- 1Department Earth and Environmental Sciences, KU Leuven, Leuven, Belgium
- 2KU Leuven Plant Institute, KU Leuven, Leuven, Belgium
- 3KU Leuven Urban Studies Institute, KU Leuven, Leuven, Belgium
- 4Public Governance Institute, KU Leuven, Leuven, Belgium
Research has shown that the collective network of domestic gardens could make a substantial contribution to climate change adaptation. One way to harness this contribution is by implementing Nature-based Solutions (NBS). However, due to the predominant focus on NBS applicable in large-scale and publicly available urban green areas, there is a lack of comprehensive research encompassing NBS specifically applicable to domestic gardens and their associated ecosystem services. Through a systematic review following the ROSES protocol, this paper provides an overview of the existing knowledge on small-scale NBS and climate resilient gardening practices, as well as, identifies research needs. This work contributes to the growing recognition of the spatial and ecological importance of domestic gardens for climate adaptation, and stresses the urgent need for more quantitative research on the range and effectiveness of ecosystem services provided by small-scale NBS. In this paper, we reflect upon the feasibility and practical implications of three specific NBS: the improvement of current lawn management practices, the reduction of sealed soil or pavement present, and the integration of trees in domestic gardens. We also acknowledge the potential of Citizen Science and governmental initiatives to engage citizens and improve the adoption of NBS in domestic gardens. Our work highlights the additional benefits and crucial role of urban planning and policy in integrating domestic gardens into broader climate adaptation strategies.
1 Introduction
Can domestic gardens play an important role in climate change adaptation? In the face of rapid urbanization and population growth, urban areas have been increasingly affected by heatwaves, air pollution, floodings and droughts, all of which will become more prevalent in the future due to climate change (Kabisch et al., 2017; Department of Economic and Social Affairs, 2019). Compared to traditional and technical measures, integrating Nature-based Solutions (NBS) into climate change adaptation policies is considered more effective and sustainable (Pauleit et al., 2017; Albert et al., 2019; Krauze and Wagner, 2019). NBS are defined by European Commission (2015) as actions which are “inspired by, supported by, or copied from nature”, and that are designed to address a range of environmental challenges in an efficient and adaptable manner, while at the same time providing economic, social, and environmental benefits (p. 5). The implementation of NBS in cities has been increasingly recognized for addressing climate-related challenges by providing diverse ecosystem services (Krauze and Wagner, 2019; Sušnik et al., 2022; McPhearson et al., 2023), including improving heat-regulation, advancing carbon sequestration, and reducing water scarcity, while also contributing to overall human wellbeing and biodiversity (Dewaelheyns, 2014; Beumer and Martens, 2016; Krols et al., 2022).
While much attention has been given to NBS applicable in large-scale and publicly available urban green areas, such as urban forests or sustainable urban drainage systems (Cameron et al., 2012; Haase et al., 2019), one vital area has remained hidden and largely unexplored—the private domain of domestic gardens. Turner (2005) describes gardens as “areas set aside for the cultivation and enjoyment of plant and other natural life”, emphasising how gardens combine cultivation (enhancing the natural environment or producing food) and enjoyment (recreational use), distinguishing them from purely agricultural or natural areas. Cameron (2012) further notes that domestic gardens are typically adjacent to residential buildings, situated within a local and individual household setting. Here, residents, whether owners or tenants, have the autonomy to manage their gardens personally or delegate this responsibility to professionals, underscoring the private nature of these spaces (Cameron et al., 2012).
Gardens are an integral part of the urban green and blue network and make up a significant portion of urban areas worldwide, ranging from 16% to 36% (Gaston et al., 2005; Mathieu et al., 2007; Loram et al., 2011; Cameron et al., 2012; Beumer and Martens, 2016; Yan et al., 2022). In Western Europe, this percentage is estimated to be even higher (Loram et al., 2011). Although specific numbers are limited, studies in the UK show that domestic gardens take up 35%–47% of the total green space in cities (Loram et al., 2007). Similarly, the extend of vegetated garden cover within the total urban area of Dunedin (New Zealand) was determined to be 36% (Mathieu et al., 2007). Research in Flanders (Belgium), the northern region of Belgium, estimated that domestic gardens cover more than one third of urban areas, and over 12% of the Flemish territory. This surpasses the present Flemish surface area of nature conservation (<5%) and forests (10%) (Strosse et al., 2020).
Despite their number and extent, domestic gardens have been largely missing in urban climate research, which may be due to their small scale, spatial fragmentation, and private character (Gaston et al., 2005; Mathieu et al., 2007; Dewaelheyns, 2014). Nevertheless, their collective network, the so-called ‘garden complex’, could make a substantial contribution to climate change adaption and the local quality of life by enhancing the various ecosystem services they provide (Dewaelheyns, 2014). Previous studies by Cameron et al. (2012), Langemeyer et al. (2019) and Krols et al. (2022) have explored the contribution of gardens to ecosystem services provisions, yet they primarily offer descriptive lists of ecosystem services without further refinement. Although these studies highlight that differences in both form and management of domestic gardens influence the ecosystem services benefits, limited research has been conducted specifically on the climatic impact of garden management (Cameron, 2023). Moreover, existing studies are limited to a particular scientific field, such as biodiversity, urban hydrology or soil research (Dewaelheyns et al., 2013; Tresch et al., 2019).
Hence, there is a need to update the understanding of the ecosystem services provided by gardens and to explore what gardens exactly contribute to climate change adaptation. One way to harness this contribution is by implementing NBS within the domestic garden context. The research questions are: (i) ‘Which NBS are applicable in domestic gardens?’ and (ii) ‘How and to what extent can these NBS provide climate change adaptation?’. Building on Turner (2005) and Cameron et al., 2012 definitions of domestic gardens, we further define NBS within this context as solutions that enhance the cultivation and enjoyment of natural life, tailored to the limited and local space of domestic gardens and easily accessible for voluntary adoption by residents. By performing a systematic review, we aim to consolidate existing knowledge on these small-scaled NBS applicable within domestic gardens and provide a well-documented inventory of their contributions to enhance the climate change adaptation potential of domestic gardens. This inventory could serve as a starting point for policymakers and planners looking to leverage that potential within their climate change adaptation strategies.
2 Systematic review
2.1 Koppen-Geiger climate zone ‘Cfb’
The review focusses specifically on the Western European region classified as Koppen-Geiger climate zone ‘Cfb’, including the UK, the Netherlands, Belgium, Luxembourg, France, Germany, and Switzerland (Peel et al., 2007). Urbanization in Western Europe has evolved organically over centuries, resulting in an intricate network of different land uses, ribbon development and urban sprawl, intertwining domestic gardens throughout the territory (Dewaelheyns et al., 2018; 2014; EEA, 2016; Vermeiren et al., 2022). This historical interconnectedness of domestic gardens with spatial development patterns specifically offers potential for local climate change adaptation (Dewaelheyns, 2014). Although the spatial context may differ in other regions, for example, the US (Akbari et al., 1997; Nassauer et al., 2014; 2009; Larson et al., 2022; 2009; Harris et al., 2012), analogies with urbanization patterns elsewhere makes Western Europe an interesting case. This includes considering the influence of policies promoting consumerism, the embrace of rural lifestyles, challenges from private housing development and governance ambiguities (den Heijer and Coppens, 2013).
The review aims to identify NBS that are particularly well-suited for addressing climate-related challenges and opportunities specific to the Western European ‘Cfb’ regions and their climatic patterns. These regions experience a maritime temperate climate with significant precipitation throughout the year and are characterized by mild summers due to cool ocean currents and winters that are usually cloudy and wet (Peel et al., 2007).
2.2 ESS garden model
To structure the systematic review, we developed ESS Garden model, built upon the ecosystem services (ESS) cascade model of CICES v5.1 (2017) (Figure 1) (Potschin et al., 2018). The CICES cascade model is a conceptual framework that was developed to explain how the ecosystem services paradigm can be used to understand relationships between people and nature (MEA, 2004; EEA, 2017). The model helps to explain benefits that ecosystem services provide for society, as well as how ecosystem services themselves are determined by natural structures and processes (Potschin and Haines-Young, 2011; Potschin and Haines-Young, 2016).
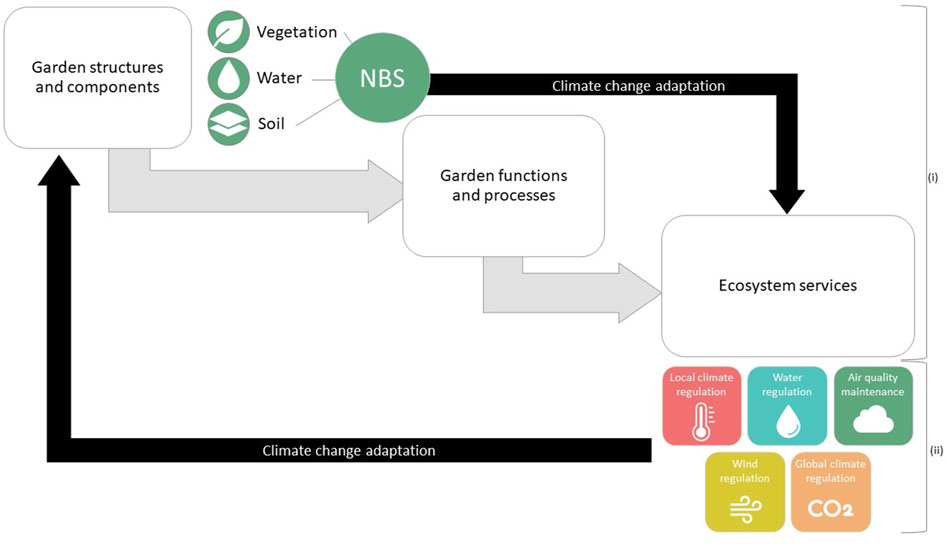
Figure 1. The ESS Garden Model based on (i) the CICES cascade model, and (ii) the MEA ecosystem services classification (MEA, 2004; EEA, 2017). Garden structures and components are divided over three garden covers where NBS can be implemented. In turn these NBS can provide relevant ecosystem services to address local urban environmental challenges related to climate change such as flooding, drought, and heat stress.
We restricted the biophysical structures and components to the garden context and focus solely on the environmental aspects of climate-related ecosystem services. We included three key garden covers to which NBS can be applied: vegetation, water, and soil. From the Millennium Ecosystem Assessment (MEA) classification (MEA, 2004), we selected relevant ecosystem services by prioritizing those that have a climatic impact or that can help alleviate local urban environmental problems, such as flooding, drought, and heat stress. These include local climate regulation, water regulation, air quality maintenance, and wind regulation. Recognizing the potential contribution of small-scale NBS in domestic gardens to increase carbon storage and other aspects of global climate regulation, we also included this aspect as part of the reviewed ecosystem services. This approach ensures a more comprehensive understanding of the overall impact of NBS across different scales.
The ESS Garden model simplifies the complex relationships between small-scale NBS and the ecosystem services they provide. Similar to the cascade model, it offers a vocabulary to better understand what ecosystem services can be provided by small-scale NBS that are useful for climate change adaptation (Potschin et al., 2018).
2.3 ROSES protocol
The ROSES review protocol is used to consolidate and document the existing knowledge on small-scale NBS suited for implementation within domestic gardens. This systematic review protocol builds upon the foundations of well-known reporting standards like PRISMA and is particularly tailored for conservation and environmental management research (Haddaway et al., 2018). It serves as a reporting and guidance tool designed to improve the efficiency and critical appraisal of the systematic review. It consists of an extensive checklist/report (Supplementary Appendix A) and flow diagram (Figure 2) designed to register all necessary information on how the systematic review was conducted to increase transparency and to ensure that the systematic review is of high standards (Haddaway et al., 2018; Drepper et al., 2021).
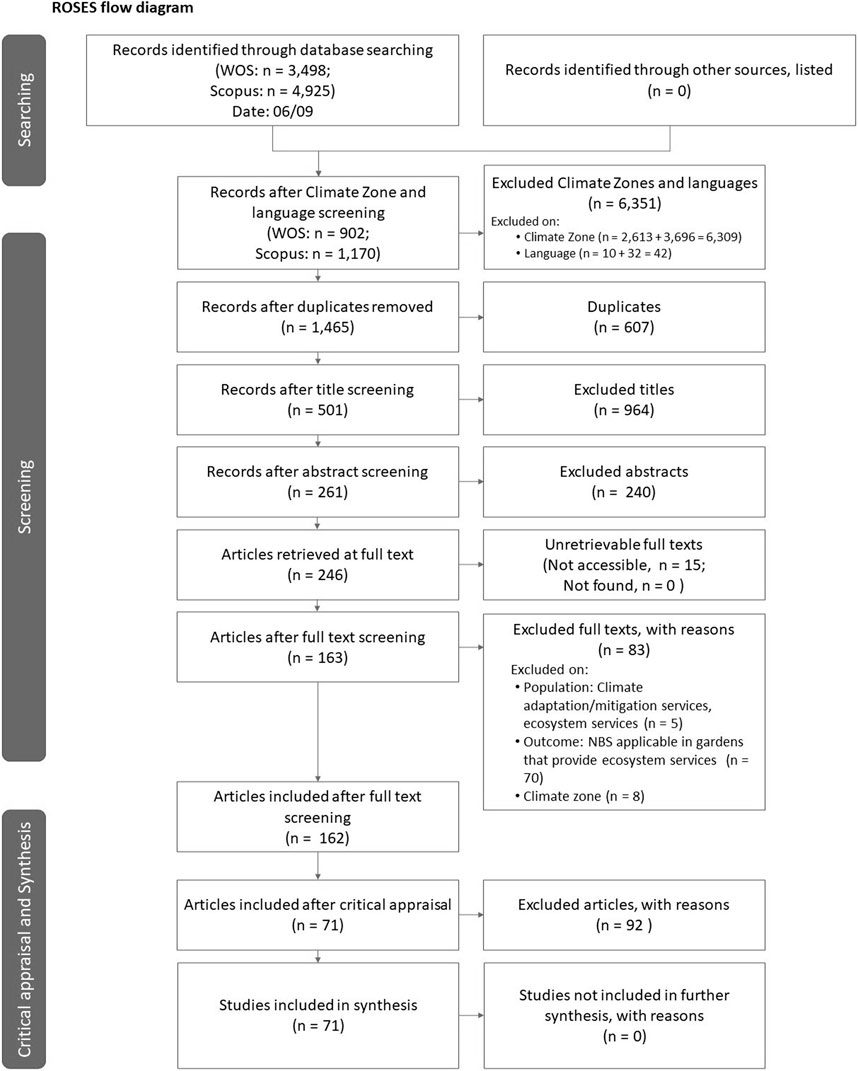
Figure 2. ROSES flow diagram of the systematic review. The flow diagram illustrates the screening and selection process of articles for the systematic review. Out of the 245 articles reviewed at full text level, 70 articles were ultimately included in the review.
Data was collected on the first of November 2023, from literature available on Web of Science Core Collection (WOS) and Scopus, using predetermined and tested search strings based on the ESS Garden model (Table 1). These search strings targeted literature exploring the interplay between NBS and gardens, and their roles in delivering ecosystem services. By including specific keywords such as “Nature-based Solutions”, “yard”, and “garden (management)” alongside with terms related to “ecosystem services” or environmental benefits, we aimed to target studies that focussed on their impact on key garden covers: vegetation, water, and soil. Since ‘ecosystem services’ or ‘Nature-based Solutions’ are both relatively recent scientific concepts, supplementary vocabulary related to urban green, nature, environment, and ecology was incorporated to reduce publication bias and cover reliability over time.
After extracting records from WOS and Scopus, the screening strategy focussed first on filtering literature on the relevant Koppen-Geiger climate zone ‘Cfb’, and literature written in English. Next, literature was screened at title-level, followed by the abstract and key-words. Based on predefined inclusion criteria (Table 2), studies covering both practical applications and theoretical explorations of the implementation of NBS within the domestic garden context were included. This approach aimed at offering a broad overview of the potential of NBS in domestic settings, with desired outcomes being improvements in ecosystem services or climate adaptation. If all the inclusion criteria were met (Table 2), literature was saved in the Mendeley Reference Manager software, and full-text screening was performed independently by three authors (Supplementary Appendix B). The ROSES flow chart in Figure 2 exposes the full body of literature.
To address potential publication bias, a comprehensive quality assessment was performed. This involved assigning a quality score to all included studies, categorizing them into low, medium, or high susceptibility to bias. Studies that are categorized as low susceptibility to bias, explicitly mention the link between NBS and the ESS they deliver, provide robust quantitative results, and detailed methodological approaches for transparency and replicability. Medium susceptibility studies similarly mention the link and provide quantitative results, however, these results are either suboptimal or derived from modelling, indicating a need for caution in interpretation. Lastly, high susceptibility studies only make reference to the link between specific NBS and ESS without providing supporting quantitative results (Supplementary Appendix B).
Data extracted from the final set of research papers is systematically organized using the ESS Garden model (Figure 1) resulting in an inventory of ecosystem services and NBS that provide them, with details covering NBS description, implementation, and management (Supplementary Appendix C). To ensure the reliability of the review, two independent authors tested the consistency of data extraction and validity assessment across a subset of six publications (8.6%), using the same indicators for inclusion or exclusion. This systematic approach aligns with the essential criteria for systematic reviews outlined by Bown and Sutton (2010).
3 Results
3.1 Global results of the systematic review
A total of 3,498 and 4,925 records were obtained from the Web of Science (WOS) and Scopus, respectively. From these initial records, 6,351 were excluded based on the language and geographic location requirements (Table 2) and 607 duplicates from both WOS and Scopus were removed. Subsequently, another 1,204 articles were excluded after title and abstract screening. Even though 246 articles were reviewed at full text level, only 71 remained for inclusion in the synthesis after screening and critical appraisal of the specific studies included in these articles (Figure 2). Most of the excluded articles focused on NBS that were specifically designed on a city-scale (e.g., urban forests, riparian buffers, large-scale bioswales) which did not align with the objectives of this research.
The distribution of articles for which the full text was screened, categorized by their year of publication, demonstrates a notable increase in literature corresponding with the introduction of the scientific term NBS (2015) (Figure 3). This expansion suggests a growing recognition and integration of the concept in research, leading to a substantial growth in the body of literature. Another noticeable trend is the increase of relevant publications that were ultimately included in the systematic review, particularly with the introduction of import garden research contributions, including (i) the first garden research in climate zone ‘Cfb’ (Gaston et al., 2005), and (ii) the first research exploring ecosystem services provided by gardens (Cameron et al., 2012; Cook et al., 2012) (Figure 3).
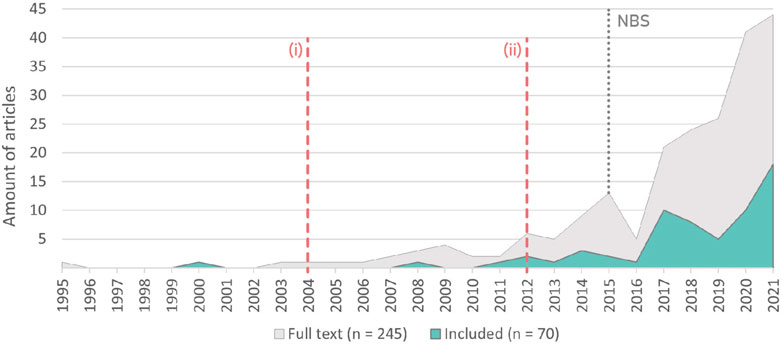
Figure 3. Illustration of the distribution of articles by publication year. The figure distinguishes the articles read at full-text level (shown in grey) and those included in the synthesis of the literature review (shown in blue). Additionally, the introduction of the term Nature-based Solutions (NBS) is highlighted, as well as, key research achievements including (i) the first garden research in climate zone ‘Cfb’, and (ii) the first research exploring ecosystem services provided by gardens.
Figure 4 illustrates the spatial representation of the research included in the systematic review. Studies concerning small-scale NBS within climate zone ‘Cfb’ have predominantly been conducted in the UK (24%) and France (13%). The remaining references do not target a specific geographical location and comprise of theoretical studies or other reviews which mainly focus on the city-scale and include only a limited number of NBS applicable to domestic gardens.
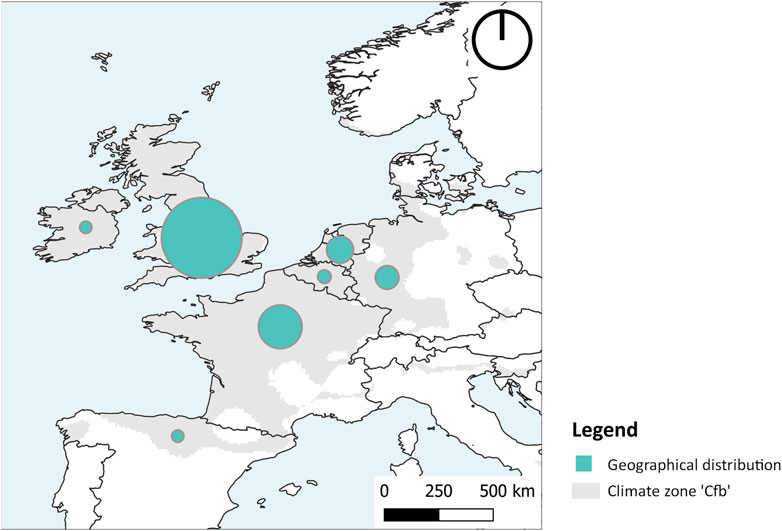
Figure 4. Illustration of the geographical distribution of articles included in the synthesis. The figure shows a spatial representation of the research included in the systematic review, with the size of each circle corresponding to the number of studies conducted in that specific area.
3.2 Nature-based Solutions applicable to domestic gardens
The systematic review resulted in a descriptive inventory of 10 small-scale NBS that support climate adaptation in domestic gardens by providing ecosystem services. These NBS are specifically suitable for implementation in domestic gardens given their spatial compatibility with domestic gardens of all sizes (Table 3) and are categorized by the garden cover they can be applied to (e.g., vegetation, water, or soil).
The primary focus of our review was to prioritize NBS supported by more robust and well-documented data. NBS focused on urban food production and soil contamination were excluded due to insufficient information regarding their specific ecosystem services or climate change adaptation potential. Similarly, NBS focused on highly specific applications like hardscaping, which involves integrating rocks, walls, and fences with plants for landscaping and energy savings (Sharath and Peter, 2019), were excluded due to a lack of supplementing information in general.
We maximized the inclusion of information from the systematic review by grouping certain NBS together when specific applications lacked sufficient data for meaningful comparisons or reliable conclusions. First, applications with similar characteristics, such as different types of water bodies, or comparable effects of practices like liming, fertilization, and pesticide use, were grouped together (e.g., ‘Installing a (natural) water body or rain garden’ and ‘Minimizing the application of liming, fertilizers, and pesticides’). Second, applications demonstrating coherence and aligning characteristics, such as ‘Greening of bare and sealed soil’ or ‘Creating a complex and diverse understory’ were grouped as they both contribute to the strengthening of a broader NBS.
Table 4 shows an overview of the links between NBS and their ecosystem services, highlighting research hot-spots as well as gaps. In the included NBS research, water regulation appears to be the primary focus, followed by local climate regulation. In contrast, papers focusing on wind regulation are rare. We found that the included literature extensively explores the contribution of the NBS ‘Increasing tree cover’ across all included ecosystem services. Concerning their climate change adaptation potential, the most studied NBS are ‘Increasing the tree cover’, ‘Installing a green roof’ and ‘Greening bare and sealed soil’. Despite being the least studied NBS, ‘Reducing lawn mowing’ was deemed important considering its potential impact, especially considering the prevalence of short-cut lawns in domestic gardens worldwide (Runfola et al., 2013; Ignatieva et al., 2020).
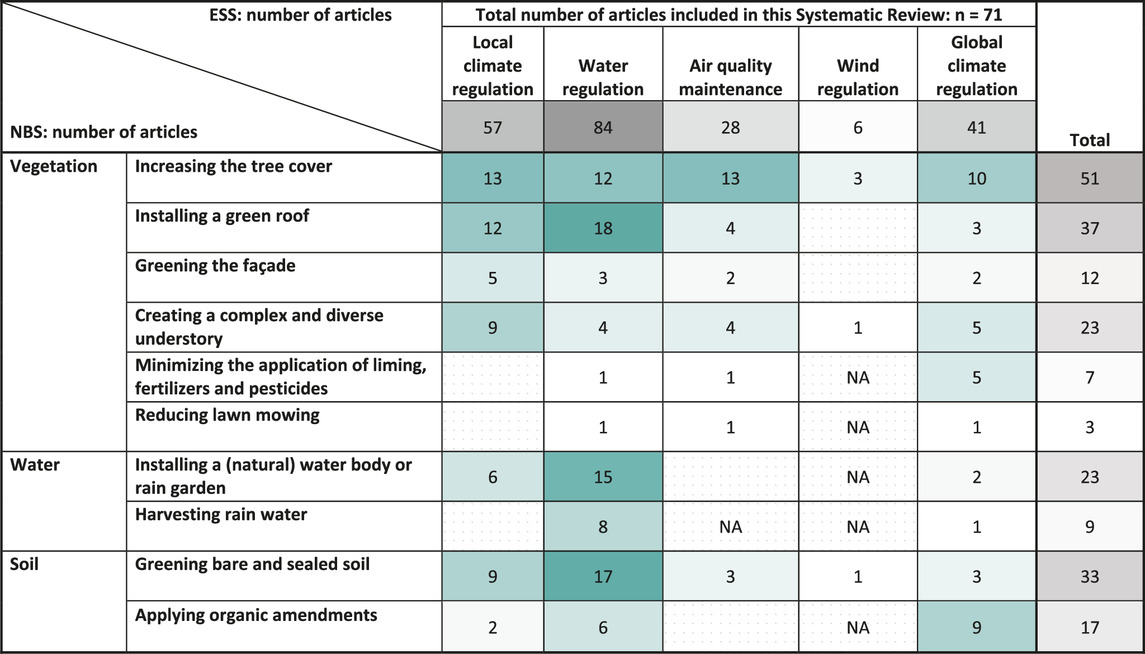
Table 4. Overview of the link between NBS and ESS mentioned in the reviewed research. The numbers in the table represent the count of articles included in the systematic review that establish a link between a specific NBS and an ESS. The total of articles mentioning a particular NBS are provided at the end of each row, while the total of articles mentioning the ESS are presented at the top of the columns. The shading intensity visually represents the proportional frequency of these associations.
3.3 Ecosystem services provided by NBS in the garden context
Our systematic review synthesizes the effectiveness and extent to which NBS applicable in domestic gardens can provide ecosystem services for climate change adaptation. With ‘extent’ we refer to the range of ecosystem services provided by the NBS, while ‘effectiveness’ refers to the degree to which these NBS successfully deliver those services, often supported by quantitative results. Therefore, we supplemented our overview of the research hotspots and gaps of the links between NBS and ecosystem services with the quantitative results from our systematic review (Table 5).
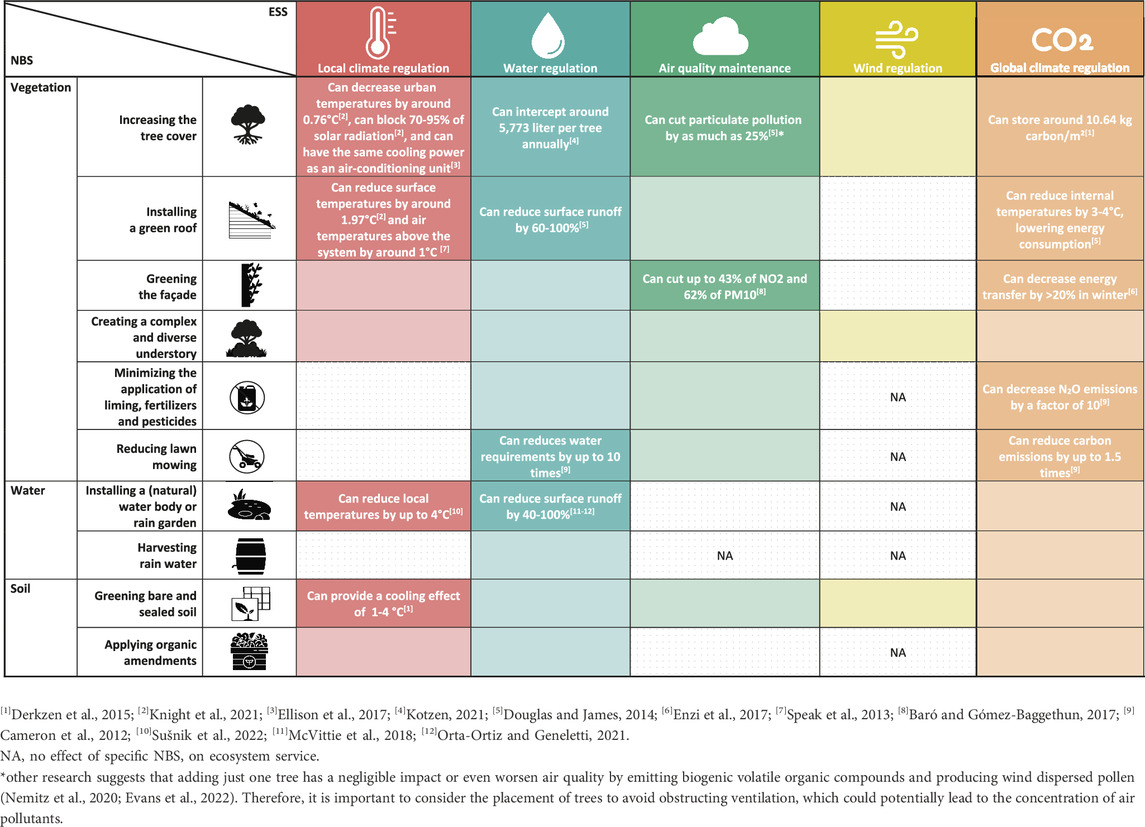
Table 5. Overview of the quantitative results derived from the systematic review. The table highlights quantitative results on the link between specific NBS and ESS, derived from the systematic review of 71 articles. Darker shading indicates quantitative results, while lighter shading indicates a mere mention of the link. Grey shading with diagonal hatching signifies that no links were found in the reviewed research, and “NA” indicates no observed effect of the NBS on the specific ESS.
When compared to Table 4, the systematic review included some explicit results, although some of them are rather general. Specifically, our review provided quantitative outcomes for only 30% of the specific connections between NBS and their roles in delivering specific ecosystem services. These outcomes were limited to seven out of the ten NBS studied. For about 44% of the researched combination of NBS and ecosystem services, general information was provided. Remarkably, our systematic review revealed that in 14% of the cases, a specific NBS was never reported in the included literature to provide the corresponding ecosystem services.
The majority of included studies are either theoretical or review studies (43%), modeling or simulation studies (26%), or experimental research (16%), while objective measurement studies are limited (14%). The remaining 3% consisted of qualitative research. Moreover, a range of different methodologies, units or metrics, and spatial ranges are used across the quantitative studies (Supplementary Appendix B).
Most of the quantitative data available concerned local climate regulation, while no quantitative data was available for wind regulation. The NBS ‘increasing the tree cover’ generated the most explicit results concerning its climate change adaptation potential across all ecosystem services. Although there is a vast amount of quantitative research conducted on trees, this systematic review identified only seven quantitative studies in Climate Zone ‘Cfb’ that were relevant to garden-scale applications. Even though garden management often involves making decisions regarding watering, soil improvement, and plant growth, no specific quantitative results were found for ‘harvesting rainwater’, ‘applying organic amendments’, or ‘greening bare and sealed soil’. Despite limited availability of literature concerning ‘reducing lawn mowing’, it yielded more quantitative results than the average NBS (Table 5).
4 Discussion
4.1 Relevance and feasibility of NBS in the garden context
The research included in the systematic review primarily focuses on NBS related to vegetation (59%). NBS concerning water (22%) and soil (19%) received less attention, indicating a need for additional efforts in these areas (Table 3). Despite this distribution, the systematic review does clearly highlight the climate change adaptation potential of NBS in domestic gardens. However, it also highlights a significant lack of quantitative data to support this claim. About 58% of the results from the systematic review provide either only general information or indicate a missing link between NBS and the ecosystem services, emphasizing this urgent need for more quantitative data on small-scale NBS.
Furthermore, even when quantitative results are available, a variety of methodologies and spatial ranges are utilized (Supplementary Appendix B), making comparisons between different NBS and the ecosystem services they provide impossible. This underscores the need for standardized approaches to enable comprehensive assessments in future research efforts.
Moreover, while spatial compatibility and the scale of NBS are crucial prerequisites for the successful implementation in domestic gardens, it is essential to consider various other factors. This includes evaluating the necessity and the feasibility of integrating specific NBS within the domestic garden context, key aspects that are not included in the reviewed articles. Surprisingly, none of the 71 included articles specifically address practical implications that significantly impact the effectiveness and long-term benefits of NBS implementation in domestic gardens. NBS are not one-size-fits-all solutions, and not every NBS is easily adopted by private gardeners, either due to technical constraints, financial limitations, or behavioral considerations. We reflect upon the necessity and the feasibility of shifting towards extensive lawn management, minimizing soil sealing, and expanding tree canopy cover in domestic gardens.
4.1.1 Shifting towards extensive lawn management
Worldwide, urban green areas are predominantly covered by lawns, covering around 50%–70% (Ignatieva and Hedblom, 2018). In the US, lawns cover over 163,000 km2 and are particularly prevalent within residential areas (Runfola et al., 2013; Lerman and Contosta, 2019). Within Sweden, their coverage is estimated to range between 23%–31% of the country, and half of its urban green areas (Hedblom et al., 2017). In Flanders (Belgium), 16% of the total surface area of gardens consists of intensively managed lawns (derived from Strosse et al., 2020). As their neat and functional appearance is perceived as a sign of wealth, the majority of lawns in domestic gardens are subjected to intensive management practices mainly including frequent mowing, fertilization, liming and irrigation. (Bormann et al., 1994; Cook et al., 2012; Lerman et al., 2018). As illustrated by our systematic review, this socially and culturally ingrained approach to lawn management can have a pronounced effect on the local environment and climate (Selhorst and Lal, 2013).
Our literature review revealed that petrol-powered lawn mowing specifically emits 1.5 times more carbon than the lawn itself is able to sequester (Cameron et al., 2012). This occurs not only due to emissions but also as a result of frequent mowing practices, often exceeding 20 times a year, which leads to reduced organic matter. Additionally, many gardeners dispose of their grass clippings, further depleting organic matter present. Furthermore, elevated pH levels resulting from excessive liming accelerate the breakdown of organic matter in lawns, hindering their ability to effectively sequester carbon in the soil. A striking 80% of soils beneath Flemish lawns have a carbon content lower than the target zone, which is determined by the optimal condition relative to the soil type and organic matter content (Dewaelheyns et al., 2013; Tits et al., 2021). Our systematic review also found that often frequently fertilized lawns emit up to 10 times more N2O compared to adjacent agricultural grasslands (Cameron et al., 2012).
In spite of that, when managed properly, grasslands have the potential to sequester a similar amount of carbon (2.8 Mg C ha-1 year-1) as forests (3.2 Mg C ha-1 year-1) (Selhorst and Lal, 2013). Turfgrass alone has demonstrated relatively rapid carbon sequestration potential, reaching 0.32–0.78 C ha-1 year-1 within the initial 4 years (Qian and Follett, 2002; Qian et al., 2010). Given the prevalence of lawns in urban areas, particularly in domestic gardens, their impact on carbon sequestration cannot be underestimated. For example, when all short-cut lawns in Flanders (Belgium), encompassing approximately 60,420 ha, are managed sustainably and even surpass the initial target zone, they have the potential to sequester an estimated extra 845,880 tons of carbon annually (derived from Tits et al., 2021; Dewaelheyns et al., 2013; Van Meerbeek et al., 2019). Next to carbon sequestration, our systematic review also indicated that opting for a less intensive lawn management reduces water requirements by up to 10 times compared to traditional lawns (Cameron et al., 2012). The NBS ‘reducing mowing frequency’ and ‘minimizing the use of lime, fertilizers, and pesticides’, are thus highly relevant as they can enhance climate adaptation in domestic gardens to impacts such as drought, heat stress, and flooding (Shwartz et al., 2013; Tresch et al., 2018). Nevertheless, our systematic review reveals that to date, no quantitative research has been done to assess the benefits resulting from shifting from an intensive to extensive lawn management in domestic gardens, specifically for local climate regulation.
The adoption of climate adaptive lawn management practices can lead to economic benefits at the individual level, such as reduced costs, time savings, and decreased environmental impact (Ignatieva and Hedblom, 2018). Citizens can reduce expenses on inputs like irrigation, pesticides, and fertilizers. Improved soil health from better management leads to reduced water usage, further lowering costs. Healthier lawns require less maintenance, saving time and energy (Selhorst and Lal, 2013). Less intensively managed lawns possess greater plant diversity, feature an abundance of flowers and attract a wider variety of fauna (Lerman et al., 2018).
Nevertheless, the successful implementation of these NBS relies entirely on adapting garden management practices and behaviors. While reducing lawn mowing frequency and minimizing the use of chemical inputs may require less frequent efforts and do not necessarily pose any economic or biophysical constraints, the need for behavioral change introduces a social constraint or hesitance. Adopting a more integrated approach to lawn management requires time and effort to study, seek advice, and explore alternative strategies. This social hesitance can arise from the additional steps and considerations involved in transitioning to new practices, which may require individuals to be receptive to change. Social hesitance can also arise from a social norm or a sense of social responsibility towards the neighborhood or community to keep lawns short and tidy (Robbins, 2008; Eisenhauer et al., 2016; Sisser et al., 2016).
The main obstacle to the effective implementation of these NBS in the garden context is the deeply ingrained preference for evergreen lawns and the societal constraints associated with this idealized image (Cook et al., 2012). Citizen science (CS) campaigns promoting climate adaptive lawn management, however, have already been successful in engaging individuals (Southon et al., 2017; Ignatieva and Hedblom, 2018; Lerman et al., 2018). Recent initiatives like ‘No Mow May’ have shown remarkable promise by encouraging reduced mowing during the month of May to support biodiversity (Plantlife, 2023). Originating in the UK, this initiative has gained widespread attention and global adoption including in Canada, Denmark, and the region of Flanders (Belgium). The Flemish version ‘MaaiMeiNiet’ has gained significant popularity over the past years, attracting more than 6,000 officially registered participants in 2021 and over 9,000 officially registered participants in 2022 (Knack, 2023). Although intensive lawn management is culturally embedded and socially ingrained, these CS campaigns do demonstrate public willingness to alter lawn management habits, highlighting the potential of these NBS in domestic gardens.
4.1.2 Minimizing soil sealing
Soil sealing is a significant issue in Europe, affecting 88,565 km2 of land, with each EU inhabitant being associated to around 200 m2 of sealed soil (Copernicus, 2018). This challenge is particularly evident in urban areas, where soil sealing occupies 20%–49% of the EU’s surface (Laćan et al., 2020). In the UK, 9,338 km2 is sealed, while Belgium and the Netherlands have approximately 8.2% of their total surface area affected. In Flanders (Belgium), 14% of the soil is sealed, which exceeds the European average by 7.2% (Departement Omgeving, 2018). This soil sealing is largely attributed to urban sprawl, the rapid urban expansion into smaller settlements connected by a dense road network (Antrop, 2004; Verbeeck et al., 2014; Vermeiren et al., 2022). Given that research has already shown elevated levels of sealed soil in front gardens, people may extent this habit of excessive paving within their domestic gardens for a number of reasons (Heikoop, 2022). Much like evergreen lawns, paved surfaces offer convenience as they require minimal maintenance and provide a uniform and tidy appearance contributing to a certain aesthetic appeal (Cook et al., 2012; Eisenhauer et al., 2016). Some people also choose to pave their gardens to create functional space for outdoor activities or parking (Perry and Nawaz, 2008; Cameron et al., 2012). Unfortunately, this trend worsens climate change impacts in urban areas, leading to increased heat stress and flooding (Kabisch et al., 2017). This is exemplified by the city of Leeds (UK) where a 13% increase in sealed soil over 30 years, primarily due to front garden paving, has been directly linked to more frequent and severe floodings in the region (Perry and Nawaz, 2008; Cameron et al., 2012). In Flanders (Belgium), almost 20% of domestic garden surfaces are paved, emphasizing the need to consider the environmental impact of excessive paving (derived from Strosse et al., 2020).
Sealed soil lacks the ability to sequester carbon (Cameron et al., 2012; Pereira et al., 2021). In addition, pavement contributes significantly to the Urban Heat Island effect leading to elevated local temperatures with increased soil sealing (Beumer and Martens, 2016; Ziter et al., 2019). The impermeable nature of pavement increases surface runoff as it prevents water from infiltrating into the ground, leading to reduced groundwater recharge, increased risks to flooding and alleviated pressure on urban drainage systems (Veerkamp et al., 2021).
Through methods like breaking out and removing pavement or utilizing alternatives like vegetated grid pavement, the NBS of ‘greening sealed soil’ offers a relatively straightforward solution to mitigate these negative consequences (Langergraber et al., 2020). Our review highlights that the cooling effect of vegetated patches, due to shading and evapotranspiration processes, can lead to temperature reduction from 1°C to 4°C depending on the spatial arrangement, the surface area and the vegetation type (Derkzen et al., 2015; Canet-Marti et al., 2021). To illustrate, grass-covered areas already show an average temperature reduction of 0.55°C compared to sealed surfaces (Knight et al., 2021). However, our systematic review also reveals a lack of quantitative results on the contribution of minimizing soil sealing to other ecosystem services.
Despite the potential benefits of ‘greening sealed soil’, implementing this NBS may face various constraints. Social constraints arise from limited awareness on the environmental impact of sealed surfaces, resistance to change, and personal preferences for paved surfaces. Furthermore, reluctancy to remove pavement can also be attributed to spatial constraints attributed to garden size or soil conditions. Additionally, greening sealed soil involves cost considerations associated with removing and processing the existing pavement or investing in alternatives like vegetated grid pavement. Moreover, it requires a substantial physical effort to carry out the necessary changes or might involve outsourcing the work to professionals in case of physical limitations.
While the process of greening sealed soil may require some social, economic, and physical effort, its benefits are substantial, offering a relatively straightforward solution. The CS campaigns ‘Nederlands Kampioenschap Tegelwippen’ (Dutch Championship Tile Flipping) in the Netherlands and ‘Vlaams Kampioenschap Tegelwippen’ (Flemish Championship Tile Flipping) in Flanders (Belgium) have already successfully promoted this NBS. Using a gamification format, these campaigns encourage citizens and municipalities to remove tiles from their outdoor spaces and replace them with green surfaces (Departement Omgeving and vzw, 2023; Frank Lee, 2023). Municipalities frequently support citizens by collecting the removed tiles and either recycling them into construction materials or ensuring their responsible disposal, highlighting the campaigns’ overall commitment to sustainability (Gemeente Waalwijk, 2023; Stad Leuven, 2023). The initiatives collect data on the number of tiles removed and the corresponding increase in green spaces, fostering community engagement and raising awareness about the importance of greening sealed soil. In the Netherlands the CS campaign has successfully resulted in the removal of an impressive 2.8 million pavement tiles in 2022 (Frank Lee, 2023), demonstrating public and governmental willingness to overcome the aforementioned social, economic and physical constraints.
4.1.3 Expanding tree canopy cover
In our increasingly urbanized world, the spatial importance of trees is evident for climate adaptation and improving the overall quality of life. The urgency and importance of trees within urban landscapes gained significance as early as 1994 by the research conducted by McPherson and colleagues. Their work revealed that an increased tree cover could reduce local air temperatures by 0.5°C and lower total heating and cooling energy use by 5%–10% annually (McPherson et al., 1994). Later studies by Akbari et al. (2001) supported these findings, indicating that urban trees have the capacity to reduce national energy use for air conditioning in the US by up to 20% (Akbari et al., 2001). However, recent studies show that global urban tree cover is declining by 40,000 ha/year, while global impervious cover is increasing by 326,000 ha/year (Nowak and Greenfield, 2020). As urban areas continue to expand, the urgency to integrate trees in the urban fabric to create more climate resilient cities becomes more pressing.
Our systematic review stresses this urgency of integrating trees to enhance climate adaptation in domestic gardens. It highlights that trees are widely recognized for above ground carbon sequestration, storing an average of 10.64 kg/m2 (Derkzen et al., 2015), with mature and diverse trees demonstrating a greater capacity (Pereira et al., 2021). They contribute to mitigating the Urban Heat Island effect by regulating micro-climates and reducing greenhouse gas emissions related to building energy use through shading and evapotranspiration (Cameron et al., 2012; Baró and Gómez-Baggethun, 2017). Broadleaf trees block 70%–95% of solar radiation (Knight et al., 2021), and deciduous trees offer a seasonal heat-blocking effect (Mancebo, 2018). Evapotranspiration cools urban areas by approximately 0.76°C on average (Knight et al., 2021), with a single mature tree providing a cooling equivalent to operating a household air-conditioning unit for a day (Ellison et al., 2017). Trees also play a crucial role in sustainable urban water management by intercepting and temporarily holding water within their canopy with an average of 5,773 L per tree annually (Kotzen, 2021). They protect soil surfaces from rainfall impact, reduce peak flow and ease demand on urban drains (Davis and Naumann, 2017; Roeland et al., 2019; Oral et al., 2020). Trees are also effective windbreaks, protecting buildings from local turbulence and high wind speeds, with vegetation density and roughness enhancing their effectiveness (Nemitz et al., 2020). However, the systematic review revealed deviating results concerning the impact of trees on air quality, with some research stating that trees can cut particulate pollution by as much as 25% (Douglas and James, 2014) and other research suggests that adding just one tree has a negligible impact or even worsens air quality by emitting biogenic volatile organic compounds and producing wind-dispersed pollen (Nemitz et al., 2020; Evans et al., 2022).
As our systematic review illustrates, the NBS of ‘increasing the tree cover’ contributes to all included ecosystem services, indicating a significant potential for climate adaptation. However, it is essential to consider the local context, spatial limitations, urban planning regulations and the social controversies surrounding trees. Planting trees is not a straightforward solution and factors such as soil type, prevailing climatic conditions, strategic tree placement to avoid obstructing ventilation, and appropriate species selection are crucial considerations for successful implementation. This stresses the importance of selecting tree species based on scientific evidence and implementing thoughtful planting strategies (Blanuša and Hadley, 2019; Blanuša et al., 2020; Orta-Ortiz and Geneletti, 2021). Integrating trees into garden designs is also not always feasible, particularly in compact spaces or due to restrictive urban planning regulations (Sousa-Silva et al., 2021). The many diverse social controversies surrounding trees—ranging from concerns related to obstructing views, leaf litter and associated insect nuisances to potential neighborhood conflicts and the need for effective tree management—should also be acknowledged as they often lead to tree removal or reluctancy to integrate trees. Even though, effective tree management can indeed involve tasks requiring time and physical effort such as pruning, thoughtful and strategic planning can significantly reduce the necessary efforts.
While the integration of trees requires thoughtful planting strategies and faces various social controversies, the systematic review highlights their significant potential for climate adaptation. Cities worldwide are actively pursuing tree planting campaigns and programs for their environmental and health benefits (Akbari et al., 2001; Lerman et al., 2018; Sousa-Silva et al., 2021). For instance, the ‘MillionTreesNYC’ campaign has successfully added one million trees, with 70% planted in public green spaces by the city and 30% by private organizations, homeowners, and community organizations. The campaign engaged citizens in planting and maintaining urban trees, fostering a sense of ownership and mitigating social controversies (MillionTreesNYC, 2015). Similarly, programs such as ‘Trees for Streets’ in London engaged citizens to sponsor local councils to facilitate increased tree planting (Trees for Cities, 2023). These abovementioned initiatives reflect a growing recognition that the environmental and health benefits of urban trees can outweigh perceived inconveniences, underscoring the potential of this NBS to transform urban landscapes for the better.
4.2 Ecosystem disservices in the garden context
While domestic gardens clearly contribute to climate change adaptation through the provision of ecosystem services, they can also be associated with ecosystem disservices such as habitat fragmentation, chemical pollution, water run-off, the introduction of invasive plant species and increased allergic reactions to pollen because of higher concentrations of flowering plants (Wang et al., 2015; Blanuša and Hadley, 2019; Cameron, 2023). Therefore, careful consideration of potential ecosystem disservices is essential, along with selecting appropriate NBS to mitigate these impacts and ensure their overall positive effect on the environment. This highlights the importance of taking a holistic and systemic approach in understanding the potential contribution of domestic gardens and implementing suitable NBS.
4.3 Planning and policy implications
Domestic gardens take up a significant share of urban environments worldwide and their design and management can contribute to climate change adaptation (Mathieu et al., 2007; Dewaelheyns, 2014; Baker and Smith, 2019). Citizen Science campaigns and governmental initiatives have already proven to be successful in engaging individuals and promoting environmentally conscious practices, contributing to the effective implementation of NBS in the domestic garden context. Such initiatives offer guidance, resources, or incentives to encourage the adoption of NBS in domestic gardens.
Urban planning and policy can significantly strengthen these benefits of engaging citizens and promoting environmentally conscious practices. By incorporating NBS for domestic gardens into urban planning frameworks and formulating supportive policies, they can further enhance the climate change adaptation potential of domestic gardens (Pauleit et al., 2017). However, empirical evidence is an indispensable basis for urban planning as well as governance guidelines and tools. The lack of quantitative data, standardized analysis approaches, and thorough insights into the decision-making patterns of gardeners hinder the development of effective urban planning and climate policies.
Furthermore, another essential urban planning and policy aspect involves exploring integrated approaches to overcome the fragmentation inherently related to domestic gardens. This entails recognizing gardens as essential elements of a larger green infrastructure network contributing to ecological, social, and climate-related benefits. This objective to go beyond the limitations of individual property lines, can be achieved by collective efforts involving various stakeholders, including individuals, local governments, experts, and organizations (Dewaelheyns et al., 2016).
In addition to the lack of empirical evidence and the need for integrated approaches, previous research has already identified a range of additional obstacles and challenges in the implementation of NBS, where urban planning and policy can play a key role. Examples of possible interventions are the development of design standards, addressing technological uncertainties, securing adequate funding, enhancing institutional capacities, and establishing robust legal frameworks to enforce NBS policies (den Heijer and Coppens, 2023; Dorst et al., 2019; Kabisch et al., 2017).
5 Conclusion
Domestic gardens can play an important role in climate change adaptation in Western Europe, given that evidence-based approaches are used–informed by quantitative results and standardized methods -, feasibility and practical implications are assessed, and ecosystem disservices are recognized. Rather than promoting to expand the spatial extent of domestic gardens, this paper emphasizes maximizing the efficiency and effectiveness of existing ones for climate change adaptation through the implementation of Nature-based Solutions (NBS).
Reviewing 71 articles, we identified ten NBS that effectively address climate-related challenges and opportunities, contributing to the growing recognition of the spatial and ecological importance of domestic gardens for climate change adaptation. However, a possible limitation of our research is that our systematic review might be influenced by publication bias. Nonetheless, our results stress both the urgent need for more quantitative research on the range and effectiveness of ecosystem services provided by small-scale NBS, and the necessity for standardized analysis approaches to enable comprehensive assessments and comparisons.
We highlight the necessity of integrating three specific NBS in the domestic garden context: the improvement of current lawn management practices, the reduction of sealed soil or pavement present and the integration of trees in domestic gardens. We reflect upon their feasibility and practical implications, including social constraints, financial requirements (implementation and management costs or material requirements), spatial limitations, labor involvement, time commitments, and necessary expertise. Even though substantial improvements can be made on these aspects, our research also confirms that NBS are no one-size-fit all solutions and require tailored approaches that are socially, ecologically and spatially explicit.
Our research acknowledges the potential of Citizen Science campaigns and governmental initiatives in addressing these challenges by engaging citizens, promoting climate adaptive practices, and improving the adoption of certain NBS in domestic gardens. Additionally, the role of urban planning and policy in integrating domestic gardens into broader climate adaptation strategies is crucial for enhancing the successful implementation of NBS in domestic gardens.
Future research should prioritize quantitative results concerning NBS in domestic gardens across various climate zones, ensuring a comprehensive understanding of NBS effectiveness in different environments. By developing practical strategies for implementing NBS through collaborations among scientists, citizens, and urban planners, the identified constraints can be addressed. Such comprehensive and holistic approach will contribute to the effective integration of NBS in broader urban planning strategies and policymaking, facilitating their widespread adoption and maximizing the climate change adaptation potential of domestic gardens.
Data availability statement
The original contributions presented in the study are included in the article/Supplementary Material, further inquiries can be directed to the corresponding author.
Author contributions
JT: Conceptualization, Data curation, Formal Analysis, Investigation, Methodology, Visualization, Writing–original draft, Writing–review and editing. KW: Formal Analysis, Investigation, Writing–review and editing. EB: Formal Analysis, Investigation, Writing–review and editing. VD: Conceptualization, Methodology, Supervision, Writing–review and editing. TS: Funding acquisition, Project administration, Supervision, Writing–review and editing. BS: Funding acquisition, Project administration, Supervision, Writing–review and editing.
Funding
The author(s) declare that financial support was received for the research, authorship, and/or publication of this article. This research has received funding from the LIFE “People-driven: Adapting Cities for Tomorrow” (PACT) project (grant number: LIFE20 CCA/BE/001710) and the KU Leuven C2 project GARLOCK (grant number: C24M/21/015).
Acknowledgments
During the preparation of this work the author(s) used ChatGPT in order to improve language and readability. After using this tool/service, the author(s) reviewed and edited the content as needed and take(s) full responsibility for the content of the publication.
Conflict of interest
The authors declare that the research was conducted in the absence of any commercial or financial relationships that could be construed as a potential conflict of interest.
Publisher’s note
All claims expressed in this article are solely those of the authors and do not necessarily represent those of their affiliated organizations, or those of the publisher, the editors and the reviewers. Any product that may be evaluated in this article, or claim that may be made by its manufacturer, is not guaranteed or endorsed by the publisher.
Supplementary material
The Supplementary Material for this article can be found online at: https://www.frontiersin.org/articles/10.3389/fenvs.2024.1430739/full#supplementary-material
SUPPLEMENTARY TABLE 1 | Appendix A: ROSES report.
SUPPLEMENTARY TABLE 2 | Appendix B: Reviewed articles.
SUPPLEMENTARY TABLE 3 | Appendix C: Full systematic review.
References
Akbari, H., Km-n, D. M., Bretz, S. E., and Hanford, J. W. (1997). Peak power and cooling energy savings of shade trees, 25, 139–148.
Akbari, H., Pomerantz, M., and Taha, H. (2001). Cool surfaces and shade trees to reduce energy use and improve air quality in urban areas. Sol. Energy 70, 295–310. doi:10.1016/S0038-092X(00)00089-X
Albert, C., Schröter, B., Haase, D., Brillinger, M., Henze, J., Herrmann, S., et al. (2019). Addressing societal challenges through nature-based solutions: how can landscape planning and governance research contribute? Landsc. Urban Plan. 182, 12–21. doi:10.1016/j.landurbplan.2018.10.003
Antrop, M. (2004). Landscape change and the urbanization process in Europe. Landsc. Urban Plan. 67, 9–26. doi:10.1016/S0169-2046(03)00026-4
Ascenso, A., Gama, C., Roebling, P., and Miranda, A. I. (2021). “How effective are nature-based solutions in different environments?,” in WITPress, CESAM, centre for environmental and marine studies. Editor J. C. L. J. L. J. B. J (Portugal: Department of Environment and Planning, University of Aveiro), 3–14. doi:10.2495/AIR210011
Baker, F., and Smith, C. (2019). A GIS and object based image analysis approach to mapping the greenspace composition of domestic gardens in Leicester, UK. Landsc. Urban Plan. 183, 133–146. doi:10.1016/j.landurbplan.2018.12.002
Baró, F., and Gómez-Baggethun, E. (2017). Assessing the potential of regulating ecosystem services as nature-based solutions in urban areas, 139–158. doi:10.1007/978-3-319-56091-5_9
Basu, A. S., Pilla, F., Sannigrahi, S., Gengembre, R., Guilland, A., and Basu, B. (2021). Theoretical framework to assess green roof performance in mitigating urban flooding as a potential nature-based solution. Sustainability 13, 13231. doi:10.3390/su132313231
Beumer, C., and Martens, P. (2016). BIMBY’s first steps: a pilot study on the contribution of residential front-yards in Phoenix and Maastricht to biodiversity, ecosystem services and urban sustainability. Urban Ecosyst. 19, 45–76. doi:10.1007/s11252-015-0488-y
Blanuša, T., and Hadley, J. (2019). Impact of plant choice on rainfall runoff delay and reduction by hedge species. Landsc. Ecol. Eng. 15, 401–411. doi:10.1007/s11355-019-00390-x
Blanuša, T., Hadley, J., Hunt, L., Alexander, P., and Hobbs, K. (2017). Provision of ecosystem services by hedges in urban domestic gardens: focus on rainfall mitigation. Acta Hortic., 519–524. doi:10.17660/ActaHortic.2017.1189.104
Blanuša, T., Qadir, Z. J., Kaur, A., Hadley, J., and Gush, M. B. (2020). Evaluating the effectiveness of urban hedges as air pollution barriers: importance of sampling method, species characteristics and site location. Environ. - MDPI 7, 81–21. doi:10.3390/environments7100081
Boldrin, D., Knappett, J. A., Leung, A. K., Brown, J. L., Loades, K. W., and Bengough, A. G. (2022). Modifying soil properties with herbaceous plants for natural flood risk-reduction. Ecol. Eng. 180, 106668. doi:10.1016/j.ecoleng.2022.106668
Bormann, F. H., Balmori, D., and Geballe, G. T. (1994). Redesigning the American lawn: a search for environmental harmony. J. Interdiscip. Hist. 25, 351. doi:10.2307/206387
Bouzouidja, R., Leconte, F., Kiss, M., Pierret, M., Pruvot, C., Détriché, S., et al. (2021). Experimental comparative study between conventional and green parking lots: analysis of subsurface thermal behavior under warm and dry summer conditions. Atmos. (Basel). 12, 994. doi:10.3390/atmos12080994
Bown, M. J., and Sutton, A. J. (2010). Quality control in systematic reviews and meta-analyses. Eur. J. Vasc. Endovasc. Surg. 40, 669–677. doi:10.1016/j.ejvs.2010.07.011
Cabral, I., Keim, J., Engelmann, R., Kraemer, R., Siebert, J., and Bonn, A. (2017). Ecosystem services of allotment and community gardens: a Leipzig, Germany case study. Urban For. Urban Green. 23, 44–53. doi:10.1016/j.ufug.2017.02.008
Cameron, R. (2023). Do we need to see gardens in a new light? Recommendations for policy and practice to improve the ecosystem services derived from domestic gardens. Urban For. Urban Green. 80, 127820. doi:10.1016/j.ufug.2022.127820
Cameron, R. W. F., Blanuša, T., Taylor, J. E., Salisbury, A., Halstead, A. J., Henricot, B., et al. (2012). The domestic garden – its contribution to urban green infrastructure. Urban For. Urban Green. 11, 129–137. doi:10.1016/j.ufug.2012.01.002
Canet-Marti, A., Pineda-Martos, R., Junge, R., Bohn, K., Paco, T. A., Delgado, C., et al. (2021). Nature-based solutions for agriculture in circular cities: challenges, gaps, and opportunities. WATER 13, 2565. doi:10.3390/w13182565
Cook, E. M., Hall, S. J., and Larson, K. L. (2012). Residential landscapes as social-ecological systems: a synthesis of multi-scalar interactions between people and their home environment. Urban Ecosyst. 15, 19–52. doi:10.1007/s11252-011-0197-0
Costa, S., Peters, R., Martins, R., Postmes, L., Keizer, J. J., and Roebeling, P. (2021). Effectiveness of nature-based solutions on pluvial flood hazard mitigation: the case study of the city of eindhoven (The Netherlands). Resources 10, 24. doi:10.3390/resources10030024
Daudin, K., Weber, C., Fouché, O., and Maton, L. (2022). Soil-water adaptive management process: the case of on-site wastewater treatment systems in peri-urban areas in France. Environ. Challenges 7, 100506. doi:10.1016/j.envc.2022.100506
Davies, Z. G., Edmondson, J. L., Heinemeyer, A., Leake, J. R., and Gaston, K. J. (2011). Mapping an urban ecosystem service: quantifying above-ground carbon storage at a city-wide scale. J. Appl. Ecol. 48, 1125–1134. doi:10.1111/j.1365-2664.2011.02021.x
Davis, M., and Naumann, S. (2017). Making the case for sustainable urban drainage systems as a nature-based solution to urban flooding. Springer Open, 123–137. doi:10.1007/978-3-319-56091-5_8
den Heijer, C., and Coppens, T. (2023). Paying for green: A scoping review of alternative financing models for nature-based solutions. J. Environ. Manage. 337, 117754. doi:10.1016/j.jenvman.2023.117754
Department of Economic and Social Affairs, U. N. (2019). World population prospects 2019: highlights, 2019. 46. New York City: Dep. Econ. Soc. Aff. World Popul. Prospect.
Derkzen, M. L., Teeffelen, A. J. A. V., and Verburg, P. H. (2015). Quantifying urban ecosystem services based on high-resolution data of urban green space: an assessment for Rotterdam, The Netherlands 1020–1032. doi:10.1111/1365-2664.12469
Dewaelheyns, V. (2014). “The garden complex in strategic perspective. The case of Flanders,” in Dissertation presented in partial fulfilment of the requirements for the degree of Doctor in Bioscience Engineering. Leuven: KU.
Dewaelheyns, V., Elsen, A., Vandendriessche, H., and Gulinck, H. (2013). Garden management and soil fertility in Flemish domestic gardens. Landsc. Urban Plan. 116, 25–35. doi:10.1016/j.landurbplan.2013.03.010
Dewaelheyns, V., Jakobsson, A., and Saltzman, K. (2018). Strategic gardens and gardening: inviting a widened perspective on the values of private green space. Urban For. Urban Green. 30, 207–209. doi:10.1016/j.ufug.2017.12.009
Dewaelheyns, V., Kerselaers, E., and Rogge, E. (2016). A toolbox for garden governance. Land use policy 51, 191–205. doi:10.1016/j.landusepol.2015.11.016
Dewaelheyns, V., Rogge, E., and Gulinck, H. (2014). Putting domestic gardens on the agenda using empirical spatial data: the case of Flanders. Appl. Geogr. 50, 132–143. doi:10.1016/j.apgeog.2014.02.011
Dobson, M. C., Crispo, M., Blevins, R. S., Warren, P. H., and Edmondson, J. L. (2021). An assessment of urban horticultural soil quality in the United Kingdom and its contribution to carbon storage. Sci. Total Environ. 777, 146199. doi:10.1016/j.scitotenv.2021.146199
Dorst, H., van der Jagt, A., Raven, R., and Runhaar, H. (2019). Urban greening through nature-based solutions – key characteristics of an emerging concept. Sustain. Cities Soc. 49, 101620. doi:10.1016/j.scs.2019.101620
Douglas, I., and James, P. (2014). Urban ecology, urban ecology: an introduction. London: Routledge. doi:10.4324/9780203108703
Drepper, B., Bamps, B., Gobin, A., and Van Orshoven, J. (2021). Strategies for managing spring frost risks in orchards: effectiveness and conditionality—a systematic review protocol. Environ. Evid. 10, 32–13. doi:10.1186/s13750-021-00247-7
Duddigan, S., Alexander, P. D., Shaw, L. J., and Collins, C. D. (2021). Effects of repeated application of organic soil amendments on horticultural soil physicochemical properties, nitrogen budget and yield. Horticulturae 7, 371. doi:10.3390/horticulturae7100371
Duddigan, S., Alexander, P. D., Shaw, L. J., Sandén, T., and Collins, C. D. (2020). The tea bag index-UK: using citizen/community science to investigate organic matter decomposition rates in domestic gardens. Sustain 12, 6895. doi:10.3390/SU12176895
Edmondson, J. L., Davies, Z. G., Gaston, K. J., and Leake, J. R. (2014a). Urban cultivation in allotments maintains soil qualities adversely affected by conventional agriculture. J. Appl. Ecol. 51, 880–889. doi:10.1111/1365-2664.12254
Edmondson, J. L., Davies, Z. G., McCormack, S. A., Gaston, K. J., and Leake, J. R. (2014b). Land-cover effects on soil organic carbon stocks in a European city. Sci. Total Environ. 472, 444–453. doi:10.1016/j.scitotenv.2013.11.025
Eisenhauer, B. W., Brehm, J. M., Stevenson, N., and Peterson, J. (2016). Changing homeowners’ lawn care behavior to reduce nutrient runoff. Soc. Nat. Resour. 29, 329–344. doi:10.1080/08941920.2015.1062946
Ellison, D., Morris, C. E., Locatelli, B., Sheil, D., Cohen, J., Murdiyarso, D., et al. (2017). Trees, forests and water: cool insights for a hot world. Glob. Environ. Chang. 43, 51–61. doi:10.1016/j.gloenvcha.2017.01.002
Emilsson, T., and Ode Sang, Å. (2017). Impacts of climate change on urban areas and nature-based solutions for adaptation, 15–27. doi:10.1007/978-3-319-56091-5_2
Enzi, V., Cameron, B., Dezsényi, P., Gedge, D., Mann, G., and Pitha, U. (2017). Nature-based solutions to climate change adaptation in urban areas, 51–64. doi:10.1007/978-3-319-56091-5
Evans, D. L., Falagan, N., Hardman, C. A., Kourmpetli, S., Liu, L., Mead, B. R., et al. (2022). Ecosystem service delivery by urban agriculture and green infrastructure-a systematic review. Ecosyst. Serv. 54, 101405. doi:10.1016/j.ecoser.2022.101405
European Commission (2015). Towards an EU Research and Innovation policy agenda for Nature-Based Solutions & Re-Naturing Cities. doi:10.2777/765301
Ferreira, C. S. S., Kalantari, Z., Hartmann, T., and Pereira, P. (2021). “Introduction: nature-based solutions for flood mitigation,” in Handbook of environmental chemistry, 1–7. doi:10.1007/698_2021_776
Frank Lee (2023). NK tegelwippen. Available at: https://www.nk-tegelwippen.nl/.
Gaston, K. J., Warren, P. H., Thompson, K., and Smith, R. M. (2005). Urban domestic gardens (IV): the extent of the resource and its associated features. Biodivers. Conserv. 14, 3327–3349. doi:10.1007/s10531-004-9513-9
Gemeente Waalwijk (2023). Tegeltaxi haalt gratis tegels op. Available at: https://duurzaam.waalwijk.nl/tegeltaxi-haalt-gratis-tegels-op.
Gerits, F., Reubens, B., Messely, L., De Smedt, P., Landuyt, D., Loos, A., et al. (2022). Disentangling the interrelated abiotic and biotic pathways linking landscape composition and crop production. J. Appl. Ecol. 59, 2742–2755. doi:10.1111/1365-2664.14269
Grard, B. J. P., Manouchehri, N., Aubry, C., Frascaria-Lacoste, N., and Chenu, C. (2020). Potential of technosols created with urban by-products for rooftop edible production. Int. J. Environ. Res. Public Health 17, 3210–3221. doi:10.3390/ijerph17093210
Haase, D., Jänicke, C., and Wellmann, T. (2019). Front and back yard green analysis with subpixel vegetation fractions from earth observation data in a city. Landsc. Urban Plan. 182, 44–54. doi:10.1016/j.landurbplan.2018.10.010
Haddaway, N. R., Macura, B., Whaley, P., and Pullin, A. S. (2018). ROSES Reporting standards for Systematic Evidence Syntheses: pro forma, flow-diagram and descriptive summary of the plan and conduct of environmental systematic reviews and systematic maps. Environ. Evid. 7, 7–11. doi:10.1186/s13750-018-0121-7
Harris, E. M., Polsky, C., Larson, K. L., Garvoille, R., Martin, D. G., Brumand, J., et al. (2012). Heterogeneity in residential yard care: evidence from Boston, Miami, and phoenix. Hum. Ecol. 40, 735–749. doi:10.1007/s10745-012-9514-3
Hedblom, M., Lindberg, F., Vogel, E., Wissman, J., and Ahrné, K. (2017). Estimating urban lawn cover in space and time: case studies in three Swedish cities. Urban Ecosyst. 20, 1109–1119. doi:10.1007/s11252-017-0658-1
Heikoop, T. H. (2022). Assessing soil sealing and ecosystem services of urban front yards using Google Street View: a case study in Bloemhof district Rotterdam, The Netherlands. IOP Conf. Ser. Earth Environ. Sci. 955, 012019. doi:10.1088/1755-1315/955/1/012019
Hellies, M., Deidda, R., and Viola, F. (2018). Retention performances of green roofs worldwide at different time scales. L. Degrad. Dev. 29, 1940–1952. doi:10.1002/ldr.2947
Hewett, C. J. M., Wilkinson, M. E., Jonczyk, J., and Quinn, P. F. (2020). Catchment systems engineering: an holistic approach to catchment management. Wiley Interdiscip. Rev. Water 7. doi:10.1002/wat2.1417
Huang, Y., Tian, Z., Ke, Q., Liu, J., Irannezhad, M., Fan, D., et al. (2020). Nature-based solutions for urban pluvial flood risk management. WIREs Water 7. doi:10.1002/wat2.1421
Ignatieva, M., Haase, D., Dushkova, D., and Haase, A. (2020). Lawns in cities: from a globalised urban green space phenomenon to sustainable nature-based solutions. Land 9, 73. doi:10.3390/land9030073
Ignatieva, M., and Hedblom, M. (2018). An alternative urban green carpet: how can we move to sustainable lawns in a time of climate change? Sci. (80-.) 362, 148–149. doi:10.1126/science.aau6974
Jaffal, I., Ouldboukhitine, S.-E., and Belarbi, R. (2012). A comprehensive study of the impact of green roofs on building energy performance. Renew. Energy 43, 157–164. doi:10.1016/j.renene.2011.12.004
Kabisch, N., Korn, H., Stadler, J., and Bonn, A. (2017). Nature-based solutions to climate change adaptation in urban areas—linkages between science, policy and practice. Policy Pract., 1–11. doi:10.1007/978-3-319-56091-5_1
Keßler, S., Bierl, R., Meyer, B., and Krein, A. (2017). Chemical effects of a near-to-nature detention pond on a small urban headwater. Limnologica 62, 118–125. doi:10.1016/j.limno.2016.11.006
Knack (2023). Doe niets voor de natuur: Maai Mei Niet. Available at: https://maaimeiniet.be/.
Knight, T., Price, S., Bowler, D., Hookway, A., King, S., Konno, K., et al. (2021). How effective is ‘greening’ of urban areas in reducing human exposure to ground-level ozone concentrations, UV exposure and the ‘urban heat island effect’? An updated systematic review. Environ. Evid. 10, 12. doi:10.1186/s13750-021-00226-y
Kotzen, B. (2018). Economic benefits and costs of green streets. Nat. Based Strategies Urban Build. Sustain., 319–331. doi:10.1016/B978-0-12-812150-4.00029-X
Kotzen, B. (2021). Future cities: speculation on the case for vertical biophilic cities. Ecocycles 7, 59–80. doi:10.19040/ecocycles.v7i2.192
Krauze, K., and Wagner, I. (2019). From classical water-ecosystem theories to nature-based solutions — contextualizing nature-based solutions for sustainable city. Sci. Total Environ. 655, 697–706. doi:10.1016/j.scitotenv.2018.11.187
Krivtsov, V., Birkinshaw, S., Olive, V., Lomax, J., Christie, D., and Arthur, S. (2022). Multiple benefits of blue-green infrastructure and the reduction of environmental risks: case study of ecosystem services provided by a SUDS pond. Springer Tracts Civ. Eng., 247–262. doi:10.1007/978-981-16-5312-4_17
Krols, J., Aerts, R., Vanlessen, N., Dewaelheyns, V., Dujardin, S., and Somers, B. (2022). Residential green space, gardening, and subjective well-being: a cross-sectional study of garden owners in northern Belgium. Landsc. Urban Plan. 223, 104414. doi:10.1016/j.landurbplan.2022.104414
Kumar, P., Debele, S. E., Sahani, J., Rawat, N., Marti-Cardona, B., Alfieri, S. M., et al. (2021). An overview of monitoring methods for assessing the performance of nature-based solutions against natural hazards. Earth-Science Rev. 217, 103603. doi:10.1016/j.earscirev.2021.103603
Laćan, I., Moanga, D., McBride, J. R., and Butsic, V. (2020). “Sealed in San José:” Paving of front yards diminishes urban forest resource and benefits in low-density residential neighborhoods. Urban For. Urban Green. 54, 126755. doi:10.1016/j.ufug.2020.126755
Lakho, F. H., Qureshi, A., Novelli, L. D. D., Depuydt, V., Depreeuw, T., Van Hulle, S. W. H., et al. (2022). Performance of a green wall (total value WallTM) at high greywater loading rates and life cycle impact assessment. Sci. Total Environ. 821, 153470. doi:10.1016/j.scitotenv.2022.153470
Langemeyer, J., Latkowska, M. J., Gómez-Baggethun, E. N., Voigt, A., Calvet-Mir, L., Pourias, J., et al. (2019). Ecosystem services from urban gardens. Urban Allot. Gard. Eur., 115–141. doi:10.4324/9781315686608-6
Langergraber, G., Castellar, J. A. C., Andersen, T. R., Andreucci, M. B., Baganz, G. F. M., Buttiglieri, G., et al. (2021). Towards a cross-sectoral view of nature-based solutions for enabling circular cities. WATER 13, 2352. doi:10.3390/w13172352
Langergraber, G., Pucher, B., Simperler, L., Kisser, J., Katsou, E., Buehler, D., et al. (2020). Implementing nature-based solutions for creating a resourceful circular city. Blue-Green Syst. 2, 173–185. doi:10.2166/bgs.2020.933
Larson, K. L., Casagrande, D., Harlan, S. L., and Yabiku, S. T. (2009). Residents’ yard choices and rationales in a desert city: social priorities, ecological impacts, and decision tradeoffs. Environ. Manag. (New York) 44, 921–937. doi:10.1007/s00267-009-9353-1
Larson, K. L., Lerman, S. B., Nelson, K. C., Narango, D. L., Wheeler, M. M., Groffman, P. M., et al. (2022). Examining the potential to expand wildlife-supporting residential yards and gardens. Landsc. Urban Plan. 222, 104396. doi:10.1016/j.landurbplan.2022.104396
Lerman, S. B., and Contosta, A. R. (2019). Lawn mowing frequency and its effects on biogenic and anthropogenic carbon dioxide emissions. Landsc. Urban Plan. 182, 114–123. doi:10.1016/j.landurbplan.2018.10.016
Lerman, S. B., Contosta, A. R., Milam, J., and Bang, C. (2018). To mow or to mow less: lawn mowing frequency affects bee abundance and diversity in suburban yards. Biol. Conserv. 221, 160–174. doi:10.1016/j.biocon.2018.01.025
Leroy, B. L. M., Herath, M. S. K., De Neve, S., Gabriels, D., Bommele, L., Reheul, D., et al. (2008). Effect of vegetable, fruit and garden (VFG) waste compost on soil physical properties. Compost Sci. Util. 16, 43–51. doi:10.1080/1065657X.2008.10702354
Loram, A., Tratalos, J., Warren, P. H., and Gaston, K. J. (2007). Urban domestic gardens (X): the extent & structure of the resource in five major cities. Landsc. Ecol. 22, 601–615. doi:10.1007/s10980-006-9051-9
Loram, A., Warren, P., Thompson, K., and Gaston, K. (2011). Urban domestic gardens: the effects of human interventions on garden composition. Environ. Manage. 48, 808–824. doi:10.1007/s00267-011-9723-3
Mancebo, F. (2018). Gardening the city: addressing sustainability and adapting to global warming through urban agriculture. Environ. - MDPI 5, 38–11. doi:10.3390/environments5030038
Mathieu, R., Freeman, C., and Aryal, J. (2007). Mapping private gardens in urban areas using object-oriented techniques and very high-resolution satellite imagery. Landsc. Urban Plan. 81, 179–192. doi:10.1016/j.landurbplan.2006.11.009
McPhearson, T., Kabisch, N., and Frantzeskaki, N. (2023). Nature-based solutions for cities, Cheltenham, UK: Edward Elgar Publishing. doi:10.4337/9781800376762
McPherson, E. G., Nowak, D. J., and Rowntree, R. A. (1994). Chicago’s urban forest ecosystem: results of the Chicago urban forest climate project.(includes executive summary). For. Serv. general Tech. Rep. (Final). doi:10.2737/NE-GTR-186
McVittie, A., Cole, L., Wreford, A., Sgobbi, A., and Yordi, B. (2018). Ecosystem-based solutions for disaster risk reduction: lessons from European applications of ecosystem-based adaptation measures. Int. J. Disaster Risk Reduct. 32, 42–54. doi:10.1016/j.ijdrr.2017.12.014
MEA (2004). Ecosystems and human well-being: a framework for assessment. Choice Rev. Online. doi:10.5860/choice.41-4645
MillionTreesNYC (2015). MillionTreesNYC | A plaNYC initiative with NYC parks and New York restoration project. Available at: https://www.milliontreesnyc.org/.
Mobilia, M., D’Ambrosio, R., Claverie, R., and Longobardi, A. (2021). “Substrate soil moisture impact on green roof performance for an experimental site in tomblaine, France,” in Computational science and its applications, iccsa 2021. Editors O. Gervasi, B. Murgante, S. Misra, C. Garau, I. Blecic, D. Taniaret al. PT II, 563–570. doi:10.1007/978-3-030-86960-1_39
Monteiro, M. V., Blanuša, T., Verhoef, A., Richardson, M., Hadley, P., and Cameron, R. W. F. (2017). Functional green roofs: importance of plant choice in maximising summertime environmental cooling and substrate insulation potential. Energy Build. 141, 56–68. doi:10.1016/j.enbuild.2017.02.011
Nassauer, J. I., Cooper, D. A., Marshall, L. L., Currie, W. S., Hutchins, M., and Brown, D. G. (2014). Parcel size related to household behaviors affecting carbon storage in exurban residential landscapes. Landsc. Urban Plan. 129, 55–64. doi:10.1016/j.landurbplan.2014.05.007
Nassauer, J. I., Wang, Z., and Dayrell, E. (2009). What will the neighbors think? Cultural norms and ecological design. Landsc. Urban Plan. 92, 282–292. doi:10.1016/j.landurbplan.2009.05.010
Nemitz, E., Vieno, M., Carnell, E., Fitch, A., Steadman, C., Cryle, P., et al. (2020). Potential and limitation of air pollution mitigation by vegetation and uncertainties of deposition-based evaluations: air pollution mitigation by vegetation. Philos. Trans. R. Soc. A Math. Phys. Eng. Sci. 378, 20190320. doi:10.1098/rsta.2019.0320
Nika, C. E., Gusmaroli, L., Ghafourian, M., Atanasova, N., Buttiglieri, G., and Katsou, E. (2020). Nature-based solutions as enablers of circularity in water systems: a review on assessment methodologies, tools and indicators. Water Res. 183, 115988. doi:10.1016/j.watres.2020.115988
Nowak, D. J., and Greenfield, E. J. (2020). The increase of impervious cover and decrease of tree cover within urban areas globally (2012–2017). Urban For. Urban Green. 49, 126638. doi:10.1016/j.ufug.2020.126638
Omar, M., Al Sayed, N., Barré, K., Halwani, J., and Machon, N. (2018). Drivers of the distribution of spontaneous plant communities and species within urban tree bases. Urban For. Urban Green. 35, 174–191. doi:10.1016/j.ufug.2018.08.018
Omgeving, D., and vzw, B. (2023). Vlaams Kampioenschap tegelwippen. Available at: https://vk-tegelwippen.be/.
Oral, H. V., Carvalho, P., Gajewska, M., Ursino, N., Masi, F., van Hullebusch, E. D., et al. (2020). A review of nature-based solutions for urban water management in European circular cities: a critical assessment based on case studies and literature. Blue-Green Syst. 2, 112–136. doi:10.2166/bgs.2020.932
Orta-Ortiz, M. S., and Geneletti, D. (2021). “Reviewing the performance of nature-based solutions for stormwater management in urban areas,” in Springer science and business media deutschland GmbH, department of civil, environmental and mechanical engineering, university of trento, via mesiano, 77, trento, 38123, Italy. Editor L. R. R. P. D, 15–22. doi:10.1007/978-3-030-68824-0_2
Orta-Ortiz, M. S., and Geneletti, D. (2022). What variables matter when designing nature-based solutions for stormwater management? A review of impacts on ecosystem services. Environ. Impact Assess. Rev. 95, 106802. doi:10.1016/j.eiar.2022.106802
Pauleit, S., and Duhme, F. (2000). Assessing the environmental performance of land cover types for urban planning. Landsc. Urban Plan. 52, 1–20. doi:10.1016/S0169-2046(00)00109-2
Pauleit, S., Zölch, T., Hansen, R., Randrup, T. B., and Konijnendijk van den Bosch, C. (2017). “Nature-based solutions and climate change – four shades of green,” in Nature-based solutions to climate change adaptation in urban areas: linkages between science, policy and practice. Editors N. Kabisch, H. Korn, J. Stadler, and A. Bonn, 29–49. doi:10.1007/978-3-319-56091-5_3
Pearlmutter, D., Pucher, B., Calheiros, C. S. C., Hoffmann, K. A., Aicher, A., Pinho, P., et al. (2021). Closing water cycles in the built environment through nature-based solutions: the contribution of vertical greening systems and green roofs. Water 13, 2165. doi:10.3390/w13162165
Pearlmutter, D., Theochari, D., Nehls, T., Pinho, P., Piro, P., Korolova, A., et al. (2020). Enhancing the circular economy with nature-based solutions in the built urban environment: green building materials, systems and sites. Blue-Green Syst. 2, 46–72. doi:10.2166/bgs.2019.928
Peel, M. C., Finlayson, B. L., and McMahon, T. A. (2007). Updated world map of the Köppen-Geiger climate classification. Hydrol. Earth Syst. Sci. 11, 1633–1644. doi:10.5194/hess-11-1633-2007
Pelfrêne, A., Sahmer, K., Waterlot, C., and Douay, F. (2019). From environmental data acquisition to assessment of gardeners’ exposure: feedback in an urban context highly contaminated with metals. Environ. Sci. Pollut. Res. 26, 20107–20120. doi:10.1007/s11356-018-3468-y
Pereira, P., Inácio, M., Karnauskaita, D., Bogdzevič, K., Gomes, E., Kalinauskas, M., et al. (2021). “Nature-based solutions impact on urban environment chemistry: air, soil, and water,” in Handbook of environmental chemistry, 89–148.
Perry, T., and Nawaz, R. (2008). An investigation into the extent and impacts of hard surfacing of domestic gardens in an area of Leeds, United Kingdom. Landsc. Urban Plan. 86, 1–13. doi:10.1016/j.landurbplan.2007.12.004
Plantlife (2023). No mow may. Available at: https://www.plantlife.org.uk/campaigns/nomowmay/.
Potschin, M., and Haines-Young, R. (2011). Ecosystem services: exploring a geographical perspective. Prog. Phys. Geogr. 35, 575–594. doi:10.1177/0309133311423172
Potschin, M., Haines-Young, R., Görg, C., Heink, U., Jax, K., and Schleyer, C. (2018). Understanding the role of conceptual frameworks: reading the ecosystem service cascade. Ecosyst. Serv. 29, 428–440. doi:10.1016/j.ecoser.2017.05.015
Qian, Y., and Follett, R. F. (2002). Assessing soil carbon sequestration in turfgrass systems using long-term SoilTesting data. Agronomy, 723–955. doi:10.2136/sssaj2009.0075
Qian, Y., Follett, R. F., and Kimble, J. M. (2010). Soil organic carbon input from urban turfgrasses. Soil Sci. Soc. Am. J. 74, 366–371. doi:10.2136/sssaj2009.0075
Qiu, Y., da Silva Rocha Paz, I., Chen, F., Versini, P.-A., Schertzer, D., and Tchiguirinskaia, I. (2021). Space variability impacts on hydrological responses of nature-based solutions and the resulting uncertainty: a case study of Guyancourt (France). Hydrol. Earth Syst. Sci. 25, 3137–3162. doi:10.5194/hess-25-3137-2021
Quaranta, E., Fuchs, S., Liefting, H. J., Schellart, A., and Pistocchi, A. (2022). Costs and benefits of combined sewer overflow management strategies at the European scale. J. Environ. Manage. 318, 115629. doi:10.1016/j.jenvman.2022.115629
Robbins, P. (2008). Lawn people: how grasses, weeds, and chemicals make us who we are. Prof. Geogr. 60, 425–426. doi:10.1080/00330120802115334
Roeland, S., Moretti, M., Amorim, J. H., Branquinho, C., Fares, S., Morelli, F., et al. (2019). Towards an integrative approach to evaluate the environmental ecosystem services provided by urban forest. J. For. Res. 30, 1981–1996. doi:10.1007/s11676-019-00916-x
Rozos, E., Makropoulos, C., and Maksimović, Č. (2013). Rethinking urban areas: an example of an integrated blue-green approach. Water Supply 13, 1534–1542. doi:10.2166/ws.2013.140
Runfola, D. M., Polsky, C., Nicolson, C., Giner, N. M., Pontius, R. G., Krahe, J., et al. (2013). A growing concern? Examining the influence of lawn size on residential water use in suburban Boston, MA, USA. Landsc. Urban Plan. 119, 113–123. doi:10.1016/j.landurbplan.2013.07.006
Salman, I. N. A., Schindler, B., Agra, H., Bawab, O., Friedman-Heiman, A., Kadas, G. J., et al. (2018). Green roof research towards enhancing urban biodiversity, storm-water retention and air pollution abatement. CABI Rev. 2018, 1–14. doi:10.1079/PAVSNNR201813039
Selhorst, A., and Lal, R. (2013). Net carbon sequestration potential and emissions in home lawn turfgrasses of the United States. Environ. Manage. 51, 198–208. doi:10.1007/s00267-012-9967-6
Sharath, M. K., and Peter, K. V. (2019). “Enviroscaping: an environment friendly landscaping,” in Sustainable Green Technologies for Environmental Management. Editors S. Shah, V. Venkatramanan, and R. Prasad (Singapore: Springer).
Shwartz, A., Muratet, A., Simon, L., and Julliard, R. (2013). Local and management variables outweigh landscape effects in enhancing the diversity of different taxa in a big metropolis. Biol. Conserv. 157, 285–292. doi:10.1016/j.biocon.2012.09.009
Sisser, J. M., Nelson, K. C., Larson, K. L., Ogden, L. A., Polsky, C., and Chowdhury, R. R. (2016). Lawn enforcement: how municipal policies and neighborhood norms influence homeowner residential landscape management. Landsc. Urban Plan. 150, 16–25. doi:10.1016/j.landurbplan.2016.02.011
Sitzenfrei, R., Kleidorfer, M., Bach, P. M., and Bacchin, T. K. (2020). Green infrastructures for urban water system: balance between cities and nature. Water 12, 1456. doi:10.3390/w12051456
Smith, P., Arneth, A., Barnes, D. K. A., Ichii, K., Marquet, P. A., Popp, A., et al. (2022). How do we best synergize climate mitigation actions to co-benefit biodiversity? Glob. Chang. Biol. 28, 2555–2577. doi:10.1111/gcb.16056
Sousa-Silva, R., Cameron, E., and Paquette, A. (2021). Prioritizing street tree planting locations to increase benefits for all citizens: experience from oliette, Canada. Front. Ecol. Evol. 9, 1–13. doi:10.3389/fevo.2021.716611
Southon, G. E., Jorgensen, A., Dunnett, N., Hoyle, H., and Evans, K. L. (2017). Biodiverse perennial meadows have aesthetic value and increase residents’ perceptions of site quality in urban green-space. Landsc. Urban Plan. 158, 105–118. doi:10.1016/j.landurbplan.2016.08.003
Speak, A. F., Mizgajski, A., and Borysiak, J. (2015). Allotment gardens and parks: provision of ecosystem services with an emphasis on biodiversity. Urban For. Urban Green. 14, 772–781. doi:10.1016/j.ufug.2015.07.007
Speak, A. F., Rothwell, J. J., Lindley, S. J., and Smith, C. L. (2013). Reduction of the urban cooling effects of an intensive green roof due to vegetation damage. Urban Clim. 3, 40–55. doi:10.1016/j.uclim.2013.01.001
Stad Leuven (2023). Leuvense tegeltaxi haalt tegels en puin gratis op tijdens VK Tegelwippen. Available at: https://pers.leuven.be/leuvense-tegeltaxi-haalt-tegels-en-puin-gratis-op-tijdens-vk-tegelwippen.
Strosse, V., Somers, B., and Van Valckenborgh, J. (2020). GARMON: the Garden Monitor - mapping and characterizing gardens using remote sensing.
Sušnik, J., Masia, S., Kravčík, M., Pokorný, J., and Hesslerová, P. (2022). Costs and benefits of landscape-based water retention measures as nature-based solutions to mitigating climate impacts in eastern Germany, Czech Republic, and Slovakia. L. Degrad. Dev. 33, 3074–3087. doi:10.1002/ldr.4373
Tits, M., Reynaert, S., Berx, C., Vaerten, J., Elsen, A., and Vandendiressche, H. (2021). Bodemvruchtbaarheid van tuinen, wijngaarden en openbaar groen in Vlaanderen en een blik op de sitatie in Wallonië en Nederland (2015-2020). Bodemkd. Dienst Belg.
Trees for Cities (2023). Trees for streets. Available at: https://www.treesforstreets.org/.
Tresch, S., Frey, D., Le Bayon, R.-C., Zanetta, A., Rasche, F., Fliessbach, A., et al. (2019). Litter decomposition driven by soil fauna, plant diversity and soil management in urban gardens. Sci. Total Environ. 658, 1614–1629. doi:10.1016/j.scitotenv.2018.12.235
Tresch, S., Moretti, M., Le Bayon, R.-C., Mäder, P., Zanetta, A., Frey, D., et al. (2018). A gardener’s influence on urban soil quality. Front. Environ. Sci. 6. doi:10.3389/fenvs.2018.00025
Valencia, E., Gross, N., Quero, J. L., Carmona, C. P., Ochoa, V., Gozalo, B., et al. (2018). Cascading effects from plants to soil microorganisms explain how plant species richness and simulated climate change affect soil multifunctionality. Glob. Chang. Biol. 24, 5642–5654. doi:10.1111/gcb.14440
Van Meerbeek, K., Muys, B., and Hermy, M. (2019). Lignocellulosic biomass for bioenergy beyond intensive cropland and forests. Renew. Sustain. Energy Rev. 102, 139–149. doi:10.1016/j.rser.2018.12.009
van Oorschot, J., Sprecher, B., van ’t Zelfde, M., van Bodegom, P. M., and van Oudenhoven, A. P. E. (2021). Assessing urban ecosystem services in support of spatial planning in the Hague, The Netherlands. Landsc. Urban Plan. 214, 104195. doi:10.1016/j.landurbplan.2021.104195
Veerkamp, C. J., Schipper, A. M., Hedlund, K., Lazarova, T., Nordin, A., and Hanson, H. I. (2021). A review of studies assessing ecosystem services provided by urban green and blue infrastructure. Ecosyst. Serv. 52, 101367. doi:10.1016/j.ecoser.2021.101367
Verbeeck, K., Van Rompuy, R., Hermy, M., and Van Orshoven, J. (2014). Infiltrating into the paved garden – a functional evaluation of parcel imperviousness in terms of water retention efficiency. J. Environ. Plan. Manag. 57, 1552–1571. doi:10.1080/09640568.2013.819317
Vermeiren, K., Crols, T., Uljee, I., De Nocker, L., Beckx, C., Pisman, A., et al. (2022). Modelling urban sprawl and assessing its costs in the planning process: a case study in Flanders, Belgium. Land use policy 113, 105902. doi:10.1016/j.landusepol.2021.105902
Wang, H. F., Qureshi, S., Knapp, S., Friedman, C. R., and Hubacek, K. (2015). A basic assessment of residential plant diversity and its ecosystem services and disservices in Beijing, China. Appl. Geogr. 64, 121–131. doi:10.1016/j.apgeog.2015.08.006
Warhurst, J. R., Parks, K. E., McCulloch, L., and Hudson, M. D. (2014). Front gardens to car parks: changes in garden permeability and effects on flood regulation. Sci. Total Environ. 485–486, 329–339. doi:10.1016/j.scitotenv.2014.03.035
Watkin, R., Vojinovic, W., Vojinovic, Z., Weesakul, S., and Torres, A. S. (2019). A framework for assessing benefits of implemented nature-based solutions. Sustainability 11, 6788. doi:10.3390/su11236788
Yan, J., Van der Linden, S., Tian, Y., Valckenborgh, J. V., Strosse, V., and Somers, B. (2022). Characterizing garden greenspace in a medieval European city: added values of spatial resolution and multi-temporal stereo imagery. Remote Sens. 14, 1169. doi:10.3390/rs14051169
Ziter, C. D., Pedersen, E. J., Kucharik, C. J., and Turner, M. G. (2019). Scale-dependent interactions between tree canopy cover and impervious surfaces reduce daytime urban heat during su. Washington, DC: pdf.
Keywords: systematic review, climate change adaptation, domestic gardens, Nature-based Solutions, ecosystem services
Citation: Teerlinck J, Wittemans K, Beele E, Dewaelheyns V, Steen T and Somers B (2024) What can Nature-based Solutions in domestic gardens contribute to climate change adaption in Western-Europe? a systematic review. Front. Environ. Sci. 12:1430739. doi: 10.3389/fenvs.2024.1430739
Received: 10 May 2024; Accepted: 05 August 2024;
Published: 23 August 2024.
Edited by:
Emma Ferranti, University of Birmingham, United KingdomReviewed by:
Giulio Senes, University of Milan, ItalyDanielle Sinnett, University of the West of England, United Kingdom
Copyright © 2024 Teerlinck, Wittemans, Beele, Dewaelheyns, Steen and Somers. This is an open-access article distributed under the terms of the Creative Commons Attribution License (CC BY). The use, distribution or reproduction in other forums is permitted, provided the original author(s) and the copyright owner(s) are credited and that the original publication in this journal is cited, in accordance with accepted academic practice. No use, distribution or reproduction is permitted which does not comply with these terms.
*Correspondence: Janne Teerlinck, janne.teerlinck@kuleuven.be