- 1Tillage and Cultivation Research Institute, Liaoning Academy of Agricultural Sciences, Shenyang, China
- 2Department of Agriculture and Environmental Science & Cooperative Research, Lincoln University of Missouri, Jefferson City, MO, United States
- 3Center of Agroforestry, University of Missouri, Columbia, MO, United States
Soil contamination by the munition explosive residues of 2,4,6-trinitrotoluene (TNT) and its metabolites resulting primarily from military operations has been identified as a threat to human health and ecosystems. Biodegradation by native plants to remove this hazardous compound or reduce its toxicity is considered a cost-effective and environmentally sound approach for the cleanup or restoration of TNT-contaminated soils. This study aims to investigate the TNT biodegradation and kinetics by two selected native grasses in the species-specific rhizosphere soils through growth chamber experiments. Native eastern gamma grass (Tripsacum dactyloides) and switchgrass (Panicum virgatum L.) were grown in soil spiked with 14C-TNT for 8 weeks. The 14C-TNT degradation and degradative metabolite profile in the rhizosphere soils were determined by liquid scintillation counter and high-performance liquid chromatography, respectively. The results indicated that both native grass species significantly enhanced the TNT degradation in the rhizosphere soils as compared with the control rhizosphere soils. More than 95% of the applied 14C-TNT was degraded in the first 7 days, and the rate then reached a steady state afterward, but less than 10% of the TNT applied was completely mineralized and transformed into CO2. The degradative reaction was found to follow second-order kinetics. Six major TNT degradative metabolites have been detected and identified in the rhizosphere soils. Overall, switchgrass appeared more effective for biodegrading TNT than eastern gamma grass. This research demonstrated that the native grass species, especially switchgrass, has the potential to mitigate the adverse human health and ecological risks of TNT-contaminated sites and can be considered an environmentally friendly, sustainable approach to safeguarding human health from TNT contamination.
Introduction
Soil contamination with munition explosives such as TNT (2,4,6-trinitrotoluene) resulting from military operations or wastewater discharge has been identified as a threat to human health and ecosystems. TNT is one of two explosive munitions most widely used in the world (USEPA, 2014). Massive TNT production started in 1902 and peaked during the two world wars (Nyanhongo et al., 2005). Since World War I, TNT has been used as a major explosive by the United States Army (Lewis et al., 2004). Annual TNT production was reported to be approximately one million kilograms (Harter and Ricket, 1985). The manufacture, use, and disposal of TNT explosives has resulted in environmental contamination, such as soil, water, or ecosystems, which is a public health and environmental concern (Pennington and Brannon, 2002). Wastewater generated during TNT production, also called “pink water,” could result in land contamination adjacent to the manufacturing facilities (Gilbert et al., 1980; Lindner, 1980). It was reported that up to 2 million liters of pink water could be produced annually by a single factory (Jenkins et al., 1986). At the Louisiana Army Ammunition Plant in Louisiana and the Cornhusker Army Ammunition Plant in Nebraska, land contamination by TNT was reported at up to 87,000 mg/kg in soil, 711,000 mg/kg in sediment, and 3,375 mg/L in surface water (Steevens et al., 2002). In the United States, more than 12 million tons of soil have been contaminated by the explosives (Lewis et al., 2004), and at least 20 of 1,397 hazardous waste sites listed on the USEPA National Priorities List have been identified as being contaminated by TNT (Agency for Toxic Substances and Disease Registry, 1995).
TNT is a highly toxic, single-ring nitroaromatic compound that is relatively persistent in the environment. Environmental contamination by TNT is a severe human health and environmental concern. Human exposure to TNT or other nitroaromatic compounds such as DNT (dinitrotoluene) and nitrophenols can cause several health problems, such as anemia, abnormal liver function, cataract development, skin irritation, and even cancers (Yinon, 1990; Honeycutt et al., 1996; Mulla and Talwar, 2013; Mulla et al., 2019). The TNT-exposure-related death of 475 people has been reported in the United States (McConnell and Flinn, 1946).
Conventional remediation approaches for TNT-contaminated sites include incineration, compost, or bioslurry. However, those remediation technologies are often destructive, high-cost, or labor-intensive (Habineza et al., 2016). In contrast, biodegradation to detoxify the TNT explosives by in situ microorganisms, green plants, or natural processes is often non-destructive with low maintenance and costs (Glick, 2003) and could be considered as a cost-effective, environmental-sound sustainable strategy for cleanup of the soils contaminated by the explosives (Habineza et al., 2016; Mulla et al., 2019).
Biodegradation of TNT by microorganisms has been extensively studied for decades (Habineza et al., 2016). Kao et al. (2016) studied indigenous microorganisms for TNT biodegradation in soil and concluded that the effectiveness was less satisfactory. Most previous studies showed that TNT or other nitroaromatic compounds in the environments preferred to be transformed into other degradative compounds rather than completely mineralized into CO2 (Habineza et al., 2016). Major TNT metabolites detected in the biodegradative pathway were 2-amino-4,6-dinitrotoluene (2-A-4,6-DNT), 4-amino-2,6- dinitrotoluene (4-A-2,6-DNT), 2,4-diamino-6-nitrotoluene (2,4-DA-6-NT), 2,6-diamino- 4-nitrotoluene (2,6-DA-4-NT) (Wang et al., 2010). Little TNT mineralization was reported (Hawari et al., 2000). This is probably due to the symmetric location of nitro groups on the toluene ring of TNT that limits enzymatic attack by classic dioxygenase during the microbial metabolism of aromatic compounds and the high electronegativity and partially positive charge of N atoms in the nitro group that make TNT easily reducible (Esteve-Nunez et al., 2001).
Biodegradation of TNT by native plants or grasses through a species-specific rhizosphere microbial community is little reported and remains largely unknown. The transgenic Arabidopsis plant study by Wang and Su (2024) indicated that TNT treatment would induce the overexpression of the CYP81D11 gene in Arabidopsis and enhance plant resistance or tolerance to TNT stress through enhanced TNT uptake rather than induced biodegradation. It has been suggested that native plants have a promising ability to degrade organic pollutants such as chlorinated hydrocarbons, nitroaromatic compounds, pesticides, and petroleum by species-specific microbial communities in rhizosphere soil (Anderson et al., 1993; Haby and Crowley, 1996; Reilley et al., 1996; Siciliano and Greer, 2000; Chaudhry and Schroeder, 2001; Schwitzguebel and Auberrt, 2001; Via and Zinnert, 2016). The objectives of this study were to use growth chamber experiments to i) investigate in situ TNT degradation and mineralization in rhizosphere soils by two selected native grasses, ii) determine the kinetics or mechanisms of native grass-specific TNT degradative processes, and iii) identify the profile of TNT metabolites in the degradative pathways.
Materials and methods
Experimental procedures: Two native grass species grown in Missouri, United States, Eastern gamma grass (Tripsacum dactyloides, EG) and Switchgrass (Panicum virgatum L, SW), were selected for this experiment based on our previous screening results. The seeds of EG and SW were sterilized in 30% ethanol for 30 s, rinsed with deionized water for 1 min, and then seeded in plastic pots (15 cm diameter, 20 cm height) filled with soil containing a mixture of 50% sand and 50% silt at a rate of 10 seeds per pot. The EG and SW pots, three pots per grass plus control (total nine pots), were grown in a walk-in growth chamber under the conditions of light intensity at 1,400 E m−2·sec−1, light/dark period at 15/9 h, humidity at 50%, and temperature at 25°C (light)/20°C (dark) for 8 weeks to develop microbial communities in grass species-specific rhizosphere soil. Soil moisture was maintained by adding deionized water weekly to a plastic pan placed under each pot. At maturity, fresh rhizosphere soils (soil near roots) were collected at the end of 8-week growth period from each of the EG or SW pots. The rhizosphere soils were collected by gently pulling five plants from each pot and carefully separating the soil from the roots. The soil samples were placed in plastic bags and stored at −4°C until treatment.
Twenty (20) grams of each of the collected fresh rhizosphere soils were treated with 0.5 μCi C14-TNT (PerkinElmer Life and Analytical Sciences) and 17.86 μg of 1,000 μg mL−1 TNT (Spex Certiprep, Inc.) to make total TNT concentration of 1.0 mg kg−1 soil. The C14 spiked soil was placed in 15-mL Erlenmeyer flasks, and a 20-mL scintillation vial containing 10 mL 1 M NaOH (Fisher Scientific) was also prepared as a 14CO2 trap. Both spiked soil flask and scintillation vial were placed in a mason jar and sealed with parafilm and duct tape. The jars were incubated at room temperature in the dark for 8 weeks. Each treatment was triplicated, and blank soil was included as controls. During the incubation period, soil moisture was maintained at 15%. The NaOH trap was replaced every week and stored at room temperature in the dark until 14CO2 analysis. Samples (1.0 g) of spiked soil were also collected from the jars at 0 days, 7 days, 14 days, 21 days, 42 days, and 56 days, respectively, and then extracted using 10 mL 100% acetonitrile (Fisher Scientific). The soil extracts were passed through a 0.45 μm filter, concentrated to 200 μL by an N2 evaporator, and stored at −200C prior to TNT analysis. After the extraction, the soils were oven-dried at 65°C overnight, ground into a fine powder, and stored in 20-mL scintillation vials at room temperature in the dark.
Analytical procedures: A 1.0 mL aliquot of the 10-mL 1 M NaOH traps was transferred into a 7 mL scintillation vial, mixed with 4 mL cocktail (Ultima Gold™ AB, PerkinElmer), and measured for 14CO2 radioactivity by a Beckman LS 6000SC liquid scintillation counter. The amount of C14-TNT in the traps was determined, and the TNT mineralization rate was calculated. A 0.5 g sample of the spiked soil after acetonitrile extraction was weighed in a 7-mL scintillation vial and mixed with 4 mL of cocktail (Ultima Gold™ AB, PerkinElmer). The mixtures were vortexed, allowed to precipitate for 3 days, and measured for 14CO2 radioactivity by a Beckman LS 6000SC liquid scintillation counter. The amount of non-extractable TNT fraction in soil was determined. Concentrations of acetonitrile-extractable C14-TNT and its metabolites were analyzed by a Shimadzu High Performance Liquid Chromatogram equipped with a Phenomenex Columbus C8110A column and a radioactivity detector. The mobile phase consisted of 100% acetonitrile and 0.1% phosphoric acid (1:9, v:v) at a flow rate of 1 mL min−1.
Statistical analysis: Data were analyzed using non-parametric two-way ANOVA for treatment using SAS software.
Results and discussion
TNT degradation
As the data in Figure 1 show, the disappearance of TNT in the rhizosphere soil appeared to be rapid during the first 7 days and gradually leveled off during the incubation period. It was estimated that more than 95% of the applied TNT degraded in the first 7 days. Two selected native grasses significantly enhanced the TNT degradation compared with the control, but the degradative capacity of the two grasses did not significantly differ. The grasses’ ability to enhance the TNT degradation was less effective than expected under the experimental conditions. Enhanced degradation in the rhizosphere soil by the native grasses could be a result of the biodegradative or biochemical reactions mediated by enriched specific microbial communities due to the activity of the grass roots. It is well known that rhizosphere soil differs in chemical and biochemical properties such as pH, redox, and organic composition from bulk soil, as induced by plant root activities, including root exudation, respiration, and nutrient uptake. Plant species could create differential or species-specific biochemical and ecological conditions in the rhizosphere because of genetic differences. The unique rhizosphere environment may help develop or differentiate a species-specific microbial group or community enabled to attack nitroaromatic compounds and promote TNT degradation or detoxification (Su et al., 2024). The rapid degradation of TNT in the early incubation stage has been observed in the previous studies by Hawari et al. (2000). This suggested that the original TNT molecule may be transformed to other nitroaromatic forms without a complete cleavage of the aromatic ring rather than a complete mineralization to CO2. As reported previously, the chemical structure of TNT could strongly limit the possibility of aromatic ring cleavage. In this case, the TNT that disappeared in the rhizosphere soil might be transformed into nitroso-, hydroxylamino-, or amino-TNT derivatives because of the easily reducible nitro groups (Wang et al., 2010).
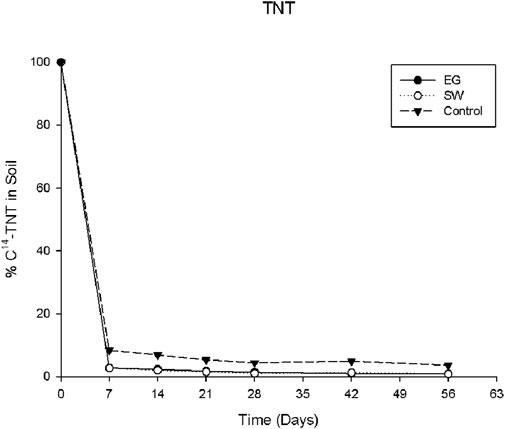
Figure 1. Percentage of applied C14-TNT remaining in the rhizosphere soil of EG and SW grasses during the 8-week incubation period.
Major mechanisms of TNT biodegradation have been proposed, including successive two-electron reduction and denitration (Nyanhongo et al., 2005; Smets et al., 2007), which involves a sequential reduction of TNT to nitroso-TNT, hydroxylamino-TNT, and amino-TNT derivatives. The degradative intermediates that were frequently detected included 2-hydroxylamino-4,6-dinitrotoluene (2HA46DNT), 4-hydroxylamino-2,6-dinitrotoluene (4HA26DNT), 2-amino-4,6-dinitrotoluene (2A46DNT), 4-amino-2,6-dinitrotoluene (4A26DNT) and 2,4-diamino-6-nitrotoluene. No nitroso derivative was detected (Koder and Miller, 1998; Esteve-Nunez et al., 2001; Yin et al., 2004; Nyanhongo et al., 2005; Yin et al., 2005). These partially reduced TNT intermediates have been reported to react with each other in the presence of oxygen to form azoxytetranitrotoluene, which is more recalcitrant and mutagenic than TNT (Haidour and Ramos, 1996; George et al., 2001). Although the oxygenolytic metabolism of TNT rarely occurred, a possible oxygenolytic attack of aminodinitrotoluene was observed. 2-amino-4,6-dinitrotoluene and 4-amino-2,6- dinitrotoluene has been reported to be oxidized to 3-amino-4-methyl-5-nitrocatechol and 3-amino-6methyl-5-nitrocatechol with the release of nitrite, respectively (Johnson et al., 2001). Hydroxylamino-TNT derivatives were observed to go through a Bamberger-like rearrangement to produce vicinal amino and hydroxyl substituents with the release of ammonium (Hughes et al., 1998; Caballero et al., 2005a; Caballero et al., 2005b; Caballero and Ramos, 2006).
Denitration refers to the direct hydride addition to the aromatic ring of TNT with the formation of a TNT hydride-Meisenheimer complex (H−-TNT) (Esteve-Nunez et al., 2001; Nyanhongo et al., 2005; Smets et al., 2007). H−-TNT would be quickly converted to a TNT dihydride-Meisenheimer complex (2H−-TNT ). 2H−-TNT is an unstable orange product that could be further transformed with nitrite release. A one-electron reduction of TNT may also occur (Spain, 1995; Esteve-Nunez et al., 2001). One nitro group of TNT could be reduced to a nitroso group with the formation of a nitroanion radical. This nitroanion radical would react with oxygen to form a superoxide and original nitro aromatic compound through a futile cycle.
Degradation kinetics
TNT degradation in soil can be considered an irreversible reaction. The rate law of TNT degradation can be expressed as follows:
where [TNT] is concentration, t is time, k is a constant, and a is kinetic order. If n = 0, the TNT degradation would be a zero-order reaction, and the equation can be rewritten as follows:
Then, the equation can be integrated as follows:
If n = 1 or 2, the TNT degradation would be a first- or second-order reaction, and the equation can be integrated as follows:
or
When [TNT], ln[TNT], or 1/[TNT] is plotted against the incubation time, a linear line would indicate the kinetic order for the TNT degradation. Based on data shown in Figure 1, in all cases, the TNT biodegradation in rhizosphere soil by both grasses presented second-order reaction kinetics (Figure 2). The regression coefficients of the linearity were r2 = 0.9525, r2 = 0.8514, and r2 = 0.8002 in EG and SW rhizosphere soils and control soil, respectively. This second-order kinetics indicated that, like most biodegradation processes of organic contaminants, the biodegradation of TNT was a microbial-driven transformation reaction. The degradation rate was initially fast and then gradually reached a steady state, as shown in Figure 1. This suggested that oxygen availability or TNT concentration may be a primary rate-limiting factor during the biodegradation process.
TNT mineralization
As indicated in Figure 1, the two selected native grasses significantly enhanced the TNT degradation or biotransformation in the rhizosphere soils. More than 95% of applied TNT was degraded in the first 7 days, and the rate remained in a steady state afterward during the incubation. Among the amount of the TNT degraded, only a fraction of the applied TNT was completely mineralized into harmless CO2 by the native grasses, as indicated in Figure 3. It was estimated that approximately 10% and 8% of applied TNT was transformed into CO2 by SW and EG, respectively, during the 8 weeks compared with 5% in the control. Both SW and EG demonstrated a significantly higher capacity than the control to enhance or stimulate the complete TNT mineralization or CO2 transformation, and SW was shown to be more effective than EG. This result was still less satisfactory and consistent with previous reports (Habineza et al., 2016), indicating that only a limited fraction of TNT applied had experienced complete cleavage of the aromatic ring and larger amounts of TNT were transformed to smaller molecules rather than CO2. A relatively low TNT mineralization rate was also reported in many other studies (Hawari et al., 2000; Nyanhongo et al., 2005). Meanwhile, the chemical-stable ring structure or properties of TNT that make the aromatic ring intact could resist or limit TNT mineralization. In addition to the TNT applied, the mineralization may also result from the TNT metabolites or smaller degradative molecules in the rhizosphere that were attacked by the species-specific activity of the soil rhizosphere microbes enhanced by native EG and SW grasses, causing the aromatic ring cleavage and transformation of CO2.
TNT residue
TNT residue was the fraction of non-extractable TNT remaining in the soil after acetonitrile extraction and presented the fraction of non-degradable TNT. TNT and its degradative metabolites may be able to interact with clays and humic substances in soil, which form relatively stable compounds resistant to acetonitrile extraction. Thus, those residual TNT compounds in soil could be considered less toxic or non-harmful fractions to the environment and human health because of their very limited bioavailability and leachability. The more non-extractable compounds are in soil, the less toxic they are to humans and ecosystems. Data presented in Figure 4 indicate that there was no significant difference among the treatments in the amounts of non-extractable TNT in the rhizosphere soils. In all cases, the non-extractable contents increased rapidly in the first 7 days of the incubation period and then remained relatively stable at around 6%–7% afterward, indicating that the rapid formation of relatively stable TNT complexes could prevent TNT from further degradation or mineralization by soil microorganisms. This was in agreement with the degradation data presented in Figure 1, which shows that approximately 95% TNT was degraded during the incubation.
TNT degradative metabolites
Six major degradative metabolites of TNT have been identified in the acetonitrile extracts of the rhizosphere soils collected (Figure 5), which included 4-nitrotoluene (4NT), 2-amino-4,6-dinitrotoluene (2A46DNT), 4-amino-2,6-dinitrotoluene (4A26DNT), 4-hydroxylamino-2,6-dinitrotoluene (4HA26DNT), and two unknowns, M1 and M2. Based on the metabolite profiles, the TNT degradative pathway in soil seemed to undergo the sequential two-electron reduction mechanism mentioned above. Hydroxylamino- and amino-TNT derivatives have been detected in this study, but no nitroso-TNT derivative was identified. This observation is in good agreement with other studies reported, in which hydroxylamino- and amino-TNT derivatives were frequently observed as TNT metabolites, and no nitroso-TNT derivatives were detected (Koder and Miller, 1998; Esteve-Nunez et al., 2001; Yin et al., 2004; Nyanhongo et al., 2005; Yin et al., 2004).
Relatively higher concentrations of the identified TNT metabolites were observed during the first 7 days of the incubation, which corresponded to the rapid dissipation of TNT in early incubation (Figure 1). Overall, 2A46DNT and 4A26DNT appeared to be the major metabolites of TNT degradation in the soils. 4HA26DNT concentrations were very low in both soils. Compared with the control, the concentrations of TNT metabolites were significantly lower in the grass rhizosphere soils during the incubation period. This indicated that both native grasses enhanced not only TNT degradation but also the degradation of TNT metabolites in soil. Nevertheless, some TNT metabolites may be more toxic than the parent molecule; for example, nitroso and hydroxylamino groups were reported to be responsible for the mutagenicity and carcinogenicity of nitroaromatic compounds. Hydroxylamino-dinitrotoluene was reported to cause hemotoxic symptoms in humans exposed to TNT (Esteve-Nunez et al., 2001). Further reduction of the nitro group to an amino group may decrease the toxicity or mutagenicity of the compounds.
Conclusion
This growth chamber experiment showed that two selected EG and SW native grasses significantly enhanced the degradation of TNT in species-specific rhizosphere soil. SW appeared to be more effective in degrading TNT than EG. More than 95% of the TNT applied was degraded or transformed to other metabolic derivatives in the soil during the incubation period. The degradation rate was initially fast at the first 7 days of incubation and then remained low and steady afterward. The biodegradation was identified as a second-order reaction. Approximately 10% of the TNT applied was completely mineralized or transformed into harmless CO2. There was approximately 6%–7% non-acetonitrile-extractable TNT or TNT residue that may form relatively stable metabolic derivatives or complexes with soil constituents and limit further TNT degradation in soil. Six major TNT degradative metabolites were identified in the degradative pathway. This study demonstrated that the native grasses have the potential to mitigate the adverse human and environmental risks of TNT-contaminated sites through enhanced biodegradation and could be considered as a cost-effective, environmental-sound remediation approach for safeguarding human health and ecosystems from TNT contamination.
Data availability statement
The original contributions presented in the study are included in the article/supplementary material; further inquiries can be directed to the corresponding author.
Author contributions
NL: writing–original draft, writing–review and editing, formal analysis, software, and validation. KY: data curation, writing–original draft, formal analysis, and methodology. CL: data curation, methodology, and writing–review and editing. JY: funding acquisition, project administration, supervision, writing–review and editing, methodology, and writing–original draft.
Funding
The author(s) declare that financial support was received for the research, authorship, and/or publication of this article. This research was funded by the USEPA-NCE-STAR and the USDA-NIFA through grants to Lincoln University of Missouri (EPA no. RD83107101; NIFA no. 0206403).
Conflict of interest
The authors declare that the research was conducted in the absence of any commercial or financial relationships that could be construed as a potential conflict of interest.
Publisher’s note
All claims expressed in this article are solely those of the authors and do not necessarily represent those of their affiliated organizations, or those of the publisher, the editors, and the reviewers. Any product that may be evaluated in this article, or claim that may be made by its manufacturer, is not guaranteed or endorsed by the publisher.
References
Agency for Toxic Substances and Disease Registry, (1995). Toxicological profile for TNT. Atlanta, GA: Dpartment of Health and Human Services.
Anderson, T., Guthrie, E., and Walton, B. T. (1993). Bioremediation in the rhizosphere: plant roots and associated microbes clean contaminated soil. Environ. Sci. Technol. 27, 2630–2636. doi:10.1021/es00049a001
Caballero, A., Esteve-Nunez, A., Zylstra, G. J., and Ramos, J. L. (2005a). Assimilation of nitrogen from nitrite and trinitrotoluene in Pseudomonas putida JLR11. Bacteriol 187, 396–399. doi:10.1128/JB.187.1.396-399.2005
Caballero, A., Lazaro, J., Ramos, J. L., and Esteve-Núñez, A. (2005b). PnrA, a new nitroreductase-family enzyme in the TNT-degrading strain Pseudomonas putida JLR11. Environ. Microbiol. 7, 1211–1219. doi:10.1111/j.1462-2920.2005.00801.x
Caballero, A., and Ramos, J. (2006). A double mutant of Pseudomonas putida JLR11 deficient in the synthesis of the nitroreductas PnrA and assimilatory nitrite reductase NasB is impaired for growth on 2,4,6-trinitrotoluen (TNT). Environ. Microbiol. 8, 1306–1310. doi:10.1111/j.1462-2920.2006.01012.x
Chaudhry, Q., Schroeder, P., Werck-Reichhar, D., Grajek W Mulla, S., Talwar, M., Ninnekar, H., et al. (2001). Prospects and limitations of phytoremediation for the removal of persistent pesticides in the environment. Environ. Sci. Pollut. Res. 9, 4–17. doi:10.1065/espr2001.09.084.1
Esteve-Nunez, A., Caballero, A., and Ramos, J. L. (2001). Biological degradation of 2,4,6-Trinitrotoluene. Microbiol. Mol. Biol. Rev. 65, 335–352. doi:10.1128/mmbr.65.3.335-352.2001
George, S., Huggins-Clark, G., and Brooks, L. (2001). Use of a Salmonella microsuspension bioassay to detect the mutagenicity of munitions compounds at low concentrations. Mutat. Res. 490, 45–56. doi:10.1016/s1383-5718(00)00150-9
Gilbert, E., Kaye, S., and Herman, H. (1980) “TNT,” in Encyclopedia of explosives and related items. Dover NJ, US Army armament research and development commmand, 235–287.
Glick, B. R. (2003). Phytoremediation: synergistic use of plants and bacteria to clean up the environment. Biotechnol. Adv. 21, 383–393. doi:10.1016/s0734-9750(03)00055-7
Habineza, A., Zhai, J., Mai, T., Mmereki, D., and Ntakirutimana, T. (2016). Biodegradation of 2, 4, 6-trinitrotoluene (TNT) in contaminated soil and microbial remediation options for treatment. Period. Polytech. Chem. Eng. 61 (3), 171–187. doi:10.3311/ppch.9251
Haby, P., and Crowley, D. (1996). Biodegradation of 3-chlorobenzoate as affected by rhizodeposition and selected carbon substrates. Environ. Qual. 25, 304–310. doi:10.2134/jeq1996.00472425002500020014x
Haidour, A., and Ramos, J. (1996). Identification of products resulting from biological reduction of 2,4,6-trinitrotoluene, 2,4-dinitrotoluene and 2,6-dinitrotoluene by Psedudomonas sp. Environ. Sci. Technol. 30, 2365–2370. doi:10.1021/es950824u
Harter, D., and Ricket, D. (1985) “The use and importance of nitroaromatic chemicals in the chemical industry,” in Toxicity of nitroaromatic chemical industry. New York: Hemisphere Publishing, 1–14.
Hawari, J., Beaudet, S., Halasz, A., Thiboutot, S., and Ampleman, G. (2000). Microbial degradation of explosives: biotransformation versus mineralization. Appl. Microbiol. Biotechnol. 54, 605–618. doi:10.1007/s002530000445
Honeycutt, M. E., Jarvis, A. S., and McFarland, V. A. (1996). Cytotoxicity and mutagenicity of 2,4,6-trinitrotoluene and its metabolites. Ecotoxicol. Environ. Saf. 35, 282–287. doi:10.1006/eesa.1996.0112
Hughes, J., Wang, C., Yesland, K., Richardson, A., Bhadra, R., Bennett, G., et al. (1998). Bamberger rearrangement during TNT metabolisn by Clostridium acetobutylicum. Environ. Sci. Technol. 32, 494–500. doi:10.1021/es970612s
Jenkins, T., Leggett, D., Grant, C. L., and Bauer, C. F. (1986). Reversed-phase high-performance liquid chromatographic determination of nitroorganics in munitions wastewater. Anal. Chem. 58, 170–175. doi:10.1021/ac00292a042
Johnson, G., Smets, B., and Spain, J. C. (2001). Oxidative transformation of aminodinitrotoluene isomers by multicomponent dioxygenases. Appl. Environ. Microbiol. 67, 5460–5466. doi:10.1128/aem.67.12.5460-5466.2001
Kao, C., Lin, B., Chen, S. C., Wei, S. F., Chen, C. C., Yao, C. L., et al. (2016). Biodegradation of trinitrotoluene (TNT) by indigenous microorganisms from TNT-contaminated soil, and their application in TNT bioremediation. Bioremediation 20 (3), 165–173. doi:10.1080/10889868.2016.1148007
Koder, R., and Miller, A. (1998). Steady-state kinetic mechanism, stereospecificity, substrate and inhibitor specificity of Enterobacter cloacae nitroreductase. Biochim. Biophys. Acta 1387, 395–405. doi:10.1016/s0167-4838(98)00151-4
Lewis, T. A., Newcombe, D. A., and Crawford, R. L. (2004). Bioremediation of soils contaminated with explosives. Environ. Manag. 70, 291–307. doi:10.1016/j.jenvman.2003.12.005
Lindner, V. (1980) “Explosives and propellants,” in Kirk-Othmer encyclopedia of chemical technology. NY: Wiley, 561–620.
McConnell, W., and Flinn, R. (1946). Summary of twenty-two trinitrotoluene fatalities in World War II. Ind. Hyg. Tox 28, 76–86.
Mulla, S., Bharagava, R., Belhaj, D., Saratale, G. D., Bagewadi, Z. K., Saxena, G., et al. (2019). “An overview of nitro group-containing compounds and herbicides degradation in microorganisms,” in Metabolism of xenobiotic compounds. Microorganisms for sustainability. Editor P. Arora (Singapore: Springer), 10, 319–335. doi:10.1007/978-981-13-7462-3_16
Mulla, S., Talwar, M., et al. (2013). “Biodegradation of 2, 4, 6-trinitrotoluene explosive residues,” in Biological remediation of explosive residues. Environmental science and engineering. Editor S. Singh (Cham: Springer), 201–233.
Nyanhongo, G. S., Schroeder, M., Steiner, W., and Gübitz, G. M. (2005). Biodegradation of 2,4,6-trinitrotoluene (TNT): an enzymatic perspective. Biocatal. Biotransformation 23 (2), 53–69. doi:10.1080/10242420500090169
Pennington, J. C., and Brannon, J. M. (2002). Environmental fate of explosives. Thermochim. Acta 384, 163–172. doi:10.1016/s0040-6031(01)00801-2
Reilley, K., Banks, M., and Schwab, A. P. (1996). Dissipation of polycyclic aromatic hydrocarbons in the rhizosphere. Environ. Qual. 25, 212–219. doi:10.2134/jeq1996.00472425002500020002x
Schwitzguebel, J., Auberrt, S., et al. (2001). Sulphonated aromatic pollutants: limits of microbial degradability and potential of phytoremediation. Environ. Sci. Pollut. Res. 9, 62–72. doi:10.1065/espr2001.09.084.4
Siciliano, S., and Greer, C. (2000). Plant-bacterial combinations to phytoremediate soil contaminated with high concentrations of 2,4,6-trinitrotoluene. Environ. Qual. 29, 311–316. doi:10.2134/jeq2000.00472425002900010039x
Smets, B. F., Yin, H., and Esteve-Nuñez, A. (2007). TNT biotransformation: when chemistry confronts mineralization. Appl. Microbiol. Biotechnol. 76, 267–277. doi:10.1007/s00253-007-1008-7
Spain, J. (1995). Biodegradation of nitroaromatic compounds. Annu. Rev. Microbiol. 49, 523–555. doi:10.1146/annurev.mi.49.100195.002515
Steevens, J. A., Duke, B. M., Lotufo, G. R., and Bridges, T. S. (2002). Toxicity of the explosives 2,4,6-trinitrotoluene, hexahydro-1,3,5-trinitro-1,3,5-triazine, and octahydro-1,3,5,7-tetranitro-1,3,5,7- tetrazocine in sediments to Chironomus tentans and Hyalella azteca: low-dose hormesis and high-dose mortality. Environ. Toxicol. Chem. 20 (7), 1475–1482. doi:10.1897/1551-5028(2002)021<1475:toteth>2.0.co;2
Su, K., Wu, Z., Liu, Y., Wang, Y., Wang, H., Liu, M., et al. (2024). UDP-glycosyltransferase UGT96C10 functions as a novel detoxification factor for conjugating the activated dinitrotoluene sulfonate in switchgrass. Plant Biotechnol. 22, 2530–2540. doi:10.1111/pbi.14366
United States Environmental Protection Agency (USEPA) (2014). Washington, DC: USEPA. Technical Fact Sheets. 2,4,6-Trinitrotoluene (TNT).
Via, S., and Zinnert, J. (2015). Impacts of explosive compounds on vegetation: a need for community scale investigations. Environ. Pollut. 208, 495–505. doi:10.1016/j.envpol.2015.10.020
Wang, H., Su, K., et al. (2024). Overexpressing CYP81D11 enhances 2,4,6-trinitrotoluene tolerance and removal efficiency in Arabidopsis. Physiol. Plantarium. doi:10.1111/pbl.14364
Wang, Z., Ye, Z., Zhang, M., and Bai, X. (2010). Degradation of 2,4,6-trinitrotoluene (TNT) by immobilized microorganism-biological filter. Process Biochem. 45, 993–1001. doi:10.1016/j.procbio.2010.03.006
Yin, H., Wood, T., and Smets, B. F. (2004). Reductive transformation of TNT by Escherichia coli: pathway description. Appl. Microbiol. Biotechnol. 67, 397–404. doi:10.1007/s00253-004-1736-x
Yin, H., Wood, T., and Smets, B. F. (2005). Reductive transformation of TNT by Escherichia coli resting cells: kinetic analysis. Appl. Microbiol. Biotechnol. 69, 326–334. doi:10.1007/s00253-005-1988-0
Keywords: TNT, biodegradation, native grass, rhizosphere soil, remediation
Citation: Li N, Yang K, Lin C and Yang J (2024) Enhanced biodegradation of trinitrotoluene in rhizosphere soil by native grasses. Front. Environ. Sci. 12:1426203. doi: 10.3389/fenvs.2024.1426203
Received: 30 April 2024; Accepted: 03 September 2024;
Published: 24 September 2024.
Edited by:
Leonardo Fernandes Fraceto, São Paulo State University, BrazilReviewed by:
Sikandar I. Mulla, REVA University, IndiaYanju Liu, The University of Newcastle, Australia
Copyright © 2024 Li, Yang, Lin and Yang. This is an open-access article distributed under the terms of the Creative Commons Attribution License (CC BY). The use, distribution or reproduction in other forums is permitted, provided the original author(s) and the copyright owner(s) are credited and that the original publication in this journal is cited, in accordance with accepted academic practice. No use, distribution or reproduction is permitted which does not comply with these terms.
*Correspondence: John Yang, yangj@lincolnu.edu