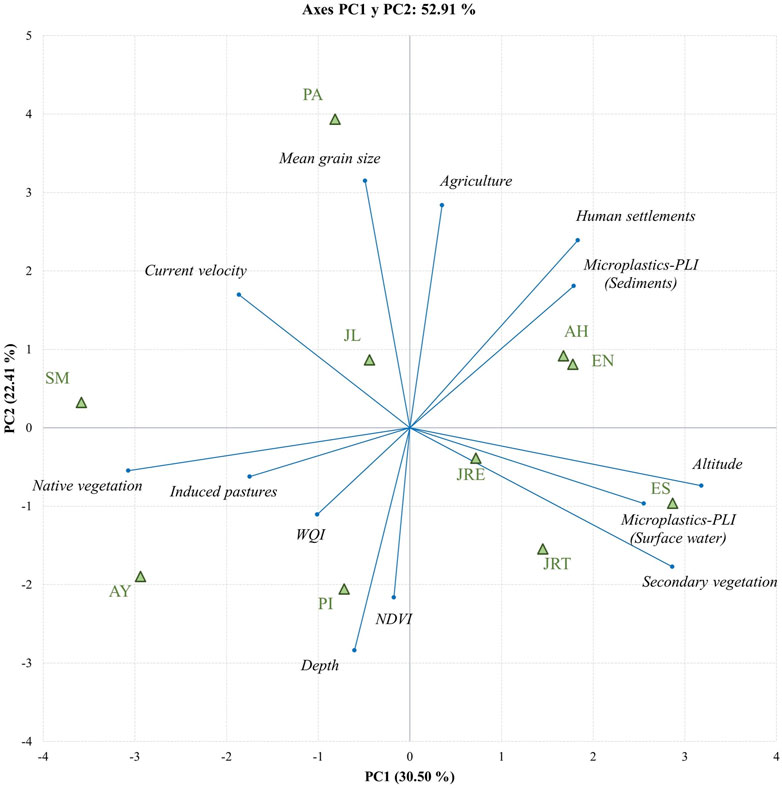
Figure 10. Biplot of the PCA of study sites and environmental variables. PI, Pizquintla; AH, Ahuacatlán; EN, Escanelilla; ES, Escanela; JRT, Jalpan Reservoir Tributary; JRE, Jalpan Reservoir Effluent; PA, Purísima; JL, Jalpan; SM, Santa María; AY, Ayutla.
4 Discussion
4.1 Environmental conditions
Sites AY and SM were located near and within core zones, which had the highest percent cover of native vegetation and the lowest impact from anthropic land uses, demonstrating the importance of designating areas for conservation and research. On the other hand, the vigor and presence of vegetation may have decreased in some places due to human settlements (impermeable soils and changes in land use) in the SGBR. Moreover, the negative correlation between NDVI and BOD5 may be associated with the low amount of organic matter entering these aquatic ecosystems. Therefore, those study sites covered with better-preserved vegetation could receive a lower contribution of organic matter. Particularly good physicochemical characteristics and water quality (WQI) have also been reported in these areas of the SGBR in previous studies (Rodríguez-Romero et al., 2021; Rico-Sánchez et al., 2022). The abundance of finer sediments at some study sites may be closely related to seasonal runoff that transports particulate matter, which may have increased in areas covered by secondary vegetation. According to Frelich et al. (2020), secondary vegetation is associated with areas that have been deforested or converted to other land uses (mainly agriculture) and that were reforested or afforested with natural or planted vegetation. This vegetation type necessarily implies an anthropic impact on the area.
4.2 Abundance of microplastics
In Mexico, MPs have only been found in marine and estuarine systems within PNAs, such as the Laguna de Términos Flora and Fauna Protection Area, located in the south of the Gulf of Mexico (Arreola-Alarcón et al., 2022), and the Espiritu Santo Island and Cabo Pulmo National Marine Parks in the Gulf of California (Celis-Hernandez et al., 2023). In contrast, studies of MPs in freshwater systems are scarce for rivers close to large populations and non-existent for rivers within PNAs. In the Atoyac River basin, MPs (333.33 items kg−1 and 266.67 items kg−1 were detected in sediments from sites along the Zahuapan and Atoyac rivers, respectively), at higher concentrations than those reported in this work (×= 55 items kg−1). Note that the Atoyac River is one of the most polluted rivers in the country (Shruti et al., 2019). Conversely, in other regions of the world, MPs have been found in freshwater ecosystems of PNAs. In the Yellow River deltas (a National Nature Reserve) in China, MP concentrations ranging from 136 items kg−1 to 2060 items kg−1 were recorded; the lowest levels were recorded in the most conserved areas (Duan et al., 2020).
Compared to other studies, the MP levels in surface water observed in the present study were higher than those reported for systems such as the Colorado River (United States), Limpopo River (South Africa), and Rhine River (Switzerland), which ranged from 0.022 items L−1 to 2.08 items L−1 (Mani et al., 2015; Baldwin et al., 2020; Dahms et al., 2020). Lake Victoria in Tanzania and Lake Ontario in Canada recorded MP concentrations (2.19 items L−1 and 1.5 items L−1, respectively) lower than those observed at sites JRT and JRE at the Jalpan Reservoir (Egessa et al., 2020; Grbić et al., 2020). On the other hand, MP concentrations in river sediments around the world vary from a few particles to thousands of them. Cases similar to our observations in SGBR are the Yangtze River (25 items kg−1 to 300 items kg−1) (Di and Wang, 2018), the Daliao River (100 items kg−1 to 467 items kg−1), and the Shuangtaizi River (133 items kg−1 to 300 items kg−1) in China (Xu et al., 2020), as well as the Danube River (x = 159.2 items kg−1) in Romania (Pojar et al., 2021). Human settlements are reported as one of the main sources associated with the presence of MPs in these works.
4.3 Physicochemical characteristics of microplastics
The high abundance of plastic fibers observed in the present work is consistent with reports for rivers and lakes in other regions (Barrows et al., 2018; Wang G. et al., 2021; Neelavannan et al., 2022; Li et al., 2024). This MP form is mainly associated with the degradation of plastic products, textiles, and domestic wastewater discharges (Napper and Thompson, 2016; Le et al., 2022). Approximately 5.6 Mt of synthetic microfibers have been discharged into the environment between 1950 and 2016, and 167.2 thousand tons per year are dumped in water bodies (Gavigan et al., 2020). The other forms found (i.e., fragments, films, and spheres) are usually associated with the fragmentation of MPs and personal care products (Badea et al., 2023). MPs of various colors are common because colorants (dyes and pigments) are additives widely used in the plastics and textiles industries (Rochman et al., 2019). Colorants can pose an additional risk to organisms; toxic effects of these pollutants have been reported in aquatic invertebrates and vertebrates (Hashemi and Kaykhaii, 2022). The high proportion of colorless MPs may result from bleaching due to exposure to UV rays in the environment (Chen et al., 2024). On the other hand, the weathering of MPs in the aquatic environment can produce surface irregularities (increasing the specific surface area of MPs) and thus influence the adsorption and aggregation of other elements present in the environmental matrix in which MPs are contained (Duan et al., 2021).
In turn, large amounts of plastic polymers have been reported in various rivers and lakes (Yang et al., 2021; Wang et al., 2022). The polymers identified in this study are widely used in the manufacturing of numerous plastic products. Polyethylene terephthalate is particularly used to make disposable water bottles, but it can also be found in containers for other liquids and food packaging. High-density polyethylene is used extensively to make containers, toys, and grocery bags. Polypropylene is stronger than polyethylene and constitutes sweet and snack wrappers, caps, tubes, microwave containers, automotive parts, etc. Polyester, polystyrene, and polyamide are commonly used in textile industries, but they can also be used in fishing nets and lines, building insulation, or electrical and electronic equipment (Wang C. et al., 2021; Plastics Europe, 2022). MPs with diverse polymeric compositions can affect aquatic biota and consequently human beings. Distinct adverse effects have been reported such as intestinal obstruction, tissue damage, inflammation, behavioural changes, cytotoxicity, neurotoxicity, oxidative stress, and even death (Anbumani and Kakkar, 2018; Bhuyan, 2022). Therefore, polymers can pose a risk to the environment and human health due to the release of residual oligomers and chemicals commonly used to synthesize these plastic products (e.g., antimony, phthalates, bisphenol A or metals) (Lithner et al., 2011; Proshad et al., 2017; Sridharan et al., 2022).
4.4 Microplastics as carriers of other pollutants
The presence of trace metals on the surface of MPs has been extensively reported and is associated with various physicochemical processes, including desorption from additives used in plastic manufacturing, adsorption through chemical binding with functional groups, or deposition on the MP surface (Cao et al., 2021; Turner and Filella, 2021; Patidar et al., 2023). In freshwater ecosystems, adsorption may be closely related to the presence of metals in water and sediments. Previous tests of water collected from rivers in the SGBR detected Al, As, Cd, Fe, Hg, Cr, Cu, and other minerals derived from geological strata and mining operations in the area (Rico-Sánchez et al., 2022).
4.5 Microplastic pollution load
The PLI has been extensively evaluated in freshwater bodies around the world. In the present study, the surface water of sites JRT and JRE, associated with the Jalpan Reservoir, showed values below the load reported for lakes Lonar and Xianghai in China (PLI = 3.21 and 2.16, respectively), also considered nature reserves (Yin et al., 2021). The water pollution load of the Jalpan (sites JL and PA), Ayutla (AY), and Santa María (SM) rivers was similar to the load observed in the Houjin River (PLI = 2.1) (Huang et al., 2023) or the Awano, Ayaragi, and Asa rivers (PLI between 1 and 3 approx.) in Japan (Kabir et al., 2021); in contrast, sites ES and EN of the Escanela River showed a higher MP load. In sediments, the load was higher in all sites studied than in the Yangtze River basin, China (river, PLI = 0.803; reservoirs, PLI = 0.605) (Yang et al., 2023), but lower than in the Subarnarekha River, India (PLI 7.54–8.17) which is considered highly polluted (Patidar et al., 2023). Although the rivers in the Reserve showed a moderate load of microplastic pollution, the observed levels were similar to those reported in some urban rivers in which pollution was associated with anthropic activities.
4.6 Potential sources and routes of microplastic pollution
One of the main challenges is identifying the potential sources of pollution and the routes that MPs follow to reach water bodies. This work included multiple environmental variables to understand the dynamics of MPs in the SGBR. The study sites ES, EN, AH, and JRT showed the highest MP loads in water and sediment. High MP levels in sediments were associated with human settlements and agriculture: therefore, as reported in other studies (Di and Wang, 2018; Xu et al., 2020; Raju et al., 2023), anthropogenic activities can be a considerable source of MPs in the Reserve. During fieldwork, we observed plastic waste inside water bodies and adjacent slopes, suggesting inadequate solid waste management and disposal in the PNA (Supplementary Figure S4). Furthermore, the significant correlation observed between human settlements and fecal coliform levels may indicate wastewater discharges, a source of MPs (Le et al., 2022) (Supplementary Figure S3). Two wastewater treatment facilities (WWTF #24004 and #24019), whose current operating status is unknown (INEGI, 2024), are located near sites AH and JL. Moreover, farming practices in the Reserve, particularly in areas such as PA, can be another source of MPs, as various materials such as seeding trays, silage bags, crop protective covers, and containers, among others, are potential sources of MPs (Wang C. et al., 2021).
This study highlighted that those sites located at higher altitudes and surrounded by secondary vegetation (ES and EN, Escanela River) showed the highest levels of MPs pollution in water. Despite NDVI indicated a good foliage condition at these sites, the vegetation has suffered human or natural disturbances, and as a consequence, some MP transport routes could be encouraged. On the one hand, wind may contribute significantly to the transport and deposition of MPs in soil, water, and vegetation. Wind has been one of the main routes of transport of MPs, particularly light ones such as fibers, to water bodies, even in remote areas (Evangeliou et al., 2020; Su et al., 2022). Thus, the characteristics and health status of the surrounding vegetation in the study sites may be key factors for the input of MPs. On the other hand, seasonal rains in the region are an additional factor that may contribute MPs to these aquatic ecosystems. Wet deposition, washing off leaf surfaces, and runoff also transport MPs from the atmosphere and soil into rivers and lakes (Wei et al., 2023). Therefore, high MP levels in surface water could be associated with these meteorological factors.
Additionally, the physicochemical characteristics of the water may influence the abundances of MPs; these showed positive and negative correlations with water hardness and temperature, respectively (Supplementary Figure S3). Harder and colder waters may have favored the suspension of MPs, especially lighter ones (Imhof et al., 2012). The relationship between sediment grain size and MPs in surface water suggests that sites with coarser sediment may capture more MPs. A similar behavior, in which sediments with coarse grains retained more MPs, has been observed experimentally elsewhere (Munz et al., 2024). However, this study presents a first approximation to the dynamics of MPs in rivers of the SGBR, so further studies should be conducted.
In contrast to sites in upstream rivers, the sites on the Santa María and Ayutla rivers, located within and near the core zones, showed that areas with well-preserved vegetation, better water quality, and low impact from land-use changes had lower MP pollution levels. A high score in water quality indicates low pollution, particularly associated with anthropogenic activities or nutrient enrichment. As a result, MPs contributions related to domestic discharges or soil washing can be minimized. On the other hand, dense (high NDVI scores) and native vegetation can function as a natural barrier against airborne MPs in these areas. The capability of vegetative spaces to capture hazardous air particles has been proved through a phenomenon known as the forest filter effect (Michael and Michael, 1998). Recent researchers have demonstrated that plant leaves capture MPs, indicating that terrestrial vegetation could be a crucial temporary sink and source of MPs (Liu et al., 2020; Jiao et al., 2024). Nevertheless, rain may reduce the effectiveness of this barrier and, in contrast, contribute to the MP pollution load to water bodies. Therefore, the MPs detected in SM and AY may have washed off from leaves and transported by upstream currents. Current velocity and channel depth probably contribute to minimizing MP deposition in sediments. Consequently, MPs transported by river water can be deposited downstream and even reach coastal areas and oceans, impacting these areas (Lebreton et al., 2017).
5 Conclusion
The results of the present study are the first in Mexico to report the presence of MPs in water and sediments of freshwater ecosystems within a PNA classified as Biosphere Reserve. This study established the relationships of NDVI and WQI with the microplastic pollution load index in water and sediments, as well as with current velocity. In turn, the multiple morphological and physicochemical characteristics of these plastic particles were documented, and metallic elements were found on their surface, confirming their role as carriers of trace metals. Furthermore, the PLI allowed the estimation of the degree of MP pollution and the potential risks along the rivers of the SGBR. By incorporating multiple environmental variables, geospatial analysis, and statistical testing, the probable sources of microplastic pollution were identified, as well as the probable transport routes and flow of MPs in the aquatic ecosystems studied. Anthropogenic activities, meteorological factors, vegetation status, and physicochemical characteristics of water were some of the factors associated with the presence and dynamism of MPs in the river systems of the Reserve. Our findings show that even protected natural areas can be polluted with MPs. However, those areas with low anthropogenic impact and better conserved, such as sites AY and SM located in core areas, may be less affected by this type of pollutants. Our results showed the close relationship between MP abundance and environmental conditions and its importance for detecting potential pollution sources. It is necessary to continue with these studies considering other aspects, such as seasonality or the presence of other pollutants.
Data availability statement
The raw data supporting the conclusions of this article will be made available by the authors, without undue reservation.
Author contributions
RG-S: Conceptualization, Formal Analysis, Investigation, Methodology, Writing–original draft, Writing–review and editing, Data curation. JS-D: Conceptualization, Methodology, Writing–review and editing, Resources, Supervision. EL-L: Conceptualization, Resources, Supervision, Writing–original draft, Writing–review and editing, Formal Analysis, Funding acquisition, Investigation, Methodology, Project administration.
Funding
The authors declare that financial support was received for the research, authorship, and/or publication of this article. This research was funded by Instituto Politécnico Nacional (IPN) through the research projects SIP- 20221711, SIP-20221886 and SIP 20240743. IPN-students-innovation project.
Acknowledgments
The authors thank the Instituto Politécnico Nacional for the support and María Elena Sánchez-Salazar for the language editing. We also recognize the National Council of Humanities, Science, and Technology (CONAHCYT) for postgraduate scholarships.
Conflict of interest
The authors declare that the research was conducted in the absence of any commercial or financial relationships that could be construed as a potential conflict of interest.
The authors declared that they were an editorial board member of Frontiers, at the time of submission. This had no impact on the peer review process and the final decision.
Publisher’s note
All claims expressed in this article are solely those of the authors and do not necessarily represent those of their affiliated organizations, or those of the publisher, the editors and the reviewers. Any product that may be evaluated in this article, or claim that may be made by its manufacturer, is not guaranteed or endorsed by the publisher.
Supplementary material
The Supplementary Material for this article can be found online at: https://www.frontiersin.org/articles/10.3389/fenvs.2024.1441340/full#supplementary-material
Abbreviations
AH, Ahuacatlán; AY, Ayutla; EN, Escanelilla; ES, Escanela; JRT, Jalpan Reservoir Tributary; JRE, Jalpan Reservoir Effluent; JL, Jalpan; MPs, Microplastics; NDVI, Normalized Difference Vegetation Index; PNA, Protected Natural Area; PLI, Pollution Load Index; PI, Pizquintla; PA, Purísima; SGBR, Sierra Gorda Biosphere Reserve; SM, Santa María; WQI, Water Quality Index.
References
Allen, S., Allen, D., Karbalaei, S., Maselli, V., and Walker, T. R. (2022). Micro(nano)plastics sources, fate, and effects: what we know after ten years of research. J. Hazard. Mater. Adv. 6, 100057. doi:10.1016/j.hazadv.2022.100057
Anbumani, S., and Kakkar, P. (2018). Ecotoxicological effects of microplastics on biota: a review. Environ. Sci. Pollut. Res. 25, 14373–14396. doi:10.1007/s11356-018-1999-x
Arreola-Alarcón, I. M., Reyes-Bonilla, H., Sakthi, J. S., Rodríguez-González, F., and Jonathan, M. P. (2022). Seasonal tendencies of microplastics around coral reefs in selected Marine Protected National Parks of Gulf of California, Mexico. Mar. Pollut. Bull. 175, 113333. doi:10.1016/j.marpolbul.2022.113333
ASTM (2007). Standard test method for particle-size analysis of soils. Philadelphia: American Society for Testing and Materials.
ATCM (2022). Antarctic treaty consultative meeting. Management plan for antarctic specially protected area No. 126 Byers Peninsula, Livingston Island, South Shetland Islands. Available at: https://www.ats.aq/devAS/Meetings/Measure/760 (Accessed November 27, 2023).1–30.
Atugoda, T., Vithanage, M., Wijesekara, H., Bolan, N., Sarmah, A. K., Bank, M. S., et al. (2021). Interactions between microplastics, pharmaceuticals, and personal care products: implications for vector transport. Environ. Int. 149, 106367. doi:10.1016/j.envint.2020.106367
Badea, M. A., Balas, M., and Dinischiotu, A. (2023). Microplastics in freshwaters: implications for aquatic autotrophic organisms and fauna health. Microplastics 2, 39–59. doi:10.3390/microplastics2010003
Baird, R. B., Eaton, A. D., and Rice, E. W. (2017). Standard methods for the examination of water and wastewater. 23rd Edn. Washington, DC, USA: American Public Health Association.
Baldwin, A. K., Spanjer, A. R., Rosen, M. R., and Thom, T. (2020). Microplastics in lake mead national recreation area, USA: occurrence and biological uptake. PLoS One 15, e0228896. doi:10.1371/journal.pone.0228896
Barrows, A. P. W., Christiansen, K. S., Bode, E. T., and Hoellein, T. J. (2018). A watershed-scale, citizen science approach to quantifying microplastic concentration in a mixed land-use river. Water Res. 147, 382–392. doi:10.1016/j.watres.2018.10.013
Bhuyan, M. S. (2022). Effects of microplastics on fish and in human health. Front. Environ. Sci. 10. doi:10.3389/fenvs.2022.827289
Brandsch, J., and Piringer, O. (2007). Characteristics of plastic materials, in Plastic packaging materials for food (Weinheim, Germany: Wiley-VCH Verlag GmbH), 9–45. doi:10.1002/9783527613281.ch02
Burns, E. E., and Boxall, A. B. A. (2018). Microplastics in the aquatic environment: evidence for or against adverse impacts and major knowledge gaps. Environ. Toxicol. Chem. 37, 2776–2796. doi:10.1002/etc.4268
Cao, F., Lu, Y., Dong, S., and Li, X. (2020). Evaluation of natural support capacity of water resources using principal component analysis method: a case study of Fuyang district, China. Appl. Water. Sci. 10, 192. doi:10.1007/s13201-020-1174-7
Cao, Y., Zhao, M., Ma, X., Song, Y., Zuo, S., Li, H., et al. (2021). A critical review on the interactions of microplastics with heavy metals: mechanism and their combined effect on organisms and humans. Sci. Total. Environ. 788, 147620. doi:10.1016/j.scitotenv.2021.147620
Carabias, J., Provencio, E., de la Maza Elvira, J., and Ruíz Corso, M. I. (1999). Programa de Manejo Reserva de la Biosfera Sierra Gorda México. Mexico D.F.: Instituto Nacional de Ecología.
Celis-Hernandez, O., Ávila, E., Rendón-von Osten, J., Briceño-Vera, E. A., Borges-Ramírez, M. M., Gómez-Ponce, A. M., et al. (2023). Environmental risk of microplastics in a Mexican coastal lagoon ecosystem: anthropogenic inputs and its possible human food risk. Sci. Total. Environ. 879, 163095. doi:10.1016/j.scitotenv.2023.163095
Chen, B., He, B., Wu, H., and Liu, A. (2024). Microplastic degradations in simulated UV light, natural light and natural water body: a comparison investigation. Emerg. Contam. 10, 100306. doi:10.1016/j.emcon.2024.100306
Citterich, F., Lo Giudice, A., and Azzaro, M. (2023). A plastic world: a review of microplastic pollution in the freshwaters of the Earth’s poles. Sci. Total. Environ. 869, 161847. doi:10.1016/j.scitotenv.2023.161847
Cormier, B., Gambardella, C., Tato, T., Perdriat, Q., Costa, E., Veclin, C., et al. (2021). Chemicals sorbed to environmental microplastics are toxic to early life stages of aquatic organisms. Ecotoxicol. Environ. Saf. 208, 111665. doi:10.1016/j.ecoenv.2020.111665
Cowger, W., Steinmetz, Z., Gray, A., Munno, K., Lynch, J., Hapich, H., et al. (2021). Microplastic spectral classification needs an open source community: open specy to the rescue. Anal. Chem. 93, 7543–7548. doi:10.1021/acs.analchem.1c00123
Dahms, H. T. J., van Rensburg, G. J., and Greenfield, R. (2020). The microplastic profile of an urban African stream. Sci. Total. Environ. 731, 138893. doi:10.1016/j.scitotenv.2020.138893
D’Avignon, G., Gregory-Eaves, I., and Ricciardi, A. (2022). Microplastics in lakes and rivers: an issue of emerging significance to limnology. Environ. Rev. 30, 228–244. doi:10.1139/er-2021-0048
Debraj, D., and Lavanya, M. (2023). Microplastics everywhere: a review on existing methods of extraction. Sci. Total. Environ. 893, 164878. doi:10.1016/j.scitotenv.2023.164878
Di, M., and Wang, J. (2018). Microplastics in surface waters and sediments of the three gorges reservoir, China. Sci. Total. Environ. 616 (617), 1620–1627. doi:10.1016/j.scitotenv.2017.10.150
Dinius, S. H. (1987). Design of an index of water quality. Water Resour. Bull. 23, 833–843. doi:10.1111/j.1752-1688.1987.tb02959.x
Duan, J., Bolan, N., Li, Y., Ding, S., Atugoda, T., Vithanage, M., et al. (2021). Weathering of microplastics and interaction with other coexisting constituents in terrestrial and aquatic environments. Water. Res. 196, 117011. doi:10.1016/j.watres.2021.117011
Duan, Z., Zhao, S., Zhao, L., Duan, X., Xie, S., Zhang, H., et al. (2020). Microplastics in Yellow River Delta wetland: occurrence, characteristics, human influences, and marker. Environ. Pollut. 258, 113232. doi:10.1016/j.envpol.2019.113232
Eerkes-Medrano, D., Thompson, R. C., and Aldridge, D. C. (2015). Microplastics in freshwater systems: a review of the emerging threats, identification of knowledge gaps and prioritisation of research needs. Water. Res. 75, 63–82. doi:10.1016/j.watres.2015.02.012
Egessa, R., Nankabirwa, A., Ocaya, H., and Pabire, W. G. (2020). Microplastic pollution in surface water of Lake Victoria. Sci. Total. Environ. 741, 140201. doi:10.1016/j.scitotenv.2020.140201
Ejecutivo, P. (1997). Decreto de la Reserva de la Biosfera Sierra Gorda. Available at: https://dof.gob.mx/nota_detalle.php?codigo=4879875&fecha=19/05/1997#gsc.tab=0 (Accessed February 22, 2024).
European Commission (2013). Joint Research Centre. Institute for environment and sustainability, MSFD technical subgroup on marine litter. Guidance on monitoring of marine litter in European seas. Luxembourg: Publications Office.
Evangeliou, N., Grythe, H., Klimont, Z., Heyes, C., Eckhardt, S., Lopez-Aparicio, S., et al. (2020). Atmospheric transport is a major pathway of microplastics to remote regions. Nat. Commun. 11, 3381. doi:10.1038/s41467-020-17201-9
Frelich, L. E., Jogiste, K., Stanturf, J., Jansons, A., and Vodde, F. (2020). Are secondary forests ready for climate change? It depends on magnitude of climate change, landscape diversity and ecosystem legacies. Forests 11, 965. doi:10.3390/f11090965
Gavigan, J., Kefela, T., Macadam-Somer, I., Suh, S., and Geyer, R. (2020). Synthetic microfiber emissions to land rival those to waterbodies and are growing. PLoS One 15, e0237839. doi:10.1371/journal.pone.0237839
Geyer, R., Jambeck, J. R., and Law, K. L. (2017). Production, use, and fate of all plastics ever made. 3. Available at: https://www.science.org.
González-Pleiter, M., Edo, C., Velázquez, D., Casero-Chamorro, M. C., Leganés, F., Quesada, A., et al. (2020). First detection of microplastics in the freshwater of an antarctic specially protected area. Mar. Pollut. Bull. 161, 111811. doi:10.1016/j.marpolbul.2020.111811
Grbić, J., Helm, P., Athey, S., and Rochman, C. M. (2020). Microplastics entering northwestern Lake Ontario are diverse and linked to urban sources. Water Res. 174, 115623. doi:10.1016/j.watres.2020.115623
Hahladakis, J. N., Velis, C. A., Weber, R., Iacovidou, E., and Purnell, P. (2018). An overview of chemical additives present in plastics: migration, release, fate and environmental impact during their use, disposal and recycling. J. Hazard. Mater. 344, 179–199. doi:10.1016/j.jhazmat.2017.10.014
Hartoyo, A. P. P., Pamoengkas, P., Mudzaky, R. H., Khairunnisa, S., Ramadhi, A., Munawir, A., et al. (2022). Estimation of vegetation cover changes using normalized difference vegetation index (NDVI) in Mount Halimun Salak National Park, Indonesia. IOP Conf. Ser. Earth Environ. Sci. 1109, 012068. doi:10.1088/1755-1315/1109/1/012068
Hashemi, S. H., and Kaykhaii, M. (2022). “Azo dyes: sources, occurrence, toxicity, sampling, analysis, and their removal methods,” in Emerging freshwater pollutants. Editors Dalu, T., and Tavengwa, N. Elsevier. 267–287. doi:10.1016/b978-0-12-822850-0.00013-2
Hoang, T., and Mitten, S. (2022). Microplastic accumulation in the gastrointestinal tracts of nestling and adult migratory birds. Sci. Total. Environ. 838, 155827. doi:10.1016/j.scitotenv.2022.155827
Huang, C. W., Li, Y. L., Lin, C., Bui, X. T., Vo, T. D. H., and Ngo, H. H. (2023). Seasonal influence on pollution index and risk of multiple compositions of microplastics in an urban river. Sci. Total. Environ. 859, 160021. doi:10.1016/j.scitotenv.2022.160021
Ibrahim, A., Ismail, A., Juahir, H., Iliyasu, A. B., Wailare, B. T., Mukhtar, M., et al. (2023). Water quality modelling using principal component analysis and artificial neural network. Mar. Pollut. Bull. 187, 114493. doi:10.1016/j.marpolbul.2022.114493
Imhof, H. K., Schmid, J., Niessner, R., Ivleva, N. P., and Laforsch, C. (2012). A novel, highly efficient method for the separation and quantification of plastic particles in sediments of aquatic environments. Limnol. Oceanogr. Methods 10, 524–537. doi:10.4319/lom.2012.10.524
INEGI (2021). Instituto Nacional de Estadística, Geografía e Informática. México en Cifras. Available at: https://www.inegi.org.mx/app/areasgeograficas/?ag=22#collapse-Resumen (Accessed February 22, 2024).
INEGI (2024) Instituto Nacional de Estadística, Geografía e Informática. Simulador de Flujos de Agua de Cuencas Hidrográficas. Available at: https://antares.inegi.org.mx/analisis/red_hidro/siatl/(Accessed February 21, 2024).
Jambeck, J. R., Geyer, R., Wilcox, C., Siegler, T. R., Perryman, M., Andrady, A., et al. (2015). Plastic waste inputs from land into the ocean. Science 347, 768–771. doi:10.1126/science.1260352
Jiao, M., Wang, Y., Yang, F., Zhao, Z., Wei, Y., Li, R., et al. (2024). Dynamic fluctuations in plant leaf interception of airborne microplastics. Sci. Total. Environ. 906, 167877. doi:10.1016/j.scitotenv.2023.167877
Kabir, A. H. M. E., Sekine, M., Imai, T., Yamamoto, K., Kanno, A., and Higuchi, T. (2021). Assessing small-scale freshwater microplastics pollution, land-use, source-to-sink conduits, and pollution risks: perspectives from Japanese rivers polluted with microplastics. Sci. Total. Environ. 768, 144655. doi:10.1016/j.scitotenv.2020.144655
Kaur, M., Ghosh, D., Guleria, S., Arya, S. K., Puri, S., and Khatri, M. (2023). Microplastics/nanoplastics released from facemasks as contaminants of emerging concern. Mar. Pollut. Bull. 191, 114954. doi:10.1016/j.marpolbul.2023.114954
Kinigopoulou, V., Pashalidis, I., Kalderis, D., and Anastopoulos, I. (2022). Microplastics as carriers of inorganic and organic contaminants in the environment: a review of recent progress. J. Mol. Liq. 350, 118580. doi:10.1016/j.molliq.2022.118580
Kowalkowski, T., Zbytniewski, R., Szpejna, J., and Buszewski, B. (2006). Application of chemometrics in river water classification. Water Res. 40, 744–752. doi:10.1016/j.watres.2005.11.042
Kutralam-Muniasamy, G., Pérez-Guevara, F., Elizalde-Martínez, I., and Shruti, V. C. (2020). Review of current trends, advances and analytical challenges for microplastics contamination in Latin America. Environ. Pollut. 267, 115463. doi:10.1016/j.envpol.2020.115463
Kutralam-Muniasamy, G., Pérez-Guevara, F., Elizalde-Martínez, I., and Shruti, V. C. (2021). How well-protected are protected areas from anthropogenic microplastic contamination? Review of analytical methods, current trends, and prospects. Trends Environ. Anal. Chem. 32, e00147. doi:10.1016/j.teac.2021.e00147
Le, L. T., Nguyen, K. Q. N., Nguyen, P. T., Duong, H. C., Bui, X. T., Hoang, N. B., et al. (2022). Microfibers in laundry wastewater: problem and solution. Sci. Total. Environ. 852, 158412. doi:10.1016/j.scitotenv.2022.158412
Lebreton, L. C. M., Van Der Zwet, J., Damsteeg, J. W., Slat, B., Andrady, A., and Reisser, J. (2017). River plastic emissions to the world’s oceans. Nat. Commun. 8, 15611. doi:10.1038/ncomms15611
Li, J., Liu, H., and Paul Chen, J. (2018). Microplastics in freshwater systems: a review on occurrence, environmental effects, and methods for microplastics detection. Water Res. 137, 362–374. doi:10.1016/j.watres.2017.12.056
Li, Y., Deng, Y., Hu, C., Li, D., Zhang, J., and Zhou, N. (2024). Microplastic pollution in urban rivers within China’s Danxia landforms: spatial distribution characteristics, migration, and risk assessment. Sci. Total. Environ. 910, 168610. doi:10.1016/j.scitotenv.2023.168610
Lithner, D., Larsson, A., and Dave, G. (2011). Environmental and health hazard ranking and assessment of plastic polymers based on chemical composition. Sci. Total. Environ. 409, 3309–3324. doi:10.1016/j.scitotenv.2011.04.038
Liu, K., Wang, X., Song, Z., Wei, N., and Li, D. (2020). Terrestrial plants as a potential temporary sink of atmospheric microplastics during transport. Sci. Total. Environ. 742, 140523. doi:10.1016/j.scitotenv.2020.140523
Malafaia, G., and Barceló, D. (2023). Microplastics in human samples: recent advances, hot-spots, and analytical challenges. Trends Anal. Chem. 161, 117016. doi:10.1016/j.trac.2023.117016
Mani, T., Hauk, A., Walter, U., and Burkhardt-Holm, P. (2015). Microplastics profile along the Rhine River. Sci. Rep. 5, 17988. doi:10.1038/srep17988
Michael, M. L., and Michael, H. (1998). Forests as filters of airborne organic pollutants: a model. Environ. Sci. Technol. 32, 413–420. doi:10.1021/es970592u
Munz, M., Loui, C., Postler, D., Pittroff, M., and Oswald, S. E. (2024). Transport and retention of micro-polystyrene in coarse riverbed sediments: effects of flow velocity, particle and sediment sizes. Microplastics Nanoplastics 4, 2. doi:10.1186/s43591-023-00077-z
Nantege, D., Odong, R., Auta, H. S., Keke, U. N., Ndatimana, G., Assie, A. F., et al. (2023). Microplastic pollution in riverine ecosystems: threats posed on macroinvertebrates. Environ. Sci. Pollut. Res. 30 (31), 76308–76350. doi:10.1007/s11356-023-27839-9
Napper, I. E., and Thompson, R. C. (2016). Release of synthetic microplastic plastic fibres from domestic washing machines: effects of fabric type and washing conditions. Mar. Pollut. Bull. 112, 39–45. doi:10.1016/j.marpolbul.2016.09.025
Neelavannan, K., Sen, I. S., Lone, A. M., and Gopinath, K. (2022). Microplastics in the high-altitude Himalayas: assessment of microplastic contamination in freshwater lake sediments, Northwest Himalaya (India). Chemosphere 290, 133354. doi:10.1016/j.chemosphere.2021.133354
Orona-Návar, C., García-Morales, R., Loge, F. J., Mahlknecht, J., Aguilar-Hernández, I., and Ornelas-Soto, N. (2022). Microplastics in Latin America and the Caribbean: a review on current status and perspectives. J. Environ. Manage. 309, 114698. doi:10.1016/j.jenvman.2022.114698
Patidar, K., Ambade, B., Mohammad, F., and Soleiman, A. A. (2023). Microplastics as heavy metal vectors in the freshwater environment: distribution, variations, sources and health risk. Phys. Chem. Earth 131, 103448. doi:10.1016/j.pce.2023.103448
Plastics Europe (2022). Plastics-the facts 2022. Available at: https://plasticseurope.org/knowledge-hub/plastics-the-facts-2022 (Accessed November 23, 2023).
Pojar, I., Stănică, A., Stock, F., Kochleus, C., Schultz, M., and Bradley, C. (2021). Sedimentary microplastic concentrations from the Romanian Danube River to the black sea. Sci. Rep. 11, 2000. doi:10.1038/s41598-021-81724-4
Proshad, R., Kormoker, T., Islam, Md. S., Haque, M. A., Rahman, Md. M., and Mithu, Md. M. R. (2017). Toxic effects of plastic on human health and environment: a consequences of health risk assessment in Bangladesh. Int. J. Health 6, 1–5. doi:10.14419/ijh.v6i1.8655
Raju, M., Gandhimathi, R., and Nidheesh, P. V. (2023). The cause, fate and effect of microplastics in freshwater ecosystem: ways to overcome the challenge. J. Water Process. Eng. 55, 104199. doi:10.1016/j.jwpe.2023.104199
Reddy, A. S., and Nair, A. T. (2022). The fate of microplastics in wastewater treatment plants: an overview of source and remediation technologies. Environ. Technol. Innov. 28, 102815. doi:10.1016/j.eti.2022.102815
Rico-Sánchez, A. E., Rodríguez-Romero, A. J., Sedeño-Díaz, J. E., López-López, E., and Sundermann, A. (2022). Aquatic macroinvertebrate assemblages in rivers influenced by mining activities. Sci. Rep. 12, 3209. doi:10.1038/s41598-022-06869-2
Rochman, C. M., Brookson, C., Bikker, J., Djuric, N., Earn, A., Bucci, K., et al. (2019). Rethinking microplastics as a diverse contaminant suite. Environ. Toxico.l Chem. 38, 703–711. doi:10.1002/etc.4371
Rodríguez-Romero, A. J., Rico-Sánchez, A. E., Sedeño-Díaz, J. E., and López-López, E. (2021). Characterization of the multidimensional functional space of the aquatic macroinvertebrate assemblages in a biosphere reserve (Central méxico). Divers. (Basel) 13, 546. doi:10.3390/d13110546
Romía, M. B., and Bernàrdez, M. A. (2009). “Chapter 3 - multivariate calibration for quantitative analysis,” in Infrared spectroscopy for food quality analysis and control. Editor Sun, D.-W. (San Diego: Academic Press), 51–82. doi:10.1016/B978-0-12-374136-3.00003-1
Schell, T., Rico, A., and Vighi, M. (2020). Occurrence, fate and fluxes of plastics and microplastics in terrestrial and freshwater ecosystems. Rev. Environ. Contam. Toxicol. 250, 1–43. doi:10.1007/398_2019_40
SGM (2024). GeoInfoMex. Available at: https://www.gob.mx/sgm/articulos/conoce-el-sistema-de-consulta-de-informacion-geocientifica-geoinfomex?idiom=es (Accessed February 25, 2024).
Shahnawaz, M., Sangale, M., and Ade, A. B. (2019). Bioremediation Technology for plastic waste. Singapore: Springer Nature. doi:10.1007/978-981-13-7492-0
Shruti, V. C., Jonathan, M. P., Rodriguez-Espinosa, P. F., and Rodríguez-González, F. (2019). Microplastics in freshwater sediments of Atoyac River basin, puebla city, Mexico. Sci. Total. Environ. 654, 154–163. doi:10.1016/j.scitotenv.2018.11.054
Sridharan, S., Kumar, M., Saha, M., Kirkham, M. B., Singh, L., and Bolan, N. S. (2022). The polymers and their additives in particulate plastics: what makes them hazardous to the fauna? Sci. Total. Environ. 824, 153828. doi:10.1016/j.scitotenv.2022.153828
Su, L., Xiong, X., Zhang, Y., Wu, C., Xu, X., Sun, C., et al. (2022). Global transportation of plastics and microplastics: a critical review of pathways and influences. Sci. Total. Environ. 831, 154884. doi:10.1016/j.scitotenv.2022.154884
Sulaiman, B., Woodward, J., and Shiels, H. A. (2023). Riverine microplastics and their interaction with freshwater fish. Water Biol. Secur. 2, 100192. doi:10.1016/j.watbs.2023.100192
Tarpley, J. D., Schneider, S. R., and Money, R. L. (1984). Global vegetation indices from the NOAA-7 meteorological satellite. J. Clim. Appl. Meteorology 23, 491–494. doi:10.1175/1520-0450(1984)023<0491:gviftn>2.0.co;2
Tofa, T. S., Kunjali, K. L., Paul, S., and Dutta, J. (2019). Visible light photocatalytic degradation of microplastic residues with zinc oxide nanorods. Environ. Chem. Let.t 17, 1341–1346. doi:10.1007/s10311-019-00859-z
Tomlinson, D. L., Wilson, J. G., Harris, C. R., and Jeffrey, D. W. (1980). Problems in the assessment of heavy-metal levels in estuaries and the formation of a pollution index. Helgoländer Meeresunters. 33, 566–575. doi:10.1007/bf02414780
Turner, A., and Filella, M. (2021). Hazardous metal additives in plastics and their environmental impacts. Environ. Int. 156, 106622. doi:10.1016/j.envint.2021.106622
Wang, C., Zhao, J., and Xing, B. (2021a). Environmental source, fate, and toxicity of microplastics. J. Hazard. Mater. 407, 124357. doi:10.1016/j.jhazmat.2020.124357
Wang, G., Lu, J., Li, W., Ning, J., Zhou, L., Tong, Y., et al. (2021b). Seasonal variation and risk assessment of microplastics in surface water of the Manas River Basin, China. Ecotoxicol. Environ. Saf. 208, 111477. doi:10.1016/j.ecoenv.2020.111477
Wang, Y., Zhou, B., Chen, H., Yuan, R., and Wang, F. (2022). Distribution, biological effects and biofilms of microplastics in freshwater systems - a review. Chemosphere 299, 134370. doi:10.1016/j.chemosphere.2022.134370
Wei, L., Yue, Q., Chen, G., and Wang, J. (2023). Microplastics in rainwater/stormwater environments: influencing factors, sources, transport, fate, and removal techniques. Trac. Trends Anal. Chem. 165, 117147. doi:10.1016/j.trac.2023.117147
Xu, Q., Xing, R., Sun, M., Gao, Y., and An, L. (2020). Microplastics in sediments from an interconnected river-estuary region. Sci. Total. Environ. 729, 139025. doi:10.1016/j.scitotenv.2020.139025
Yang, H., Sun, F., Liao, H., Guo, Y., Pan, T., and Wu, F. (2023). The pollution of microplastics in sediments of the Yangtze River Basin: occurrence, distribution characteristics, and basin-scale multilevel ecological risk assessment. Water Res. 243, 120322. doi:10.1016/j.watres.2023.120322
Yang, L., Zhang, Y., Kang, S., Wang, Z., and Wu, C. (2021). Microplastics in freshwater sediment: a review on methods, occurrence, and sources. Sci. Total. Environ. 754, 141948. doi:10.1016/j.scitotenv.2020.141948
Yin, K., Wang, D., Zhao, H., Wang, Y., Guo, M., Liu, Y., et al. (2021). Microplastics pollution and risk assessment in water bodies of two nature reserves in Jilin Province: correlation analysis with the degree of human activity. Sci. Total. Environ. 799, 149390. doi:10.1016/j.scitotenv.2021.149390
Keywords: microplastics, emerging pollutants, trace elements, land use, SEM-EDX analyses, FTIR spectroscopy
Citation: Granados-Sánchez RR, Sedeño-Díaz JE and López-López E (2024) Microplastic pollution and associated trace metals in freshwater ecosystems within protected natural areas: the case of a biosphere reserve in Mexico. Front. Environ. Sci. 12:1441340. doi: 10.3389/fenvs.2024.1441340
Received: 30 May 2024; Accepted: 28 August 2024;
Published: 11 September 2024.
Edited by:
Oladele Ogunseitan, University of California, Irvine, United StatesReviewed by:
Soha Shabaka, National Institute of Oceanography and Fisheries (NIOF), EgyptEvdokia Syranidou, Technical University of Crete, Greece
Copyright © 2024 Granados-Sánchez, Sedeño-Díaz and López-López. This is an open-access article distributed under the terms of the Creative Commons Attribution License (CC BY). The use, distribution or reproduction in other forums is permitted, provided the original author(s) and the copyright owner(s) are credited and that the original publication in this journal is cited, in accordance with accepted academic practice. No use, distribution or reproduction is permitted which does not comply with these terms.
*Correspondence: Eugenia López-López, ZXVsb3BlekBpcG4ubXg=