- 1Harbin Normal University, Harbin, Heilongjiang, China
- 2Key Laboratory of Mollisols Agroecology, Northeast Institute of Geography and Agroecology, Chinese Academy of Sciences, Harbin, Heilongjiang, China
- 3College of Resources and Environment, Jilin Agricultural University, Changchun, China
Strip tillage is an effective tillage technique, which included the strip seedbed tilled area (ST-IR) and the straw-covered area between the seedbeds (ST-BR). However, soil hydrothermal conditions and soil disturbance varies with strip tillage widths, which might affect crop growth and yields. Therefore, this study explored the effect of strip tillage width on the soil hydrothermal conditions and soil structure of ST-IR and ST-BR, to determine the optimal strip tillage width. A field experiment with three replicates was conducted to analyze soil moisture and soil temperature variation and to clarify the difference of soil structure in the IR and BR. Three strip tillage widths, 20 cm (ST20), 30 cm (ST30), and 40 cm (ST40), were examined in this study. Soil temperature in both the ST-IR and ST-BR increased as the strip tillage width increased, and the average soil temperature of ST30-IR and ST40-IR in the seedling stage was 2.11°C and 2.62°C higher than that of ST20-IR, respectively. Moreover, the daily soil temperature range in both the IR and BR was greater for ST40 than for ST20 and ST30 in the seedling stage; soil temperature differences in other crop growth stages were small. No significant difference in soil temperature was observed between ST30-IR and ST40-IR. Conversely, soil moisture was greater in ST20-IR and ST30-IR than in ST40-IR; soil moisture was also greater in ST30-BR and ST40-BR than in ST20-BR during the monitoring period. Soil moisture in ST40-IR and ST20-BR increased and decreased more rapidly during rainfall events than in the other treatments. Moreover, soil temperature was higher in the ST-IR than in the ST-BR for all 3 strip tillage widths, and the opposite results were observed for soil moisture. In addition, strip tillage widths had no significant differences on soil structure. In conclusion, a 30 cm strip tillage width had higher soil temperature and remained more soil moisture than other strip tillage widths, so this strip tillage width is thus recommended for use by farmers in Northeast China.
1 Introduction
Tillage is a critically important agricultural management technique for improving soil properties and grain production (Munkholm et al., 2013; Pires et al., 2017). However, long-term conventional tillage practices (CT), such as rotary tillage and moldboard plowing, can increase soil erosion and decrease soil productivity (Bessam and Mrabet, 2003; Reicosky, 2015; Carretta et al., 2021). To address these problems, many studies have reported that conservation tillage measures, which leave at least 30% of the crop residues on the soil surface, and crop rotation can improve soil quality and control soil erosion (Farooq and Siddique, 2015; Blevins et al., 2018; Klik and Rosner, 2020; Chen et al., 2024).
The Mollisols region of Northeast China is a major soybean and corn-producing region. Recently, CT has been shown to lead to soil degradation, and the on-going soil degradation has an obvious influence on soil quality and crop yields in this region (He et al., 2010; Liu et al., 2010). Ensuring the national food security by rational use of cultivated land is an important challenge in the Mollisols region of Northeast China. Therefore, a shift from CT to conservation tillage is needed to improve soil quality and reduce soil loss (Liu et al., 2010; Xu et al., 2018). In this region, many studies have reported that conservation tillage obviously increased soil water storage, and long-term conservation tillage can improve soil aggregate structure and soil organic carbon content, compared with CT (Li et al., 2019; Zhang et al., 2012; Zhang et al., 2015; Zhou et al., 2022; Zhang et al., 2018). However, some studies have shown that conservation tillage with straw mulching (e.g., no-tillage) reduces soil temperature by 1.5°C–1.8°C in spring and increases soil penetration resistance (Chen et al., 2011; Blanco-Canqui and Ruis, 2018; Shen et al., 2018; Chen et al., 2021; Li et al., 2022). Chen et al. (2011) found that short-term no-tillage reduces maize yields by up to 30%. Li et al. (2022) also observed the maize yields of no tillage with 100% residue coverage was 10.35% less than conventional tillage. Due to crop production losses and their economic benefits reduction, no tillage is hard to be popularized and applied by the farmers in this region, which significantly limit the wide use of no tillage.
Strip tillage (ST) is an effective conservation tillage measure for increasing the seed emergence percentage and maize yields by 8.34% and by 2.82%, respectively, compared with no-tillage in the Mollisols region of Northeast China (Chen et al., 2021). It divides the soil into a strip seedbed zone with no straw mulching (ST-IR) and a straw-covered zone between the seedbeds (ST-BR). The ST-IR is worked mechanically down to a depth of 10–20 cm to enhance the microclimate and surface microtopography for seed emergence and crop growth, and the undisturbed, straw-covered zone between the seedbeds is untilled (Morrison, 2002; Celik et al., 2013). Hence, ST combines the main advantages of no-tillage and conventional tillage. Due to straw mulching in ST-BR, ST can reduce soil evaporation and increase soil moisture, and straw mulching also can increase surface roughness reduce raindrop impact, which led to reduction of soil loss compared with conventional tillage (Al-Kaisi and Licht, 2004; Carretta et al., 2021; Chen et al., 2021). In addition, ST also can increase the surface soil temperature during the seedling period, and seed germination and soil structure are enhanced under ST compared with under no-tillage, as a result of soil loosening in ST-IR (Al-Kaisi and Licht, 2004; Licht and Al-Kaisi, 2005a; Licht and Al-Kaisi, 2005b; Celik et al., 2013; Pöhlitz et al., 2018). Several studies have reported that crop yields are higher under ST than under no-tillage, but crop yields under ST are slightly lower or similar to those under conventional tillage (Opoku et al., 1997; Vetsch and Randall, 2002; Lamm and Aiken, 2007; Chen et al., 2021).
Soil moisture and soil temperature conditions are the important factors affecting seed germination and crop growth (Roberts et al., 2005; Ramakrishna et al., 2006). Soil temperature has a significant effect on soil evaporation, soil aeration, and soil microbial activity (Licht and Al-Kaisi, 2005a; Licht and Al-Kaisi, 2005b; Gharabaghi et al., 2015; Jahanfar et al., 2018). Soil moisture is also a key factor affecting soil heat flow and crop growth and yields (Zhang et al., 2009; Holzman et al., 2014). Meanwhile, better soil structure can improve the ability of crop roots to grow and to supply the leaves with water and nutrients (Passioura, 1991; Zhang et al., 2021; Hartmann and Six, 2023).
Therefore, clarifying the effects of ST on soil water–heat conditions and soil structure is important for optimizing the efficacy of ST practices. Celik et al. (2013) found that differences in strip tillage widths affected soil physical properties, seed emergence, and crop yields. In addition, the soil hydrothermal environment and soil structure might differ in ST-IR and ST-BR for different strip tillage widths due to differences in soil disturbance and surface cover. Therefore, identifying the optimum strip tillage width is essential for the application of ST in the Mollisols region of Northeast China. The aims of this study were (1) to characterize the changes in the soil temperature and soil moisture of ST-IR and ST-BR, and (2) to investigate the differences in soil structure during the crop growing season.
2 Materials and methods
2.1 Field experiments
Field experiments were carried out at the Hailun Monitoring and Research Station of Soil and Water Conservation, CAS, located in Hailun, Heilongjiang Province (47°21′N, 126°50′E) from May to October in 2022. This region was characterized by a typical sub-humid climate with mean annual precipitation of 530 mm, mean annual temperature of 1.5°C, and mean annual accumulated temperature of (>0°C) 3,550°C. The soil of the experimental field is a Mollisol according to the USDA classification. The soil properties (0–20 cm) are presented in Table 1.
Three strip tillage width treatments (20 cm, 30 cm, and 40 cm) were established in 2021, and the experiment was conducted in a randomized complete block design with three replicates per plot (5 m × 7 m). For each treatment, the maize straw was crushed and then covered evenly on the soil surface in the region of ST-BR in October after harvest, and the biomass was approximately 8.0 t hm–2. In 2022, maize was planted in the region of ST-IR by artificial sowing in May 1st. No other management measures were applied in all the plots during the other crop growth stage. The plant density was 4.8 plants per m2, and the fertilizer applied comprised 138 kg N·hm–2, 51.75 kg P·hm–2, and 15 kg K·hm–2. The herbicides acetochlor and thifensulfuron-methyl were applied before and after seeding to control weeds. Any remaining weeds were removed manually.
2.2 Measurement of soil moisture and temperature
The soil moisture and soil temperature of ST-IR and ST-BR were measured every 30 min in each treatment using an SMEC 300 device (WaterScout, Graton, CA, United States) at the depth of 5 cm, 10 cm and 20 cm, respectively. Soil temperature and soil moisture in the 0–20 cm depth were calculated as the average values of three monitoring soil layers. Data on air temperatures and the amount of rainfall in the experimental area were collected from the weather station near the plots. The time interval of air temperature data collection was consistent with that of soil temperature.
2.3 Measurement of soil physical properties
After harvest in 2022, bulk density (BD) for each plot was measured by using a steel cylinder measuring 5 cm in diameter and 5 cm high, and repeated three times. Soil samples were dried at 105°C for at least 8 h and then weighed. The mean BD results from the 0–5 cm, 5–10 cm and 13–18 cm cylinders were used to represent the 0–20 cm layers.
Three random soil samples of 0–20 cm depth were collected by the self-made sampling shovel, and placed in the self-sealing plastic bags. All the samples were air dried and crop roots were removed. As aggregate stability is measured on various aggregate sizes depending on the high vacuum slow wetting method (Sun et al., 2014). Three aggregate size classes (2 mm, 0.25 mm, and 0.053 mm) have been used in order to test the influence of initial aggregate size on the size distribution of breakdown fragments. The mass of each of four aggregate fractions (<0.053 mm, 0.053–0.25 mm, 0.25–2 mm and >2 mm) was recorded as Wwi, and the relative percentage was calculated as:
Wi was the proportion of the weight of the size aggregates fraction, and the formula is as follows:
The mean weight diameter (MWD) was calculated as:
Where Xi is the mean particle diameter (mm) of the ith size class, the mean particle diameter was taken as the arithmetic mean of the upper and lower sieve sizes.
2.4 Statistical analysis
All data were analyzed using a one-way ANOVA, and the significance of differences in means was evaluated using Tukey tests with SPSS analytical software 22.0 (SPSS Inc., Chicago, Illinois, United States). Multiple comparisons were performed using the least significant difference tests (p < 0.05). Figures were generated using Origin 2022 software.
3 Results
3.1 Seasonal variation in soil moisture
In this study region, soil moisture plays an important role in crop growth, especially in the seedling stage, due to less rainfall. Average soil moisture in the ST-IR was significantly greater with a 20 cm strip tillage width (ST20-IR) and 30 cm strip tillage width (ST30-IR) than with a 40 cm strip tillage width (ST40-IR) during the growth stages (Figure 1A; Figure 2A). Soil moisture was 4.14% and 6.15% higher in the seedling stage (from May 8th to June 18th), 7.01% and 7.96% higher in the jointing stage (from June 19th to July 16th), 6.45% and 7.61% higher in the filling stage (from July 16th to August 29 th), and 6.83% and 6.60% higher in the mature stage (from August 30th to October 1st) in ST40-IR than in ST20-IR and ST30-IR, respectively. Soil moisture varied with the amount of rainfall, but little differences in soil moisture among strip tillage width treatments were observed when rainfall events occurred. However, in the absence of rain, soil moisture decreased more rapidly in ST40-IR than in the other treatments. For example, from May 25th to May 29th (Figure 1A), the average soil moisture of ST40-IR in this period was 5.40% and 7.33% lower than that of ST20-IR and ST30-IR, respectively.
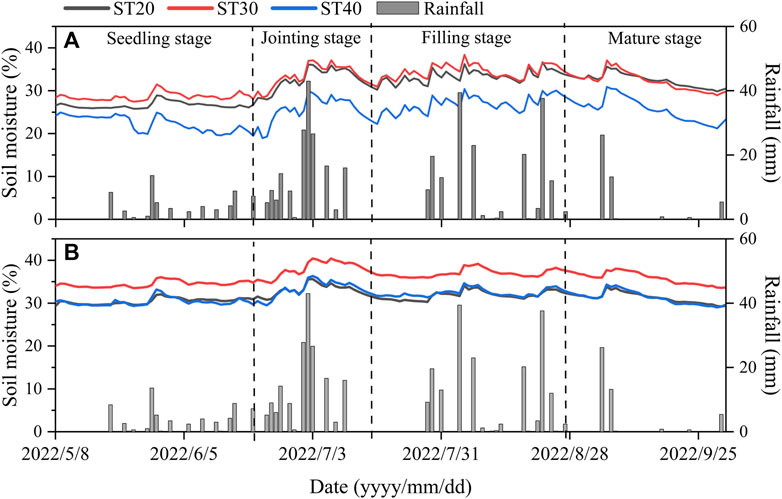
Figure 1. Variation in soil moisture in the seedbeds (A) and between the seedbeds (B) in the growth stages. ST20, strip width 20 cm; ST30, strip width 30 cm; ST40, strip width 40 cm.
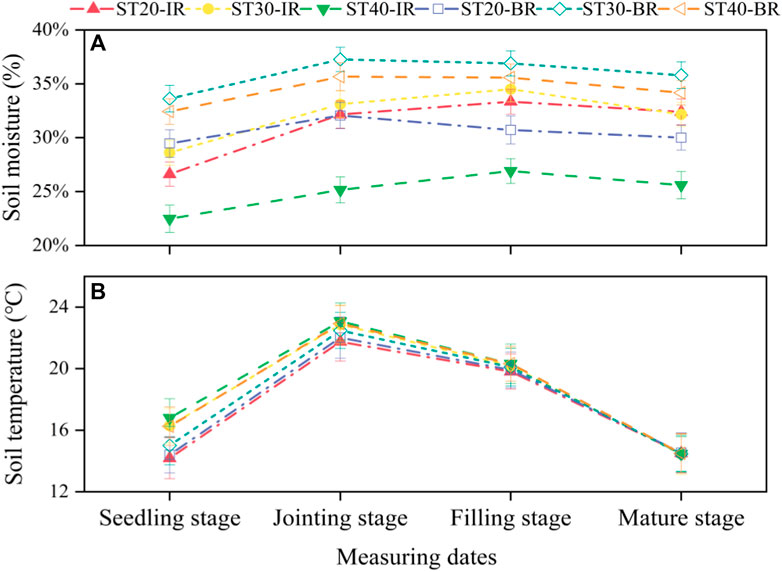
Figure 2. Soil moisture (A) and soil temperature (B) of the seedbeds (IR) and between the seedbeds (BR) in the crop growing season. ST20, strip width 20 cm; ST30, strip width 30 cm; ST40, strip width 40 cm.
Average soil moisture was significantly higher in ST30-BR and ST40-BR than in ST20-BR (Figure 1B; Figure 2A). Average soil moisture was 5.80% and 4.17% higher in ST30-BR and ST40-BR than in ST20-BR in the mature stage. The smallest difference in soil moisture among all treatments was observed in the seedling stage. ST30-BR had the greatest soil moisture in the growth stage, with the exception of the heavy rainfall events. Three heavy rainfall events occurred on July 1st, August 5th, and September 5th; soil moisture was 1.37%, 4.30%, and 1.21% higher in ST40-BR than in ST30-BR on July 1st, August 5th, and September 5th, respectively. The soil moisture of BR was higher than that of IR in all treatments, especially for ST40. Average soil moisture was 9.42% higher in ST40-BR than in ST40-IR during the growing period.
3.2 Seasonal variation in soil temperature
The soil temperature of ST-IR varied with air temperature in all treatments, especially in the seedling stage (Figure 2B; Figure 3A). The soil temperature of ST-IR increased and decreased more rapidly as the strip tillage width increased compared with ST-BR. During the whole growth period, the average soil temperature in ST40-IR was 1.14°C and 0.24°C higher than that in ST20-IR and ST30-IR, respectively. Furthermore, large differences in soil temperature among these treatments were observed in the seedling stage, but little difference was observed in the mature stage. The average soil temperature of ST40-IR in the seedling stage was 2.62°C and 0.51°C higher than that of ST20-IR and ST30-IR, respectively.
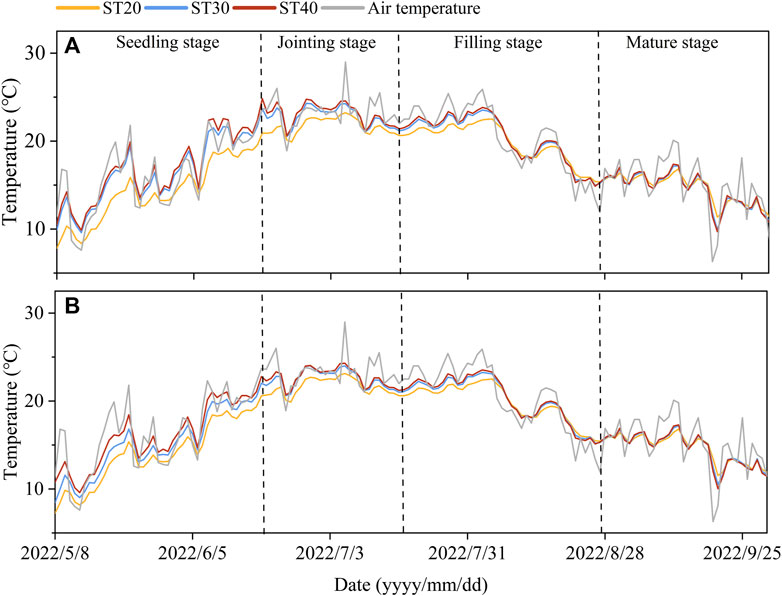
Figure 3. Variation in soil temperature in the seedbeds (A) and between the seedbeds (B) at different growth stages. ST20, strip width 20 cm; ST30, strip width 30 cm; ST40, strip width 40 cm.
The soil temperature of ST-BR varied with air temperature, but the range of variation in soil temperature was lower for ST-BR than for ST-IR in the three treatments. During the whole growth period, the average soil temperature was higher in ST40-BR than in the other treatments (Figure 2B; Figure 3B). For example, on September 20th, the minimum soil temperature of ST40-BR was 1.53°C and 0.71°C lower than that of ST20-BR and ST30-BR, respectively. The soil temperature range was higher for ST40-BR than for ST20-BR and ST30-BR. The largest difference in soil temperature among treatments was observed in the seedling stage, and average soil temperature was 1.85°C and 1.25°C higher in ST40-BR than in ST20-BR and ST30-BR, respectively. Soil temperature slightly varied among treatments in the mature stage. Additionally, the average soil temperature of ST30-IR and ST40-IR was greater than that of ST30-BR and ST40-BR, respectively. The soil temperature of ST20-BR was slightly higher than that of ST20-IR during the growing period.
The response of soil moisture to soil moisture during the no rainfall period (May 14th to May 18th) are shown in Figure 4. Soil moisture of ST-IR and ST-BR decreased with the increasing soil temperature for all the strip tillage widths in the form of linear function (y = −a·x + b). The relationship was negative for all the treatments, but was not significant. In addition, the a value of the ST-IR was greater than that of ST-BR, which indicated that soil moisture of ST-IR varied greatly with soil temperature than that of ST-BR.
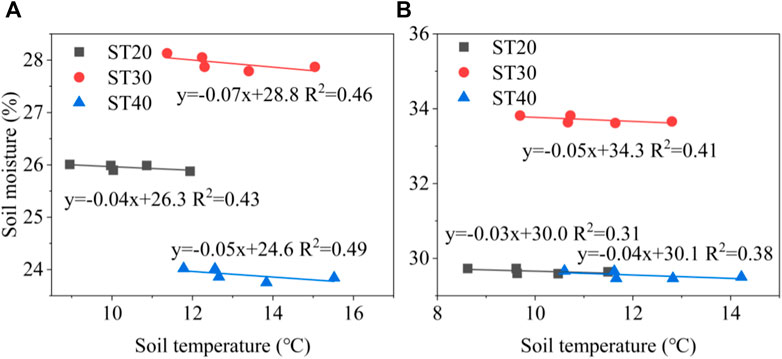
Figure 4. Relationships between soil moisture and soil temperature of the seedbeds (A) and between the seedbeds (B). ST20, strip width 20 cm; ST30, strip width 30 cm; ST40, strip width 40 cm.
3.3 Variation in soil moisture during rainfall events
During the monitoring period (July 2nd), changes in soil moisture under different strip tillage widths were analyzed during three continuous rainfall events (RE): RE1 (6.8 mm), RE2 (12.0 mm), and RE3 (5.4 mm) (Figure 5). Soil moisture in ST20-IR, ST30-IR, and ST40-IR was increased by 10.3%, 4.8%, and 11.4%, respectively, during RE1. Soil moisture in ST40-IR (ranging from 38.9% to 31.4%) decreased slightly more rapidly than that in ST20-IR (ranging from 44.0% to 37.0%) and ST30-IR (ranging from 39.8% to 37.8%) after RE1. Similar results were observed in RE2 and RE3. During the three rainfall events, average soil moisture was 6.2% and 7.1% higher in ST20-IR and ST30-IR than in ST40-IR, respectively.
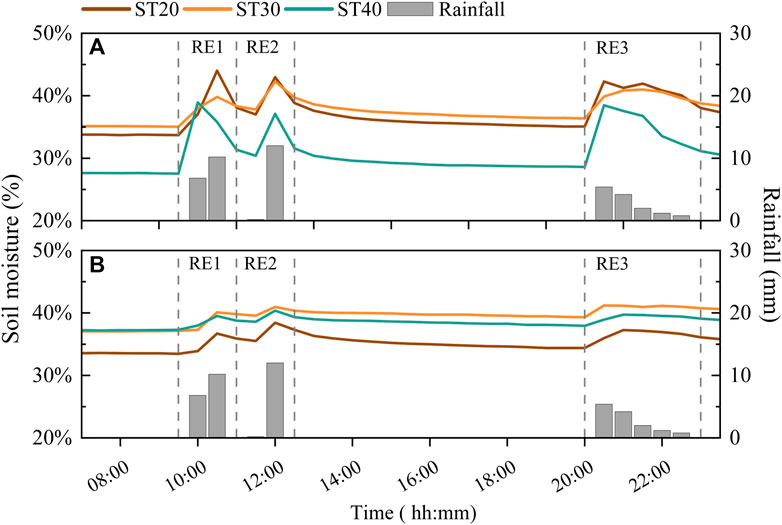
Figure 5. Variation in soil moisture of the seedbeds (A) and between the seedbeds (B) under different strip tillage widths during rainfall events. ST20, strip width 20 cm; ST30, strip width 30 cm; ST40, strip width 40 cm.
During the monitoring period, average soil moisture in ST20-BR, ST30-BR, and ST40-BR was 34.85%, 38.84%, and 38.18%, respectively. Soil moisture increased and decreased more rapidly during all rainfall events in ST20-BR than in ST30-BR and ST40-BR (Figure 5B). The average soil moisture in ST20-BR, ST30-BR, and ST40-BR increased by 3.01%, 2.08%, and 1.92%, respectively. After rainfall events, soil moisture decreased by 1.21%, 0.47%, and 0.82% in ST20-BR, ST30-BR, and ST40-BR, respectively. In this study region, rainfall is the major factor affecting soil moisture variability, due to no irrigation. Hence, maintaining higher soil moisture during the rainfall event is good for crop coots to absorb more water and nutrients, and thus improve crop growth and yields.
Furthermore, average soil moisture in ST20-IR, ST30-IR, and ST40-IR was 3.0%, 1.4%, and 5.9% greater than that in ST20-BR, ST30-BR, and ST40-BR, respectively. The highest value of soil moisture in ST-IR were observed earlier in the experimental period than that in ST-BR for all the strip tillage width treatments.
3.4 Daily changes in topsoil temperature
Figure 6 shows daily variation in soil temperature and air temperature under different strip tillage widths on May 20th. Air temperature ranged from 10.0°C to 30.4°C, with an average of 19.7°C. Daily soil temperature ranges were 11.87°C–15.63°C for ST20-IR, 12.03°C–21.40°C for ST30-IR, and 12.33°C–22.03°C for ST40-IR. The maximum values of soil temperature in ST20-IR, ST30-IR, and ST40-IR were observed at 17:30, 13:30, and 13:00, respectively. The mean daily soil temperature was 2.84°C and 3.82°C higher in ST30-IR and ST40-IR than in ST20-IR, respectively.
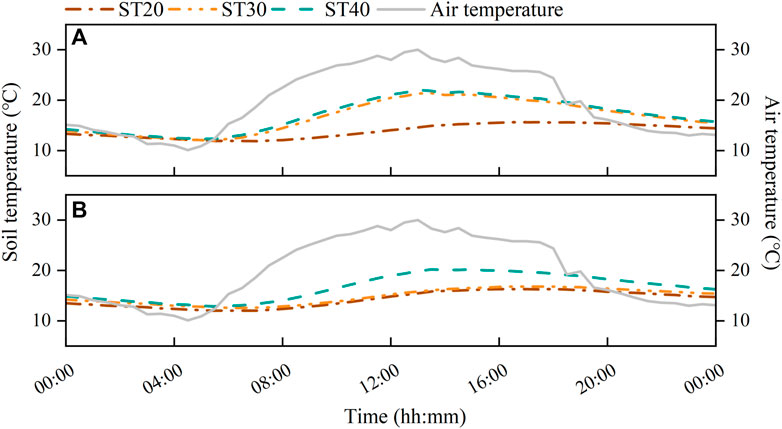
Figure 6. Daily variation in soil temperature of the seedbeds (A) and between the seedbeds (B) under different strip tillage widths during the seedling stage (in May 20th). ST20, strip width 20 cm; ST30, strip width 30 cm; ST40, strip width 40 cm.
Little variation in soil temperature was observed between the seedbeds for ST20 and ST30 compared with the soil temperature of the seedbeds. Soil temperature ranged from 12.01°C to 16.32°C, 12.57°C–16.80°C, and 12.87°C–20.20°C for ST20-BR, ST30-BR, and ST40-BR, respectively. The maximum values of soil temperature in ST20-BR, ST30-BR, and ST40-BR were observed at 16:30, 17:00, and 13:30, respectively. The minimum values of soil temperature in ST20-BR, ST30-BR, and ST40-BR were observed at 6:30, 6:30, and 5:30, respectively. The daily mean soil temperature of ST40-BR was 2.40°C and 1.87°C greater than that of ST20-BR and ST30-BR, respectively.
3.5 Soil structure
As shown in Table 2, 3 strip tillage widths did not significantly affect the BD of ST-IR and ST-BR, and its value ranged from 1.32 g cm−3 to 1.39 g cm−3. Meanwhile, it was found that the BD of ST-BR was slightly higher than that of ST-IR for each treatment.
Similar to BD, strip tillage widths had no significant effect on water stable aggregate distribution. In both ST-IR and ST-BR, the highest proportion of soil aggregate was observed in the 0.25–2 mm size fractions. Meanwhile, no significant differences of MWD were observed among the different strip tillage widths. Furthermore, for each treatment, the >2 mm aggregate size fractions of ST-BR were slightly lower than that of ST-IR, but the MWD values exhibited the opposite trend.
4 Discussion
4.1 Soil moisture
Soil moisture and soil temperature have major effects on the formation and development of soil, the movement of materials and energy in the soil, and the growth of crops. Conservation tillage with straw mulching can improve soil structure and soil water conservation, control variation in soil temperature and moisture, and enhance soil quality (Li et al., 2019; Chen et al., 2021; Dai et al., 2021; Tian et al., 2023). Previous studies (Licht and Al-Kaisi, 2005a; He et al., 2010; Pires et al., 2017; Rai et al., 2019; Song et al., 2019) have reported that clarifying variation in soil moisture and temperature is essential for evaluating the effects of ST on the soil environment. Differences in strip tillage widths have a marked effect on soil water–heat characteristics and crop yield (Celik et al., 2013). Our study found that strip tillage width had a significant effect on soil moisture in seedbeds and between seedbeds during the crop-growing season (Figure 1). Soil moisture was significantly greater in ST20-IR and ST30-IR than in ST40-IR, and the opposite patterns were observed between the seedbeds. These results could be attributed to (1) soil evaporation in the seedbeds increasing as the strip tillage width increases due to the lack of straw mulching and the high intensity of soil disturbance, which reduces the soil water content of ST-IR. These results were consistent with Liao et al. (2021). They found that mulching increased infiltration after rainfall and it can effectively reduce soil evaporation, and a positive significant correlation was found between evaporation and soil moisture. (2) The surface biomass after harvest was used in three treatments in this study; thus, the thickness of straw mulch between the seedbeds increased as the strip tillage width increased. A thick layer of straw mulch can reduce soil surface net radiation and soil evaporation, increase soil surface roughness and soil infiltration rate, which lead to greater soil moisture (Diaz et al., 2005; Dahiya et al., 2007; Qiu et al., 2014). Therefore, the average soil moisture was significantly higher in ST30-BR and ST40-BR than in ST20-BR during the growing season. Furthermore, our study revealed that the average soil moisture in ST30-BR was similar to that in ST40-BR, which might be related to soil water transport between ST-IR and ST-BR.
Many studies have shown that soil moisture is affected by rainfall conditions (Roberts et al., 2005; Wang et al., 2018). Similar results were observed in our study; specifically, our findings indicate that soil moisture in all three treatments varied with the amount of rainfall during the monitoring period. After rainfall, the rise and fall in soil moisture was more pronounced in ST40-IR than in ST20-IR and ST30-IR (Figure 5). The soil moisture range was greater in ST20-BR than in the other treatments. Furthermore, the soil moisture of ST-IR was significantly lower than that of ST-BR. These results indicate that straw mulching is an effective measure for increasing soil moisture by promoting the transmission and retention of water. Many studies have shown that straw mulching can promote soil infiltration, reduce water evaporation, protect the soil surface from the effects of raindrops, and reduce splash erosion (Fabrizzi et al., 2005; Ramakrishna et al., 2006; Liao et al., 2021).
4.2 Soil temperature
Compared with no-tillage, ST enhanced seed emergence and crop yields by creating loose seedbeds and increasing soil temperature, especially in our study region, which experiences a cold and dry climate during the spring (Chen et al., 2021; Dou et al., 2024). Licht and Al-Kaisi (2005a) found that the soil temperature was lower (by 1.2°C–1.5°C) under no-tillage than under ST and chisel tillage at a depth of 5 cm. A high seedbed soil temperature is important for seed emergence. Soil thermal diffusivity was higher under no-tillage practices than in tilled soil (Abu-Hamdeh, 2000; Fabrizzi et al., 2005; Blanco-Canqui and Ruis, 2018). The results of our study found that the three different strip tillage width treatments had a significant effect on soil temperature in seedbeds and between seedbeds (Figure 3). Soil temperature in ST40-IR increased and decreased more rapidly with air temperature than in the other treatments, and the daily ranges of soil temperature were highest in ST40-IR. The greatest differences in soil temperature among the three treatments were observed in the seedling stage; soil temperature in ST40-IR was 2.51°C and 0.52°C higher than that in ST20-IR and ST30-IR, respectively. However, these results were not consistent with those of Celik et al. (2013). They observed that the average seedbed soil temperature did not significantly differ among strip tillage width treatments during the seed emergence period. This might stem from differences in climate conditions, as spring in our study region is characterized by large differences in day and night air temperatures. Large differences in soil moisture in the seedbeds were observed in our study, and no significant differences in soil moisture were observed among the different strip tillage width treatments in Celik et al. (2013). Several studies have found that the effect of soil moisture on soil temperature can be attributed to its ability to increase soil thermal conductivity (Al-Kayssi et al., 1990; Obia et al., 2020). Furthermore, our results showed that soil temperature increased as the strip tillage width increased; these findings are consistent with those of Celik et al. (2013). This indicates that the area of soil disturbance increases as the strip tillage width increases. Soils disturbed by tillage show increased soil surface roughness and have more air pockets, which accelerates soil drying and heating when evaporation occurs (Morris et al., 2010; Schwartz et al., 2010).
Similar to ST-IR, the average soil temperature of ST40-BR was higher than that of ST20-BR and ST30-BR during the whole growth period, especially in the spring. These results indicate that the heat flow between ST-IR and ST-BR had no effect on the thickness of straw mulch between the seedbeds and that the strip tillage width and soil disturbance explained the variation in average soil temperature. Moreover, the average soil temperature of the seedbeds was significantly greater than that between the seedbeds for ST30 and ST40, and differences in the average soil temperature were small in the ST20 treatments. Generally, disturbed soil with no straw mulching in the seedbeds is much warmer and drier due to the pores, which facilitate the circulation of air, compared with undisturbed areas covered with residue (Abu-Hamdeh, 2000; Chang et al., 2020; Dai et al., 2021). Meanwhile, some studies have found that tillage can reduce soil aggregate size and stability; given that soil aggregates have a lower heat capacity and greater heat conductivity than water, dry soils typically warm and cool faster than wet soils (Licht and Al-Kaisi, 2005a; Licht and Al-Kaisi, 2005b; Blanco-Canqui and Ruis, 2018).
In addition, our study also found that soil moisture was negative related to soil temperature (Figure 4), and soil moisture of ST30-BR and ST40-BR was significantly higher than that of ST30-IR and ST40-IR, which might be a major factor for the lower soil temperature of ST-BR. Previous studies have reported that low soil temperature could inhibit germination and seedling growth of crop (Bhattacharyya and De Datta, 1971; Bollero et al., 1996; Chen et al., 2007). Barlow et al. (1977) found that the changes of soil temperature by 1°C had a significant influence on crop growth. Meanwhile, low soil temperature caused the death of lower leaves and chlorosis of the upper leaves, and both vegetative growth and dry matter production were retarded, which lead to reduction in grain yields (Bhattacharyya and De Datta, 1971; White et al., 1991; Calleja-Cabrera et al., 2020).
4.3 Soil physical properties
Tillage plays a vital role in crop growth and yields by improving soil structure (Opoku et al., 1997; Dahiya et al., 2007; Song et al., 2019). However, long-term CT measures, such as moldboard rotary plowing and chisel plowing, increase soil bulk density and penetration resistance and reduce soil aggregate stability, can directly lead to land degradation and enhance weed growth, and ultimately reduce crop yield (Alvarez and Steinbach, 2009; Chen et al., 2011; Sithole et al., 2016; Chen et al., 2021). Compared with CT, long term ST had a significant enhancement in soil pore and aggregate structure, which conducive to solute transport and root growth (Chen et al., 2011; Han et al., 2024). In this study, only the soil disturbance areas of three treatments were different and other soil management practices were same. Therefore, our study indicated that strip tillage widths did not significantly affect soil bulk density, aggregate distribution and stability.
Furthermore, differences in soil structure were observed between ST-IR and ST-BR. The BD of ST-IR was lower than that of ST-BR, due to the seedbed loosening after harvest (Kukal and Aggarwal, 2003; Jaskulska et al., 2020). Meanwhile, soil aggregate stability of ST-IR was slightly greater than that of ST-BR. Similar to no-tillage with no soil disturbance and straw mulching, short-term ST increased soil compaction and reduced soil aggregate stability of ST-BR. However, no significant differences in BD and soil aggregate stability were found between ST-IR and ST-BR. This could be due to the short duration of this study and sampling time (in October, the mature stage). Although loosening the soils of the seedbeds causes the lower soil bulk density in the seedling stage, the soil bulk density increased with the growing season due to the influence of rainfall and soil compaction (Karunatilake and Van Es, 2002; Keller et al., 2019). Tian et al. (2023) found that no significant difference of BD was between CT and NT in the mature stage, meanwhile, no significant differences in aggregate stability was observed in NT than CT. These results indicated that short-term mulching and soil disturbance treatments had a slight influence on soil structure. The land management practices of ST-IR and ST-BR was similar to that of CT and NT, respectively. Hence, no significant difference in soil structure was found between ST-IR and ST-BR. In addition, Li et al. (2019) reported that long-term NT could improve soil aggregate stability and decrease the BD with the increasing experimental period. They also found that conservation tillage practices positively affected many soil physical properties. In the similar region, Chen et al. (2021) also confirmed that long-term strip tillage can improve soil structure of ST-BR than that of ST-IR, as a result of straw mulching.
5 Conclusion
The results of this study demonstrated that the soil temperature of the seedbeds and between the seedbeds increased as the strip tillage width increased during the growing stage, and the greatest difference among the 3 strip tillage width treatments was observed in the seedling stage. The average soil temperature in ST40-IR was 2.62°C and 0.51°C higher than that in ST20-IR and ST30-IR, respectively, and the mean soil temperature was 1.85°C and 1.25°C higher in ST40-BR than in ST20-BR and ST30-BR, respectively. The daily warming rate and maximum values of soil temperature were greater in ST30-IR and ST40-IR than in ST20-IR. The soil moisture of IR decreased as the strip tillage width increased. During the whole growth stage, the average soil moisture was 6.61% and 7.08% higher in ST20-IR and ST30-IR than in ST40-IR, respectively. However, for BR, average soil moisture was higher in ST30 than in the other treatments, and ST40 had the greatest soil moisture during heavy rainfall events. Furthermore, soil moisture increased and decreased more rapidly in ST40-IR and ST20-BR during rainfall events compared with the other treatments. The soil moisture of BR was higher than that of IR in the 3 strip tillage width treatments, and the opposite patterns were observed for soil temperature. In addition, strip tillage widths had no significant effect on soil physical properties. In a word, we concluded that a strip tillage width of 30 cm can maintain a high soil temperature of IR in the spring and better conserve soil moisture. For local farmers and land managers, a strip width of 30 cm is thus recommended when conversion of conventional tillage to strip tillage in the Mollisols region of Northeast China.
This study confirmed that short-term ST can improve the soil temperature of the seedbeds and maintain high moisture. However, due to the single-year duration, we need to further explore the effect of long-term different strip tillage widths on soil properties and crop yields, and determine the suitable strip tillage width for different crops.
Data availability statement
The original contributions presented in the study are included in the article/supplementary material, further inquiries can be directed to the corresponding author.
Author contributions
CW: Data curation, Writing–original draft, Investigation, Methodology, Project administration, Supervision. SA: Formal Analysis, Investigation, Writing–original draft. QC: Conceptualization, Data curation, Funding acquisition, Investigation, Methodology, Resources, Writing–review and editing. JL: Investigation, Methodology, Resources, Validation, Writing–review and editing. JD: Investigation, Project administration, Writing–original draft. FY: Data curation, Formal Analysis, Methodology, Resources, Writing–review and editing.
Funding
The author(s) declare that financial support was received for the research, authorship, and/or publication of this article. This study was funded by the National Natural Science Foundation of China (42101281); Natural Science Foundation of Heilongjiang Province of China (no. TD 2023D005).
Conflict of interest
The authors declare that the research was conducted in the absence of any commercial or financial relationships that could be construed as a potential conflict of interest.
Publisher’s note
All claims expressed in this article are solely those of the authors and do not necessarily represent those of their affiliated organizations, or those of the publisher, the editors and the reviewers. Any product that may be evaluated in this article, or claim that may be made by its manufacturer, is not guaranteed or endorsed by the publisher.
References
Abu-Hamdeh, N. H. (2000). Effect of tillage treatments on soil thermal conductivity for some Jordanian clay loam and loam soils. Soil and Tillage Res. 56 (3-4), 145–151. doi:10.1016/S0167-1987(00)00129-X
Al-Kaisi, M., and Licht, M. A. (2004). Effect of strip tillage on corn nitrogen uptake and residual soil nitrate accumulation compared with no-tillage and chisel plow. Agron. J. 96 (4), 1164–1171. doi:10.2134/agronj2004.1164
Al-Kayssi, A. W., Al-Karaghouli, A. A., Hasson, A. M., and Beker, S. A. (1990). Influence of soil moisture content on soil temperature and heat storage under greenhouse conditions. J. Agric. Eng. Res. 45, 241–252. doi:10.1016/S0021-8634(05)80152-0
Alvarez, R., and Steinbach, H. S. (2009). A review of the effects of tillage systems on some soil physical properties, water content, nitrate availability and crops yield in the Argentine Pampas. Soil and Tillage Res. 104 (1), 1–15. doi:10.1016/j.still.2009.02.005
Barlow, E. W. R., Boersma, L., and Young, J. L. (1977). Photosynthesis, transpiration, and leaf elongation in corn seedlings at suboptimal soil temperatures 1. Agron. J. 69 (1), 95–100. doi:10.2134/agronj1977.00021962006900010025x
Bessam, F., and Mrabet, R. (2003). Long-term changes in soil organic matter under conventional tillage and no-tillage systems in semiarid Morocco. Soil Use Manag. 19 (2), 139–143. doi:10.1111/j.1475-2743.2003.tb00294.x
Bhattacharyya, A. K., and De Datta, S. K. (1971). Effects of soil temperature regimes on growth characteristics, nutrition, and grain yield of IR22 rice 1. Agron. J. 63 (3), 443–449. doi:10.2134/agronj1971.00021962006300030029x
Blanco-Canqui, H., and Ruis, S. J. (2018). No-tillage and soil physical environment. Geoderma 326, 164–200. doi:10.1016/j.geoderma.2018.03.011
Blevins, R. L., Lal, R., Doran, J. W., Langdale, G. W., and Frye, W. W. (2018). Conservation tillage for erosion control and soil quality. in Advances in soil and water conservation (New York: Routledge), 51–68.
Bollero, G. A., Bullock, D. G., and Hollinger, S. E. (1996). Soil temperature and planting date effects on corn yield, leaf area, and plant development. Agron. J. 88, 385–390. doi:10.2134/agronj1996.00021962008800030005x
Calleja-Cabrera, J., Boter, M., Oñate-Sánchez, L., and Pernas, M. (2020). Root growth adaptation to climate change in crops. Front. Plant Sci. 11, 544. doi:10.3389/fpls.2020.00544
Carretta, L., Tarolli, P., Cardinali, A., Nasta, P., Romano, N., and Masin, R. (2021). Evaluation of runoff and soil erosion under conventional tillage and no-till management: a case study in northeast Italy. Catena 197, 104972. doi:10.1016/j.catena.2020.104972
Celik, A., Altikat, S., and Way, T. R. (2013). Strip tillage width effects on sunflower seed emergence and yield. Soil and Tillage Res. 131, 20–27. doi:10.1016/j.still.2013.03.004
Chang, L., Han, F., Chai, S., Cheng, H., Yang, D., and Chen, Y. (2020). Straw strip mulching affects soil moisture and temperature for potato yield in semiarid regions. Agron. J. 112 (2), 1126–1139. doi:10.1002/agj2.20103
Chen, Q., Zhang, J., Guo, M., Zhang, X., Tian, J., Zhou, P., et al. (2024). Comparison of the effects of five long-term land use and management practices on runoff, soil erosion, and nutrient loss under natural rainfall in the Mollisol region of Northeast China. Earth Surf. Process. Landforms 49, 1606–1620. doi:10.1002/esp.5789
Chen, Q., Zhang, X. Y., Sun, L., Ren, J. H., Yuan, Y. R., and Zang, S. Y. (2021). Influence of tillage on the Mollisols physicochemical properties, seed emergence and yield of maize in Northeast China. Agriculture 11 (10), 939. doi:10.3390/agriculture11100939
Chen, S. Y., Zhang, X. Y., Pei, D., Sun, H. Y., and Chen, S. L. (2007). Effects of straw mulching on soil temperature, evaporation and yield of winter wheat: field experiments on the North China Plain. Ann. Appl. Biol. 150 (3), 261–268. doi:10.1111/j.1744-7348.2007.00144.x
Chen, Y., Liu, S., Li, H., Li, X. F., Song, C. Y., Cruse, R. M., et al. (2011). Effects of conservation tillage on corn and soybean yield in the humid continental climate region of Northeast China. Soil and Tillage Res. 115, 56–61. doi:10.1016/j.still.2011.06.007
Dahiya, R., Ingwersen, J., and Streck, T. (2007). The effect of mulching and tillage on the water and temperature regimes of a loess soil: experimental findings and modeling. Soil and Tillage Res. 96 (1-2), 52–63. doi:10.1016/j.still.2007.02.004
Dai, Z., Hu, J., Fan, J., Fu, W., Wang, H., and Hao, M. (2021). No-tillage with mulching improves maize yield in dryland farming through regulating soil temperature, water and nitrate-N. Agric. Ecosyst. Environ. 309, 107288. doi:10.1016/j.agee.2020.107288
Diaz, F., Jimenez, C. C., and Tejedor, M. (2005). Influence of the thickness and grain size of tephra mulch on soil water evaporation. Agric. water Manag. 74 (1), 47–55. doi:10.1016/j.agwat.2004.10.011
Dou, S., Wang, Z., Tong, J., Shang, Z., Deng, A., Song, Z., et al. (2024). Strip tillage promotes crop yield in comparison with no tillage based on a meta-analysis. Soil and Tillage Res. 240, 106085. doi:10.1016/j.still.2024.106085
Fabrizzi, K. P., Garcıa, F. O., Costa, J. L., and Picone, L. I. (2005). Soil water dynamics, physical properties and corn and wheat responses to minimum and no-tillage systems in the southern Pampas of Argentina. Soil and Tillage Res. 81 (1), 57–69. doi:10.1016/j.still.2004.05.001
Farooq, M., and Siddique, K. H. (2015) Conservation agriculture: concepts, brief history, and impacts on agricultural systems. Springer International Publishing, 3–17.
Gharabaghi, B., Safadoust, A., Mahboubi, A. A., Mosaddeghi, M. R., Unc, A., Ahrens, B., et al. (2015). Temperature effect on the transport of bromide and E. coli NAR in saturated soils. J. Hydrology 522, 418–427. doi:10.1016/j.jhydrol.2015.01.003
Han, J., Li, J., Jiang, Y., Wang, D., Chen, Q., and Zhang, X. (2024). Effect of long-term strip tillage on soil macropore structure in cool black soil region of Northeast China. Land Degrad. Dev. 35 (1), 484–495. doi:10.1002/ldr.4931
Hartmann, M., and Six, J. (2023). Soil structure and microbiome functions in agroecosystems. Nat. Rev. Earth Environ. 4 (1), 4–18. doi:10.1038/s43017-022-00366-w
He, J., Li, H., Kuhn, N. J., Wang, Q., and Zhang, X. Y. (2010). Effect of ridge tillage, no-tillage, and conventional tillage on soil temperature, water use, and crop performance in cold and semi-arid areas in Northeast China. Soil Res. 48 (8), 737–744. doi:10.1071/SR09155
Holzman, M. E., Rivas, R., and Piccolo, M. C. (2014). Estimating soil moisture and the relationship with crop yield using surface temperature and vegetation index. Int. J. Appl. Earth Observation Geoinformation 28, 181–192. doi:10.1016/j.jag.2013.12.006
Jahanfar, A., Drake, J., Sleep, B., and Gharabaghi, B. (2018). A modified FAO evapotranspiration model for refined water budget analysis for Green Roof systems. Ecol. Eng. 119, 45–53. doi:10.1016/j.ecoleng.2018.04.021
Jaskulska, I., Romaneckas, K., Jaskulski, D., Gałęzewski, L., Breza-Boruta, B., Dębska, B., et al. (2020). Soil properties after eight years of the use of strip-till one-pass technology. Agronomy 10 (10), 1596. doi:10.3390/agronomy10101596
Karunatilake, U. P., and Van Es, H. M. (2002). Rainfall and tillage effects on soil structure after alfalfa conversion to maize on a clay loam soil in New York. Soil and Tillage Res. 67, 135–146. doi:10.1016/S0167-1987(02)00056-9
Keller, T., Sandin, M., Colombi, T., Horn, R., and Or, D. (2019). Historical increase in agricultural machinery weights enhanced soil stress levels and adversely affected soil functioning. Soil and Tillage Res. 194, 104293. doi:10.1016/j.still.2019.104293
Klik, A., and Rosner, J. (2020). Long-term experience with conservation tillage practices in Austria: impacts on soil erosion processes. Soil and Tillage Res. 203, 104669. doi:10.1016/j.still.2020.104669
Kukal, S. S., and Aggarwal, G. C. (2003). Puddling depth and intensity effects in rice–wheat system on a sandy loam soil: I. Development of subsurface compaction. Soil and Tillage Res. 72 (1), 1–8. doi:10.1016/s0167-1987(03)00093-x
Lamm, F. R., and Aiken, R. M. (2007). “Tillage and irrigation capacity effects on corn production,” in 2007 ASAE annual meeting (American Society of Agricultural and Biological Engineers), 1. doi:10.13031/2013.22942
Li, R., Zheng, J., Xie, R., Ming, B., Peng, X., Luo, Y., et al. (2022). Potential mechanisms of maize yield reduction under short-term no-tillage combined with residue coverage in the semi-humid region of Northeast China. Soil and Tillage Res. 217, 105289. doi:10.1016/j.still.2021.105289
Li, Y., Li, Z., Cui, S., Jagadamma, S., and Zhang, Q. (2019). Residue retention and minimum tillage improve physical environment of the soil in croplands: a global meta-analysis. Soil and Tillage Res. 194, 104292. doi:10.1016/j.still.2019.06.009
Liao, Y., Cao, H. X., Liu, X., Li, H. T., Hu, Q. Y., and Xue, W. K. (2021). By increasing infiltration and reducing evaporation, mulching can improve the soil water environment and apple yield of orchards in semiarid areas. Agric. Water Manag. 253, 106936. doi:10.1016/j.agwat.2021.106936
Licht, M. A., and Al-Kaisi, M. (2005a). Strip-tillage effect on seedbed soil temperature and other soil physical properties. Soil and Tillage Res. 80 (1), 233–249. doi:10.1016/j.still.2004.03.017
Licht, M. A., and Al-Kaisi, M. (2005b). Corn response, nitrogen uptake, and water use in strip-tillage compared with no-tillage and chisel plow. Agron. J. 97, 705–710. doi:10.2134/agronj2004.0102
Liu, X. B., Zhang, X. Y., and Herbert, S. J. (2010). Feeding China’s growing needs for grain. Nature 465, 420. doi:10.1038/465420a
Morris, N. L., Miller, P. C. H., Orson, J. H., and Froud-Williams, R. J. (2010). The adoption of non-inversion tillage systems in the United Kingdom and the agronomic impact on soil, crops and the environment—a review. Soil and Tillage Res. 108 (1-2), 1–15. doi:10.1016/j.still.2010.03.004
Morrison, J. E., and Jr., (2002). Strip tillage for “no–till” row crop production. Appl. Eng. Agric. 18 (3), 277. doi:10.13031/2013.8593
Munkholm, L. J., Heck, R. J., and Deen, B. (2013). Long-term rotation and tillage effects on soil structure and crop yield. Soil and Tillage Res. 127, 85–91. doi:10.1016/j.still.2012.02.007
Obia, A., Cornelissen, G., Martinsen, V., Smebye, A. B., and Mulder, J. (2020). Conservation tillage and biochar improve soil water content and moderate soil temperature in a tropical Acrisol. Soil and Tillage Res. 197, 104521. doi:10.1016/j.still.2019.104521
Opoku, G., Vyn, T. J., and Swanton, C. J. (1997). Modified no-till systems for corn following wheat on clay soils. Agron. J. 89 (4), 549–556. doi:10.2134/agronj1997.00021962008900040003x
Passioura, J. B. (1991). Soil structure and plant growth. Soil Res. 29 (6), 717–728. doi:10.1071/SR9910717
Pires, L. F., Borges, J. A., Rosa, J. A., Cooper, M., Heck, R. J., Passoni, S., et al. (2017). Soil structure changes induced by tillage systems. Soil and Tillage Res. 165, 66–79. doi:10.1016/j.still.2016.07.010
Pöhlitz, J., Rücknagel, J., Koblenz, B., Schlüter, S., Vogel, H. J., and Christen, O. (2018). Computed tomography and soil physical measurements of compaction behaviour under strip tillage, mulch tillage and no tillage. Soil and Tillage Res. 175, 205–216. doi:10.1016/j.still.2017.09.007
Qiu, Y., Xie, Z., Wang, Y., Ren, J., and Malhi, S. S. (2014). Influence of gravel mulch stratum thickness and gravel grain size on evaporation resistance. J. Hydrology 519, 1908–1913. doi:10.1016/j.jhydrol.2014.09.085
Rai, V., Pramanik, P., Das, T. K., Aggarwal, P., Bhattacharyya, R., Krishnan, P., et al. (2019). Modelling soil hydrothermal regimes in pigeon pea under conservation agriculture using Hydrus-2D. Soil and Tillage Res. 190, 92–108. doi:10.1016/j.still.2019.02.021
Ramakrishna, A., Tam, H. M., Wani, S. P., and Long, T. D. (2006). Effect of mulch on soil temperature, moisture, weed infestation and yield of groundnut in northern Vietnam. Field crops Res. 95 (2-3), 115–125. doi:10.1016/j.fcr.2005.01.030
Reicosky, D. C. (2015). Conservation tillage is not conservation agriculture. J. Soil water conservation 70 (5), 103A–108A. doi:10.2489/jswc.70.5.103A
Roberts, S. D., Harrington, C. A., and Terry, T. A. (2005). Harvest residue and competing vegetation affect soil moisture, soil temperature, N availability, and Douglas-fir seedling growth. For. Ecol. Manag. 205 (1-3), 333–350. doi:10.1016/j.foreco.2004.10.036
Schwartz, R. C., Baumhardt, R. L., and Evett, S. R. (2010). Tillage effects on soil water redistribution and bare soil evaporation throughout a season. Soil and Tillage Res. 110 (2), 221–229. doi:10.1016/j.still.2010.07.015
Shen, Y., McLaughlin, N., Zhang, X. P., Xu, M. G., and Liang, A. Z. (2018). Effect of tillage and crop residue on soil temperature following planting for a Black soil in Northeast China. Sci. Rep. 8, 4500. doi:10.1038/s41598-018-22822-8
Sithole, N. J., Magwaza, L. S., and Mafongoya, P. L. (2016). Conservation agriculture and its impact on soil quality and maize yield: a South African perspective. Soil and Tillage Res. 162, 55–67. doi:10.1016/j.still.2016.04.014
Song, K., Zheng, X., Lv, W., Qin, Q., Sun, L., Zhang, H., et al. (2019). Effects of tillage and straw return on water-stable aggregates, carbon stabilization and crop yield in an estuarine alluvial soil. Sci. Rep. 9 (1), 4586. doi:10.1038/s41598-019-40908-9
Sun, T., Chen, Q., Chen, Y., Cruse, R. M., Li, X. F., Song, C. Y., et al. (2014). A novel soil wetting technique for measuring wet stable aggregates. Soil Tillage Res. 141, 19–24. doi:10.1016/j.still.2014.03.009
Tian, J., Wu, X., Li, J., Guo, M., Zhang, X., and Chen, Q. (2023). Seasonal and long-term variability in soil structure and erodibility under different land-use patterns in the Mollisols region of NortheastNortheast China. Agronomy 13 (2), 449. doi:10.3390/agronomy13020449
Vetsch, J. A., and Randall, G. W. (2002). Corn production as affected by tillage system and starter fertilizer. Agron. J. 94 (3), 532–540. doi:10.2134/agronj2002.5320
Wang, G., Sun, W., Xue, B., and Kiem, A. (2018). Stratification response of soil water content during rainfall events under different rainfall patterns. Hydrol. Process. 32 (20), 3128–3139. doi:10.1002/hyp.13250
White, P. J., Cooper, H. D., Clarkson, D. T., Earnshaw, M. J., and Loughman, B. C. (1991). Effects of low temperature on growth and nutrient accumulation in rye (Secale cereale) and wheat (Triticum aestivum). Ann. Bot. 68 (1), 23–31. doi:10.1093/oxfordjournals.aob.a088214
Xu, X., Zheng, F., Wilson, G. V., He, C., Lu, J., and Bian, F. (2018). Comparison of runoff and soil loss in different tillage systems in the Mollisol region of Northeast China. Soil Tillage Res. 177, 1–11. doi:10.1016/j.still.2017.10.005
Zhang, S., Lövdahl, L., Grip, H., Tong, Y., Yang, X., and Wang, Q. (2009). Effects of mulching and catch cropping on soil temperature, soil moisture and wheat yield on the Loess Plateau of China. Soil Tillage Res. 102 (1), 78–86. doi:10.1016/j.still.2008.07.019
Zhang, S. X., Chen, X. W., Jia, S. X., Liang, A. Z., Zhang, X. P., Yang, X. M., et al. (2015). The potential mechanism of long-term conservation tillage effects on maize yield in the black soil of Northeast China. Soil Tillage Res. 154, 84–90. doi:10.1016/j.still.2015.06.002
Zhang, S. X., Li, Q., Zhang, X. P., Wei, K., Chen, L. J., and Liang, W. J. (2012). Effects of conservation tillage on soil aggregation and aggregate binding agents in black soil of Northeast China. Soil Tillage Res. 124, 196–202. doi:10.1016/j.still.2012.06.007
Zhang, Y., Li, X. J., Gregorich, E. G., McLaughlin, N., Zhang, X. P., Guo, Y. F., et al. (2018). No-tillage with continuous maize cropping enhances soil aggregation and organic carbon storage in Northeast China. Geoderma 330, 204–211. doi:10.1016/j.geoderma.2018.05.037
Zhang, Y., Tan, C., Wang, R., Li, J., and Wang, X. (2021). Conservation tillage rotation enhanced soil structure and soil nutrients in long-term dryland agriculture. Eur. J. Agron. 131, 126379. doi:10.1016/j.eja.2021.126379
Zhou, M., Xiao, Y., Zhang, X. Y., Xiao, L. L., Ding, G. W., Cruse, R. M., et al. (2022). Fifteen years of conservation tillage increases soil aggregate stability by altering the contents and chemical composition of organic carbon fractions in Mollisols. Land Degrad. Dev. 33, 2932–2944. doi:10.1002/ldr.4365
Keywords: strip tillage, strip width, soil temperature, soil moisture, soil structure
Citation: Wang C, Ai S, Chen Q, Li J, Ding J and Yang F (2024) Effect of strip tillage widths on soil moisture, soil temperature and soil structure in northeast China. Front. Environ. Sci. 12:1404971. doi: 10.3389/fenvs.2024.1404971
Received: 22 March 2024; Accepted: 06 May 2024;
Published: 20 May 2024.
Edited by:
Ilan Stavi, Dead Sea and Arava Science Center, IsraelCopyright © 2024 Wang, Ai, Chen, Li, Ding and Yang. This is an open-access article distributed under the terms of the Creative Commons Attribution License (CC BY). The use, distribution or reproduction in other forums is permitted, provided the original author(s) and the copyright owner(s) are credited and that the original publication in this journal is cited, in accordance with accepted academic practice. No use, distribution or reproduction is permitted which does not comply with these terms.
*Correspondence: Qiang Chen, chenqiang2016@hrbnu.edu.cn