- International Rice Research Institute South Asia Regional Centre, Varanasi, Uttar Pradesh, India
Arthropods may make a significant fraction of the total number of soil organisms. They function as plant litter transformers or ecosystem engineers, and thus contribute positively to soil health. The present study was conducted during the 2020–2022 at International Rice Research Institute, South Asia Regional Centre, Varanasi. Study investigates the impact of different farming methods-conventional (Scenario 1; Sc1), LINF- Low-input natural farming (Sc 2); BBEF- Biochar-based ecological farming (Sc 3); CROF- Climate-resilient organic farming (Sc 4); RF- Regenerative farming (Sc 5) practices on soil arthropod populations in rice-wheat systems. Study utilized pitfall traps across various experimental setups. The findings revealed a significant increase in arthropod diversity and abundance, particularly in organic farming scenarios, where the Formicidae family (ants) and the Araneae family (spiders) were most prevalent. In an organically rich soil system, the five most diverse groups (Isopoda, Myriapoda, Insecta, Acari, and Collembola) were reported. This increase can be attributed to the nutrient-rich amendments that positively influence soil organisms. This study highlights a gradual increase in specific taxa, such as cockroaches, spiders, ants, and grasshoppers, following the transition to organic farming. Principal component analysis (PCA) further revealed distinct arthropod distribution patterns in the different farming systems, indicating the unique ecological impact of each method. Interestingly, predator populations in zero-till wheat fields under regenerative agriculture were greater than those in conventionally tilled fields. These results underscore the substantial role of organic and regenerative farming practices in promoting sustainable agricultural ecosystems. This study reveals the complex interplay between farming practices and arthropod dynamics and highlights the ecological benefits of sustainable agricultural methods, emphasizing their potential to enhance biodiversity and ecosystem health.
Highlights
1. Arthropod diversity and abundance were found to be significantly greater under organic agricultural practices than under conventional methods.
2. Specific taxa such as cockroaches, spiders, ants, and grasshoppers gradually increased after the transition to organic farming, indicating a shift in ecological balance.
3. The study revealed that arthropod dynamics and farming techniques interact intricately, with organic additions influencing community structure and increasing soil organic carbon.
4. Different arthropod distribution patterns were found in each of the farming systems, emphasizing the distinctive effects of each technique.
5. The results highlight how organic and sustainable farming methods may improve arthropod variety and abundance while also having positive ecological effects.
1 Introduction
Countries like China, India, Pakistan, Nepal, Bangladesh, and the Philippines are distinguished by their predominant rice‒wheat cropping systems. This system spans approximately 10.3 million hectares in India alone, forming the cornerstone of the nation’s food security (Khedwal et al., 2023). However, the sustainability of this system is increasingly questioned due to various environmental and agricultural challenges (Yadav et al., 2021). The question here is whether ecosystem services are maintained and supported by existing agricultural practices. Soil arthropods, one of the most significant elements of soil-living communities, are crucial for preserving the health and quality of soil as well as for providing ecosystem services (Gonçalves et al., 2021). They play a highly recognized role in various ecosystem service-related processes, including the breakdown and decomposition of organic matter, the cycling of nutrients, the creation of soil structure, and ultimately the management of soil water holding and availability (Parisi and Menta, 2008; Bengtsson et al., 2005). Furthermore, since they are incredibly suited to certain soil conditions and because they live, feed, and reproduce in the soil, some groups are especially sensitive to changes in the quality of the soil (Holland and Luff, 2000).
Additionally, wheat is particularly susceptible to heat waves, and rice as a highly water-demanding crop, contributes significantly to greenhouse gas emissions. Moreover, there is a declining response per fertilizer unit, compelling farmers to use increased quantities to achieve similar yields, leading to groundwater contamination with nitrates (Datta et al., 2022). This situation underscores the growing importance of organic (farmyard manure, vermicompost, and jivamrit) and regenerative practices (ecological engineering for pest control, zero-tillage, and on-site crop residue management) for ensuring long-term production sustainability.
Advocated for integrating a short-duration mungbean crop and incorporating its residues during summer to enhance system sustainability. Prasad and Misra (2001) also recommend applying farmyard manure (FYM) at 10 t ha-1 alongside recommended NPK doses. These practices aim to maintain the viability of the rice-wheat cropping system.
Moreover, the success of the rice-wheat-mungbean production system is heavily influenced by effective weed management. Conservation agriculture, which has gained widespread adoption for its contributions to sustainability, plays a crucial role in this context. It is essential to recognize that altering faunal habitats, often through vegetation removal, significantly impacts arthropods, which are crucial for agroecosystem ecological functions (Quinn et al., 2016). Arthropods, which are sensitive to changes in vegetation, contribute to detritus degradation and organic matter cycling, thereby supplying nutrients to other organisms (Giesy et al., 2000; Roy et al., 2018). The mechanical alteration of soil, variations in plant residue quantity and placement, and changes in weed communities all affect arthropod communities (Ponce et al., 2011). Effects of tillage are important on soil arthropods frequency and intensity (Bhan and Behera, 2014). In direct planting systems, crop residues are concentrated at the surface, fostering a more intricate biological system and maintaining stable microclimatic conditions, particularly regarding soil humidity and temperature. This creates a more favorable habitat for soil fauna, and this was well reviewed by Chen et al. (2024).
Additionally, the diversity of natural enemies, including larger arthropods, plays a pivotal role in the biological control of arthropods (Menta and Remelli, 2020; Huot et al., 2018; Ponce et al., 2011). It has been found that agricultural expansion affects the species composition of communities, has a detrimental impact on the diversity of their functional traits, and thus attenuates the functioning of ecosystems and the benefits they provide (Emmerson et al., 2016). Contemporary agricultural practices such as conventional tillage, deep tillage, intensive and imbalanced use of chemical fertilizers, and widespread pesticide application have contributed to a decline in arthropod populations (Gonçalves et al., 2021). These practices result in complex interactions among natural enemies, leading to positive, negative, and neutral outcomes. A stable insect community emerging from these interactions forms an intricate food chain and web, providing significant opportunities for community interaction and compensation if one pathway is disturbed (Menta and Remelli, 2020; Huot et al., 2018).
Furthermore, Hong et al. (2022) demonstrated the connection between biodiversity and ecosystem health. The excessive use of fertilizers and pesticides, coupled with poorly coordinated development and landscape transformation in modern intensive agricultural practices, has led to pollution in water and soil environments and eradicated the biological refuge and habitat provided by wheat and rice fields (Hong et al., 2022). The biodiversity established in rice‒wheat fields is essential for stable ecosystems, contributing significantly to the ecosystem services offered by paddy fields and creating economic value for society.
Unfortunately, sufficient database on soil organisms (including arthropods) and their role in ecosystem functions are still lacking (Havlicek, 2012). Their significance in agriculture is presented only as worldwide statistics and in the form of straightforward facts suitable for mass audiences. The growing concern about sustainability in agriculture and the food industry, as well as the role of soil quality, may shed light on the significance of a thorough understanding of soil arthropod communities and the critical role they play in maintaining soil quality and health (Menta and Remelli, 2020). Thus, understanding soil arthropod communities under different soil nutrient management practices can aid in the development of management plans for sustainable agriculture. The hypothesis of the study is that different organically managed crop fields may help increase soil arthropod biodiversity for soil and agricultural sustainability. Thus, the study aimed to explore, evaluate, and compare the abundance, family diversity, and prey-predator composition of arthropods in rice-wheat cropping systems under conventional (chemical fertilizer- and pesticide-based agricultural practices), organically amended (use of compost and organic solutions), and regenerative (zero-tillage, crop residue management in the field, etc.) agricultural practices over three-year post-conversion from conventional practices. This study focused on a diverse group of arthropods with distinct functions, including insects such as spiders, ants, grasshoppers, earthworms, and beetle insects, and elucidated the importance of this existing cropping system.
2 Materials and methods
2.1 Experimental site
A field experiment involving rice (Oryza sativa), wheat (Triticum aestivum), and mungbean (Vigna radiata L.) crops was established seasonally in 2020, 2021, and 2022 at the International Rice Research Institute, Varanasi (25.302887 82.947973, 83 m above mean sea level), Uttar Pradesh, India. The average minimum and maximum temperatures at the site were 15 and 30°C, respectively. The annual rainfall ranges between 700 and 800 mm, with more than 70% falling between July and September during the monsoon season. The treatments included organic input, chemical base fertilizer, RA (75% chemical fertilizer and 25% organic input), and a sequence cropping rice‒wheat-mung bean system. The data were collected at the end of the season, which is twice a year. Initial soil parameters of the experimental agricultural soil are given in Table 1.
Agricultural field soil samples were collected from the top layer (0–15 cm) randomly within each plot with the help of an auger. Three samples within a plot were thoroughly mixed to make composite samples followed by air drying. A part of soil sample was ground and the filtered using 2.0 mm sieve as per the requirement of different parameter analysis.
The initial soil properties (pH, EC, organic carbon, and available N, P, K) were estimated in air-dried samples using standard protocols.
2.2 Experimental details and descriptions of the scenarios
This study evaluated a portfolio of management practices under different crops and cropping systems (Figure 1). Five combinations of treatments with different crop rotations and associated management practices, referred to as scenarios (Sc), were evaluated according to the prevailing conditions in North India. Each scenario was replicated three times in a production-scale plot (14 m × 5 m = 70 m2) in a randomized complete block design. Distance between replicate field was 0.5 m and distance between treatments was 0.25 m. All the management practices in scenario 1 (Sc1) were based on current farmer practices (Table 2) in the region. Whereas, management practices in Sc2, Sc3, and Sc4 were based on organic farming, and that in Sc5 was based on regenerative agricultural farming (Table 3).
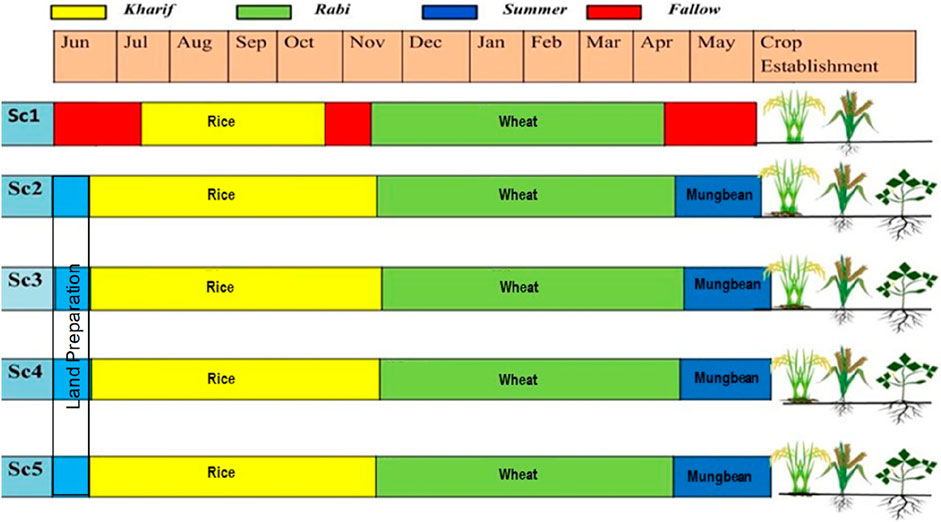
Figure 1. Schematic diagram of different crops and cropping sequences under different crop establishment methods (Sc1, conventional farming; Sc2, low-input natural farming; Sc3, biochar-based ecological farming; Sc4, climate-based ecological farming; and Sc5, regenerative agriculture).
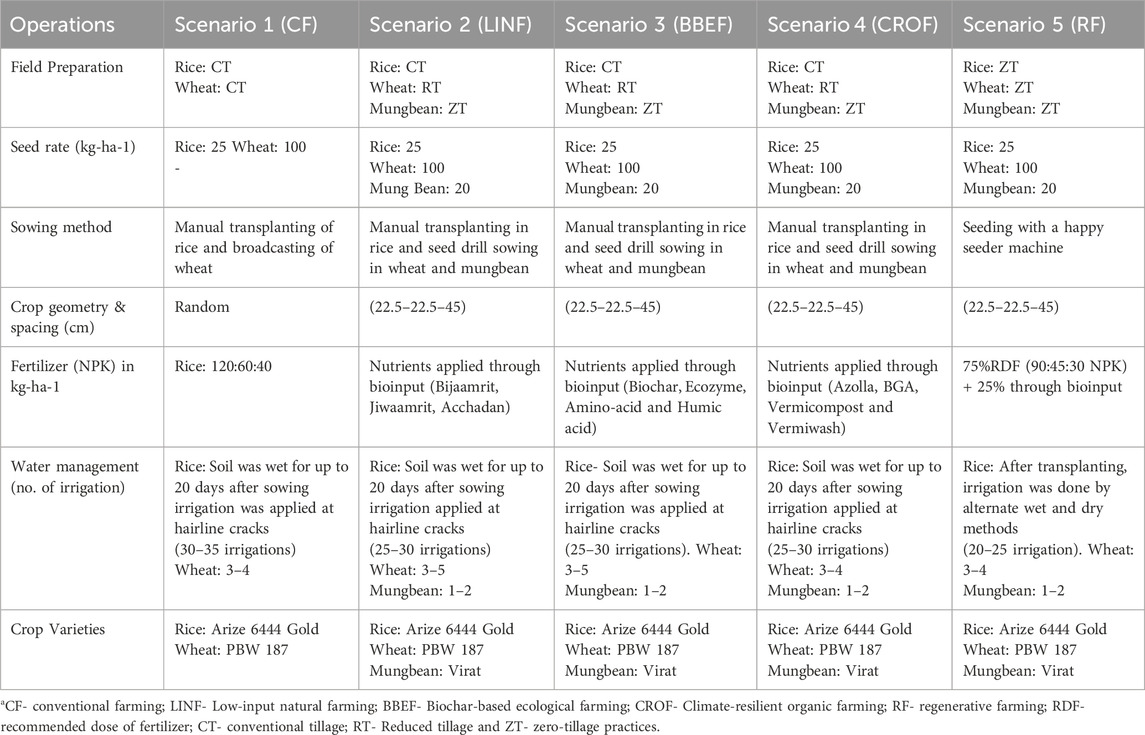
Table 2. Tillage, seed rate, cropping system, and agronomic management practices followed in organic farming systems and the five scenarios.
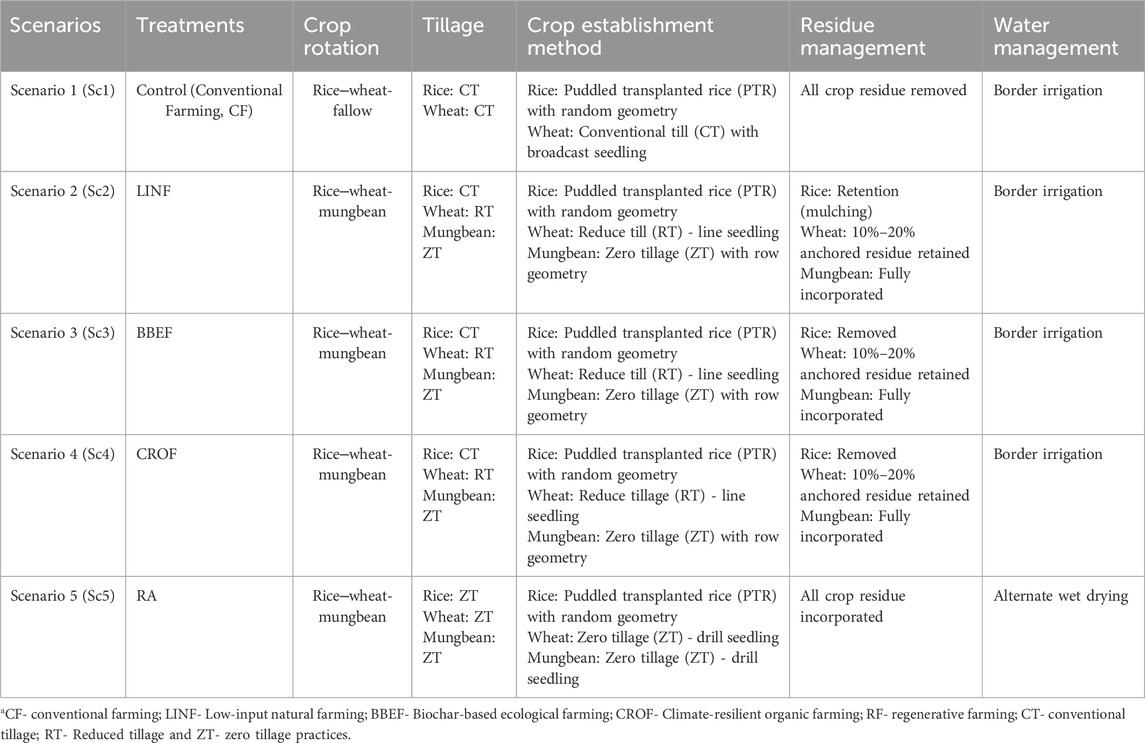
Table 3. Crop rotation, tillage and crop establishment methods, residue management, and water management protocols under different scenarios. CT: conventional tillage, ZT: zero-tillage, DSR: direct seeded rice.
The following are the five scenarios with different agricultural management practices:
Sc1- Conventional farming, CF-puddled transplanted rice (PTR), conventional tillage (CT), wheat without residue (-R), and chemical fertilizer use were used.
Sc2-Conventional tillage direct seeded rice (CTDSR) and conventional tillage wheat (CTW) with no residue (-R) Zero-tillage mungbean without residue (+R): This scenario is based on low-input natural farming (Bijamrit, Jeevamrit, Acchadan).
Sc3: No residue conventional tillage direct seeded rice (CTDSR) and wheat (CTW): This scenario is based on biochar-based ecological farming (biochar, ecozyme, amino acids, humic acid).
Sc4.-Rice was followed by conventional tillage direct seeded rice (CTDSR). Conventional tillage wheat (CTW) with no residue (-R). Zero-tillage mungbean (ZTM): This scenario is based on climate-resilient organic farming (Azolla, BGA, vermicompost, and vermiwash).
Sc-5: Zero-tillage direct seeded rice (ZTDSR); zero-tillage wheat (ZTW) with residue (-R) Zero-tillage mungbean (ZTM) with residue (+R) is based on regenerative agriculture (75% chemical and 25% organic fertilizer used).
2.3 Pitfall traps
The pitfall traps were made using plastic cups of 8 cm in diameter at the top, 5 cm in diameter at the bottom, and 10 cm tall when buried in the soil (lined on top with the bottom of the leaf litter level) as described by Ponce et al. (2011) and Ruiz-Lupión et al. (2019). A 10 × 10 × 1 cm3 wood lid was gently placed on top, just sitting on the litter. Because the study aimed to capture active fauna within the leaf litter layer, which moves mainly in the dark, a lid was used to reduce incoming light in the traps. An open pitfall trap would have skewed the results toward nocturnal fauna or the small number of taxa that are active on top of the litter layer during the day (e.g., some species of carabid beetles) according to Moya-Larano (2011), and personal observations. Most meso-fauna and macro-fauna were prevented from escaping through the trap’s bottom while allowing water to drain. Furthermore, we included some leaves inside the trap to reduce the occurrence of predation as much as possible (i.e., to minimize the predation of small trapped arthropods by bigger arthropods) and have the same potential attractiveness as the other two trap types. However, we only used six leaves (dry mass mean: 2.67 ± 0.03 g) at the bottom of each pitfall trap, up to a height of 3 cm, far enough from the trap opening to prevent the larger organisms from climbing out (Figure 2).
2.4 Statistical analysis
All experiments were independently performed with at least three replicates. All mean data were analyzed by one-way analysis of variance (ANOVA) using the least significant difference method (LSD) in SPSS statistical software (version 18.0 for Windows, SPSS, Inc.) to determine whether there was any significant difference in arthropod biodiversity among the treatments. The level of statistical significance was set at p < 0.05. Principal component analysis (PCA) was applied to the present datasets (families of arthropods). The effects of different scenarios on arthropods (as a response to the practices) were compared for higher inputs through PCA. PCA provides visual analysis of whether the responses are dependent or independent of the independent variables (practices/scenarios). The general patterns of arthropods in various soil environments across practices were also described.
3 Results
3.1 Effect on the different taxonomic groups
Different arthropods taxon were observed depending on the year and tillage system (Figure 3), namely, Araneae (spiders), collembola (springtails), Acarina (mites), Formicidae (ants), Coleoptera (beetles), Diptera (flies), Homoptera (hoppers), Acarina, Gryllidae, Culicinae (mosquito), Acarina, Forficulidae, and Lumbricidae (earthworms).
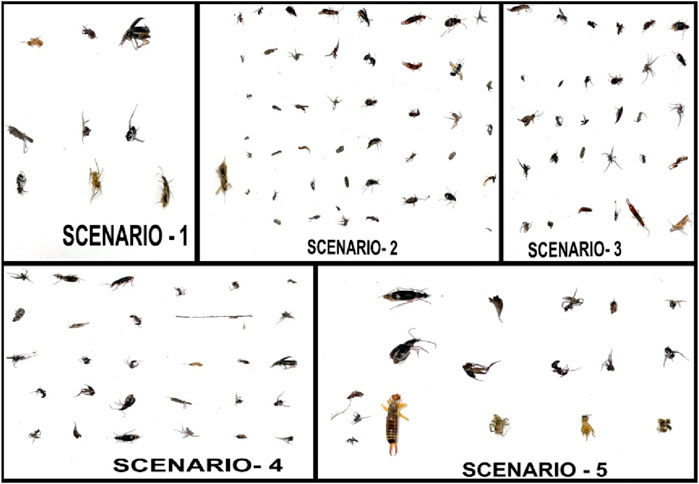
Figure 3. Photographs of arthropod diversity under different scenarios (Sc1, conventional farming; Sc2, low-input natural farming; Sc3, biochar-based ecological farming; Sc4, climate-based ecological farming; and Sc5, regenerative agriculture).
In 2022, there was a notable increase in arthropod populations compared to those in 2020 and 2021. Among the various scenarios (Sc1, Sc2, Sc3, Sc4, and Sc5), Sc1 had lower counts of total sampled arthropods, and these were Araneae, Collembola, Acarina, Formicidae, and others, as details provided in Table 3.
3.2 Comprehensive analysis of arthropod distribution across farming systems
PCA was utilized to explore the distribution of arthropod families in various farming systems over several years, as depicted in Figure 4. This analysis revealed distinct patterns, with certain families predominating in specific farming scenarios. Notably, a considerable dispersion of arthropods was observed in Sc1, contrasting with the high diversity and number in Sc2. Over the mentioned study period, the variability among arthropod families in the rice‒wheat cropping system was clearly reported. Families such as Araneae and Formicidae exhibited specific associations with conventional (Sc1) and regenerative farming (Sc5) systems, respectively, while Coleoptera and Homoptera were more abundant in Sc2, Sc3, and Sc4 (detailed mean data is provided in Table 4).
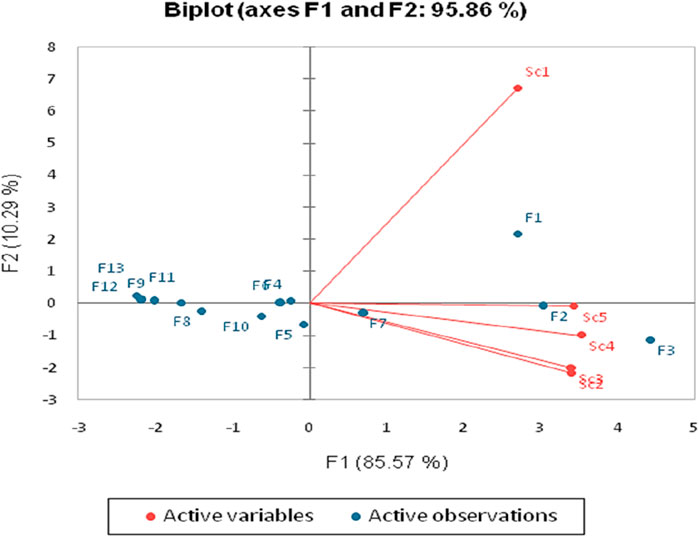
Figure 4. PCA (biplot) between agricultural systems and the number of arthropod families under different scenarios (Sc1-conventional farming, Sc2-low-input natural farming, Sc3-biochar-based ecological farming, Sc4-climate-based ecological farming, and Sc5-regenerative agriculture).
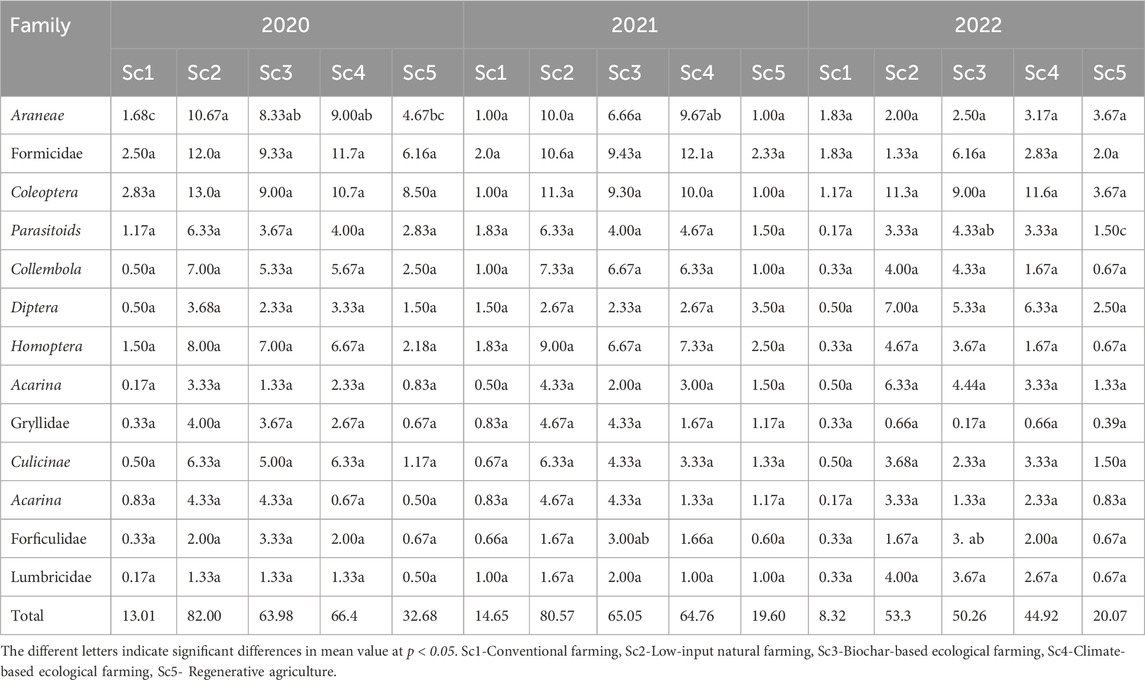
Table 4. The mean number of principal groups of arthropods collected under different scenarios in 2020, 2021, and 2022.
In the biplot analysis, the relationship between variables is denoted by the angle between vectors. This method accounted for 95.7% of the observed variability, demonstrating the intricate relationships among arthropod families and farming practices (Figure 4). Different arthropod families, represented by points, were analyzed in relation to the farming systems denoted by arrows. The analysis showed positive correlations for some families with specific farming systems, while others exhibited negative correlations, indicating varied impacts on specific farming practices.
3.3 Predator and prey population and species variation in wheat
Predator populations in zero-till wheat-sown fields demonstrated significant variations in regenerative agriculture practice from 2020 to 2022. The reported counts (average value) of arthropods were 6.6, 5.6, and 4.2 per square meter, respectively, which were notably higher (approximately double) than those in conventionally tilled fields within conventional farming settings (Figure 5). This trend was consistent for various species, with notable differences in population density based on tillage treatments. For instance, spider populations averaged 3.59 per square meter in zero-tillage wheat fields, in contrast to the average of 1.36 in conventionally sown fields. Rove beetles followed a similar pattern, with counts of 3.56 in ZT fields and 1.58 in conventional fields. This study emphasizes the critical role of rice stubbles in preserving predator populations within rice‒wheat systems. This finding underscores the importance of biodiversity in agroecosystems for maintaining stability in predator conservation and controlling pest outbreaks. If comparing the scenarios for all the 3 years, there was significant difference noted between all the scenarios for the predator population and difference was more prominently visible during the 2022.
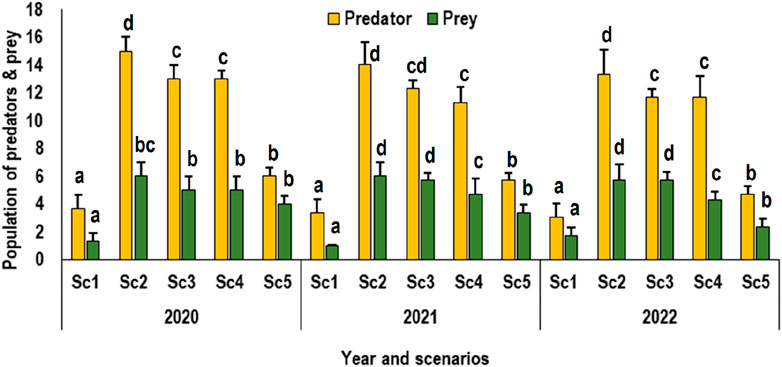
Figure 5. Predator and prey population dynamics in rice‒wheat cropping systems under different tillage treatments (Sc1-conventional farming, Sc2-low-input natural farming, Sc3-biochar-based ecological farming, Sc4-climate-based ecological farming, and Sc5-regenerative agriculture). Alphabetical symbols on the bars shows the significance difference (at p < 0.05) between the treatment.
3.4 Rice population and species variations
Cultivating rice after harvesting reduced-tillage wheat crop in Sc2, Sc3, and Sc4 resulted in a notably greater predator population as compared to rice cropping following conventional wheat farming (Figure 5). In both locations, there was a marginal increase in the predator population for rice planted in conventionally sown wheat. Notably, the disparity in predator populations between zero-till and conventional-tillage wheat crops was less pronounced in the rice crop than in the wheat crop. This observation suggests that the impact of tillage practices on predator populations may vary across different crops within the agricultural system.
3.5 Macrofauna and mesofauna population dynamics in the rice‒wheat cropping system
Explored fauna within a three-year rice–wheat cropping system revealed significantly positive trend. Analyses for 2020, 2021, and 2022 revealed that Sc2 had the greatest macrofaunal population in 2020 and 2022, while Sc3 showed peaked data in 2021. Mesofaunal numbers were highest in Sc2 during 2020 and, in Sc4 during 2021. Conventional farming consistently had the lowest populations of both macrofauna and mesofauna, likely due to the use of pesticides and chemical fertilizers (Figure 6). In contrast, organic farming exhibited the greatest population, surpassing conventional, regenerative, and other farming practices. This study also sheds light on the temporal dynamics of the transition from conventional to organic farming and its impact on arthropod abundance. The population trends sometimes followed a nonlinear saturation pattern, suggesting that the equilibrium in arthropod populations might take up to a decade to stabilize post transition to organic farming. If comparing the scenarios for all the 3 years, there was significant difference noted between Sc1 and Sc5. Sc2, Sc3, and Sc4 did not show any regular trend for both macrofauna and mesofauna during the experimental period.
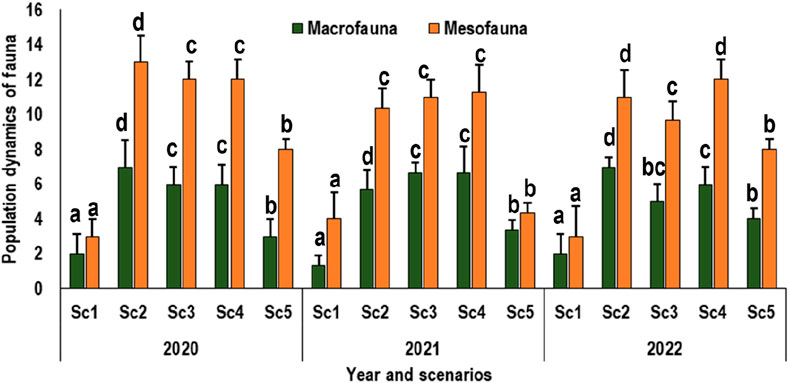
Figure 6. Macrofauna and Mesofauna population dynamics in rice‒wheat cropping systems under different tillage treatments (Sc1-conventional farming, Sc2-low-input natural farming, Sc3-biochar-based ecological farming, Sc4-climate-based ecological farming, and Sc5-regenerative agriculture). Alphabetical symbols on the bars shows the significance difference (at p < 0.05) between the treatment.
4 Discussion
The study, encompassing five scenarios and 13 arthropod families, explored the fauna within a three-year rice-wheat cropping system and revealed significant results of the management The present study aimed to assess the impacts of various long-term crop establishment, residue management and sustainable agricultural practices on arthropod diversity and composition under the subtropical humid climate of the Eastern Indo-Gangetic Plain of Uttar Pradesh, India. Soil flora–fauna diversity has been reported to be strongly affected by tillage in our findings and supported by literature (Moretti et al., 2017; Gora et al., 2022). As a significant number of arthropoda (13 arthropod families) were found in the study, it is to be noted that the number of soil-dwelling Diptera in temperate zones can reach higher, may be 50–150 species (Frouz, 1999; Ponce et al., 2011; Vera-Aviles et al., 2020). Depending on the family to which they belong—for example, Chironomidae in wet grasslands and Empididae in deciduous tree formations—they are more prevalent in forests than on arable land (Menta and Ramelli, 2020). Coleoptera, another arthropod reported in the study, is the largest order of beetles, and are frequently utilized as indicators because of their quick reaction to disturbances (forest cutting, grazing, fertilization, and habitat fragmentation). Additionally, they are also dependent on numerous biotic and abiotic variables that regulate their population as well (Rainio and Niemelä, 2003). However, the integration of organic farming is expected to result in a delayed positive response in species groups due to slow changes in soil properties and limitations in organism dispersal. Spiders, which are more abundant in the food web, may respond more slowly than other arthropods.
The decrease in Acarina abundance in Sc1, attributed to habitat modification rather than glyphosate use (van Eekeren et al., 2022; Giesy et al., 2000; George et al., 2017). Furthermore, the increased survival of specific mite groups in tilled soil could be due to their resilience to water scarcity, which is consistent with the findings of Moretti et al. (2017). This analysis describes and supports the complex interplay between population dynamics and environmental factors.
It is well known that organic amendments significantly enhance soil organic carbon and micronutrient levels, and thus influencing soil organisms (Crystal-Ornelas et al., 2021). In the compost-treated plots, it was found in our study that spiders exhibited larger abdomens, suggesting increased prey availability and reduced leaf damage. Despite no decrease in herbivore numbers, the larger spiders indicate potential shifts in predator‒prey dynamics. Spiders also played a significant role in neem-treated rice plots, as reported by Baitha et al. (2000a and 2000b). These findings highlight the complex relationships within the ecosystem and the positive impact of organic farming on plant resistance and pest control (Muneret et al., 2018) and meta-analyses by Crystal-Ornelas et al. (2021).
Furthermore, Formicidae are the most commonly utilized hymenopteran bio-indicator at the family level. Collembolan, mite, spider, and beetle species are richer than ant species and are more frequently used as environmental indicators. However, counting and identifying them takes longer than identifying ant species. They are also better indicators of assemblage composition than other groups and have a well-established ecology (Hoffmann and Anderson, 2003; Majer et al., 2007). Ants are well-known social insects that exhibit stationary nesting behavior. This characteristic of their ecology enables ant associations with the regions from which they are collected. Ants have been effectively employed as bio-indicators in Australia (Andersen and Majer, 2004), where their richness is connected with microbial activity in restored mining sites, as well as markers of pollution, the health of forests, and the state of rangelands. Since species assemblage, abundance, and richness are related to soil management factors, soil variables, and cropping practices, ants may have potential as biological indicators of soil conditions and management in agroecosystems. Previous research has indicated that ants respond to land changes in predictable ways. Additionally, because they may improve soil drainage, aeration, and nutrient supply, ants are crucial ecosystem engineers who help to implement low-impact farming practices. As indicated in Table 3, the Formicidae (ant) family boasts the greatest number of members, followed by the Araneae (spider) family. Table 3 shows the delayed or gradual responses of arthropods, potentially attributed to factors such as dispersal extent (referred to as “colonization credit”), residual effects of pesticides, the presence of chemical fertilizers in the soil, and the lag time associated with organic matter mineralization. Within the scope of the current study, specific taxa, including cockroaches, spiders, ants, and grasshoppers, gradually increased after the shift to organic farming. Among the factors mentioned above, dispersion limitation may not be the primary reason for the gradual increase, particularly in units adopting organic farming, such as the individual Sc2, Sc3, and Sc4 scenarios. Consequently, rapid immigration from neighboring habitats to these areas is anticipated. However, dispersal limitation could account for the observed changes in spiders and ants. These particular spiders are recognized for congregating in regions abundant in prey populations, yet their modus operandi revolve around daily site changes.
The study also highlighted the enhanced predator populations in zero-till wheat fields compared to conventionally sown fields (PCA analysis), suggesting that predator conservation could be a viable strategy for insect pest management in rice-wheat systems. The comprehensive PCA sheds light on the dynamic interplay of arthropod families in different agricultural contexts and underscores the potential of specific farming practices in promoting ecological balance.
A diverse ecosystem provides vital environmental buffers, offering necessary hosts, food, shelter, and overwintering sites, thereby reducing the likelihood of pest outbreaks and contributing to agricultural sustainability (Salim et al., 2003; Emmerson et al., 2016). This relationship, as provided in our study sites, between biodiversity and agricultural health highlights the need for integrated pest management strategies prioritizing ecological balance and sustainability. Additionally, this study provides vital insights into the time-dependent responses of organisms in rice‒wheat fields, highlighting the benefits of organic farming in supporting arthropod diversity. This diversity is crucial and is influenced by the scale and context of the agricultural system. The findings suggest that the arthropod population observed is sufficient for the needs of the organic farming community, emphasizing the overall benefits of organic practices in agricultural ecosystems.
5 Conclusion
The results of this study revealed a significant increase in arthropod populations in rice-wheat cultivation under organic farming practices (Sc2 and Sc3) as compared to conventional methods. The use of organic fertilizers and the integration of legume (mungbean) crops in this study showed a critical factor in enhancing the abundance and diversity of arthropods in organic (Sc2) and no-till (ZT) systems. A notable increase in arthropod populations as well predator-prey population was observed in Scenario 2, where implementing cover crops such as mulch played a critical role (no-tillage). Macrofauna and mesofauna were higher in Sc4 supporting the climate based ecological farming. This research underscores the complex relationship between agricultural practices and arthropod dynamics, emphasizing the ecological advantages of adopting organic and sustainable farming approaches for enhancing biodiversity and contributing to a more sustainable ecosystem.
Data availability statement
The original contributions presented in the study are included in the article/supplementary material, further inquiries can be directed to the corresponding author.
Author contributions
AM: Conceptualization, Data curation, Funding acquisition, Resources, Supervision, Visualization, Writing–original draft. AT: Formal Analysis, Writing–original draft. PM: Formal Analysis, Writing–original draft. SS: Conceptualization, Funding acquisition, Project administration, Resources, Visualization, Writing–review and editing.
Funding
The author(s) declare that no financial support was received for the research, authorship, and/or publication of this article.
Acknowledgments
The authors wish to acknowledge their respective institutions and funding agencies for the infrastructure support. The authors also wish to acknowledge the funding support from the Ministry of Agriculture and Farmers’ Welfare, the government of India. We are highly thankful to the International Rice Research Institute (IRRI) South Asia Regional Centre for providing an agricultural field in Varanasi, India, and for their scientific contributions to the study. We thank Pidikiti Pavithra, Manas Ranjan Sahoo, and Kshitikant Rout for supporting field data collection and trail management and PM for data tabulation and analysis.
Conflict of interest
The authors declare that the research was conducted in the absence of any commercial or financial relationships that could be construed as a potential conflict of interest.
Publisher’s note
All claims expressed in this article are solely those of the authors and do not necessarily represent those of their affiliated organizations, or those of the publisher, the editors and the reviewers. Any product that may be evaluated in this article, or claim that may be made by its manufacturer, is not guaranteed or endorsed by the publisher.
References
Andersen, A. N., and Majer, J. D. (2004). Ants show the way down under: invertebrates as bioindicators in land management. Front. Ecol. Environ. 2, 291. doi:10.2307/3868404
Baitha, A., Hameed, S. F., and Singh, R. (2000a). Effectiveness and economics of various treatments and their impact on spider population in rice ecosystem. Ann. Pl. Protec Sci. 8 (1), 13–17. doi:10.5555/20001109017
Baitha, A., Hameed, S. F., and Singh, R. (2000b). Relative toxicity of neem products against the larvae of rice folder. Indian J. Ent 62 (1), 66–68. doi:10.5555/20003030909
Bengtsson, J., Ahnström, J., and Weibull, A. C. (2005). The effects of organic agriculture on biodiversity and abundance: a meta-analysis. J. Appl. Ecol. 42 (2), 261–269. doi:10.1111/j.1365-2664.2005.01005.x
Bhan, S., and Behera, U. K. (2014). Conservation agriculture in India–Problems, prospects and policy issues. Int. Soil Water Conservation Res. 2 (4), 1–12. doi:10.1016/s2095-6339(15)30053-8
Blake, G. R., and Hartage, K. H. (1986). “Bulk density,” in 0, methods of soil analysis part 1-physical and mineralogical methods. Amer. Soc. Agron., Inc., and soil sci. Soc. Amer., Editor A. Klute second edition (Madison, Wis: Inc.), 363–375.
Chen, J., North, M., and Franklin, J. F. (2024). The contributions of microclimatic information in advancing ecosystem science. Agric. For. Meteorology 355, 110105. doi:10.1016/j.agrformet.2024.110105
Crystal-Ornelas, R., Thapa, R., and Tully, K. L. (2021). Soil organic carbon is affected by organic amendments, conservation tillage, and cover cropping in organic farming systems: a meta-analysis. Agric. Ecosyst. and Environ. 312, 107356. doi:10.1016/j.agee.2021.107356
Datta, P., Behera, B., and Rahut, D. B. (2022). Climate change and Indian agriculture: a systematic review of farmers’ perception, adaptation, and transformation. Environ. Challenges 8, 100543. doi:10.1016/j.envc.2022.100543
Emmerson, M., Morales, M. B., Oñate, J. J., Batary, P., Berendse, F., Liira, J., et al. (2016). How agricultural intensification affects biodiversity and ecosystem services. Adv. Ecol. Res. 55, 43–97. doi:10.1016/bs.aecr.2016.08.005
George, P. B. L., Keith, A. M., Creer, S., Barrett, G. L., Lebron, I., Emmett, B. A., et al. (2017). Evaluation of mesofauna communities as soil quality indicators in a national-level monitoring programme. Soil Biol. biochem. 115, 537–546. doi:10.1016/j.soilbio.2017.09.022
Giesy, J. P., Dobson, S., and Solomon, K. R. (2000). Ecotoxicological risk assessment for roundup herbicide. Rev. Environ. Contam. Toxicol. 167, 35–120. doi:10.1007/978-1-4612-1156-3_2
Gonçalves, F., Carlos, C., Crespo, L., Zina, V., Oliveira, A., Salvação, J., et al. (2021). Soil arthropods in the douro demarcated region vineyards: general characteristics and ecosystem services provided. Sustainability 13 (14), 7837. doi:10.3390/su13147837
Gora, M. K., Kumar, S., Jat, H. S., Kakraliya, S. K., Choudhary, M., Dhaka, A. K., et al. (2022). Scalable diversification options deliver sustainable and nutritious food in Indo-Gangetic plains. Sci. Rep. 12 (1), 1–15. doi:10.1038/s41598-022-18156-1
Hanway, J. J., and Heidel, H. (1952). Soil analysis methods as used in Iowa state college soil testing laboratory. Low State Coll. Agric. Bull. 57, 1–13.
Havlicek, E. (2012). Soil biodiversity and bioindication: from complex thinking to simple acting. Eur. J. Soil Biol. 49, 80–84. doi:10.1016/j.ejsobi.2012.01.009
Hoffmann, B. D., and Andersen, A. N. (2003). Responses of ants to disturbance in Australia, with particular reference to functional groups. Austral Ecol. 28, 444–464. doi:10.1046/j.1442-9993.2003.01301.x
Holland, J. M., and Luff, M. L. (2000). The effects of agricultural practices on Carabidae in temperate agroecosystems. Integr. Pest. Manag. Rev. 5, 109–129. doi:10.1023/a:1009619309424
Hong, P., Schmid, B., De Laender, F., Eisenhauer, N., Zhang, X., Chen, H., et al. (2022). Biodiversity promotes ecosystem functioning despite environmental change. Ecol. Lett. 25 (2), 555–569. doi:10.1111/ele.13936
Huot, H., Cortet, J., Watteau, F., Milano, V., Nahmani, J., Sirguey, C., et al. (2018). Diversity and activity of soil fauna in an industrial settling pond managed by natural attenuation. Appl. Soil Ecol. 132, 34–44. doi:10.1016/j.apsoil.2018.08.020
Khedwal, R. S., Chaudhary, A., Sindhu, V. K., Yadav, D. B., Kumar, N., Chhokar, R. S., et al. (2023). Challenges and technological interventions in rice–wheat system for resilient food–water–energy-environment nexus in Northwestern Indo-Gangetic Plains: a review. Cereal Res. Commun. 51, 785–807. doi:10.1007/s42976-023-00355-9
Majer, J. D., Orabi, G., and Bisevac, L. (2007). Ants (Hymenoptera: Formicidae) pass the bioindicator scorecard. Myrmecol. News. 10, 69–76.
Menta, C., and Remelli, S. (2020). Soil health and arthropods: from complex system to worthwhile investigation. Insects 11 (1), 54. doi:10.3390/insects11010054
Moretti, M., Dias, A. T. C., de Bello, F., Altermatt, F., Chown, S. L., Azcárate, F. M., et al. (2017). Handbook of protocols for standardized measurement of terrestrial invertebrate functional traits. Funct. Ecol. 31, 558–567. doi:10.1111/1365-2435.12776
Moya-Laraño, J. (2011). Genetic variation, predator–prey interactions and food web structure. Philosophical Trans. R. Soc. B Biol. Sci. 366 (1569), 1425–1437. doi:10.1098/rstb.2010.0241
Muneret, L., Mitchell, M., Seufert, V., Aviron, S., Djoudi, E. A., Pétillon, J., et al. (2018). Evidence that organic farming promotes pest control. Nat. Sustain. 1, 361–368. doi:10.1038/s41893-018-0102-4
Olsen, S. R. (1954). Estimation of available phosphorus in soils by extraction with sodium bicarbonate (No. 939). US Department of Agriculture.
Parisi, V., and Menta, C., 2008. Microarthropods of the soil: convergence phenomena and evaluation of soil quality using QBS-ar and QBS-C.
Ponce, C., Bravo, C., de León, D. G., Magana, M., and Alonso, J. C. (2011). Effects of organic farming on plant and arthropod communities: a case study in Mediterranean dryland cereal. Agric. Ecosyst. and Environ. 141 (1-2), 193–201. doi:10.1016/j.agee.2011.02.030
Prasad, R., and Misra, B. N. (2001). Effect of addition of organic residues, farmyard manure and fertilizer nitrogen on soil fertility in rice-wheat cropping system. Archives Agron. Soil Sci. 46 (5-6), 455–463. doi:10.1080/03650340109366190
Quinn, N. F., Brainard, D. C., and Szendrei, Z. (2016). The effect of conservation tillage and cover crop residue on beneficial arthropods and weed seed predation in acorn squash. Environ. Entomol. 45 (6), 1543–1551. doi:10.1093/ee/nvw139
Rainio, J., and Niemelä, J. (2003). Ground beetles (Coleoptera: carabidae) as bioindicators. Biodivers. and Conservation 12, 487–506. doi:10.1023/a:1022412617568
Roy, S., Roy, M. M., Jaiswal, A. K., and Baitha, A. (2018). Soil arthropods in maintaining soil health: thrust areas for sugarcane production systems. Sugar Tech. 20 (4), 376–391. doi:10.1007/s12355-018-0591-5
Ruiz-Lupión, D., Pascual, J., Melguizo-Ruiz, N., Verdeny-Vilalta, O., and Moya-Laraño, J. (2019). New litter trap devices outperform pitfall traps for studying arthropod activity. Insects 10 (5), 147. doi:10.3390/insects10050147
Salim, M., Ramzan, M., and Rehman, A. (2003). Zero tillage technology of sowing wheat: effects on Predators in rice‒wheat cropping systems. SAARC J. Agric. 1, 199–207. doi:10.5555/20103304562
Subbiah, B. V., and Asija, G. L. (1956). A rapid method for estimation of available Nitrogen in soils. Curr. Sci. 25, 259–260. doi:10.5555/19571900070
van Eekeren, N., Jongejans, E., van Agtmaal, M., Guo, Y., van der Velden, M., Versteeg, C., et al. (2022). Microarthropod communities and their ecosystem services restore when permanent grassland with mowing or low-intensity grazing is installed. Agric. Ecosyst. and Environ. 323, 107682. doi:10.1016/j.agee.2021.107682
Vera-Aviles, D., Suarez-Capello, C., Llugany, M., Poschenrieder, C., De Santis, P., and Cabezas-Guerrero, M. (2020). Arthropod diversity influenced by two musa-based agroecosystems in Ecuador. Agriculture 10 (6), 235. doi:10.3390/agriculture10060235
Keywords: soil arthropods, organic farming, regenerative agriculture, rice-wheat cropping system, biodiversity, ecosystem health, sustainable practice
Citation: Mishra AK, Tiwari A, Maurya PK and Sharma S (2024) Enhancing ecosystem dynamics: organic and regenerative practices in rice–wheat systems and their impact on soil arthropod biodiversity. Front. Environ. Sci. 12:1407954. doi: 10.3389/fenvs.2024.1407954
Received: 27 March 2024; Accepted: 26 September 2024;
Published: 15 October 2024.
Edited by:
Johann G. Zaller, University of Natural Resources and Life Sciences Vienna, AustriaReviewed by:
Amit Anil Shahane, Central Agricultural University, IndiaSneh Gautam, G. B. Pant University of Agriculture and Technology, India
Copyright © 2024 Mishra, Tiwari, Maurya and Sharma. This is an open-access article distributed under the terms of the Creative Commons Attribution License (CC BY). The use, distribution or reproduction in other forums is permitted, provided the original author(s) and the copyright owner(s) are credited and that the original publication in this journal is cited, in accordance with accepted academic practice. No use, distribution or reproduction is permitted which does not comply with these terms.
*Correspondence: Ajay Kumar Mishra, a.k.mishra@irri.org; Sheetal Sharma, sheetalhpkvv@gmail.com