- 1Arctic and Aquatic Research Division, Fisheries and Oceans Canada, Winnipeg, Canada
- 2Department of Arctic and Marine Biology, UiT - The Arctic University of Norway, Tromsø, Norway
- 3Greenland Climate Research Centre, Greenland Institute of Natural Resources, Nuuk, Greenland
- 4Department of Biology, Arctic Research Centre, Aarhus University, Aarhus, Denmark
- 5Takuvik International Research Laboratory, Laval University (Canada) and CNRS (France), Département de biologie and Québec-Océan, Université Laval, Québec City, QC, Canada
The polymer-facilitated flux of ice algae on Arctic shelves can initiate benthic activity and growth after the nutritionally constrained winter period. Lipid-rich ice algae are readily consumed by benthos and those entering the sediment can benefit deposit feeders. Ice algae assimilated by benthic organisms cascade up multiple trophic levels within the benthic sub-web, re-entering the pelagic sub web through habitat coupling species. Pelagic predators can have significant ice-algal carbon signals obtained from the benthic compartment. Sympagic-pelagic-benthic coupling on Arctic shelves is expected to weaken with ongoing sea-ice change. This review discusses the phenology, quantity, and quality of ice-algal contributions to coupling, linked to thinning snow and ice cover including multi-year ice replacement. Predicting future coupling between marine sub-webs requires focused research that considers trophic markers of multiple carbon sources.
1 Introduction
Sympagic-pelagic-benthic (SPB) coupling has a key role in the functioning of pelagic and benthic compartments of Arctic marine ecosystems including the trophic interactions of species (i.e., sub-web) within each compartment (Søreide et al., 2013; Wassmann et al., 2020; Amiraux et al., 2023a). This coupling can support the benthic sub-web for two reasons: i) the vertical export of Arctic primary production is highly pulsative, which can limit degradation processes within the pelagic zone, and ii), over >50% of the Arctic marine area consists of shallow Arctic shelves (e.g., <200 m) where coupling occurs more direct than in deep areas. On the Arctic shelves, ice algae grow attached to and within all ice types, first year (FYI) and multi-year ice (MYI) as well as land fast and pack ice. Sympagic production rapidly transitions from a heterotrophic to a diatom-dominated autotrophic community following the end of the polar night (Mundy and Meiners, 2021; Marquardt et al., 2023). Under scenarios of Arctic environmental change, the export of ice algae to the benthos is expected to be less efficient as a greater proportion of organic matter is predicted to be retained within the water column thereby weakening SPB coupling (Wassmann et al., 2011).
Herein, we present recent (primarily post 2010) assessments of ice algae supply and current insights into the benthic use of ice algae in a time of sea ice change (Figure 1). We acknowledge the importance of ice algae for pelagic diets, including for key species of copepods (Kohlbach et al., 2016; Kohlbach et al., 2021; Koch et al., 2023) and pelagic higher trophic levels such as certain seals (Kunisch et al., 2021; Carlyle et al., 2022) and polar bears (Brown et al., 2018). In this review, we primarily focus on the contributions of ice algae to the benthic sub-web, and the less frequently considered reintroduction of ice algal-derived organic carbon back into the pelagic sub-web. There are significant changes occurring in the Central Arctic Ocean in regards to sea ice and productivity, however, for the purpose of this review, we have chosen to focus on the highly productive shelf seas of the Arctic where there is relatively strong SPB coupling and sea-ice dynamics are shifting rapidly.
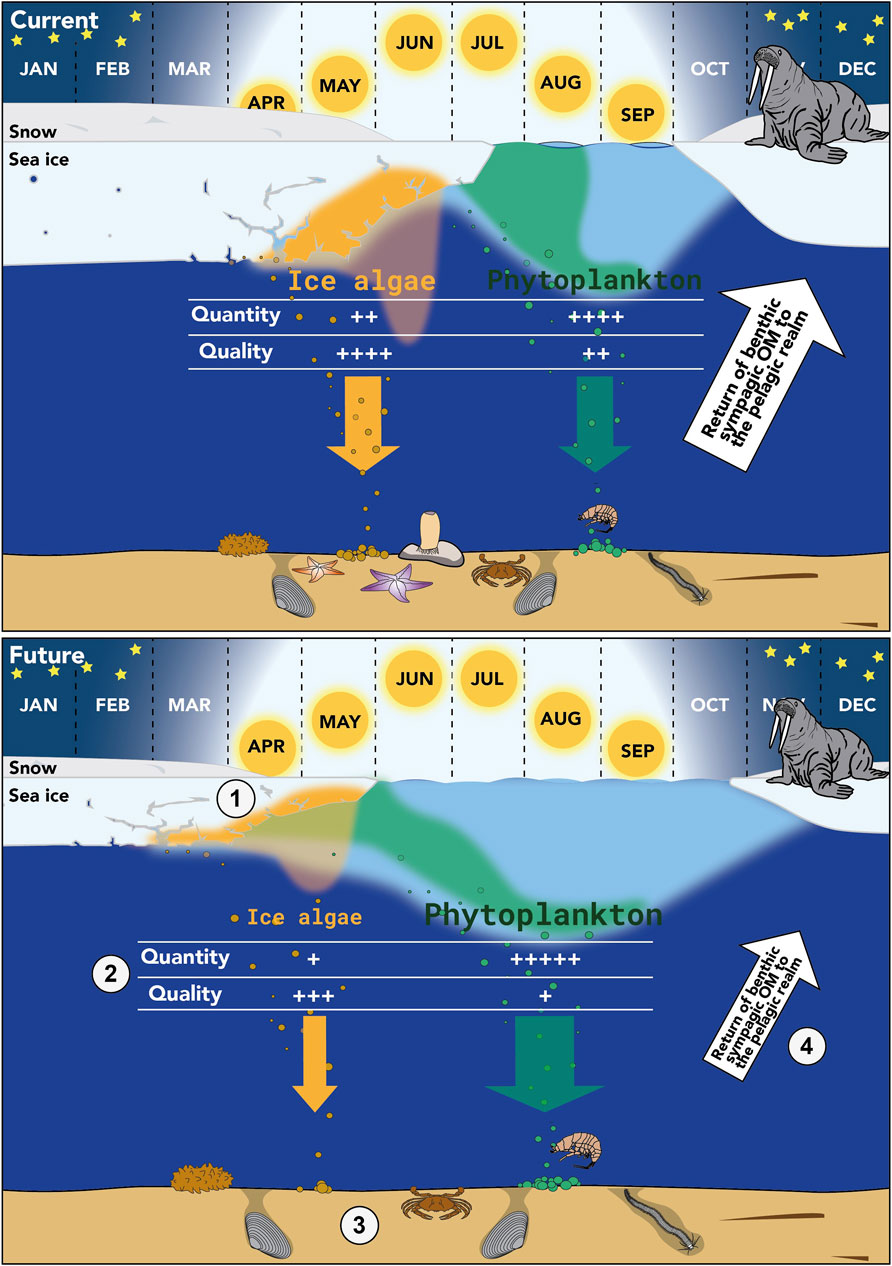
Figure 1. In this scenario, future conditions are driven by thinning snow and ice cover that allows light conditions suitable for ice algal and under-ice primary production to occur earlier in the year. Orange and green shading represent ice algal and phytoplankton, respectively, in the euphotic zone (light-blue shading). Numbering is related to the following expected mechanisms of change: 1) increased light availability early in the spring allows for potential co-occurrence of ice algal and under-ice phytoplankton blooms with diminished strength of the spring ice-edge phytoplankton bloom, 2) truncated ice algal bloom could reduce supply (quantity) relative to phytoplankton, and fresher/warmer surface waters could increase algal degradation in the water column reducing the quality of the downward flux, 3) different food sources altering community structure and function, and 4) overall weakened connection between benthic and pelagic food webs due to altered benthic structure.
2 Ice algae supply – dynamics in the ice and pelagic compartments
2.1 Sea-ice compartment
Ice algae represent a variable and relatively low contribution to total annual net primary production (phytoplankton + ice algae) on productive Arctic shelves in recent periods of sea-ice change (1%–26%, Legendre et al., 1992; Payne et al., 2021). However, the contribution of ice algae to total primary production on Arctic shelves can be significantly higher early in the season when the ice algal bloom occurs prior to an under-ice or spring phytoplankton bloom (e.g., Gradinger, 2009; Hegseth and von Quillfeldt, 2022). From this early season perspective, ice algae can be the primary source of energy for pelagic and benthic consumers and their associated predators (e.g., nearshore amphipods and benthic juvenile polychaetes) (Gradinger and Bluhm, 2010; McConnell et al., 2012; Pitusi et al., 2023). Overall, the relative contribution of ice algal production remains poorly constrained as it cannot be remotely assessed at meaningful seasonal and spatial scales (Lalande et al., 2019; Figure 2).
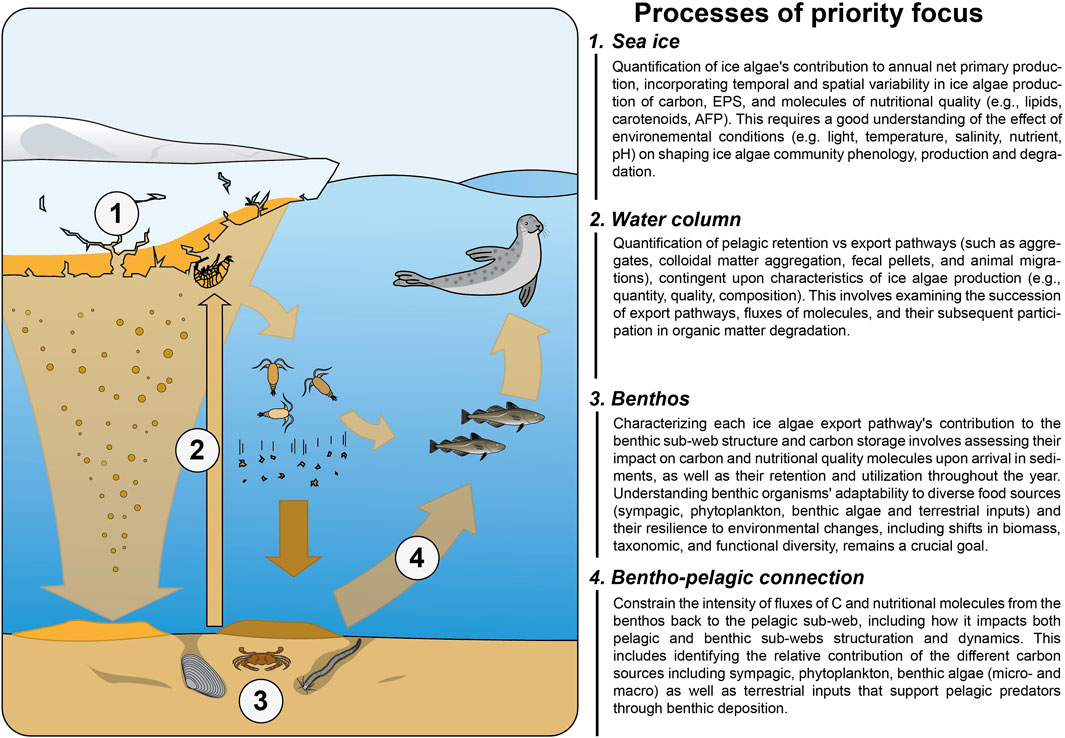
Figure 2. Conceptual diagram depicting ice algal processes requiring investigation to understand both the present and future significance of ice algae in SPB coupling. Research needs are provided for the sea ice, water column and benthos compartments as well as the re-entry of ice algae to the pelagic compartment (benthos-pelagic connection).
In the sea ice, ice algae are highly concentrated in the bottom 3–10 cm at the ice-water interface and are easily accessible for ice-associated grazers. During the bloom period the ice algal community consists of diatom-dominated assemblages rich in cellular levels of long chain polyunsaturated fatty acids (LC-PUFA) and antioxidant carotenoids (Harwood, 2019; Amiraux et al., 2021a; Amiraux et al., 2022). Ice algal cell quality is sustained by physiological and metabolic adaptations that maintain favorable micro-environments for growth in the ice (Boetius et al., 2015), and through the release of exopolymeric substances (EPS: polysaccharides released by ice algae that accumulate as gels around the cells, Krembs et al., 2002; Riedel et al., 2006) that limit abiotic and biotic degradation of the ice algae. The contribution of ice algae to pelagic and benthic sub-webs may, hence, be best represented by measurements of both biomass and diatom composition, rather than production alone (Lalande et al., 2021).
The quantity of ice algae produced in and released from the ice is expected to increase on Arctic shelves with sea-ice change (Leu et al., 2015; Tedesco et al., 2019; Wassmann et al., 2020). Two climate-related processes, driven by the same mechanism are mainly responsible for that. First, replacement of MYI by FYI, and second, an increase in the number of ice algae growing days. Both processes are mechanistically driven by improved light transmission due to thinner snow-on-ice cover and thinner ice (Lim et al., 2022; Figure 1) that facilitates, a) increased growth of ice algae where previously there was expected light limitation, and b) an earlier start of ice algae growth. Light transmittance represents the amount of photosynthetically active radiation (PAR) reaching ice algae after considering the amount of incoming solar radiation as influenced by atmospheric attenuation and Arctic seasonality (i.e., photoperiod), and loss factors related to different snow and ice properties. Very low light transmission (i.e., 0.014%; Lange et al., 2017, or 0.17 µmol photons m−2 s−1; Hancke et al., 2018) is required for ice algal growth, highlighting their value as a carbon source beyond when phytoplankton are capable of growth.
2.1.1 Multi-year ice replacement
Seasonal and spatial studies of sympagic communities (Leu et al., 2015; Mundy and Meiners, 2021) and modelling of sympagic primary production under climate change scenarios (e.g., Tedesco et al., 2012; Tedesco et al., 2019; Wassmann et al., 2020; Payne et al., 2021) all indicate increased ice algae supply with MYI replacement and eventual complete MYI loss. This shift has already taken place in many locations including the Chukchi Sea shelf (Payne et al., 2021), currently exceeding ice algae lost due to overall decreases in sea ice extent (Lannunzel et al., 2020; Lim et al., 2022). However, comparative field measurements from the Lincoln Sea shelf found similar ice algal biomass, nutritional quality, and net community production at the bottommost layer of MYI and FYI on Arctic shelves (Lange et al., 2015; Kohlbach et al., 2020; Campbell et al., 2022a) such that on the last remaining MYI-dominated shelf region, the Lincoln Sea, MYI replacement may result in little to no future increase in ice algae supply (Lange et al., 2017; Lange et al., 2019; Campbell et al., 2022b) and controlling factors other than light transmission may be highly relevant in this Arctic shelf region. The extent to which ice algal supply increases requires further research. For example, the photophysiology of ice-algal species could result in a shift in ice-algal community composition, resulting in decreased ice algal quality, rather than increased supply with MYI replacement on the Lincoln Sea shelf (Campbell et al., 2022a; Figure 2).
In pack ice with increasing dominance of FYI, ice algal supply could be affected by increased variability of snow cover on FYI relative to MYI (Lange et al., 2019) as well as increased wind-driven formation of ridges or infiltration layers (Fernández-Méndez et al., 2018; Granskog et al., 2020). Increased wind-driven lead formation has been hypothesized to favor ice algal supply from new ice. However, colonizing species (i.e., inoculum), potentially originating from a dwindling MYI source (Olsen et al., 2017), may not be available for new ice production, or the species that are available may not photoacclimate to the high-light conditions of the new ice (Kauko et al., 2017; Kauko et al., 2019; Campbell et al., 2022a).
2.1.2 Increased growing days
The duration (i.e., number of growing days) of ice algae production is a critical factor to understand variability in the quantity and timing of ice algal supply for SPB coupling (e.g., Beaufort Sea shelf, Dezutter et al., 2019; Dezutter et al., 2021; Nadaï et al., 2021, Chukchi Sea shelf, Selz et al., 2018). This duration varies over geographical scales and relates to sea ice decline. It also relates to snow cover as illustrated in pack ice of the Chukchi Sea shelf where ice algal growth started in mid-May and a growth duration of 70 days was recorded under low snow cover (0.02 m). Nearby, in contrast, growth was delayed by 4 weeks and the magnitude of the ice algal bloom was lower and shortened under high snow cover (0.4 m; Hill et al., 2022). These observations support expectations for a longer growth period and consequently higher ice algal biomass as the Arctic ice snow pack becomes thinner reducing light limitation early in the spring (Wassmann et al., 2020). Currently, however, increased ice algae supply due to overall earlier/longer growing season is not strongly apparent. Between 1985 and 2018, days suitable for ice algal growth (see Lim et al., 2022 for details) are estimated to have increased by ≤ 2.5 days decade−1 for the Chukchi, Beaufort and Kara seas, and decreased by < 2 days decade−1 for the Barents Sea and Baffin Bay. In the Barents, a shallow seasonally ice-covered shelf sea, any benefits of thinning ice and snow allowing for earlier ice algal growth may have already been erased by early snow or ice melt that precedes or truncates the ice algal bloom (Leu et al., 2015; Onarheim and Årthun, 2017).
If increased light transmission allows for a longer ice algal growth period, nutrient limitation could be an increasingly limiting factor for ice algal supply and quality (Hill et al., 2022). Gravity drainage during ice growth, turbulent diffusion (Duarte et al., 2022; Hill et al., 2022), and forced convection from tides in coastal regions (e.g., Kitikmeot Sea, Dalman et al., 2019) could contribute to relieving nutrient limitation thereby supporting higher ice algal supply for SPB coupling. The resuspension of remineralized nutrients induced by strong tidal mixing could also reduce nutrient limitation in surface waters of the shallow Siberian shelf (Lalande et al., 2014).
Increasing light and heat transmission will also affect ice algal growth at the scale of brine channels. The warming Arctic air (Rantanen et al., 2022) and subsequent warming of snow and ice will change bulk ice properties (e.g., brine volume and bulk salinity; Wang et al., 2020; Wang et al., 2023) altering connections between brine channels and the distribution of habitable space within the sea ice (Leu et al., 2015; Mundy and Meiners, 2021). For example, in land fast FYI of western Baffin Oziel et al. (2019) detected unexpected homogenous vertical profiles of ice brine, potentially due to an early warming and episodic snow melt event that altered brine volume for the rest of the season. Episodic and seasonal warming could thus affect both habitable space and drive earlier termination of the ice algal bloom (Mundy and Meiners, 2021).
2.2 Pelagic compartment
The release of ice algae into the pelagic compartment can typically be detected from February to July on Arctic shelves (Juul-Pedersen et al., 2008; Lalande et al., 2020; Dezutter et al., 2021; Bodur et al., 2023). Peak periods of release and the eventual complete sloughing of ice algae coincide with snow and ice melt (Oziel et al., 2019; Lim et al., 2022; Figure 1A). With continued Arctic change, it is possible that the number of flux events, prior to complete sloughing, could increase due to temperature fluctuations that lead to intermittent snow melt events (e.g., as observed by Oziel et al., 2019 in western Baffin Bay). SPB coupling is expected to weaken due to shorter periods of intense flux (e.g., on Pacific Arctic shelves, Lalande et al., 2021) and increased recycling of material during flux (Fadeev et al., 2021; Koch et al., 2020a; Dybwad et al., 2022; Figure 1B). However, on Arctic shelves where seasonal, and in particular, land fast ice persist, the strength of SPB coupling has not currently been diminished (Kohlbach et al., 2019; Cautain, et al., 2022).
The flux of intact and repackaged (e.g., fecal pellets) ice algae to the seafloor is mediated by herbivorous meiofauna within the sea ice, grazers immediately under the ice that can access ice algal cells within the bottom ice skeletal layer, and further grazing within the epipelagic and deep waters. These grazers can use the lipid-rich ice algae to help fill their polar-night depleted lipid stores and synthesize membranes composed of polar lipids (Leu et al., 2011; Kunisch et al., 2023). Grazing impact by under-ice/pelagic herbivorous fauna is higher than by sea-ice meiofauna (Kohlbach et al., 2016; Gradinger and Bluhm, 2020; Ehrlich et al., 2021). Coupling to pelagic grazers rather than directly to the benthos is dependent on zooplankton abundance at the time of ice algae release (i.e., potential mismatches, Dezutter et al., 2019; Nadaï et al., 2021) and zooplankton species composition (Ehrlich et al., 2020). Pelagic zooplankton can include meroplankton (i.e., planktonic early life stages of benthic invertebrates) such that sympagic support of benthic species is not limited to processes occurring in the benthic compartment. Meroplankton in the water column can graze on ice algae, benefiting the benthos during these critical early benthic life stages (Stübner et al., 2016; Dezutter et al., 2019).
From multi-year studies with variable sea-ice conditions, it is clear that under-ice and pelagic grazers can take advantage of early ice melt and ice algal release, supporting earlier development of copepod nauplii (Søreide et al., 2010; Søreide et al., 2013; Dezutter et al., 2021). Consequently, for an early ice algae release to successfully bypass pelagic grazers, the timing must extend beyond the natural reproductive and feeding plasticity of the pelagic grazers. It is expected that access to ice algae will continue to influence copepods as a trophic nexus for Arctic food web function (Renaud et al., 2018; Ershova et al., 2021), as pulses of both ice algal and phytoplankton production are critical for zooplankton growth and reproduction (Søreide et al., 2010; Kohlbach et al., 2022; Koch et al., 2023).
When ice algae arrive at the seafloor it is generally of higher quality relative to the phytoplankton flux (McMahon et al., 2006; Figure 1A). The pulsative, rapid, flux of ice algae and associated exopolymeric substances at the time of snow or ice melt (Amiraux et al., 2021a; Salter et al., 2023) can evade grazers (Fadeev et al., 2021; Nadaï et al., 2021) and limit degradation processes within the pelagic zone. Consequently, this material provides substantial nutritional contributions to the Arctic benthic sub-web (Juul-Pedersen et al., 2008; Kohlbach et al., 2019; Yunda-Guarin et al., 2020; Amiraux et al., 2021b). The polymer-facilitated sinking of ice algae beyond the euphotic zone limits abiotic (light-induced) degradation of their cellular compounds. Additionally, the presence of exopolymeric substances around ice algae restricts remineralization by bacteria whose remineralization efficiencies are higher than bacteria in the sea ice compartment (Amiraux et al., 2017; Amiraux et al., 2021b; Rontani et al., 2022).
3 Fate of ice algae in the benthic compartment
3.1 Ice algae use in the benthic compartment
The deposition of ice algae during ice melt allows for the intake of carbon that initiates benthic growth and reproduction after the nutritionally constrained winter period (North et al., 2014). Individual diatom cells, ice-algal aggregates, and ice algae in zooplankton fecal pellets that enter the benthic sub-web during the ice algal bloom can invoke rapid benthic feeding responses due to the early accessibility, concentrated deposition, and the high quality of the ice algal material (Renaud et al., 2007; Amiraux et al., 2021a).
During the ice-covered spring on Arctic shelf areas that have long periods of land fast ice coverage, sea ice-derived carbon can contribute a substantial portion (e.g., >75%,) of the benthic carbon pool in both invertebrates and sediments (Kohlbach et al., 2019; Yunda-Guarin et al., 2020). The high quality of ice algae means they are readily consumed and assimilated by the Arctic benthos (McMahon et al., 2006; Amiraux et al., 2021a). Yet, Arctic shelves can have sedimentary stores of sea-ice carbon created during peak ice algal flux (Koch et al., 2023). Although there is limited information about ice algal storage in sediments of Arctic shelves (Figure 2), sediment samples from the southeast Beaufort Sea shelf indicate the presences of ice associated carbon within the upper 70 mm of sediment, with sedimentation rates of ca. 1 mm y−1. This indicates that burrowing infauna could have year-round access to sea-ice carbon deposited from the last century (Koch et al., 2023). The quality of this ice algal source requires further study.
The responsiveness of benthic organisms to ice algae varies, even among taxa with similar feeding behaviors. Amiraux et al. (2021a) showed that, despite both bivalves, Serripes groenlandicus and Mya truncate, being slow-moving burrowing suspension feeders, their responsiveness to settling primary production differs due to their distinct bioturbation affinities. Unlike M. truncata, which induces diffusive mixing bioturbation (Lacoste et al., 2018) and is expected to ingest a variable and non-negligible quantity of buried organic matter, S. groenlandicus solely consumes settling organic matter and exhibits a high responsiveness to these inputs. Therefore, since S. groenlandicus enables monitoring of SPB coupling, it is regarded as a sentinel of this process (Amiraux et al., 2021a).
Differences in the strength of ice algal signals exist between benthic feeding types as suspension feeders are less reliant on ice algae than deposit feeders (both surface and subsurface; Koch et al., 2020b), likely because the latter can use sediment that may be a reservoir of ice algae material throughout the year. Benthic detritivores can also benefit from utilizing sediments with stores of ice algal material (Amiraux et al., 2021b; Rontani et al., 2022). However, this benefit applies almost exclusively to ice algae exported in aggregate form during peak periods of flux that allow for sequestration/burial. The quality of ice algae exported in fecal pellet form undergoes significant reduction due to grazing and the presence of bacteria from zooplankton, rather than from sea ice, which can effectively degrade the fecal pellet material during sinking (Burot et al., 2021).
3.2 Re-entry of ice algae from the benthic to the pelagic compartment
On Arctic shelves, pelagic and benthic sub-webs share similar structures and complexities, encompassing three to five trophic levels and featuring their respective top predators (Iken et al., 2010; Stasko et al., 2018; Amiraux et al., 2023a). Therefore, the sympagic carbon assimilated by lower trophic level benthic organisms, including deposit or filter feeders, can cascade up multiple trophic levels within the benthic sub-web (Yurkowski et al., 2020; Koch et al., 2021; Amiraux et al., 2023b).
Pelagic and benthic sub-webs are closely linked through habitat coupling species. These species serve as bridges between compartments, facilitating the transfer of organic carbon, including sympagic material, from benthic to pelagic organisms and vice versa. Habitat couplings arises from mobile, opportunistic species (Baustian et al., 2014), which in the Arctic include, but are not limited to, walrus feeding on benthic bivalves (Fisher and Stewart, 1997; Ray et al., 2006; Mann et al., 2020), grey whales feeding primarily on benthic prey in the Arctic (Stewart et al., 2023), the Arctic finned octopods, shorthorn sculpin and Greenland halibut (demersal fish) feeding on both benthic and pelagic prey (Giraldo et al., 2018; Landry et al., 2018; Golikov et al., 2023), as well as numerous mobile invertebrates such as cumaceans and amphipods. Sympagic carbon that moves through the benthic sub-web can re-enter the pelagic sub-web at various trophic levels, ultimately reaching pelagic apex predators. Evidence for this was given by Amiraux et al. (2023a) who demonstrated that the relatively high dependence of Arctic marine mammals (53% ± 22% of body carbon) on sympagic carbon in the coastal area of Southampton Island (Hudson Bay, Nunavut) results from their direct or indirect (trophic cascade) use of sympagic carbon from the benthic compartment.
This re-entry of ice algae to the pelagic sub-web can vary over time and latitude. For example, despite a sea ice decline of 18% in both mid- and high-latitudes Canadian Arctic shelves (Foxe Basin and Jones Sound, respectively) from 1982 to 2016, Yurkowski et al. (2020) found that walrus sympagic carbon presence, resulting from a benthivorous diet, remained high (e.g., 89%–98%) at high latitudes but had decreased by 75% at mid-latitudes. For these walrus, ice algae dependency has already weakened at the lower latitudes such that further ice decline, reducing ice algal supply, could have a greater impact on the high latitude walrus. Decoupling from ice algae is not necessarily detrimental for higher trophics relying on the benthic compartment for their diet due to adaptability facilitated by flexible habitat use or migration.
4 Future perspectives for the benthic sub-web
Ice algae currently contribute to the growth and reproduction of species in the pelagic and benthic sub-webs. Therefore, it is reasonable to expect that any decline or shift in ice algae supply, including shifts in quality, could induce a restructuring of Arctic food webs (Moore and Stabeno, 2015). This restructuring will be driven by directional regulations within the Arctic marine ecosystem. Variations in ice algae supply will exert an influence on the benthic sub-web through a bottom-up regulatory process. This influence will propagate upward, impacting the benthic top-down controls, potentially initiating trophic feedback within the pelagic realm. Although not yet documented, less ice algae within the benthic sub web could ultimately impact benthic predators (e.g., sea stars, Amiraux et al., 2023b), thereby reducing predation pressure on benthic prey that support pelagic predators (i.e., bivalves for walrus, Yurkowski et al., 2020).
Arctic fauna can rely on multiple carbon sources including ice algae, phytoplankton, benthic algae and terrestrial inputs (Bell et al., 2016; Gaillard et al., 2017; Harris et al., 2018). Omnivory and trophic plasticity contribute to the stability of a food web in the event of changing food supply (Bridier et al., 2021; Yunda-Guarin et al., 2022; Yunda-Guarin et al., 2023). Therefore, while not all benthic species may react equally (Bridier et al., 2023), it is probable that many species will be able to adapt to changing ice algal supply and organic matter quality. The Arctic’s complex food webs highlight the adaptability of Arctic benthic species to diverse food sources that are supplied in abundance (Wei et al., 2020). However, sea ice change and the potential decrease in SPB coupling efficiency (Zhulay et al., 2023), will inevitably change the composition of food sources, potentially impacting benthic community functioning, including endemic species (Figure 1B). These assumptions are supported by Yunda-Guarin et al. (2023), showing that a diversity of food resources facilitate the development of epibenthic communities characterized by a more complex structure (more trophic levels) and higher interconnectedness (larger individual niche size) compared to trophic structures based on lower food diversity. The extent to which benthic biodiversity and/or biomass responds to changing food source diversity and shifting predator-prey interactions in different regions of Arctic shelves requires further, mechanistic study (Figure 2).
5 Conclusion
The impact of global warming on ice algae supply has already been observed in many Arctic regions, and future changes in ice-algal production will be closely linked to snow cover and ice thickness. These factors influence the availability of light for ice algal growth, affecting the onset and duration of the bloom, community composition, succession, degradation from both abiotic (light-induced, autoxidative) and biotic (bacterial mineralization, zooplankton grazing) processes and ultimately nutritional quality of the ice algae. Consequently, the quantitative and qualitative contribution of ice algae to SPB coupling has and will be altered. Whether and how this anticipated loss for the benthos gets compensated for is rather unclear, though recent work suggest that other carbon sources such as microphytobenthos, macroalgae and terrestrial carbon may play increasing roles on Arctic shelves (Krause-Jensen et al., 2020; Attard et al., 2024).
Given the pivotal role of light as an abiotic driver of SPB coupling, there is a current imperative for research focused on identifying changes in ice algae production, degradation, and quality in response to light-driven phenology (Figure 2). This involves examining morphological aspects like algal cell size and shape, as well as physiological traits such as exopolymer production. These characteristics are influenced by microalgal phenology and contribute to the sinking of algal material out of the euphotic zone. Additionally, the export pathways should be better constrained through a deeper understanding of the contribution of ice algae export from aggregates, fecal pellets, migrations and heteroaggregation of the colloidal fraction (Figure 2). Furthermore, exploring lipid content throughout ice algal succession, including variations in type and quantity, and examining how both abiotic and biotic processes influence these contents, is crucial in determining the flux of high-quality organic matter to the benthic environment.
In the benthic compartment, a primary focus should center on understanding the spatial and temporal variability of ice algal contribution compared to other carbon sources (Figure 2). This understanding could be advanced by studies of species acting as sentinels for the SPB coupling, along with a comprehensive investigation of their trophic links. Moreover, it is imperative to improve quantification of how changes in ice algae, encompassing both quantity and quality, will impact the trophic structure and functioning of the benthic sub-web and, consequently, extend back to the pelagic sub-web. We suggest that the resolution of such a challenge should rely on a multitude of trophic markers (e.g., fatty acids, highly branched isoprenoids, stable isotopes) and approaches (e.g., quantification of ice algae-derived carbon, isotopic niche and redundancy) as well as using integrating models (e.g., Bayesian models) to comprehensively assess the current and future contribution of ice algae and alternate carbon sources to the Arctic marine ecosystems.
Author contributions
AN: Conceptualization, Data curation, Funding acquisition, Project administration, Writing–original draft, Writing–review and editing. BB: Conceptualization, Writing–review and editing. TJ-P: Writing–original draft, Writing–review and editing. DK: Writing–review and editing. MR: Writing–review and editing. DS: Writing–review and editing. RA: Conceptualization, Visualization, Writing–original draft, Writing–review and editing.
Funding
The author(s) declare that no financial support was received for the research, authorship, and/or publication of this article.
Conflict of interest
The authors declare that the research was conducted in the absence of any commercial or financial relationships that could be construed as a potential conflict of interest.
The reviewer CK declared a past co-authorship with the authors DK and RA to the handling editor.
Publisher’s note
All claims expressed in this article are solely those of the authors and do not necessarily represent those of their affiliated organizations, or those of the publisher, the editors and the reviewers. Any product that may be evaluated in this article, or claim that may be made by its manufacturer, is not guaranteed or endorsed by the publisher.
References
Amiraux, R., Archambault, P., Moriceau, B., Lemire, M., Babin, M., Memery, L., et al. (2021a). Efficiency of sympagic-benthic coupling revealed by analyses of n-3 fatty acids, IP25 and other highly branched isoprenoids in two filter-feeding Arctic benthic molluscs: Mya truncata and Serripes groenlandicus. Org. Geochem. 151, 104160. doi:10.1016/j.orggeochem.2020.104160
Amiraux, R., Belt, S. T., Vaultier, F., Galindo, V., Gosselin, M., Bonin, P., et al. (2017). Monitoring photo-oxidative and salinity-induced bacterial stress in the Canadian Arctic using specific lipid tracers. Mar. Chem. 194, 89–99. doi:10.1016/j.marchem.2017.05.006
Amiraux, R., Bonin, P., Burot, C., and Rontani, J.-F. (2021b). Use of stress signals of their attached bacteria to monitor sympagic algae preservation in Canadian Arctic Sediments. Microorganisms 9, 2626. doi:10.3390/microorganisms9122626
Amiraux, R., Lavaud, J., Cameron-Bergeron, K., Matthes, L. C., Peeken, I., Mundy, C. J., et al. (2022). Content in fatty acids and carotenoids in phytoplankton blooms during the seasonal sea ice retreat in Hudson Bay complex, Canada. Elem. Sci. Anthr. 10, 00106. doi:10.1525/elementa.2021.00106
Amiraux, R., Mundy, C., Pierrejean, M., Niemi, A., Hedges, K. J., Brown, T. A., et al. (2023a). Tracing carbon flow and trophic structure of a coastal Arctic marine food web using highly branched isoprenoids and carbon, nitrogen and sulfur stable isotopes. Ecol. Indic. 147, 109938. doi:10.1016/j.ecolind.2023.109938
Amiraux, R., Yurkowski, D. J., Archambault, P., Pierrejean, M., and Mundy, C. (2023b). Top predator sea stars are the benthic equivalent to polar bears of the pelagic realm. Proc. Natl. Acad. Sci. 120, e2216701120. doi:10.1073/pnas.2216701120
Attard, K., Singh, R. K., Gattuso, J. P., Filbee-Dexter, K., Krause-Jensen, D., Kühl, M., et al. (2024). Seafloor primary production in a changing Arctic Ocean. Proc. Natl. Acad. Sci. 121, e2303366121. doi:10.1073/pnas.2303366121
Baustian, M., Hansen, G. A., De Kluijver, A., Robinson, K., Henry, E., Knoll, L., et al. (2014). Linking the bottom to the top in aquatic ecosystems: mechanisms and stressors of benthic-pelagic coupling. Eco-DAS X Symp. Proc. 3, 25–47. (Association for the Sciences of Limnology and Oceanography). doi:10.4319/ecodas.2014.978-0-9845591-4-5.38
Bell, L. E., Bluhm, B. A., and Iken, K. (2016). Influence of terrestrial organic matter in marine food webs of the Beaufort Sea shelf and slope. Mar. Ecol. Prog. Ser. 550, 1–24. doi:10.3354/meps11725
Bodur, Y. V., Renaud, P. E., Goraguer, L., Amargant-Arumí, M., Assmy, P., Dąbrowska, A. M., et al. (2023). Seasonal patterns of vertical flux in the northwestern Barents Sea under Atlantic Water influence and sea-ice decline. Prog. Oceanogr. 219, 103132. doi:10.1016/j.pocean.2023.103132
Boetius, A., Anesio, A., Deming, J., Mikucki, J. A., and Rapp, J. Z. (2015). Microbial ecology of the cryosphere: sea ice and glacial habitats. Nat. Rev. Microbiol. 13, 677–690. doi:10.1038/nrmicro3522
Bridier, G., Olivier, F., Chauvaud, L., Sejr, M. K., and Grall, J. (2021). Food source diversity, trophic plasticity, and omnivory enhance the stability of a shallow benthic food web from a high-Arctic fjord exposed to freshwater inputs. Limnol. Ocean. 66, S259–S272. doi:10.1002/lno.11688
Bridier, G., Olivier, F., Grall, J., Chauvaud, L., Sejr, M. K., and Tremblay, R. (2023). Seasonal lipid dynamics of four Arctic bivalves: implications for their physiological capacities to cope with future changes in coastal ecosystems. Ecol. Evol. 13, e10691. doi:10.1002/ece3.10691
Brown, T. A., Galicia, M. P., Thiemann, G. W., Belt, S. T., Yurkowski, D. J., and Dyck, M. G. (2018). High contributions of sea ice derived carbon in polar bear (Ursus maritimus) tissue. PLOS ONE 13, e0191631. doi:10.1371/journal.pone.0191631
Burot, C., Amiraux, R., Bonin, P., Guasco, S., Babin, M., Joux, F., et al. (2021). Viability and stress state of bacteria associated with primary production or zooplankton-derived suspended particulate matter in summer along a transect in Baffin Bay (Arctic Ocean). Sci. Total Environ. 770, 145252. doi:10.1016/j.scitotenv.2021.145252
Campbell, K., Lange, B., Landy, J. C., Katlein, C., Nicolaus, M., Anhaus, P., et al. (2022b). Net heterotrophy in High Arctic first-year and multi-year spring sea ice. Elem. Sci. Anth. 10, 00040. doi:10.1525/elementa.2021.00040
Campbell, K., Matero, I., Bellas, C., Turpin-Jelfs, T., Anhaus, P., Graeve, M., et al. (2022a). Monitoring a changing Arctic: recent advancements in the study of sea ice microbial communities. Ambio 51, 318–332. doi:10.1007/s13280-021-01658-z
Carlyle, C. G., Roth, J. D., Yurkowski, D. J., Kohlbach, D., Young, B. G., Brown, T. A., et al. (2022). Spatial variation in carbon source use and trophic position of ringed seals across a latitudinal gradient of sea ice. Ecol. Indic. 145, 109746. doi:10.1016/j.ecolind.2022.109746
Cautain, I. J., Last, K. S., McKee, D., Bluhm, B. A., Renaud, P. E., Ziegler, A. F., et al. (2022). Uptake of sympagic organic carbon by the Barents Sea benthos linked to sea ice seasonality. Front. Mar. Sci. 9. doi:10.3389/fmars.2022.1009303
Dalman, L. A., Else, B. G., Barber, D., Carmack, E., Williams, W. J., Campbell, K., et al. (2019). Enhanced bottom-ice algal biomass across a tidal strait in the Kitikmeot Sea of the Canadian Arctic. Elem. Sci. Anth. 7, 22. doi:10.1525/elementa.361
Dezutter, T., Lalande, C., Darnis, G., and Fortier, L. (2021). Seasonal and interannual variability of the Queen Maud Gulf ecosystem derived from sediment trap measurements. Limnol. Oceanogr. 66, S411–S426. doi:10.1002/lno.11628
Dezutter, T., Lalande, C., Dufresne, C., Darnis, G., and Fortier, L. (2019). Mismatch between microalgae and herbivorous copepods due to the record sea ice minimum extent of 2012 and the late sea ice break-up of 2013 in the Beaufort Sea. Prog. Ocean. 173, 66–77. doi:10.1016/j.pocean.2019.02.008
Duarte, P., Assmy, P., Campbell, K., and Sundfjord, A. (2022). The importance of turbulent ocean–sea ice nutrient exchanges for simulation of ice algal biomass and production with CICE6. 1 and Icepack 1.2. Geosci. Model Dev. 15, 841–857. doi:10.5194/gmd-15-841-2022
Dybwad, C., Lalande, C., Bodur, Y. V., Henley, S. F., Cottier, F., Ershova, E., et al. (2022). The influence of sea ice cover and Atlantic water advection on annual particle export north of Svalbard. J. Geophys. Res. 127, e2022JC018897. doi:10.1029/2022JC018897
Ehrlich, J., Bluhm, B., Peeken, I., Massicotte, P., Schaafsma, F. L., Castellani, G., et al. (2021). Sea-ice associated carbon flux in Arctic spring. Elem. Sci. Anth. 9, 00169. doi:10.1525/elementa.2020.00169
Ehrlich, J., Schaafsma, F. L., Bluhm, B. A., Peeken, I., Castellani, G., Brandt, A., et al. (2020). Sympagic fauna in and under Arctic pack ice in the annual sea-ice system of the new Arctic. Front. Mar. Sci. 7, 452. doi:10.3389/fmars.2020.00452
Ershova, E. A., Kosobokova, K. N., Banas, N. S., Ellingsen, I., Niehoff, B., Hildebrandt, N., et al. (2021). Sea ice decline drives biogeographical shifts of key Calanus species in the central Arctic Ocean. Glob. Change Biol. 27, 2128–2143. doi:10.1111/gcb.15562
Fadeev, E., Rogge, A., Ramondenc, S., Nöthig, E.-M., Wekerle, C., Bienhold, C., et al. (2021). Sea ice presence is linked to higher carbon export and vertical microbial connectivity in the Eurasian Arctic Ocean. Commun. Biol. 4, 1255. doi:10.1038/s42003-021-02776-w
Fernández-Méndez, M., Olsen, L. M., Kauko, H. M., Meyer, A., Rösel, A., Merkouriadi, I., et al. (2018). Algal hot spots in a changing Arctic Ocean: sea-ice ridges and the snow-ice interface. Front. Mar. Sci. 5, 75. doi:10.3389/fmars.2018.00075
Fisher, K., and Stewart, R. (1997). Summer foods of atlantic walrus, Odobenus rosmarus rosmarus, in northern foxe basin, northwest territories. Can. J. Zool. 75, 1166–1175. doi:10.1139/z97-139
Gaillard, B., Meziane, T., Tremblay, R., Archambault, P., Blicher, M. E., Chauvaud, L., et al. (2017). Food resources of the bivalve Astarte elliptica in a sub-Arctic fjord: a multi-biomarker approach. Mar. Ecol. Prog. Ser. 567, 139–156. doi:10.3354/meps12036
Giraldo, C., Stasko, A., Walkusz, W., Majewski, A., Rosenberg, B., Power, M., et al. (2018). Feeding of Greenland halibut (Reinhardtius hippoglossoides) in the Canadian Beaufort Sea. J. Mar. Syst. 183, 32–41. doi:10.1016/j.jmarsys.2018.03.009
Golikov, A. V., Stauffer, J. B., Schindler, S. V., Taylor, J., Boehringer, L., Purser, A., et al. (2023). Miles down for lunch: deep-sea in situ observations of Arctic finned octopods Cirroteuthis muelleri suggest pelagic–benthic feeding migration. Proc. R. Soc. B 290, 20230640. doi:10.1098/rspb.2023.0640
Gradinger, R. (2009). Sea-ice algae: major contributors to primary production and algal biomass in the Chukchi and Beaufort Seas during May/June 2002. Deep Sea Res. 56, 1201–1212. doi:10.1016/j.dsr2.2008.10.016
Gradinger, R., and Bluhm, B. (2010). Timing of ice algal grazing by the Arctic nearshore benthic amphipod Onisimus litoralis. Arctic. 63, 355–358. Available at: www.jstor.org/stable/20799602
Gradinger, R., and Bluhm, B. A. (2020). First of an Arctic sea ice meiofauna food web analysis based on abundance, biomass and stable isotope ratios. Mar. Ecol. Prog. Ser. 634, 29–43. doi:10.3354/meps13170
Granskog, M. A., Assmy, P., and Koç, N. (2020). “Emerging traits of sea ice in the atlantic sector of the arctic,” in Emerging traits of sea ice in the atlantic sector of the arctic in climate Change and the white world, 3–10. Cham: Springer International Publishing, 3–10. doi:10.1007/978-3-030-21679-5_1
Hancke, K., Lund-Hansen, L. C., Lamare, M. L., Højlund Pedersen, S., King, M. D., Andersen, P., et al. (2018). Extreme low light requirement for algae growth underneath sea ice: a case study from Station Nord, NE Greenland. J. Geophys. Res. 123, 985–1000. doi:10.1002/2017JC013263
Harris, C. M., McTigue, N. D., McClelland, J. W., and Dunton, K. H. (2018). Do high Arctic coastal food webs rely on a terrestrial carbon subsidy? Food Webs 15, e00081. doi:10.1016/j.fooweb.2018.e00081
Harwood, J. L. (2019). Algae: critical sources of very long-chain polyunsaturated fatty acids. Biomolecules 9, 708. doi:10.3390/biom9110708
Hegseth, E. N., and von Quillfeldt, C. (2022). The sub-ice algal communities of the Barents Sea pack ice: temporal and spatial distribution of biomass and species. J. Mar. Sci. Eng. 10, 164. doi:10.3390/jmse10020164
Hill, V., Light, B., Steele, M., and Sybrandy, A. L. (2022). Contrasting sea-ice algae blooms in a changing arctic documented by autonomous drifting buoys. J. Geophys. Res. 127. doi:10.1029/2021JC017848
Iken, K., Bluhm, B., and Dunton, K. (2010). Benthic food-web structure under differing water mass properties in the southern Chukchi Sea. Deep Sea Res. Part II Top. Stud. Oceanogr. 57, 71–85. doi:10.1016/j.dsr2.2009.08.007
Juul-Pedersen, T., Michel, C., Gosselin, M., and Seuthe, L. (2008). Seasonal changes in the sinking export of particulate material, under first-year sea ice on the Mackenzie Shelf (western Canadian Arctic). Mar. Ecol. Prog. Ser. 353, 13–25. doi:10.3354/meps07165
Kauko, H. M., Pavlov, A. K., Johnsen, G., Granskog, M. A., Peeken, I., and Assmy, P. (2019). Photoacclimation state of an Arctic underice phytoplankton bloom. J. Geophys. Res. 124, 1750–1762. doi:10.1029/2018JC014777
Kauko, H. M., Taskjelle, T., Assmy, P., Pavlov, A. K., Mundy, C., Duarte, P., et al. (2017). Windows in Arctic sea ice: light transmission and ice algae in a refrozen lead. J. Geophys. Res. Biogeo. 122, 1486–1505. doi:10.1002/2016JG003626
Koch, C. W., Brown, T. A., Amiraux, R., Ruiz-Gonzalez, C., MacCorquodale, M., Yunda, G., et al. (2023). Year-round utilization of sea ice-associated carbon in Arctic ecosystems. Nat. Commun. 14, 1964. doi:10.1038/s41467-023-37612-8
Koch, C. W., Cooper, L. W., Grebmeier, J. M., Frey, K., and Brown, T. A. (2020b). Ice algae resource utilization by benthic macro-and megafaunal communities on the Pacific Arctic shelf determined through lipid biomarker analysis. Mar. Ecol. Prog. Ser. 651, 23–43. doi:10.3354/meps13476
Koch, C. W., Cooper, L. W., Lalande, C., Brown, T. A., Frey, K. E., and Grebmeier, J. M. (2020a). Seasonal and latitudinal variations in sea ice algae deposition in the Northern Bering and Chukchi Seas determined by algal biomarkers. PloS ONE 15, e0231178. doi:10.1371/journal.pone.0231178
Koch, C. W., Cooper, L. W., Woodland, R. J., Grebmeier, J. M., Frey, K. E., Stimmelmayr, R., et al. (2021). Female Pacific walruses (Odobenus rosmarus divergens) show greater partitioning of sea ice organic carbon than males: evidence from ice algae trophic markers. PLOS ONE 16, e0255686. doi:10.1371/journal.pone.0255686
Kohlbach, D., Duerksen, S. W., Lange, B. A., Charette, J., Reppchen, A., Tremblay, P., et al. (2020). Fatty acids and stable isotope signatures of first-year and multiyear sea ice in the Canadian High Arctic. Elem. Sci. Anth. 8, 054. doi:10.1525/elementa.2020.054
Kohlbach, D., Ferguson, S. H., Brown, T. A., and Michel, C. (2019). Landfast sea ice-benthic coupling during spring and potential impacts of system changes on food web dynamics in Eclipse Sound, Canadian Arctic. Mar. Ecol. Prog. Ser. 627, 33–48. doi:10.3354/meps13071
Kohlbach, D., Graeve, M., A. Lange, B., David, C., Peeken, I., and Flores, H. (2016). The importance of ice algae-produced carbon in the central Arctic Ocean ecosystem: food web relationships revealed by lipid and stable isotope analyses. Limnol. Oceanogr. 61, 2027–2044. doi:10.1002/lno.10351
Kohlbach, D., Hop, H., Wold, A., Schmidt, K., Smik, L., Belt, S. T., et al. (2021). Multiple trophic markers trace dietary carbon sources in Barents Sea zooplankton during late summer. Front. Mar. Sci. 7, 610248. doi:10.3389/fmars.2020.610248
Kohlbach, D., Smik, L., Belt, S. T., Hop, H., Wold, A., Graeve, M., et al. (2022). A multi-trophic marker approach reveals high feeding plasticity in Barents Sea under-ice fauna. Prog. Ocean. 208, 102895. doi:10.1016/j.pocean.2022.102895
Krause-Jensen, D., Archambault, P., Assis, J., Bartsch, I., Bischof, K., Filbee-Dexter, K., et al. (2020). Imprint of climate change on pan-Arctic marine vegetation. Front. Mar. Sci. 7. doi:10.3389/fmars.2020.617324
Krembs, C., Eicken, H., Junge, K., and Deming, J. W. (2002). High concentrations of exopolymeric substances in Arctic winter sea ice: implications for the polar ocean carbon cycle and cryoprotection of diatoms. Deep Sea Res. 49, 2163–2181. doi:10.1016/S0967-0637(02)00122-X
Kunisch, E., Graeve, M., Gradinger, R., Haug, T., Kovacs, K. M., Lydersen, C., et al. (2021). Ice-algal carbon supports harp and ringed seal diets in the European Arctic: evidence from fatty acid and stable isotope markers. Mar. Ecol. Prog. Ser. 675, 181–197. doi:10.3354/meps13834
Kunisch, E. H., Graeve, M., Gradinger, R., Flores, H., Varpe, Ø., and Bluhm, B. A. (2023). What we do in the dark: prevalence of omnivorous feeding activity in Arctic zooplankton during polar night. Limnol. Ocean. 68, 1835–1851. doi:10.1002/lno.12389
Lacoste, É., Piot, A., Archambault, P., McKindsey, C. W., and Nozais, C. (2018). Bioturbation activity of three macrofaunal species and the presence of meiofauna affect the abundance and composition of benthic bacterial communities. Mar. Environ. Res. 136, 62–70. doi:10.1016/j.marenvres.2018.02.024
Lalande, C., Grebmeier, J. M., Hopcroft, R. R., and Danielson, S. L. (2020). Annual cycle of export fluxes of biogenic matter near Hanna Shoal in the northeast Chukchi Sea. Deep Sea Res. Part II Top. Stud. Oceanogr. 177, 104730. doi:10.1016/j.dsr2.2020.104730
Lalande, C., Grebmeier, J. M., McDonnell, A. M. P., Hopcroft, R. R., O’Daly, S., and Danielson, S. L. (2021). Impact of a warm anomaly in the Pacific Arctic region derived from time-series export fluxes. PLoS ONE 16, e0255837. doi:10.1371/journal.pone.0255837
Lalande, C., Nöthig, E.-M., Somavilla, R., Bauerfeind, E., Shevchenko, V., and Okolodkov, Y. (2014). Variability in under-ice export fluxes of biogenic matter in the Arctic Ocean. Glob. Biogeochem. Cy. 28, 571–583. doi:10.1002/2013GB004735
Landry, J. J., Fisk, A. T., Yurkowski, D. J., Hussey, N. E., Dick, T., Crawford, R. E., et al. (2018). Feeding ecology of a common benthic fish, shorthorn sculpin (Myoxocephalus scorpius) in the high arctic. Polar Biol. 41, 2091–2102. doi:10.1007/s00300-018-2348-8
Lange, B. A., Flores, H., Michel, C., Beckers, J. F., Bublitz, A., Casey, J. A., et al. (2017). Pan-Arctic sea ice-algal chl a biomass and suitable habitat are largely underestimated for multiyear ice. Glob. Change Biol. 23, 4581–4597. doi:10.1111/gcb.13742
Lange, B. A., Haas, C., Charette, J., Katlein, C., Campbell, K., Duerksen, S., et al. (2019). Contrasting ice algae and snow-dependent irradiance relationships between first-year and multiyear sea ice. Geophys. Res. Lett. 46, 10834–10843. doi:10.1029/2019GL082873
Lange, B. A., Michel, C., Beckers, J. F., Casey, J. A., Flores, H., Hatam, I., et al. (2015). Comparing springtime ice-algal chlorophyll a and physical properties of multi-year and first-year sea ice from the Lincoln Sea. PloS ONE 10, e0122418. doi:10.1371/journal.pone.0122418
Lannuzel, D., Tedesco, L., van Leeuwe, M., Campbell, K., Flores, H., Delille, B., et al. (2020). The future of Arctic sea-ice biogeochemistry and ice-associated ecosystems. Nat. Clim. Change 10, 983–992. doi:10.1038/s41558-020-00940-4
Legendre, L., Ackley, S. F., Dieckmann, G. S., Gullicksen, B., Horner, R., Hoshiai, T., et al. (1992). Ecology of sea ice biota: Part 2. Global significance. Polar Biol. 12, 429–444. doi:10.1007/BF00243114
Lalande, C., Nöthig, E. M., and Fortier, L. (2019). Algal export in the arctic ocean in times of global warming. Geophy. Res. Lett. 46, 5959–5967. doi:10.1029/2019GL083167
Leu, E., Mundy, C. J., Assmy, P., Campbell, K., Gabrielsen, T. M., Gosselin, M., et al. (2015). Arctic spring awakening – steering principles behind the phenology of vernal ice algal blooms. Prog. Ocean. 139, 151–170. doi:10.1016/j.pocean.2015.07.012
Leu, E., Søreide, J. E., Hessen, D. O., Falk-Petersen, S., and Berge, J. (2011). Consequences of changing sea-ice cover for primary and secondary producers in the European Arctic shelf seas: timing, quantity, and quality. Prog. Ocean. 90, 18–32. doi:10.1016/j.pocean.2011.02.004
Lim, S. M., Payne, C. M., van Dijken, G. L., and Arrigo, K. R. (2022). Increases in Arctic sea ice algal habitat, 1985–2018. Elem. Sci. Anthr. 10, 00008. doi:10.1525/elementa.2022.00008
Mann, R., Powell, E. N., and Munroe, D. M. (2020). The case of the ‘missing’ Arctic bivalves and the walrus: the biggest [overlooked] clam fishery on the planet. J. Shellfish Res. 39, 501–509. doi:10.2983/035.039.0301
Marquardt, M., Goraguer, L., Assmy, P., Bluhm, B. A., Aaboe, S., Down, E., et al. (2023). Seasonal dynamics of sea-ice protist and meiofauna in the northwestern Barents Sea. Prog. Oceanogr. 218, 103128. doi:10.1016/j.pocean.2023.103128
McConnell, B., Gradinger, R., Iken, K., and Bluhm, B. A. (2012). Growth rates of arctic juvenile Scolelepis squamata (Polychaeta: spionidae) isolated from Chukchi Sea fast ice. Polar Biol. 35, 1487–1494. doi:10.1007/s00300-012-1187-2
McMahon, K. W., Ambrose Jr, W. G., Johnson, B. J., Yi Sun, M., Lopez, G. R., Clough, L. M., et al. (2006). Benthic community response to ice algae and phytoplankton in Ny Ålesund, Svalbard. Mar. Ecol. Prog. Ser. 310, 1–14. doi:10.3354/meps310001
Moore, S. E., and Stabeno, P. J. (2015). Synthesis of arctic research (SOAR) in marine ecosystems of the pacific arctic. Prog. Ocean. 136, 1–11. doi:10.1016/j.pocean.2015.05.017
Mundy, C., and Meiners, K. M. (2021) “Ecology of arctic sea ice,” in Arctic ecology, 261–288. John Wiley and Sons Ltd, 261–288. doi:10.1002/9781118846582.ch10
Nadaï, G., Nöthig, E.-M., Fortier, L., and Lalande, C. (2021). Early snowmelt and sea ice breakup enhance algal export in the Beaufort Sea. Prog. Ocean. 190, 102479. doi:10.1016/j.pocean.2020.102479
North, C. A., Lovvorn, J. R., Kolts, J. M., Brooks, M. L., Cooper, L. W., and Grebmeier, J. M. (2014). Deposit-feeder diets in the Bering Sea: potential effects of climatic loss of sea ice-related microalgal blooms. Ecol. Appl. 24, 1525–1542. doi:10.1890/13-0486.1
Olsen, L. M., Laney, S. R., Duarte, P., Kauko, H. M., Fernández-Méndez, M., Mundy, C. J., et al. (2017). The seeding of ice algal blooms in Arctic pack ice: the multiyear ice seed repository hypothesis. J. Geophys. Res. Biogeo. 122, 1529–1548. doi:10.1002/2016JG003668
Onarheim, I. H., and Årthun, M. (2017). Toward an ice-free Barents Sea. Geophys. Res. Lett. 44, 8387–8395. doi:10.1002/2017GL074304
Oziel, L., Massicotte, P., Randelhoff, A., Ferland, J., Vladoiu, A., Lacour, L., et al. (2019). Environmental factors influencing the seasonal dynamics of spring algal blooms in and beneath sea ice in western Baffin Bay. Elem. Sci. Anthr. 7, 34. doi:10.1525/elementa.372
Payne, C., Bianucci, L., Van Dijken, G., and Arrigo, K. (2021). Changes in under-ice primary production in the Chukchi Sea from 1988 to 2018. J. Geophys. Res. 126, e2021JC017483. doi:10.1029/2021JC017483
Pitusi, V., Gradinger, R. R., and Søreide, J. (2023). Temporal and spatial variability of sympagic metazoans in a high-Arctic fjord, Svalbard. Front. Mar. Sci. 10. doi:10.3389/fmars.2023.1201359
Rantanen, M., Karpechko, A. Y., Lipponen, A., Nordling, K., Hyvärinen, O., Ruosteenoja, K., et al. (2022). The Arctic has warmed nearly four times faster than the globe since 1979. Commun. Earth Environ. 3, 168. doi:10.1038/s43247-022-00498-3
Ray, G. C., McCormick-Ray, J., Berg, P., and Epstein, H. E. (2006). Pacific walrus: benthic bioturbator of beringia. J. Exp. Mar. Bio. Ecol. 330, 403–419. doi:10.1016/j.jembe.2005.12.043
Renaud, P. E., Daase, M., Banas, N. S., Gabrielsen, T. M., Søreide, J. E., Varpe, Ø., et al. (2018). Pelagic food-webs in a changing Arctic: a trait-based perspective suggests a mode of resilience. ICES J. Mar. Sci. 75, 1871–1881. doi:10.1093/icesjms/fsy063
Renaud, P. E., Riedel, A., Michel, C., Morata, N., Gosselin, M., Juul-Pedersen, T., et al. (2007). Seasonal variation in benthic community oxygen demand: a response to an ice algal bloom in the Beaufort Sea, Canadian Arctic? J. Mar. Syst. 67, 1–12. doi:10.1016/j.jmarsys.2006.07.006
Riedel, A., Michel, C., and Gosselin, M. (2006). Seasonal study of sea-ice exopolymeric substances on the Mackenzie shelf: implications for transport of sea-ice bacteria and algae. Aquat. Microb. Ecol. 45, 195–206. doi:10.3354/ame045195
Rontani, J.-F., Lalande, C., Vilgrain, L., Vaultier, F., and Amiraux, R. (2022). Control of the preservation of sympagic algal material in surficial sediments of central and eastern Baffin Bay by bactericidal hydroperoxides and free fatty acids. Mar. Chem. 247, 104177. doi:10.1016/j.marchem.2022.104177
Salter, I., Bauerfeind, E., Fahl, K., Iversen, M. H., Lalande, C., Ramondenc, S., et al. (2023). Interannual variability (2000–2013) of mesopelagic and bathypelagic particle fluxes in relation to variable sea ice cover in the eastern Fram Strait. Front. Earth Sci. 11, 1210213. doi:10.3389/feart.2023.1210213
Selz, V., Saenz, B. T., van Dijken, G. L., and Arrigo, K. R. (2018). Drivers of ice algal bloom variability between 1980 and 2015 in the Chukchi Sea. J. Geophys. Res. 123, 7037–7052. doi:10.1029/2018JC014123
Søreide, J. E., Carroll, M. L., Hop, H., Ambrose, W. G., Hegseth, E. N., and Falk-Petersen, S. (2013). Sympagic-pelagic-benthic coupling in Arctic and Atlantic waters around Svalbard revealed by stable isotopic and fatty acid tracers. Mar. Biol. Res. 9, 831–850. doi:10.1080/17451000.2013.775457
Søreide, J. E., Leu, E. V. A., Berge, J., Graeve, M., and Falk-Petersen, S. (2010). Timing of blooms, algal food quality and Calanus glacialis reproduction and growth in a changing Arctic. Glob. Change Biol. 16, 3154–3163. doi:10.1111/j.1365-2486.2010.02175.x
Stasko, A. D., Bluhm, B. A., Michel, C., Archambault, P., Majewski, A., Reist, J. D., et al. (2018). Benthic-pelagic trophic coupling in an Arctic marine food web along vertical water mass and organic matter gradients. Mar. Ecol. Prog. Ser. 594, 1–19. doi:10.3354/meps12582
Stewart, J. D., Joyce, T. W., Durban, J. W., Calambokidis, J., Fauquier, D., Fearnbach, H., et al. (2023). Boom-bust cycles in gray whales associated with dynamic and changing Arctic conditions. Science 382, 207–211. doi:10.1126/science.adi1847
Stübner, E., Søreide, J., Reigstad, M., Marquardt, M., and Blachowiak-Samolyk, K. (2016). Year-round meroplankton dynamics in high-Arctic Svalbard. J. Plank. Res. 38, 522–536. doi:10.1093/plankt/fbv124
Tedesco, L., Vichi, M., and Scoccimarro, E. (2019). Sea-ice algal phenology in a warmer Arctic. Sci. Adv. 5, eaav4830. doi:10.1126/sciadv.aav4830
Tedesco, L., Vichi, M., and Thomas, D. N. (2012). Process studies on the ecological coupling between sea ice algae and phytoplankton. Ecol. Model. 226, 120–138. doi:10.1016/j.ecolmodel.2011.11.011
Wang, Q., Liu, Y., Lu, P., and Li, Z. (2023). The porosity effect on the mechanical properties of summer sea ice in the Arctic. Cryosphere Discuss, 1–21. doi:10.5194/tc-2023-31
Wang, Q., Lu, P., Leppäranta, M., Cheng, B., Zhang, G., and Li, Z. (2020). Physical properties of summer sea ice in the Pacific sector of the Arctic during 2008–2018. J. Geophys. Res. 125, e2020JC016371. doi:10.1029/2020JC016371
Wassmann, P., Carmack, E., Bluhm, B., Duarte, C. M., Berge, J., Brown, K., et al. (2020). Towards a unifying pan-arctic perspective: a conceptual modelling toolkit. Prog. Ocean. 189, 102455. doi:10.1016/j.pocean.2020.102455
Wassmann, P., Duarte, C. M., Agustí, S., and Sejr, M. K. (2011). Footprints of climate change in the Arctic marine ecosystem. Glob. Change Biol. 17, 1235–1249. doi:10.1111/j.1365-2486.2010.02311.x
Wei, C. L., Cusson, M., Archambault, P., Belley, R., Brown, T., Burd, B. J., et al. (2020). Seafloor biodiversity of Canada's three oceans: patterns, hotspots and potential drivers. Divers. Distrib. 26, 226–241. doi:10.1111/ddi.13013
Yunda-Guarin, G., Brown, T. A., Michel, L. N., Saint-Béat, B., Amiraux, R., Nozais, C., et al. (2020). Reliance of deep-sea benthic macrofauna on ice-derived organic matter highlighted by multiple trophic markers during spring in Baffin Bay, Canadian Arctic. Elem. Sci. Anthr. 8, 047. doi:10.1525/elementa.2020.047
Yunda-Guarin, G., Michel, L. N., Nozais, C., and Archambault, P. (2022). Interspecific differences in feeding selectivity shape isotopic niche structure of three ophiuroids in the Arctic Ocean. Ecol. Prog. Ser. 683, 81–95. doi:10.3354/meps13965
Yunda-Guarin, G., Michel, L. N., Roy, V., Friscourt, N., Gosselin, M., Nozais, C., et al. (2023). Trophic ecology of epibenthic communities exposed to different sea-ice concentrations across the Canadian Arctic Ocean. Prog. Ocean. 217, 103105. doi:10.1016/j.pocean.2023.103105
Yurkowski, D. J., Brown, T. A., Blanchfield, P. J., and Ferguson, S. H. (2020). Atlantic walrus signal latitudinal differences in the long-term decline of sea ice-derived carbon to benthic fauna in the Canadian Arctic. Proc. R. Soc. B 287, 20202126. doi:10.1098/rspb.2020.2126
Keywords: sea ice, ice algae, Arctic change, benthos, sympagic-pelagic-benthic coupling
Citation: Niemi A, Bluhm BA, Juul-Pedersen T, Kohlbach D, Reigstad M, Søgaard DH and Amiraux R (2024) Ice algae contributions to the benthos during a time of sea ice change: a review of supply, coupling, and fate. Front. Environ. Sci. 12:1432761. doi: 10.3389/fenvs.2024.1432761
Received: 14 May 2024; Accepted: 05 August 2024;
Published: 16 August 2024.
Edited by:
Per Fauchald, Norwegian Institute for Nature Research (NINA), NorwayReviewed by:
Chelsea Koch, American University, United StatesCopyright © 2024 Niemi, Bluhm, Juul-Pedersen, Kohlbach, Reigstad, Søgaard and Amiraux. This is an open-access article distributed under the terms of the Creative Commons Attribution License (CC BY). The use, distribution or reproduction in other forums is permitted, provided the original author(s) and the copyright owner(s) are credited and that the original publication in this journal is cited, in accordance with accepted academic practice. No use, distribution or reproduction is permitted which does not comply with these terms.
*Correspondence: Andrea Niemi, QW5kcmVhLk5pZW1pQERGTy1NUE8uZ2MuY2E=