- 1Department of Soil and Environmental Sciences, University of Sargodha, Sargodha, Pakistan
- 2Department of Environmental Sciences, Government College University, Faisalabad, Pakistan
- 3Institute of Molecular Biology and Biotechnology, University of Lahore, Lahore, Pakistan
- 4Department of Agronomy, Arid Agriculture University, Rawalpindi, Pakistan
- 5Department of Botany, Sardar Bahadur Khan Women’s University, Quetta, Pakistan
- 6Department of Botany and Microbiology, College of Science, King Saud University, Riyadh, Saudi Arabia
- 7Office of Engagement and Extension, Colorado State University, Fort Collins, CO, United States
- 8USDA-NRCS Canon City Field Office, Canon, CO, United States
- 9Department of Biological Sciences, Superior University, Lahore, Pakistan
- 10Colorado Water Center, Colorado State University, Fort Collins, CO, United States
- 11Central Great Plains Resources Management Research, USDA-Agricultural Research Service (ARS), Akron, CO, United States
Wastewater is considered a good reservoir of mineral elements that can be used for agriculture, aquaculture, and some other activities after adopting suitable measures. The gap between supply and demand for water is increasing exponentially because of the abrupt boost to the world’s population. This poses a threat to human life as it has reached alarming levels in some parts of the globe. Normally, wastewater consists of liquid waste produced by commercial or industrial sources for daily use, consumption, and production. It is time to refocus our attention on a kind of circulating water system by reusing municipal wastewater for agricultural purposes, particularly irrigation. The recycled or treated water would be used as an alternative to fresh water. In the current study, the impact of various organic amendments was studied to mitigate the toxic effects of pollutants present in wastewater by cultivating maize as a test crop. The present study comprised five treatments replicated four times with a randomized complete block design under field conditions. In this experiment, the treatments included T1 (treatment 1) = control (wastewater-polluted soil without the application of any amendment), T2 = farmyard manure (FYM) at 2.5 tons ha-1 (hectare-1), T3 = FYM at 5.0 tons ha-1, T4 = compost at 2.5 tons ha-1, and T5 = compost at 5.0 tons ha-1. The application of FYM at 5.0 tons ha-1 (T3) was recorded as being the most effective as the maximum improvement was observed in soil characteristics such as pH, electrical conductivity (EC), sodium adsorption ratio (SAR), and organic matter, and for T3, these were 7.33, 2.22 dS m-1, 8.16, and 0.94%, respectively. T3 remained most superior in reducing the concentration of heavy metals in the soil; for example, lead, cadmium, nickel, and arsenic for T3 were 8.64, 1.34, 10.44, and 2.25 mg kg-1 (milligrams per kg), respectively. Maximum fresh biomass (fodder yield) of 9.98 tons ha-1 was harvested when FYM was applied at 5.0 tons ha-1 to the soil compared to 6.2 tons ha-1 in the control plot. The highest contents of nitrogen (1.20%), phosphorus (0.41%), and potassium (3.97%) were observed in maize plants for T3. In maize plants (T3), the concentration of lead, cadmium, nickel, and arsenic was reduced to levels of 1.92, 0.23, 2.28, and 1.25 mg kg-1, respectively. Therefore, it can be concluded from the findings of the experiment that the application of FYM significantly reduced heavy metal concentrations and improved soil health, along with maize crop growth and productivity.
Introduction
Water scarcity poses an important challenge to the progress of sustainable farming, particularly in the dryland agricultural parts of the world (Smith et al., 2018). In these areas, farmers now face the necessity of utilizing urban wastewater for irrigation purposes (Johnson and Thompson, 2019). As the global population continues to rise and the demand for food increases, water resources are dwindling, and long-distance transport of water imposes a substantial strain on agricultural productivity (Brown et al., 2022). Utilizing treated urban wastewater for irrigation offers a potential solution by mitigating water shortages, enhancing water usage efficiency, and reducing water pollution (Gomez et al., 2021). The optimal utilization of all available water resources, including treated urban wastewater, is becoming crucial in dry and semi-arid climates due to the immense pressure on non-renewable water sources, prolonged drought periods, and rapid urbanization (White et al., 2017). Hence, the reuse of treated urban wastewater has emerged as an unconventional yet promising water resource that can alleviate water scarcity concerns to some extent (Garcia et al., 2020). As wastewater contains essential plant nutrients, it becomes imperative to establish proper guidelines for its utilization to minimize the adverse effects associated with wastewater irrigation and maximize its performance (Adams et al., 2021). Furthermore, it is indispensable to judge other effects of wastewater, for example, changes in soil and plant elemental composition, pollutants, and heavy metal accumulation (Lee et al., 2019).
The most common method is to dump municipal wastewater (untreated and treated) on land in both developing and developed countries. The majority of the wastewater is used to irrigate fiber, seed, and feed crops, and, to some extent, vineyards, orchards, and other crops in developed countries by following environmental standards. Standards have been established in developing countries, but they are not always strictly enforced. Hussain et al. (2002) reported that in Mexico, India, and China, untreated wastewater has been most commonly used in aquaculture and agriculture for 100 years. Heavy metals can enter the food chain as a result of wastewater irrigation because they accumulate in the soil and are then taken up by plants. Huang M et al. (2016) reported that soil serves as both a source and a filter, assisting in the removal of contaminants from wastewater. The quantity and origin of the metal, the properties of the soil, and the rate at which plant species absorb certain metals are only a few of the variables that affect the transmission of metals into the food chain (Chen et al., 2018). Many health problems can result from the buildup of toxic metals in the food chain (Smith et al., 2018). Long-term irrigation with domestic and industrial wastewater can cause toxic metal accumulation in soils, and the affected soils not only deteriorate the quality of the produce, particularly the edible sections of plants but also the productivity of field crops (Lee et al., 2019; Nte et al., 2022; Rizwan et al., 2022).
Elements with metallic characteristics including malleability, ductility, conductivity, cation stability, and ligand selectivity are known as heavy metals. These often have atomic weights above 20 and high densities (Briffa et al., 2020). Heavy metals, also known as metalloids, such as Pb, Cd, Hg, and As, are regarded as serious hazards because they have no beneficial effects on organisms and are extremely dangerous to both plants and animals. Heavy metal-contaminated soils frequently have poor biological, physical, and chemical characteristics. Low organic matter content, insufficient nutrient levels, micronutrient imbalances, toxicity, decreased N and P utilization, high salinity, and severe pH levels are examples of unfavorable soil conditions (Gill et al., 2014). The presence of heavy metals in foodstuffs is associated with health risks for human beings (Gupta et al., 2018).
Organic soil additives play an important role in enhancing the structure and texture of soils and increasing soil organic concentration, including the retention capacity for water and nutrients. The application of biochar, animal manure, and compost are some common organic practices or soil amendments to minimize the impact of potentially toxic elements (PTEs) (Shaheen and Rinklebe, 2015; Ahmad et al., 2017; Pangaribuan et al., 2023) and ultimately improve crop productivity (Abd-El-Fattah et al., 2022; Hussan et al., 2022). The dairy, beef, pig, and poultry industries produce livestock wastes. These are widely used by farmers as fertilizers due to their high nutritional value. These are also thought to be ideal for reducing soil-based PTE migration (Bolan et al., 2003). Houben et al. (2012) reported that applying bone meal and cow dung to polluted soils can significantly reduce Zn and Cd leaching. Houben et al. (2012) reported that applying soil amendments can lessen the mobility of toxic metals like lead, zinc, and cadmium. Shaheen et al. (2014) concluded that the contents of copper, iron, nickel, manganese, and zinc can be reduced by the application of bone meal. The utilization of compost can easily reduce the risk of crop failure and its impact on human health. Vegetable compostable organic additives/green compost and cultivating halophytes are usually used to fix PTEs in contaminated soils (Huang X et al., 2016; Ghous et al., 2022). This study took a comprehensive and novel approach to address wastewater-created soil pollutant toxicity, including the evaluation of heavy metal immobilization, soil fertility improvement, and crop growth promotion. Wastewater can be safely used if coupled with organic amendments, particularly farmyard manure (FYM). FYM has the potential to alleviate the noxious impact of contaminants in wastewater on maize plants, refining soil healthiness, and decreasing heavy metal contents. Keeping in view the current scenario of water deficiency, the current research assessed the efficiency of organic materials to mitigate wastewater-created soil pollutant toxicity and shortlisted the most effective measures.
Materials and methods
The present study was conducted from February 2020 to September 2021 at the College of Agriculture, University of Sargodha, Sargodha, Punjab, Pakistan. The efficiency of organic amendments was evaluated with respect to mitigating soil toxicity created using untreated municipal wastewater irrigation and concluded with final recommendations for farmers about the use of wastewater irrigation. In this experiment, organic amendments (farmyard manure and compost) at two levels (2.5 tons and 5 tons ha-1) with four replications were applied along with the control and evaluated on clay loam soil under field conditions using maize as the test crop.
The FYM was prepared from the decomposition of animal waste, typically from farms or rural areas, and consisted of a mixture of animal excreta (cow dung, urine, and bedding material) and leftover crop materials (straw, hay, or other plant residues). The decomposition process involves the microbial breakdown of organic matter, resulting in a nutrient-rich fertilizer. Typically, FYM contains plenty of nutrients like nitrogen, phosphorus, potassium, and other micronutrients, making it a valuable soil amendment for improving soil fertility and structure. Compost was prepared from organic matter such as fruits, vegetables, tea bags, leaves, grass clippings, plant residues like crop waste, and weeds. These organic matter were mixed and allowed to decompose, resulting in a nutrient-rich soil amendment that can be used to improve the soil fertility and structure.
A 5 × 5 m2 plot was created, treatments were applied accordingly, and wastewater (black water) was used as a source of irrigation. In this experiment, the treatments included T1 (treatment 1) = control (wastewater-polluted soil without the application of any amendment), T2 = FYM at 2.5 tons ha-1 (hectare-1), T3 = FYM at 5.0 tons ha-1, T4 = compost at 2.5 tons ha-1, and T5 = compost at 5.0 tons ha-1. These treatments were applied after laboratory analysis (Table 1) and randomized using a randomized complete block design (RCBD) with four replications. The layout is shown in Figure 1.
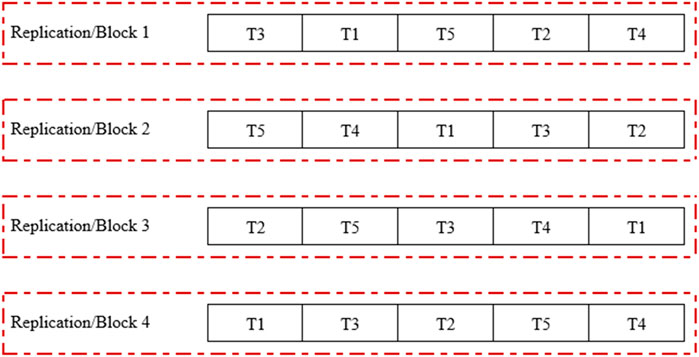
Figure 1. Field layout of the randomized complete block design with four replications. T1 (treatment 1) = control (wastewater-polluted soil without application of any amendments), T2 = farmyard manure (FYM) at 2.5 tons ha-1 (hectare-1), T3 = FYM at 5.0 tons ha-1, T4 = compost at 2.5 tons ha-1, and T5 = compost at 5.0 tons ha-1.
At the maturity stage, maize plants were harvested, and plant samples were collected. Soil samples were also collected from all plots. Both plant and soil samples were analyzed for different organic and inorganic pollutants.
Analysis of soil, plant, and water samples
All laboratory analyses for soil, plant, and water samples were made according to the methods in the USDA Handbook 60 of U.S. Salinity Laboratory Staff (1969). These methods were used for the determination of pH, EC, SAR, Ca, and K in soil and water samples. Similarly, wet digestion of plant samples (leaves) was done accordingly. Subsequently, P and K contents in maize plant samples (leaves) were determined according to the referenced methods.
Soil texture
A 50 g soil sample was placed in a 500-mL beaker. Next, we added 50 mL of a 1% sodium hexametaphosphate solution and 250 mL of distilled water to the beaker. The mixture was left to stand overnight. Afterward, the soil was stirred for 10 min using a Bouyoucos apparatus/mechanical stirrer. The volume of the suspension was then adjusted to obtain the desired level before being placed into a 1,000-mL graduated cylinder. Readings from a Bouyoucos hydrometer were recorded both 4 s and 2 h after the suspensions were mixed. The International Textural Triangle, which offers a categorization system based on the proportions of sand, silt, and clay in the soil (Moreno-Maroto and Alonso-Azcarate, 2022), was used to identify the soil textural class.
The pH of the soil was determined using a pH meter (Jenway 3510). To do this, a saturated soil paste was prepared (Method 2) and the pH of the soil was then determined after calibrating the pH meter using buffer solutions of values 7.0 and 10.0 (Method 54). Using an EC meter, soil samples were prepared to measure the amount of soluble salts using Method 4b. The EC meter was calibrated with a 0.1 N KCl solution.
The formula used to compute the sodium adsorption ratio (SAR) was
Each cation was written as me L-1 (USDA Handbook 60).
The Eaton (1950) equation was used to compute the residual sodium carbonate (RSC):
The method outlined by Walkley and Black (1934) was used to calculate the total soil organic carbon content. The sample was oxidized by adding concentrated H2SO4 and 1N K2Cr2O7 and the subsequent addition of H3PO4. Titration was performed for the oxidized carbon content against 0.5 M ferrous ammonium sulfate. Finally, the organic C content was obtained after multiplying the value by a factor of 1.334. Dissolved organic C was extracted using a K2SO4 solution.
Heavy metal protocols
The samples were analyzed using an atomic absorption spectrophotometer (AAS) (Analytik Jena, model novAA-400P, Germany) to determine the concentrations of heavy metals. The AAS is a reliable tool for measuring the concentration of heavy metals. Its operation is based on the principle of light absorption by the ground state of the metals at specific wavelengths. In this technique, the liquid sample containing metal particles is transformed into an atomic state by passing it through a flame. The flame consists of combustible gases such as air and acetylene mixture. A specific wavelength of light is directed through the flame, and the reduction in light intensity caused by the absorption of the analyte is measured against a standard reference. During the analysis, the sample is prepared in a liquid form, aerosolized, and introduced into the flame. The temperature of the flame typically ranges from 2,100°C to 2,800°C. As the sample burns, the atoms of the target element are reduced to unexcited ground-state free particles that absorb light at precise wavelengths unique to each element. These wavelengths, which are accurate to a range of 0.01–0.1 nm, are used to determine the specific wavelength for each element of interest. To identify the absorption wavelength, a light beam with an electrode made of the element in question is passed through the flame. The reduction in light intensity caused by the absorption of the analyte is detected by a photon multiplier. The concentration of the element in the sample can be used to correlate this decrease in intensity (Smith et al., 2018).
Nitrogen measurement
A Kjeldahl distillation apparatus was used for the estimation of nitrogen content in plant samples (O’Sullivan, 2011). Method 61 from the USDA Handbook 60 was used to determine the total phosphorus concentration in maize plant samples. A spectrophotometer was used for the determination of phosphorus with the pink Wade reagent [0.03% FeCl3 solution containing 0.3% sulfosalicylic acid solution, 3/1 (v/v)]. Method 58a from the USDA Handbook 60 was used to determine the total potassium concentration in maize plant samples by standard solutions of K2SO4 through a flame photometer. Ca was determined using an atomic absorption spectrophotometer. For the determination of Ca, standard solutions of Ca prepared from CaCO3 and HCl were used.
Plant height was taken as the length from the base of the plant to the tip at its maturity stage. A meter rod was used to measure the plant height. A Vernier caliper was used to note the stem diameter of the plants. At maturity, the maize crop was harvested, and the biomass yield was recorded.
Therefore, the values for various soil parameters (pH, EC, SAR, Pb, Cd, Ni, and As) reported in this research paper are the average of four analyses carried out throughout the study period after the harvest of each maize crop. Similarly, maize plant samples were collected after each harvest (four times) throughout a study period of 2 years and were analyzed in the laboratory for concentrations of nitrogen, phosphorus, potassium, lead, cadmium, nickel, and arsenic. In this paper, average values for these parameters are given and explained to make the results more concise and comprehensive.
Statistical analysis
Using the software program “Statistics 10,” the data collected on soil and crop properties were subjected to statistical analysis (ANOVA). The data from two seasons were tested for homogeneity using Bartlett’s test (Bartlett, 1937), and were found to be homogeneous; therefore, the data from all seasons were combined for analysis. According to the procedure outlined by Steel et al. (1997), significant differences between treatment effects were identified using the LSD test at the 1% probability level, and these differences were marked with various alphabets (a, b, c, etc.).
Results
This experiment was continued for 2 years, during which time four maize crops were harvested. Wastewater was used as a source of irrigation throughout the study period. Wastewater was analyzed after each month, and the average values of these analyses are given in Table 2. Similarly, soil samples were collected from each treatment plot of the experiment after harvesting each crop and were analyzed in the laboratory for various parameters.
Impact of organic amendments on the chemical characteristics of the soil
Figure 2 provides information about soil EC, pH, SAR, and OM for different treatments. The highest EC of 2.78 ± 0.020 dS m-1 was recorded under the control treatment, whereas the minimum EC value for T3 was 2.22 ± 0.017 dS m-1. The maximum soil pH of 8.16 ± 0.017 was observed in the control treatment (Figure 2). The soil pH for T2 was 7.44 ± 0.016 and that for T3 was 7.33 ± 0.021. The soil pH for compost at 2.5 t ha-1 (T4) was 7.51 ± 0.011. Information about the soil sodium adsorption ratio (SAR) for the different treatments is provided in Figure 2. The control treatment (T1) represents the baseline condition without any organic amendments. The SAR value for the control treatment was 13.18 ± 0.014. The SAR value for FYM at 2.5 t ha-1 (T2) was 8.28 ± 0.015, whereas the same value for FYM at 5.0 t ha-1 (T3) was 8.16 ± 0.011. Soil organic matter is an important factor in soil health and fertility as it contributes to nutrient availability, water-holding capacity, and overall soil structure. The amount of soil organic matter determined for the control treatment was 0.78% ± 0.009% (Figure 2). The soil organic matter content for FYM at 2.5 t ha-1 (T2) was 0.86% ± 0.017% in contrast to the organic matter content of 0.94% ± 0.014% for T3 (FYM at 5.0 t ha-1). The soil organic matter content for T4 (compost at 2.5 t ha-1) was 0.84% ± 0.014%. Similarly, T5 (compost at 5.0 t ha-1) indicated 0.86% ± 0.018% soil organic matter Impact of organic amendments on heavy metals contents in soil
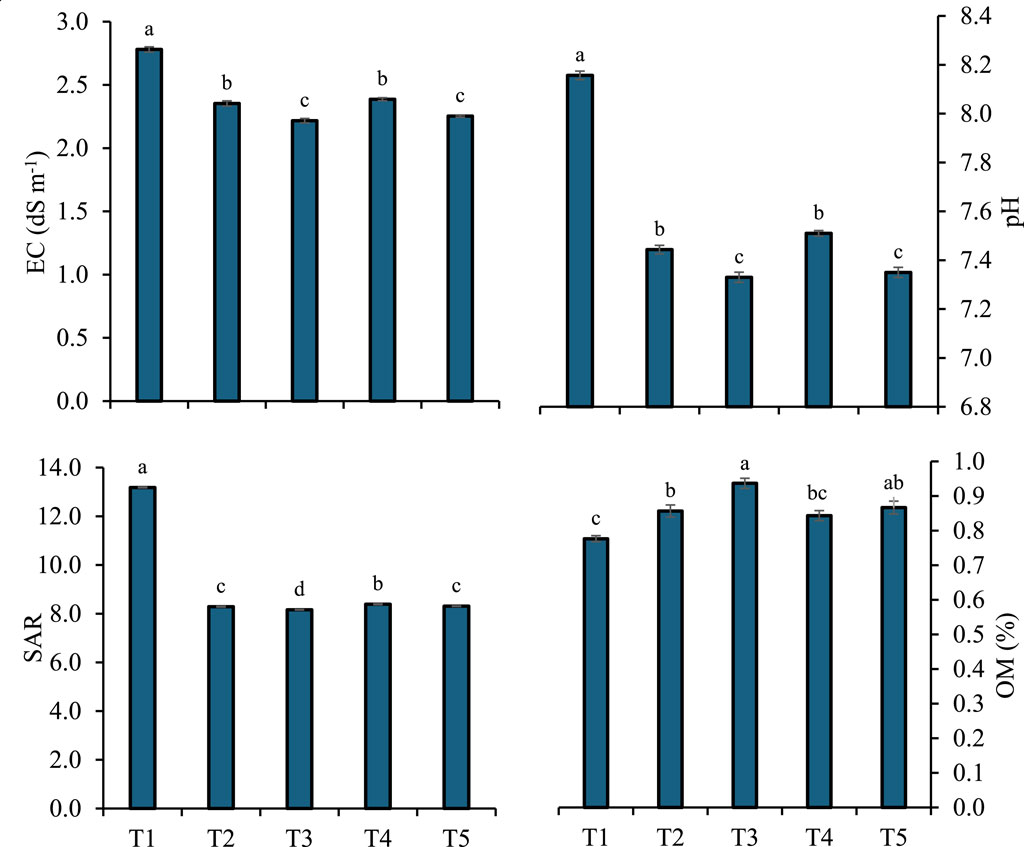
Figure 2. Impact of organic amendments on soil characteristics (EC, pH, SAR, and OM). T1 = control (wastewater-polluted soil without application of any amendment), T2 = FYM at 2.5 tons ha-1 (hectare-1), T3 = FYM at 5.0 tons ha-1, T4 = compost at 2.5 tons ha-1, and T5 = compost at 5.0 tons ha-1. Bars containing the same letter did not differ significantly at p ≤ 0.05 for a particular attribute.
Figure 3 presents the concentrations of lead, cadmium, nickel, and arsenic in soils for different treatments. The control treatment (T1) exhibited the highest lead concentration at 12.24 ± 0.011 mg/kg in the soil. Treatment (T2) involving soil amended with FYM at 2.5 t ha-1 resulted in a reduced lead concentration of 8.85 ± 0.011 mg/kg. Furthermore, increasing the FYM concentration to 5.0 t ha-1 (T3) resulted in a further decrease in lead content to 8.64 ± 0.017 mg/kg. This indicates that higher concentrations of FYM have a more pronounced effect on reducing lead contamination. In the case of compost amendments, the treatment (T4) with compost at 2.5 t ha-1 resulted in a lead concentration of 8.87 ± 0.011 mg/kg. Similarly, the treatment (T5) with compost at 5.0 t ha-1 exhibited a lead concentration of 8.75 ± 0.014 mg/kg. Figure 3 shows that the control treatment (T1) exhibited the highest cadmium concentration at 1.81 ± 0.012 mg/kg in untreated soil. The treatment (T2) involving soil amended with FYM at 2.5 t ha-1 resulted in a reduced cadmium concentration of 1.42 ± 0.020 mg/kg. Furthermore, increasing the FYM concentration to 5.0 t ha-1 (T3) caused a further decrease in cadmium quantity to 1.34 ± 0.008 mg/kg. In the case of compost amendments, treatment with compost at 2.5 t ha-1 (T4) resulted in a cadmium concentration of 1.46 ± 0.014 mg/kg. Similarly, the treatment with compost at 5.0 t ha-1 (T5) exhibited a cadmium concentration of 1.36 ± 0.014 mg/kg. Data on nickel concentrations in soils for each treatment are also provided in Figure 3. The control treatment (T1) exhibited the highest nickel concentration at 13.63 ± 0.015 mg/kg in untreated soil. The application of FYM at 5.0 t ha-1 (T3) caused the maximum reduction in nickel content to 10.44 ± 0.017 mg/kg. In the case of compost application, treatment with compost at 2.5 t ha-1 (T4) and 5 t ha-1 (T5) resulted in a nickel concentration of 10.66 ± 0.021 mg/kg and 10.48 ± 0.020 mg/kg, respectively. Likewise, the control treatment (T1) exhibited the highest arsenic concentration at 4.25 ± 0.011 mg/kg in untreated soil (Figure 3), whereas a minimum concentration of 2.25 ± 0.020 mg/kg was found under the application of FYM concentration to 5.0 t ha-1 (T3).
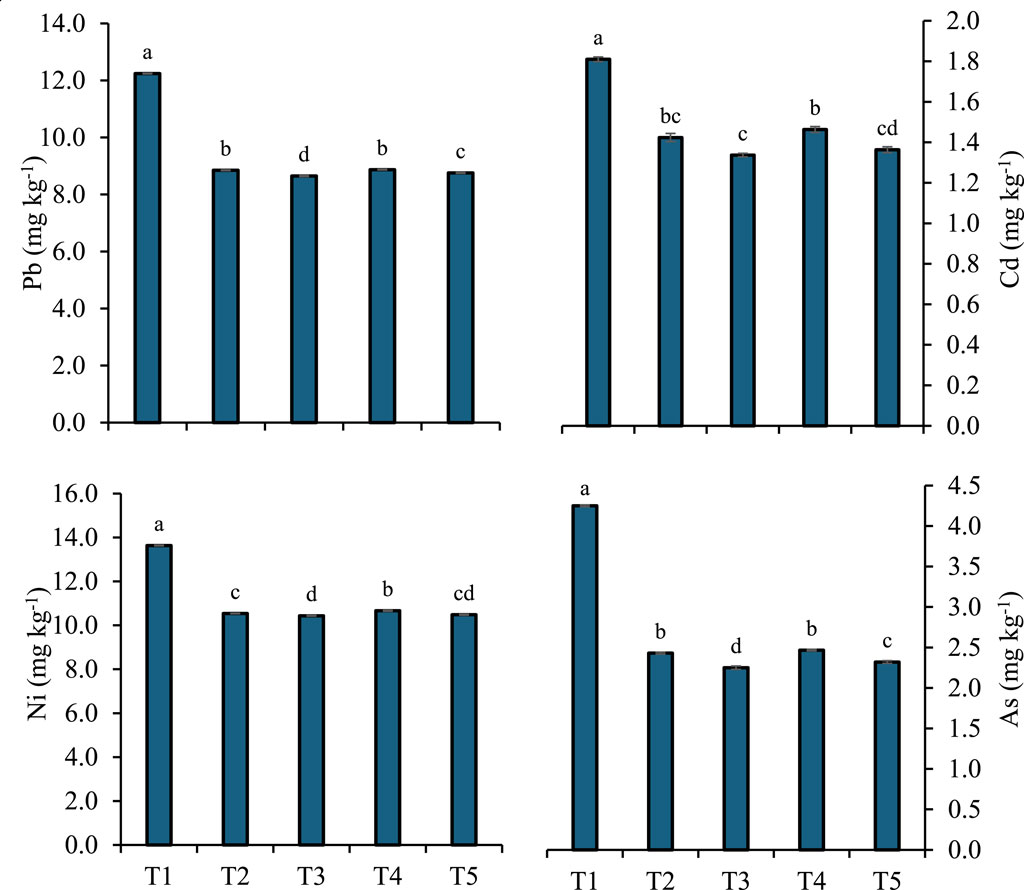
Figure 3. Impact of organic amendments on concentrations of heavy metals (Pb, Cd, Ni, and As) in the soil. T1 = control (wastewater-polluted soil without the application of any amendments), T2 = FYM at 2.5 tons ha-1 (hectare-1), T3 = FYM at 5.0 tons ha-1, T4 = compost at 2.5 tons ha-1, and T5 = compost at 5.0 tons ha-1. Bars containing the same letter did not differ significantly at p ≤ 0.05 for a particular attribute.
Impact of organic amendments on the yield parameters of maize
Data on the plant height in different treatments are given in Figure 4. Under the control treatment (T1), the average plant height was measured to be 167.33 ± 1.20 cm. The average plant height recorded under T2 (FYM at 2.5 t ha-1) was 176.33 ± 1.45 cm. T3 involved adding a higher concentration of FYM to the soil at 5.0 t ha-1, and the average plant height observed under T3 was 188.33 ± 1.45 cm, which is significantly higher than that in the control treatment and T2. The average plant height recorded under T4 (compost at a concentration of 2.5 t ha-1) was 173.67 ± 0.88 cm in contrast to T5 (compost at 5.0 t ha-1) with a height of 182.33 ± 1.76 cm. The average stem diameter recorded in the control treatment (T1) was 27.33 ± 1.20 cm (Figure 4). T3 with FYM at 5.0 t ha-1 produced a stem diameter of 42.33 ± 1.76 cm, which was significantly higher than that in the control treatment and T2. Figure 4 presents the forage yield of maize plants under different treatments, including the control (T1). Among all the five treatments, T3 (FYM at 5.0 t ha-1) displayed the maximum forage yield of 9.98 ± 1.76 t ha-1, whereas the lowest forage yield among all the treatments was in the control treatment.
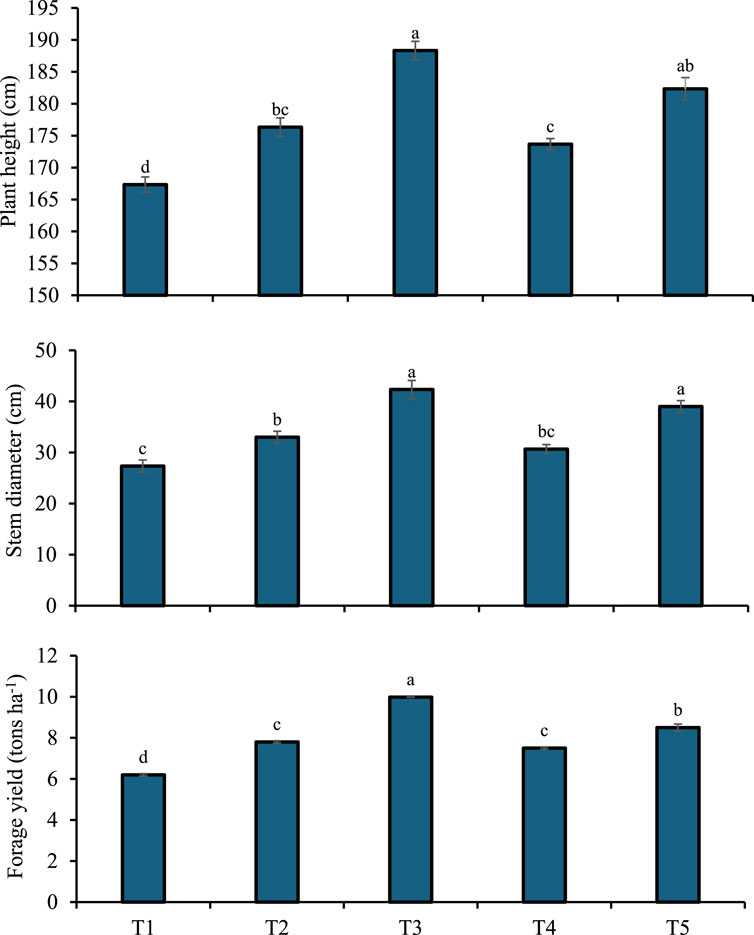
Figure 4. Impact of organic amendments on maize plant height, stem diameter, and forage yield. T1 = control (wastewater-polluted soil without application of any amendments), T2 = FYM at 2.5 tons ha-1 (hectare-1), T3 = FYM at 5.0 tons ha-1, T4 = compost at 2.5 tons ha-1, and T5 = compost at 5.0 tons ha-1. Bars containing the same letter did not differ significantly at p ≤ 0.05 for a particular attribute.
Impact of organic amendments on the mineral elements of maize plants
Figure 5 shows N, P, K, and Ca contents in leaves of maize plants under different treatments. The results showed that the application of organic matter generally increased the nitrogen content in the leaves of maize plants in contrast to the control (T1). Among all treatments, T3 (FYM at 5.0 t ha-1) has the highest nitrogen value of 1.27% ± 0.012%, followed by T5 (compost at 5.0 t ha-1) with a nitrogen content of 1.2% ± 0.057%. Among all treatments, T3 (FYM at 5.0 t ha-1) displayed the maximum P content with 0.41% ± 0.011% (Figure 5). T5 (compost at 5.0 t ha-1) followed T3 with a P content of 0.370.012%. The application of FYM at 5.0 t ha-1 exhibited the highest K value of 3.97% ± 0.12%, followed by T5 (compost at 5.0 t ha-1) with a value of 3.460.09% (Figure 5). T3 (FYM at 5.0 t ha-1) showed the highest calcium content with a value of 5.85% ± 0.011% (Figure 5). T5 (compost at 5.0 t ha-1) followed T3 with a Ca content of 5.51% ± 0.015%. T2 (soil + FYM at 2.5 t ha-1) exhibited a slightly lower calcium content of 5.24% ± 0.009% than T3 and T5.
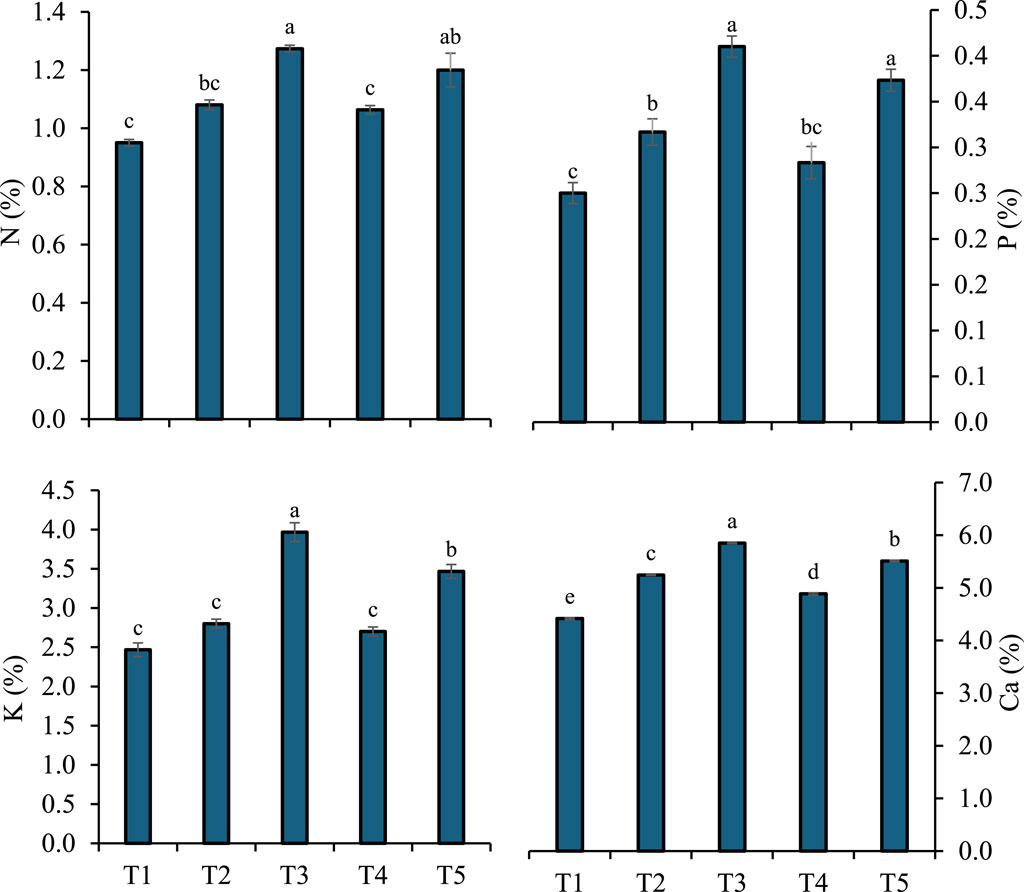
Figure 5. Impact of organic amendments on the accumulation of mineral elements (N, P, K, and Ca) in maize leaves. T1 = control (wastewater-polluted soil without application of any amendments), T2 = FYM at 2.5 tons ha-1 (hectare-1), T3 = FYM at 5.0 tons ha-1, T4 = compost at 2.5 tons ha-1, and T5 = compost at 5.0 tons ha-1. Bars containing the same letter did not differ significantly at p ≤ 0.05 for a particular attribute.
Impact of organic amendments on the concentration of heavy metals in maize plants
Figure 6 presents the concentration of heavy metals (Pb, Cd, Ni, and As) accumulated in maize plants under different treatments. Among all the treatments, the control (T1) has the highest lead content with a value of 4.81 ± 0.008 mg/kg. T3 (FYM at 5.0 t ha-1) exhibited the lowest lead value of 1.92 ± 0.017 mg/kg. T5 (compost at 5.0 t ha-1) showed a relatively high lead content of 2.1 ± 0.02 mg/kg when compared with T3. The control treatment (T1) has the highest cadmium content with a value of 0.47 ± 0.007 mg/kg. T3 (FYM at 5.0 t ha-1) exhibited the lowest cadmium value of 0.23 ± 0.012 mg/kg (Figure 6). The control treatment (T1) had the highest nickel content, with a value of 6.76 ± 0.013 mg/kg. T3 (FYM at 5.0 t ha-1) exhibited the lowest nickel value of 2.28 ± 0.019 mg/kg. T5 (compost at 5.0 t ha-1) showed a relatively high nickel content of 3.45 ± 0.023 mg/kg compared with T3. Treatments T2 (FYM at 2.5 t ha-1) and T4 (compost at 2.5 t ha-1) indicated nickel values of 3.42 ± 0.015 and 4.23 ± 0.014 mg/kg, respectively (Figure 6). Figure 6 shows that the control treatment (T1) had the highest arsenic content with a value of 2.75 ± 0.021 mg/kg. T3 (FYM at 5.0 t ha-1) exhibited the lowest arsenic value of 1.25 ± 0.022 mg/kg. T5 (compost at 5.0 t ha-1) showed a relatively high arsenic content of 1.42 ± 0.018 mg/kg compared with T3. T2 (FYM at 2.5 t ha-1) and T4 (compost at 2.5 t ha-1) indicated arsenic values of 1.95 ± 0.012 and 2.25 ± 0.011 mg/kg, respectively (Figure 6).
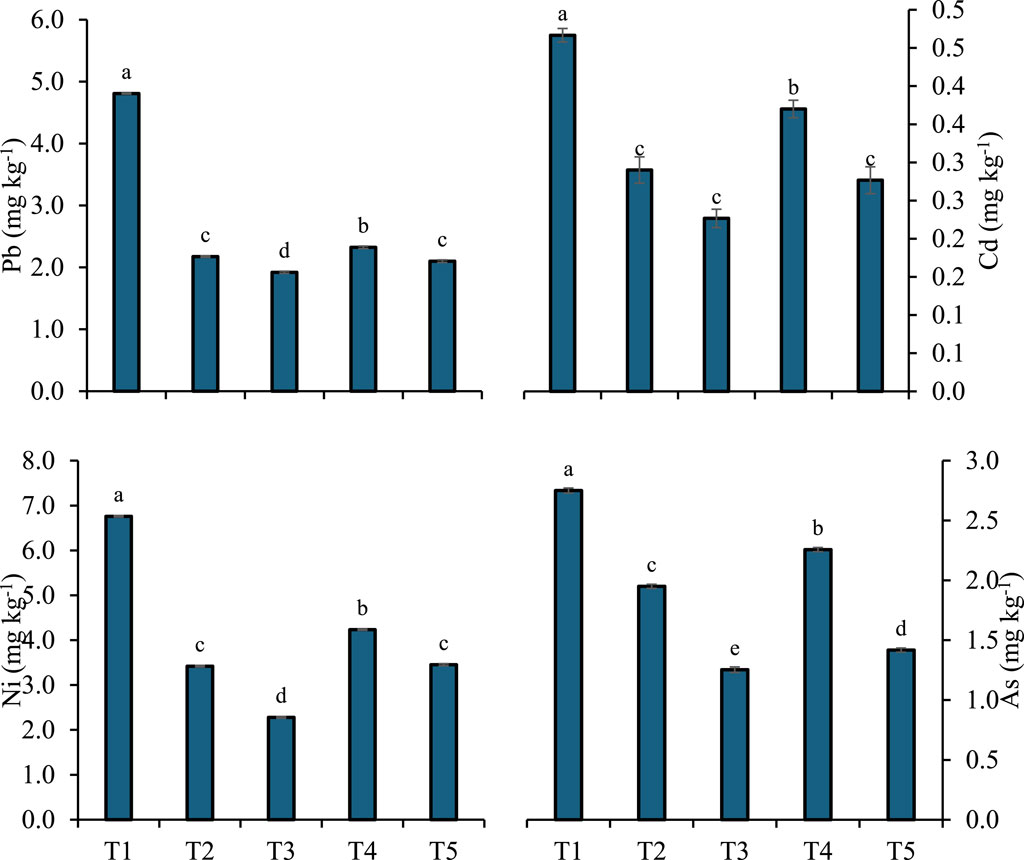
Figure 6. Impact of organic amendments on concentrations of heavy metals (Pb, Cd, Ni, and As) in maize. T1 = control (wastewater-polluted soil without application of any amendments), T2 = FYM at 2.5 tons ha-1 (hectare-1), T3 = FYM at 5.0 tons ha-1, T4 = compost at 2.5 tons ha-1, and T5 = compost at 5.0 tons ha-1. Bars containing the same letter did not differ significantly at p ≤ 0.05 for a particular attribute.
Discussion
This study revealed that untreated wastewater used for agricultural purposes indeed contains alarmingly high concentrations of heavy metals, posing a serious threat to the environment and human health. These heavy metals, including lead, cadmium, chromium, and arsenic, are well known for their toxic effects and ability to persist in the environment. The results demonstrated the significant accumulation of heavy metals in these soils over time. The toxicity levels observed in both water and soil samples underscored the urgent need for effective remediation measures to reduce heavy metal contamination and mitigate its adverse effects. In addition to reducing heavy metal concentrations, the current research examines the broader impact of wastewater treatment with organic amendments on soil quality. The soil reaction (pH) is the only parameter that illuminates a complete representation of growth media for crop development, counting nutrient supply tendency, the fortune of supplementary nutrients, salt position and soil airing, soil mineral composition, and final climate situations of the area. Soil pH is basic in several parts of Pakistan, comprising arid and semi-arid regions (Sarwar, 2005). Any land management strategy that results in a decrease in soil pH is responsible for altering the soil to improve the crop productivity. Our findings are in line with the findings of Taeprayoon et al. (2022), who focused on the application of organic fertilizers in cadmium-contaminated soils for the phytoremediation of three bioenergy crops. Their study examined the effectiveness of organic additives in stabilizing cadmium concentrations and stimulating the growth of bioenergy crops in contaminated soils. Furthermore, Wang et al. (2020) studied the effect of soil fertilizers on the fixation and accumulation of heavy metals in maize grown in multi-metal-contaminated soils. Van-Zwieten (2018) discussed the enduring role of organic materials in eliminating soil problems for sustainable crop production.
Alam et al. (2020) investigated the impacts of organic modification on the bioavailability of heavy metals in mining-affected soils and the associated health risks in humans. The results showed that organic modifiers could effectively reduce the bioavailability of heavy metals, thereby minimizing potential risks to human health. Organic fertilizers are natural alternatives to synthetic inorganic fertilizers and are popular with farmers. Organic fertilizers, which are derived from the remains or by-products of a single organism, have many benefits. They gradually release nutrients, improve soil structure, improve nutrient content, and stimulate microbial activity (Chen et al., 2018). The use of organic waste such as sewage sludge, bird droppings, and cow dung as fertilizer maximizes the value of resources and solves the problem of waste disposal. Furthermore, organic waste used as fertilizer is environmentally friendly and critical to wildlife and human safety (Sharma et al., 2019). The usage of organic fertilizers in soils can increase the fertility status of the soil, increase yields, improve the overall soil condition, and, at the same time, solve the problems of pesticide residues and water-source nutrient pollution. Monitoring of enzymes such as urease, sucrase, and phosphatase provides valuable information about soil health and microbial activity. Common practices such as composting organic waste such as sewage sludge, bird droppings, and garden waste are often used to improve soil structure, nutrient levels, and microbial activity (Johnson and Thompson, 2019; Bui et al., 2023). Poultry manure and horse manure are also valuable sources of essential nutrients for plants and are often used as fertilizers. However, trace elements present in sewage sludge and fertilizers require careful handling to prevent their accumulation in food plants and reduce potential adverse effects on human health (Sharma et al., 2019).
In a recent study, Rani and Singh (2022) focused on addressing heavy metal contamination in soils through the addition of organic matter like farm and poultry manure, compost, and biochar. Their findings demonstrated the effectiveness of these amendments in reducing heavy metal concentrations and enhancing soil quality. Similarly, Rashmi et al. (2022) emphasized the environmentally friendly approach of using soil amendments to promote soil health and sustainable oilseed production. Their research explored various organic amendments and their potential to recover soil fertility and production. Yasir et al. (2022) specifically explored the efficacy of organic amendments in reducing the phytotoxicity of cadmium (Cd) assimilation in wheat. Their study showed that organic modifiers can reduce cadmium toxicity and plant uptake. Similarly, Koishi et al. (2020) studied the impact of organic fertilizers on the quantity and quality of soil organic matter in conventional agriculture. Their research revealed the positive outcome of organic fertilizers in increasing soil organic matter and improving the overall condition of the soil. From a broader perspective, Goss et al. (2013) conducted a comprehensive review of the use of organic modifiers in agriculture and their potential impact on human health. Their research assessed the potential benefits and dangers of organic farming practices. On the other hand, Kwiatkowska-Malina (2018) studied the function of organic matter in contaminated soils and investigated how organic additives affect the availability of heavy metals to plants in contaminated soils.
Zhao et al. (2014) reported a reduction in lead concentration in contaminated soils from 15.3 mg/kg to 10.2 mg/kg with the application of compost at 3 tons ha-1. Huang M et al. (2016) found that the application of organic amendments, including FYM, reduced lead concentrations in soils by up to 35% compared to control treatments. The reductions observed in the current study (Figure 3) are consistent with the findings of Zhao et al. (2014) and Huang X et al. (2016), demonstrating the effectiveness of organic amendments in reducing lead contamination in soils. Bolan et al. (2010) showed that the application of compost reduced cadmium concentrations in soils by up to 30%. Khan et al. (2013) found a significant reduction in cadmium levels in contaminated soils with the application of FYM, from 2.0 mg/kg to 1.5 mg/kg. The cadmium reduction in this study (Figure 3) aligns well with the findings of Bolan et al. (2010) and Khan et al. (2013), indicating the beneficial impact of organic amendments on cadmium contamination.
Clemente et al. (2006) observed a reduction in nickel concentrations in contaminated soils from 14.5 mg/kg to 11.3 mg/kg with the application of compost. Zhang et al. (2017) found that organic amendments, including FYM, reduced nickel concentrations in soils by approximately 20%. The results of this study (Figure 3) are in agreement with those of Clemente et al. (2006) and Zhang et al. (2017), further supporting the role of organic amendments in nickel reduction. Peryea and Kammereck (2001) reported a reduction in arsenic concentration in contaminated soils from 5.0 mg/kg to 3.0 mg/kg with the application of organic matter. Chirenje et al. (2003) stated that the use of compost reduced arsenic concentrations in soils by up to 50%. The arsenic reduction observed in this study (Figure 3) is comparable to findings obtained by Peryea and Kammereck (2001) and Chirenje et al. (2003), highlighting the efficacy of FYM and compost in mitigating arsenic contamination. This comparison demonstrates the consistency of the findings of the current study with the broader body of research, reinforcing the effectiveness of FYM and compost in reducing heavy metal concentrations in soils.
Incorporating soil additives into agricultural practices has the potential to transform the industry by optimizing crop yields and ensuring environmental sustainability. Poor soil fertility, salinity, low organic matter, and limited availability of sulfur and trace elements hinder oilseed production. Therefore, it is important to combine soil improvement with management strategies to increase soil productivity and yield (Rashmi et al., 2022). Samadi et al. (2024) found that drought stress negatively impacted maize growth. However, both sulfate of potash (SOP) and biofertilizers can increase maize yield, with SOP having a stronger effect. Interestingly, the effect of biofertilizers on shoot and root weight was more pronounced under drought stress than that of SOP.
Organic amendments such as organic fertilizers, composts, biosolids, biochar, and biofertilizers, and inorganic amendments such as gypsum, pyrite, lime, and zeolites can be used to increase crop yields (Sindesi et al., 2023). These soil amendments not only provide essential nutrients, but also add organic matter to improve the physical, chemical, and biological properties of the soil. In agroecosystems, increasing the soil organic carbon (SOC) plays an important role in mitigation, adaptation, and food security (Brown et al., 2019). An ongoing field trial in Switzerland showed the effect of various organic fertilizers, such as green manure, cereal straw, agricultural manure, and cow manure, on the accumulation and distribution of SOC in different soil fractions (Anderson et al., 2022). Novair et al. (2024) determined that the application of organic amendments is one way to manage low-water irrigation in paddy soils. However, soil amendments are in line with circular economy principles and are valuable resources for agriculture. Before applying soil fertilizers to oilseeds, a thorough analysis should be performed to assess the potential, limitations, and impact of soil fertilizers on soil health and crop quality. Local soil amendments such as organic fertilizers, composts, green manures, plant residues, and biofertilizers are recommended to improve soil health and yield production in a sustainable way (Rashmi et al., 2022).
In another study, Hong et al. (2020) carried out research on the elimination of heavy metal pollution in soils by chemical additives and their effects on the antioxidant activity of lettuce and soil enzyme activity. The aim of the study was to understand how chemical tweaks can reduce heavy metal pollution and assess their effects on plant and soil health. Similarly, Taeprayoon et al. (2022) focused on the application of organic fertilizers in cadmium-contaminated soils for the phytoremediation of three bioenergy crops. Their study examined the effectiveness of organic additives in stabilizing cadmium concentrations and stimulating the growth of bioenergy crops in contaminated soils. Nayeri et al. (2023) claimed that phytoremediation is a promising remediation technology that is a green, sustainable, and cost-effective strategy to bio-remediate pollutants, especially heavy metals from natural soils, and water resources.
Wang et al. (2020) studied the effect of soil fertilizers on the fixation and accumulation of heavy metals in maize grown in multi-metal-contaminated soils. The objective of this research was to assess the ability of soil fertilizers to lessen the bioavailability of heavy metals and ensure safe crop production. Van-Zwieten (2018) discussed the enduring role of organic matter in eliminating soil problems for sustainable crop production. This study highlights the importance of using organic fertilizers to improve soil value and fertility to ensure sustainable crop productivity.
Conclusion
The application of organic amendments like farmyard manure and compost not only immobilized the heavy metals in the soil profile but also improved the biomass of the maize crop. However, the maximum potential of farmyard manure was observed to immobilize the heavy metals in the soil profile when it was applied at a rate of 5 tons ha-1. Among the organic amendments, the application of farmyard manure proved superior and more effective regarding soil health, as its addition reduced the concentration of heavy metals (lead, cadmium, nickel, and arsenic) in soil samples compared with compost application. It is suggested that farmers, particularly those cultivating land on the periphery of industrial cities, can use organic amendments to minimize the toxic impact of wastewater when used as irrigation water. The main benefit of using farmyard manure as an organic amendment is that it immobilizes heavy metals in the soil profile, reducing their concentration and mitigating their toxic impact on soil health and crop growth. Additionally, it improves soil characteristics and increases crop biomass, making it an effective and sustainable solution for managing heavy metal pollution in industrial crop cultivation.
Data availability statement
The original contributions presented in the study are included in the article/Supplementary Material; further inquiries can be directed to the corresponding author.
Author contributions
MZM: conceptualization, data curation, formal analysis, methodology, resources, software, visualization, writing–original draft, and writing–review and editing. GS: conceptualization, investigation, project administration, supervision, validation, visualization, and writing–review and editing. MI: conceptualization, project administration, resources, supervision, visualization, and writing–review and editing. SSR: conceptualization, formal analysis, methodology, software, visualization, writing–original draft, and writing–review and editing. ZH: data curation, methodology, supervision, and writing–review and editing. AR: data curation, formal analysis, methodology, project administration, supervision, and writing–original draft. SG: conceptualization, data curation, investigation, methodology, project administration, validation, and writing–review and editing. ATA: resources, software, data analysis, writing–review and editing. BOM: conceptualization, methodology, resources, software, visualization, writing–original draft, and writing–review and editing. KM: conceptualization, data curation, resources, formal analysis, software, writing–original draft, and writing–review and editing. SK: formal analysis, methodology, resources, software, supervision, writing–original draft, and writing–review and editing.
Funding
The authors declare that no financial support was received for the research, authorship, and/or publication of this article.
Acknowledgments
The authors would like to extend their sincere appreciation to the Researchers Supporting Project, number (RSPD2024R558), King Saud University, Riyadh, Saudi Arabia.
Conflict of interest
Author BM was employed by USDA-NRCS Canon City Field Office, Canon.
The remaining authors declare that the research was conducted in the absence of any commercial or financial relationships that could be construed as a potential conflict of interest.
Publisher’s note
All claims expressed in this article are solely those of the authors and do not necessarily represent those of their affiliated organizations, or those of the publisher, the editors, and the reviewers. Any product that may be evaluated in this article, or claim that may be made by its manufacturer, is not guaranteed or endorsed by the publisher.
References
Abd-El-Fattah, D. A., Hashem, F. A., and Abd-Elrahman, S. H. (2022). Impact of applying organic fertilizers on nutrient content of soil and lettuce plants, yield quality, and benefit-cost ratio under water stress conditions. Asian J. Agric. Biol. 2022 (2), 202102086. doi:10.35495/ajab.2021.02.086
Adams, J. M., Smith, B., and Johnson, K. L. (2021). Sustainable agricultural development: challenges and opportunities. J. Sustain. Agric. 455, 472–490.
Ahmad, M., Lee, S. S., Lee, S. E., Al-Wabel, M. I., Tsang, D. C., and Ok, Y. S. (2017). Biochar-induced changes in soil properties affected the immobilization/mobilization of metals/metalloids in contaminated soils. J. soils sediments 17, 717–730. doi:10.1007/s11368-015-1339-4
Alam, M., Hussain, Z., Khan, A., Khan, M. A., Rab, A., Asif, M., et al. (2020). The effects of organic amendments on heavy metals bioavailability in mine impacted soil and associated human health risk. Sci. Hortic. 262, 109067. doi:10.1016/j.scienta.2019.109067
Anderson, A., Anbarasu, A., Pasupuleti, R. R., Manigandan, S., Praveenkumar, T. R., and Kumar, J. A. (2022). Treatment of heavy metals containing wastewater using biodegradable adsorbents: a review of mechanism and future trends. Chemosphere 295, 133724. doi:10.1016/j.chemosphere.2022.133724
Bartlett, M. S. (1937). The statistical conception of mental factors. Br. J. Psychol. 281, 97–104. doi:10.1111/j.2044-8295.1937.tb00863.x
Bolan, N., Adriano, D., Mani, S., and Khan, A. (2003). Adsorption, complexation, and phytoavailability of copper as influenced by organic manure. Environ. Toxicol. Chem. 222, 450–456. doi:10.1002/etc.5620220228
Bolan, N. S., Adriano, D. C., and Naidu, R. (2010). Impact of organic amendments on the reduction of cadmium uptake by plants. Environ. Sci. Tech. 44 (1), 15–23.
Briffa, J., Sinagra, E., and Blundell, R. (2020). Heavy metal pollution in the environment and their toxicological effects on humans. Heliyon 6, e04691. doi:10.1016/j.heliyon.2020.e04691
Brown, D. R., Johnston, S. G., Santos, I. R., Holloway, C. J., and Sanders, C. J. (2019). Significant organic carbon accumulation in two coastal acid sulfate soil wetlands. Geophys. Res. Lett. 466, 3245–3251. doi:10.1029/2019GL082076
Brown, R. T., Thompson, W. R., and Johnson, A. S. (2022). Water scarcity and food security: global challenges for sustainable agriculture. Food secur. 142, 267–285.
Bui, V. H., Nguyen, H. C., and Ngo, Q. H. (2023). Establishment of rice yield prediction model using soil compaction. Asian J. Agric. Biol. 2023 (2), 202109327. doi:10.35495/ajab.2021.09.327
Chen, Y., Camps-Arbestain, M., Shen, Q., Singh, B., and Cayuela, M. L. (2018). The long-term role of organic amendments in building soil nutrient fertility: a meta-analysis and review. Nutr. Cycl. Agroecosyst. 111, 103–125. doi:10.1007/s10705-017-9903-5
Chirenje, T., Ma, L. Q., and Reeves, M. (2003). Arsenic sorption by organic matter. Environ. Sc. Tech. 37 (20), 4951–4957.
Clemente, R., Escolar, A., and Bernal, M. P. (2006). Heavy metals in soils amended with composts. Chemo 64 (6), 1003–1009.
Eaton, E. M. (1950). Significance of carbonate in irrigation water. Soil Sci. 69, 123–133. doi:10.1097/00010694-195002000-00004
Garcia, D. L., Gomez, R. M., and White, C. J. (2020). Managing water scarcity: an assessment of alternative water sources for agriculture. Environ. Res. Lett. 152, 024006.
Ghous, M., Iqbal, S., Bakhtavar, M. A., Nawaz, F., Haq, T. U., and Khan, S. (2022). Halophyte quinoa: a potential hyperaccumulator of heavy metals for phytoremediation. Asian J. Agric. Biol. 2022 (4), 2021444. doi:10.35495/ajab.2021.444
Gill, R. T., Harbottle, M. J., Smith, J. W. N., and Thornton, S. F. (2014). Electrokinetic-enhanced bioremediation of organic contaminants: a review of processes and environmental applications. Chemosphere 107, 31–42. doi:10.1016/j.chemosphere.2014.03.019
Gomez, R. M., Johnson, A. S., and Thompson, W. R. (2021). Enhancing water use efficiency in agriculture: challenges and strategies. Environ. Manag. 291, 112623.
Goss, M. J., Tubeileh, A., and Goorahoo, D. (2013). A review of the use of organic amendments and the risk to human health. Adv. Agron. 120, 275–379. doi:10.1016/b978-0-12-407686-0.00005-1
Gupta, S. K., Ansari, F. A., Nasr, M., Chabukdhara, M., and Bux, F. (2018). Multivariate analysis and health risk assessment of heavy metal contents in foodstuffs of Durban, South Africa. Environ. Monit. Assess. 190, 151. doi:10.1007/s10661-018-6546-1
Hong, Y. K., Kim, J. W., Lee, S. P., Yang, J. E., and Kim, S. C. (2020). Heavy metal remediation in soil with chemical amendments and its impact on activity of antioxidant enzymes in Lettuce (Lactuca sativa) and soil enzymes. Appl. Biol. Chem. 631, 42–10. doi:10.1186/s13765-020-00526-w
Houben, D., Pircar, J., and Sonnet, P. (2012). Heavy metal immobilization by cost-effective amendments in a contaminated soil: effects on metal leaching and phytoavailability. J. Geochem. Explor. 123, 87–94. doi:10.1016/j.gexplo.2011.10.004
Huang, M., Zhu, Y., Li, Z., Huang, B., Luo, N., Liu, C., et al. (2016). Compost as a soil amendment to remediate heavy metal-contaminated agricultural soil: mechanisms, efficacy, problems, and strategies. Water Air Soil Pollut. 227, 359. doi:10.1007/s11270-016-3068-8
Huang X, X., Chen, Z., and Chen, Y. (2016). Impact of organic amendments on the bioavailability of heavy metals in soil. J. Hazar. Mater. 302, 269–276.
Hussain, I., Raschid, L., Hanjra, M. A., Marikar, F., and van der Hoek, W. (2002). Wastewater use in agriculture: review of impacts and methodological issues in valuing impacts. Colombo, Sri Lanka: International Water Management Institute, 37.
Hussan, M. U., Saleem, M. F., Hafeez, M. B., Khan, S., Hussain, S., Ahmad, N., et al. (2022). Impact of soil applied humic acid, zinc and boron supplementation on the growth, yield and zinc translocation in wheat. Asian J. Agric. Biol. (1), 202102080. doi:10.35495/ajab.2021.02.080
Johnson, K. L., and Thompson, W. R. (2019). Urban wastewater as a resource for agricultural irrigation: challenges and opportunities. Water Resour. Manag. 333, 1153–1169.
Khan, S., Afzal, M., Iqbal, S., and Khan, Q. M. (2013). Plant–bacteria partnerships for the remediation of hydrocarbon contaminated soils. Chemo 90 (4), 1317–1332. doi:10.1016/j.chemosphere.2012.09.045
Koishi, A., Bragazza, L., Maltas, A., Guillaume, T., and Sinaj, S. (2020). Long-term effects of organic amendments on soil organic matter quantity and quality in conventional cropping systems in Switzerland. Agron 1012, 1977. doi:10.3390/agronomy10121977
Kwiatkowska-Malina, J. (2018). Functions of organic matter in polluted soils: the effect of organic amendments on phytoavailability of heavy metals. Appl. Soil Ecol. 123, 542–545. doi:10.1016/j.apsoil.2017.06.021
Lee, J., Adams, J. M., and Garcia, D. L. (2019). Assessing the impacts of wastewater irrigation on soil and plant elemental composition: a review. Agric. Water Manag. 214, 317–327.
Moreno-Maroto, J. M., and Alonso-Azcarate, J. (2022). Evaluation of the USDA soil texture triangle through Atterberg limits and an alternative classification system. Appl. Clay Sci. 229, 106689. doi:10.1016/j.clay.2022.106689
Nayeri, S., Dehghanian, Z., Lajayer, B. A., Thomson, A., Astatkie, T., and Price, G. W. (2023). CRISPR/Cas9-Mediated genetically edited ornamental and aromatic plants: a promising technology in phytoremediation of heavy metals. J. Clean. Prod. 428, 139512. doi:10.1016/j.jclepro.2023.139512
Novair, S. B., Hosseini, H. M., Etesami, H., Pirmoradian, N., Lajayer, B. A., and Price, G. W. (2024). Straw application improved soil biological properties and the growth of rice plant under low water irrigation. Environ. Res. 255, 119138. doi:10.1016/j.envres.2024.119138
Nte, N. J., Sunday, I. T., Solomon, A. O., Onyeogoziri, E. C., Uguru, N. S., Nwangele, M. B., et al. (2022). Response of groundnut to lime sources on acid sandy loam. Asian J. Agric. Biol. (3). doi:10.35495/ajab.2020.08.452
O’Sullivan, M. (2011). “Analytical methods: proximate and other chemical analyses,” in Encyclopedia of dairy sciences. Editor J. W. Fuquay Second Edition (Elsevier Ltd). doi:10.1016/B978-0-12-374407-4.00517-3
Pangaribuan, D. H., Widagdo, S., Hariri, A. M., Siregar, S., and Sardio, M. I. (2023). The effect of rice straw mulch and cow urine on growth, yield, quality on sweet corn and pest population density. Asian J. Agric. Biol. 2023 (1), 202103123. doi:10.35495/ajab.2021.03.123
Peryea, F. J., and Kammereck, R. (2001). Phytoremediation of arsenic-contaminated soils: the role of compost and organic matter. J. Environ. Qual. 30 (4), 1124–1131.
Rani, N., and Singh, M. (2022). “Remediation of soil impacted by heavy metal using farm yard manure, vermicompost, biochar and poultry manure,” in Soil science - emerging technologies, global perspectives and applications. Editors M. Aide, and I. Braden doi:10.5772/intechopen.105536
Rashmi, I., Kumawat, A., Munawery, A., Karthika, K. S., Sharma, G. K., Kala, S., et al. (2022). “Soil amendments: an ecofriendly approach for soil health improvement and sustainable oilseed production,” in Oilseed crops-uses, biology and production. Editors M. Hasanuzzaman, and K. Nahar (London, United Kingdom: IntechOpen). doi:10.5772/intechopen.106606
Rizwan, M., Ahmed, K., Bhatti, M. K., Sarfraz, M., Irfan, M., Hussain, S. S., et al. (2022). Effect of sowing techniques and tillage practices on paddy yield of direct seeded rice in salt affected soils. Asian J. Agric. Biol. 2022 (1), 202101043. doi:10.35495/ajab.2021.01.043
Samadi, A., Derafshi, M., Hassani, A., Gholamhoseini, M., Asgari Lajayer, B., Astatkie, T., et al. (2024). Effects of biofertilizers and potassium sulfate on nutrients uptake and physiological characteristics of maize (Zea mays L.) under drought stress. J. Crop Health 76 (1), 209–218. doi:10.1007/s10343-023-00954-w
Sarwar, G. (2005). Use of compost for crop production in Pakistan. Kassel, Germany: Ökologie und Umweltsicherung - Universität. Ph.D. Dissertation.
Shaheen, S. M., Hooda, P. S., and Tsadilas, C. D. (2014). Opportunities and challenges in the use of coal fly ash for soil improvements–a review. Environ. Manag. 145, 249–267. doi:10.1016/j.jenvman.2014.07.005
Shaheen, S. M., and Rinklebe, J. (2015). Impact of emerging and low-cost alternative amendments on the (im) mobilization and phytoavailability of Cd and Pb in a contaminated floodplain soil. Ecol. Eng. 74, 319–326. doi:10.1016/j.ecoleng.2014.10.024
Sharma, B., Vaish, B., Monika, , Singh, U. K., Singh, P., and Singh, R. P. (2019). Recycling of organic wastes in agriculture: an environmental perspective. Int. J. Environ. Res. 13, 409–429. doi:10.1007/s41742-019-00175-y
Sindesi, O. A., Ncube, B., Lewu, M. N., Mulidzi, A. R., and Lewu, F. B. (2023). Cabbage and Swiss chard yield, irrigation requirement and soil chemical responses in zeolite-amended sandy soil. Asian J. Agric. Biol. 2023 (1), 202111387. doi:10.35495/ajab.2021.11.387
Smith, B., Brown, R. T., and Adams, J. M. (2018). Water scarcity and agriculture: a review. Water 108, 966.
Steel, R. G. D., Torrie, J. H., and Dickey, D. A. (1997). Principles and procedures of statistics. A biometrd rical approach. 3rd edition. Boston. Massachusetts: McGrawHill Publishing Co, 635.
Taeprayoon, P., Homyog, K., and Meeinkuirt, W. (2022). Organic amendment additions to cadmium-contaminated soils for phytostabilization of three bioenergy crops. Sci. Rep. 121, 13070. doi:10.1038/s41598-022-17385-8
U.S. Salinity Laboratory Staff (1969). Diagnosis and Improvements of saline and alkali soils. Washington, DC, USA: USDA. U.S. Govt. Printing Office. Handbook No. 60.
Van-Zwieten, L. (2018). The long-term role of organic amendments in addressing soil constraints to production. Nutr. Cycl. Agroecosyst 11123, 99–102. doi:10.1007/s10705-018-9934-6
Walkley, A., and Black, I. A. (1934). An examination of the Degtjareff method for determining soil organic matter, and a proposed modification of the chromic acid titration method. Soil Sci. 371, 29–38. doi:10.1097/00010694-193401000-00003
Wang, F., Zhang, S., Cheng, P., Zhang, S., and Sun, Y. (2020). Effects of soil amendments on heavy metal immobilization and accumulation by maize grown in a multiple-metal-contaminated soil and their potential for safe crop production. Toxics 84, 102. doi:10.3390/toxics8040102
White, C. J., Gomez, R. M., and Johnson, A. S. (2017). Impacts of urbanization on water scarcity in arid and semi-arid regions: a review. J. Hydrol. 553, 890–908.
Yasir, T. A., Aslam, S., Rizwan, M. S., Wasaya, A., Ateeq, M., Khan, M. N., et al. (2022). Role of organic amendments to mitigate Cd toxicity and its assimilation in Triticum aestivum L. Phyton 9111, 2491. doi:10.32604/phyton.2022.022473
Zhang, X., Zhang, S., and Wang, L. (2017). Application of organic amendments to reduce soil pollution. Environ. Manag. 203, 156–164.
Keywords: arsenic, cadmium, compost, farmyard manure, lead, nickel, polluted water
Citation: Zeeshan Manzoor M, Sarwar G, Ibrahim M, Rehan SS, Hasnain Z, Rais A, Gul S, Alfagham AT, Manono BO, Mehmood K and Khan S (2024) Remediation quantum of organic amendments to immobilize potentially toxic heavy metals in wastewater-contaminated soils through maize cultivation. Front. Environ. Sci. 12:1420705. doi: 10.3389/fenvs.2024.1420705
Received: 21 April 2024; Accepted: 17 September 2024;
Published: 09 October 2024.
Edited by:
Rubén Forján, University of Oviedo, SpainReviewed by:
Rakhwe Kama, South China Agricultural University, ChinaBehnam Asgari Lajayer, Dalhousie University, Canada
Copyright © 2024 Zeeshan Manzoor, Sarwar, Ibrahim, Rehan, Hasnain, Rais, Gul, Alfagham, Manono, Mehmood and Khan. This is an open-access article distributed under the terms of the Creative Commons Attribution License (CC BY). The use, distribution or reproduction in other forums is permitted, provided the original author(s) and the copyright owner(s) are credited and that the original publication in this journal is cited, in accordance with accepted academic practice. No use, distribution or reproduction is permitted which does not comply with these terms.
*Correspondence: Shahbaz Khan, c2hhaGJhei5raGFuQGNvbG9zdGF0ZS5lZHU=