- 1China Energy Engineering Group Guangxi Electric Power Design Institute Co.,LTD., Nanning, China
- 2School of Environment, Northeast Normal University, Changchun, China
- 3Technical Innovation Center of Mine Geological Environmental Restoration Engineering in Southern Karst Area, Ministry of Natural Resources, Nanning, China
- 4China Energy Engineering Group Guangdong Electric Power Desing Institute Co.,LTD., Guangzhou, China
- 5Key Laboratory of Vegetation Ecology, Ministry of Education, Northeast Normal University, Changchun, China
- 6Institute of Grassland Science, Northeast Normal University, Changchun, China
Mercury pollution in emerging industrial zones and surrounding areas, especially in industrial concentration areas, has attracted much attention. So as to clarify the characteristics of Hg in the environment in China’s small and medium-sized industrial emerging and surrounding areas, central urban area of Gongzhuling, a traditional agricultural town with a high degree of industrialization in northeast Changchun industrial base, is taken as the scope. Geological accumulation trin (Igeo) was used to study the degree of soil mercury contamination in Gongzhuling area, latent Er (ecological risk) trin was used to appraise the Er of soil Hg in the study area, and non-carcinogenic risk assessment of mercury in soil using human exposure risk assessment model. The results showed that 34% of soil samples had higher Hg content than the background value of the province’s soil (0.04 mg kg−1). The Igeo results showed that Hg pollution rate of soil in study area was 9% (index >0). In conclusion, the level of soil Hg pollution in Gongzhuling area was low, and the pollution area is mainly concentrated in the northwestern part of the study range. The highest and lowest Er values of soil Hg from the study sample were 2.23 and 214.83, and 24% of the samples had Er > 40, that means they pose a moderate or higher potential ecological risk, and most of these points are located in the northeast of the study range. The main route of human exposure to Hg is oral ingestion. The HQ (non-carcinogenic risk index) and HI (total non-carcinogenic risk value) of soil mercury were both much less than 1, it indicates that the present level of soil Hg in the study range does not pose a threat to local adult health for the time being. This study provides reference for other urban pollution risk assessment, and further defines the direction of future work.
1 Introduction
Mercury (Hg) pollution is global (Driscoll et at., 2013), with the characteristics of easy migration, endorance, concealment, high bioaccumulation, and high biotoxicity (Wang et at., 2022). Uneven distribution of mercury in nature, migration, and transformation are closely associated with the human activities, vegetation, geological conditions, and so on (Lin and Pehkonen, 1999; Feng and Chen, 2013; Karthikeyan et at., 2021; Wang et at., 2022). A newly tapped urban area with mechanic plants zone, conducting complicated procedures and using multiple power re-sources, might have high background mercury levels thus affecting human health (Henríquez-Hernández et at., 2017; Li et at., 2020). And mercury concentrations in regional soil rise with the rapid industrial transformation, along with a large amount of coal to supply heat and energy (Balasundaram and Sharma, 2019). In China, the average mercury content in coal is 0.20 mg kg−1 (Tian et al., 2013), and In northeast China, the average mercury content in coal is 0.158 mg kg−1, All of them were 0.10 mg kg−1 higher than the world-wide average (Nriagu and Pacyna, 1988; Yudovich and Ketris, 2005). We found that in the 234.93 million tons of standard coal produced in Jilin Province in 2020, 10%–15% is coal gangue (Khan et al., 2016). Meanwhile, automotive interior parts and bearing linings contain mercury (Nadal et al., 2016), and although the circulation of auto-motive parts containing mercury has been banned since 2006, there are still vehicles containing mercury on the market that are not scrapped or in the process of scrapping. In addition, there is a great risk of mercury release or environmental exposure in the disposal of mercury containing accessories, so it is important to analyze the accumulation and enrichment of mercury in industrial development areas to evaluate regional ecological and environmental security (Yan et al., 2018).
As the city and towns grew, the number of people accommodated by the city and the density of the urban population gradually increased, which puts urban population at an increasing risk of exposure to mercury pollution (Li R. X. et al., 2020), directly or indirectly affecting the health and natural resource welfare of residents. Fast-growing economy and industrialization, boosting the middle class in certain zones, have meant more imports of consumer goods and in the generation of large quantities of coal-based fuels and industrial wastes (Henríquez-Hernández et al., 2017). Progress of industrialization is always accompanied by the large consumption of fossil fuel, huge amounts of municipal waste exhausts and medical waste incineration (Wang et al., 2019; George et al., 2020; Senior et al., 2020). In addition, a large number of industry-related activities or proceedings, like non-ferrous metal smelting, chloralkaline industry, cement manufacturing, clay gold, automotive parts and accessories manufacturing, and mercury refining activities, have the risk of emitting by-products containing Hg or compounds of mercury species (Yan et al., 2018). Therefore, it is in need to clear up the present situation regarding mercury concentration and accumulation levels in the soil environments of an emerging peri-industrial town.
Precedential researches have paid much attention on the risk analysis of heavy metal pollution in coal mines, little is known about the hierarchical and spatial distribution of soil mercury pollution within city limits after newly-tapped industrializing areas in China (Bi et al., 2020; Fei et al., 2020). There is very heavy information on city-wide soil mercury pollution, which relates to the ongoing exposure of urban residents to mercury that accumulates in urban soils. (Khan et al., 2016). This exposure may take the form of inhalation, oral ingestion, or skin contact. For now, the mercury content levels in urban soils and other parts soils in some of the Chinese cities like Tianjin, Chongqing, Zhuzhou and so on have been investigated by many researchers (Shi et al., 2010; Lei and Yu, 2014; Yan et al., 2018; Sun et al., 2020; Zhao et al., 2020; Wang et al., 2021; Li et al., 2022; Kun and Cai, 2023). However, scarcity of knowledge has been revealed on the comprehensive risk assessment of mercury in peri-industrializing cities (Ouyang et al., 2021; Li et al., 2022). Hence, the focus of this study is Hg levels and their health risk assessment in the industry-agriculture integrated town.
The main purpose of this study was to evaluate the possible risk of heavy metal mercury contamination in soil of Gongzhuling area in Northeastern China as a county, which has prospered due to comprehensive forestry and agricultural resources and has undergone an industrialization process for decades. It is commonly believed that the chronic industrialization process causes soil Hg pollution, and this is despite the fact that changes in the energy mix have led to mercury pollution, the environmental retention of mercury may pose a risk to humans living in areas contaminated with mercury (Yao and Fang, 2017). Samples of surface soil in Gongzhuling area were collected to determine the concentration of mercury. The eco-logical risks of the Hg in soil were assessed by calculating the geo-accumulation index (Igeo) (Muller, 1969; Christophoridis et al., 2020) and the potential ecological risk index (Er) (Hakanson, 1980; Liu et al., 2020). The associated health risks were then assessed by determining the levels of metal exposure through oral ingestion, inhalation, and dermal contact, and then calculating HQ (the non-carcinogenic risk index) (EPA, 2001) and HI (the total non-carcinogenic risk value) (EPA, 2000). The results of this study will display a more comprehensive outlook of the risks arising from human exposure to heavy metal mercury in classic industry-agriculture integrated towns. It provides reference for health risk assessment of other industrial cities.
2 Materials and methods
2.1 Study area
The research area is located in Gongzhuling in southwest Jilin Province, 54 km southwest of the main urban area of Changchun, between E124°45′to 124°53′and N43°32′to 43°27′, with an area of 4,172.55 km2 and 36 km2 in the central urban area, with a permanent population of 86.2313 in 2021 (Statistics, 2021). The population density is about 212 people/km2 (Khan et al., 2016). It is a temperate continental monsoon climate, with an annual average temperature of 5.6°C, annual precipitation of 594.8 mm (Zhang et al., 2016), the main wind direction is southwest wind, and the green space coverage rate is 0.03% (Khan et al., 2016).
As an emerging industrial base and main grain-producing area, Gongzhuling City gives full play to its geographical and resource advantages and gives priority to the development of automobile parts and agricultural products processing industries (Statistics, 2021). Since then, Gongzhuling has formed two dominant industries and continuously promoted the development of related industries. In return, the two industries have standing sustained the social and economic development of the city and affected its industrial layout. For now, Gongzhuling plays the utmost role in corn production, and the food yield out has been stable at a level above 6 billion kilograms, ranking top among many counties. And even its corn-unit-yield also accounts for the most portion in China.
With the short distance between Gongzhuling and Changchun, Gongzhuling ac-tively develops and improves the auto parts production and assembly industry, espe-cially for China First Automobile Works Group industrial chain customization, since the establishment of the Changchun Automobile Industry Development Zone in 2005. From then on, Gongzhuling has trained a group of professional, technical per-sonnel, and enterprises, engaged in brake bars, adjusting arms, bearings, gas storage and other key products supply. At the same time, Gongzhuling is an important car accessories supply base with a considerable casting production scale.
Gongzhuling District is now constructing itself to be an essential part of Chang-chun city, playing the role of city backyard and new-industry base. It is gradu-ally de-veloping into an important agricultural scientific research base in Jilin Province and a livable city with agricultural and sideline product processing, auto parts pro-cessing, and warehousing and logistics as the leading industries. The goal of Gongzhuling is to form an important agricultural and sideline product processing and auto parts base in Jilin Province and drive the development of the logistics industry mainly focusing on auto parts distribution and agricultural products distribution. From the geographical view, Harbin-Dalian transportation in the south of the city, as well as the industry and urban corridor of the Gongzhuling section are the main axis of Gongzhuling’s indus-trial development, focusing on the development of industry and the third industry; Provincial highway S105 is an important east-west secondary in-dustry development axis of Gongzhuling City, focusing on the development of agri-culture-related industry, resource industry, and agriculture-related service industry. To investigate the mercury exposure risk, the research area of Gongzhuling was di-vided into three parts: the south, the middle and the north. The southern ecological environment, the central agricul-tural production area, and the northern animal hus-bandry and new energy develop-ment area were studied respectively.
2.2 Sampling and analysis processes
Reference Gongzhuling urban spatial pattern, select the research scope, including built-up area and newly developed industrial area. The research scope includes the early development zone and Gongzhuling New Area, which fully reflects the basic characteristics of the integrated development of agriculture and production. We divided 100 sampling cells (1 km × 1 km) using a random grid distribution method, and the position of each sampling point is plotted according to its latitude and longitude. Figure 1 shows the location of 100 sample points. In order to promote subsequent relationship research between mercury concentration and wind direction in urban soil, according to the urban regional planning of Gongzhuling, the research area was divided into four regions: east, south, west and north, with the intersection of Yingbin Avenue and Guowen Avenue as the center.
On a typical meteorological day, five-point mixed sampling method was used to collect samples in the 5 m × 5 m range. All samples were collected from 0 to 10 cm of topsoil and other debris were removed. And all samples are kept sealed in zipped polyethylene bags with each sample weighing approximately 500 g. For soil samples obtained in areas without hardening sampling points, dust in a certain area around the sampling point is taken as a test sample. After the sample collection process is complete, the sample is kept in a cool place until all analyses are completed.
The Zeeman LUMEX RA-915+ effect mercury analyzer was used to monitor the atmospheric total mercury content, the accuracy is 0.1 ng/g. All sampling points are divided into 0 cm and 100 cm vertical air layers, and the monitoring process is carried out using an on-board mercury meter (Zeeman LUMEX RA-915+) according to the sampling point number. After the instrument is self-calibrated, data is collected every 10 s 12–18 monitoring data points were obtained at each sampling point, with a data accuracy of 1 ng m−3. Select blank samples from open clean green space or urban green space and measure corresponding background values as reference. Combined with the UMA solid sample detection device, 50–200 mg air-dried soil samples were weighed in the unidirectional light channel sampling pool of the LUMEX RA-915+ mercury meter to determine the total mercury content in the soil. The minimum detection limit of solid samples determined by the instrument is 0.5 ng g−1. Quality control (QC) procedures calibrate XRF instruments against U.S. SRM(Standard Reference Material). The correlation coefficient R2 of soil sample test standard curve was 0.9999. The air transport speed of the instrument is 4 L min−1. Every soil sample, 3–8 parallel analyses are performed to eliminate abnormal data. GBW07424 Songnen Plain soil composition standard material (GSS-10) was used to construct the standard curve.
2.3 Ecological risk assessment
2.3.1 Geo-accumulation index (Igeo)
The Igeo is used to assess the levels of contamination of a particular metal in soil by assessing the degree of metal enrichment above baseline or background values. The Igeo is calculated according to Equation 1 as follows (Sabouhi et al., 2020; Meng, 1995):
Igeo: Geological accumulation index; Bi: Urban soil background value (0.040 mg kg−1); K: Correction index (usually 1.5) to account for changes in background values that may be due to differences in rock characteristics at different locations; Ci: Measured concentration of mercury (mg·kg−1). The classification of the Igeo is shown in Table 1.
2.3.2 Potential ecological risk index (Er)
The potential ecological harm degree of mercury in soil and atmosphere was evaluated by Er. Calculate Er according to Equation 2, as follows (Xu et al., 2008; Maanan et al., 2015; Gao et al., 2024):
Er: Potential ecological harm coefficient of the mercury; Tr: Mercury toxicity factor, set to 40; Ci: measured value of mercury content; and C0: Background value of Hg, set to 0.040 mg kg−1. Table 2 shows the relationship between potential ecological risk coefficient and the degree of Er hazard.
2.4 Health risk assessments
2.4.1 Exposure assessments
This study referred to the environmental health risk assessment method recom-mended by the United States Environmental Protection Agency (US EPA) for soil mercury health risk assessment (EPA, 1989). Soil mercury mainly enters the human body through oral ingestion, inhalation, and dermal contact (Liu et al., 2016). The long-term daily non-carcinogenic risk exposure doses of the above three exposure routes were calculated using the human exposure risk assessment model. The equations are as follows (Du et al., 2013; Vilavert et al., 2012):
In Equations 3–5, ADIinh: average daily exposure dose by inhalation [mg·(kg·d)−1]; ADIoral: The average daily exposure dose of oral intake [mg·(kg·d)−1]; ADIdermal: The average daily exposure dose by skin contact [mg·(kg·d)−1]; C: Measured concentration of mercury in soil (mg·kg−1); IRinh: Respiratory intake (m3·d−1), reference value is 20 m3 d−1; EF: Exposure frequency (d·a−1), reference value 350 days·a−1; ED: Exposure year (a), reference value is 25 a; BW: Body weight (kg), reference value is 55.9 kg; AT: Exposure time (d), reference value is 365 × ED (d); PEF: Particle emission factor (m3·kg−1), reference value 1.32 × 109 m3 kg−1; IRoral: Oral intake (mg·d−1), reference value is 114 mg d−1; SA: skin exposed area (cm2·d−1), the reference value is 5,000 cm2 d−1; SL: Skin adhesion [mg·(cm2)−1], the reference value is 1 mg·(cm2)−1; ABS: Skin absorption factor, reference value of 0.001.
2.4.2 Non-carcinogenic risk assessments
The heavy metal toxicity of mercury is not carcinogenic, so we use the non-carcinogenic risk index (HQ) to evaluate the non-carcinogenic risk of mercury. The non-carcinogenic risk index is calculated according to Equation 6, as follows (EPA, 2001):
ADIi: average daily exposure dose [mg·(kg·d)−1]; RfDi: reference dose [mg·(kg·d)−1]; RfDoral = 3.0 × 10−4 mg·(kg·d)−1; RfDdermal = 2.4 × 10−5 mg·(kg·d)−1; RfDinh = 1. 07 × 10−4 mg·(kg·d)−1.
The total non-carcinogenic risk value of mercury for the different pathways is indicated by HI (EPA, 2000), according to Equation 7:
When HI or HQ < 1, the risk is considered small and can be ignored; and when HI or HQ > 1, there is a potential non-carcinogenic risk (EPA, 1997; EPA, 2001).
3 Results
3.1 Mercury levels in the environment
3.1.1 Mercury in surface soils
Statistically, the average content of Hg in soil samples was 0.0354 ± 0.0570 mg kg−1, and the concentration ranged from 0.00130 to 0.632 mg kg−1. It can be seen that there is a great difference in soil mercury content in Gongzhuling District, and Table 3 shows Hg levels in these four parts. In 100 samples, 24% more than the background value of soil mercury in Jilin Province (0.04 mg kg−1), and the highest level reached 15.80 times of the background value. Overall, the mercury concentration in different regions of Gongzhuling is akin to the normal background value, but there are a few sample points that exceed the standard value highly.
3.1.2 Atmospheric mercury in urban areas
Atmospheric mercury concentration in the four directions within the study area are shown in Figure 2. For measurements of the atmosphere at 0 and 100 cm, and the results showed that the average concentrations at the two altitudes were 3.3 ± 6.2 ng m−3 and 4.9 ± 11.9 ng m−3, respectively, and the concentrations ranged from 0 to 64.0 ng m−3 and 0–116 ng m−3, respectively. There is a higher mercury concentration at 100 cm. The blank samples had the same atmospheric mercury content at 0 cm and 100 cm, averaging 1.2 ± 1.5 ng m−3. The results showed that the atmospheric mercury content of 54.63% samples at 0 cm was higher than that of the blank control, and the maximum mercury content was 53.33 times higher than that of the blank control. The atmospheric mercury content of 47.58% samples at 100 cm was higher than that of blank control, and the highest mercury content reached 97.67 times of that of blank control. The atmospheric mercury content in Gongzhuling District changed greatly. It can also be seen from Figure 2 that the atmospheric mercury content in different regions of the study area has an obvious trend. In the southern region, atmospheric mercury concentrations of 0 cm and 100 cm are higher.
3.2 Risk assessment
3.2.1 Ecological risk assessment of soil mercury
According to Igeo, 9% of the samples showed mercury contamination (index >0), among which samples 7 and 49 were highly contaminated. Sample 86 is medium; Samples 34, 42, 43, 44, 59 and 82 were mildly moderate; Other samples were judged to be free of contamination.
Overall, the Hg pollution degree in Gongzhuling area is low. The results showed that the soil mercury pollution samples were concentrated in the industrial center, near the railway station and on both sides of the main traffic circle in the study area. Soil mercury contamination samples were distributed near driving schools, automobile markets, commercial centers and logistics parks. According to the literature, mercury in soil may be partly caused by atmospheric deposition (Zhou et al., 2021), but coal burning emissions, industrial production and automobile exhaust are also important sources of pollution (Singh et al., 2023). Combined with the industrial distribution of Gongzhuling, we hypothesize that soil mercury contamination at these sites is mainly caused by mercury emissions from transportation.
The results showed that the minimum Er value of soil mercury was 2.23 and the maximum was 214.83, and 24% of the samples had Er values higher than 40, indicating moderate or higher potential ecological risk. It is worth noting that some samples have strong potential ecological risks (Er > 160). The probable cause is the sample location was close to the automobile accessory workshops and the automotive repair shop, resulting in the potential ecological risk score of soil mercury in this area is higher. The results show that all samples have potential ecological risks, of which 6% have strong potential ecological risks and 16% have moderate potential ecological risks. In summary, the mercury pollution level in Gongzhuling area has a slight ecological risk.
3.2.2 Health risk assessment of soil mercury
The average HI values for each direction in the study area are ranked as follows: west (0.236 × 10−1) > east (0.202 × 10−1) > north (0.556 × 10−2) > south (0.447 × 10−2). The west region contains concentrated automobile workshops, and therefore the total health risk value is high. The southern part of Gongzhuling District’s is primarily made up of newly tapped residential areas and integrated municipal infrastructure. And also, because its upwind situation of this city, the environment quality is generally high, and has the lowest overall health risk in the city. In the east section, with a cluster of driving schools, and intensive industrial transportations between the Changchun City and automobile industry development zone, large traffic flow and exhausts contributed a lot to the accumulation of the soil mercury (Li Y. M. et al., 2020; Wang and Song, 2021), so the mercury level is also high. Overall, the HI value in Gongzhuling was determined to be much less than 1 (Li and Zhao, 2022). At present, mercury levels in urban soil do not pose a health threat to local adults.
This study was evaluated with reference to the environmental health risk assessment method recommended by the United States Environmental Protection Agency (EPA, 1989). The non-carcinogenic risks of the three major soil mercury exposure routes were compared by oral ingestion (mean 0.352 × 10−3)> skin contact (mean 0.193 × 10−3)> inhalation (mean 0.131 × 10−6). Food ingestion is the primary route of human exposure to soil mercury, and respiratory exposure contributes little compared to ingestion exposure.
4 Discussion
4.1 Mercury distribution
4.1.1 Spatial distribution characteristics of soil mercury
ArcGIS 10.7 was used to analyze and intuitively express the spatial distribution characteristics of soil Hg in the study area as shown in Figure 3. The Kriging interpolation method was used for the analysis to display the research result (Li et al., 2022). As is showed in the figure, there were significant differences in soil mercury content in the study area. The north is significantly higher than the south. The northwest of the research area has a cluster of auto repair shop and auto parts factory, along with a large traffic flow. It has been figured out that mercury could be emitted through gas emissions and the leaching of accessories that had been put in use before the ban on mercury application on the decorations and gadgets of automobiles. Besides, most Hg enriched in the soil exits in stable states and has a slight ability to reenter the surface water and other media, exerting a heterogeneous difference amongst each part of the city (Da Silva et al., 2022). Some studies found that mercury could be accumulated in the soil in the vicinity of iron and steel production areas, accessory allocation plants, as well as coal-fired power plants and thermal plants in general (Zhao et al., 2019; Qureshi, 2022).
In addition, rapid urbanization in recent years has brought development to new urban areas located in the southern part of the study area. With the increase in the built-up area and the enrichment of municipal infrastructure, resulting in the increasing population density in Gongzhuling District, the spatial distribution of mercury in urban soil was diffuse (Sodango et al., 2018). The soil mercury content in the southern new urban area is relatively low, but there are a few soil mercury rich areas, which are mainly distributed in large residential areas and high-tech parks.
High levels of mercury were also detected in the soil in commercial areas located in the middle of the study area and residential areas located in the north. Despite the large traffic and vehicle exhausts, the garbage yields are large, and mercury containing waste is relatively high, and finally form the high mercury contents in the surroundings and soils in the vicinity of commercial area. It is worth noting that there is also a Railway Station in this area. The main categories of goods transported at this station are not only contain households, fertilizer, corn, and mostly coals and accessories of automobiles. Part of the soil Hg in the region is brought by coal dust and fly ash produced during coal transportation. The residential areas in the northeast are part of the old city, where many large and medium-sized heavy industries were once located, including cement factories and thermal power plants (Wang et al., 2021; Liu et al., 2023). Previous studies have shown that the mercury content in the soil around coal burning enterprises is much higher than the world average (0.03 mg kg−1). This is directly affected by the industrial activities with Hg-containing, and there was an inverse relationship between soil mercury concentration and site distance (Nadal et al., 2016; Jones et al., 2021). This is consistent with the results of this study.
The comparison of soil Hg content between other towns and Gongzhuling District is shown in Table 4. The ten different areas of Shanghai, Shannxi, Changchun, Guannan, Zhuzhou, Huhhot, Nanjing, Changzhou, Linyi, Guizhou, and Chongqing have all in the process of industrialization. Shanghai, Tianjin, Chongqing, and Changzhou have comprehensive and specific industrial lines, whereas the Jinghui, Huhhot, and other cities are still in the intensive industrial constructions. It was found that urban areas with coal burning, commercial and logistics activities had higher soil mercury content than other areas, indicating that industrial activities had an impact on the mercury level in nearby soil. By comparing the soil Hg content between the central urban area with frequent traffic and logistics, we found that the Hg content in soil is inversely proportional to the distance from the city center. The mercury concentrations in the industry developing areas were generally lower than the industrial intensive areas, which indicated that the mercury values varied in accordance with the industrial levels. This implied that industrial process of Gongzhuling district could possibly have impacts on mercury accumulations and enrichments in this study area. In general, as shown in Figure 3, the overall high soil mercury content in the southwest of the study area showed a decreasing spatial distribution trend from the center to the suburbs.
4.1.2 Spatial distribution characteristics of atmospheric mercury
The spatial distribution of 0 cm and 100 cm atmospheric mercury concentration in Gongzhuling is shown in Figure 4. The data showed that the content of mercury at 0 cm and 100 cm is generally at the same level. Specifically, atmospheric sample points in the southern part had the highest mercury level of all the tested re-gions both in the 0 cm and 100 cm levels. This might be because of the long-distance mitigation of mercury due to the southwestern dominant wind direction.
Located around the railway station and expressway, the samples in the south were highly impacted by the large traffic flow and goods distributions. The waste gas and exhausts discharged by the vehicles and other kinds of automobiles could possibly contain different concentration of Hg, which mostly enters the atmosphere and arise the mercury level of the southern part environment medium of the city. It is figured out by some articles that traffic exhausts fumes have the most side-effect of terrain mercury on sides of urban streets (Lei and Yu, 2014). And so the main sources of the high atmospheric mercury levels in the southern part of the city are mercury-contained exhausts and domestic wastes.
4.1.3 Relationship between soil mercury concent and atmospheric mercury levels
As shown in Figure 5, atmospheric Hg levels at 0 cm and 100 cm and soil Hg concentration were presented as scatter plots, and no obvious linear correlation was found between atmospheric mercury levels and soil mercury content. In the research, the atmospheric deposition of mercury had little impacts on the soil mercury levels. This may be due to the greater ability of atmospheric mercury to travel long distances. In this study, Cloudy weather with little solar radiation and the content of oxidized gaseous element mercury is low, thus enhancing the transport of gaseous mercury, which in turn greatly affects the migration transformation of gaseous mercury between the atmosphere and the soil (Lin and Pehkonen, 1999).
Scatter plots of atmospheric mercury concentration and soil mercury content at 0 cm and 100 cm were drawn, which showed that there was no significant linear correlation between atmospheric mercury levels and soil mercury content. So atmospheric mercury deposition had little affection on soil mercury levels in this study, and this is in accordance with the high mobility of mercury species to migrate over some distances.
4.1.4 Relationship between soil mercury concentration and wind direction
The effects of north, south, east and west wind directions on soil Hg concentration in Gongzhuling main urban area were analyzed by using box diagram and variance analysis. The results are shown in Figure 6.
According to Figure 6, the soil mercury concentration was different in different wind direction, and the highest was in the west wind direction. The results of one-way ANOVA showed that there were significant differences in soil mercury concentration between north and south (p = 0.754 × 10−15 << 0.05). The concentrations of mercury in the north and west soils were relatively equal. There was no significant difference in soil Hg content between East and west (p = 0.9955 > 0.05). Soil mercury concentrations in the west, east, and north were all slightly different relative to the south (p-values of 0.1197, 0.1308, and 0.0507 for the three relative to the south, respectively). In summary, there is an obvious relationship between soil Hg pollution and wind direction in Gongzhuling area. During the sampling period from early July to early October, the prevailing southwest wind in Gongzhuling area affected the migration of air pollutants containing mercury from southwest to northeast. Atmospheric Hg enters regional soils by dry and wet deposition, henceforth resulting the regional mercury pollution (Li R. X. et al., 2020; Zhang et al., 2020).
4.2 Risk assessment of soil mercury pollution in Gongzhuling District
4.2.1 Ecological risk assessments of the soil mercury
Figure 7 shows the descending plot of Igeo. Figure 8 depicts the Igeo distribution of soil mercury.
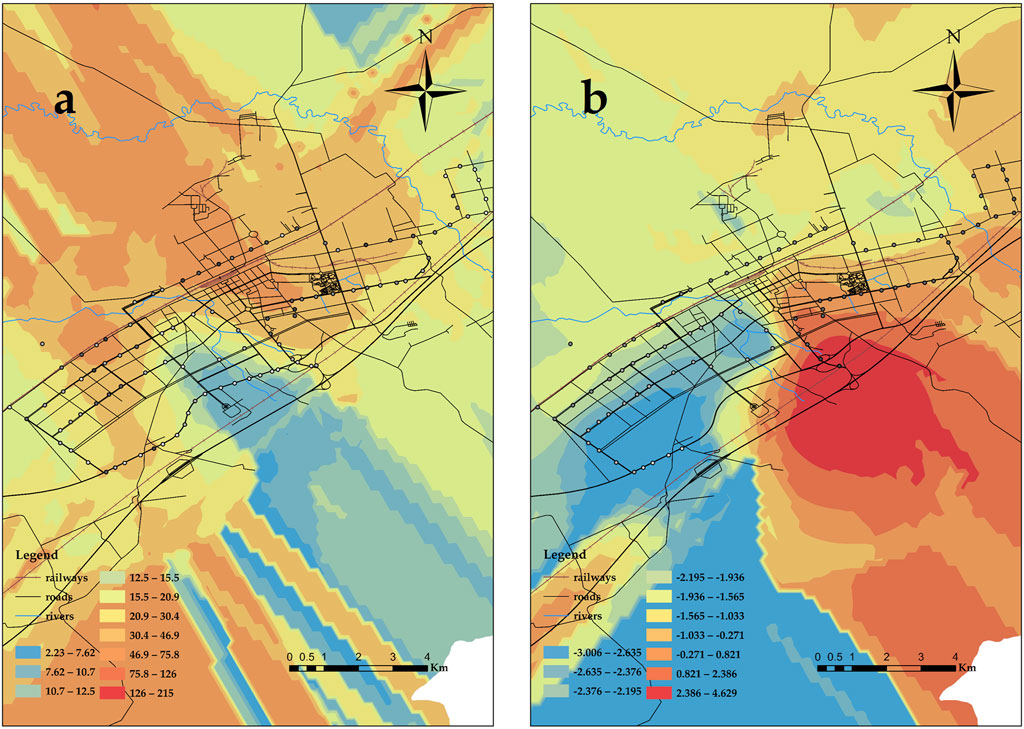
Figure 8. Characteristic distribution of soil mercury pollution: (A) distribution of the soil mercury Igeo in Gongzhuling District; (B) distribution of the soil mercury Er in Gongzhuling District.NE.Bib.
Igeo results showed that only 9% of the sample had contaminated (index >0), and two samples of them were seriously polluted, 7% sample showed moderate contamination, and most of the samples were not contaminated. Overall, the results of soil mercury accumulation index showed that the level of soil Hg pollution in Gongzhuling was low, and majority concentrated in specific areas. According to Figure 8A, the contaminated area is concentrated mainly on the northwest of Gongzhuling, which in accordance with the detection results of soil mercury concentration. The northwest region approach Gongzhuling industrial concentration area, manifesting that industrial activities bring a lot of mercury pollution to the surrounding soil. Besides, some of the polluted sam-ples were concentrated in the vicinity of commercial area of the urban, both sides of the main avenues, and around small automobile repair shops, suggesting that the traffic of urban contributes lots to the soil mercury pollution in the investigated area obviously.
Figure 8B shows soil Hg pollution from potential ecological risk levels of spatial distribution. The Er values of soil Hg ranged from 2.3 to 214.83 with significant difference. According to Figure 8B, soil mercury content in the northeastern part of the study area was the highest overall. Based on the urban industrial layout of Gongzhuling area, it is observed that eastern spots have the industrial cluster and the frequent logistical transportations towards Changchun. In summary, the potential ecological risk of soil mercury in this area is high.
4.2.2 Assessment of the potential health risks of soil mercury
As a peri-industrialized town, Gongzhuling District is a relatively industries in-tensive town. Since large-scale industry investments began, the content and spatial distribution of mercury in urban soil were partially affected (Rodrigues et al., 2006; Zhang and Wong, 2007; Henríquez-Hernández et al., 2017; Yan et al., 2018; Li R. X. et al., 2020). The results prove that soil Hg was enriched near the intensive industrial park. Figure 9 shows the source and location of mercury in Gongzhuling City through the investigation about Hg sources in Gongzhuling City, combined result about experimental and analysis from this study and related literature of other industrial cities. The industrial activities of automobile on the loop circle of the area are the main source of soil Hg. The agglomeration trend of automobile industry has led to the main layout of Gongzhuling’s industry structure, and caused a distribution pattern of Hg discharge in this space, such as iron smelters, flume exhausts, and gas emissions from coal-fired thermal plants (Yan et al., 2018; Lv et al., 2023). Urban soil Hg take on spatial distribution traits of slight and large scale amongst the whole area since the source in the study area also contains waste incineration, transportation, and so on (Xu and Lu, 2016). In summary, the study found that the main urban area of soil mercury enrichment area is very few, and the control of mercury-contain fuel played a vital role on mercury management.
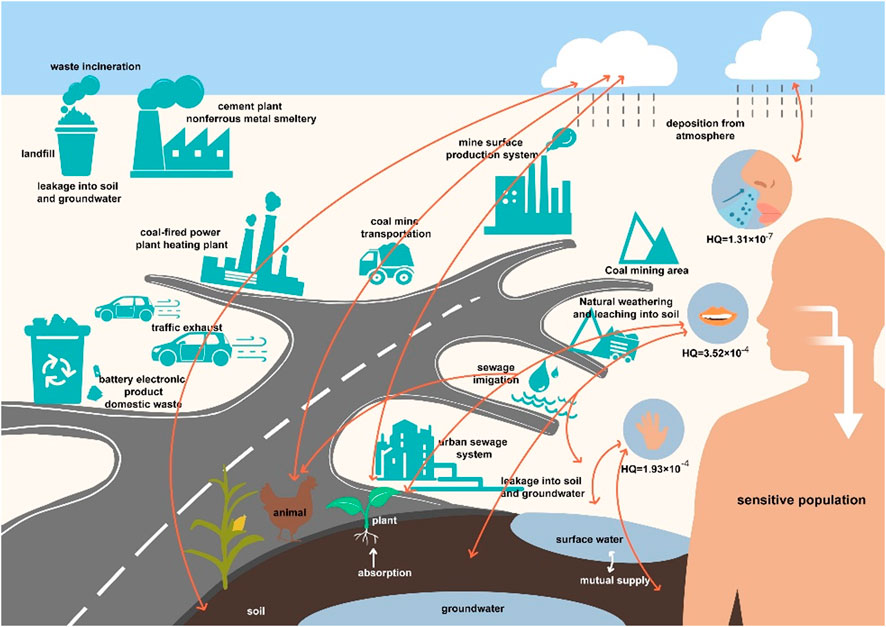
Figure 9. Schematic diagram of sources and locations of environmental elemental mercury in typical industrial city and human mercury exposure pathways.
Combined with the soil mercury exposure risk investigation in typical industrial cities and this study results, the mercury exposure diagram of residents in industrial cities was drawn, as shown in Figure 9, with the purpose of identifying the sources and ways of soil Hg exposure risk of urban residents in intensive automobile industry. Typically, citizens in industrial areas contact with Hg through inhalation of mercury-containing exhaust gases or dust from human activities; skin contact with solids or liquids containing mercury; or get Hg from food.
As shown in Figure 10, this study mapped non-carcinogenic risk levels in four different directions for three exposure pathways in the study area.
The results of Figure 10 show that the non-carcinogenic risk ranking of the three exposure routes is: oral ingestion > skin contact > inhalation, The three are mainly taken oral ingestion. In other studies, concerns have been raised that mercury exposure through air, water and other media also poses risks to human health, including intensive contact with the mercury-enriched working environment, municipal solid wastes and incinerations, and many disposals on digital devices as well. Besides, exposure may occur due to the in-take, thus leading to mercury bioaccumulation effects in animals, which could result in a large amount of mercury exposure (Henríquez-Hernández et al., 2017; Li Y. M. et al., 2020; Da Silva et al., 2022). In the north of Gongzhuling District, there are a cluster of automobile industry. The south area of Gongzhuling District is newly tapped area with comprehensive and complete municipal infrastructure. In that the industrial exhausts and automobile gas emissions in the region may potentially put environment at risk. It is known from the literature that the soil mercury in farmland near intensive industrial parks poses a higher health risk. For instance, fast-growing economy and industrialization, boosting the middle class in certain areas, have meant a parallel increase in the importation of consumer goods and in the gen-eration of large quantities of coal-based fuels and industrial wastes (Henríquez-Hernández et al., 2017). Besides, there could be difficulties on scrutinize the instant vary among industrial atmosphere, but more extensive in terms of number of samples, should be conducted to assure that gaseous mercury has been progressively degraded or transformed through time (Nadal et al., 2016). Overall, the health risk and total health risk levels for all three exposure routes of soil mercury are well below 1, indicating that soil mercury contamination does not pose a health hazard to local adults in the survey area. Our next work direction should be to control the existing soil pollution in cities and towns, improve the environment, and promote the construction of urban ecological civilization.
5 Conclusion
The average soil mercury concentration in the Gongzhuling District urban area was 0.0354 ± 0.0570 mg kg−1, and the range was 0.00130∼0.632 mg kg−1. The mercury content of 24% of the soil samples exceeded the regional soil background value of Jilin Province (0.04 mg kg−1). According to the Igeo, 9% of the sample sites in the study area were polluted (index >0). In general, the soil mercury pollution level in Gongzhuling District was low, and the polluted areas were mainly concentrated in the northeast of Gongzhuling District’s main urban area. The Er of the soil mercury in the study area ranged from 2.23 to 214.83, and 24% of the sampling sites presented a moderate or above potential ecological risk (Er > 40). The potential ecological risk level of the soil mercury was higher in the north of the study area. In terms of non-carcinogenic risk, the three main soil mercury exposure pathways were ranked as follows: oral ingestion (average 0.352 × 10−3) > dermal contact (average 0.193 × 10−3) >> inhalation (average 0.131 × 10−6). The oral ingestion of soil is the main route of human mercury exposure. In addition, the health risk and total health risk values of the three types of soil mercury exposure were far less than 1, indicating that the soil mercury pollution in the study area poses no harm to the health of local adults.
Data availability statement
The original contributions presented in the study are included in the article/supplementary material, further inquiries can be directed to the corresponding author.
Author contributions
TZ: Writing–original draft, Writing–review and editing. YH: Software, Validation, Writing–original draft. JW: Formal Analysis, Investigation, Writing–review and editing. SY: Data curation, Writing–review and editing. JH: Writing–original draft. WH: Writing–original draft. HH: Supervision, Writing–review and editing. GZ: Conceptualization, Methodology, Project administration, Resources, Visualization, Writing–review and editing.
Funding
The author(s) declare that financial support was received for the research, authorship, and/or publication of this article. This research was funded by the Major science and technology project of China Power Engineering Consulting Group Co., LTD. (No.DG3-P01-2022); Science and Technology Development Plan Project of Jilin Province, China (20240101067JC); the Science and Technology Research Project of Jilin Provincial Education Department (No. JJKH20231316KJ); the Chinese National Natural Science Foundation of China (Grant No. 31230012, 31770520); the Fundamental Research Funds for the Central Universities (No. 134-135132028); and the Chinese Postdoctoral Science Foundation (2021M700496).
Acknowledgments
We are grateful to the Key Laboratory of Vegetation ecology of the Ministry of Education for its help and support.
Conflict of interest
Authors TZ, WH, and HH were employed by China Energy Engineering Group Guangxi Electric Power Design Institute Co., LTD.
Author SY was employed by China Energy Engineering Group Guangdong Electric Power Desing Institute Co., LTD.
The remaining authors declare that the research was conducted in the absence of any commercial or financial relationships that could be construed as a potential conflict of interest.
The authors declare that this study received funding from China Power Engineering Consulting Group Co., LTD. The funder was involved in the whole process of the study, including the design of the study, the collection, analysis, interpretation of the data, the writing of the paper, and the decision on whether to submit it for publication.
Publisher’s note
All claims expressed in this article are solely those of the authors and do not necessarily represent those of their affiliated organizations, or those of the publisher, the editors and the reviewers. Any product that may be evaluated in this article, or claim that may be made by its manufacturer, is not guaranteed or endorsed by the publisher.
References
Balasundaram, K., and Sharma, M. (2019). Technology for mercury removal from flue gas of coal based thermal power plants: a comprehensive review. Crit. Rev. Environ. Sci. Technol. 49 (18), 1700–1736. doi:10.1080/10643389.2019.1583050
Bi, X. Y., Zhang, M. H., Wu, Y., Fu, Z., Sun, G., Shang, L., et al. (2020). Distribution patterns and sources of heavy metals in soils from an industry undeveloped city in Southern China. Ecotoxicol. Environ. Saf. 205, 111115. doi:10.1016/j.ecoenv.2020.111115
Christophoridis, C., Evgenakis, E., Bourliva, A., Papadopoulou, L., and Fytianos, K. (2020). Concentration, fractionation, and ecological risk assessment of heavy metals and phosphorus in surface sediments from lakes in N. Greece. Environ. Geochem. Health 42 (9), 2747–2769. doi:10.1007/s10653-019-00509-x
Da Silva, D., Bittencourt, L. O., Baia-da-Silva, D. C., Chemelo, V. S., Eiró-Quirino, L., Nascimento, P. C., et al. (2022). Methylmercury causes neurodegeneration and downregulation of myelin basic protein in the spinal cord of offspring rats after maternal exposure. Int. J. Mol. Sci. 23 (7), 3777. doi:10.3390/ijms23073777
Deng, C., Ping, X., Yang, W., Huang, X., and Zhang, L. (2012). Selection of the background value for enrichment factor. Environ. Chem. 31 (9), 1362–1367.
Driscoll, C. T., Mason, R. P., Chan, H. M., Jacob, D. J., and Pirrone, N. (2013). Mercury as a global pollutant: sources, pathways, and effects. Environ. Sci. & Technol. 47 (10), 4967–4983. doi:10.1021/es305071v
Du, Y., Gao, B., Zhou, H., Ju, X., Hao, H., and Yin, S. (2013). “Health risk assessment of heavy metals in road dusts in urban parks of Beijing, China,” in Proceedings of the 4th international symposium on environmental science and technology (ISEST) (Dalian: PEOPLES R CHINA), 299–309. 2013, Jun, 04-07, 2013.
EPA (1989). “Risk-assessment guidance for superfund volume 1,” in Human health evaluation manual (Part A). U.S. EPA-540/1-89/002.
EPA (1997). “Mercury study report to congress. Health effect of mercury compounds,”. U.S. EPA-452/R-97-007.
EPA (2001). Supplemental guidance for developing soil screening levels for superfund sites. Washington: U.S.
Fei, X. F., Lou, Z. H., Xiao, R., Ren, Z., and Lv, X. (2020). Contamination assessment and source apportionment of heavy metals in agricultural soil through the synthesis of PMF and GeogDetector models. Sci. Total Environ. 747, 141293. doi:10.1016/j.scitotenv.2020.141293
Feng, X., Chen, J., Fu, X., Hu, H., Li, P., Qiu, G., et al. (2013). Progresses on environmental geochemistry of mercury. Bull. Mineralogy Petrology Geochem. 32 (5), 503–530.
Gao, S., Dong, Y., Jia, Q., Wu, S., Bai, J., Cui, C., et al. (2024). Hazards of toxic metal(loid)s: exploring the ecological and health risk in soil-crops systems with long-term sewage sludge application. Sci. Total Environ. 948, 174988–175014. doi:10.1016/j.scitotenv.2024.174988
George, A., Shen, B. X., Kang, D., Yang, J., and Luo, J. (2020). Emission control strategies of hazardous trace elements from coal-fired power plants in China. J. Environ. Sci. 93, 66–90. doi:10.1016/j.jes.2020.02.025
Hakanson, L. (1980). An ecological risk index for aquatic pollution control.a sedimentological approach. Water Res. 14 (8), 975–1001. doi:10.1016/0043-1354(80)90143-8
Henríquez-Hernández, L. A., Luzardo, O. P., Boada, L. D., Carranza, C., Pérez Arellano, J. L., González-Antuña, A., et al. (2017). Study of the influencing factors of the blood levels of toxic elements in Africans from 16 countries. Environ. Pollut. 230, 817–828. doi:10.1016/j.envpol.2017.07.036
Hu, S., Yuan, X., Chen, H., Li, T., and Liu, Q. (2015). Multi-scale spatial distribution and source discrimination of heavy metals in suburb soils: a case study of junctional zone between nanjing city and zhenjiang city. J. Agro-Environment Sci. 34 (12), 2295–2303.
Jones, I., Zhu, M. M., Zhang, J., Zhang, Z., Preciado-Hernandez, J., Gao, J., et al. (2021). The application of spent tyre activated carbons as low-cost environmental pollution adsorbents: a technical review. J. Clean. Prod. 312, 127566. doi:10.1016/j.jclepro.2021.127566
Karthikeyan, O. P., Smith, T. J., Dandare, S. U., Parwin, K. S., Singh, H., Loh, H. X., et al. (2021). Metal(loid) speciation and transformation by aerobic methanotrophs. Microbiome 9 (1), 156. doi:10.1186/s40168-021-01112-y
Khan, S., Munir, S., Sajjad, M., and Li, G. (2016). Urban park soil contamination by potentially harmful elements and human health risk in Peshawar City, Khyber Pakhtunkhwa, Pakistan. J. Geochem. Explor. 165, 102–110. doi:10.1016/j.gexplo.2016.03.007
Kun, Z., Cai, Y. K., Chen, W., and Peng, P. (2023). Source identification and spatial distribution of heavy metals in soil of central urban area of Chongqing, China. Soil & Sediment Contam. 32 (6), 771–788.
Lei, L., Yu, D., Chen, Y., Song, W., Liang, D., and Wang, Z. (2014). Spatial distribution and sources of heavy metals in soils of Jinghui Irrigated Area of Shaanxi, China. Trans. Chin. Soc. Agric. Eng. 30 (6), 88–96.
Li, R., Wu, H., Ding, J., Fu, W., Gan, L., and Li, Y. (2017). Mercury pollution in vegetables, grains and soils from areas surrounding coal-fired power plants. Sci. Rep. 7, 46545. doi:10.1038/srep46545
Li, R. X., Yuan, Y., Sun, W., Yang, M., and Wang, X. (2020a). Environmental health and ecological risk assessment of soil heavy metal pollution in the coastal cities of estuarine bay-A case study of hangzhou bay, China. Toxics 8 (3), 75. doi:10.3390/toxics8030075
Li, X., Zhao, L., Teng, Y., Luo, Y., Huang, B., Liu, C., et al. (2022). Characteristics, spatial distribution and risk assessment of combined mercury and cadmium pollution in farmland soils surrounding mercury mining areas in Guizhou. Ecol. Environ. Sci. 31 (8), 1629–1636.
Li, Y., Yu, J. L., Liu, Y., Huang, R., Wang, Z., and Zhao, Y. (2022). A review on removal of mercury from flue gas utilizing existing air pollutant control devices (APCDs). J. Hazard. Mater. 427, 128132. doi:10.1016/j.jhazmat.2021.128132
Li, Y. M., Chen, L., Liang, S., Qi, J., Zhou, H., Feng, C., et al. (2020b). Spatially explicit global hotspots driving China's mercury related health impacts. Environ. Sci. & Technol. 54 (22), 14547–14557. doi:10.1021/acs.est.0c04658
Li, Z. G., Feng, X. B., Li, G., Bi, X., Zhu, J., Qin, H., et al. (2013). Distributions, sources and pollution status of 17 trace metal/metalloids in the street dust of a heavily industrialized city of central China. Environ. Pollut. 182, 408–416. doi:10.1016/j.envpol.2013.07.041
Lin, C. J., and Pehkonen, S. O. (1999). The chemistry of atmospheric mercury: a review. Atmos. Environ. 33 (13), 2067–2079. doi:10.1016/s1352-2310(98)00387-2
Liu, C., Liao, Q., Huang, S., Jin, Y., Zhu, B., and Hua, M. (2009). Studies on trace elements geochemistry of atmospheric dust and related issues in urban geological survey in Jiangsu. J. Geol. 33 (02), 138–149.
Liu, K. H., Li, C. M., Tang, S., Shang, G., Yu, F., and Li, Y. (2020). Heavy metal concentration, potential ecological risk assessment and enzyme activity in soils affected by a lead-zinc tailing spill in Guangxi, China. Chemosphere 251, 126415. doi:10.1016/j.chemosphere.2020.126415
Liu, T., Xiong, Z., Ni, P., Ma, Z., Tan, Y., Li, Z., et al. (2023). Review on adsorbents in elemental mercury removal in coal combustion flue gas, smelting flue gas and natural gas. Chem. Eng. J. 454, 140095. doi:10.1016/j.cej.2022.140095
Liu, Y., Lei, S. G., and Xiaoyang, C. (2016). Assessment of heavy metal pollution and human health risk in urban soils of a coal mining city in East China. Hum. Ecol. Risk Assess. 22 (6), 1359–1374. doi:10.1080/10807039.2016.1174924
Lv, D. W., Wu, Q. R., Ouyang, D., Wen, M., Zhang, G., Wang, S., et al. (2023). Differentiated emission control strategy based on comprehensive evaluation of multi-media pollution: case of mercury emission control. J. Environ. Sci. 123, 222–234. doi:10.1016/j.jes.2022.03.028
Maanan, M., Saddik, M., Chaibi, M., Assobhei, O., and Zourarah, B. (2015). Environmental and ecological risk assessment of heavy metals in sediments of Nador lagoon, Morocco. Ecol. Indic. 48, 616–626. doi:10.1016/j.ecolind.2014.09.034
Meng, X. X. L. S. Z. (1995). in Background value of soil elements in Jilin province (Beijing, China: Science Press).
Muller, G. (1969). Index of geoaccumulation in sediments of the rhine river. GeoJournal 2 (3), 109–118.
Nadal, M., Rovira, J., Díaz-Ferrero, J., Schuhmacher, M., and Domingo, J. L. (2016). Human exposure to environmental pollutants after a tire landfill fire in Spain: health risks. Environ. Int. 97, 37–44. doi:10.1016/j.envint.2016.10.016
Nriagu, J. O., and Pacyna, J. M. (1988). Quantitative assessment of worldwide contamination of air, water and soils by trace metals. Nature 333 (6169), 134–139. doi:10.1038/333134a0
Ouyang, D. W., Liu, K. Y., Wu, Q., Wang, S., Tang, Y., Li, Z., et al. (2021). Effect of the coal preparation process on mercury flows and emissions in coal combustion systems. Environ. Sci. & Technol. 55 (20), 13687–13696. doi:10.1021/acs.est.1c03689
Qureshi, A. (2022). Mercury in the environment around industrially impacted locations in India: a mini-review. Bull. Environ. Contam. Toxicol. 109 (6), 937–942. doi:10.1007/s00128-022-03548-w
Rodrigues, S., Pereira, M. E., Duarte, A., Ajmone-Marsan, F., Davidson, C., Grčman, H., et al. (2006). Mercury in urban soils:: a comparison of local spatial variability in six European cities. Sci. Total Environ. 368 (2-3), 926–936. doi:10.1016/j.scitotenv.2006.04.008
Sabouhi, M., Ali-Taleshi, M. S., Bourliva, A., Nejadkoorki, F., and Squizzato, S. (2020). Insights into the anthropogenic load and occupational health risk of heavy metals in floor dust of selected workplaces in an industrial city of Iran. Sci. Total Environ. 744, 140762. doi:10.1016/j.scitotenv.2020.140762
Senior, C., Granite, E., Linak, W., and Seames, W. (2020). Chemistry of trace inorganic elements in coal combustion systems: a century of discovery. Energy & Fuels 34 (12), 15141–15168. doi:10.1021/acs.energyfuels.0c02375
Shi, R. G., Lv, J. A., Cai, Y., Liu, Y., Wang, Z., Feng, J., et al. (2010). Levels, spatial distribution and possible sources of heavy metals contamination of suburban soils in Tianjin, China. Bull. Environ. Contam. Toxicol. 85 (3), 287–290. doi:10.1007/s00128-010-0070-5
Singh, A. D., Khanna, K., Kour, J., Dhiman, S., Bhardwaj, T., Devi, K., et al. (2023). Critical review on biogeochemical dynamics of mercury (Hg) and its abatement strategies. Chemosphere 319, 137917–137926. doi:10.1016/j.chemosphere.2023.137917
Sodango, T. H., Li, X., Sha, J., and Bao, Z. (2018). Review of the spatial distribution, source and extent of heavy metal pollution of soil in China: impacts and mitigation approaches. J. Health & Pollut. 8 (17), 53–70. doi:10.5696/2156-9614-8.17.53
Sun, G., Feng, X. B., Yang, C., Zhang, L., Yin, R., Li, Z., et al. (2020). Levels, sources, isotope signatures, and health risks of mercury in street dust across China. J. Hazard. Mater. 392, 122276. doi:10.1016/j.jhazmat.2020.122276
Tang, S. L., Wang, L. N., Feng, X., Feng, Z., Li, R., Fan, H., et al. (2016). Actual mercury speciation and mercury discharges from coal-fired power plants in Inner Mongolia, Northern China. Fuel 180, 194–204. doi:10.1016/j.fuel.2016.04.037
Tian, H. Z., Lu, L., Hao, J. M., Gao, J. J., Cheng, K., Liu, K. Y., et al. (2013). A review of key hazardous trace elements in Chinese coals: abundance, occurrence, behavior during coal combustion and their environmental impacts. Energy & Fuels 27 (2), 601–614. doi:10.1021/ef3017305
Vilavert, L., Nadal, M., Schuhmacher, M., and Domingo, J. L. (2012). Concentrations of metals in soils in the neighborhood of a hazardous waste incinerator: assessment of the temporal trends. Biol. Trace Elem. Res. 149 (3), 435–442. doi:10.1007/s12011-012-9441-6
Wang, J. X., Ma, L., Letcher, R., Bradford, S. A., Feng, X., and Rinklebe, J. (2022). Biogeochemical cycle of mercury and controlling technologies: publications in critical reviews in environmental science & technology in the period of 2017-2021. Crit. Rev. Environ. Sci. Technol. 52 (24), 4325–4330. doi:10.1080/10643389.2022.2071210
Wang, P., Hu, Y. A., and Cheng, H. (2019). Municipal solid waste (MSW) incineration fly ash as an important source of heavy metal pollution in China. Environ. Pollut. 252, 461–475. doi:10.1016/j.envpol.2019.04.082
Wang, Q., Song, Y., Wang, C., Xu, R., Peng, M., Zhou, Y., et al. (2021). Source identification and spatial distribution of soil heavy metals in Western Yunnan. China Environ. Sci. 41 (8), 3693–3703.
Wang, Z. J., Wang, L., Xiao, T., Yu, T., Li, X., Tang, Z., et al. (2021). Pollution characteristics and risk assessments of mercury in the soil of the main urban regions in a typical Chinese industrial city: Changchun. Pol. J. Environ. Stud. 30 (4), 3829–3841. doi:10.15244/pjoes/131051
Xu, Y., Lu, F., Dong, L., Yang, J., Nai, C., and Liu, Y. (2016). Human health effects of heavy metals around an incineration plant through multi-media exposure. Acta Sci. Circumstantiae 36 (9), 3464–3471.
Xu, Z., Ni, S., Tuo, X., and Zhang, C. (2008). Calculation of heavy metals toxicity coefficient in the evaluation of potential ecological risk index. Enuivonmental Sci. Technol. 31 (2), 112–115.
Yan, G., Mao, L. C., Liu, S., Mao, Y., Ye, H., Huang, T., et al. (2018). Enrichment and sources of trace metals in roadside soils in Shanghai, China: a case study of two urban/rural roads. Sci. Total Environ. 631-632, 942–950. doi:10.1016/j.scitotenv.2018.02.340
Yang, Z. P., Lu, W. X., Long, Y., Bao, X., and Yang, Q. (2011). Assessment of heavy metals contamination in urban topsoil from Changchun City, China. J. Geochem. Explor. 108 (1), 27–38. doi:10.1016/j.gexplo.2010.09.006
Yao, Y., Fang, F., Wu, J., Zhu, Z., Lin, Y., Zhang, D., et al. (2017). Concentrations and pollution assessment of mercury in surfaces dust at urban streets and elementary school campuses of Huainan City. Acta Sci. Circumstantiae 37 (3), 844–852.
Yudovich, Y. E., and Ketris, M. P. (2005). Mercury in coal: a review. Int. J. Coal Geol. 62 (3), 107–134. doi:10.1016/j.coal.2004.11.002
Zhang, H. C., Wang, T., Zhang, Y., Wang, J., Sun, B., and Pan, W. P. (2020). A review on adsorbent/catalyst application for mercury removal in flue gas: effect of sulphur oxides (SO2, SO3). J. Clean. Prod. 276, 124220. doi:10.1016/j.jclepro.2020.124220
Zhang, J. J., Wang, Y., Liu, J., Liu, Q., and Zhou, Q. (2016). Multivariate and geostatistical analyses of the sources and spatial distribution of heavy metals in agricultural soil in Gongzhuling, Northeast China. J. Soils Sediments 16 (2), 634–644. doi:10.1007/s11368-015-1225-0
Zhang, L., and Wong, M. H. (2007). Environmental mercury contamination in China: sources and impacts. Environ. Int. 33 (1), 108–121. doi:10.1016/j.envint.2006.06.022
Zhao, L., Liang, Y., Chen, Q., Xu, Q., and Jing, H. W. (2020). Spatial distribution, contamination assessment, and sources of heavy metals in the urban green space soils of a city in north China. Huan jing ke xue= Huanjing kexue 41 (12), 5552–5561. doi:10.13227/j.hjkx.202004277
Zhao, S. L., Pudasainee, D., Duan, Y., Gupta, R., Liu, M., and Lu, J. (2019). A review on mercury in coal combustion process: content and occurrence forms in coal, transformation, sampling methods, emission and control technologies. Prog. Energy Combust. Sci. 73, 26–64. doi:10.1016/j.pecs.2019.02.001
Keywords: pollution character, risk evaluation, mercury, industrialization, urban soil
Citation: Zhang T, Hu Y, Wu J, Yin S, Huang J, Huang W, Huang H and Zhang G (2024) Characteristics and risk assessments of mercury pollution in a county region undergoing industrialization in northeastern China: a case study in Gongzhuling. Front. Environ. Sci. 12:1440426. doi: 10.3389/fenvs.2024.1440426
Received: 26 July 2024; Accepted: 30 August 2024;
Published: 25 September 2024.
Edited by:
Željka Fiket, Ruđer Bošković Institute, CroatiaCopyright © 2024 Zhang, Hu, Wu, Yin, Huang, Huang, Huang and Zhang. This is an open-access article distributed under the terms of the Creative Commons Attribution License (CC BY). The use, distribution or reproduction in other forums is permitted, provided the original author(s) and the copyright owner(s) are credited and that the original publication in this journal is cited, in accordance with accepted academic practice. No use, distribution or reproduction is permitted which does not comply with these terms.
*Correspondence: Gang Zhang, emhhbmdnMjE3QG5lbnUuZWR1LmNu