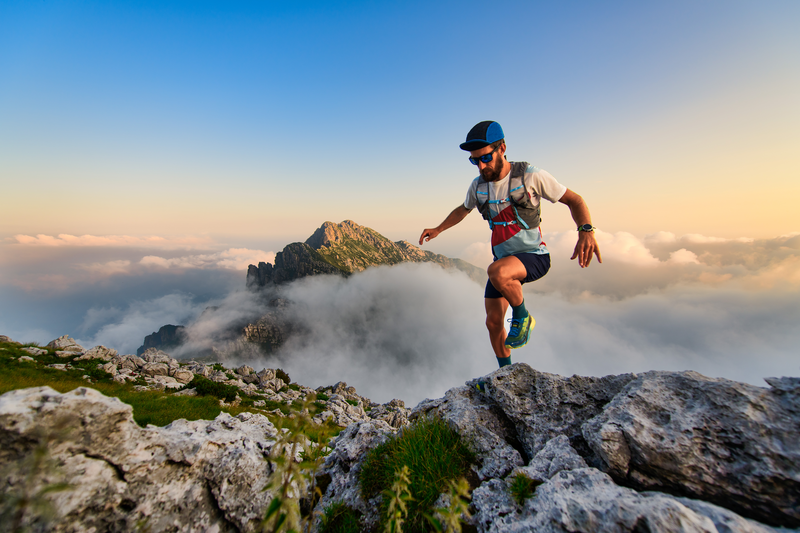
95% of researchers rate our articles as excellent or good
Learn more about the work of our research integrity team to safeguard the quality of each article we publish.
Find out more
MINI REVIEW article
Front. Environ. Sci. , 24 July 2024
Sec. Freshwater Science
Volume 12 - 2024 | https://doi.org/10.3389/fenvs.2024.1410821
This article is part of the Research Topic Freshwater Biodiversity Crisis: Multidisciplinary Approaches as Tools for Conservation Volume II View all 10 articles
Human-induced environmental changes, including climate change and pollution, significantly affect host-parasite interactions, potentially altering the geographical spread and severity of various parasitic diseases. These changes may particularly influence the dynamics of diseases like schistosomiasis, posing significant public health concerns. This review explores how pollutants such as organophosphate pesticides, antibiotics, heavy metals, cities’ landfills, and microplastics can affect the development and transmission dynamics of parasites, especially Schistosoma spp. Our researches highlight that pesticides promote parasitic disease development, while pharmaceuticals have mixed effects on the life cycles of these parasites. Similarly, heavy metals found in water systems disrupt host-pathogen interactions, and microplastics are linked to significant changes in snail stressor genes, a critical intermediate host for several parasites. With the rising impacts of anthropogenic activity on the environment, there is an urgent need to reassess and adjust regulatory policies to minimize these threats. By studying the implications of pollution on host-parasite interactions, we can develop better strategies for disease control and improve the preservation of our ecosystem’s health.
Freshwater ecosystems, including lakes, rivers, streams, wetlands, and ponds play a crucial role in regulating biodiversity, water quality, nutrient cycling, and mitigating the impacts of droughts providing crucial ecosystem services to humans (Gray et al., 2022). These ecosystems are worldwide distributed and figure among the most diverse habitats on the planet while dealing with faster degradation than terrestrial ones (Turak et al., 2017). Freshwater species populations have experienced a staggering decline of 84% since 1970 (McRae et al., 2020), mainly as a consequence of water contamination by human activities (Aryal et al., 2020; Albert et al., 2021). Four main sources of chemical contamination can be identified: Firstly, agricultural contamination via the use of pesticides to control pests, the use of which is regulated, however, these substances can persist and run-off, contaminating the environment for long periods (Syafrudin et al., 2021). Secondly, industrial chemicals, including micro- and nano-plastics, heavy metals, and organic compounds used on production lines. Thirdly, chemicals used in the health sector, such as antibiotics, antidepressants, and endocrine disruptors, among others, are found in trace amounts in the environment. Finally, the full range of substances that can be detected in cities, including organic contaminants, microplastics, or products contained in cigarette butts. Depending on the conditions and the substance in question, they can contaminate several links in the trophic chain and their persistence in the environment depends on physico-chemicals factors (Chevalier et al., 2016; Tudi et al., 2021).
The disruption of ecosystem balance as a consequence of the exacerbation of climate crisis intensify the situation further. Rising temperatures associated to unbalance water cycle have serious consequences, not only for natural ecosystems but also for agriculture, industry and health sector, as they lead to more frequent, severe, and prolonged extreme events (VijayaVenkataRaman et al., 2012). Stratification, water levels, and flow regimes are all significantly impacted with broad-ranging impacts on biodiversity (Birk et al., 2020; Sures et al., 2023).
The emergence of freshwater zoonotic diseases, infectious maladies transmissible between animals and humans, has witnessed a notable increase. Approximately 60% of established infectious diseases and up to 75% of novel or emerging infectious diseases are estimated to have zoonotic origins, either transmitted or not transmitted by freshwater (Salyer et al., 2017). The dynamic interactions among humans, animals, and pathogens cohabiting the same environment align with a “One Health” approach, emphasizing interconnections between human, animal, and environmental health—a pivotal aspect of disease control and prevention strategies (Kahn, 2011). This concept is based on the sophisticated principle that protecting human health involves protecting the health of animals and their interactions with the environment, and is driven by three main objectives; vigilance against infectious diseases, combat resistance to anti-infectives, and recognize the disruptions in our environment that are likely to encourage the emergence of new diseases (Destoumieux-Garzón et al., 2018; Parodi, 2021). Freshwater-snail-borne parasites, such as those causing schistosomiasis, play instrumental roles in species biodiversity contributing to the dynamics of natural ecosystems (Sures et al., 2023). It strives to comprehend how environmental conditions, freshwater pollution between them, impact parasite life cycles, and their evolution, including their effects on host behaviour, physiology, and population dynamics, as well as their influence on food webs and ecosystem functioning (Nachev and Sures, 2016; Sures et al., 2017a). By unravelling the environmental factors that influence parasites spreading, it is possible to develop strategies for disease prevention, control, and management. However, new approaches are needed to counter the progression of freshwater diseases, which are on the rise in Europe (Levy et al., 2018; Semenza et al., 2022). Although some studies have examined the impact of pollution on vector biology, further research is required to understand its effect on the pathogens themselves. Parasites, such as schistosomes, pose an enduring threat to organisms across various phyla, with their virulence defined as the impact on their host’s fitness, being shaped by host-parasite combinations and environmental conditions. In this intricate interplay, biological and chemical features act as drivers emerging as a potential factor affecting parasite virulence, a still neglected aspect of water pollution effect (Augusto et al., 2019). Systems biology approaches, combining functional genomics, offer promising methods to address challenges by elucidating the molecular mechanisms underlying host-pathogen interactions in changing environmental scenarios. A key area of research in functional genomics is the use of CRISPR-based technology to edit vector host or pathogen genomes, potentially leading to more effective treatment and prevention strategies for infectious diseases. Despite the difficulties and uncertainties associated with using CRISPR-based methods in mosquito-borne malaria models, it is recognized that there is a need for further technological advancements in vector hosts or their associated parasites in the context of freshwater-related diseases (Tajudeen et al., 2023).
This review elucidates the influence of freshwater quality and its potential ramifications on the spread of waterborne diseases in Europe. Concentrating on Schistosomiasis, a parasitic ailment traditionally confined to Africa, Asia, and Central/South America, but recently observed in France (Corsica), we explored the repercussions of freshwater pollution on the snail-parasite relationship that potentially impacts disease dissemination within Europe.
Schistosomiasis or bilharziasis is the world’s second most common parasitic disease. This parasitic anthropozoonosis disease is endemic to sub-Saharan Africa, Central and South America, and Asia which recently spread to countries in temperate zones as a result of migratory movements, globalisation, and tourism (Tchouanguem et al., 2016). Currently, this disease is progressing in Europe and has already been endemic in Corsica for several years, responsible for multiple contaminations every year (Berry et al., 2016; Kincaid-Smith et al., 2017; Gabrielli and Garba Djirmay 2023). Several species of parasites are responsible for this disease, two of which are the majority in terms of infection, but they differ in their intermediate hosts. First of all, Schistosoma mansoni, (Silva, 1908), transmitted by the snail Biomphalaria spp., (Say, 1818) is mainly studied because it is widespread in Africa and present in Latin America. Secondly, Schistosoma haematobium (Bilharz, 1852), having the snail Bulinus spp. (Müller OF Geschichte der Perlen-Blasen) as a vector, which is mainly present in sub-Saharan Africa and that tends much more to migrate towards Europe, already present in Corsica, Spain, Portugal, Greece, and Italy (Gabrielli and Garba Djirmay 2023). These two parasites mainly have humans as definitive hosts but can also affect other mammals which may also contribute to human infections (McManus et al., 2018; Liang et al., 2022). In humans, Schistosomiasis can cause numerous symptoms, infecting the intestine, liver, kidneys, and genitals, causing inflammation in both men and women and even death (McManus et al., 2018). According to the World Health Organization (WHO), in 2021, at least 251.4 million people needed preventive treatment against Schistosomiasis, but only a fifth received it, demonstrating that new measures need to be put in place to counter the advance of this disease and reduce the number of deaths (Kokaliaris et al., 2022). Currently, the fight against schistosomiasis aims to reduce the number of sufferers through the development of new treatments and improved sanitary conditions, which only serve to reduce transmission (Kokaliaris et al., 2022).
This conjuncture will only intensify in the years to come, with the amplification of climate change which promotes the transmission and spatio-temporal distribution of parasites by affecting their reproduction, their duration of maturation, and the incubation period of which they need to become their preferred vector (Semenza et al., 2022). The increase in migratory flows contribute also to extending the geographical distribution of schistosomiasis (Aagaard-Hansen et al., 2010) underlining the need to find solutions to curb this parasitic disease. Moreover, warmer climates are also associated with faster disease transmission as well as the persistence of more virulent parasitic strains (Altizer et al., 2013; Cable et al., 2017). In addition, genetic mixtures between species of schistosomiasis can also take place, producing hybrids like those found in Corsica (Noël et al., 2018). These hybridization phenomena promote contamination or extend the range of hosts that can be affected, which further complicates the determination of sources and the methods of action to fight against epidemics (Gabrielli and Garba Djirmay 2023). All of these factors make this disease, no longer tropical but global, a major public and veterinary health problem (Berger et al., 2022).
The situation is all the more alarming from the point of view of aquatic ecosystems, which represent important poles of biodiversity (Gray et al., 2022). As the life cycle of these parasites is closely linked to aquatic environments, their state under the effect of climate change is central to understanding the evolution of this disease and finding solutions. By their very nature, freshwater ecosystems are subject to many fluctuations in physico-chemical parameters (water availability, temperature, salinity, and pH), which affect both the parasite and its host population dynamics (Woodward et al., 2010). Anthropogenic modifications to these ecosystems add a new level of complexity, affecting these populations in deleterious or healthy ways, mainly through the expansion of agriculture, which leads to the contamination of these environments (Prajapati et al., 2022). Indeed, under the effect of higher temperatures, the parasitic life cycle could be affected at different layers, the populations of snails or cercariae can thus increase in numbers but the lifespan of the snails can be found reduced, also reducing the period of release of the cercariae. In addition, the presence of fertilisers and pesticides facilitates the development of food resources, such as algae (periphyton) as well as the development of intermediate hosts (Douchet et al., 2023) and could also increase parasites’ virulence (Mennerat et al., 2010). Other factors are more deleterious in the overall dynamics of transmission, such as predation or competition (Douchet et al., 2023). Faced with all these factors, the host’s metabolism and immune system are decisive in the response to infection: tolerance, resistance, or susceptibility (Downs et al., 2019). Despite these numerous findings, it is necessary to consider the net effects on the entire life cycle of the parasite as well as the entire ecosystem to glimpse the links between climate change and the development of parasitic diseases within aquatic ecosystems (Marcogliese et al., 2015).
Numerous studies have shown that the presence of chemical compounds in the environment can also have synergistic or antagonistic effects on parasite development (Table 1). Organophosphate pesticides have an impact on the development of parasitic diseases, either by promoting or hindering their development cycle, as in the case of schistosomiasis (Sures et al., 2017b). Indeed, it has been shown that Esfenvalerate, authorised in Europe, can promote the disease by reducing snail predators, thus favouring their biomass, or can negatively impact snail-susceptible competitors such as Macrobrachium shrimp species, thus increasing their abundance and the rate of trematode transmission (Haggerty et al., 2023). There is also evidence that Glyphosate, also authorised in Europe, reduces transmission by affecting snail reproduction (Hoover et al., 2020). On the other hand, the pesticides Atrazine, Chlorpyrifos and Profenofos, present in ecosystems even though they are not authorised in Europe, have demonstrated an impact on the dynamics of algae, affected by these substances, thus favouring snail populations and amplifying transmission. At the same time, predator mortality is increased, which also favours transmission (Hoover et al., 2020). Snail hosts have also been shown to have a high tolerance to even high concentrations of pesticides, which, combined with the effects of these substances on predators and the food reservoir of this intermediate host, further increases the risk of disease transmission (Ganatra et al., 2023).
These effects are likely to be amplified by the eutrophication of environments due to the migration of nutrients from crops to water (increase in population densities, land occupation), which is constantly growing and leading to increased exposure to trematode cercariae (larval forms that infect the definitive host). However, these observations remain highly context-dependent (type and concentration of pesticide, distance from agriculture, population status, predation, climatic factors, etc.). Besides the effect of pesticides on the host-parasite relationship, there are other chemical contaminants due to anthropogenic activity, such as pollution from health facilities, cities and industry that could also impact it. Pollution related to human health is commonly linked to pharmaceuticals and personal care products (PPCPs), hormones, and endocrine disruptors released into the environment. One of the components of PPCPs easily found in freshwater is antibiotic residues (Danner et al., 2019). A recent study showed the influence of antibiotics on parasite dynamics. This study explored the effect of tetracycline, a common broad-spectrum antibiotic, on the host-parasite life cycle of the parasite S. mansoni and its intermediate host, Bi. glabrata. They studied the impact of an ecologically relevant low-dose concentration on the host and parasite. Hosts affected by tetracycline contamination showed an increase in parasite production as the infection developed. Subsequently, the presence of antibiotics increased egg-laying in snails, potentially making new hosts for the parasite (Melchiorre et al., 2023). Another study showed the impact of polyhexamethylene biguanide hydrochloride (PHMB) used as a disinfectant and antiseptic, capable of inhibiting the embryonic hatching of Bi. glabrata (de Oliveira Melo et al., 2019; de Oliveira Melo et al., 2019). Another study demonstrates the harmful effects of antiviral drugs that are often released into the environment. Lamivudine and stavudine were found to have a stimulatory effect on Bu. tropicus embryonic development, producing mean embryo lengths greater than controls. In contrast, efavirenz and nevirapine showed an overall inhibitory effect on embryonic growth. Antiretroviral exposure to Bu. tropicus affected embryo length, hatching and mortality. The influence of these antiretrovirals on the development and growth of the snail vector confirms the need for further studies into the ecological impact of these pharmaceutical compounds (Bouwman et al., 2020).
Meanwhile, cities represent a substantial part of global pollution with residential and industrial processes. First, polycyclic aromatic hydrocarbons (PAHs) from organic material combustion or discarded cigarette butts have been detected in elevated levels in snails, including Bu. globosus (Dobaradaran et al., 2020; Ediagbonya et al., 2022). In addition, terrestrial snails Eobania vermiculata (Müller OF 1774) demonstrated the profound effects of high concentrations of pollutants such as PAHs; their findings revealed a statistically significant rise in reactive oxygen species (ROS) production, protein carbonylation, and DNA damage (Itziou et al., 2011). However, no studies about the impact of these contaminants have been performed on aquatic snails such as Bi. glabrata or Bu. truncatus so far.
Moreover, microplastics, found in an average abundance of 28.13 ± 4.18 particles across all snail populations (An et al., 2022), have been linked to notable changes in the behaviour of the aquatic snail, Potamopyrgus antipodarum (Gray, 1843) (Romero-Blanco et al., 2021). Furthermore, studies have demonstrated that the build-up of nanoplastics within Bi. glabrata can trigger changes in the expression of numerous stress response genes. These include heat shock protein-70 (HSP70), cytochrome P450 (CYP450), and macrophage migration inhibitory factor (MIF) (Merrill, 2022). These potentially hazardous compounds, by influencing snails physiology, may also adversely affect the virulence of Schistosoma, thereby posing further ecological risks, further research is needed.
Finally, heavy metals found in polluted water such as cadmium, lead, and mercury are responsible of disbalanced in host-pathogens interaction. It has been shown that cadmium concentration in Bi. alexandrina snails is positively correlated to their infection rate by S. mansoni (El-Gohary et al., 2003; El-Din et al., 2010). Table 1 have also shown that chronic lead exposure of S. mansoni and S. haematobium infected hamsters showed significant reductions in worm burden, tissue egg load, and ova excretion in stool, liver, and intestine while increasing the oxidative stress compared to the non-infected group.
However, the effect of freshwater contamination on parasites has not been well studied. Previous work has revealed that the use of pesticides could not just affect the snail host but also exerts a long-lasting impact on parasite virulence (Mello-Silva et al., 2011; Augusto et al., 2017; 2021). Therefore, the intricate relationship of the abovementioned pollutants on host and parasite outcomes contributes to the need to study the effects of schistosomiasis in different environmental conditions, such as in Europe. At the same time, however, it is imperative to note that in real life, these contaminants do not normally present themselves as pure, as evidenced by laboratory experiments. In natural settings, an acute or latent concentration of a dangerous substance mixture is present in the environment; however, freshwater snails and parasites are also present. Therefore, more research is needed to determine the impact of environmental pollution on the prevalence of schistosomiasis. This type of crosscutting may contain crucial data and optimal solutions for parasitic illnesses due to pollution.
Here, we reviewed how freshwater pollution modifies the host-parasite relationship, significantly impacting snail hosts and the transmission risk of schistosomiasis. However, an often overlooked aspect is the potential effect of pollution on parasite virulence. The virulence of metazoan parasites isn't solely determined by their genetics; environmental factors play a crucial role in controlling virulence gene expression. Specifically, pollution may enhance the expression of these genes, thus influencing the spread of schistosomiasis in Europe. Additionally, studies in realistic environmental settings associated to functional genomic approach are essential to better understand and control the spread of freshwater-borne diseases like schistosomiasis.
AC: Conceptualization, Data curation, Investigation, Writing–original draft, Writing–review and editing. HG: Conceptualization, Data curation, Investigation, Writing–original draft, Writing–review and editing. AS: Conceptualization, Data curation, Investigation, Writing–original draft, Writing–review and editing. MT: Conceptualization, Data curation, Investigation, Writing–original draft, Writing–review and editing. RA: Conceptualization, Data curation, Formal Analysis, Funding acquisition, Investigation, Methodology, Project administration, Resources, Software, Supervision, Validation, Visualization, Writing–original draft, Writing–review and editing.
The author(s) declare that financial support was received for the research, authorship, and/or publication of this article. This study was supported by the École Universitaire de Recherche TULIP-GS (ANR-18-EURE-0019) and ANR (ANR-22-CPJ1-0056-01 - Tropical diseases of today, European diseases of tomorrow: a systems biology approach to understand, predict and control their emergence).
The authors declare that the research was conducted in the absence of any commercial or financial relationships that could be construed as a potential conflict of interest.
All claims expressed in this article are solely those of the authors and do not necessarily represent those of their affiliated organizations, or those of the publisher, the editors and the reviewers. Any product that may be evaluated in this article, or claim that may be made by its manufacturer, is not guaranteed or endorsed by the publisher.
Aagaard-Hansen, J., Nombela, N., and Alvar, J. (2010). Population movement: a key factor in the epidemiology of neglected tropical diseases: population movement and neglected tropical diseases. Trop. Med. Int. Health 15, 1281–1288. doi:10.1111/j.1365-3156.2010.02629.x
Albert, J. S., Destouni, G., Duke-Sylvester, S. M., Magurran, A. E., Oberdorff, T., Reis, R. E., et al. (2021). Scientists’ warning to humanity on the freshwater biodiversity crisis. Ambio 50, 85–94. doi:10.1007/s13280-020-01318-8
Altizer, S., Ostfeld, R. S., Johnson, P. T. J., Kutz, S., and Harvell, C. D. (2013). Climate change and infectious diseases: from evidence to a predictive framework. Science 341, 514–519. doi:10.1126/science.1239401
An, L., Cui, T., Zhang, Y., and Liu, H. (2022). A case study on small-size microplastics in water and snails in an urban river. Sci. Total Environ. 847, 157461. doi:10.1016/j.scitotenv.2022.157461
Aryal, N., Wood, J., Rijal, I., Deng, D., Jha, M. K., and Ofori-Boadu, A. (2020). Fate of environmental pollutants: a review. Water Environ. Res. 92, 1587–1594. doi:10.1002/wer.1404
Augusto, R. D. C., Duval, D., and Grunau, C. (2019). Effects of the environment on developmental plasticity and infection success of schistosoma parasites – an epigenetic perspective. Front. Microbiol. 10, 1475. doi:10.3389/fmicb.2019.01475
Augusto, R. D. C., Rey, O., Cosseau, C., Chaparro, C., Vidal-Dupiol, J., Allienne, J. F., et al. (2021). A simple ATAC-seq protocol for population epigenetics. Wellcome Open Res. 5, 121. doi:10.12688/wellcomeopenres.15552.2
Augusto, R. D. C., Tetreau, G., Chan, P., Walet-Balieu, M. L., Mello-Silva, C. C., Santos, C. P., et al. (2017). Double impact: natural molluscicide for schistosomiasis vector control also impedes development of Schistosoma mansoni cercariae into adult parasites. PLoS Negl. Trop. Dis. 11, e0005789. doi:10.1371/journal.pntd.0005789
Berger, D. J., Léger, E., Sankaranarayanan, G., Sène, M., Diouf, N. D., Rabone, M., et al. (2022). Genomic evidence of contemporary hybridization between Schistosoma species. PLOS Pathog. 18, e1010706. doi:10.1371/journal.ppat.1010706
Berry, A., Fillaux, J., Martin-Blondel, G., Boissier, J., Iriart, X., Marchou, B., et al. (2016). Evidence for a permanent presence of schistosomiasis in Corsica, France, 2015. Eurosurveillance 21. doi:10.2807/1560-7917.ES.2016.21.1.30100
Birk, S., Chapman, D., Carvalho, L., Spears, B. M., Andersen, H. E., Argillier, C., et al. (2020). Impacts of multiple stressors on freshwater biota across spatial scales and ecosystems. Nat. Ecol. Evol. 4, 1060–1068. doi:10.1038/s41559-020-1216-4
Bouwman, H., Bezuidenhout, C., Horn, S., Vogt, T., Bothma, L., Gerber, E., et al. (2020). Quantification, fate and hazard assessment of HIV-ARVs in water resources - chapter 1.5: the presence of pharmaceuticals in water edn. Water Research Commission, South Africa.
Cable, J., Barber, I., Boag, B., Ellison, A. R., Morgan, E. R., Murray, K., et al. (2017). Global change, parasite transmission and disease control: lessons from ecology. Philos. Trans. R. Soc. B Biol. Sci. 372, 20160088. doi:10.1098/rstb.2016.0088
Chevalier, F. D., Le Clec’h, W., Eng, N., Rugel, A. R., Assis, R. R. d., Oliveira, G., et al. (2016). Independent origins of loss-of-function mutations conferring oxamniquine resistance in a Brazilian schistosome population. Int. J. Parasitol. 46, 417–424. doi:10.1016/j.ijpara.2016.03.006
Danner, M.-C., Robertson, A., Behrends, V., and Reiss, J. (2019). Antibiotic pollution in surface fresh waters: occurrence and effects. Sci. Total Environ. 664, 793–804. doi:10.1016/j.scitotenv.2019.01.406
de Oliveira Melo, A., Santos, D. B. dos, Silva, L. D., Rocha, T. L., and Bezerra, J. C. B. (2019). Molluscicidal activity of polyhexamethylene biguanide hydrochloride on the early-life stages and adults of the Biomphalaria glabrata (Say, 1818). Chemosphere 216, 365–371. doi:10.1016/j.chemosphere.2018.10.035
Destoumieux-Garzón, D., Mavingui, P., Boetsch, G., Boissier, J., Darriet, F., Duboz, P., et al. (2018). The one health concept: 10 Years old and a long road ahead. Front. Vet. Sci. 5, 14. doi:10.3389/fvets.2018.00014
Dobaradaran, S., Schmidt, T. C., Lorenzo-Parodi, N., Kaziur-Cegla, W., Jochmann, M. A., Nabipour, I., et al. (2020). Polycyclic aromatic hydrocarbons (PAHs) leachates from cigarette butts into water. Environ. Pollut. 259, 113916. doi:10.1016/j.envpol.2020.113916
Douchet, P., Gourbal, B., Loker, E. S., and Rey, O. (2023). Schistosoma transmission: scaling-up competence from hosts to ecosystems. Trends Parasitol. S1471492223000934, 563–574. doi:10.1016/j.pt.2023.04.001
Downs, C. J., Schoenle, L. A., Han, B. A., Harrison, J. F., and Martin, L. B. (2019). Scaling of host competence. Trends Parasitol. 35, 182–192. doi:10.1016/j.pt.2018.12.002
Ediagbonya, T. F., Oluwafemi, A. P., Ikuesan, F. A., Ogunjobi, J. A., and Odinaka, C. V. (2022). The accumulation of polycyclic aromatic hydrocarbons in snails and soil in Igbekebo, Ondo State, Nigeria. Reg. Stud. Mar. Sci. 56, 102625. doi:10.1016/j.rsma.2022.102625
El-Din, A. T. S., Mohamed, A. M., Mohamed, A. H., El-Hommossany, K. M., and Habib, M. R. (2010). Relationship between some heavy metals and schistosoma mansoni infection rates in Biomphalaria alexandrina snails collected from different Egyptian localities.
El-Gohary, M., Yassin, A.-E., and Shalabya, M. A. (2003). The effect of chronic lead exposure on the course of schistosomiasis in hamsters and the protective effect of the antioxidant preparation ‘Antox. ’ Hum. Exp. Toxicol. 22, 481–490. doi:10.1191/0960327103ht391oa
Gabrielli, A. F., and Garba Djirmay, A. (2023). Schistosomiasis in Europe. Curr. Trop. Med. Rep. 10, 79–87. doi:10.1007/s40475-023-00286-9
Ganatra, A. A., Becker, J. M., Shahid, N., Kaneno, S., Hollert, H., Liess, M., et al. (2023). Agricultural pesticides do not suppress infection of Biomphalaria (Gastropoda) by Schistosoma mansoni (Trematoda). Syst. Biol. doi:10.1101/2023.02.13.528426
Gray, E., Cappelli, G., Gammell, M. P., Roden, C. M., and Lally, H. T. (2022). A review of dystrophic lake and pool habitat in Europe: an Irish perspective. J. Nat. Conserv. 68, 126189. doi:10.1016/j.jnc.2022.126189
Gray, J. E. (1843). Catalogue of the species of Mollusca and their shells, which have hitherto been recorded as found at New Zealand, with the description of some lately discovered species, in Dieffenbach, E. Travels in New Zealand; with contributions to the geography, geology, botany, and natural history of that country 2, 228–265.
Haggerty, C. J. E., Delius, B. K., Jouanard, N., Ndao, P. D., De Leo, G. A., Lund, A. J., et al. (2023). Pyrethroid insecticides pose greater risk than organophosphate insecticides to biocontrol agents for human schistosomiasis. Environ. Pollut. 319, 120952. doi:10.1016/j.envpol.2022.120952
Hoover, C. M., Rumschlag, S. L., Strgar, L., Arakala, A., Gambhir, M., de Leo, G. A., et al. (2020). Effects of agrochemical pollution on schistosomiasis transmission: a systematic review and modelling analysis. Lancet Planet Health 4, e280–e291. doi:10.1016/S2542-5196(20)30105-4
Itziou, A., Kaloyianni, M., and Dimitriadis, V. K. (2011). Effects of organic contaminants in reactive oxygen species, protein carbonylation and DNA damage on digestive gland and haemolymph of land snails. Chemosphere 85, 1101–1107. doi:10.1016/j.chemosphere.2011.07.043
Kahn, L. H. (2011). The need for one health degree programs. Infect. Ecol. Epidemiol. 1, 7919. doi:10.3402/iee.v1i0.7919
Kincaid-Smith, J., Rey, O., Toulza, E., Berry, A., and Boissier, J. (2017). Emerging schistosomiasis in Europe: a need to quantify the risks. Trends Parasitol. 33, 600–609. doi:10.1016/j.pt.2017.04.009
Kokaliaris, C., Garba, A., Matuska, M., Bronzan, R. N., Colley, D. G., Dorkenoo, A. M., et al. (2022). Effect of preventive chemotherapy with praziquantel on schistosomiasis among school-aged children in sub-Saharan Africa: a spatiotemporal modelling study. Lancet Infect. Dis. 22, 136–149. doi:10.1016/S1473-3099(21)00090-6
Levy, K., Smith, S. M., and Carlton, E. J. (2018). Climate change impacts on waterborne diseases: moving toward designing interventions. Curr. Environ. Health Rep. 5, 272–282. doi:10.1007/s40572-018-0199-7
Liang, S., Ponpetch, K., Zhou, Y.-B., Guo, J., Erko, B., Stothard, J. R., et al. (2022). Diagnosis of Schistosoma infection in non-human animal hosts: a systematic review and meta-analysis. PLoS Negl. Trop. Dis. 16, e0010389. doi:10.1371/journal.pntd.0010389
Marcogliese, D. J., Blaise, C., Cyr, D., de Lafontaine, Y., Fournier, M., Gagné, F., et al. (2015). Effects of a major municipal effluent on the St. Lawrence River: a case study. AMBIO 44, 257–274. doi:10.1007/s13280-014-0577-9
McManus, D. P., Dunne, D. W., Sacko, M., Utzinger, J., Vennervald, B. J., and Zhou, X. N. (2018). Schistosomiasis. Nat. Rev. Dis. Primer 4, 13. doi:10.1038/s41572-018-0013-8
McRae, L., Deinet, S., Marconi, V., Scott-Gatty, K., and Freeman, R. (2020). Bending the curve of biodiversity loss—chapter 1: an SOS for nature. Gland: WWF.
Melchiorre, H. G., Gutierrez, S. O., Minchella, D. J., and Vannatta, J. T. (2023). Downstream effects: impact of antibiotic pollution on an aquatic host–parasite interaction. Ecosphere 14, e4513. doi:10.1002/ecs2.4513
Mello-Silva, C. C., de Vasconcellos, M. C., Bezerra, J. C. B., Rodrigues, M. d. L. A., and Pinheiro, J. (2011). The influence of exposure to Euphorbia splendens var. hislopii latex on the concentrations of total proteins and nitrogen products in Biomphalaria glabrata infected with Schistosoma mansoni. Acta Trop. 117, 101–104. doi:10.1016/j.actatropica.2010.10.007
Mennerat, A., Nilsen, F., Ebert, D., and Skorping, A. (2010). Intensive farming: evolutionary implications for parasites and pathogens. Evol. Biol. 37, 59–67. doi:10.1007/s11692-010-9089-0
Merrill, M. L. (2022). Assessing embryonic toxicity and end fates of nanoplastics in freshwater environments using gastropod biomphalaria glabrata. Corpus Christi: Texas A&M University-Corpus Christi.
Müller OF 1774 “Vermivm terrestrium et fluviatilium, seu animalium infusoriorum, helminthicorum, et testaceorum, non-marinorum, succincta historia,” in Havniae lipsiae.
Nachev, M., and Sures, B. (2016). Environmental parasitology: parasites as accumulation bioindicators in the marine environment. J. Sea Res. 113, 45–50. doi:10.1016/j.seares.2015.06.005
Noël, H., Ruello, M., Maccary, A., Pelat, C., Sommen, C., Boissier, J., et al. (2018). Large outbreak of urogenital schistosomiasis acquired in Southern Corsica, France: monitoring early signs of endemicization? Clin. Microbiol. Infect. 24, 295–300. doi:10.1016/j.cmi.2017.06.026
Parodi, A. L. (2021). The ``One health’’ concept: reality and future prospect. Bull. Académie Natl. Médecine.
Prajapati, S., Challis, J. K., Jardine, T. D., and Brinkmann, M. (2022). Levels of pesticides and trace metals in water, sediment, and fish of a large, agriculturally-dominated river. Chemosphere 308, 136236. doi:10.1016/j.chemosphere.2022.136236
Romero-Blanco, A., Remón-Elola, A., and Alonso, Á. (2021). Assessment of the effects of environmental concentrations of microplastics on the aquatic snail Potamopyrgus antipodarum. Water Air Soil Pollut. 232, 438. doi:10.1007/s11270-021-05379-7
Salyer, S. J., Silver, R., Simone, K., and Barton Behravesh, C. (2017). Prioritizing zoonoses for global health capacity building—themes from one health zoonotic disease workshops in 7 countries, 2014–2016. Emerg. Infect. Dis. 23, S55–S64. doi:10.3201/eid2313.170418
Say, T. (1818). Account of two new genera, and several new species, of fresh water and land shells. J. Acad. Nat. Sci. Phila 2, 276–284.
Semenza, J. C., Rocklöv, J., and Ebi, K. L. (2022). Climate change and cascading risks from infectious disease. Infect. Dis. Ther. 11, 1371–1390. doi:10.1007/s40121-022-00647-3
Sures, B., Nachev, M., Pahl, M., Grabner, D., and Selbach, C. (2017a). Parasites as drivers of key processes in aquatic ecosystems: facts and future directions. Exp. Parasitol. 180, 141–147. doi:10.1016/j.exppara.2017.03.011
Sures, B., Nachev, M., Schwelm, J., Grabner, D., and Selbach, C. (2023). Environmental parasitology: stressor effects on aquatic parasites. Trends Parasitol. 39, 461–474. doi:10.1016/j.pt.2023.03.005
Sures, B., Nachev, M., Selbach, C., and Marcogliese, D. J. (2017b). Parasite responses to pollution: what we know and where we go in ‘Environmental Parasitology. ’ Parasit. Vectors 10, 65. doi:10.1186/s13071-017-2001-3
Syafrudin, M., Kristanti, R. A., Yuniarto, A., Hadibarata, T., Rhee, J., Al-onazi, W. A., et al. (2021). Pesticides in drinking water—a review. Int. J. Environ. Res. Public Health 18, 468. doi:10.3390/ijerph18020468
Tajudeen, Y. A., Oladipo, H. J., Oladunjoye, I. O., Oladipo, M. K., Shittu, H. D., Abdulmumeen, I. F., et al. (2023). Transforming malaria prevention and control: the prospects and challenges of gene drive technology for mosquito management. Ann. Med. 55, 2302504. doi:10.1080/07853890.2024.2302504
Tchouanguem, H. N., Fouelifack, F. Y., Keugoung, B., Fouelifa, L. D., and Moyou, R. S. (2016). Current status of schistosomiasis in Santchou health area, Santchou Health District, Cameroon western region. Pan Afr. Med. J. 24, 137. doi:10.11604/pamj.2016.24.137.8778
Tudi, M., Daniel Ruan, H., Wang, L., Lyu, J., Sadler, R., Connell, D., et al. (2021). Agriculture development, pesticide application and its impact on the environment. Int. J. Environ. Res. Public Health 18, 1112. doi:10.3390/ijerph18031112
Turak, E., Harrison, I., Dudgeon, D., Abell, R., Bush, A., Darwall, W., et al. (2017). Essential Biodiversity Variables for measuring change in global freshwater biodiversity. Biol. Conserv. 213, 272–279. doi:10.1016/j.biocon.2016.09.005
VijayaVenkataRaman, S., Iniyan, S., and Goic, R. (2012). A review of climate change, mitigation and adaptation. Renew. Sustain Energy Rev. 16, 878–897. doi:10.1016/j.rser.2011.09.009
Keywords: water quality, aquatic ecosystems, environmental parasitology, Schistosoma spp., chemical pollutants
Citation: Costa A, Guerrero H, Sureau A, Tassaint M and Augusto RdC (2024) Contaminated freshwater as a Harbinger of tropical disease spread in Europe. Front. Environ. Sci. 12:1410821. doi: 10.3389/fenvs.2024.1410821
Received: 01 April 2024; Accepted: 24 June 2024;
Published: 24 July 2024.
Edited by:
Renato Tavares Martins, National Institute of Amazonian Research (INPA), BrazilReviewed by:
Qinghui Huang, Tongji University, ChinaCopyright © 2024 Costa, Guerrero, Sureau, Tassaint and Augusto. This is an open-access article distributed under the terms of the Creative Commons Attribution License (CC BY). The use, distribution or reproduction in other forums is permitted, provided the original author(s) and the copyright owner(s) are credited and that the original publication in this journal is cited, in accordance with accepted academic practice. No use, distribution or reproduction is permitted which does not comply with these terms.
*Correspondence: Ronaldo de Carvalho Augusto, cm9uYWxkby5hdWd1c3RvQHVuaXYtcGVycC5mcg==
Disclaimer: All claims expressed in this article are solely those of the authors and do not necessarily represent those of their affiliated organizations, or those of the publisher, the editors and the reviewers. Any product that may be evaluated in this article or claim that may be made by its manufacturer is not guaranteed or endorsed by the publisher.
Research integrity at Frontiers
Learn more about the work of our research integrity team to safeguard the quality of each article we publish.