- 1U.S. Fish and Wildlife Service (retired affiliate), Anchorage, AK, United States
- 2Fisheries and Oceans Canada, Winnipeg, MB, Canada
- 3Department of Ecoscience, Aarhus University, Roskilde, Denmark
- 4International Arctic Research Center, University of Alaska Fairbanks, Fairbanks, AK, United States
- 5Research Department, Norwegian Polar Institute, Tromsø, Norway
- 6College of Fisheries and Ocean Sciences, University of Alaska Fairbanks, Fairbanks, AK, United States
Climate change is impacting marine ecosystems throughout the circumpolar Arctic, altering seasonal habitats and the food bases for fishes, seabirds, and marine mammals. Arctic and Subarctic regions provide resources for resident species and for species that migrate to the north from more southerly regions. Changes in northerly latitudes thus impact endemic as well as non-endemic animals. Herein, we review what is known about climate-driven changes in the migration patterns of Arctic and Subarctic marine vertebrates, including: 1) Arctic residents with seasonal movements – those fishes, seabirds, and marine mammals that complete their entire life cycle within the Arctic but exhibit seasonal movements; 2) Breeding migrants – many seabirds enter the Arctic to breed and subsequently migrate south in the fall; and 3) Summer visitors for feeding – certain species of boreal fishes, seabirds and marine mammals arrive during the northern summer to feed on abundant prey though they breed elsewhere. Migratory movements are often driven by the timing and extent of sea ice, which defines suitable habitat for some animals and limits access to open water and prey for others. Longer open-water seasons, warmer ocean temperatures, and stronger winds have resulted in earlier production blooms in spring and often, extended open-ocean plankton blooms into late summer, resulting in altered prey types and distributions. A common thread among taxa is that shifts in distribution and timing of migrating animals indicate they are traveling farther north, or shifting longitudinally, and migrations are occurring over longer seasonal time frames. Species performing multiple lifetime migrations or long-distance migrants may need to adjust migration timing or routing iteratively to match changes in marine productivity. Altered animal distributions or phenology, and reduced sea ice, affects access to animals that are critical nutritional, economical, and cultural components of Indigenous people’s lives in the Arctic. Ongoing changes challenge the resilience and adaptability of Arctic people and ecosystems, and will require adaptive research and management approaches.
1 Introduction
Animal migrations have evolved in response to spatial and temporal changes in resource availability (Newton, 2008; Milner-Gulland et al., 2011; Evans and Bearhop, 2022). In the Arctic, much of the marine-based prey that support upper trophic level taxa are only available during the brief Arctic summer and fall. Animals that remain in the Arctic year-round often conduct seasonal movements within Arctic areas (Alerstam et al., 2007; Pettitt-Wade et al., 2021), while Subarctic and temperate animals that depend on the Arctic’s summer production period migrate north as sea ice retreats and return south as winter advances and darkness and low temperatures prevail (Berge et al., 2015a). Migration strategies depend on reasonable levels of ecosystem predictability to ensure timely access to prey for replenishment of energy stores and for breeding success. Among migratory species, the ability to adjust to spatial and temporal variability in resources varies along a continuum from narrow to flexible (Corkeron and Conner, 1999; Evans and Bearhop, 2022). However, climate change is altering marine systems such that resources and other environmental conditions are becoming more unpredictable in space and time (UNEP/CMS, 2006; Robinson et al., 2009; Gilg et al., 2012). This unpredictability is a current and future challenge for marine vertebrates that rely on migration to or within the Arctic to maximize their survival and reproductive success. Indigenous communities that rely on animal harvests are also impacted (Huntington et al., 2016; Hauser et al., 2021).
As climate change alters the timing and spatial distribution of resources, animals may need to alter their phenologies and migratory routes or staging areas. Changes in their traditional patterns and altered resource bases are likely to result in expending more energy searching for food and issues with finding adequate prey densities to support large-bodied animals (Bauer et al., 2008; Howard et al., 2018). Concurrently, other species may gain access to more direct routes, and more or higher-quality food over a longer season, reducing individual energetic expenditures of migration. Climate change induced impacts to migration can therefore affect reproductive success and overwintering survival, with repercussions to entire populations (Harrison et al., 2011). Ultimately, ecosystems will be impacted, as some fish species that are being negatively affected by climate change constitute key species in Arctic marine food webs, upon which many of the top trophic feeders depend (Welch et al., 1992; Hop and Gjøsæter, 2013). As upper trophic level consumers, predatory fishes, seabirds, and marine mammals serve as sentinels of ecosystem health (Moore et al., 2014; Sydeman et al., 2015; Moore and Kuletz, 2019). These three animal groups also constitute important food resources to people who rely on Arctic marine ecosystems for subsistence and many species serve as cultural cornerstones (Guyot et al., 2006; Huntington et al., 2013; 2016).
Migratory animals have significant impacts upon the marine ecosystems to which they migrate, serving as prey, predators, contributors to, and cyclers of, nutrients (Bauer and Hoye, 2014). Because animal migrations cross national boundaries, the United Nations established a global treaty on the Conservation of Migratory Species of Wild Animals (UNEP-WCMC, 2024), which defines a migratory species as: “The entire population or any geographically separate part of the population of any species or lower taxon of wild animals, a significant proportion of whose members cyclically and predictably cross one or more national jurisdictional boundaries.” While this definition overlooks migratory movements that only occur within national boundaries, it does recognize the impact of climate change on animal migrations, and the need to address how current and future environmental changes will impact animals that rely on migration strategies to maintain their populations.
The timing and extent of sea-ice retreat is a primary driver of Arctic marine systems, and in spring it affects water-column characteristics, phenology and spatial extent of primary production, and consequently, abundance of lower trophic organisms, species composition, and nutritional quality (Gaston et al., 2009; Daase et al., 2013; Leu et al., 2015; Stige et al., 2019). The decline in area, thickness, and seasonal duration of sea ice within the last 20 years has greatly altered Arctic ecosystems, and these declining trends in sea ice will continue as northern latitude air and water temperatures increase (Barber et al., 2015; Hunt et al., 2016; Meredith et al., 2019; IPCC et al., 2023). With warmer conditions, early sea-ice melt in spring has resulted in earlier spring blooms, increased chlorophyll, and higher zooplankton biomass that experiences higher turnover, albeit of smaller, low-nutrient prey species, from zooplankton to fishes (Österblom et al., 2008; Hop and Gjøsæter, 2013; Renaud et al., 2018; Møller and Nielsen, 2020; Copeman et al., 2022). Such changes also affect competition among species at different trophic levels (Bogstad et al., 2015; Stige et al., 2018). Subsequently, the distribution of zooplankton, pelagic and benthic invertebrates, and forage fishes affect the upper trophic level vertebrates that migrate to or within the Arctic, including annual (e.g., seabirds and marine mammals) and life-cycle stages (e.g., anadromous fishes).
Most migration to the Arctic occurs latitudinally, in the extreme, from the Antarctic to the Arctic (Egevang et al., 2010), but migration also occurs longitudinally across the circumpolar realm (Alerstam and Gudmundsson, 1999; Alerstam et al., 2007). Migratory patterns can be influenced by the presence or absence of ice and impacts vary across taxa (McKeon et al., 2016; Briscoe et al., 2017; Clairbaux et al., 2019). Migration patterns of nearshore foragers and anadromous fishes could also be affected by changes in riverine and coastal systems, as freshwater runoff, thawing terrestrial permafrost, retreating glaciers, increased sedimentation, and lack of shore-fast ice alter the coastal domains (Carmack et al., 2015). Likewise, populations that rely on transport by surface currents for successful migration may be affected by changes in ocean circulation patterns (Frederiksen et al., 2016; Eriksen et al., 2020).
The forms of migration vary widely within and among taxa. Resident species may spend most or all of their life cycle within Subarctic or Arctic areas but exhibit regular movements within those areas. Such patterns are exhibited by some species of anadromous and marine fishes (Pettitt-Wade et al., 2021), certain marine mammals (Von Duyke et al., 2020; Shuert et al., 2023; Storrie et al., 2023) and at least three species of seabirds (Divoky et al., 2016; Gilg et al., 2016a; b; Frederiksen et al., 2021). Breeding migrants include some seabird species that migrate from the Subarctic and elsewhere to breed in the Arctic. Non-breeding migrants arrive during summer and fall only to feed in the Arctic. Among these species, some arrive as post-breeders from the Subarctic or nearby temperate realms, including species of seabirds (Kuletz et al., 2015; Gaston et al., 2017; Piatt et al., 2021) and migrant baleen whales (Storrie et al., 2018; Moore et al., 2022). This pattern also includes some marine fishes that migrate seasonally to the Arctic, e.g., capelin (Mallotus villosus) and several gadid species (Haug et al., 2017; Mueter et al., 2021). A few avian species are long-distance migrants from the Southern Hemisphere and travel to the Subarctic and Arctic only to feed and increase body reserves (Kuletz et al., 2015; Yamamoto et al., 2015; Nishizawa et al., 2017). Regardless of origin or strategy, animals need to match the phenology of their movements to the availability of food resources, which can be particularly critical for species that move to the Arctic to breed (Klaassen, 2003). For example, most seabirds are highly philopatric to colony breeding sites (Hamer et al., 2001), and for certain anadromous fishes, migration to freshwater breeding sites may be site-specific and rather inflexible (Hendry and Sterns, 2004).
Migratory strategies of animals can range from extreme “income migrants” (also known as a “fly-and-forage” strategy) to “capital migrants” (Evans and Bearhop, 2022). Income migrants forage as they move towards their migration endpoint, with little or no physiological alteration to prepare for migration and rely on multiple ecosystems for replenishment while en route. At the opposite extreme are capital migrants, individuals that must store adequate reserves for the entire journey, often undergoing physiological alterations to conserve energy until they reach their endpoint (Evans and Bearhop, 2022). For capital migrants, the timing of movements is critical and depends on the availability of food and suitable environmental conditions immediately upon arrival. As our knowledge regarding foraging patterns of migrants increases, it appears that most animals fall somewhere along this continuum, and some may even alter their strategies at different stages of their migratory journey (e.g., Lydersen et al., 2020). Our goal was to review available scientific literature to provide a synthesis of information on the impact of climate change on migratory Arctic marine vertebrates (fishes, seabirds, and marine mammals), and thereby inform decision-makers about these conservation challenges.
2 Methods
2.1 Spatial and temporal scope
For our review we primarily considered the marine and associated freshwater inflows across the circumpolar Arctic as defined by the Arctic Council’s Conservation of Arctic Flora and Fauna (CAFF) boundaries, which includes Arctic and Subarctic waters (Figure 1; https://caff.is/work/projects/arctic-biodiversity-data-service/). The Arctic Ocean incorporates an area of approximately 14,060,000 km2, with most of its waters north of 66° N latitude, but including some Subarctic regions generally north of 60° N. The Arctic coastline within this region is ∼45,390 km, although the CAFF boundary incorporates regions farther south. In the Pacific Arctic, the Subarctic includes the entire Bering Sea and portions of the northwestern Gulf of Alaska shelf. In the Atlantic Arctic, the boundary includes Hudson Bay, the Labrador Sea, and waters off southern Iceland and northern Norway (Figure 1). Because many of the migratory animals we review herein spend portions of their life cycle south of these boundaries, we also refer to areas outside our primary study area as appropriate. Our temporal scope emphasizes changes that have occurred in animal migration reported in publications over the last two decades.
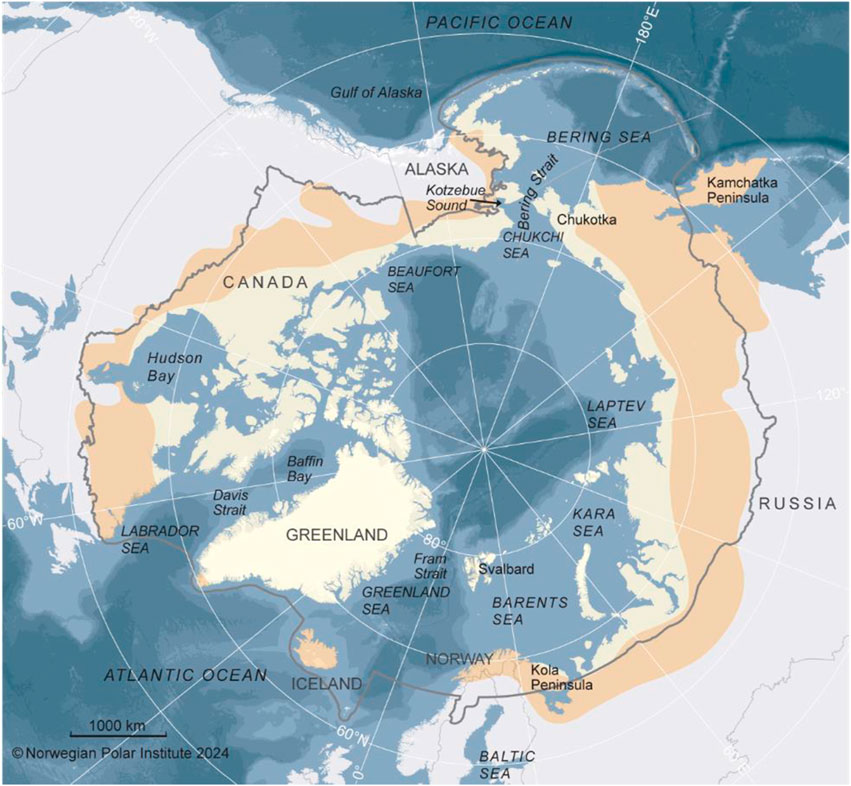
Figure 1. The Circumpolar study region, showing the CAFF boundaries, which include Arctic (pale yellow shading) and Subarctic (light brown shading) regions.
In the High Arctic, the deep (mean of ∼3,000 m) Central Arctic Ocean (∼3.3 million km2) borders five of seven Arctic Marine Areas (AMAs) as defined by the Circumpolar Marine Biodiversity Monitoring Program (CAFF, 2017). The Arctic Ocean is bordered by six countries, with the largest basins adjacent to Russia (Eurasian Basin) and Canada (Amerasian Basin). Warm, saline water flows from lower latitudes into the Arctic via two major “gateways.” In the Pacific, the narrow (∼82 km wide) and shallow (90 m) Bering Strait has inflow from the Bering Sea, with the nutrient-rich Anadyr Current on the west and the warmer, fresher Alaska Coastal Current on the east, merging with Bering Shelf Water through Bering Strait and diverging across the shallow (∼80 m) Chukchi Shelf (Weingartner et al., 1999). The Chukchi shelf slope is also influenced by the eastward-flowing deep Atlantic water of the Arctic Basin and the westward-flowing Beaufort Gyre. The Atlantic gateway is the wide (∼450 km) and deep (5,500 m) Fram Strait, with the relatively warm West Spitsbergen Current as the primary inflow. It continues eastwards along the shelf north of Svalbard as the Atlantic Water Boundary Current (Renner et al., 2018) into the Nansen Basin of the Arctic Ocean. The Fram Strait is also a primary outflow on the west side of the Atlantic with the colder and fresher East Greenland Current (Karpouzoglou et al., 2024). Large river systems drain into the Arctic Ocean from multiple points, especially the large Russian rivers, resulting in fresher, cooler waters near coastal areas, which influence coastal currents (Feng et al., 2021).
Throughout the circumpolar Arctic and Subarctic, seasonal and long-term sea ice have been important structural components of marine ecosystems, which have been changing dramatically in recent decades (Taylor et al., 2022; IPCC et al., 2023). Historically, seasonal sea-ice formation expanded from November to March. On the Pacific side, sea ice could extend south across the central Bering Sea shelf and retract in late spring, and the Bering Strait would not be ice-free until June (Stabeno and Bell, 2019). As seasonal sea ice retreated, a layer of cool, fresh water influenced water masses, currents, and productivity (Lin et al., 2019; Danielson et al., 2020). The Atlantic Arctic is changing more rapidly than any other Arctic area. The region is heavily influenced by mixed Atlantic Water entering the Barents Sea from the south in two currents–one that goes eastward into the southern Barents Sea and one that flows north along the west coast of Svalbard (Ingvaldsen et al., 2021). The volume and temperature of these currents have changed over recent decades, altering sea-ice conditions at high latitudes dramatically (Efstathiou et al., 2022; Isaksen et al., 2022; Polyakov et al., 2023). In recent decades, sea-ice loss has drastically altered the physical and biological properties of Arctic marine systems (Fossheim et al., 2015; Descamps et al., 2017; Baker et al., 2020; Danielson et al., 2020). The open water season in the gateway areas currently extends from May to December (Stabeno and Bell, 2019; Efstathiou et al., 2022; Isaksen et al., 2022; Urbanski and Litwicka, 2022).
2.2 Review process
Our review of Arctic marine ecology as related to migration includes fishes, seabirds, and marine mammals, with expert representation from both the Pacific and Atlantic Arctic sectors. We included sources published in scientific journals, books, and publicly accessible agency reports. We relied on the expertise of the team and used internet search engines, including Google Scholar and Web of Science to survey the literature. Key words searched included taxa-specific names, plus combinations of: climate change, Arctic, migration, global warming impacts, timing of migrations, distributional shift, phenology, and movement patterns.
3 Results
3.1 Anadromous and marine fishes
3.1.1 Life histories and migrations
The migration of fishes is typically a “migration triangle,” as they move seasonally or in life stages among nursery, feeding, and spawning grounds (Harden-Jones, 1968). In Arctic and Subarctic regions, migratory fishes consist of two general types: anadromous and strictly marine. Anadromous fishes hatch in fresh water, migrate to the ocean, and return to reproduce in freshwater; individuals of some species perform multiple seasonal migrations during their lives (Hendry et al., 2004). In contrast, strictly marine fishes complete their entire life cycle in the ocean. Marine migrations, the focus of this review (i.e., freshwater stages of anadromous fishes are not considered), are influenced by a myriad of factors, including water temperature, ice phenology, ice extent, wind direction, and primary and secondary production. Changes in these factors can cause changes in timing and spatial extent of fish migrations. Because of their different life histories and migratory patterns, and their relative importance to subsistence (predominantly anadromous) versus commercial fisheries (predominantly marine) in the north, we address anadromous and marine fishes separately.
The most abundant anadromous fishes in the Arctic are from the sub-family Salmoninae (Pacific salmon species Oncorhynchus spp., Atlantic salmon and sea trout Salmo spp., and various char Salvelinus spp.) and members of the Coregoninae (whitefishes and ciscoes Coregonus spp.) (Reist et al., 2006). They contribute to a variety of fisheries, including subsistence, commercial, and recreational in North America and Eurasia (e.g., Falardeau et al., 2022). Collectively, salmonids exhibit a great diversity in life-history characteristics including semelparous (single) or iteroparous (multiple) reproductive cycles in a lifetime, facultative or obligatory migration, partial migration, and phenotypic plasticity (Hendry and Sterns, 2004). Species that have received relatively more attention on how climate change may affect migration are Atlantic salmon (Salmo salar), Arctic char (Salvelinus alpinus), and brown trout (Salmo trutta), while coregonines and endemic populations of Pacific salmon north of the Bering Strait have received less research attention.
Marine fish in the Arctic consist of two main categories with respect to migratory behavior: Arctic resident species and boreal species. Arctic residents comprise a diverse fish community living in environments characterized by low temperatures and strong seasonality in photoperiod and sea-ice cover. They typically have limited migrations within Arctic waters, as they move between spawning, feeding, and overwintering areas. Most Arctic marine species are small (<35 cm) benthic forms, dominated by sculpins (Cottoidea), eelpouts (Zoarcidae), and snailfishes (Liparidae), that typically have large demersal eggs and benthic larvae or short pelagic larval phases. Thus, they generally have poor dispersal potentials and their seasonal migrations are local, often between shallow and deeper waters (Brand and Fischer, 2016). However, there are exceptions, such as the Greenland shark (Somniosus microcephalus), which can reach a length of 5.5 m (Lynghammar et al., 2024). Boreal species’ main centers of distributions occur farther south and they undertake seasonal migrations to the Arctic to feed, typically arriving in early summer and leaving before freeze-up (Lynghammar et al., 2024). Such migrants include both pelagic and demersal species. The migration timing of Arctic marine fish is closely linked to seasonal changes in the environment, particularly temperature and sea-ice conditions, and varies among species based on their specific life history traits and ecological requirements. However, for marine fish there is no information on temporal changes in migration. Most data are based on trawling, typically performed annually rather than seasonally, which reveals information on changes in distribution over time rather than migration timing (e.g., Fossheim et al., 2015; Frainer et al., 2017; Levine et al., 2023). Distributions are generally shifting farther north with warming conditions, and this is more apparent for pelagic species than for demersal species; the latter tend to tolerate a wider range of thermal conditions (Baker, 2021).
3.1.2 Phenology of migration for anadromous fishes
Among iteroparous anadromous fishes, several factors affect the timing of seasonal migration, including ocean temperatures and in some cases, ice cover. Marine habitats in Arctic North America and eastern Eurasia are ice covered during winter, unlike areas affected by the North Atlantic Current that keep the northern coasts of Iceland and Norway ice-free year-round. Sea ice forms when seawater (35 ppt) freezes at −1.8°C, and while doing so, ejects colder briny water from the ice. Anadromous fishes are not known to contain antifreeze compounds (e.g., glycoproteins), and are thus generally limited to occupying water temperatures >−0.7°C (Cheng, 2009). Therefore, sea ice and cold water act as barriers to the movements of anadromous fishes. Increased air temperatures in the Arctic have induced earlier spring ice melt, which can determine when fish undertake their marine feeding migration to take advantage of seasonal ocean productivity (Cooley et al., 2020; Falardeau et al., 2022). Similarly, ice formation in the fall is now occurring later, resulting in a longer ice-free season and increased sea surface temperatures (SST). As many anadromous fish species’ movements are governed by by ice cover and water temperature (Bond and Quinn, 2013), some have responded by shifting their migration timing earlier in the spring (Kovach et al., 2013; Dempson et al., 2017) and later in the fall. Migration of char species is associated with ice presence, and some species can move below the ice in the ocean during winter and spring (Hammer et al., 2022; Smith et al., 2022), but spring coastal movements are limited by ice and are also influenced by marine temperatures (Hollins et al., 2022). Dolly Varden (Salvelinus malma) enter the ocean after land-fast ice has broken up and its marine dispersal is limited by sea-ice extent in summer (Gallagher et al., 2021).
3.1.3 Distributional shifts in anadromous fishes
Marine water temperatures influence the spatial distribution of anadromous salmonids during summer months (Mulder et al., 2020; Gallagher et al., 2021; Rikardsen et al., 2021; Nordli et al., 2023). In Arctic North America, the cold-adapted species Arctic char and Dolly Varden are surface oriented, typically occupying depths <5 m, and prefer temperatures between approximately 2°C and 8°C (Courtney et al., 2016; Harris et al., 2020; Mulder et al., 2020). Both species track their preferred isopleths in the ocean, which can shift spatially interannually, altering their marine distribution from year to year (Jensen et al., 2014; Harris et al., 2020; Gallagher et al., 2021). For example, Dolly Varden often occupy nearshore habitats in years with cooler SSTs but use more offshore areas in years with warmer SSTs (Gallagher et al., 2021). Additionally, SSTs impact estuarine use by Arctic char, as these habitats are typically occupied when the ocean is relatively cold, thus warming temperatures could decrease movement into estuaries (Harris et al., 2020). Increased water temperatures and higher productivity are thought to be related to the increasingly northward extent of migration in Subarctic and temperate species, such as observed for Pacific salmon species (Dunmall et al., 2013).
Other factors influencing the spatial extent of anadromous fish migrations include oceanographic fronts that typically have relatively high primary and secondary productivity. For example, an oceanographic front with high productivity, where the warm North Atlantic Current intersects with cold polar waters of the Northeast Atlantic Arctic, is an important feeding area for Atlantic salmon (Rikardsen et al., 2021). Similarly, Dolly Varden feed at a front in the Chukchi Sea where euphausiid abundance is high (Courtney et al., 2016). As conditions in the Arctic change, frontal boundaries may move (Lu et al., 2015), which will impact location, duration, and energetic costs of migration (Rikardsen et al., 2021). Finally, prevailing wind direction may affect juvenile fish migration. For example, the intensified strength of easterly winds in the western parts of the North American Arctic (Falardeau et al., 2023), may improve juvenile Arctic cisco (Coregonus autumnalis) dispersal towards nursery and feeding areas favorable for recruitment (Fechhelm et al., 2007; Zimmerman et al., 2013).
3.1.4 Ontogenetic and seasonal migrations of Arctic marine fishes
The few relatively large demersal species in the Arctic that extend to the Subarctic include flatfishes, such as Greenland halibut (Reinhardtius hippoglossiodes), Arctic skate (Amblyraja hyperborea) and the Greenland shark. Most flounders undertake ontogenetic migrations, moving to deeper water as they grow (Bassi et al., 2024). Older fish also move seasonally into fjords and shallow waters during the ice-free season, and into deeper water during winter (Barkley et al., 2018). In the North Atlantic, the population structure of Greenland halibut is complex, with three main components that have different migratory patterns (Vihtakari et al., 2022). In the Northeast Atlantic, the spawning area extends from Vesterålen (68° 41′N, 15° 24′E), Norway, north to Bear Island (74° 30′N, 19° 00′E). The pelagic eggs and larvae drift northward with the West Spitsbergen Current and pelagic juveniles occur north of Svalbard and in the Arctic Ocean (Ingvaldsen et al., 2023).
Arctic skates occur from moderate depths (300 m) to the lower continental slopes (2,500 m). Tagging studies indicate that their migration routes are relatively limited, generally within 30–40 km of capture sites at Baffin Island (Peklova et al., 2014). The Atlantic is the most important dispersal route for skates into the Arctic Ocean, whereas on the Pacific side, the cold bottom water in the Bering Strait restricts their dispersal into the Arctic (Lynghammar et al., 2012). Climate warming and reduction of the Bering Sea “Cold Pool” thermal barrier (bottom water ∼ −1.5°C), could facilitate increased migration of skates and other fishes from the Bering Sea through the Bering Strait into the Arctic Ocean (Mueter et al., 2021).
In contrast to skates and other benthic fishes in the Arctic, the demersal Greenland shark is the most migratory elasmobranch in the Arctic, undertaking movements that are similar in extent to the Arctic whales. The Greenland shark is capable of long-distance migrations exceeding 1,000 km, and often conducts excursions between inshore and offshore waters (Campana et al., 2015; Edwards et al., 2022). Greenland sharks typically occur in coastal fjords during ice-free periods to feed (McMeans et al., 2013) and they move offshore during the period of ice reformation (November to July). On the other side of the Arctic Ocean, the Pacific sleeper shark (Somnious pacificus) ranges from the Bering Sea throughout the deep waters in the Pacific and into the Southern Ocean (Yano et al., 2004), and this fish also undertakes seasonal migrations from onshore to offshore waters. Little is known about how climate warming will affect these sharks, except when they occur in shallower coastal waters to feed and can then be caught and outfitted with biotelemetry instruments (Lydersen et al., 2016).
Arctic pelagic fishes include the Arctic cod (Boreogadus saida) and the polar cod (Arctogadus glacialis), both of which are important forage fishes for Arctic and Subarctic predators (e.g., Welch et al., 1992, 1993; Pettitt-Wade et al., 2021). On the Pacific side, the saffron cod (Eleginus gracilis) is abundant in the western Arctic, where it plays a significant role in food webs (e.g., Crawford et al., 2015). It is more demersal than Arctic and polar cod and it has a more southerly and nearshore distribution (De Robertis et al., 2017). Seasonal movements by Arctic cod are typically inshore/shallow during summer and are associated with juvenile development, adult feeding, and pre-spawning schooling, whereas movements to deeper areas are linked to spawning and overwintering below sea ice (Hop et al., 1997; Geoffroy et al., 2011; Benoit et al., 2014; Bouchard et al., 2016). Adult polar cod may perform long seasonal movements (<200 km) within the Arctic (Kessel et al., 2017). In the eastern Barents Sea, polar cod migrate northward in May-June, following the ice edge, with the largest fish moving first (Aune et al., 2021). In the Chukchi Sea, seasonal differences in Arctic cod abundance suggest that their migration between nursery grounds as juveniles, feeding grounds as subadults, and spawning grounds as adults, is linked to ice cover and seasonal production patterns (Forster et al., 2020). Polar cod also move between inshore and offshore areas in relation to spawning, feeding, and juvenile development, respectively (Bouchard et al., 2016; Pettitt-Wade et al., 2021). Saffron cod have different seasonal movements since they migrate inshore to spawn during winter and offshore to feed during summer (Logerwell et al., 2015). This seasonal offshore movement allows them to access abundant food resources in deeper waters. The impacts of reduced sea ice on these life stage migratory movements are largely unknown.
3.1.5 Seasonal movements of boreal fish species into the Arctic
Several boreal fish species migrate seasonally to the Arctic to feed on abundant and lipid-rich prey. In the Atlantic, the main species are capelin, Atlantic cod (Gadus morhua), and haddock (Melanogrammus aeglefinus). Capelin juveniles and sub-adults undertake feeding migrations along the east coast of Greenland and in the eastern Fram Strait and in the Barents Sea over to the slopes of the Arctic Ocean (Carscadden et al., 2013). Their northern distributions overlap with Arctic cod and they compete for some of the same zooplankton food sources (Hop and Gjøsæter, 2013; McNicholl et al., 2018).
Atlantic cod perform feeding migrations to Svalbard and the northern Barents Sea to the slopes of the Arctic Ocean, where they feed on capelin, Arctic cod, and other species (Haug et al., 2017). Such migrations are part of their life history, after pelagic eggs are released at spawning sites in northern Norway and larvae drift into the Barents Sea via the Norwegian Coastal Current (Ottersen et al., 2014). Juveniles and sub-adults disperse in the feeding areas, which include the Barents Sea as well as the shelf west and north of Svalbard to the slope of the Arctic Ocean. Adults perform annual spawning migrations to the Norwegian coast in March and subsequently migrate northwards after spawning. An increasing cod stock represents more prey for marine mammals, but also competes for some of the same food sources (Bogstad et al., 2015). The more demersal haddock migrate northwards to areas also used by Atlantic cod, particularly on the western and northern coasts of Svalbard and adjacent slopes, where they predominately feed on benthos rather than fish (Landa et al., 2014).
In the Pacific, the reduced Bering Sea “Cold Pool” has facilitated the dispersal of juvenile walleye pollock (Gadus chalcogrammus) from the Bering Sea into the Chukchi Sea (Eisner et al., 2020; Grüss et al., 2021; Levine et al., 2023). This has created a migratory pattern for walleye pollock, from a source population in the northern Bering Sea, with larvae and juveniles advected into the Chukchi Sea with presumed return migration (Levine et al., 2023). However, some individuals migrate across the Arctic Ocean to the North Atlantic (Orlov et al., 2021). The same process has brought Pacific cod (Gadus macrocephalus) into the northern Bering Sea, and although juveniles have passed through the Bering Strait they are unlikely to survive beyond age-1 because of poor growth conditions for this species in the Chukchi Sea (Cooper et al., 2023).
Mackerel (Scomber scombrus) and Atlantic bluefin tuna (Thunnus thynnus) can occasionally undertake migrations to the Arctic from their more typical southern ranges, with respective spawning areas in the eastern North Atlantic and in the Mediterranean Sea (Mackenzie et al., 2014; Berge et al., 2015b). These expansions involve longer seasonal migrations to feeding areas, since their southern spawning areas have not changed. If ocean warming facilitates more efficient predatory fishes to move into the Arctic, this could cause cascading top-down effects on marine food webs.
3.2 Seabirds
3.2.1 Seabird life history patterns in circumpolar regions
For this review, we use the term “seabirds” to encompass species that spend most of their lives at sea and return to land to nest and raise young (families Procellariidae, Phalacrocoracidae, Laridae, Alcidae), as well as other marine-feeding birds that spend more time in terrestrial or freshwater habitats, and do not typically nest in colonies (families Anatidae, Gaviidae, Stercoraridae, and phalaropes [Scolopacidae]). In general, seabirds are highly mobile and have evolved to find food in a dynamic environment, with a wide range of life history traits among species. Approximately 64 seabird species use the Arctic, ∼30 of which breed only within the CAFF boundaries of the Arctic (Irons et al., 2015). Seabirds are highly philopatric to their nesting sites, becoming central place foragers while raising chicks, and are free-ranging the rest of the year. Some seabird species and most sea ducks and phalaropes stage in marine areas prior to post-breeding migration, and then typically use a “fly and forage” strategy (e.g., red phalaropes (Phalaropus fulicarius) (Saalfeld et al., 2024) and track areas with high prey densities (e.g., little auks (Alle alle) (Amélineau et al., 2018). Other species nest far south of our study area (e.g., Ardenna shearwaters) or in Subarctic regions (e.g., Aethia auklets), but migrate seasonally to the Arctic where they can forage opportunistically during their post-breeding periods.
Seabirds that breed in the Arctic display an array of migratory patterns, ranging from movements within an ocean basin to species that migrate between the Arctic and Antarctic (Arctic terns, Sterna paradisaea). Foraging behavior and diets include surface foragers and divers, zooplanktivores, piscivores, benthivores, and omnivores. It is thus difficult to generalize regarding climate change impacts on seabirds, and indeed, the variability in foraging and migratory patterns guarantees that different responses are exhibited across families and species (Cairns, 1987; Piatt et al., 2007; Woehler and Hobday, 2024).
Most seabirds cannot store large amounts of body fat and thus require close synchronization of seasonal life phases with environmental cues. In general, photoperiod is the most important cue for avian species to initiate migratory movements, breeding, and molting (Coppack and Pulido, 2004, and citations therein). Given the Arctic’s brief summer season and seasonal extremes in daylight, temperature, open water access, and subsequent prey availability, the spatio-temporal match between a seabird and its prey is critical. Although they are highly mobile, seabirds often depend on concentrated, high densities of prey for efficient foraging (Russell et al., 1999; Jahncke et al., 2005), particularly when feeding chicks (Møller et al., 2018), and prey must be near the surface or at least within species-specific diving depth range (Benoit-Bird et al., 2013).
A few seabird species rely directly on ice-associated fauna. The Mandt’s black guillemot (Cepphus grylle mandtii) in the Pacific Arctic (Divoky et al., 2016) and the ivory gull (Pagophila eburnean) (Spencer et al., 2014) and Ross’s gull (Rhodostethia rosea) in the Atlantic Arctic (Gilg et al., 2016b) are closely tied to sea ice year-round, often following the marginal ice zone. Ivory gulls also forage on sea ice, scavenging on the remains from polar bear (Ursus maritimus) or polar fox (Vulpes lagopus) kills (Karnovsky et al., 2009). The red-legged kittiwake (Rissa brevirostris), which nests on islands in the central Bering Sea, shows a strong association with sea ice-influenced waters during the winter (Orben et al., 2015a). As their current primary habitat disappears, such pagophilic species will be forced to adapt their migratory patterns in the future, if they are to survive (Spencer et al., 2014; see Case Study No.1).
3.2.2 Evidence of changes in migration routes
Few seabird surveys have been conducted in the High Arctic and little is known about the extent to which seabirds rely on cross-Arctic routes for migration between breeding and overwintering areas. However, satellite tracking of individual birds and studies utilizing tracking radar have found that such routes do exist. Alerstam et al. (2007) documented Arctic Ocean migrations that were estimated to include over 2 million birds–comprised primarily of shorebird species, but also including terns (sub-family Sternidae) and skuas (Stercorarius). Reduced sea ice could facilitate increases in cross-Arctic migration (Clairbaux et al., 2019). For income migrants (that depend on feeding en route, i.e., most seabirds), more open water increases potential access to prey, making such transits possible (McKeon et al., 2016; Briscoe et al., 2017). At least a portion of one population of black-legged kittiwake (Rissa tridactyla) has been documented to travel over the Arctic from a colony in the Barents Region to overwintering sites in the Chukchi and Bering seas, and more cross-Arctic migrations of kittiwakes could occur as sea ice declines (Ezhov et al., 2021).
We lack long-term studies that can detect changes to migratory movements of most Arctic seabird species, but tagging studies indicate extensive cross-Arctic and ice-associated migrations that could be impacted by changes to this habitat. Ivory gulls that nest in the Atlantic Arctic migrate to the Bering Sea during their non-breeding season (Gilg et al., 2010), and Ross’s gulls conduct cross-Arctic migrations in and among the Russian, Alaskan, and Canadian Arctic regions (Maftei et al., 2015; Gilg et al., 2016b), with most of the estimated world population moving westward past northern Alaska during October-November (Maftei et al., 2014). One four-decade study that combined distribution modeling and tracking data for thick-billed murres (Uria lomvia) in the Hudson Bay region, found that the greatest shift in distribution (westward and to recently ice-free waters) occurred during the fall migration period (Patterson et al., 2021).
Long-term monitoring via at-sea surveys can be used to examine seabird response to anomalously warm or ice-free conditions, perhaps a prelude to a warmer Arctic. One long-term pattern in the Pacific Arctic suggests that with longer open water seasons and increased zooplankton productivity, planktivorous seabirds have shifted post-breeding movements northward. In the eastern Chukchi Sea, zooplankton feeders such as Aethia auklets and short-tailed shearwaters (Ardenna tenuirostris) were more abundant in offshore waters during the 2000s than the local-nesting, fish-eating seabirds (alcids and larids), which had predominated in offshore waters during 1974–1995 (Gall et al., 2017). In fact, short-tailed shearwaters have gradually shifted their migratory route northward since ca. 2014 (Kuletz et al., 2020). Because the auklets and shearwaters do not nest in the region, their influx reflects a shift in their post-breeding migration, to forage on newly abundant late summer resources. However, this shift has not been a linear response to the warming Arctic; during a recent, anomalously warm period (2017–2019), substantially fewer auklets migrated into the Chukchi Sea in late summer and fall, and shearwaters altered their distribution across the Chukchi Shelf (Kuletz et al., 2024; Case Study No. 2).
Based on both satellite-tagged birds and at-sea surveys, some seabird species that nest in temperate and Subarctic regions migrate to Arctic waters for brief periods (weeks) after their breeding periods. In the North Pacific, ancient murrelets (Synthliboramphus antiquus) that breed on islands in British Columbia and the Aleutian Archipelago migrate to the northern Bering and Chukchi seas (Day et al., 2013; Gaston et al., 2017). Kittlitz’s murrelets (Brachyramphus brevirostris) nest in Subarctic coastal areas of the northern Gulf of Alaska and migrate to the Arctic post-breeding (Day et al., 2013; Piatt et al., 2021). While abundance in the Arctic for both of these (primarily piscivorous) small alcid species have varied interannually, the long-term trend shows increasing numbers during late summer and fall in the northern Bering and Chukchi seas (Day et al., 2013; Kuletz et al., 2020). These species return south in the late fall when the hours of darkness increase and formation of sea ice begins (currently, October-November).
The philopatry shown by most seabird species to their traditional breeding sites limits shifts in colony formation as a rapid response to environmental change. However, northward expansions of seabird species are occurring and shifts in migrations northward to new feeding areas during the non-breeding portion of their life cycles have occurred with climate warming. For example, models based on at-sea surveys revealed that the three albatross species (Phoebastria) that spend portions of their non-breeding season in the Gulf of Alaska and Bering Sea have increased in numbers and two of those species have shifted their center of distribution northward in the Bering Sea (Kuletz et al., 2014). These changes indicate new migratory and overwintering patterns because the albatrosses breed on islands in the tropical North Pacific. The locally-breeding northern fulmar (Fulmarus glacialis) has also shown a northward shift in its center of distribution in the Bering Sea (Renner et al., 2013), but there is so far no evidence of a shift in breeding distribution. However, in the Atlantic Arctic, a northward shift in breeding has been documented with the establishment of northern gannets (Morus bassanus) on the northernmost coasts of Norway and Russia, and as far north as the Bear Island (in 2011), a major northward extension of their breeding distribution. These new colonies in the Arctic are associated with the northward movement of the gannet’s prey species, such as herring (Clupea harengus) and mackerel, as the Barents Sea is warming (Barrett et al., 2017).
3.2.3 Evidence of changes in stop-over and wintering areas
Migration strategies include the locations of stop-over, staging, and over-wintering areas (Baurer et al., 2008; Newton, 2008), and observations indicate that these portions of the migratory cycle are also undergoing climate-related changes. For example, Steller’s eiders (Polysticta stelleri) have shifted their main wintering areas from the Baltic Sea to the White Sea, as the latter has become increasingly ice-free in winter (Aarvak et al., 2013).
Documented shifts in the distribution of molting spectacled eiders (Somateria fischeri) in the Bering Sea between the 1990’s and ca. 2010 could not be correlated with shifts in the distribution of benthic prey or other environmental variables (Sexson et al., 2016). Although the eiders did not change their wintering areas in response to changes in ice conditions, eider survival rates were lowest at either extreme in ice conditions (i.e., having no ice for resting, or lack of open water for foraging) (Christie et al., 2018). In the northern Gulf of Alaska, benthic feeding sea ducks seem to be more resilient to changes in environmental conditions than pelagic-feeding seabirds, highlighting the importance of considering trophic interactions when modeling responses to climate change (Cushing et al., 2018; Robinson et al., 2024).
Some species exhibit long stopovers, sometimes for molting, prior to movements to overwintering sites (Mosbech et al., 2006; Frederiksen et al., 2012; Fort et al., 2013). For example, red-legged kittiwakes leave their central Bering Sea island colonies and move to the western Bering Sea in the fall, before relocating to the southwestern Bering Sea near Kamchatka and Japan in late winter (Drummond et al., 2021). Migration patterns can differ between closely related species (e.g., kittiwakes, Orben et al., 2015a; murres, Takahashi et al., 2021; and puffins, Shoji et al., 2023), and between sexes (e.g., murres, Mosbech et al., 2006; Frederiksen et al., 2016; Huffeldt et al., 2024; Ross’s gulls, Gilg et al., 2016b). Both tagging studies and at-sea surveys indicate that interannual variability in spatial distribution during migration is common (e.g., short-tailed shearwaters, Yamamoto et al., 2015; black-legged kittiwakes, Orben et al., 2015b; red phalaropes, Saalfeld et al., 2024). Thick-billed murres in Hudson Bay, Canada, have adjusted timing of departure in fall and arrival in spring as the open-water season has become extended by nearly 3 weeks, and modeling indicates that overwintering sites have shifted 211 km west and 50 km north per decade (Patterson et al., 2021). Behavioral flexibility during the non-breeding season suggests that while changes in sea ice may be a key feature in the High Arctic and can influence actively migrating seabirds, birds may not alter use of overwintering areas in Subarctic regions based solely on ice conditions (e.g., red-legged kittiwakes in the Bering Sea; Orben et al., 2018).
3.2.4 Energetic aspects of climate related changes in migration
Pre-breeding and post-breeding migrations by seabirds often include the need to replenish fat reserves lost during winter or breeding, respectively, or to fuel molting (Markones et al., 2010; Fort et al., 2013). These energetic needs require most seabirds to be “income migrants,” taking advantage of concentrations of prey along their migratory paths. However, short-tailed shearwaters make few foraging stops when migrating from the Arctic back to their Australian breeding grounds (Woehler and Hobday, 2024), and the energetic costs of extending the Arctic portion of their non-breeding route (Kuletz et al., 2020; 2024) could have implications for their survival and reproductive success (Glencross et al., 2021).
3.3 Marine mammals
The Arctic marine mammal community consists of 11 resident Arctic endemic species and ca. 25 seasonally-resident species that migrate into Arctic waters to take advantage of seasonally productive foraging opportunities during the open-water period. Climate change is impacting endemic Arctic marine mammals via both direct alterations to their habitats and indirectly via ecosystem change, as well as changing patterns of human activity (e.g., Kovacs and Lydersen, 2008; Kovacs et al., 2011; 2012; 2021; Gilg et al., 2012; George et al., 2015; Laidre et al., 2015; Descamps et al., 2017; Halliday et al., 2022). Many seasonal migrants are benefitting from longer open-water seasons and are tracking northward shifts of their prey species. Northward shifts in range to track optimal environmental conditions are a predicted response to climate change for Arctic marine mammals, which are highly mobile, large animals (Gilg et al., 2012). The ongoing climate changes are influencing movement patterns on both large and small scales.
3.3.1 Shifts in phenology of Arctic endemic species
Variability in the timing of migration, induced by global warming, has been recorded for many marine mammal species, including Arctic endemics. Most populations of bowhead whales (Balaena mysticetus) migrate from key foraging areas, where they have access to seasonally abundant zooplankton prey during the polar summer and fall, to habitats used for mating in winter and calving in spring. Migration timing is linked to the northward sea-ice retreat in spring and the southward sea-ice expansion in the fall for most populations of bowhead whales (but see Lydersen et al., 2012). In recent years, variability in timing of migration has been linked to variability in the timing of the open water season (Druckenmiller et al., 2018). Phenological changes with both earlier migration in the spring (Noongwook et al., 2007; Huntington et al., 2021; Szesciorka and Stafford, 2023) and later migrations in fall (Stafford et al., 2021; Szesciorka et al., 2024) have been documented in recent decades in the Pacific Arctic, where acoustical time-series data extend over decades and multi-generational Indigenous Knowledge has been documented. Sea surface temperatures have been suggested as one potential cue triggering (or delaying) migration (Tsjuii et al., 2021), along with changes in sea-ice concentrations (Ferguson et al., 2010). Some individual bowheads have ceased migration entirely, remaining in their summering grounds in the Amundsen Gulf and in the eastern Beaufort Sea (Insley et al., 2021; Diogou et al., 2023) and in Cumberland Sound (Fortune et al., 2020), although this pattern may have existed undetected prior to telemetry studies.
Many populations of beluga whales (Delphinapterus leucas) undertake cyclical annual migrations, moving between summering grounds and offshore wintering areas, which are often within drift-ice areas or near ice edges. Large beluga whale populations that occupy High Arctic areas tend to migrate greater distances relative to small populations, which have relatively localized regional movements (Luque and Ferguson, 2009; McGuire et al., 2020; Lydersen and Kovacs, 2021). Tagging data from 1998 to 2002 and 2007–2012 indicated that during the latter period (which had less sea ice and a later freeze-up), Chukchi Sea beluga whales delayed fall migration by 2 to >4 weeks (Hauser et al., 2017).
Most narwhal (Monodon monoceros) populations aggregate in predictable coastal areas during summer and then migrate to ice-covered areas offshore before the onset of heavy ice formation. Kenyon et al. (2018) and Shuert et al. (2022) documented significant delays in the timing of fall migrations using tracking data that extend over two decades in the Canadian Arctic; these time frames match climate-driven rates of sea-ice loss for the region.
Few long-term tracking studies exist for Arctic endemic seals, although some species undertake extensive migrations. The Atlantic drift-ice seals, harp seals (Pagophilus groenlandicus) and hooded seals (Cystophora cristata), breed in spring in southern parts of their ranges, but migrate northward to molt and to forage throughout the summer in Arctic waters, before returning south again in fall (see Vacquié-Garcia et al., 2024). Hooded seals have undergone significant changes in phenology of their movements, with earlier departures north and delayed departures southward (see Case Study No. 3), resulting in an extended period of Arctic residency. Summer foraging migrations of ringed seals (Pusa hispida) to preferred sea-ice concentrations start at similar times compared to the past, but now require longer travel distances compared to decades ago (Freitas et al., 2008; Lone et al., 2019; Ogloff et al., 2021).
Most Pacific walruses (Odobenus rosmarus divergens) follow seasonal migration patterns between southern wintering areas and northern summering areas. Sea-ice changes in the Pacific Arctic have resulted in changes in the phenology of migration, with walruses arriving earlier and staying later in their Chukchi Sea summering areas during 2008–2011, compared to past decades, until they return south to the Bering Sea following the expansion of winter sea ice (Jay et al., 2012).
Female polar bears generally migrate between foraging areas on offshore sea ice during summer and denning areas on land for the winter, and landfast sea ice is an important spring foraging area for mothers with young-of-the-year cubs (Rode et al., 2015). However, there are exceptions to these patterns, e.g., denning occurs on the sea ice in the Beaufort Sea, and a proportion of the Barents Sea polar bear population remains ashore year-round (Aars et al., 2017; Rode et al., 2022). It is likely that some immature or adult male bears remain in offshore habitats year-round, but tracking data is lacking for these groups of bears. Reductions in sea ice are resulting in phenological changes to the movement patterns of bears, with some moving onto land earlier in the fall and departing later in the spring (Rode et al., 2015; Escajeda et al., 2018; Laidre et al., 2018; Atwood et al., 2021).
3.3.2 Shifts in phenology of seasonal migrants
Based on passive acoustic monitoring (2012–2019), gray whales (Eschrichtius robustus) have shown little change in spring arrival timing in the Pacific Arctic, but they have been departing the northern Bering Sea and southern Chukchi Sea earlier each year since 2016; it is uncertain whether this is a major phenological change or whether it is simply an east-west range shift away from the recorder sites (Moore et al., 2022). Other seasonal migrant cetaceans, including blue (Balaenoptera musculus), fin (Balaenoptera physalus), humpback (Megaptera novaeangliae), minke (B. acutorostrata), sperm (Physeter macrocephalus), and killer whales (Orcina orca) arrive earlier to high latitudes, and depart later in the northeast Atlantic Arctic (Storrie et al., 2018; Bengtsson et al., 2022; Stafford et al., 2022; 2024). Some species (e.g., fin whales) that were summer residents in the past are currently detected acoustically at high latitudes year-round (Ahonen et al., 2021). Killer whales have extended their season of residence both in the Canadian High Arctic and in the Pacific Arctic (Higdon et al., 2014; Stafford, 2019), indicative of expanded migratory routes and timing, with evidence that some killer whales traveled to the Arctic from tropical waters (Matthews et al., 2020; 2024).
3.3.3 Shifts in distribution of Arctic endemic species
Bowhead whales in the Bering-Chukchi-Beaufort region have wintered farther north into the Chukchi Sea during 2018 and 2019, away from their typical winter home range in the western Bering Sea, which was devoid of sea ice those years (Citta et al., 2023). Aerial survey data indicate a westward shift (closer to Point Barrow) during fall migration of bowheads during 1997–2014, compared to 1982–1996, which corresponds to a shift closer to shore with more open water in recent years (Druckenmiller et al., 2018). In West Greenland, bowhead whale movements away from traditional summering areas in Disko Bay have occurred with the animals shifting southward, tracking SST patterns, with the whales specifically targeting a narrow range of temperatures, between −0.5°C and 2°C (Chambault et al., 2018).
Shifting of migratory pathways to reach new areas has been documented for narwhals that formerly resided in Eclipse Sound in the eastern Canadian Arctic, but now travel to Admiralty Inlet and Prince Regent Inlet (Kenyon et al., 2018). High levels of human disturbance associated with heavy vessel traffic servicing an industrial mine (Witting, 2023), as well as killer whale predation, have been suggested as the principle causes of this change (Lefort et al., 2020). Influx of warm Atlantic water (with associated boreal prey) has changed the coastal distribution of belugas on the west side of Svalbard. Previously, belugas in this area foraged on Arctic cod almost exclusively at tidewater glacier fronts and migrated between such areas along the coastlines (Lydersen et al., 2001; 2014; Vacquié-Garcia et al., 2018). However, they now spend much more time away from shore in the fjords, occupying different water masses, presumably to feed on small boreal fish species (Hamilton et al., 2019). In contrast, belugas in the Pacific Arctic have not significantly shifted their habitats despite loss of sea-ice cover during 1990–2014 (Hauser et al., 2018a).
Changes in sea-ice distribution (mainly, summer sea-ice edges occurring farther north) have resulted in much longer migrations for ringed seals in the Barents Region, as well as changes in their foraging behavior (Hamilton et al., 2015). Young animals must travel farther north to reach sea ice and adult ringed seals that stay in coastal areas around Svalbard now have very restricted movement patterns compared to a few decades ago, with small home ranges, where they show increased foraging effort (Hamilton et al., 2016; 2019).
Previously, when sea ice remained throughout the year over the shallow shelf of the Pacific Arctic, female walruses and young animals remained year-round near the sea ice, which serves as a haul-out platform. With the retreat of the sea ice northward over the deep Arctic Ocean, females and young now move to land-based haul-out sites along the coasts of Alaska and Russia between feeding trips, where male walruses also gather (Jay et al., 2012). Disturbances at these mixed-sex, high-density haul-outs have resulted in increased calf mortality from stampedes, at levels that can negatively impact population demographics (Udevitz et al., 2012).
Polar bear migrations have tracked sea-ice reduction in some parts of the species’ range. In Svalbard, bears no longer migrate south to islands (e.g., Hopen Island) that were previously key sites for denning, because sea ice no longer forms this far south in the late fall-early winter (Andersen et al., 2012). Female bears leave the coastal sea ice in early summer and travel on shore, feeding at ground nesting bird colonies along the west coast (Hamilton et al., 2017; Bengtsson et al., 2021). Olson et al. (2017) have documented a trend toward less denning on sea ice and more denning on land for the polar bear population in the southern Beaufort Sea. In this region, declines in sea ice have resulted in divergent movement patterns, with most of the subpopulation remaining on sea ice during summer, though some animals remain on land. The bears on ice have increased their home range sizes and migrate longer distances, while bears on land exhibit vastly smaller (88% less) annual utilization distributions (Pagano et al., 2021). Similarly, the Chukchi Sea polar bears are moving onto land for longer time periods due to reduced sea-ice cover (Rode et al., 2015; 2022). In Baffin Bay in the 1990s, summer offshore sea ice allowed polar bears to move widely, but its disappearance in the 2000s led to reduced movement rates (Laidre et al., 2018). During this time, adult females favored lower sea-ice concentrations when it provided access to the continental shelf and delayed den entry accounting for shorter denning durations (Escajeda et al., 2018). In Hudson Bay, polar bears are spending more time on sea ice that is distributed farther north than in the past (Miller et al., 2022), with their migrations to and from coastal areas being tightly coupled to sea-ice concentrations and fragmentation (Biddlecombe et al., 2021). Migrations involve significantly more swimming time for polar bears in areas where sea ice has declined, which has energetic implications for individuals (Pilfold et al., 2017).
3.3.4 Shifts in distribution of seasonal migrants
Northward shifts in migration endpoints (seasonal distributions) for many migratory cetaceans including blue, fin, humpback, minke, killer, and sperm whales have been documented over recent decades (Brower et al., 2018; Storrie et al., 2018; Stafford, 2019; Stafford et al., 2022; Lydersen et al., 2020; Ahonen et al., 2021; Bengtsson et al., 2022; Heide-Jørgensen et al., 2022; Pöyhönen et al., 2024). The Nunavummiut, Inuit residents of Nunavut, have observed an increase in killer whales in Nunavut waters associated with reductions in sea ice and changing movement patterns of other marine mammals that killer whales prey upon (Higdon et al., 2014). Increasing presence of sperm whales and northern bottlenose whales (Hyperoodon ampullatus) has been observed in Baffin Bay, coincident with the longer open-water season and expanded northward migratory routes (Posdaljian et al., 2022; Davidson et al., 2023). Harbor porpoises (Phocoena phocoena) have been detected in mid-winter in Kotzebue Sound in the Chukchi Sea, which likely reflects a recent northward extension of their migration patterns (Whiting et al., 2020).
4 Discussion
The decline in area, thickness, and seasonal duration of sea ice within the last 20 years has greatly altered the Arctic ecosystem, and this trajectory is predicted to continue as northern-latitude temperatures increase (Barber et al., 2015; Hunt et al., 2016; Ballinger and Overland, 2022; IPCC et al., 2023), due to anthropogenic carbon emissions (Kumar et al., 2020; Carvalho et al., 2021). The biological importance of the marginal ice zone (MIZ), or ice edge, to Arctic vertebrates has long been recognized (Divoky, 1977; Bradstreet and Cross, 1982; Hunt et al., 1996; Stirling, 1997). For many Arctic upper trophic level animals, their migratory timing and distribution track the MIZ because the mix of open water and ice-associated primary productivity support prey from invertebrates to forage fishes, and from benthic to pelagic food webs (Bradstreet and Cross, 1982; Kędra et al., 2015; Axler et al., 2023). As the MIZ has retreated earlier in spring and moved farther north (Perrette et al., 2011), it has potential to impact migratory movements at multiple trophic levels. Although we lack historic data on the migration patterns of many Arctic species, there is mounting evidence that the rapid changes occurring in the Arctic, particularly during spring through fall, have already resulted in changes in marine vertebrate migrations.
Climate change continues to alter open water patterns, sea-ice habitat and associated prey, timing of prey availability, and accessibility of foraging and travel routes. Changes in the temporal and spatial characteristics of habitat and prey in these circumpolar regions will alter species composition, biodiversity, and food web characteristics. The reduction in sea ice will also influence human activities, with increases in vessel traffic, oil, gas, and mining exploration and extraction, and coastal construction being likely in many areas, introducing artificial light sources, ocean noise, and risks of collisions that can impact migratory behaviors of some species (Reeves et al., 2014; Ivanova et al., 2020; Gjerdrum et al., 2021; Burt et al., 2023; Witting, 2023).
Sea-ice cover influences basic marine productivity (Arrigo et al., 2008) and access to prey (Benoit et al., 2010), and therefore affects both temporal and spatial aspects of migration. However, because of the extreme seasonal patterns of daylight in the Arctic, the temporal boundaries on primary and secondary production likely restrain the effectiveness of phenological responses of marine vertebrates to environmental change, especially for species that breed in the Arctic. Adaptation will thus often require alterations in spatial patterns of migration. The extent to which upper trophic level taxa can adjust their phenologies, habitat use, prey type, and spatial distribution will determine their resilience to ecosystem changes brought about by climate change. Where data are available, the emerging pattern among Arctic vertebrates is to change both spatial routing and temporal duration of migrations.
4.1 Influence of ecological barriers and bridges on migratory routes and ecosystems
Sea-ice losses remove an “ecological barrier” for some marine animals, as more open water creates “ecological bridges” across the Arctic, with more access to prey and travel corridors (Heide-Jørgensen et al., 2011; McKeon et al., 2016; Briscoe et al., 2017). Less ice in the Central Arctic Ocean will also open up new and energetically favorable migration routes for birds (Clairbaux et al., 2019). Shifting ranges northward to track optimal environmental conditions is a predicted response to climate change for Arctic animals, particularly for highly mobile, large-bodied marine mammals (Gilg et al., 2012). Consequently, changes in migratory patterns functionally result in shifts in ranges for these animals. Northward shifts by invertebrates and fish are also likely to play a role in changes in other marine animal distributions, although predation, competition, anthropogenic noise, and other human activities might limit geographic adjustments for some species (Gilg et al., 2012; Reeves et al., 2014; Blackwell et al., 2015; Matthews et al., 2020a; ; 2020b; Halliday et al., 2022).
Northward expansions of Subarctic and temperate marine vertebrates in response to global changes (referred to as borealization, or Atlantification and Pacification), are difficult to separate from changes in migration, and in some cases may just be a matter of semantics. Clearly, migratory pathways have shifted northward or otherwise changed to track favorable habitats (e.g., glacial fjords, or shoals that entrap ice during summer), which effectively becomes a range expansion or contraction. Ectothermic fishes are particularly responsive to temperature, and warmer water temperatures have led to northward expansions of both anadromous and marine species (Dunmall et al., 2024; Haug et al., 2017; Baker, 2021). Warm phases and marine heatwaves can facilitate northward excursions by species such as juvenile Chinook salmon (Oncorhynchus tshawytscha) that have moved north in the Bering Sea (Murphy et al., 2017) or sub-adult Chinook salmon from the Pacific Northwest occurring in the Bering Sea (Guthrie et al., 2021). The massive and unprecedented influx of juvenile walleye pollock and Pacific cod into the Chukchi Sea during the 2017–2019 warm phase precipitated ecosystem-wide impacts (Ballinger and Overland, 2022; Cooper et al., 2023; Levine et al., 2023; Kuletz et al., 2024; Case Study No. 2). Conceivably, such shifts in seasonal migratory patterns and accompanying ecosystem impacts, could become permanent.
The retraction of sea ice also removes essential habitat for some animals and intensifies spatial overlap of species. For example, declines in landfast ice in fjords and along Arctic coasts have increased seasonal mixes of Arctic and Subarctic fish species (Watt et al., 2016; Vihtakari et al., 2018), as well as avian and mammalian species (Higdon et al., 2014; Madsen et al., 2019; Stafford et al., 2022). Ice concentration, wind speed, and wind direction can interact to affect migratory movements; for example, polar bears in Hudson Bay move onto land when wind conditions and temperatures result in early seasonal ice breakup (Bohart et al., 2021). In the western Beaufort Sea, bowhead whales and beluga aggregate where and when favorable wind and current conditions tend to concentrate euphausiids and fish (Ashjian et al., 2010; Okkonen et al., 2011; Stafford et al., 2013). Under similar conditions, aggregations are seen for bowhead whales at Arctic hotspots in Russia, Canada, and Greenland (Citta et al., 2015; Banas et al., 2021). In the Chukchi Sea, such episodic conditions can create hotspots of foraging for seabirds and marine mammals during the late summer and fall migration period (Kuletz et al., 2015), which may influence timing and spatial patterns of migration.
Recently documented overlaps due to new migratory movements indicate disruptions in food webs and altered predator-prey relationships, such as the competition for prey between Atlantic cod and marine mammals in the Barents Sea (Bogstad et al., 2015). Increased numbers of Subarctic species can result in greater competition (e.g., diet overlap), potentially causing declines in endemic populations and affecting ecosystem structure, as postulated for Arctic cod in the Atlantic Arctic, where Subarctic fish species have encroached (Renaud et al., 2012; Christiansen, 2017). While new prey species may be available to predators, the nutrient quality vs capture effort may not be comparable. For example, Subarctic fishes often contain less lipids than Arctic species, or can be an unpredictable food source due to variable abundance, reducing their energetic value to − or predictability for − seabirds and marine mammals (Österblom et al., 2008; Gaston et al., 2009; Hop and Gjøsæter, 2013; Descamps and Strøm, 2021).
While Subarctic species are expanding their distributions by altering migratory routes, the distributional ranges of some Arctic species are contracting northward to areas more influenced by sea ice, or to cold deep waters along slopes or basins of fjords (Huserbråten et al., 2019; Bonnet-Lebrun et al., 2021). As Subarctic fish species expand into the Arctic, mainly driven by increasing water temperatures and declining sea-ice distribution (Mueter et al., 2021), there is evidence of ecosystem impacts (Falk-Petersen et al., 2007; Eriksen et al., 2017). Arctic endemic fish species may reach limits in spatial adaptability when moving between breeding and non-breeding areas. The distance to summer Arctic feeding areas may limit the effectiveness of migratory adaptations, due to longer transits and new predators. For example, models for Barents Sea capelin indicate that their populations will shift east and north in summer as ocean temperatures increase, and their spawning areas could potentially also change from the Norwegian coast to include Arctic islands (Huse and Ellingsen, 2008). Increasing distances to northern ice edges are likely to become energetically limiting for some marine mammals (e.g., Freitas et al., 2008; Lydersen et al., 2014). Likewise, for seabirds that migrate to the Arctic for post-breeding replenishment, the additional distance to prey fields that have moved farther north (Kuletz et al., 2024) may no longer be energetically beneficial for those birds. When summer ice edges occur north of the shelf seas, over deep Arctic Basin waters, low productivity because of limited nutrients affects the abundance of prey species and, thus, will likely limit the northward range expansion of predators.
4.2 Timing of migratory movements and energetic implications
The cues that trigger migratory movements may vary in importance among taxa, and will affect different aspects of their life histories. Ectothermic fishes are more directly impacted by changes in water temperatures, and larval and juvenile stages are subject to passive “migratory” movements, and thus show a rapid, and sometimes dramatic response to altered environmental conditions (Langangen et al., 2016). Fish species that perform multiple lifetime migrations may be particularly vulnerable, given the multiple times they must contend with various stressors to time their migration to match pulses of marine production during spring-summer. Some endothermic long-distance migrants, such as shearwaters, may face similar issues matching movements with prey availability as they navigate between hemispheres. Shearwaters may act more as capital migrants while in the Arctic and Subarctic, by storing fat reserves for long southerly transits with minimal foraging (Woehler and Hobday, 2024). However, large die offs of shearwaters have been observed in Alaska during marine heatwave events (Baduini et al., 2001; Jones et al., 2023), suggesting that this migratory strategy may not be adequate with continued warming of northern oceans. Alternatively, animals that are restricted to a specific type of habitat during migration, or that can only move within a small area, may face even greater impacts from alterations of that habitat due to climate change.
Seabirds are highly mobile, but their breeding season restrictions mean that the greatest shift in timing and habitat use for them are the migratory periods immediately before and after breeding. Tracking data suggest that for some species, overwintering areas can generally be consistent among years (Orben et al., 2015b), although other studies indicate that individuals show flexibility and variability (Guilford et al., 2011). Photoperiod appears to be the critical element for timing of migratory movements for seabirds, even if migratory routes vary, and this may be how mated pairs that migrate and winter apart are able to synchronize their returns to the nest site (e.g., thick-billed murres, Huffeldt et al., 2024). These evolutionary-scale relationships will be strained by the rapid changes in the Arctic environment.
Many marine mammals, which can rely on body fat reserves due to their large body size, have adjusted to changing conditions by arriving earlier and remaining longer in their summer Arctic foraging areas (Hauser et al., 2017; Szesciorka et al., 2024). Some species have individuals that currently remain in High Arctic areas year-round (Insley et al., 2021), thereby increasing opportunities for exchange among populations (Heide-Jørgenson et al., 2011; Ahonen et al., 2021; Diogou et al., 2023). Changes in Arctic marine mammal migratory routes indicate a primarily northward shift to track suitable oceanographic conditions, ice-edge upwelling areas, or preferred prey (Storrie et al., 2018; Bengtsson et al., 2021; 2022; Citta et al., 2023). Reduced sea-ice conditions have apparently created a “boom time” for large whales in the Pacific Arctic in newly exposed areas (Moore, 2016). However, several Unusual Mortality Events among ice-dependent species of marine mammals in the Pacific Arctic have been linked to reductions in sea ice (Barratclough et al., 2023), highlighting variable results for different species in response to changes in a single driver.
4.3 Social and ecological impacts
Increases in ship traffic and other human activities have already been observed in the Arctic, with anticipated effects on movements of some fishes (e.g., Arctic cod, Ivanova et al., 2020), seabirds (Merkel and Johansen, 2011; Gjerdrum et al., 2021) and marine mammals (Reeves et al., 2014; Hauser et al., 2018b; Silber and Adams, 2019; Halliday et al., 2021). Another risk for endemic Arctic species is the possibility of novel pathogens arriving via Subarctic species spending more time in the Arctic (Greer et al., 2008; Barratclough et al., 2023).
For most Arctic, and many Subarctic species, limited historic and current data make forecasts regarding changes in migratory phenology and routes challenging. Changes that have occurred also impact the quality of species’ monitoring; for example, new wintering areas may be more remote than traditional sites already monitored (e.g., Fox et al., 2019), or new migration routes and stopovers are not yet discovered. Establishing monitoring programs that are adaptable to such changes will require planning for such contingencies and working with people that live in the Arctic (Kochanowicz et al., 2021; Hauser et al., 2023; Clairbaux et al., 2024).
Ongoing climate changes challenge the resilience and adaptability of Arctic people and ecosystems and will require a close coupling of adaptive monitoring and research, with ecosystem-based management of human activities at all levels, including those involved with Indigenous harvest practices (see Case Study No. 4), as recommended by the Arctic Council (Siron et al., 2008; https://pame.is/projects/ecosystem-approach). In nearshore Arctic areas, earlier ice break-up and warming air and SST impact food security and traditional cultural practices that rely on availability and access to migratory marine vertebrates (ICC-Alaska, 2015; Regehr et al., 2017; Falardeau and Bennett, 2020; Ford et al., 2021; Hauser et al., 2021; Falardeau et al., 2022; Ovitz et al., 2024). Indigenous Peoples have been adapting to changing conditions since time immemorial, yet rapid climate-driven changes require adoption of new strategies. Indigenous Knowledge and perspectives in decision-making contribute to interpretation of observed changes in animal migration and equitable solutions to Arctic change (Wong et al., 2020; Hauser et al., 2021; Yua et al., 2022). Additionally, Indigenous Knowledge provides information on long-term changes, including changes in migration phenology or evidence of population fluctuations (Mallory et al., 2003; Moore and Hauser, 2019; Clairbaux et al., 2024).
4.4 Conclusion
Unpredictability in Arctic physical and biological conditions is a key current and future challenge for marine vertebrates that rely on migration for survival and reproductive success. Fish species may respond more directly and quickly to physical changes, but many fish species are more limited in mobility than seabirds and marine mammals. Seabirds are highly mobile, but may be more restrained by photoperiod, and less able to use a capital migratory strategy because of their relatively small body sizes, thus temporal and spatial changes to prey could impede their adaptability. Marine mammals have altered their timing and spatial migratory patterns, but prey availability and new predation regimes (i.e., new and longer presence of top predators) may limit the effectiveness of altering migratory patterns. Increased human activity levels in previously ice-covered areas will also influence Arctic ecosystems, while altered animal migration routes and loss of sea ice that facilitated hunting impact Indigenous communities. Monitoring Arctic populations of fishes, seabirds, and marine mammals will require adaptive practices to track shifts in timing and distribution of these important ecosystem components. Habitat declines for ice-dependent Arctic species is a serious threat to global biodiversity, and collaboration among stakeholders is imperative to address these challenges effectively.
5 Case Studies
5.1 Case Study No.1. Ivory gull: an ice-dependent seabird
The ivory gull is probably the most Arctic of all marine birds. It is tightly linked to sea ice habitats throughout the year, where it feeds on a variety of prey ranging from marine invertebrates to seal carcasses (Gilg et al., 2016b; Spencer et al., 2016; Dumas et al., 2022). Ivory gull breeding colonies are located in the High Arctic in Canada, Greenland, and in the High Arctic archipelagos of Svalbard (Norway) and Franz Josef Land (Russia). Tracking studies and observations have shown that the main wintering areas are along ice edges in the Davis Strait and the Bering Sea (Gilg et al., 2010; Spencer et al., 2014; Frederiksen et al., 2021). Notably, ivory gulls consistently migrate along ice edges to areas south of the Arctic Circle, and overwinter where daylight remains available in mid-winter. This pattern is consistent with visual foragers that depend on sufficient light to feed. Winter sea ice is projected to retract northwards, and little ice is expected to occur south of the Arctic Circle in mid-winter by the late 21st century, particularly in the Bering Sea (DeRepentigny et al., 2020; Dörr et al., 2021). The available tracking data are insufficient to demonstrate changes in the timing or pathways of ivory gull migrations, but given the species’ close link to ice-edge habitats and the observed and expected changes in this habitat, changes are likely occurring. The retreat of the marginal ice zone favoured by the ivory gull will impact its migratory pathways, and most of the non-breeding habitat of the ivory gull is projected to disappear. Unless the ivory gull can alter its migratory patterns, such changes in the ice habitat it uses for migration and overwintering, combined with the impact of receding summer sea ice near breeding colonies, puts this iconic species at high risk of decline and possible extinction before the end of the century.
5.2 Case Study No. 2. Seabirds respond to changes in the Chukchi Sea
In the eastern Chukchi Sea of the Pacific Arctic, reduced sea ice and warmer ocean temperatures have led to longer open-water seasons, higher zooplankton abundance and increases in boreal fish species (Mueter et al., 2021). These changes have led to changes in the offshore avian community during the post-breeding period, when seabirds must replenish body reserves and prepare for winter and migrations south. Piscivorous seabirds that nest along the southern Chukchi Sea coast used to be the most abundant seabirds in offshore waters during summers (1970s–1980s), but ship-based surveys during 2007–2012 found that planktivorous seabirds had become more abundant offshore (Gall et al., 2017). The offshore increase in planktivores was primarily due to abundance increases for two species of Aethia auklets, which feed on copepods and small euphausiids, and short-tailed shearwaters, which feed on larger euphausiids and small fish; both groups migrate through the Bering Strait into the Chukchi Sea to forage on abundant prey in late summer (Kuletz et al., 2015; Gall et al., 2017). Auklets and other alcids conduct such post-breeding northward migrations from breeding sites in the Bering Sea or even the Gulf of Alaska (Kuletz et al., 2024 and references therein). For the shearwaters, the Chukchi Sea is the northernmost extent of their seasonal migration from Australian breeding grounds (Yamamoto et al., 2015).
Continued warming of the Pacific Arctic may further alter seabird migratory patterns. During a recent period (2017–2019) with multiple marine heatwave events, the Chukchi Sea had high biomass of small zooplankton, but low abundance of large nutrient-rich copepods and euphausiids (Spear et al., 2019; Kim et al., 2020). Concurrently, fish abundance was elevated, including an unprecedented movement of age-0 walleye pollock into the region (Levine et al., 2023). The lack of a thermal barrier deep in the Subarctic Bering Sea known as the “Cold Pool” allowed adult pollock to shift northward and spawn near the Bering Strait, where warmer conditions led to increased advection through the strait and facilitated juvenile pollock movement into the Chukchi Sea (Baker et al., 2020; Levine et al., 2023; Figure 2). The pollock may have provided new prey to piscivorous birds but could also have competed for zooplankton with planktivorous seabirds and other forage fishes. Concurrently, abundance of the more omnivorous short-tailed shearwater increased greatly, but they were distributed across the shallow Chukchi Shelf (Kuletz et al., 2024) rather than in previously identified hotspots (Kuletz et al., 2015). In contrast, only low numbers of auklets, which experienced breeding failures in the northern Bering Sea (Will et al., 2020), traveled to the Chukchi Sea in 2019 (Kuletz et al., 2024). For highly mobile animals like seabirds, rapid changes in migratory patterns may be possible, but there are likely energetic costs incurred in traveling farther or for longer periods.
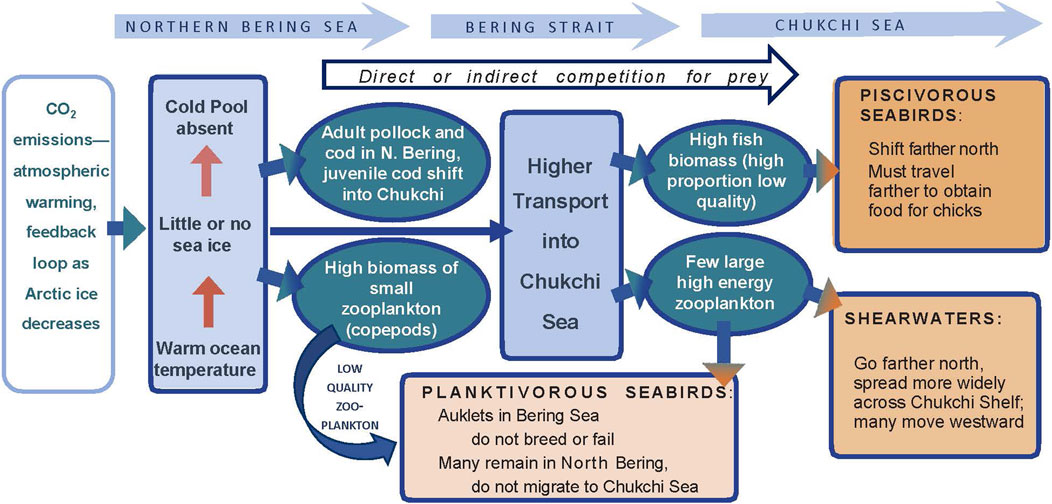
Figure 2. A conceptual model showing the physical drivers and changes in the Chukchi Sea ecosystem during a period characterized by marine heatwaves (2017–2019) and impacts to seabirds. Modified from Figure 12 in Kuletz et al. (2024).
5.3 Case Study No. 3. Climate change impacts on hooded seal migration patterns
Hooded seals are Arctic endemic, drift-ice breeding, true seals that migrate between traditional pupping areas in southern parts of their range and traditional molting sites farther north, before dispersing over vast areas where they forage during summer and fall, mostly in High Arctic waters of the North Atlantic (Andersen et al., 2009; Vacquié-Garcia et al., 2017). Climate warming has resulted in increased ocean temperatures and marked reductions in sea-ice thickness, extent, and seasonal duration across the hooded seals’ North Atlantic/Arctic range over the past three decades, with Atlantification of both physical and biological conditions (Kovacs et al., 2021). Few long-term data series are available for marine mammals that allow for exploration of how such changes influence animals’ migration patterns, but a unique 30 years-long tracking data set for hooded seals from the Northwest Atlantic allowed Vacquié-Garcia et al. (2024) to explore movement patterns in the context of climate change. They found that hooded seals from two breeding areas off the east coast of Canada targeted different environmental conditions. However, each breeding group has remained faithful in their selection of the same oceanographic conditions for their foraging activities over the whole of the study period. Despite different temperature, sea-ice concentration, depth, and salinity preferences, both populations had shifted the phenology of their migration–leaving breeding areas earlier–and also shifted their migratory endpoints to places farther north (Figure 3), to access similar oceanographic characteristics across the decades covered in the study. This northward shift is likely to continue into the future, but the limits to adaptive capacity of hooded seals with respect to the loss of traditional breeding and molting sites are unknown. Hooded seals are already experiencing markedly increased rates of polar bear predation off the east coast of Greenland, where the drift ice now occurs very close to shore compared to decades ago (McKinney et al., 2013), which has become a driver to negative populations trends for Greenland Sea hooded seals (Øigård et al., 2014).
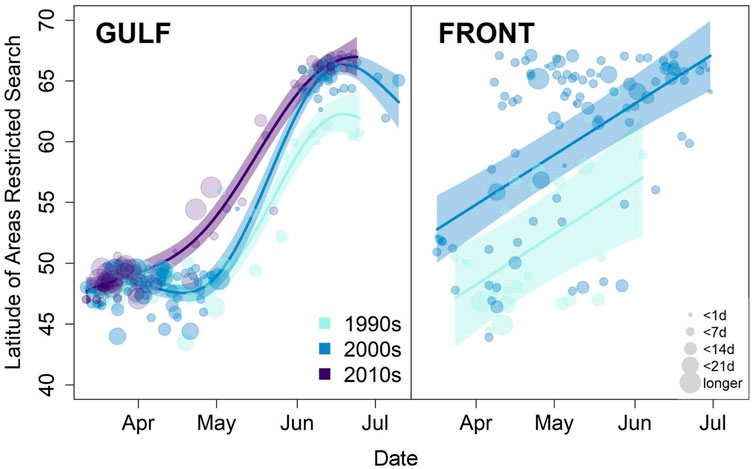
Figure 3. Foraging areas (identified along tracks by Areas Restricted Search [ARS] in hierarchical switching state-space models) for hooded seals, with respect to latitude and date, from the Gulf of St Lawrence (GULF) and the FRONT north of Newfoundland, over three decades (in colors). The size of the circles represents durations of ARSs. Solid lines and shaded areas represent the fitted estimates and their respective confidence intervals (From Vacquié-Garcia et al., 2024). Results showed earlier and more northerly locations over time (decades).
5.4 Case Study No. 4. Arctic changes impact subsistence harvests by Indigenous Peoples
Lifestyles of Indigenous coastal communities in Arctic North America, Greenland, and eastern Eurasia are closely associated with seasonal sea ice. The ability of these communities to engage in the traditional harvests on which they rely are being heavily impacted by a warming environment, largely because of changes to the spatiotemporal patterns of migratory marine mammals, seabirds, and anadromous fishes (Badjeck et al., 2010; Brewster et al., 2016; ICC-Alaska, 2015; Savo et al., 2017; Huntington et al., 2020). In western Arctic North America, Inuit harvests of seals and anadromous fishes have been impacted by climate change (Hauser et al., 2021; Falardeau et al., 2022).
Many migratory marine mammal species are fundamental to the nutritional, spiritual, and cultural aspects of Indigenous life in coastal Arctic Alaska communities (Huntington et al., 2017). These animals are dependent on sea ice and ocean conditions, and wind and weather conditions can impact the ability of hunters to safely harvest bowhead whales and walruses during the seasonal periods in which they are available (Hansen et al., 2013; Huntington et al., 2013). In Northwest Alaska, an interdisciplinary team of researchers worked with an Iñupiaq Elder Advisory Council to understand how bearded seal harvest phenology was changing relative to changing environmental conditions (Hauser et al., 2021). Bearded seals (“ugruk” in Iñupiaq), migrate into the region during spring sea ice break-up, relying on ice floes for resting between foraging bouts. This study found that the duration of harvests declined significantly as break-up shifted earlier (Figure 4); this was determined by integrating harvest records, Indigenous Knowledge on conditions impacting bearded seal spring habitat and accessibility of seals to hunters, and remotely-sensed sea-ice data. Indigenous hunters have so far been able to compensate for decreased harvesting periods by changing how they searched for and hunted seals.
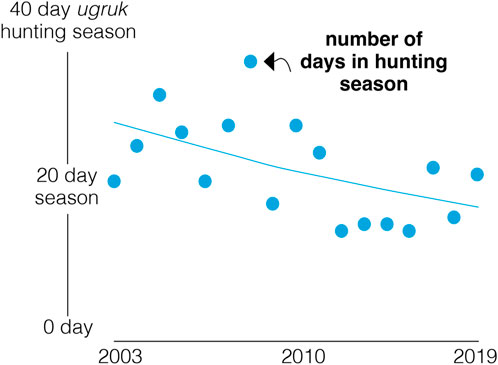
Figure 4. The length of the hunting season (2003–2019) for bearded seal (ugruk) by Iñupiaq harvesters in northwest Alaska. Over this period, the length of the harvest season has declined significantly, due to early start and end dates in correlation with earlier sea ice break-up. The hunting season now ends in mid-June rather than early July. Modified from Figure 2 in Hauser et al. (2021).
Climate change effects on fisheries for Arctic char and Dolly Varden during the open water season, and their quality as a food source for Inuit people, raise concerns pertaining to altered marine habitat use, diet changes affecting flesh quality, exposure to contaminants, and interactions with non-endemic species (Tran et al., 2019; Chila et al., 2022; Harris et al., 2022; Bolduc et al., 2024; Pearce et al., 2024). Arctic char might become harder to harvest in shore-based gill net fisheries during summer, as the char follows preferred cooler temperatures to deeper areas farther from shore (Harris et al., 2022). Nearshore habitat-use and marine spatial distribution of Dolly Varden are influenced by SST and ice conditions (Gallagher et al., 2021). The increasing loss of coastal ice during summer has impacted Dolly Varden harvests as the presence of drifting ice improves catches of fish (Brewster et al., 2016; WMAC and Aklavik HTC, 2018). Additionally, increased frequency and strength of wind and wave activity impedes the travel to coastal fishing camps and also the ability to deploy gill nets, which is further exacerbated by coastal erosion (Brewster et al., 2016; Kokelj et al., 2021; Pearce et al., 2024). The challenges posed by changes in fish habitat use, accessibility to fishing locations, and long-term changes in the fish community composition in the western Arctic (Brewster et al., 2016; Priest et al., 2022; von Biela et al., 2023) require harvesters to adjust fishing practices, which will have social and economic consequences for Indigenous communities.
Author contributions
KJK: Conceptualization, Investigation, Methodology, Project administration, Supervision, Writing–original draft, Writing–review and editing, Visualization. SF: Conceptualization, Investigation, Methodology, Writing–original draft, Writing–review and editing. MF: Conceptualization, Investigation, Methodology, Writing–original draft, Writing–review and editing. CG: Conceptualization, Investigation, Methodology, Writing–original draft, Writing–review and editing. DH: Conceptualization, Investigation, Methodology, Writing–original draft, Writing–review and editing, Visualization. HH: Conceptualization, Investigation, Methodology, Validation, Writing–original draft, Writing–review and editing. KMK: Conceptualization, Investigation, Methodology, Visualization, Writing–original draft, Writing–review and editing, Resources. CL: Conceptualization, Investigation, Methodology, Writing–original draft, Writing–review and editing. AM: Conceptualization, Investigation, Methodology, Writing–original draft, Writing–review and editing. AS: Conceptualization, Investigation, Methodology, Writing–original draft, Writing–review and editing.
Funding
The author(s) declare financial support was received for the research, authorship, and/or publication of this article. Support was provided to MF and AM by the Danish Energy Agency. Publication costs for this review were provided by the Norwegian Polar Institute.
Acknowledgments
Author’s contributions were supported by their respective agencies, including U.S. Fish and Wildlife Service (KJK), Fisheries and Oceans Canada (SHF, CG), University of Alaska Fairbanks (DDWH, AS), Aarhus University Roskilde (MF, AM) and the Norwegian Polar Institute (HH, KMK, CL). We thank Oddveig (NPI) for adapting Figure 1, Pam Odom (ABR, Inc., Fairbanks, Alaska) for adapting Figure 2, and Jade Vacquie-Garcia for alterations to Figure 3. We thank Lori Quakenbush and David Ainley for their constructive comments and edits, which greatly improved the final manuscript.
Conflict of interest
The authors declare that the research was conducted in the absence of any commercial or financial relationships that could be construed as a potential conflict of interest.
The reviewer LQ declared a past co-authorship with the authors KMK and CL to the handling editor.
Publisher’s note
All claims expressed in this article are solely those of the authors and do not necessarily represent those of their affiliated organizations, or those of the publisher, the editors and the reviewers. Any product that may be evaluated in this article, or claim that may be made by its manufacturer, is not guaranteed or endorsed by the publisher.
References
Aars, J., Marques, T. A., Lone, K., Andersen, M., Wiig, Ø., Fløystad, I. M. B., et al. (2017). The number and distribution of polar bears in the western Barents Sea. Polar Res. 36, 1374125. doi:10.1080/17518369.2017.1374125
Aarvak, T., Øien, I. J., Krasnov, Y. V., Gavrilo, M. V., and Shavykin, A. A. (2013). The European wintering population of Steller’s eider Polysticta stelleri reassessed. Bird. Conserv. Int. 23, 337–343. doi:10.1017/S0959270912000251
Ahonen, H., Stafford, K. M., Lydersen, C., Berchock, C. L., Moore, S. E., and Kovacs, K. M. (2021). Interannual variability in acoustic detection of blue and fin whale calls in the Northeast Atlantic High Arctic between 2008 and 2018. Endang. Species Res. 45, 209–224. doi:10.3354/esr01132
Alerstam, T., Bäckman, J., Gudmundsson, G. A., Hedenström, A., Henningsson, S. S., Karlsson, H., et al. (2007). A polar system of intercontinental bird migration. Proc. R. Soc. B. 274, 2523–2530. doi:10.1098/rspb.2007.0633
Alerstam, T., and Gudmundsson, G. (1999). Bird orientation at high latitudes: flight routes between Siberia and North America across the Arctic Ocean. Proc. R. Soc. B 266, 2499–2505. doi:10.1098/rspb.1999.0952
Amélineau, F., Fort, J., Mathewson, P. D., Speirs, D. C., Courbin, N., Perret, S., et al. (2018). Energyscapes and prey fields shape a North Atlantic seabird wintering hotspot under climate change. R. Soc. Open Sci. 5, 171883. doi:10.1098/rsos.171883
Andersen, J. M., Wiersma, Y. F., Stenson, G., Hammill, M. O., and Rosing-Asvid, A. (2009). Movement patterns of hooded seals (Cystophora cristata) in the northwest Atlantic Ocean during the post-moult and pre-breed seasons. J. N. Atl. Fish. Sci. 42, 1–11. doi:10.2960/J.v42.m649
Andersen, M., Derocher, A. E., Wiig, Ø., and Aars, J. (2012). Polar bear (Ursus maritimus) maternity den distribution in Svalbard, Norway. Polar Biol. 35, 499–508. doi:10.1007/s00300-011-1094-y
Arrigo, K. R., van Dijken, G., and Pabi, S. (2008). Impact of a shrinking Arctic ice cover on marine primary production. Geophys. Res. Lett. 35, L19603. doi:10.1029/2008GL035028
Ashjian, C. J., Braund, S. R., Campbell, R. G., George, J. C., Kruse, J., Maslowski, W., et al. (2010). Climate variability, oceanography, bowhead whale distribution, and Iñupiat subsistence whaling near Barrow, Alaska. Arctic 63, 179–194. doi:10.14430/arctic973
Atwood, T. C., Rode, K. D., Douglas, D. C., Simac, K., Pagano, A. M., and Bromaghin, J. E. (2021). Long-term variation in polar bear body condition and maternal investment relative to a changing environment. Glob. Ecol. Cons. 32, e01925. doi:10.1016/j.gecco.2021.e01925
Aune, M., Raskhozheva, E., Andrade, H., Augustine, S., Bambulyak, A., Camus, L., et al. (2021). Distribution and ecology of polar cod (Boreogadus saida) in the eastern Barents Sea: a review of historical literature. Mar. Environ. Res. 166, 105262. doi:10.1016/j.marenvres.2021.105262
Axler, K. E., Goldstein, E. D., Nielsen, J. M., Deary, A. L., and Duffy-Anderson, J. T. (2023). Shifts in the composition and distribution of Pacific Arctic larval fish assemblages in response to rapid ecosystem change. Glob. Change Biol. 29, 4212–4233. doi:10.1111/gcb.16721
Badjeck, M. C., Allison, E. H., Halls, A. S., and Dulvy, N. K. (2010). Impacts of climate variability and change on fishery-based livelihoods. Mar. Policy 34, 375–383. doi:10.1016/j.marpol.2009.08.007
Baduini, C. L., Lovvorn, J. R., and Hunt Jr., G. L. (2001). Determining the body condition of short-tailed shearwaters: implications for migratory flight ranges and starvation events. Mar. Ecol. Prog. Ser. 222, 265–277. doi:10.3354/meps222265
Baker, M. R. (2021). Contrast of warm and cold phases in the Bering Sea to understand spatial distributions of Arctic and sub-Arctic gadids. Polar Biol. 44, 1083–1105. doi:10.1007/s00300-021-02856-x
Baker, M. R., Kivva, K. K., Pisareva, M. N., Watson, J. T., and Selivanova, J. (2020). Shifts in the physical environment in the Pacific Arctic and implications for ecological timing and conditions. Deep Sea Res. II 177, 104802. doi:10.1016/j.dsr2.2020.104802
Ballinger, T. J., and Overland, J. E. (2022). The Alaskan Arctic regime shift since 2017: a harbinger of years to come? Polar Sci. 32, 100841. doi:10.1016/j.polar.2022.100841
Banas, N. S., Møller, E. F., Laidre, K. L., Simon, M., Ellingsen, I. H., and Nielsen, T. G. (2021). Reconciling behavioural, bioenergetic, and oceanographic views of bowhead whale predation on overwintering copepods at an Arctic hotspot (Disko Bay, Greenland). Front. Mar. Sci. 8, 614582. doi:10.3389/fmars.2021.614582
Barber, D. G., Hop, H., Mundy, C. J., Else, B., Dmitrenko, I. A., Tremblay, J. É., et al. (2015). Selected physical, biological and biogeochemical implications of a rapidly changing Arctic Marginal Ice Zone. Progr. Oceanogr. 139, 122–150. doi:10.1016/j.pocean.2015.09.003
Barkley, A. N., Hussey, N. E., Fisk, A. T., Hedges, K. J., and Treble, M. A. (2018). Transient movements of a deep-water flatfish in coastal waters: implications of inshore-offshore connectivity for fisheries management. J. Appl. Ecol. 55, 1071–1081. doi:10.1111/1365-2664.13079
Barratclough, A., Ferguson, S. H., Lydersen, C., Thomas, P. O., and Kovacs, K. M. (2023). A review of circumpolar Arctic marine mammal health – a call to action in a time of rapid environmental change. Pathogens 12, 937. doi:10.3390/pathogens12070937
Barrett, R. T., Strøm, H., and Melnikov, M. (2017). On the polar edge: the status of the northern gannet (Morus bassanus) in the Barents Sea in 2015-16. Polar Res. 366, 1390384. doi:10.1080/17518369.2017.1390384
Bassi, L., Tremblay, R., Morissette, O., and Sirois, P. (2024). Connectivity of Greenland halibut in the northwestern Atlantic Ocean inferred from otolith chemistry. Front. Mar. Sci. 10, 1282264. doi:10.3389/fmars.2023.1282264
Bauer, S., and Hoye, B. J. (2014). Migratory animals couple biodiversity and ecosystem functioning worldwide. Science 344, 1242552. doi:10.1126/science.1242552
Bauer, S., Van Dinther, M., Høgda, K. A., Klaassen, M., and Madsen, J. (2008). The consequences of climate-driven stop-over sites changes on migration schedules and fitness of Arctic geese. J. Anim. Ecol. 77, 654–660. doi:10.1111/j.1365-2656.2008.01381.x
Bengtsson, O., Hamilton, C. D., Lydersen, C., Andersen, M., and Kovacs, K. M. (2021). Distribution and habitat characteristics of pinnipeds and polar bears in the Svalbard Archipelago, 2005–2018. Polar Res. 40, 5326. doi:10.33265/polar.v40.5326
Bengtsson, O., Lydersen, C., and Kovacs, K. M. (2022). Cetacean spatial trends over time (2005-2019) in Svalbard, Norway: climate change and Svalbard's whales. Polar Res. 41, 7773. doi:10.33265/polar.v41.7773
Benoit, D., Simard, Y., and Fortier, L. (2014). Pre-winter distribution and habitat characteristics of polar cod (Boreogadus saida) in southeastern Beaufort Sea. Polar Biol. 37, 149–163. doi:10.1007/s00300-013-1419-0
Benoit, D., Simard, Y., Gagné, J., Geoffroy, M., and Fortier, L. (2010). From polar night to midnight sun: photoperiod, seal predation, and the diel vertical migrations of polar cod (Boreogadus saida) under landfast ice in the Arctic Ocean. Polar Biol. 33, 1505–1520. doi:10.1007/s00300-010-0840-x
Benoit-Bird, K. J., Battaile, B. C., Heppell, S. A., Hoover, B., Irons, D., Jones, N., et al. (2013). Prey patch patterns predict habitat use by top marine predators with diverse foraging strategies. PLoS ONE 8, e53348. doi:10.1371/journal.pone.0053348
Berge, J., Heggland, K., Lønne, O. J., Cottier, F., Hop, H., Gabrielsen, G. W., et al. (2015b). First records of Atlantic mackerel (Scomber scombrus) from the Svalbard Archipelago, Norway, with possible explanations for the extension of its distribution. Arctic 68, 54–61. doi:10.14430/arctic4455
Berge, J., Renaud, P., Darnis, G., Cottier, F., Last, K., Gabrielsen, T. M., et al. (2015a). In the dark: a review of ecosystem processes during the Arctic polar night. Prog. Oceanogr. 139, 258–271. doi:10.1016/j.pocean.2015.08.005
Biddlecombe, B. A., Bayne, E. M., Lunn, N. J., McGeachy, D., and Derocher, A. E. (2021). Effects of sea ice fragmentation on polar bear migratory movement in Hudson Bay. Mar. Ecol. Prog. Ser. 666, 231–241. doi:10.3354/meps13684
Blackwell, S. B., Nations, C. S., McDonald, T. L., Thode, A. M., Mathias, D., Kim, K. H., et al. (2015). Effects of airgun sounds on bowhead whale calling rates: evidence for two behavioral thresholds. PLoS ONE 10, e0125720. doi:10.1371/journal.pone.0125720
Bogstad, B., Gjøsæter, H., Haug, T., and Lindstrøm, U. (2015). A review of the battle for food in the Barents Sea: cod vs. marine mammals. Front. Ecol. Evol. 3, 29. doi:10.3389/fevo.2015.00029
Bohart, A. M., Lunn, N. J., Derocher, A. E., and McGeachy, D. (2021). Migration dynamics of polar bears (Ursus maritimus) in western Hudson Bay. Behav. Ecol. 32, 440–451. doi:10.1093/beheco/araa140
Bolduc, S., Lemire, M., Tremblay, J.-É., Falardeau, M., Dallaire, X., Brochu, M., et al. (2024). Assessment of inter-regional dietary differences in anadromous Arctic Char (Salvelinus alpinus) in Nunavik, Canada, and links with flesh quality indicators. Arct. Sci. 10, 372–385. doi:10.1139/AS-2023-0018
Bond, M. H., and Quinn, T. P. (2013). Patterns and influences on Dolly Varden migratory timing in the Chignik Lakes, Alaska, and comparison of populations throughout the northeastern Pacific and Arctic oceans. Can. J. Fish. Aquat. Sci. 70, 655–665. doi:10.1139/cjfas-2012-0416
Bonnet-Lebrun, A.-S., Larsen, T., Thórarinsson, T. L., Kolbeinsson, Y., Frederiksen, M., Morley, T. I., et al. (2021). Cold comfort: arctic seabirds find refugia from climate change and potential competition in marginal ice zones and fjords. Ambio 51, 345–354. doi:10.1007/s13280-021-01650-7
Bouchard, C., Mollard, S., Suzuki, K., Robert, D., and Fortier, L. (2016). Contrasting the early life histories of sympatric Arctic gadids Boreogadus saida and Arctogadus glacialis in the Canadian Beaufort Sea. Polar Biol. 39, 1005–1022. doi:10.1007/s00300-014-1617-4
Bradstreet, M. S., and Cross, W. E. (1982). Trophic relationships at high Arctic ice edges. Arctic 35, 1–12. doi:10.14430/arctic2303
Brand, M., and Fischer, P. (2016). Species composition and abundance of the shallow water fish community of Kongsfjorden, Svalbard. Polar Biol. 39, 2155–2167. doi:10.1007/s00300-016-2022-y
Brewster, J. D., Neumann, D., Ostertag, S. K., and Loseto, L. L. (2016). Traditional ecological knowledge (TEK) at shingle point, YT: observations on changes in the environment and fish populations. Can. Tech. Rep. Fish. Aquat. Sci. 3174, 23.
Briscoe, D. K., Hobday, A. J., Carlisle, A., Scales, K., Eveson, J. P., Arrizabalaga, H., et al. (2017). Ecological bridges and barriers in pelagic ecosystems. Deep Sea Res. II 140, 182–192. doi:10.1016/j.dsr2.2016.11.004
Brower, A. A., Clarke, J. T., and Ferguson, M. C. (2018). Increased sightings of subArctic cetaceans in the eastern Chukchi Sea, 2008–2016: population recovery, response to climate change, or increased survey effort? Polar Biol. 41, 1033–1039. doi:10.1007/s00300-018-2257-x
Burt, C. S., Kelly, J. F., Trankina, G. E., Silva, C. L., Khalighifar, A., Jenkins-Smith, H. C., et al. (2023). The effects of light pollution on migratory animal behavior. Trends Ecol. Evol. 38, 355–368. doi:10.1016/j.tree.2022.12.006
CAFF (2017) State of the Arctic Marine Biodiversity Report. CAFF International Secretariat: Akureyri, Iceland.
Cairns, D. K. (1987). Seabirds as indicators of marine food supplies. Biol. Oceanogr. 5, 261–271. doi:10.1080/01965581.1987.10749517
Campana, S. E., Fisk, A. T., and Kimberly, A. P. (2015). Movements of Arctic and northwest Atlantic Greenland sharks (Somniosus microcephalus) monitored with archival satellite pop-up tags suggest long-range migrations. Deep Sea Res. II 115, 109–115. doi:10.1016/j.dsr2.2013.11.001
Carmack, E., Winsor, P., and Williams, W. (2015). The contiguous panarctic Riverine Coastal Domain: a unifying concept. Progr. Oceanogr. 139, 13–23. doi:10.1016/j.pocean.2015.07.014
Carscadden, J. E., Gjøsæter, H., and Vilhjálmsson, H. (2013). A comparison of recent changes in distribution of capelin (Mallotus villosus) in the Barents Sea, around Iceland and in the Northwest Atlantic. Progr. Oceanogr. 114, 64–83. doi:10.1016/j.pocean.2013.05.005
Carvalho, K. S., Smith, T. E., and Wang, S. (2021). Bering Sea marine heatwaves: patterns, trends and connections with the Arctic. J. Hydrol. 600, 126462. doi:10.1016/j.jhydrol.2021.126462
Chambault, P., Albertsen, C. M., Patterson, T. A., Hansen, R. G., Tervo, O., Laidre, K. L., et al. (2018). Sea surface temperature predicts the movements of an Arctic cetacean: the bowhead whale. Sci. Rep. 8, 9658. doi:10.1038/s41598-018-27966-1
Cheng, C.-H. C. (2009). “Freezing avoidance in polar fishes,” in Encyclopedia of life support systems. Extremophiles. Editors C. Gerday, and N. Glansdorf (Oxford, UK: Eolss Publishers Co. Ltd), II, 215–232.
Chila, Z., Dunmall, K. M., Proverbs, T. A., and Lantz, T. C.Aklavik Hunters and Trappers Committee, Inuvik Hunters and Trappers Committee, Sachs Harbour Hunters and Trappers Committee, Olokhaktomiut, Hunters and Trappers Committee, and Paulatuk Hunters and Trappers Committee (2022). Inuvialuit knowledge of Pacific salmon range expansion in the western Canadian Arctic. Can. J. Fish. Aquat. Sci. 79, 1042–1055. doi:10.1139/cjfas-2021-0172
Christiansen, J. S. (2017). No future for Euro-Arctic ocean fishes? Mar. Ecol. Prog. Ser. 575, 217–227. doi:10.3354/meps12192
Christie, K. S., Hollmen, T. E., Flint, P., and Douglas, D. (2018). Non-linear effect of sea ice: spectacled eider survival declines at both extremes of the ice spectrum. Ecol. Evol. 8, 11808–11818. doi:10.1002/ece3.4637
Citta, J. J., Breed, G. A., Okkonen, S. R., Druckenmiller, M. L., Quakenbush, L., George, J. C., et al. (2023). Shifts in bowhead whale distribution, behavior, and condition following rapid sea ice change in the Bering Sea. Cont. Shelf Res. 256, 104959. doi:10.1016/j.csr.2023.104959
Citta, J. J., Lowry, L. F., Quakenbush, L. T., Kelly, B. P., Fischbach, A. S., London, J. M., et al. (2015). A multi-species synthesis of satellite telemetry data in the Pacific Arctic (1987–2015): overlap of marine mammal distributions and core use areas. Deep Sea Res. Part II 152, 132–153. doi:10.1016/j.dsr2.2018.02.006
Clairbaux, M., Fort, J., Mathewson, P., Porter, W., Strøm, H., and Grémillet, D. (2019). Climate change could overturn bird migration: transarctic flights and high-latitude residency in a sea ice free Arctic. Sci. Rep. 9, 17767. doi:10.1038/s41598-019-54228-5
Clairbaux, M., Rönkä, M., Anker-Nilssen, T., Artukhin, Y., Danielsen, J., Gavrilo, M., et al. (2024). An ecologically sound and participatory monitoring network for pan-Arctic seabirds. Conserv. Biol., e14287. doi:10.1111/cobi.14287
Cooley, S. W., Ryan, J. C., Smith, L. C., Horvat, C., Pearson, B., Dale, B., et al. (2020). Coldest Canadian Arctic communities face greatest reductions in shorefast sea ice. Nat. Clim. Chang. 10, 533–538. doi:10.1038/s41558-020-0757-5
Cooper, D. W., Cieciel, K., Copeman, L., Emelin, P. O., Logerwell, E., Ferm, N., et al. (2023). Pacific cod or tikhookeanskaya treska (Gadus macrocephalus) in the Chukchi Sea during recent warm years: distribution by life stage and age-0 diet and condition. Deep Sea Res. II 208, 105241. doi:10.1016/j.dsr2.2022.105241
Copeman, L. A., Salant, C. D., Stowell, M. A., Spencer, M. L., Kimmel, D. G., Pinchuk, A. I., et al. (2022). Annual and spatial variation in the condition and lipid storage of juvenile Chukchi Sea gadids during a recent period of environmental warming (2012 to 2019). Deep Sea Res. II 205, 105180. doi:10.1016/j.dsr2.2022.105180
Coppack, T., and Pulido, F. (2004). Photoperiodic response and the adaptability of avian life cycles to environmental change. Adv. Ecol. Res. 35, 131–150. doi:10.1016/S0065-2504(04)35007-5
Corkeron, P. J., and Conner, R. C. (1999). Why do baleen whales migrate? Mar. Mamm. Sci. 15, 1228–1245. doi:10.1111/j.1748-7692.1999.tb00887.x
Courtney, M. B., Scanlon, B. S., Rikardsen, A. H., and Seitz, A. C. (2016). Marine behavior and dispersal of an important subsistence fish in Arctic Alaska, the Dolly Varden. Environ. Biol. Fish. 99, 209–222. doi:10.1007/s10641-015-0468-3
Crawford, J. A., Quakenbush, L. T., and Citta, J. J. (2015). A comparison of ringed and bearded seal diet, condition and productivity between historical (1975–1984) and recent (2003–2012) periods in the Alaskan Bering and Chukchi seas. Progr. Oceanogr. 136, 133–150. doi:10.1016/j.pocean.2015.05.011
Cushing, D. A., Roby, D. D., and Irons, D. B. (2018). Patterns of distribution, abundance, and change over time in a subarctic marine bird community. Deep Sea Res. II 147, 148–163. doi:10.1016/j.dsr2.2017.07.012
Daase, M., Falk-Petersen, S., Varpe, Ø., Darnis, G., Søreide, J. E., Wold, A., et al. (2013). Timing of reproductive events in the marine copepod Calanus glacialis: a pan- Arctic perspective. Can. J. Fish. Aquat. Sci. 70, 871–884. doi:10.1139/cjfas-2012-0401
Danielson, S. L., Ahkinga, O., Ashjian, C., Basyuk, E., Cooper, L. W., Eisner, L., et al. (2020). Manifestation and consequences of warming and altered heat fluxes over the Bering and Chukchi Sea continental shelves. Deep Sea Res. II 177, 104781. doi:10.1016/j.dsr2.2020.104781
Davidson, E. R., Ferguson, S. H., Higdon, J. W., and Treble, M. A. (2023). Opportunistic sightings from fisheries surveys inform habitat suitability for northern bottlenose whales Hyperoodon ampullatus and sperm whales Physeter macrocephalus in Baffin Bay and Davis Strait, Canadian Arctic. Mar. Ecol. Prog. Ser. 723, 57–71. doi:10.3354/meps14444
Day, R. H., Gall, A. E., Morgan, T. C., Rose, J. R., Plissner, J. H., Sanzenbacher, P. M., et al. (2013). Seabirds new to the Chukchi and Beaufort seas, Alaska: response to a changing climate? West. Birds 44, 174–182.
Dempson, B., Schwarz, C. J., Bradbury, I. R., Robertson, M. J., Veinott, G., Poole, R., et al. (2017). Influence of climate and abundance on migration timing of adult Atlantic salmon (Salmo salar) among rivers in Newfoundland and Labrador. Ecol. Freshw. Fish. 26, 247–259. doi:10.1111/eff.12271
DeRepentigny, P., Jahn, A., Holland, M. M., and Smith, A. (2020). Arctic sea ice in two configurations of the CESM2 during the 20th and 21st centuries. J. Geoph. Res. Oceans 125, e2020JC016133. doi:10.1029/2020JC016133
De Robertis, A., Taylor, K., Wilson, C. D., and Farley, E. V. (2017). Abundance and distribution of Arctic cod (Boreogadus saida) and other pelagic fishes over the U.S. Continental shelf of the northern Bering and Chukchi seas. Deep Sea Res. II 135, 51–65. doi:10.1016/j.dsr2.2016.03.002
Descamps, S., Aars, J., Fuglei, E., Kovacs, K. M., Lydersen, C., Pavlova, O., et al. (2017). Climate change impacts on wildlife in a High Arctic archipelago – Svalbard, Norway. Glob. Change Biol. 23, 490–502. doi:10.1111/gcb.13381
Descamps, S., and Strøm, H. (2021). As the Arctic becomes boreal: ongoing shifts in a high-Arctic seabird community. Ecology 102, e03485. doi:10.1002/ecy.3485
Diogou, N., Halliday, W. D., Dosso, S. E., Mouy, X., Niem, A., and Insley, S. (2023). Bowhead whale year-round acoustic presence and habitat associations in the Amundsen Gulf, Western Canadian Arctic, 2018-2019. Progr. Ocean. 213, 103004. doi:10.1016/j.pocean.2023.103004
Divoky, G. J. (1977). “Sea ice as a factor in seabird distribution and ecology in the Beaufort, Chukchi and Bering Seas,” in Conservation of marine birds of northern North America. Washington, D.C.: U.S. Fish and wildlife service, wildlife research, department. Editors J. C. Bartonek, and D. N. Nettleship, 11, 9–19.
Divoky, G. J., Douglas, D. C., and Stenhouse, I. J. (2016). Arctic sea ice a major determinant in Mandt's black guillemot movement and distribution during non-breeding season. Biol. Lett. 12, 20160275. doi:10.1098/rsbl.2016.0275
Dörr, J., Årthun, M., Eldevik, T., and Madonna, E. (2021). Mechanisms of regional winter sea-ice variability in a warming Arctic. J. Clim. 34, 8635–8653. doi:10.1175/JCLI-D-21-0149.1
Druckenmiller, M. L., Citta, J. J., Ferguson, M. C., Clarke, J. T., George, J. C., and Quakenbush, L. (2018). Trends in sea-ice cover within bowhead whale habitats in the Pacific Arctic. Deep Sea Res. II 152, 95–107. doi:10.1016/j.dsr2.2017.10.017
Drummond, B. A., Orben, R. A., Christ, A. M., Fleishman, A. B., Renner, H. M., Rojek, N. A., et al. (2021). Comparing non-breeding distribution and behavior of red-legged kittiwakes from two geographically distant colonies. PLoS ONE 16, e0254686. doi:10.1371/journal.pone.0254686
Dumas, K., Gilg, O., Courbin, N., Corregidor-Castro, A., Evanno, G., Strøm, H., et al. (2022). Influence of sea-ice-related features and anthropogenic subsidies on the foraging behaviour of a high-Arctic seabird, the ivory gull (Pagophila eburnea). Mar. Biol. 169, 151. doi:10.1007/s00227-022-04137-5
Dunmall, K. M., Langan, J. A., Cunningham, C. J., Reist, J. D., Melling, H., Hunters, A., et al. (2024). Pacific salmon in the Canadian Arctic highlight a range-expansion pathway for sub-Arctic fishes. Glob. Change Biol. 30, e17353. doi:10.1111/gcb.17353
Dunmall, K. M., Reist, J. D., Carmack, E. C., Babaluk, J. A., Heide-Jørgensen, M. P., and Docker, M. F. (2013). “Pacific salmon in the arctic: harbingers of change,” in Responses of arctic marine ecosystems to climate change. Editors F. J. Mueter, D. M. S. Dickson, H. P. Huntington, J. R. Irvine, E. A. Longerwell, S. A. MacLeanet al. (Fairbanks, AK: University of Alaska Fairbanks: Alaska Sea Grant), 141–163. doi:10.4027/ramecc.2013.07
Edwards, J. E., Hedges, K. J., Kessel, S. T., and Hussey, N. E. (2022). Multi-year acoustic tracking reveals transient movements, recurring hotspots, and apparent seasonality in the coastal-offshore presence of Greenland sharks (Somniosus microcephalus). Front. Mar. Sci. 9, 902854. doi:10.3389/fmars.2022.902854
Efstathiou, E., Eldevik, T., Årthun, M., and Lind, S. (2022). Spatial patterns, mechanisms, and predictability of Barents Sea ice change. J. Clim. 35, 2961–2973. doi:10.1175/JCLI-D-21-0044.1
Egevang, C., Stenhouse, I. J., Phillips, R. A., Petersen, A., Fox, J. W., and Silk, J. R. D. (2010). Tracking of Arctic terns Sterna paradisaea reveals longest animal migration. Proc. Natl. Acad. Sci. USA. 107, 2078–2081. doi:10.1073/pnas.0909493107
Eisner, L. B., Zuenko, Y. I., Basyuk, E. O., Britt, L. L., Duffy-Anderson, J. T., Kotwicki, S., et al. (2020). Environmental impacts on walleye pollock (Gadus chalcogrammus) distribution across the Bering Sea shelf. Deep Sea Res. II 182, 104881. doi:10.1016/j.dsr2.2020.104881
Eriksen, E., Huserbråten, M., Gjøsæter, H., Vikebø, F., and Albretsen, J. (2020). Polar cod egg and larval drift patterns in the Svalbard archipelago. Polar Biol. 43, 1029–1042. doi:10.1007/s00300-019-02549-6
Eriksen, E., Skjoldal, H. R., Gjøsæter, H., and Primicerio, R. (2017). Spatial and temporal changes in the Barents Sea pelagic compartment during the recent warming. Progr. Ocean. 151, 206–226. doi:10.1016/j.pocean.2016.12.009
Escajeda, E., Laidre, K. L., Born, E. W., Wiig, Ø., Atkinson, S., Dyck, M., et al. (2018). Identifying shifts in maternity den phenology and habitat characteristics of polar bears (Ursus maritimus) in Baffin Bay and Kane Basin. Polar Biol. 41, 87–100. doi:10.1007/s00300-017-2172-6
Evans, S. R., and Bearhop, S. (2022). Variation in movement strategies: capital versus income migration. J. Anim. Ecol. 91, 1961–1974. doi:10.1111/1365-2656.13800
Ezhov, A. V., Gavrilo, M. V., Krasnov, Y. V., Bråthen, V. S., Moe, B., Baranskaya, A. V., et al. (2021). Transpolar and bi-directional migration strategies of black-legged kittiwakes Rissa tridactyla from a colony in Novaya Zemlya, Barents Sea, Russia. Mar. Ecol. Prog. Ser. 676, 189–203. doi:10.3354/meps13889
Falardeau, J., de Vernal, A., Fréchette, B., Hillaire-Marcel, C., Archambault, P., Fritz, M., et al. (2023). Impacts of stronger winds and less sea ice on Canadian Beaufort Sea shelf ecosystems since the late 1990s. Estuar. Coast. Shelf Sci. 294, 108520. doi:10.1016/j.ecss.2023.108520
Falardeau, M., and Bennett, E. M. (2020). Towards integrated knowledge of climate change in Arctic marine systems: a systematic literature review of multidisciplinary research. Arct. Sci. 6, 1–23. doi:10.1139/as-2019-0006
Falardeau, M., Bennett, E. M., Else, B., Fisk, A., Mundy, C. J., Choy, E. S., et al. (2022). Biophysical indicators and Indigenous and Local Knowledge reveal climatic and ecological shifts with implications for Arctic Char fisheries. Glob. Environ. Change. 74, 102469. doi:10.1016/j.gloenvcha.2022.102469
Falk-Petersen, S., Timofeev, S., Pavlov, V., and Sargent, J. R. (2007). “Climate variability and the effect on arctic food chains: the role of Calanus,” in Arctic-alpine ecosystems and people in a changing environment. Editors J. B. Ørbæk, R. Kallenborn, I. Tombre, E. N. Hegseth, S. Falk-Petersen, and A. H. Hoel (Berlin: Springer Verlag), 147–166.
Fechhelm, R. G., Streever, B., and Gallagway, B. J. (2007). The Arctic cisco (Coregonus autumnalis) subsistence and commercial fisheries, Colville River, Alaska: a conceptual model. Arctic 60, 421–429. doi:10.14430/arctic199
Feng, D., Gleason, C. J., Lin, P., Yang, X., Pan, M., and Ishitsuka, Y. (2021). Recent changes to Arctic river discharge. Nat. Commun. 12, 6917. doi:10.1038/s41467-021-27228-1
Ferguson, S. H., Dueck, L., Loseto, L. L., and Luque, S. P. (2010). Bowhead whale Balaena mysticetus seasonal selection of sea ice. Mar. Ecol. Prog. Ser. 411, 285–297. doi:10.3354/meps08652
Ford, J. D., Pearce, T., Canosa, I. V., and Harper, S. (2021). The rapidly changing Arctic and its societal implications. WIREs Clim. Change 12, e735. doi:10.1002/wcc.735
Forster, C. E., Norcross, B. L., Mueter, F. J., Logerwell, E. A., and Seitz, A. C. (2020). Spatial patterns, environmental correlates, and potential seasonal migration triangle of polar cod (Boreogadus saida) distribution in the Chukchi and Beaufort seas. Polar Biol. 43, 1073–1094. doi:10.1007/s00300-020-02631-4
Fort, J., Steen, H., Strøm, H., Tremblay, Y., Grønningsæter, E., Pettex, E., et al. (2013). Energetic consequences of contrasting winter migratory strategies in a sympatric Arctic seabird duet. J. Avian Biol. 44, 255–262. doi:10.1111/j.1600-048X.2012.00128.x
Fortune, S. M., Young, B. G., and Ferguson, S. H. (2020). Age-and sex-specific movement, behaviour and habitat-use patterns of bowhead whales (Balaena mysticetus) in the Eastern Canadian Arctic. Polar Biol. 43, 1725–1744. doi:10.1007/s00300-020-02739-7
Fossheim, M., Primicerio, R., Johannesen, E., Ingvaldsen, R. B., Aschan, M. M., and Dolgov, A. V. (2015). Recent warming leads to a rapid borealization of fish communities in the Arctic. Nat. Clim. Change 5, 673–677. doi:10.1038/NCLIMATE2647
Fox, A. D., Nielsen, R. D., and Petersen, I. K. (2019). Climate-change not only threatens bird populations but also challenges our ability to monitor them. Ibis 161, 467–474. doi:10.1111/ibi.12675
Frainer, A., Primiciero, R., Kotsch, S., Aune, M., Dolgov, A. V., Fossheim, M., et al. (2017). Climate-riven changes in functional biogeography of Arctic marine fish communities. PNAS 114, 12202–12207. doi:10.1073/pnas.1706080114
Frederiksen, M., Descamps, S., Erikstad, K. E., Gaston, A. J., Gilchrist, H. G., Grémillet, D., et al. (2016). Migration and wintering of a declining seabird, the thick-billed murre Uria lomvia, on an ocean basin scale: conservation implications. Biol. Cons. 200, 26–35. doi:10.1016/j.biocon.2016.05.011
Frederiksen, M., Gilg, O., and Yannic, G. (2021). Cross-icecap spring migration confirmed in a high-Arctic seabird, the Ivory Gull Pagophila eburnea. Ibis 163, 706–714. doi:10.1111/ibi.12903
Frederiksen, M., Moe, B., Daunt, F., Phillips, R. A., Barrett, R. T., Bogdanova, M. I., et al. (2012). Multi-colony tracking reveals the winter distribution of a pelagic seabird on an ocean basin scale. Divers. Distrib. 18, 530–542. doi:10.1111/j.1472-4642.2011.00864.x
Freitas, C., Kovacs, K. M., Ims, R. A., and Lydersen, C. (2008). Predicting habitat use by ringed seals (Phoca hispida) in a warming Arctic. Ecol. Model. 217, 19–32. doi:10.1016/j.ecolmodel.2008.05.014
Gall, A. E., Morgan, T. C., Day, R. H., and Kuletz, K. J. (2017). Ecological shift from piscivorous to planktivorous seabirds in the Chukchi Sea, 1975-2012. Polar Biol. 40, 61–78. doi:10.1007/s00300-016-1924-z
Gallagher, C. P., Courtney, M. B., Seitz, A. C., Lea, E. V., and Howland, K. L. (2021). Ocean-entry timing and marine habitat-use of Canadian Dolly Varden: dispersal among conservation, hydrocarbon exploration, and shipping areas in the Beaufort Sea. Estuar. Coast. Shelf Sci. 262, 107609. doi:10.1016/j.ecss.2021.107609
Gaston, A. J., Bertram, D. F., Boyne, A. W., Chardine, J. W., Davoren, G., Diamond, A. W., et al. (2009). Changes in Canadian seabird populations and ecology since 1970 in relation to changes in oceanography and food webs. Environ. Rev. 17, 267–286. doi:10.1139/a09-013
Gaston, A. J., Hashimoto, Y., and Wilson, L. (2017). Post-breeding movements of Ancient Murrelet Synthliboramphus antiquus family groups, subsequent migration of adults and implications for management. PLoS ONE 12, e0171726. doi:10.1371/journal.pone.0171726
Geoffroy, M., Robert, D., Darnis, G., and Fortier, L. (2011). The aggregation of polar cod (Boreogadus saida) in the deep Atlantic layer of ice-covered Amundsen Gulf (Beaufort Sea) in winter. Polar Biol. 34, 1959–1971. doi:10.1007/s00300-011-1019-9
George, J. C., Druckenmiller, M. L., Laidre, K. L., Suydam, R., and Person, B. (2015). Bowhead whale body condition and links to summer sea ice and upwelling in the Beaufort Sea. Progr. Ocean. 136, 250–262. doi:10.1016/j.pocean.2015.05.001
Gilg, O., Andreev, A., Aebischer, A., Kondratyev, A., Sokolov, A., and Dixon, A. (2016b). Satellite tracking of Ross’s gull Rhodostethia rosea in the Arctic Ocean. J. Ornithol. 157, 249–253. doi:10.1007/s10336-015-1273-7
Gilg, O., Istomina, L., Heygster, G., Strøm, H., Gavrilo, M. V., Mallory, M. L., et al. (2016a). Living on the edge of a shrinking habitat: the ivory gull, Pagophila eburnea, an endangered sea-ice specialist. Biol. Lett. 12, 20160277. doi:10.1098/rsbl.2016.0277
Gilg, O., Kovacs, K. M., Aars, J., Fort, J., Gauthier, G., Gramillet, D., et al. (2012). Climate change and the ecology and evolution of Arctic vertebrates. Ann. N. Y. Acad. Sci. 1249, 166–190. doi:10.1111/j.1749-6632.2011.06412.x
Gilg, O., Strøm, H., Aebischer, A., Gavrilo, M. V., Volkov, A. E., Miljeteig, C., et al. (2010). Post-breeding movements of northeast Atlantic ivory gull Pagophila eburnea populations. J. Avian Biol. 41, 532–542. doi:10.1111/j.1600-048X.2010.05125.x
Gjerdrum, C., Ronconi, R. A., Turner, K. L., and Hamer, T. E. (2021). Bird strandings and bright lights at coastal and offshore industrial sites in Atlantic Canada. Avian Conserv. Ecol. 16, art22. doi:10.5751/ACE-01860-160122
Glencross, J. S., Lavers, J. L., and Woehler, E. J. (2021). Breeding success of short-tailed shearwaters following extreme environmental conditions. Mar. Ecol. Prog. Ser. 672, 193–203. doi:10.3354/meps13791
Greer, A., Ng, V., and Fisman, D. (2008). Climate change and infectious diseases in North America: the road ahead. Can. Med. Assoc. J. 178, 715–722. doi:10.1503/cmaj.081325
Grüss, A., Thorson, J. T., Stawitz, C. C., Reum, J. C. P., Rohan, S. K., and Barnes, C. L. (2021). Synthesis of interannual variability in spatial demographic processes supports the strong influence of cold-pool extent on eastern Bering Sea walleye pollock (Gadus chalcogrammus). Progr. Oceanogr. 194, 102569. doi:10.1016/j.pocean.2021.102569
Guilford, T., Freeman, R., Boyle, D., Dean, B., Kirk, H., Phillips, R. A., et al. (2011). A dispersive migration in the Atlantic puffin and its implications for migratory navigation. PLoS ONE 6, e21336. doi:10.1371/journal.pone.0021336
Guthrie, C. M., Nguyen, H. T., Karpan, K., Watson, J. T., and Larson, W. A. (2021). Genetic stock composition analysis of Chinook salmon (Oncorhynchus tshawytscha) bycatch samples from the 2019 Bering Sea trawl pollock trawl fishery. U.S. Dep. Commer. NOAA Tech. Memo., 33. doi:10.25923/6aeb-dv24
Guyot, M., Dickson, C., Paci, C., Furgal, C., and Chan, H. M. (2006). Local observations of climate change and impacts on traditional food security in two northern Aboriginal communities. Int. J. Circumpolar Health 65, 403–415. doi:10.3402/ijch.v65i5.18135
Halliday, W. D., Dawson, J., Yurkowski, D. J., Doniol-Valcroze, T., Ferguson, S. H., Gjerdrum, C., et al. (2022). Vessel risks to marine wildlife in the Tallurutiup Imanga National Marine Conservation Area and the eastern entrance to the Northwest Passage. Environ. Sci. Policy 127, 181–195. doi:10.1016/j.envsci.2021.10.026
Halliday, W. D., Pine, M. K., Citta, J. J., Harwood, L., Hauser, D. D., Hilliard, R. C., et al. (2021). Potential exposure of beluga and bowhead whales to underwater noise from ship traffic in the Beaufort and Chukchi Seas. Ocean. Coast. Manage. 204, 105473. doi:10.1016/j.ocecoaman.2020.105473
Hamer, K. C., Schreiber, E. A., and Burger, J. (2001). Breeding biology, life histories, and life history-environment interactions in seabirds. Biol. Mar. Birds 45, 217–261. doi:10.1201/9781420036305
Hamilton, C. D., Kovacs, K. M., Ims, R. A., Aars, J., and Lydersen, C. (2017). An Arctic predator-prey system in flux: climate change impacts on coastal space use by polar bears and ringed seals. J. Anim. Ecol. 86, 1054–1064. doi:10.1111/1365-2656.12685
Hamilton, C. D., Lydersen, C., Ims, R. A., and Kovacs, K. M. (2015). Predictions replaced by facts: a keystone species' behavioural responses to declining arctic sea-ice. Biol. Lett. 11, 20150803. doi:10.1098/rsbl.2015.0803
Hamilton, C. D., Lydersen, C., Ims, R. A., and Kovacs, K. M. (2016). Coastal habitat use by ringed seals Pusa hispida following a regional sea-ice collapse: importance of glacial refugia in a changing Arctic. Mar. Ecol. Prog. Ser. 545, 261–277. doi:10.3354/meps11598
Hamilton, C. D., Vacquié-Garcia, J., Kovacs, K. M., Ims, R. A., and Lydersen, C. (2019). Contrasting changes in space use induced by climate change in two Arctic marine mammal species. Biol. Lett. 15, 20180834. doi:10.1098/rsbl.2018.0834
Hammer, L. J., Hussey, N. E., Marcoux, M., Pettitt-Wade, H., Hedges, K., Tallman, R., et al. (2022). Arctic char Salvelinus alpinus movement dynamics relative to ice breakup in a high Arctic embayment. Mar. Ecol. Prog. Ser. 682, 221–236. doi:10.3354/meps13939
Hansen, W. D., Brinkman, T. J., Leonawicz, M., Chapin, F. S., and Kofinas, G. P. (2013). Changing daily wind speeds on Alaska’s North Slope: implications for rural hunting opportunities. Arctic 66, 448–458. doi:10.14430/arctic4331
Harris, L., Moore, J.-S., Dunmall, K., Evans, M., Falardeau, M., Gallagher, C., et al. (2022). Arctic char in a rapidly changing North. Polar Knowl. Aqhaliat Rep. 4, 34–57. doi:10.35298/pkc.2021.02.eng
Harris, L. N., Yurkowski, D. J., Gilbert, M. J. H., Else, B. G. T., Duke, P. J., Ahmed, M. M. M., et al. (2020). Depth and temperature preference of anadromous Arctic char Salvelinus alpinus in the Kitikmeot Sea, a shallow and low-salinity area of the Canadian Arctic. Mar. Ecol. Prog. Ser. 634, 175–197. doi:10.3354/meps13195
Harrison, X. A., Blount, J. D., Inger, R., Norris, D. R., and Bearhop, S. (2011). Carry-over effects as drivers of fitness differences in animals. J. Anim. Ecol. 80, 4–18. doi:10.1111/j.1365-2656.2010.01740.x
Haug, T., Bogstad, B., Chierici, M., Gjøsæter, H., Hallfredsson, E. H., Høines, Å. S., et al. (2017). Future harvest of living resources in the Arctic Ocean north of the Nordic and Barents Seas: a review of possibilities and constraints. Fish. Res. 188, 38–57. doi:10.1016/j.fishres.2016.12.002
Hauser, D. D., Glenn, R. T., Lindley, E. D., Pikok, K. K., Heeringa, K., Jones, J., et al. (2023). Nunaaqqit Savaqatigivlugich—working with communities: evolving collaborations around an Alaska Arctic observatory and knowledge hub. Arct. Sci. 9, 635–656. doi:10.1139/as-2022-0044
Hauser, D. D. W., Laidre, K. L., Stafford, K. M., Stern, H. L., Suydam, R. S., and Richard, P. R. (2017). Decadal shifts in autumn migration timing by Pacific Arctic beluga whales are related to delayed annual sea ice formation. Glob. Change Biol. 23, 2206–2217. doi:10.1111/gcb.13564
Hauser, D. D. W., Laidre, K. L., and Stern, H. (2018b). Vulnerability of arctic marine mammals to vessel traffic in the increasingly ice-free Northwest Passage and Northern Sea Route. Proc. Natl. Acad. Sci. USA. 115, 7617–7622. doi:10.1073/pnas.1803543115
Hauser, D. D. W., Laidre, K. L., Stern, H. L., Suydam, R. S., and Richard, P. R. (2018a). Indirect effects of sea ice loss on summer-fall habitat and behaviour for sympatric populations of an Arctic marine predator. Divers. Distrib. 24, 791–799. doi:10.1111/ddi.12722
Hauser, D. D. W., Whiting, A. V., Mahoney, A. R., Goodwin, J., Harris, C., Schaeffer, R. J., et al. (2021). Co-production of knowledge reveals loss of Indigenous hunting opportunities in the face of accelerating Arctic climate change. Environ. Res. Lett. 16, 095003. doi:10.1088/1748-9326/ac1a36
Heide-Jørgensen, M. P., Chambault, P., Jansen, T., Gjelstrup, C. V. B., Rosing-Asvid, A., Macrander, A., et al. (2022). A regime shift in the Southeast Greenland marine ecosystem. Glob. Change Biol. 29, 668–685. doi:10.1111/gcb.16494
Heide-Jørgensen, M. P., Laidre, K. L., Quakenbush, L. T., and Citta, J. J. (2011). The Northwest Passage opens for bowhead whales. Biol. Lett. 8, 270–273. doi:10.1098/rsbl.2011.0731
Hendry, A. P., Bohlin, T., Jonsson, B., and Berg, O. (2004). “To sea or not to sea: anadromy versus non-anadromy in salmonids,” in Evolution illuminated: salmon and their relatives. Editors A. P. Hendry, and S. C. Stearns (Oxford, UK: Oxford University Press), 92–125.
Hendry, A. P., and Sterns, S. C. (2004). Evolution illuminated: salmon and their relatives. Oxford, UK: Oxford University Press.
Higdon, J. W., Westdal, K. H., and Ferguson, S. H. (2014). Distribution and abundance of killer whales (Orcinus orca) in Nunavut, Canada – an Inuit knowledge survey. J. Mar. Biol. Assoc. U. K. 94, 1293–1304. doi:10.1017/S0025315413000921
Hollins, J., Pettitt-Wade, H., Gallagher, C. P., Lea, E. V., Loseto, L. L., and Hussey, N. E. (2022). Distinct freshwater migratory pathways in Arctic char (Salvelinus alpinus) coincide with separate patterns of marine spatial habitat-use across a large coastal landscape. Can. J. Fish. Aquat. Sci. 79, 1447–1464. doi:10.1139/cjfas-2021-0291
Hop, H., and Gjøsæter, H. (2013). Polar cod (Boreogadus saida) and capelin (Mallotus villosus) as key species in marine food webs of the Arctic and the Barents Sea. Mar. Biol. Res. 9, 878–894. doi:10.1080/17451000.2013.775458
Hop, H., Welch, H. E., and Crawford, R. E. (1997). “Population structure and feeding ecology of Arctic cod schools in the Canadian High Arctic,” in Fish ecology in Arctic NorthNorth America. Editor J. B. Reynolds (Bethesda, Maryland, USA: American Fisheries Society Symposium), 19, 68–80.
Howard, C., Stephens, P. A., Tobias, J. A., Sheard, C., Butchart, S. H. M., and Willis, S. G. (2018). Flight range, fuel load and the impact of climate change on the journeys of migrant birds. Proc. R. Soc. B 285, 20172329. doi:10.1098/rspb.2017.2329
Huffeldt, N. P., Ballesteros, M., Helm, B., Linnebjerg, J. F., Merkel, F. R., Mosbech, A., et al. (2024). Thick-billed murres in breeding pairs migrate and overwinter far apart but in similar photic environments. J. Ornith. 165, 881–888. doi:10.1007/s10336-024-02176-x
Hunt Jr., G. L., Bakken, V., and Mehlum, F. (1996). Marine birds in the marginal ice zone of the Barents Sea in late winter and spring. Arctic 49, 53–61. doi:10.14430/arctic1183
Hunt Jr., G. L., Drinkwater, K. F., Arrigo, K., Berge, J., Daly, K. L., Danielson, S., et al. (2016). Advection in polar and sub-polar environments: impacts on high latitude marine ecosystems. Progr. Ocean. 149, 40–81. doi:10.1016/j.pocean.2016.10.004
Huntington, H. P., Braem, N. M., Brown, C. L., Hunn, E., Krieg, T. M., Lestenkof, P., et al. (2013). Local and traditional knowledge regarding the Bering Sea ecosystem: selected results from five indigenous communities. Deep Sea Res. II 94, 323–332. doi:10.1016/j.dsr2.2013.04.025
Huntington, H. P., Danielson, S. L., Wiese, F. K., Baker, M., Boveng, P., Citta, J. J., et al. (2020). Evidence suggests potential transformation of the Pacific Arctic ecosystem is underway. Nat. Clim. Change 10, 342–348. doi:10.1038/s41558-020-0695-2
Huntington, H. P., Quakenbush, L. T., and Nelson, M. (2016). Effects of changing sea ice on marine mammals and subsistence hunters in northern Alaska from traditional knowledge interviews. Biol. Lett. 12, 20160198. doi:10.1098/rsbl.2016.0198
Huntington, H. P., Quakenbush, L. T., and Nelson, M. (2017). Evaluating the effects of climate change on indigenous marine mammal hunting in Northern and Western Alaska using traditional knowledge. Front. Mar. Sci. 4, 319. doi:10.3389/fmars.2017.00319
Huntington, H. P., Sakakibara, C., Noongwook, G., Kanayurak, N., Skhauge, V., Zdor, E., et al. (2021). “Whale hunting in indigenous Arctic cultures,” in The bowhead whale. Editors J. C. George, and J. G. M. Thewissen (NY, USA: Academic Press), 501–517.
Huse, G., and Ellingsen, I. (2008). Capelin migrations and climate change – a modelling analysis. Clim. Change 87, 177–197. doi:10.1007/s10584-007-9347-z
Huserbråten, M. B. O., Eriksen, E., Gjøsæter, H., and Vikebø, F. (2019). Polar cod in jeopardy under the retreating Arctic sea ice. Commun. Biol. 2, 407. doi:10.1038/s42003-019-0649-2
ICC-Alaska (2015). Alaskan inuit food security conceptual framework: how to assess the Arctic from an Inuit perspective: summary report and recommendations report. Anchorage, Alaska: ICC-Alaska.
Ingvaldsen, R. B., Assmann, K. M., Primicierio, R., Fossheim, M., Polyakov, I. V., and Dolgov, A. V. (2021). Physical manifestations and ecological implications of Arctic Atlantification. Nat. Rev. Earth Environ. 2, 874–889. doi:10.1038/s43017-021-00228-x
Ingvaldsen, R. B., Eriksen, E., Gjøsæter, H., Engås, A., Schuppe, B. K., Assmann, K. M., et al. (2023). Under-ice observations by trawls and multi-frequency acoustics in the Central Arctic Ocean reveals abundance and composition of pelagic fauna. Sci. Rep. 13, 1000. doi:10.1038/s41598-023-27957-x
Insley, S. J., Halliday, W. D., Mouy, X., and Diogou, N. (2021). Bowhead whales overwinter in the Amundsen Gulf and eastern Beaufort Sea. R. Soc. Open Sci. 8, 202268. doi:10.1098/rsos.202268
IPCC (2023). “Summary for policymakers,” in Climate change 2023: synthesis report. Contribution of working groups I, II and III to the sixth assessment report of the intergovernmental panel on climate change. Editors H. Lee, and J. Romero (Geneva, Switzerland: IPPC), 1–34.
Irons, D. B., Petersen, A., Anker-Nilssen, T., Arthukin, Y., Barrett, R., Boertman, D., et al. (2015). Circumpolar seabird monitoring plan. CAFF monitoring report No. 17. Akureyri, Iceland: CAFF International Secretariat.
Isaksen, K., Nordli, Ø., Ivanov, B., Køltzow, M. A. Ø., Aaboe, S., Gjelten, H. M., et al. (2022). Exceptional warming over the Barents area. Sci. Rep. 12, 9371. doi:10.1038/s41598-022-13568-5
Ivanova, S. V., Kessel, S. T., Espinoza, M., McLean, M. F., O’Neill, C., Landry, J., et al. (2020). Shipping alters the movement and behavior of Arctic cod (Boreogadus saida), a keystone fish in Arctic marine ecosystems. Ecol. Appl. 30, e02050. doi:10.1002/eap.2050
Jahncke, J., Coyle, K. O., Zeeman, S. I., Kachel, N. B., and Hunt Jr., G. L. (2005). Distribution of foraging shearwaters relative to inner front of SE Bering Sea. Mar. Ecol. Prog. Ser. 305, 219–233. doi:10.3354/meps305219
Jay, C., Fishchbach, A. S., and Kochnev, A. A. (2012). Walrus area use in the Chukchi Sea during sparse sea ice cover. Mar. Ecol. Prog. Ser. 468, 1–13. doi:10.3354/meps10057
Jensen, J. L. A., Rikardsen, A. H., Thorstad, E. B., Suhr, A. H., Davidsen, J. G., and Primicerio, R. (2014). Water temperatures influence the marine area use of Salvelinus alpinus and Salmo trutta. J. Fish. Biol. 84, 1640–1653. doi:10.1111/jfb.12366
Jones, T., Parrish, J. K., Lindsey, J., Wright, C., Burgess, H. K., Dolliver, J., et al. (2023). Marine bird mass mortality events as an indicator of the impacts of ocean warming. Mar. Ecol. Prog. Ser. 737, 161–181. doi:10.3354/meps14330
Karnovsky, N. J., Hobson, K. A., Brown, Z. W., and Hunt Jr., G. L. (2009). Distribution and diet of ivory gulls (Pagophila eburnea) in the North Water Polynia. Arctic 62, 65–74. doi:10.14430/arctic113
Karpouzoglou, T., De Steur, L., Smedsrud, L. H., Karcher, M., and Sumata, H. (2024). Three forcing mechanisms of freshwater transport in Fram Strait. J. Geophys. Res. Oceans 129, e2024JC020930. doi:10.1029/2024JC020930
Kędra, M., Moritz, C., Choy, E. S., David, C., Degen, R., Duerksen, S., et al. (2015). Status and trends in the structure of Arctic benthic food webs. Polar Res. 34, 23775. doi:10.3402/polar.v34.23775
Kenyon, K. A., Yurkowski, D. J., Orr, J., Barber, D., and Ferguson, S. H. (2018). Baffin Bay narwhal (Monodon monoceros) select bathymetry over sea ice during winter. Polar Biol. 41, 2053–2063. doi:10.1007/s00300-018-2345-y
Kessel, S. T., Hussey, N. E., Crawford, R. E., Yurkowski, D. J., Webber, D. M., Dick, T. A., et al. (2017). First documented large-scale horizontal movements of individual Arctic cod (Boreogadus saida). Can. J. Fish. Aquat. Sci. 74, 292–296. doi:10.1139/cjfas-2016-0196
Kim, J. H., Cho, K. H., La, H. S., Choy, E. J., Matsuno, K., Kang, S. H., et al. (2020). Mass occurrence of Pacific copepods in the southern Chukchi Sea during summer: implications of the high-temperature Bering summer water. Front. Mar. Sci. 7, 612. doi:10.3389/fmars.2020.00612
Klaassen, M. (2003). “Relationships between migration and breeding strategies in arctic breeding birds,” in Avian migration. Editors P. Berthold, E. Gwinner, and E. Sonnenschein (Berlin, Germany: Springer), 237–249.
Kochanowicz, Z., Dawson, J., Halliday, W. D., Sawada, M., Copland, L., Carter, N. A., et al. (2021). Using western science and Inuit knowledge to model ship-source noise exposure for cetaceans (marine mammals) in Tallurutiup Imanga (Lancaster Sound), Nunavut, Canada. Mar. Policy 130, 104557. doi:10.1016/j.marpol.2021.104557
Kokelj, S. V., Kokoszka, J., van der Sluijs, J., Rudy, A. C. A., Tunnicliffe, J., Shakil, S., et al. (2021). Thaw-driven mass wasting couples slopes with downstream systems, and effects propagate through Arctic drainage networks. Cryosphere 15, 3059–3081. doi:10.5194/tc-15-3059-2021
Kovach, R. P., Joyce, J. E., Echave, J. D., Lindberg, M. S., and Tallmon, D. A. (2013). Earlier migration timing, decreasing phenotypic variation, and biocomplexity in multiple salmonid species. PLoS ONE 8, e53807. doi:10.1371/journal.pone.0053807
Kovacs, K. M., Aguilar, A., Aurioles, D., Burkanov, V., Campagna, C., Gales, N., et al. (2012). Global threats to pinnipeds. Mar. Mamm. Sci. 28, 414–436. doi:10.1111/j.1748-7692.2011.00479.x
Kovacs, K. M., Belikov, S., Boveng, P., Desportes, G., Ferguson, S., Hansen, R., et al. (2021). 2021 State of the Arctic Marine Biodiversity Report (SAMBR) update: marine mammals. Technical Report. Akureyri, Iceland: CAFF International Secretariat.
Kovacs, K. M., and Lydersen, C. (2008). Climate change impacts on seals and whales in the North Atlantic Arctic and adjacent shelf seas. Sci. Progr. 91, 117–150. doi:10.3184/003685008X324010
Kovacs, K. M., Lydersen, C., Overland, J. E., and Moore, S. (2011). Impacts of changing sea-ice conditions on Arctic marine mammals. Mar. Biodiv. 41, 181–194. doi:10.1007/S12526-010-0061-0
Kuletz, K., Cushing, D., and Labunski, E. (2020). Distributional shifts among seabird communities of the Northern Bering and Chukchi seas in response to ocean warming during 2017 – 2019. Deep Sea Res. II 181, 104913. doi:10.1016/j.dsr2.2020.104913
Kuletz, K. J., Ferguson, M. C., Hurley, B., Gall, A. E., Labunski, E. A., and Morgan, T. C. (2015). Seasonal spatial patterns in seabird and marine mammal distribution in the eastern Chukchi and western Beaufort seas: identifying biologically important pelagic areas. Progr. Oceanogr. 136, 175–200. doi:10.1016/j.pocean.2015.05.012
Kuletz, K. J., Gall, A. E., Morgan, T. C., Prichard, A. K., Eisner, A. B., Kimmel, D. G., et al. (2024). Seabird responses to ecosystem changes driven by marine heatwaves in a warming Arctic. Mar. Ecol. Prog. Ser. 737, 59–88. doi:10.3354/meps14493
Kuletz, K. J., Renner, M., Labunski, E. A., and Hunt Jr., G. L. (2014). Changes in the distribution and abundance of albatrosses in the eastern Bering Sea: 1975-2010. Deep Sea Res. II 109, 282–292. doi:10.1016/j.dsr2.2014.05.006
Kumar, A., Yadav, J., and Mohan, R. (2020). Global warming leading to alarming recession of the Arctic sea-ice cover: insights from remote sensing observations and model reanalysis. Heliyon 6, e04355. doi:10.1016/j.heliyon.2020.e04355
Laidre, K. L., Stern, H., Born, E. W., Heagerty, P., Atkinson, S., Wiig, Ø., et al. (2018). Changes in winter and spring resource selection by polar bears Ursus maritimus in Baffin Bay over two decades of sea-ice loss. Endang. Species Res. 36, 1–14. doi:10.3354/esr00886
Laidre, K. L., Stern, H., Kovacs, K. M., Lowry, L., Moore, S. E., Regehr, E. V., et al. (2015). Arctic marine mammal population status, sea ice habitat loss, and conservation recommendations for the 21st century. Conserv. Biol. 29, 724–737. doi:10.1111/cobi.12474
Landa, C. S., Ottesen, G., Sundby, S., Dingsør, G. E., and Stiansen, J. E. (2014). Recruitment, distribution boundary and habitat temperature of an arcto-boreal gadoid in a climatically changing environment: a case study on Northeast Arctic haddock (Melanogrammus aeglefinus). Fish. Oceanogr. 23, 506–520. doi:10.1111/fog.12085
Langangen, Ø., Ottersen, G., Ciannelli, L., Vikebø, F. B., and Stige, L. C. (2016). Reproductive strategy of a migratory fish stock: implications of spatial variations in natural mortality. Can. J. Fish. Aquat. Sci. 73, 1742–1749. doi:10.1139/cjfas-2015-0321
Lefort, K. J., Garroway, C. J., and Ferguson, S. H. (2020). Killer whale abundance and predicted narwhal consumption in the Canadian Arctic. Glob. Change Biol. 26, 4276–4283. doi:10.1111/gcb.15152
Leu, E., Mundy, C. J., Assmy, P., Campbell, K., Gabrielsen, T. M., Gosselin, M., et al. (2015). Arctic spring awakening–Steering principles behind the phenology of vernal ice algal blooms. Progr. Oceanogr. 139, 151–170. doi:10.1016/j.pocean.2015.07.012
Levine, R., De Robertis, A., Grunbaum, D., Wildes, S., Farley, E., Stabeno, P. J., et al. (2023). Climate-driven shifts in pelagic fish distributions in a rapidly changing Pacific Arctic. Deep Sea Res. II 208, 105244. doi:10.1016/j.dsr2.2022.105244
Lin, P., Pickart, R. S., McRaven, L. T., Arrigo, K. R., Bahr, F., Lowry, K. E., et al. (2019). Water mass evolution and circulation of the northeastern Chukchi Sea in summer: implications for nutrient distributions. J. Geophys. Res. Oceans 124, 4416–4432. doi:10.1029/2019JC015185
Logerwell, E., Busby, M., Carothers, C., Cotton, S., Duffy-Anderson, J., Farley, E., et al. (2015). Fish communities across a spectrum of habitats in the western Beaufort Sea and Chukchi Sea. Prog. Oceanogr. 136, 115–132. doi:10.1016/j.pocean.2015.05.013
Lone, K., Hamilton, C. D., Aars, J., Lydersen, C., and Kovacs, K. M. (2019). Summer habitat selection by ringed seals (Pusa hispida) in the drifting sea ice of the northern Barents Sea. Polar Res. 38, 3483. doi:10.33265/polar.v38.3483
Lu, K., Weingartner, T., Danielson, S., Winsor, P., Dobbins, E., Martini, K., et al. (2015). Lateral mixing across ice meltwater fronts of the Chukchi Sea shelf. Geophys. Res. Lett. 42, 6754–6761. doi:10.1002/2015GL064967
Luque, S. P., and Ferguson, S. H. (2009). Ecosystem regime shifts have not affected growth and survivorship of eastern Beaufort Sea belugas. Oecologia 160, 367–378. doi:10.1007/s00442-009-1300-6
Lydersen, C., Assmy, P., Falk-Petersen, S., Kohler, J., Kovacs, K. M., Reigstad, M., et al. (2014). The importance of tidewater glaciers for marine mammals and seabirds in Svalbard, Norway. J. Mar. Syst. 129, 452–471. doi:10.1016/j.jmarsys.2013.09.006
Lydersen, C., Fisk, A. T., and Kovacs, K. M. (2016). A review of Greenland shark (Somniosus microcephalus) studies in the Kongsfjorden area, Svalbard Norway. Polar Biol. 39, 2169–2178. doi:10.1007/s00300-016-1949-3
Lydersen, C., and Kovacs, K. M. (2021). A review of the ecology and status of white whales (Delphinapterus leucas) in Svalbard, Norway. Polar Res. 40, 5509. doi:10.33265/polar.v40.5509
Lydersen, C., Martin, A. R., Kovacs, K. M., and Gjertz, I. (2001). Summer and autumn movements of white whales Delphinapterus leucas in Svalbard, Norway. Mar. Ecol. Prog. Ser. 219, 265–274. doi:10.3354/meps219265
Lydersen, C., Vacquié-Garcia, J., Heide-Jørgensen, M. P., Øien, N., Guinet, C., and Kovacs, K. M. (2020). Autumn movements of fin whales (Balaenoptera physalus) from Svalbard, Norway, revealed by satellite tracking. Sci. Rep. 10 (16966), 16966–17013. doi:10.1038/s41598-020-73996-z
Lydersen, C., Wiig, Ø., Bachmann, L., Heide-Jørgensen, M. P., Swift, R., Kovacs, K. M., et al. (2012). Lost highway not forgotten: satellite tracking of a bowhead whale (Balaena mysticetus) from the critically endangered Spitsbergen stock. Arctic 65, 76–86. doi:10.14430/arctic4167
Lynghammar, A., Christiansen, J. S., Mecklenburg, C. V., Karamushko, O. V., Møller, P. R., and Gallucci, V. F. (2012). Species richness and distribution of chondrichthyan fishes in the Arctic Ocean and adjacent seas. Biodiversity 14, 57–66. doi:10.1080/14888386.2012.706198
Lynghammar, A., Hop, H., and Præbel, K. (2024). “Fish fauna in a pan-Arctic scenario of declining sea ice,” in Elements of a pan-Arctic Ocean ecology. Editor P. Wassmann (Stamsund Norway: Orkana Forlag), 337–350.
Mackenzie, B. R., Payne, M. R., Boje, J., Høyer, J. L., and Siegstad, H. (2014). A cascade of warming impacts brings bluefin tuna to Greenland waters. Glob. Change Biol. 20, 2484–2491. doi:10.1111/gcb.12597
Madsen, J., Jaspers, C., Frikke, J., Gundersen, O. M., Nolet, B. A., Nolet, K., et al. (2019). A gloomy future for light-bellied brent geese in Tusenøyane, Svalbard, under a changing predator regime. Polar Res. 38, 3393. doi:10.33265/polar.v38.3393
Maftei, M., Davis, S. E., and Mallory, M. L. (2015). Confirmation of a wintering ground of ross's gull Rhodostethia rosea in the northern Labrador Sea. Ibis 157, 642–647. doi:10.1111/ibi.12261
Maftei, M., Davis, S. E., Uher-Koch, B. D., Gesmundo, C., Suydam, R., and Mallory, M. L. (2014). Quantifying fall migration of Ross’s gulls (Rhodostethia rosea) past Point Barrow, Alaska. Polar Biol. 37, 1705–1710. doi:10.1007/s00300-014-1552-4
Mallory, M. L., Gilchrist, H. G., Fontaine, A. J., and Akearok, J. A. (2003). Local ecological knowledge of ivory gull declines in Arctic Canada. Arctic 56, 293–298. doi:10.14430/arctic625
Markones, N., Dierschke, V., and Garthe, S. (2010). Seasonal differences in at-sea activity of seabirds underline high energetic demands during the breeding period. J. Ornithol. 151, 329–336. doi:10.1007/s10336-009-0459-2
Matthews, C. J. D., Yarnes, C. T., Lefort, K. J., Edkins, T. L., Kiszka, J. J., and Ferguson, S. H. (2024). Dietary plasticity and broad North Atlantic origins inferred from bulk and amino acid-specific δ15N and δ13C favour killer whale range expansions into Arctic waters. J. Anim. Ecol. 93, 1049–1064. doi:10.1111/1365-2656.14123
Matthews, C. J. D., Ghazal, M., Lefort, K. J., and Inuarak, E. (2020a). Epizoic barnacles on Arctic killer whales indicate residency in warm waters. Mar. Mamm. Sci. 36, 1010–1014. doi:10.1111/mms.12674
Matthews, C. J. D., Breed, G. A., Leblanc, B., and Ferguson, S. (2020b). Killer whale presence drives bowhead whale selection for sea ice in Arctic seascapes of fear. Proc. Natl. Acad. Sci. USA. 117, 6590–6598. doi:10.1073/pnas.1911761117
McGuire, T. L., Himes-Boor, G. K., McClung, J., Stephens, A. D., Garner, C., Shelden, K. E. W., et al. (2020). Distribution and habitat use by endangered Cook Inlet beluga whales: patterns observed during a photo-identification study, 2005–2017. Aquat. Conserv. Mar. Freshw. Ecosyst. 30, 2402–2427. doi:10.1002/aqc.3378
McKeon, C. S., Weber, M. X., Alter, S. E., Seavy, N. E., Crandall, E. D., Barshis, D. J., et al. (2016). Melting barriers to faunal exchange across ocean basins. Glob. Change Biol. 22, 465–473. doi:10.1111/gcb.13116
McKinney, M. M., Iverson, S. J., Fish, A. T., Sonne, C., Riget, F. F., Letcher, R. J., et al. (2013). Global change effects on the long-term feeding ecology and contaminant exposures of East Greenland polar bears. Glob. Change Biol. 19, 2360–2372. doi:10.1111/gcb.12241
McMeans, B. C., Arts, M. T., Lydersen, C., Kovacs, K. M., Hop, H., Falk-Petersen, S., et al. (2013). The role of Greenland sharks (Somniosus microcephalus) in an Arctic ecosystem: assessed via stable isotopes and fatty acids. Mar. Biol. 160, 1223–1238. doi:10.1007/s00227-013-2174-z
McNicholl, D. G., Davoran, G. K., Majeswki, A. R., and Reist, J. D. (2018). Isotopic niche overlap between co-occurring capelin (Mallotus villosus) and polar cod (Boreogadus saida) and the effect of lipid extraction on stable isotope ratios. Polar. Biol. 41, 423–432. doi:10.1007/s00300-017-2199-8
Meredith, M., Sommerkorn, M., Cassotta, S., Derksen, C., Ekaykin, A., Hollowed, A., et al. (2019). “Polar regions,” in IPCC special report on the ocean and cryosphere in a changing climate. Editors H.-O. Pörtner, D. C. Roberts, V. Masson-Delmotte, P. Zhai, M. Tignor, E. Poloczanskaet al. (Cambridge UK: Cambridge University Press), 203–320.
Merkel, F. R., and Johansen, K. L. (2011). Light-induced bird strikes on vessels in Southwest Greenland. Mar. Pollut. Bull. 62, 2330–2336. doi:10.1016/j.marpolbul.2011.08.040
Miller, E. N., Lunn, N. J., McGeachy, D., and Derocher, A. E. (2022). Autumn migration phenology of polar bears (Ursus maritimus) in Hudson Bay, Canada. Polar Biol. 45, 1023–1034. doi:10.1007/s00300-022-03050-3
Milner-Gulland, E. J., Fryxell, J. M., and Sinclair, A. R. E. (2011). Animal migration: a synthesis. Oxford, UK: Oxford University Press.
Møller, E. F., Johansen, K. L., Agersted, M. D., Rigét, F., Clausen, D. S., Larsen, J., et al. (2018). Zooplankton phenology may explain the North Water Polynya’s importance as a breeding area for little auks. Mar. Ecol. Prog. Ser. 605, 207–223. doi:10.3354/meps12745
Møller, E. F., and Nielsen, T. G. (2020). Borealization of Arctic zooplankton - smaller and less fat zooplankton species in Disko Bay, Western Greenland. Limnol. Oceanogr. 65, 1175–1188. doi:10.1002/lno.11380
Moore, S. E. (2016). Is it “boom times” for baleen whales in the Pacific Arctic region? Biol. Lett. 12, 20160251. doi:10.1098/rsbl.2016.0251
Moore, S. E., Clarke, J. T., Okkonen, S. R., Grebmeier, J. M., Berchok, C. L., and Stafford, K. M. (2022). Changes in gray whale phenology and distribution related to prey variability and ocean biophysics in the northern Bering and eastern Chukchi seas. PLoS ONE 17, e0265934. doi:10.1371/journal.pone.0265934
Moore, S. E., and Hauser, D. D. (2019). Marine mammal ecology and health: finding common ground between conventional science and indigenous knowledge to track arctic ecosystem variability. Environ. Res. Lett. 14, 075001. doi:10.1088/1748-9326/ab20d8
Moore, S. E., and Kuletz, K. J. (2019). Marine birds and mammals as ecosystem sentinels in and near Distributed Biological Observatory regions: an abbreviated review of published accounts and recommendations for integration to ocean observatories. Deep Sea Res. II 162, 211–217. doi:10.1016/j.dsr2.2018.09.004
Moore, S. E., Logerwell, E., Eisner, L., Farley, E., Harwood, L., Kuletz, K., et al. (2014). “Marine fishes, birds and mammals as sentinels of ecosystem variability and reorganization in the Pacific Arctic region,” in The pacific arctic region: ecosystem status and trends in a rapidly changing environment. Editors J. Grebmeier, and W. Maslowski (Dordrecht, Germany: Springer), 337–392.
Mosbech, A., Gilchrist, G., Merkel, F., Sonne, C., Flagstad, A., and Nyegaard, H. (2006). Year-round movements of northern common eiders Somateria mollissima borealis breeding in Arctic Canada and West Greenland followed by satellite telemetry. Ardea 94, 651–665.
Mueter, F. J., Planque, B., Hunt Jr., G. L., Alabia, I. D., Hirawake, T., Eisner, L., et al. (2021). Possible future scenarios in the gateways to the Arctic for Subarctic and Arctic marine systems: II. prey resources, food webs, fish, and fisheries. ICES J. Mar. Sci. 78, 3017–3045. doi:10.1093/icesjms/fsab122
Mulder, I. M., Morris, C. J., Dempson, J. B., Fleming, I. A., and Power, M. (2020). Marine temperature and depth use by anadromous Arctic char correlates to body size and diel period. Can. J. Fish. Aquat. Sci. 77, 882–893. doi:10.1139/cjfas-2019-0097
Murphy, J. M., Howard, K. G., Gann, J. C., Cieciel, K. C., Templin, W. D., and Guthrie, C. M. (2017). Juvenile Chinook salmon abundance in the northern Bering Sea: implications for future returns and fisheries in the Yukon River. Deep Sea Res. II 135, 156–167. doi:10.1016/j.dsr2.2016.06.002
Nishizawa, B., Matsuno, K., Labunski, E., Kuletz, K., Yamaguchi, A., and Watanuki, Y. (2017). Seasonal distribution of short-tailed shearwaters and their prey in the Bering and Chukchi seas. Biogeosciences 14, 203–214. doi:10.5194/bg-14-203-2017
Noongwook, G., Huntington, H. P., and George, J. C. (2007). Traditional knowledge of the bowhead whale (Balaena mysticetus) around St. Lawrence Island, Alaska. Arctic 60, 47–54.
Nordli, E., Strøm, J. F., Bøhn, T., Thorstad, E. B., Serra-Llinares, R. M., Nilsen, R., et al. (2023). Behaviour and habitat use of first-time migrant Arctic charr: novel insights from a subarctic marine area. Mar. Ecol. Prog. Ser. 709, 77–90. doi:10.3354/meps14279
Ogloff, W. R., Ferguson, S. H., Fisk, A. T., Marcoux, M., Hussey, N. E., Jaworenko, A., et al. (2021). Long-distance movements and associated diving behaviour of ringed seals (Pusa hispida) in the eastern Canadian Arctic. Arct. Sci. 7, 494–511. doi:10.1139/as-2019-0042
Øigård, T. A., Haug, T., and Nilssen, K. T. (2014). Current status of hooded seals in the Greenland Sea. Victims of climate change and predation? Biol. Cons. 172, 29–36. doi:10.1016/j.biocon.2014.02.007
Okkonen, S., Ashjian, C. A., Campbell, R., Clarke, J. T., Moore, S. E., and Taylor, K. D. (2011). Satellite observations of circulation features associated with a bowhead whale feeding ‘hotspot’ near Barrow, Alaska. Remote Sens. Environ. 115, 2168–2174. doi:10.1016/j.rse.2011.04.024
Olson, J. W., Rode, K. D., Eggett, D., Smith, T. S., Wilson, R. R., Durner, G. M., et al. (2017). Collar temperature sensor data reveal long-term patterns in southern Beaufort Sea polar bear den distribution on pack ice and land. Mar. Ecol. Prog. Ser. 564, 211–224. doi:10.3354/meps12000
Orben, R. A., Irons, D. B., Paredes, R., Roby, D. D., Phillips, R. A., and Shaffer, S. A. (2015a). North or south? Niche separation of endemic red-legged kittiwakes and sympatric black-legged kittiwakes during their non-breeding migrations. J. Biogeog. 42, 401–412. doi:10.1111/jbi.12425
Orben, R. A., Kokubun, N., Fleishman, A. B., Will, A. P., Yamamoto, T., Shaffer, S. A., et al. (2018). Persistent annual migration patterns of a specialist seabird. Mar. Ecol. Prog. Ser. 593, 231–245. doi:10.3354/meps12459
Orben, R. A., Paredes, R., Roby, D. D., Irons, D. B., and Shaffer, S. A. (2015b). Wintering North Pacific black-legged kittiwakes balance spatial flexibility and consistency. Mov. Ecol. 3, 36. doi:10.1186/s40462-015-0059-0
Orlov, A. M., Rybakov, M. O., Vedishcheva, E. V., Volkov, A. A., and Orlova, S. Y. (2021). Walleye pollock Gadus chalcogrammus, a species with continuous range from the Norwegian Sea to Korea, Japan, and California: New Records from the Siberian Arctic. J. Mar. Sci. Eng. 9, 1141. doi:10.3390/jmse9101141
Österblom, H., Olsson, O., Bleckner, T., and Furness, R. W. (2008). Junk-food in marine ecosystem. Oikos 117, 967–977. doi:10.1111/j.0030-1299.2008.16501.x
Ottersen, G., Bogstad, B., Yaragina, N. A., Stige, L. C., Vikebø, F. B., and Dalpadado, P. A. (2014). Review of early life history dynamics of Barents Sea cod (Gadus morhua). ICES J. Mar. Sci. 71, 2064–2087. doi:10.1093/icesjms/fsu037
Ovitz, K. L., Matari, K. G. A., O'Hara, S., Esagok, D., Hunters, I., Committee, T., et al. (2024). Observations of social and environmental change on Kendall Island (Ukiivik), a traditional whaling camp in the inuvialuit settlement region. Arct. Sci. 10, 140–168. doi:10.1139/as-2022-0016
Pagano, A. M., Durner, G. M., Atwood, T. C., and Douglas, D. C. (2021). Effects of sea ice decline and summer land use on polar bear home range size in the Beaufort Sea. Ecosphere 12, e03768. doi:10.1002/ecs2.3768
Patterson, A., Gilchrist, H. G., Gaston, A., and Elliott, K. (2021). Northwest range shifts and shorter wintering period of an Arctic seabird in response to four decades of changing ocean climate. Mar. Ecol. Prog. Ser. 679, 163–179. doi:10.3354/meps13890
Pearce, T., Gallagher, C. P., Lea, E. V., Kudlak, G., Pettitt-Wade, H., Smart, J., et al. (2024). Inuit traditional ecological knowledge of anadromous Arctic char, iqalukpik (Salvelinus alpinus) under changing climatic conditions in the Amundsen Gulf, western Canadian Arctic. Arctic. doi:10.14430/arctic79391
Peklova, I., Hussey, N. E., Hedges, K. J., Treble, M. A., and Fisk, A. T. (2014). Movement, depth and temperature preferences of an important bycatch species, Arctic skate Amblyraja hyperborea, in Cumberland Sound, Canadian Arctic. Endang. Species Res. 23, 229–240. doi:10.3354/esr00563
Perrette, M., Yool, A., Quartly, G. D., and Popova, E. E. (2011). Near-ubiquity of ice-edge blooms in the Arctic. Biogeosciences 8, 515–524. doi:10.5194/bg-8-515-2011
Pettitt-Wade, H., Loseto, L. L., Majewski, A., and Hussey, N. E. (2021). Cod movement ecology in a warming world: circumpolar arctic gadids. Fish. Fish. 22, 562–591. doi:10.1111/faf.12536
Piatt, J. F., Douglas, D. C., Arimitsu, M. L., Kissling, M. L., Madison, E. N., Schoen, S. K., et al. (2021). Kittlitz’s murrelet seasonal distribution and post–breeding migration from the Gulf of Alaska to the Arctic Ocean. Arctic 74, 482–495. doi:10.14430/arctic73992
Piatt, J. F., Harding, A. M., Shultz, M., Speckman, S. G., Van Pelt, T. I., Drew, G. S., et al. (2007). Seabirds as indicators of marine food supplies: cairns revisited. Mar. Ecol. Prog. Ser. 352, 221–234. doi:10.3354/meps07078
Pilfold, N. W., McCall, A., Derocher, A. E., Lunn, N. J., and Richardson, E. (2017). Migratory response of polar bears to sea ice loss: to swim or not to swim. Ecography 40, 189–199. doi:10.1111/ecog.02109
Polyakov, I. V., Ingvaldsen, R. B., Pnyushkov, A. V., Bhatt, U. S., Francis, J. A., Janout, M., et al. (2023). Fluctuating Atlantic inflows modulate Arctic atlantification. Science 381, 972–979. doi:10.1126/science.adh5158
Posdaljian, N., Soderstjerna, C., Jones, J. M., Solsona-Berga, A., Hildebrand, J. A., Westdal, K., et al. (2022). Changes in sea ice and range expansion of sperm whales in the Eclipse Sound region of Baffin Bay, Canada. Glob. Change Biol. 28, 3860–3870. doi:10.1111/gcb.16166
Pöyhönen, V., Thomisch, K., Kovacs, K. M., Lydersen, C., and Ahonen, H. (2024). High Arctic “hotspots” for sperm whales (Physeter macrocephalus) off western and northern Svalbard, Norway, revealed by multi-year Passive Acoustic Monitoring (PAM). Sci. Rep. 14, 5825. doi:10.1038/s41598-024-56287-9
Priest, J. T., Mueter, F. J., Raborn, S. W., and Sutton, T. M. (2022). Effects of environmental variables on a nearshore arctic fish community, 2001–2018. Polar Biol. 45, 585–599. doi:10.1007/s00300-022-03013-8
Reeves, R. R., Ewins, P. J., Agbayani, S., Heidi-Jorgensen, M. P., Kovacs, K. M., Lydersen, C., et al. (2014). Distribution of endemic cetaceans in relation to hydrocarbon development and commercial shipping in a warming Arctic. Mar. Policy 44, 375–389. doi:10.1016/j.marpol.2013.10.005
Regehr, E. V., Wilson, R. R., Rode, K. D., Runge, M. C., and Stern, H. L. (2017). Harvesting wildlife affected by climate change: a modelling and management approach for polar bears. J. Appl. Ecol. 54, 1534–1543. doi:10.1111/1365-2664.12864
Reist, J. D., Wrona, F. J., Prowse, T. D., Power, M., Dempson, J. B., King, J. R., et al. (2006). An overview of effects of climate change on selected Arctic freshwater and anadromous fishes. Ambio 35, 381–387. doi:10.1579/0044-7447(2006)35[381:AOOEOC]2.0.CO;2
Renaud, P. E., Berge, J., Varpe, Ø., Lønne, O. J., Nahrgang, J., Ottesen, C., et al. (2012). Is the poleward expansion by Atlantic cod. and haddock threatening native polar cod, Boreogadus saida? Polar Biol. 35, 401–412. doi:10.1007/s00300-011-1085-z
Renaud, P. E., Daase, M., Banas, N. S., Gabrielsen, T. M., Søreide, J. E., Varpe, Ø., et al. (2018). Pelagic food-webs in a changing Arctic: a trait-based perspective suggests a mode of resilience. ICES J. Mar. Sci. 75, 1871–1881. doi:10.1093/icesjms/fsy063
Renner, A. H. H., Sundfjord, A., Janout, M. A., Ingvaldsen, R. B., Beszczynska-Möller, A., Pickart, R. S., et al. (2018). Variability and redistribution of heat in the Atlantic Water Boundary Current north of Svalbard. J. Geophys. Res.-Oceans 123, 6373–6391. doi:10.1029/2018JC013814
Renner, M., Parrish, J. K., Piatt, J. F., Kuletz, K. J., Edwards, A. E., and Hunt Jr., G. L. (2013). Modeled distribution and abundance of a pelagic seabird reveal trends in relation to fisheries. Mar. Ecol. Prog. Ser. 484, 259–277. doi:10.3354/meps10347
Rikardsen, A. H., Righton, D., Strøm, J. F., Thorstad, E. B., Gargan, P., Sheehan, T., et al. (2021). Redefining the oceanic distribution of Atlantic salmon. Sci. Rep. 11, 12266. doi:10.1038/s41598-021-91137-y
Robinson, B., Coletti, H. A., Ballachey, B., Bodkin, J. L., Kloecker, K., Traiger, S. B., et al. (2024). Lack of strong responses to the Pacific marine heatwave by benthivorous marine birds indicates importance of trophic drivers. Mar. Ecol. Prog. Ser. 737, 215–226. doi:10.3354/meps14384
Robinson, R. A., Crick, H. Q., Learmonth, J. A., Maclean, I. M., Thomas, C. D., Bairlein, F., et al. (2009). Travelling through a warming world: climate change and migratory species. Endang. Species Res. 7, 87–99. doi:10.3354/esr00095
Rode, K. D., Douglas, D. C., Atwood, T. C., Durner, G. M., Wilson, R. E., and Pagano, A. M. (2022). Observed and forecasted changes in land use by polar bears in the Beaufort and Chukchi seas, 1985- 2040. Glob. Ecol. Conserv. 40, e02319. doi:10.1016/J.Gecco.2022.e02319
Rode, K. D., Wilson, R. R., Regehr, E. V., St. Martin, M., Douglas, D. C., and Olson, J. (2015). Increased land use by Chukchi Sea polar bears in relation to changing sea ice conditions. PLoS ONE 10, e0142213. doi:10.1371/journal.pone.0142213
Russell, R. W., Harrison, N. M., and Hunt Jr., G. L. (1999). Foraging at a front: hydrography, zooplankton, and avian planktivory in the northern Bering Sea. Mar. Ecol. Prog. Ser. 182, 77–93. doi:10.3354/meps182077
Saalfeld, S. T., Valcu, M., Brown, S., English, W., Giroux, M. A., Harrison, A. L., et al. (2024). From land to sea: the fall migration of the red phalarope through the Western Hemisphere. Mar. Ecol. Prog. Ser. 729, 1–29. doi:10.3354/meps14531
Savo, V., Morton, C., and Lepofsky, D. (2017). Impacts of climate change for coastal fishers and implications for fisheries. Fish. Fish. 18, 877–889. doi:10.1111/faf.12212
Sexson, M. G., Petersen, M. R., Breed, G. A., and Powell, A. N. (2016). Shifts in the distribution of molting spectacled eiders (Somateria fischeri) indicate ecosystem change in the Arctic. Ornithol. Appl. 118, 463–476. doi:10.1650/CONDOR-15-139.1
Shoji, A., Whelan, S., Cunningham, J. T., Hatch, S. A., Niizuma, Y., Nakajima, C., et al. (2023). Ecological niche partitioning in two Pacific puffins. Mar. Ecol. Prog. Ser. 709, 125–139. doi:10.3354/meps14282
Shuert, C. R., Hussey, N. E., Marcoux, M., Heide-Jørgensen, M. P., Dietz, R., and Auger-Méthé, M. (2023). Divergent migration routes reveal contrasting energy-minimization strategies to deal with differing resource predictability. Mov. Ecol. 11, 31. doi:10.1186/s40462-023-00397-y
Shuert, C. R., Marcoux, M., Hussey, N. E., Heide-Jørgensen, M. P., Dietz, R., and Auger-Méthé, M. (2022). Decadal migration phenology of a long-lived Arctic icon keeps pace with climate change. Proc. Natl. Acad. Sci. USA. 119, e2121092119. doi:10.1073/pnas.2121092119
Silber, G. K., and Adams, J. D. (2019). Vessel operations in the Arctic, 2015–2017. Front. Mar. Sci. 6, 573. doi:10.3389/fmars.2019.00573
Siron, R., Sherman, K., Skjoldal, H. R., and Hiltz, E. (2008). Ecosystem-based management in the Arctic Ocean: a multi-level spatial approach. Arctic 61, 86–102. doi:10.14430/arctic104
Smith, R., Hitkolok, E., Loewen, T., Dumond, A., Kristensen, K., and Swanson, H. (2022). Overwintering ecology and movement of anadromous Arctic char (Salvelinus alpinus) in a large, ice-covered river in the Canadian Arctic. J. Fish. Biol. 100, 1432–1446. doi:10.1111/jfb.15054
Spear, A., Duffy-Anderson, J., Kimmel, D., Napp, J., Randall, J., and Stabeno, P. (2019). Physical and biological drivers of zooplankton communities in the Chukchi Sea. Polar Biol. 42, 1107–1124. doi:10.1007/s00300-019-02498-0
Spencer, N. C., Gilchrist, H. G., and Mallory, M. L. (2014). Annual movement patterns of endangered ivory gulls: the importance of sea ice. PLoS ONE 9, e115231. doi:10.1371/journal.pone.0115231
Spencer, N. C., Gilchrist, H. G., Strøm, H., Allard, K. A., and Mallory, M. L. (2016). Key winter habitat of the ivory gull Pagophila eburnea in the Canadian Arctic. Endang. Species Res. 31, 33–45. doi:10.3354/esr00747
Stabeno, P. J., and Bell, S. W. (2019). Extreme conditions in the Bering Sea (2017–2018): record-breaking low sea-ice extent. Geophys. Res. Lett. 46, 8952–8959. doi:10.1029/2019GL083816
Stafford, K. M. (2019). Increasing detections of killer whales (Orcinus orca), in the Pacific Arctic. Mar. Mamm. Sci. 35, 696–706. doi:10.1111/mms.12551
Stafford, K. M., Citta, J. J., Okkonen, S. R., and Zhang, J. (2021). Bowhead and beluga whale acoustic detections in the western Beaufort Sea 2008–2018. PLoS ONE 16, e0253929. doi:10.1371/journal.pone.0253929
Stafford, K. M., Farley, E. V., Ferguson, M., Kuletz, K. J., and Levine, R. (2022). Northward range expansion of subarctic upper trophic level animals into the Pacific Arctic region. Oceanography 35, 158–166. doi:10.5670/oceanog.2022.101
Stafford, K. M., George, J. C., Harcharek, Q., and Moore, S. E. (2024). Humpback whale sightings in northern Arctic Alaska. Mar. Mamm. Sci. 40, 246–253. doi:10.1111/mms.13051
Stafford, K. M., Okkonen, S. R., and Clarke, J. T. (2013). Correlation of a strong Alaska Coastal Current with the presence of beluga whales Delphinapterus leucas near Barrow, Alaska. Mar. Ecol. Prog. Ser. 474, 287–297. doi:10.3354/meps10076
Stige, L. C., Eriksen, E., Dalpadado, P., and Ono, K. (2019). Direct and indirect effects of sea ice cover on major zooplankton groups and planktivorous fishes in the Barents Sea. ICES J. Mar. Sci. 76 (Suppl. l.), i24–i36. doi:10.1093/icesjms/fsz063
Stige, L. C., Kvile, K. Ø., Bogstad, B., and Langangen, Ø. (2018). Predator-prey interactions cause apparent competition between marine zooplankton groups. Ecology 99, 632–641. doi:10.1002/ecy.2126
Stirling, I. (1997). The importance of polynyas, ice edges, and leads to marine mammals and birds. J. Mar. Syst. 10, 9–21. doi:10.1016/s0924-7963(96)00054-1
Storrie, L., Loseto, L. L., Sutherland, E. L., MacPhee, S. A., O’Corry-Crowe, G., and Hussey, N. E. (2023). Do beluga whales truly migrate? Testing a key trait of the classical migration syndrome. Mov. Ecol. 11, 53. doi:10.1186/s40462-023-00416-y
Storrie, L., Lydersen, C., Andersen, M., Wynn, R. B., and Kovacs, K. M. (2018). Determining the species assemblage and habitat use of cetaceans in the Svalbard Archipelago, based on observations from 2002 to 2014. Polar Res. 37, 1463065. doi:10.1080/17518369.2018.1463065
Sydeman, W. J., Poloczanska, E., Reed, T. E., and Thompson, S. A. (2015). Climate change and marine vertebrates. Science 350, 772–777. doi:10.1126/science.aac9874
Szesciorka, A. R., and Stafford, K. M. (2023). Sea ice directs changes in bowhead whale phenology through the Bering Strait. Mov. Ecol. 11, 8. doi:10.1186/s40462-023-00374-5
Szesciorka, A. R., Stafford, K. M., and Berchok, C. L. (2024). Basin-wide shift in bowhead whale migration in the Pacific Arctic. Geophys. Res. Lett. 51, e2023GL1064. doi:10.1029/2023GL106416
Takahashi, A., Thiebot, J. B., Will, A., Tsukamoto, S., Merkel, B., and Kitaysky, A. (2021). Breeding together, wintering an ocean apart: foraging ecology of the northern Bering Sea thick-billed and common murres in years of contrasting sea-ice conditions. Polar Sci. 27, 100552. doi:10.1016/j.polar.2020.100552
Taylor, P. C., Boeke, R. C., Boisvert, L. N., Feldl, N., Henry, M., Huang, Y., et al. (2022). Process drivers, inter-model spread, and the path forward: a review of amplified Arctic warming. Front. Earth Sci. 9, 758361. doi:10.3389/feart.2021.758361
Tran, L., Reist, J. D., Gallagher, C. P., and Power, M. (2019). Comparing total mercury concentrations of northern Dolly Varden, Salvelinus malma malma, in two Canadian Arctic rivers 1986–1988 and 2011–2013. Polar Biol. 42, 865–876. doi:10.1007/s00300-019-02476-6
Tsjuii, K., Otsuki, M., Akamatsu, T., Amakasu, K., Kitamura, M., Kikuchi, T., et al. (2021). Annual variation of oceanographic conditions changed migration timing of bowhead whales Balaena mysticetus in the southern Chukchi Sea. Polar Biol. 44, 2289–2298. doi:10.1007/s00300-021-02960-y
Udevitz, M., Taylor, R., Garlich-Miller, J., Quakenbush, L., and Snyder, J. (2012). Potential population-level effects of increased haulout-related mortality of Pacific walrus calves. Polar Biol. 36, 291–298. doi:10.1007/s00300-012-1259-3
UNEP/CMS (2006). Migratory species and climate change: impacts of a changing environment on wild animals. Bonn, Germany: UNEP/CMS Secretariat.
Urbanski, J. A., and Litwicka, D. (2022). The decline of Svalbard land-fast sea ice extent as a result of climate change. Oceanologia 64, 535–545. doi:10.1016/j.oceano.2022.03.008
Vacquié-Garcia, J., Lydersen, C., Biuw, M., Haug, T., Fedak, M. A., and Kovacs, K. M. (2017). Hooded seal Cystophora cristata foraging areas in the Northeast Atlantic Ocean - investigated using three complementary methods. PLoS ONE 30, e0187889. doi:10.1371/journal.pone.0187889
Vacquié-Garcia, J., Lydersen, C., Ims, R. A., and Kovacs, K. M. (2018). Habitats and movement patterns of white whales Delphinapterus leucas in Svalbard, Norway in a changing climate. Mov. Ecol. 6, 21. doi:10.1186/s40462-018-0139-z
Vacquié-Garcia, J., Spitz, J., Hammill, M., Stenson, G. B., Kovacs, K. M., Lydersen, C., et al. (2024). Foraging habits of Northwest Atlantic hooded seals over the past 30 years: future habitat suitability under global warming. Glob. Change Biol. 30, e17186. doi:10.1111/gcb.17186
Vihtakari, M., Elvarsson, B. T., Treble, M., Nogueira, A., Hedges, K., Hussey, N. E., et al. (2022). Migration patterns of Greenland halibut in the North Atlantic revealed by a compiled mark–recapture dataset. ICES J. Mar. Sci. 79, 1902–1917. doi:10.1093/icesjms/fsac127
Vihtakari, M., Welcker, J., Moe, B., Chastel, O., Tartu, S., Hop, H., et al. (2018). Black-legged kittiwakes as messengers of Atlantification in the Arctic. Sci. Rep. 8, 1178. doi:10.1038/s41598-017-19118-8
von Biela, V. R., Laske, S. M., Stanek, A. E., Brown, R. J., and Dunton, K. H. (2023). Borealization of nearshore fishes on an interior Arctic shelf over multiple decades. Glob. Change Biol. 29, 1822–1838. doi:10.1111/gcb.16576
Von Duyke, A. L., Douglas, D. C., Herreman, J. K., and Crawford, J. A. (2020). Ringed seal (Pusa hispida) seasonal movements, diving, and haul-out behavior in the Beaufort, Chukchi, and Bering Seas (2011-2017). Ecol. Evol. 10, 5595–5616. doi:10.1002/ece3.6302
Watt, C. A., Orr, J., and Ferguson, S. H. (2016). A shift in foraging behaviour of beluga whales Delphinapterus leucas from the threatened Cumberland Sound population may reflect a changing Arctic food web. Endang. Species Res. 31, 259–270. doi:10.3354/esr00768
Weingartner, T. J., Danielson, S., Sasaki, Y., Pavlov, V., and Kulakov, M. (1999). The Siberian Coastal Current: a wind- and buoyancy-forced Arctic coastal current. J. Geophys. Res. 104, 29697–29713. doi:10.1029/1999JC900161
Welch, H. E., Bergmann, M. A., Siferd, T. D., Martin, K. A., Curtis, M. F., Crawford, R. E., et al. (1992). Energy flow through the marine ecosystem of the Lancaster Sound Region, Arctic Canada. Arctic 45, 343–357. doi:10.14430/arctic1413
Welch, H. E., Crawford, R. E., and Hop, H. (1993). Occurrence of Arctic cod (Boreogadus saida) schools and their vulnerability to predation in the Canadian High Arctic. Arctic 46, 331–339. doi:10.14430/arctic1361
Whiting, A., Castellote, M., Small, R. J., Frost, K. J., and Suydam, R. (2020). Unexpected mid-winter presence of harbor porpoises (Phocoena phocoena) in Kotzebue Sound, Alaska. Mar. Mamm. Sci. 36, 354–358. doi:10.1111/mms.12641
Will, A., Takahashi, A., Thiebot, J., Martinez, A., Kitaiskaia, E., Britt, L., et al. (2020). The breeding seabird community reveals that recent sea ice loss in the Pacific Arctic does not benefit piscivores and is detrimental to planktivores. Deep Sea Res. II 181, 104902. doi:10.1016/j.dsr2.2020.104902
Witting, L. (2023). Huge narwhal displacement following shipping of iron ore in the Canadian Arctic Archipelago. Prepr. bioRxiv, 1–18. doi:10.1101/2023.05.29.542701
WMAC and Aklavik HTC (2018). Wildlife management advisory Council (North Slope and Aklavik hunters and trappers committee). Inuvialuit traditional knowledge of wildlife habitat. Whitehorse, USA: North Slope Wildlife Management Advisory Council.
Woehler, E. J., and Hobday, A. J. (2024). Impacts of marine heatwaves may be mediated by seabird life history strategies. Mar. Ecol. Prog. Ser. 737, 9–23. doi:10.3354/meps14333
Wong, C., Ballegooyen, K., Ignace, L., Johnson, M. J., and Swanson, H. (2020). Towards reconciliation: 10 Calls to Action to natural scientists working in Canada. FACETS 5, 769–783. doi:10.1139/facets-2020-0005
Yamamoto, T., Hoshina, K., Nishizawa, B., Meathrel, C. E., Phillips, R. A., and Watanuki, Y. (2015). Annual and seasonal movements of migrating short-tailed shearwaters reflect environmental variation in sub-Arctic and Arctic waters. Mar. Biol. 162, 413–424. doi:10.1007/s00227-014-2589-1
Yano, K., Stevens, J. D., and Compagno, L. J. V. (2004). A review of the systematics of the sleeper shark genus Somniosus with redescriptions of Somniosus (Somniosus) antarcticus and Somniosus (Rhinoscymnus) longus (Squaliformes: somniosidae). Ichthyol. Res. 51, 360–373. doi:10.1007/s10228-004-0244-4
Yua, E., Raymond-Yakoubian, J., Aluaq, D. R., and Behe, C. (2022). A framework for co-production of knowledge in the context of Arctic research. Ecol. Soc. 27, 34. doi:10.5751/ES-12960-270134
Zimmerman, C. E., Ramey, A. M., Turner, S. M., Mueter, F. J., Murphy, S., and Nielsen, J. L. (2013). Genetics, recruitment, and migration patterns of Arctic cisco (Coregonus autumnalis) in the Colville River, Alaska, and Mackenzie River, Canada. Polar Biol. 36, 1543–1555. doi:10.1007/s00300-013-1372-y
Keywords: Arctic, distributional shift, fish migrations, marine mammal migrations, phenology, sea-ice loss, seabird migrations, subsistence harvest
Citation: Kuletz KJ, Ferguson SH, Frederiksen M, Gallagher CP, Hauser DDW, Hop H, Kovacs KM, Lydersen C, Mosbech A and Seitz AC (2024) A review of climate change impacts on migration patterns of marine vertebrates in Arctic and Subarctic ecosystems. Front. Environ. Sci. 12:1434549. doi: 10.3389/fenvs.2024.1434549
Received: 18 May 2024; Accepted: 23 September 2024;
Published: 25 October 2024.
Edited by:
Per Fauchald, Norwegian Institute for Nature Research (NINA), NorwayReviewed by:
David Ainley, H.T. Harvey and Associates, United StatesLori Quakenbush, Alaska Department of Fish and Game, United States
Copyright © 2024 Kuletz, Ferguson, Frederiksen, Gallagher, Hauser, Hop, Kovacs, Lydersen, Mosbech and Seitz. This is an open-access article distributed under the terms of the Creative Commons Attribution License (CC BY). The use, distribution or reproduction in other forums is permitted, provided the original author(s) and the copyright owner(s) are credited and that the original publication in this journal is cited, in accordance with accepted academic practice. No use, distribution or reproduction is permitted which does not comply with these terms.
*Correspondence: Katherine J. Kuletz, Kathy_kuletz@fws.gov