- 1Division of Applied Life Science (BK21+ Program), Gyeongsang National University, Jinju, Republic of Korea
- 2Department of Soil Science, College of Agriculture, Central Mindanao University, Maramag, Philippines
- 3Soil and Fertilizer Management Division, National Institute of Agricultural Sciences, Rural Development Administration, Wanju, Republic of Korea
- 4Institute of Agriculture and Life Sciences, Gyeongsang National University, Jinju, Republic of Korea
Manure application is a recommended method to improve soil quality and mitigate global warming via soil carbon (C) sequestration. However, such application can significantly increase ammonia (NH3) volatilization loss and cause secondary environmental problems, such as acidification, eutrophication, and particulate matter formation. To investigate the potential of reducing NH3 emissions in flooded rice paddies, three types of stabilized swine manure amendments (fresh, composted, and biochar) were applied at a rate of 12 Mg ha−1 (dry weight) under standard fertilization (N–P2O5–K2O = 90–45–57 kg ha−1), and NH3 emission was characterized using the static chamber method. Regardless of manure management practices, NH3 fluxes increased significantly immediately after application of an inorganic nitrogen (N) fertilizer (urea). The manure was applied completely as the basal fertilizer before rice transplanting, but the NH3 emission rates increased more dramatically following urea application in the manure treatments. Fresh and composted manure applications significantly increased seasonal NH3 volatilization losses compared to the control but the biochar did not. Compost manure significantly increased rice grain productivity owing to the higher N content, while fresh and biochar manures did not increase rice productivity because of fewer panicles per hill and fewer grains per panicle. Consequently, biochar application resulted in lower NH3 flux intensity and seasonal NH3 flux per grain yield, whereas fresh and compost manures substantially increased this intensity. Therefore, biochar manure may be a more reasonable organic amendment than fresh and composted manures for reducing NH3 emission impacts on rice paddies.
1 Introduction
Livestock manure is considered to be a practical organic amendment for enhancing soil productivity as substitutes to chemical fertilizers in croplands (Du et al., 2020; Hua et al., 2020). However, improper application of livestock manure can create various environmental problems. Manure-derived nitrogen (N) can be lost through leaching, runoff, volatilization, denitrification, and other processes. Significant proportions of N are often lost via ammonia (NH3) emissions from manure-amended soils. For instance, an average of 12.8% and 17.9% of the applied N was reported to have volatilized as NH3 gas in Chinese upland and paddy fields, respectively (Zhang et al., 2013). The emitted NH3 can lead to increased N deposition and contribute to soil acidification, water eutrophication, and particulate matter (PM) formation (Wu et al., 2016; Ehrnsperger and Klemm, 2021).
Primary PM comprise mixed liquid droplets and solid particles in the air (e.g., soot, dust, smoke). However, secondary PM₂.₅ having diameters less than 2.5 μm may be formed through reactions of NH3 with other gaseous acidic precursors (e.g., NOx, SOx). NH3 is a reactive gas that readily combines with sulfate (SO₄2⁻) and nitrate (NO₃⁻) components in acidic cloud droplets to form particulates (Asman et al., 1998). When PM₂.₅ is inhaled, it penetrates deep into the lungs to impair respiration by irritating and corroding the alveolar walls (Xing et al., 2016). Globally, over 4.2 million premature deaths and 103 million disability-adjusted life years have been attributed to PM₂.₅ pollution (Cohen et al., 2017).
Global N losses via NH3 emissions are estimated to be 51–54 Tg N year⁻1 (Bouwman et al., 1997). Approximately 10%–20% of these emissions originate from natural sources, while the remaining 80%–90% are from anthropogenic sources (Zhang et al., 2018). Among the anthropogenic activities, agriculture contributes to about 80%–90% of NH3 emissions, with 63% from livestock feeding and 37% from crop cultivation (Bouwman et al., 1997; Piwowar, 2020). In soils, NH₃ emission intensities are influenced by several factors, such as N fertilization level and soil conditions (e.g., temperature, moisture content, pH) (Kang et al., 2016; Klimczyk et al., 2021). Compared to upland soils, flooded rice paddies may provide more favorable conditions for higher NH3 emissions. Applied N in soils can mineralize into ammonium (NH₄⁺) and then be aerobically nitrified into nitrate (NO₃⁻) (Sah and Mikkelsen, 1983). However, the nitrification rate is strongly regulated by oxygen availability (Jechalke et al., 2011; Yang et al., 2016), which generally results in the prolonged presence of inorganic NH₄⁺ in flooded rice paddies and increased volatilization into NH3 gas (Buresh et al., 2008).
Hypothetically, stabilized organic amendments (e.g., biochar, compost) may be more effective at reducing NH3 emissions than fresh manures. In compost, inorganic N is predominantly available in the form of NO₃⁻, which does not revert to NH₄⁺. Stabilized organic matter can slow mineralization and thereby decrease NH3 emissions (Lü et al., 2013; Agyarko-Mintah et al., 2017). Similarly, biochar contains less inorganic N owing to the expulsion of labile compounds during the pyrolysis process (Bruun et al., 2011; Buss et al., 2022). The organic C and inorganic N in biochar are hence more stable and resistant to decomposition (Haider et al., 2020). However, there are no readily available reports on the potentials of these stabilized organic amendments to reduce NH3 emissions in flooded rice paddy soils.
To investigate the feasibility of stabilized manure amendments in reducing NH3 emissions in rice paddies, three types of manures (fresh, composted, and biochar) were selected as the main treatments. The NH3 emission losses and rice yield properties were characterized during rice cultivation to identify the organic amendment with the lowest NH3 flux intensity.
2 Materials and methods
2.1 Preparation of manure amendments
To evaluate the impacts of manure amendments on NH₃ emissions during rice cultivation, three types of swine manures were prepared: fresh, compost, and biochar. Fresh swine manure was collected from a medium-scale pig farm in Jinju, South Korea; this manure was combined with sawdust in equal proportions (50% kg kg⁻1) and air-dried inside a greenhouse. The air-dried manure mixture had a neutral pH (6.7 with H₂O) and contained 44.8% total C and 1.8% total N (Table 1).
To prepare the compost, 1 Mg of the manure mixture was moistened to 60% (wt wt⁻1) and placed in a 1.2 m³ (1 m (width) × 1 m (length) × 1.2 m (height)) box covered with Styrofoam to retain heat. The manure pile was periodically mixed for aeration and adequately moistened during the 45-day composting period; the pile temperature increased sharply to 65–75°C immediately after piling and was maintained for approximately 3 weeks before gradually declining to the background temperature. The compost had an alkaline pH (7.4 with H₂O) and contained 43.4% total C and 2.2% total N.
To prepare the biochar, the dried manure mixture was pyrolyzed using a muffle furnace under closed conditions at 400°C for 4 h; the mixture was then placed inside the furnace and gradually heated at a rate of 10°C per minute before being stabilized at 400°C. The biochar had an alkaline pH (8.2 with H₂O) and contained 42.2% total C and 1.2% total N.
2.2 Experimental plot preparation for rice cultivation
A typical mono-rice paddy field was selected at the Gyeongsang National University experimental station (latitude 35° 8′56.73″ N, longitude 128° 5′46.27″ E) in the Republic of Korea. The paddy field has been exclusively used for rice farming for over 50 years, and the soil is classified as belonging to the Jisan series (fine silty, mixed, non-acid mesic Typic Endoaquepts). Before the experiments, the soil had a slightly acidic pH (6.0 in H₂O) and low fertility, with total C and N contents of 18.9 and 1.4 g kg⁻1, respectively.
A total of four treatments (control, fresh, compost, and biochar) were assigned with three replications each. The experimental plots (10 m × 10 m in size) were arranged in a randomized complete block design (RCBD). Irrigation inlets and outlets were prepared in each plot to control the flooded water levels; a metal barrier was deeply installed around each plot to avoid lateral water flow and fertilizer treatment mixing. In South Korea, the general recommendation for organic amendment application is 12 Mg ha⁻1 (RDA, 2017). One day before transplanting in the manure treatment plots, 12 Mg ha⁻1 of each of the manure amendments (on a dry weight basis) were manually mixed into the surface layers of the corresponding plots. The recommended levels of chemical fertilizers for rice cultivation (N–P₂O₅–K₂O = 90–45–57 kg ha⁻1) were applied to all the treatment plots (RDA, 2017). Based on the recommended rates, 50% of N, 100% of P₂O₅, and 70% of K₂O were applied as the basal fertilizers. The remaining fertilizers were side dressed on the 15th day (20% of N) and 45th day (30% of N and K₂O) after transplanting.
Twenty-five-day-old Dongjinbyeo cultivar (Japonica) rice seedlings were used in this study. Three to five seedling plants were manually transplanted per hill, with distances of 15 cm each between the hills and 30 cm each between the rows. The irrigated water in each plot was maintained at 5–7 cm above the surface soil layer. The floodwater was drained 4 weeks before rice harvesting. At the harvesting stage, 10 representative rice plants were collected from each plot. The plant samples were air-dried for a week in the greenhouse, and the seeds were detached from the panicles before counting. Finally, the harvest index was determined as the ratio of grain yield to total aboveground biomass.
2.3 Evaluation of ammonia volatilization loss
To assess the influences of manure amendment applications on NH₃ emissions during rice cultivation, the static chamber method was used. The chamber (inner diameter: 12 cm; height: 25 cm) was inserted into the soil to a depth of 15 cm without the rice plants. Two acid-impregnated sponges (diameter: 12 cm; height: 2 cm) were placed inside the chamber to trap the NH₃ gas from the soil and outside air separately. Phosphoric acid was used to trap the NH₃ gas, and 4% glycerin was used to maintain the moisture in the sponge. NH₃ samples were collected daily for 1 week after N fertilization, followed by collection at 2- to 3-day intervals for 2 weeks, and finally once per week during the rest of the rice cultivation period.
During sampling, the lower sponges were collected and placed in an icebox for preservation. They were then completely immersed in a 2 M KCl solution and shaken using a rotating shaker for 1 h. Subsequently, the sponges were squeezed by hand, and the NH₄⁺–N concentration was quantified using the indophenol blue method (Novamsky et al., 1974). The NH₃ emission rate was estimated from the amount of NH₃ trapped per unit area over a specific sampling interval:
where M (mg) is the NH₄⁺–N collected in the chamber, A (m2) is the chamber area, and D (day) is the duration for NH₃ sampling.
The seasonal NH₃ flux was calculated using the daily NH₃ emission rate and sampling interval:
where Ri is the daily NH3 emission rate (kg N ha−1 day−1) in the ith sampling interval, Di is the number of days between the ith and (i−1)th sampling, and n is the number of samplings.
2.4 Analysis of manure amendment, soil, and water characteristics
The manure amendments were sampled and their chemical properties analyzed for pH and electrical conductivity (EC) (1:10 wt v−1, sample: H2O); total C and N contents using an automated true macro element analyzer; and inorganic NH₄⁺ and NO₃⁻ contents using 2 M KCl extraction and measurement with the indophenol blue and brucine methods, respectively. During rice cultivation, the soil temperature and redox potential (Eh) changes were continually recorded using an automatic sensor at a soil depth of 15–20 cm. The floodwater and surface soil were sampled periodically to monitor changes in pH and NH₄⁺ concentration following the same analysis procedures as noted above.
2.5 Statistical analysis
The Statistical Tool for Agricultural Research 2.0.1 (STAR) software was used for the statistical analyses. The data were analyzed using one-way analysis of variance (ANOVA) for the RCBD; the statistically significant results were subjected to comparison of means using the honestly significant difference (HSD) test at the 5% significance level.
3 Results
3.1 Changes in soil temperature and Eh values
During rice cultivation, the soil temperature and Eh values were continuously monitored at the surface layer (Figure 1). The soil temperature fluctuated similarly to the air temperature but showed no significant differences among the treatments or years; the temperature increased gradually after rice transplantation, reached a peak value during the reproductive stage, and declined gradually thereafter. The soil Eh values exhibited a typical pattern throughout the entire rice cultivation period, with no marked differences among the various manure treatments. Within 2 weeks of submerging for rice transplanting, the Eh value declined sharply to –200 mV, indicating a highly reduced soil environment that persisted throughout the flooded cropping period. Following water drainage 1 month before rice harvesting, the soil Eh value increased rapidly, but no significant differences were observed among the different fertilizer treatments.
3.2 Changes in NH3 emissions
Irrespective of the manure types and investigation years, NH₃ gas was emitted in a similar pattern (Figure 2). The recommended levels of chemical fertilizers were applied in all the treatments; the NH₃ emission rates increased sharply immediately after the addition of N fertilizer (urea). The total urea was split into three applications as the basal (50%) fertilizer 1 day before rice transplantation, at tillering (20%) 15 days after transplantation, and at panicle initiation (30%) 45 days after transplantation. However, manure addition significantly increased the NH₃ emission rates.
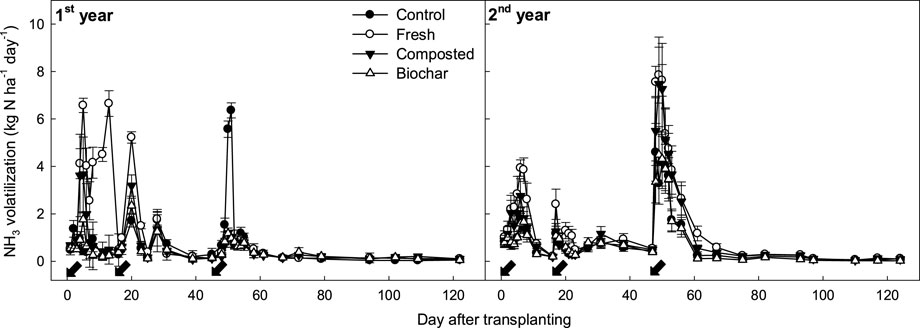
Figure 2. Changes in NH3 emission rates of rice paddy supplied with different types of manure amendments. The arrows indicate split N fertilization days, while the vertical bars indicate standard deviations (n = 3).
In the no-manure treatment (control), a total of 70–97 kg N ha⁻1 of NH₃ gas was emitted over the entire rice cultivation period, but the addition of 12 Mg ha⁻1 (dry weight) of fresh manure largely increased this seasonal flux by 42%–80% over the control (Figure 3). Stable manure types, such as the biochar and compost, were effective at reducing the increased NH₃ fluxes. Compost manure reduced the seasonal NH₃ flux by approximately 16%–21% compared to fresh manure. However, biochar exhibited even greater efficacy, reducing the seasonal NH₃ flux by 36%–46% compared to fresh manure. Furthermore, biochar showed a 4%–9% lower seasonal NH₃ flux than the control, although no statistically significant difference was observed between the two treatments.
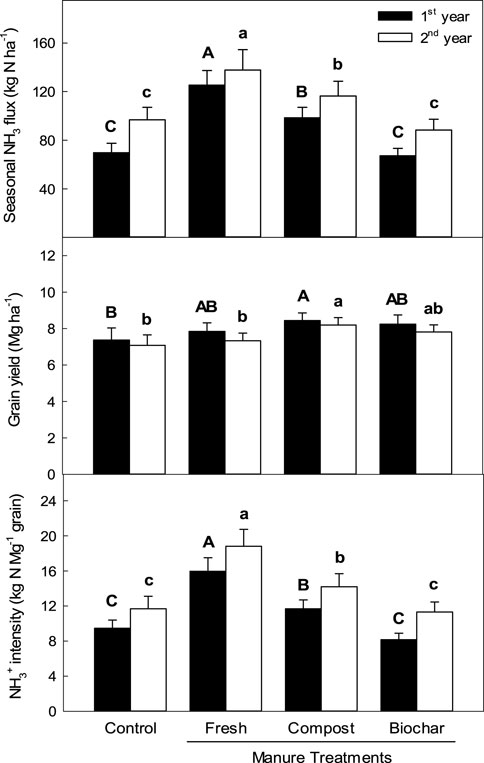
Figure 3. Seasonal NH3 fluxes, grain yields, and NH3 flux intensities during rice cultivation when supplied with different types of manure amendments. The vertical bars indicate standard deviations (n = 3).
3.3 Changes in floodwater and soil chemical properties
The floodwater pH and NH₄⁺ concentrations fluctuated similarly during the 2 years of rice cultivation (Figure 4). Regardless of manure application, these two properties increased sharply after urea addition and then decreased gradually. Manure treatments had more pronounced influences on these properties. Fresh manure application significantly increased the water pH and NH₄⁺ concentration during the early rice cropping season, but these changes were less evident in the late cropping season.
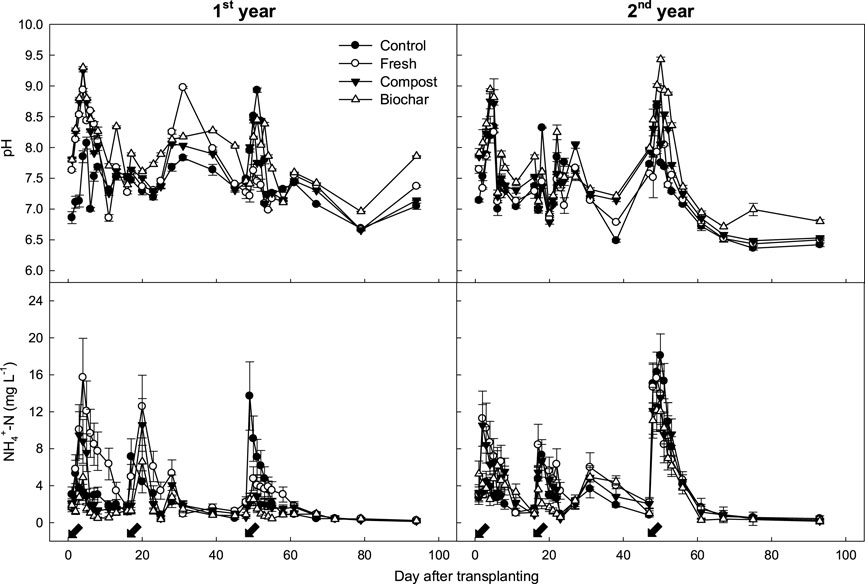
Figure 4. Changes in the pH and NH4+ concentrations in rice paddy floodwaters supplied with different types of manure amendments. The arrows indicate split N fertilization days, and the vertical bars indicate standard deviations (n = 3).
In contrast to the floodwater changes, the soil properties (pH and inorganic NH₄⁺ concentration) remained relatively stable during rice cultivation (Figure 5). The soil pH was initially low when the soil Eh value was higher; as the soil condition became highly reduced, the soil pH increased. Manure application enhanced soil pH during the early rice-growing season, with biochar proving more effective than fresh and compost manures in this regard. Regardless of the fertilization background, the soil NH₄⁺ concentration increased slightly over time. Manure application comparably increased the inorganic NH₄⁺ contents in the soils. Among the manure treatments, biochar application significantly increased the soil NH₄⁺ level throughout the cropping period, although the differences were comparable among the various manure treatments.
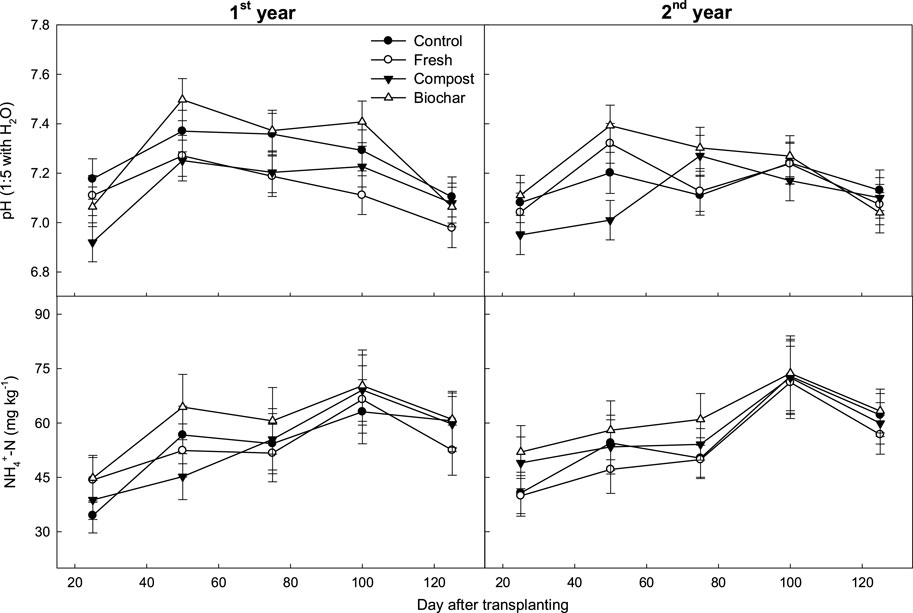
Figure 5. Changes in the pH and NH4+ concentrations in rice paddy soils supplied with different types of manure amendments. The vertical bars indicate standard deviations (n = 3).
3.4 Rice productivity and ammonia flux intensity
The rice yield properties differed between the cropping years, but the organic amendment application increased rice productivity consistently (Figure 3). The average grain yield was 7.2 Mg ha⁻1 for the control treatment. Compost application significantly increased grain productivity owing to the higher panicle numbers per hill and number of seeds per panicle, whereas the fresh and biochar manure applications increased rice productivity slightly without any statistically significant differences (Table 2). The NH₃ flux intensity, indicating the seasonal NH₃ flux per grain yield, was approximately 9.5 kg NH₃–N Mg⁻1 grain for the control treatment (Figure 3). The fresh and compost manure applications significantly increased the average NH₃ flux intensities by 69% and 38% over the control, respectively; however, biochar manure application did not increase the flus intensity over that of the control.
4 Discussion
The N losses via NH₃ emissions from the field-applied amendments have garnered significant attention, owing to their impacts on reduced fertilizer effectiveness and more environmental problems (Misselbrook and Powell, 2005; Monaco et al., 2012). Flooded rice paddies possess soil conditions that are particularly conducive to NH₃ volatilization compared to upland conditions owing to the reduced oxygen levels and higher pH during the flooded cropping season (Ventura and Yoshida, 1977; Zhao et al., 2009; Zhang et al., 2013). Urea-N applied to the soil is rapidly converted to NH₄⁺ ions, which can either volatilize into NH₃ gas or undergo biological nitrification to form NO₃⁻ ions (Saggar et al., 2013; Barth et al., 2019). However, the rate of nitrification is influenced by oxygen availability (Jechalke et al., 2011; Hsiao et al., 2014). In flooded rice paddies, NH₄⁺ ions can persist due to the low-oxygen environment, leading to higher NH₃ volatilization losses.
In the present study, soil Eh values indicating the oxidation and reduction states decreased rapidly from 150 mV to –260 mV immediately after flooding (Figure 1). This decrease signifies a significant reduction in the soil oxygen concentration after flooding, creating favorable conditions for NH₃ volatilization loss. The low-oxygen environment inhibits the activity of the nitrifying bacteria, which normally convert NH₄⁺ to NO₃⁻. Consequently, the NH₄⁺ ions accumulate in the soil and are more prone to volatilization as NH₃ gas (Cui et al., 2021). Furthermore, this soil reduction increases the soil pH to 7.5–9.0, which is another favorable condition for NH₃ emission that persists throughout the flooded period (Figure 4).
In the field study, similar levels of chemical fertilizers were applied to all treatments, and the N fertilizer (urea) was split into three application times (basal, tillering, and panicle initiation stages) during rice cultivation. Regardless of the manure management conditions, the NH₃ gas emissions fluctuated in similar patterns (Figure 2), sharply increasing within 2–3 days after urea application and then rapidly decreasing to the background levels. The results indicate that the NH₃ emission rates were highly correlated with NH₄⁺ concentrations in the floodwaters rather than in the soils (Figure 6). Unlike the general notion that NH₃ volatilization loss is influenced by inorganic NH₄⁺ content in the soil (Du Plessis and Kroontje, 1964), inorganic NH₄⁺–N concentration in the floodwater was found to be the most influential factor determining NH₃ flux in the rice paddy (Figure 6).
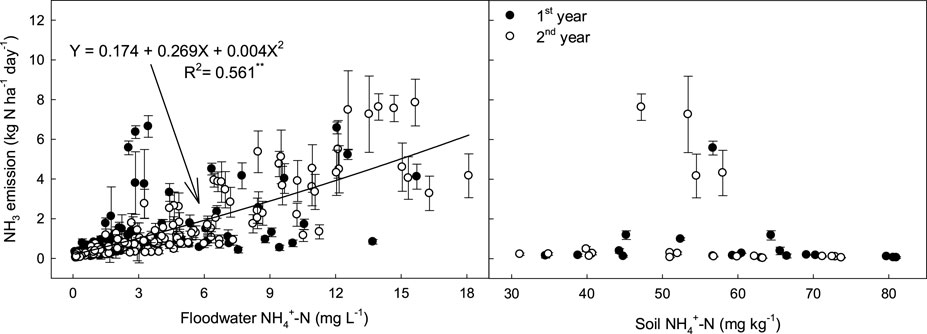
Figure 6. Relationship between NH3 emission rates and NH4+ concentrations in floodwaters and soils supplied with different types of manure amendments. The vertical bars indicate standard deviations (n = 3).
Interestingly, while the entire manure amendment was incorporated into the surface soil as the basal fertilizer before transplanting, the addition of urea through side-dressing significantly increased NH₃ volatilization loss in the manure treatments. This suggests that the added urea may have stimulated microbial activity (Xiang et al., 2023). Under favorable conditions, the soil microorganisms can metabolize urea and organic matter more rapidly, leading to increased NH₃ production as a metabolic byproduct (Lee et al., 2021; Li et al., 2022).
For example, the same amounts of urea N were applied in all treatments at the tillering stage (15 days after transplanting), but this addition increased NH₃ emission rates from tillering to panicle initiation fertilization by an average of 60% and 12% in the fresh and compost treatments, respectively. However, under biochar manure treatment, the NH₃ emissions were comparable to those without manure (Figure 2). The applied urea-N might boost microbial activity in the soil, accelerate the decomposition of organic amendments and soil organic matter, and subsequently increase NH₃ volatilization under anaerobic and high-pH conditions (He and Suzuki, 2004; Wang et al., 2021).
Among the selected manure amendments, the fresh and compost applications significantly increased the average seasonal NH₃ fluxes by 58% and 29% over no manure application, respectively (Figure 3). Biochar had an alkaline pH (8.4 ± 0.2 in water suspension) (Table 1), but its application did not increase NH₃ volatilization loss during the cropping season; instead, it decreased the seasonal NH₃ flux by approximately 7% compared to no manure application. Biochar is a C-rich solid product produced by the low-temperature pyrolysis of biomass (Lehmann and Joseph, 2015; Tomczyk et al., 2020); it contains oxygen functional groups, such as carboxyl and keto groups, which are responsible for NH₄⁺ adsorption through hydrogen bonding and electrostatic interactions (Cai et al., 2016). The reduction in NH₃ emission loss may be related to biochar’s high negativity (Gundale and DeLuca, 2007) and low inorganic N content (Table 1). Labile N in the biomass could volatilize during pyrolysis, resulting in biochar having very low inorganic N content (Ohtsuka et al., 1994; Clark et al., 2017). Some studies have shown the effectiveness of biochar in reducing N leaching losses (Major et al., 2012), with a high C/N ratio enhancing N immobilization (DeLuca et al., 2015).
However, compost contains higher concentrations of inorganic N (NH₄⁺ and NO₃⁻) compared to fresh manure (see Table 1), which makes it a less-effective application for reducing NH₃ volatilization losses. Rice grain productivity significantly increased with compost manure application (Figure 3), likely due to the higher N content (Table 1). However, fresh and biochar applications increased the average rice productivities by 5% and 11% over the control, respectively, although this was not a statistically significant difference compared to the control. The NH₃ intensity, indicating seasonal NH₃ flux per grain yield, was 12 kg NH₃–N Mg⁻1 grain for the control treatment. Fresh and compost manure applications significantly increase this intensity by approximately 50% and 12% over the control, respectively (Figure 3), primarily due to the higher NH₃ emissions. However, biochar application decreased NH₃ intensity during rice cultivation by around 16% compared to that of the control.
5 Conclusion
Regardless of the organic amendment management condition, NH₃ gas was mainly volatilized immediately after application of the chemical N fertilizer (urea). However, manure-based amendments, when incorporated as basal fertilizers before rice transplantation, can significantly increase NH₃ emission rates following urea application. Both fresh and compost manures, which are characterized by high contents of inorganic N, notably increase NH₃ volatilization during rice cultivation. In contrast, biochar having high material pH but low inorganic N content, does not elevate NH₃ emission loss over that of the control. Notably, NH₃ emission rates are primarily governed by the NH₄⁺ contents in the flooded waters, rather than those in the soils. Although compost manure application significantly boosts rice grain yield, fresh and biochar manure applications result in slight productivity increments without statistical significance. Consequently, the application of fresh and compost manures can markedly increase NH₃ emission intensities, indicating the seasonal NH₃ flux per grain yield, whereas biochar manure does not exhibit such intensity. In summary, biochar as an organic amendment is a more viable option compared to fresh and compost manures for mitigating NH₃ emissions and enhancing soil productivity in rice paddies.
Data availability statement
The original contributions presented in the study are included in the article/Supplementary Material; further inquiries can be directed to the corresponding author.
Author contributions
RC: formal analysis, investigation, writing–original draft, and writing–review and editing. SC: methodology and writing–review and editing. SJG: formal analysis and writing–review and editing. SP: investigation and writing–review and editing. PK: conceptualization, writing–original draft, and writing–review and editing.
Funding
The author(s) declare that financial support was received for the research, authorship, and/or publication of this article. This work was funded by the Basic Science Research Program through the National Research Foundation of Korea funded by the Ministry of Education (No. NRF-2023R1A2C3004842). Authors RC, SG, and SP were supported by scholarships from the BK21 Four Program of the Ministry of Education and Human Resources Development, Republic of Korea.
Acknowledgments
The authors gratefully acknowledge Gyeongsang National University for granting the necessary permissions and providing the facilities to carry out this work.
Conflict of interest
The authors declare that the research was conducted in the absence of any commercial or financial relationships that could be construed as a potential conflict of interest.
Publisher’s note
All claims expressed in this article are solely those of the authors and do not necessarily represent those of their affiliated organizations, or those of the publisher, the editors, and the reviewers. Any product that may be evaluated in this article, or claim that may be made by its manufacturer, is not guaranteed or endorsed by the publisher.
References
Agyarko-Mintah, E., Cowie, A., Van Zwieten, L., Singh, B. P., Smillie, R., Harden, S., et al. (2017). Biochar lowers ammonia emission and improves nitrogen retention in poultry litter composting. Waste Manag. 61, 129–137. doi:10.1016/j.wasman.2016.12.009
Asman, W. A., Sutton, M. A., and Schjørring, J. K. (1998). Ammonia: emission, atmospheric transport and deposition. New phytol. 139 (1), 27–48. doi:10.1046/j.1469-8137.1998.00180.x
Barth, G., Otto, R., Almeida, R. F., Cardoso, E. J. B. N., Cantarella, H., and Vitti, G. C. (2019). Conversion of ammonium to nitrate and abundance of ammonium-oxidizing-microorganism in Tropical soils with nitrification inhibitor. Sci. Agric. 77. doi:10.1590/1678-992x-2018-0370
Bouwman, A. F., Lee, D. S., Asman, W. A., Dentener, F. J., Van Der Hoek, K. W., and Olivier, J. G. J. (1997). A global high-resolution emission inventory for ammonia. Glob. Biogeochem. cycles 11 (4), 561–587. doi:10.1029/97gb02266
Bruun, E. W., Müller-Stöver, D., Ambus, P., and Hauggaard-Nielsen, H. (2011). Application of biochar to soil and N2O emissions: potential effects of blending fast-pyrolysis biochar with anaerobically digested slurry. Eur. J. Soil Sci. 62 (4), 581–589. doi:10.1111/j.1365-2389.2011.01377.x
Buresh, R. J., Ramesh Reddy, K., and Van Kessel, C. (2008). Nitrogen transformations in submerged soils. Nitrogen Agric. Syst. 49, 401–436. doi:10.2134/agronmonogr49.c11
Buss, W., Wurzer, C., Manning, D. A., Rohling, E. J., Borevitz, J., and Mašek, O. (2022). Mineral-enriched biochar delivers enhanced nutrient recovery and carbon dioxide removal. Commun. Earth Environ. 3 (1), 67–11. doi:10.1038/s43247-022-00394-w
Cai, Y., Qi, H., Liu, Y., and He, X. (2016). Sorption/desorption behavior and mechanism of NH4+ by biochar as a nitrogen fertilizer sustained-release material. J. Agric. food Chem. 64 (24), 4958–4964. doi:10.1021/acs.jafc.6b00109
Clark, S. C., Ryals, R., Miller, D. J., Mullen, C. A., Pan, D., Zondlo, M. A., et al. (2017). Effluent gas flux characterization during pyrolysis of chicken manure. ACS Sustain. Chem. Eng. 5 (9), 7568–7575. doi:10.1021/acssuschemeng.7b00815
Cohen, A. J., Brauer, M., Burnett, R., Anderson, H. R., Frostad, J., Estep, K., et al. (2017). Estimates and 25-year trends of the global burden of disease attributable to ambient air pollution: an analysis of data from the Global Burden of Diseases Study 2015. Lancet 389 (10082), 1907–1918. doi:10.1016/s0140-6736(17)30505-6
Cui, L., Li, D., Wu, Z., Xue, Y., Xiao, F., Zhang, L., et al. (2021). Effects of nitrification inhibitors on soil nitrification and ammonia volatilization in three soils with different pH. Agronomy 11 (8), 1674. doi:10.3390/agronomy11081674
DeLuca, T. H., Gundale, M. J., MacKenzie, M. D., and Jones, D. L. (2015). Biochar effects on soil nutrient transformations. Biochar Environ. Manag. Sci. Technol. Implement. 2, 421–454.
Du, Y., Cui, B., Wang, Z., Sun, J., and Niu, W. (2020). Effects of manure fertilizer on crop yield and soil properties in China: a meta-analysis. Catena 193, 104617. doi:10.1016/j.catena.2020.104617
Du Plessis, M. C. F., and Kroontje, W. (1964). The relationship between pH and ammonia equilibria in soil. Soil Sci. Soc. Am. J. 28 (6), 751–754. doi:10.2136/sssaj1964.03615995002800060022x
Ehrnsperger, L., and Klemm, O. (2021). Source apportionment of urban ammonia and its contribution to secondary particle formation in a mid-size European city. Aerosol Air Qual. Res. 21 (5), 200404. doi:10.4209/aaqr.2020.07.0404
Gundale, M. J., and DeLuca, T. H. (2007). Charcoal effects on soil solution chemistry and growth of Koeleria macrantha in the ponderosa pine/Douglas-fir ecosystem. Biol. Fertil. soils 43 (3), 303–311. doi:10.1007/s00374-006-0106-5
Haider, G., Joseph, S., Steffens, D., Müller, C., Taherymoosavi, S., Mitchell, D., et al. (2020). Mineral nitrogen captured in field-aged biochar is plant-available. Sci. Rep. 10 (1), 13816–13912. doi:10.1038/s41598-020-70586-x
He, X., and Suzuki, A. (2004). Effects of urea treatment on litter decomposition in Pasania edulis forest soil. J. Wood Sci. 50 (3), 266–270. doi:10.1007/s10086-003-0546-6
Hsiao, S. Y., Hsu, T. C., Liu, J. W., Xie, X., Zhang, Y., Lin, J., et al. (2014). Nitrification and its oxygen consumption along the turbid Chang Jiang River plume. Biogeosciences 11 (7), 2083–2098. doi:10.5194/bg-11-2083-2014
Hua, W., Luo, P., An, N., Cai, F., Zhang, S., Chen, K., et al. (2020). Manure application increased crop yields by promoting nitrogen use efficiency in the soils of 40-year soybean-maize rotation. Sci. Rep. 10 (1), 14882–14910. doi:10.1038/s41598-020-71932-9
Jechalke, S., Rosell, M., Vogt, C., and Richnow, H. H. (2011). Inhibition of nitrification by low oxygen concentrations in an aerated treatment pond system with biofilm promoting mats. Water Environ. Res. 83 (7), 622–626. doi:10.2175/106143011x12928814444493
Kang, Y., Liu, M., Song, Y., Huang, X., Yao, H., Cai, X., et al. (2016). High-resolution ammonia emissions inventories in China from 1980 to 2012. Atmos. Chem. Phys. 16 (4), 2043–2058. doi:10.5194/acp-16-2043-2016
Klimczyk, M., Siczek, A., and Schimmelpfennig, L. (2021). Improving the efficiency of urea-based fertilization leading to reduction in ammonia emission. Sci. Total Environ. 771, 145483. doi:10.1016/j.scitotenv.2021.145483
Lee, J., Choi, S., Lee, Y., and Kim, S. Y. (2021). Impact of manure compost amendments on NH3 volatilization in rice paddy ecosystems during cultivation. Environ. Pollut. 288, 117726. doi:10.1016/j.envpol.2021.117726
Lehmann, J., and Joseph, S. (2015). “Biochar for environmental management: an introduction,” in Biochar for environmental management (Earthscan, London: Routledge), 1–13.
Li, Y., Moinet, G. Y., Clough, T. J., and Whitehead, D. (2022). Organic matter contributions to nitrous oxide emissions following nitrate addition are not proportional to substrate-induced soil carbon priming. Sci. Total Environ. 851, 158274. doi:10.1016/j.scitotenv.2022.158274
Lü, D. A., Yan, B. X., Wang, L. X., Deng, Z. Q., and Zhang, Y. B. (2013). Changes in phosphorus fractions and nitrogen forms during composting of pig manure with rice straw. J. Integr. Agric. 12 (10), 1855–1864. doi:10.1016/s2095-3119(13)60400-1
Major, J., Rondon, M., Molina, D., Riha, S. J., and Lehmann, J. (2012). Nutrient leaching in a Colombian savanna Oxisol amended with biochar. J. Environ. Qual. 41 (4), 1076–1086. doi:10.2134/jeq2011.0128
Misselbrook, T. H., and Powell, J. M. (2005). Influence of bedding material on ammonia emissions from cattle excreta. J. dairy Sci. 88 (12), 4304–4312. doi:10.3168/jds.s0022-0302(05)73116-7
Monaco, S., Sacco, D., Pelissetti, S., Dinuccio, E., Balsari, P., Rostami, M., et al. (2012). Laboratory assessment of ammonia emission after soil application of treated and untreated manures. J. Agric. Sci. 150 (1), 65–73. doi:10.1017/s0021859611000487
Novamsky, I., Van Eck, R., Van Schouwenburg, C. H., and Walinga, I. (1974). Total nitrogen determination in plant material by means of the indophenol-blue method. Neth. J. Agric. Sci. 22 (1), 3–5. doi:10.18174/njas.v22i1.17230
Ohtsuka, Y., Mori, H., Watanabe, T., and Asami, K. (1994). Nitrogen removal during atmospheric-pressure pyrolysis of brown coal with iron. Fuel 73 (7), 1093–1097. doi:10.1016/0016-2361(94)90243-7
Piwowar, A. (2020). Farming practices for reducing ammonia emissions in Polish agriculture. Atmosphere 11 (12), 1353. doi:10.3390/atmos11121353
Rural Development Administration (RDA) (2017). Fertilization standard of crop plants. Jeonju, South Korea: National Institute of Agricultural Science and Technology.
Saggar, S., Singh, J., Giltrap, D. L., Zaman, M., Luo, J., Rollo, M., et al. (2013). Quantification of reductions in ammonia emissions from fertiliser urea and animal urine in grazed pastures with urease inhibitors for agriculture inventory: New Zealand as a case study. Sci. Total Environ. 465, 136–146. doi:10.1016/j.scitotenv.2012.07.088
Sah, R. N., and Mikkelsen, D. S. (1983). Availability and utilization of fertilizer nitrogen by rice under alternate flooding: I. Kinetics of available nitrogen under rice culture. Plant soil 75, 221–226. doi:10.1007/bf02375567
Tomczyk, A., Sokołowska, Z., and Boguta, P. (2020). Biochar physicochemical properties: pyrolysis temperature and feedstock kind effects. Rev. Environ. Sci. Bio/Technology 19 (1), 191–215. doi:10.1007/s11157-020-09523-3
Ventura, W. B., and Yoshida, T. (1977). Ammonia volatilization from a flooded tropical soil. Plant Soil 46 (3), 521–531. doi:10.1007/bf00015911
Wang, Y., Wang, H., Gao, C., Seglah, P. A., and Bi, Y. (2021). Urea Application rate for crop straw decomposition in temperate China. Appl. Environ. Soil Sci. 2021, 1–12. doi:10.1155/2021/2240807
Wu, Y., Gu, B., Erisman, J. W., Reis, S., Fang, Y., and Lu, X. (2016). PM2. 5 pollution is substantially affected by ammonia emissions in China. Environm. polluti. 218, 86–94.
Xiang, Y., Liu, Y., Niazi, N. K., Bolan, N., Zhao, L., Zhang, S., et al. (2023). Biochar addition increased soil bacterial diversity and richness: large-scale evidence of field experiments. Sci. Total Environ. 893, 164961. doi:10.1016/j.scitotenv.2023.164961
Xing, Y. F., Xu, Y. H., Shi, M. H., and Lian, Y. X. (2016). The impact of PM2.5 on the human respiratory system. J. Thorac. Dis. 8 (1), E69–E74. doi:10.3978/j.issn.2072-1439.2016.01.19
Yang, M., Fang, Y., Sun, D., and Shi, Y. (2016). Efficiency of two nitrification inhibitors (dicyandiamide and 3, 4-dimethypyrazole phosphate) on soil nitrogen transformations and plant productivity: a meta-analysis. Sci. Rep. 6 (1), 22075–22110. doi:10.1038/srep22075
Zhang, L., Chen, Y., Zhao, Y., Henze, D. K., Zhu, L., Song, Y., et al. (2018). Agricultural ammonia emissions in China: reconciling bottom-up and top-down estimates. Atmos. Chem. Phys. 18 (1), 339–355. doi:10.5194/acp-18-339-2018
Zhang, W. F., Dou, Z. X., He, P., Ju, X. T., Powlson, D., Chadwick, D., et al. (2013). New technologies reduce greenhouse gas emissions from nitrogenous fertilizer in China. Proc. Natl. Acad. Sci. 110 (21), 8375–8380. doi:10.1073/pnas.1210447110
Keywords: ammonia emission intensity, compost, swine manure, grain yield, biochar
Citation: Canatoy RC, Cho SR, Galgo SJC, Park SY and Kim PJ (2024) Biochar manure decreases ammonia volatilization loss and sustains crop productivity in rice paddy. Front. Environ. Sci. 12:1421320. doi: 10.3389/fenvs.2024.1421320
Received: 22 April 2024; Accepted: 18 June 2024;
Published: 18 July 2024.
Edited by:
Zisheng Xing, Agriculture and Agri-Food Canada (AAFC), CanadaReviewed by:
Taek-Keun Oh, Chungnam National University, Republic of KoreaYuan Li, Lanzhou University, China
Copyright © 2024 Canatoy, Cho, Galgo, Park and Kim. This is an open-access article distributed under the terms of the Creative Commons Attribution License (CC BY). The use, distribution or reproduction in other forums is permitted, provided the original author(s) and the copyright owner(s) are credited and that the original publication in this journal is cited, in accordance with accepted academic practice. No use, distribution or reproduction is permitted which does not comply with these terms.
*Correspondence: Pil Joo Kim, pjkim@gnu.ac.kr