- 1College of Life and Environmental Science, Central South University of Forestry and Technology, Changsha, China
- 2Yuelu Shan Laboratory, Changsha, China
Soil cadmium (Cd) contamination has emerged as a significant global environmental concern, posing numerous risks to individual organisms and entire ecosystems. Concurrently, the global increase in pesticide usage has elevated the influx of chloride ions (Cl−) into the soil. Given Cl−’s robust ability to coordinate and complex with various heavy metal ions, understanding its influence on the migration and transformation of Cd in soil-rice systems is essential for the rational application of pesticides and the effective mitigation of soil heavy metal pollution. In this paper, we explained the effect of Cl− on the environmental behavior of Cd in the soil-rice system in terms of growth traits, Cd uptake and accumulation by rice, and Cd solid-solution phase interface behavior through pot experiments and sand culture experiments. The results showed that Cd concentrations in all parts of the rice treated with CaCl2 during the filling period were lower than those in the Ca(NO3)2-treated group, with Cd accumulation diminishing as Cl− concentration increased. This suggests that the filling period is critical for Cd uptake and accumulation in rice. Unlike the accompanying anion NO3−, exogenous Cl− reduced Cd concentrations in the soil solution but increased them in rice. Notably, when the Cd/Cl ratio ranged from 0.625 to 2.5, Cl− formed predominantly CdCl+-complexes with free Cd2+ in the soil solution, enhancing the mobilization of Cd bound to soil particles and its subsequent absorption by rice. This study aims to assess Cl−’s effect on Cd migration and transformation in soil-rice systems, providing insights for safe rice production on Cd-contaminated soils and rational use of chlorine-containing pesticides.
1 Introduction
Cadmium (Cd) represents a significant biotoxic heavy metal and the primary contaminant in agricultural soils across China (Fu et al., 2021). Beyond natural sources, human activities constitute the primary contributors to soil Cd pollution. The extensive application of cadmium and cadmium-bearing minerals in agriculture and industry facilitates the entry of this heavy metal into agricultural soils through various pathways (Wang et al., 2019; Hussain et al., 2020). Cadmium, a non-essential metal, enters the human body primarily through the soil-food-human transfer chain (Feng et al., 2020). Compared to other heavy metals, cadmium exhibits higher mobility in soils, facilitating its absorption, transfer, and accumulation by animals, plants, and microorganisms in significant quantities. This accumulation impairs the biological functions of soil organisms and poses severe health risks to humans (Xu et al., 2020). Additionally, cadmium disrupts nutrient uptake and accumulation in plants, hinders photosynthesis, and induces oxidative stress and genetic damage, consequently retarding plant growth. Exposure to cadmium inhibits the growth of various plant parts, such as leaves, stems, and roots. For instance, 6 mg kg−1 of cadmium exposure significantly reduces the root and stem length, area, and number of rice seedlings (Song et al., 2015). Given these impacts, cadmium contamination has attracted extensive global attention in recent years.
The extensive use of agricultural chemicals has significantly contributed to the surge in annual rice production in China (Li et al., 2015; Deng et al., 2020; Huang et al., 2020). This widespread application of inorganic fertilizers introduces a diverse array of ions into the soil solution, facilitating various reactions such as precipitation, dissolution, adsorption, and desorption (Zhang et al., 2018a; Sun et al., 2019). Conversely, research indicates that the addition of other divalent cations, like manganese, zinc, or silicon, to the growth solution can reduce Cd uptake and translocation from roots to shoots in several plant species (Sterckeman et al., 2015; Ge et al., 2016). However, studies in agrochemistry have predominantly concentrated on the effects of cations on the soil environment, often overlooking the roles of the accompanying anions.
Chloride (Cl−) is a predominant anion in soils, originating from multiple sources. Soluble salts such as NaCl, MgCl2, and CaCl2, resulting from the weathering of parent material, along with Cl−-containing agricultural chemicals, represent major sources of chloride in soils (Christoph-Martin, 2019). Since 2012, Chinese agriculture has utilized approximately 11 million tons of ammonium chloride annually, a figure that continues to rise each year (Lu et al., 2019a). The consistent introduction of Cl− into the soil is significant; its strong leaching properties not only reduce the content of exchangeable Ca2+ and Mg2+ ions but also reduce the soil’s cation exchange capacity (CEC). Additionally, the negative charge of Cl− disrupts the soil solution’s neutrality, leading to reduced adsorption of other anions like SO42− and NO3− by soil particles (Zhang et al., 2016; León-Romero et al., 2017). Chloride’s limited adsorption onto soil colloids and its robust complexing capability allow it to form stable complexes with metal cations, thereby influencing the adsorption of cations by the soil (Yuan et al., 2017; Zheng et al., 2022).
Numerous studies have examined the environmental chemical behaviors of Cl− in soil, particularly its interactions with heavy metal ions such as Cd2+, Pb2+, and Zn2+. These ions can form soluble metal-Cl complexes with chloride, thereby increasing the dissolution of heavy metals into the soil solution. Elevated ionic strength in the soil solution has been shown to facilitate a greater release of Cd compared to other metals (Acosta et al., 2011; Zhai et al., 2018; Li et al., 2019a). Specifically, Zhang et al. (2018a) demonstrated that Cl− forms highly soluble Cd-chloro complexes (CdCln2−n) with Cd2+, enhancing Cd’s bioavailability in the soil, which leads to increased Cd absorption by C. rossii. Contrarily, some researchers argue differently. Ishtiyaq et al. (2023) observed that Cl− could enhance A. halimus’s resistance to Cd toxicity by reducing heavy metal uptake and boosting the synthesis of osmoprotective compounds, a finding echoed by (Filipović et al., 2018).
Rice (Oryza sativa L.), a crop cultivated since ancient times and a principal staple in China, exhibits an average consumption rate of 219 g/capita/day—nearly 50% higher than the global average of 148 g/capita/day (Hu et al., 2016; Lu et al., 2019b). Notably, Hunan Province, a major rice-producing region, also ranks among the provinces most severely contaminated with Cd. Results indicate that the Yangtze River basin, especially Hunan, required more attention due to the elevated Cd concentrations in soil-rice ecosystems (Zou et al., 2021). This study aims to investigate the quantitative relationship between Cl− and Cd interactions. To our knowledge, the effects of Cl− on the interface behavior of Cd in the soil-water medium and its migration in rice remain unexplored. Thus, we analyzed the morphology of Cd in soil, the fluctuations in Cd concentrations in the soil solution, and the Cd levels in rice at various growth stages under different Cl− concentrations during a rice pot experiment. The soil solution was collected via field capacity-derived extraction, and the Cd concentration in the soil liquid phase was measured under ambient conditions to explore Cl−’s influence on Cd transformation in the soil liquid phase. Our findings clarify the impact of Cl− on Cd bioavailability within the soil-rice system and unveil the underlying mechanisms, providing insights for the rational use of agricultural chemicals in farmland management and heavy metal pollution prevention.
2 Materials and methods
2.1 Sand culture experiment
The sand culture experiment provides a controlled environment to precisely investigate the impact of chloride ions on cadmium morphology. In this experiment, the high Cd accumulation variety, Huang Huazhan (HH), was first sterilized using a 30% (v/v) hydrogen peroxide (H2O2) solution for 1 h and subsequently immersed in deionized water. The seeds were then germinated in the dark at a constant temperature of 28°C ± 0.5°C for 48 h.
Following germination, seeds were cleaned and wound, and 250 g of quartz sand was placed in each black nonporous plastic pot. A solution of Cd(NO3)2 was added to achieve a concentration of 5 mg kg−1. The substrate was saturated with 1/2 Kimura B nutrient solution (Chen and Xiong, 2021). To establish a chloride concentration gradient, CaCl2 was added at 0, 2, 4, 6, and 8 mmol kg−1 levels. Similarly, to mitigate the effects of Ca2+, a matching gradient of Ca(NO3)2 was used as a control.
Five treatments were established: control (SCK), CaCl2 (ST1–ST4 without Cd), Ca(NO3)2 (SCK1–SCK4 without Cd), CaCl2 with Cd (ST1–ST4), and Ca(NO3)2 with Cd (SCK1–SCK4). Each treatment was replicated three times, totaling 54 potted plants. Seeds with uniform germination rates were selected and placed in the prepared plastic pots, which were refreshed with 1/2 Kimura B nutrient solution every 3 days. The pots were maintained in incubators set at 30°C and 25°C with 75% relative humidity, a light intensity of 150 umol m−2 s−1, and a 16/8 h day/night cycle.
2.2 Pot experiment
The experimental soil was collected from the upper cultivating layer (0–20 cm) of a typical purple paddy field in Xiangtan County (27°32′47.26″N, 112°41′1.87″E), Xiangtan City, Hunan Province. Visible debris such as stone particles and root fragments were removed using wooden blocks. The soil was then air-dried, ground, and sieved through a 10-mesh screen to ensure uniformity for subsequent experiments. The basic physical and chemical properties of the soil are detailed in Table 1.
The study utilized the conventional double-cropping rice cultivar, Xiang wanxian13 (WX13), grown in cylindrical plastic pots (200 mm diameter × 200 mm depth). The rice seedlings, sourced from a rice base in Ningxiang County (28°21′N, 112°38′E), Hunan Province, underwent a cadmium analysis before transplantation, which indicated negligible Cd content.
This experiment was carried out during the rice growing season from July 15 to 20 October 2023, in a greenhouse located at the Rice Quality and Safety Control Hunan Engineering Laboratory, Central South University of Forestry Science and Technology. The laboratory is situated in a region characterized by a subtropical monsoon climate, with four distinct seasons and abundant rainfall. The average annual temperature, sunshine duration, and precipitation are 17.2°C, 1529.3 h·a−1, and 1361.6 mm, respectively.
After grinding and sieving, 1.5 kg of the test soil was placed into each cylindrical plastic pot. Cd(NO3)2 solution was added to achieve a Cd concentration of 5 mg·kg−1 soil. The mixture was then aged for 30 days to stabilize. Following the aging period, base fertilizers N (0.15 g·kg−1), P2O5 (0.1 g·kg−), and K2O (0.15 g·kg−1) were applied. During the rice growth stage, additional fertilizations of 1/2 and 1/4 base fertilizer doses of urea were administered.
A gradient of CaCl2 concentrations (0, 2, 4, 6, and 8 mmol·kg−1 Cl−, designated as CK, T1, T2, T3, and T4, respectively) was employed. The same concentration gradient of Ca(NO3)2 served as a control (CK1, CK2, CK3, and CK4, respectively). Given that Ca(NO3)2 contains binary anions, using it as a control helps mitigate the potential interference of Ca2+ in the experimental results. Each treatment was replicated three times to ensure the reliability of the results. NO3− from the Ca(NO3)2 treatment introduced excess elemental N, potentially impacting rice growth; thus, urea was added to balance the nitrogen content in each pot.
For consistent growth conditions, two healthy rice seedlings were transplanted into each pot of aging soil. The water management strategy involved maintaining flooded conditions throughout the reproductive period. The experimental design included four growth stages of rice (tillering, heading, filling, and maturity), with each stage divided into blocks. Each block received treatments across the five Cl− levels plus the Ca(NO3)2 control, totaling 108 pots.
2.3 Sample collection
Destructive sampling methods were employed in both experiments, meaning the samples collected were not used in subsequent tests. Samples from the rice plants, soil, and soil solution were collected at four critical growth stages: tillering, heading, filling, and maturity. The soil samples were air-dried in a cool area, finely ground, and sieved through 10-mesh and 100-mesh nylon sieves before being stored in sealed plastic bags for analysis.
Rice samples were thoroughly rinsed with tap water followed by ultrapure water. The roots, stems, leaves, and brown rice grains were then separated and initially dried at 105°C for 30 min, followed by a final drying at 70°C until a constant weight was achieved. The dried samples were then ground to pass through a 60-mesh sieve using a grinder and stored for subsequent analysis.
Soil solution samples were collected using the field capacity-derived soil solution extraction (SSE) technique as described by Chen et al. (2019a). For this method, 450 g of fresh soil was placed into a centrifuge tube and centrifuged at 8000 r·min−1 for 10 min.
2.4 Sample determination and quality control
Soil pH values were measured using a glass electrode (PHS-3C, Leici, China) in a 1:2.5 soil-to-water suspension ratio (Kargas et al., 2020). Soil organic matter (OM) was quantified calorimetrically through oxidation with potassium dichromate (Wu et al., 2017), while the CEC was assessed using the ammonium acetate method followed by distillation in a Kjeldahl bottle (Zhang et al., 2020a). The concentration of Cd extractable with 0.005 mol·L−1 DTPA (soil-DTPA solution ratio, 1:2) was determined using inductively coupled plasma–optical emission spectroscopy (ICAP 6000, Thermo Elemental). Additionally, the Cd concentration in the soil solution was measured with graphite furnace atomic absorption spectroscopy (Hitachi Z5000).
Soil samples were digested using a mixture of HCl-HNO3-HClO4 (7.5:2.5:3, v:v:v) for total Cd analysis (Gaudino et al., 2007), and plant powder was digested in a mixture of HNO3-HClO4 (8:2, v:v) for the same purpose (Yang et al., 2019a). The chemical forms of Cd in soils were characterized using the modified European Community Bureau of Reference (BCR) sequential extraction procedure (Bakircioglu et al., 2011). Cd concentrations in all soil fractions and rice plants were determined using flame atomic absorption spectrometry and graphite furnace atomic absorption spectrometry (AAS: iCE-3500, Thermo Fisher Scientific, Waltham, MA, United States). The content of Cl in rice plants was quantified by silver nitrate titration.
To ensure the reliability and accuracy of the data, each sample was measured three times during the assessment of soil and plant Cd concentrations. Additionally, a quality control protocol was implemented using the national standard soil sample GBW(E)-070009 and the Chinese plant sample GSB-23. The relative standard deviation (RSD) for these measurements was maintained below 5%.
2.5 Statistical methods
The data were statistically evaluated using an F-test to determine overall significance, supplemented by Duncan’s new multiple range test (MRT) for differentiating between treatment means at a significance level of p < 0.05. Analysis was conducted using Excel 2013, SPSS 22.0, and Origin 9.0 (OriginLab and IBM, USA). The accumulated cadmium (Cd) in plant tissue, expressed as mg·plant−1, was computed by multiplying the dry weight of the tissue (g·plant−1) by the Cd concentration in each tissue (mg·kg−1).
2.6 Calculation of the complex components in soil solution
(1) The ionic strength in soil solution was calculated with the following Eq. 1:
where I is ionic strength (mol·kg-1), and EC is the electrical conductivity measured in the soil solution (μs·cm−1).
(2) The activity coefficient was calculated using the modified Debye-Huckel equation and equation below (2):
where r is the ratio of an ion’s activity to its concentration in a solution, A is a parameter associated with the absolute of the solvent (when the solvent is water, at 25°C, A = 0.509 (mol·kg-1)−1/2), and z is the valence.
(3) Because the concentration of organic ligands in the soil solution was minimal, and the propensity of Cd to form organic ligands was inferior to that of other heavy metals, the influence of organic ligands was not accounted for in this study. The interaction between Cd2+ and Cl− in forming complexes was characterized using their conditional formation constants as reported by Griffin and Jurinak (1973):
where r1 and r2 are the activity coefficients of Cl− and Cd2+ respectively, and [Cl−] and [Cd2+] represent the concentrations of free Cl− and Cd2+ ions (mol·L−1), respectively.
3 Results
3.1 Effect of Cl− on rice biomass
The influence of Cl− on rice biomass was investigated by recording the fresh weight and plant height at various growth stages (Figure 1). In the sand culture experiment, there were significant differences in plant height among the treatments (Figure 1B). Without Cd, the plant height in treatments ST1 to ST4 was 11.19%–17.16% higher than in the SCK group. Conversely, with Cd present, plant height decreased by 15.45%–43.64%.
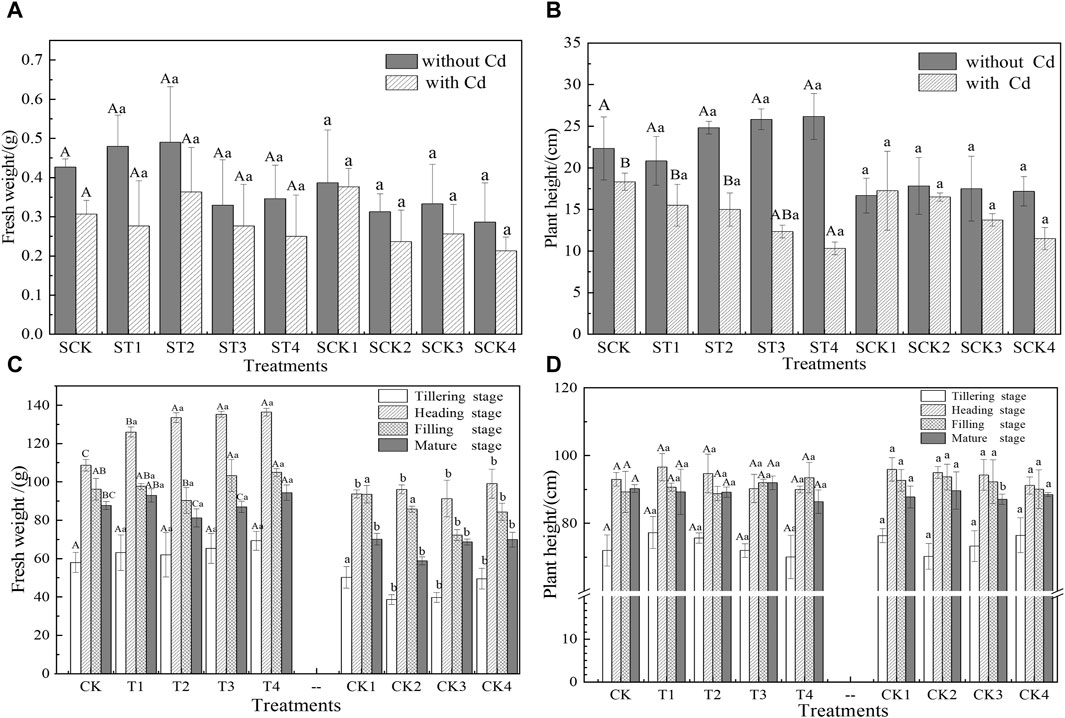
Figure 1. Effects of Cl− on the growth of rice. (A,B) and (C,D) are the results of sand culture and rice pot experiments, respectively. Different capital letters indicate that there was a significant difference between the effects of different Cl− additions on rice in the same stage (p < 0.05). Different lowercase letters indicate that there was a significant difference between the effects of Cl− on rice after offsetting the effect of Ca2+ in the same period (p < 0.05).
In the pot experiment, the fresh weight of rice consistently increased with the exogenous addition of Cl−, with significant variations noted among the treatments throughout the rice’s growth period. Specifically, the fresh weight in the T1–T4 treatments rose by 1.6%–25.5% compared to the control group (CK), and by 4.4%–60.2% relative to the Ca(NO3)2-treated groups (CK1–CK4), with the most substantial increases observed during the heading stage. This suggests that Cl− can enhance rice growth within certain concentration limits (Figure 1C). Additionally, no significant differences in plant height were found between the T1–T4 treatments, the control group (CK), and the Ca(NO3)2-treated groups during any growth stage, with peak plant heights achieved during the heading stage (Figure 1D).
3.2 Cd concentrations in different parts of rice at different growth stages
Figure 2 illustrates the distribution of Cd concentrations in different parts of rice at various growth stages. In the sand culture experiment, Cd content was markedly higher in the roots compared to the shoots. Furthermore, the addition of Cl− led to a gradual increase in the Cd content in these components (Figure 2A).
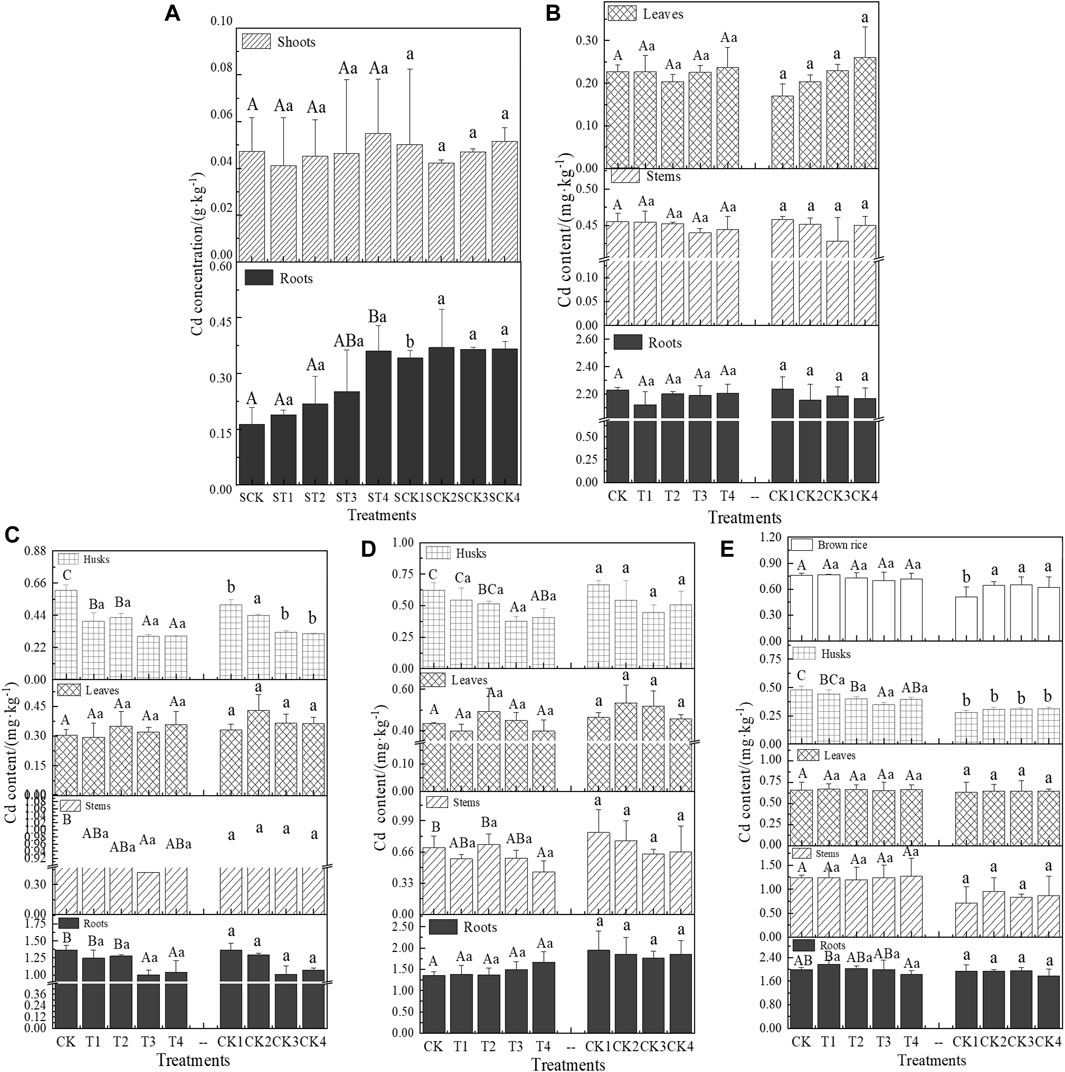
Figure 2. Cadmium concentrations in various parts of rice at different growth stages. (A) is the sand culture experiment. (B–E) are the tillering, heading, filling, and maturity stages of rice growth, respectively. Different capital letters indicate that there was a significant difference between the effects of different Cl− additions on the same part of the rice in the same stage (p < 0.05). Different lowercase letters indicate that there was a significant difference between the effects of Cl− on rice after offsetting the effect of Ca2+ in the same period (p < 0.05).
In the pot experiment, during the tillering stage, the differences in Cd concentrations across different parts of the rice were minimal among the treatments. Specifically, root Cd concentrations ranged from 2.12 to 2.24 mg·kg−1, averaging 2.19 mg·kg−1. Stem Cd concentrations were between 0.43 and 0.46 mg·kg−1, with an average of 0.45 mg·kg−1, and leaf Cd concentrations varied from 0.17 to 0.26 mg·kg−1, with an average of 0.22 mg·kg−1 (Figure 2B). During the heading and filling stages, Cd concentrations in each part of the T1–T4 treated rice were consistently lower than those in the Ca(NO3)2 treatment groups (CK1–CK4), showing reductions ranging from 3.45% to 32.17%, 1.52%–26.17%, 0.43%–25.67%, and 1.14%–32.03%, respectively (Figures 2C,D). These stages were identified as critical periods during which Cl− notably influenced Cd uptake in rice. At the maturity stage, the Cd content in each part of T1–T4 treated rice exceeded that in the corresponding parts of the control group, with increases spanning 5.31%–42.56%, 2.74%–23.04%, 1.27%–32.73%, and 2.48%–31.68%, respectively (Figure 2E).
3.3 Cd accumulation in rice plants under different treatments
The study quantified total plant Cd accumulation by measuring the Cd concentration and dry weight of various parts of the rice (roots, stems, leaves, husks, and brown rice). Figure 3 presents the effects of Cl− on Cd accumulation at different growth stages. As depicted in Figure 3, Cd accumulation predominantly occurred during the maturity stage. During the tillering stage, the addition of Cl− resulted in varying trends of Cd accumulation. Relative to the CK, there was a decrease in Cd accumulation of 4.02% and 4.51% in the T1 and T2 treatments, respectively, while an increase of 7.34% and 2.96% was observed in the T3 and T4 treatments, respectively. In the subsequent heading and filling stages, unlike the tillering stage, increased Cl− addition led to a general decrease in Cd accumulation, with the most pronounced decrease occurring during the filling stage. This suggests that the filling stage is particularly critical in influencing Cd accumulation in rice. Compared to the CK treatment group, the Cd accumulation in the T1–T4 treatments decreased by 5.87%–31.24%. In the maturity stage, only the T4 treatment exhibited higher Cd accumulation than the control (CK), showing an increase of 7.71%. Conversely, in the other treatments, Cd accumulation decreased by 6.44%–12.02%, which may be due to the varying concentrations of Cl−. Throughout the four growth stages, Cd accumulation in each treatment (T1–T4) was consistently higher than in the Ca(NO3)2 treatment groups (CK1–CK4), indicating that compared to NO3−, Cl− has a more substantial effect on Cd uptake by rice.
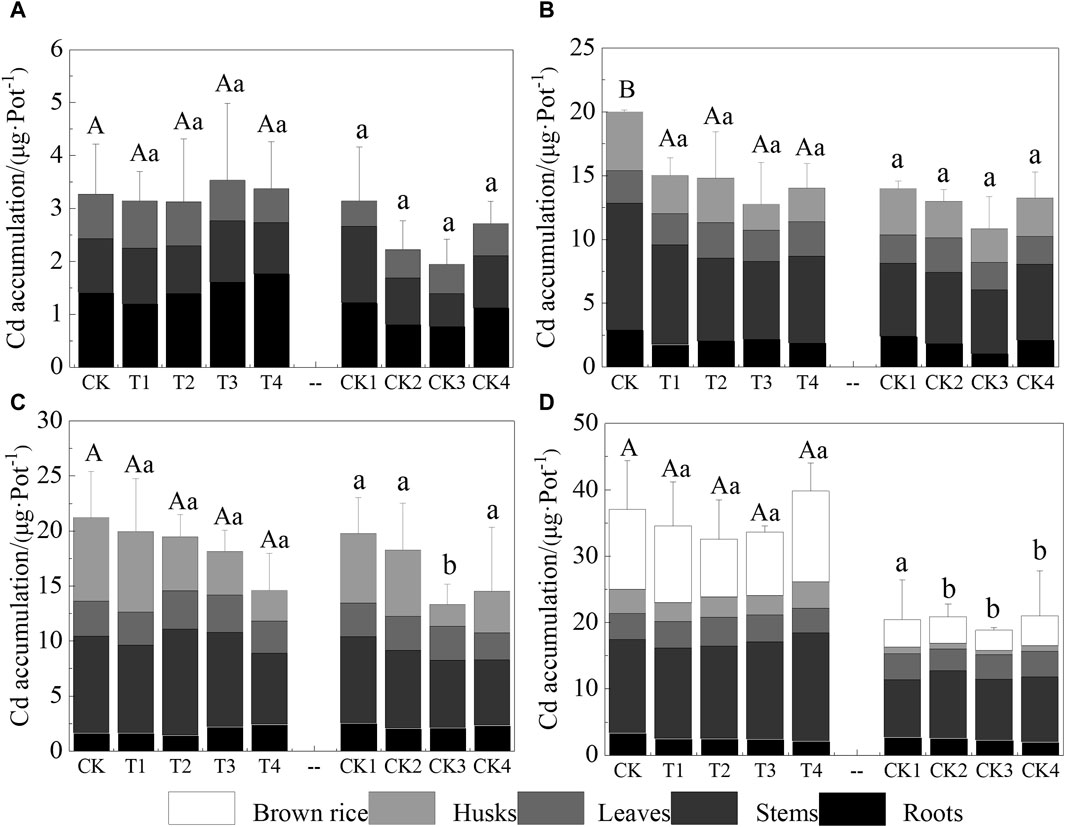
Figure 3. Cadmium accumulation in rice at different growth stages. (A–D) are the tillering, heading, filling, and maturity stages of rice growth, respectively. Different capital letters indicate that there was a significant difference between the effects of different Cl− additions on the Cd accumulation of rice in the same stage (p < 0.05). Different lowercase letters indicate that there was a significant difference between the effects of Cl− on the Cd accumulation of rice after offsetting the effect of Ca2+ in the same period (p < 0.05).
3.4 Changes in the Cd concentration in soil solution at different growth stages
Figure 4 illustrates the Cd concentrations measured using the field capacity-SSE method across different rice growth stages. There were significant differences in the Cd concentration of the soil solution between the initial growth periods (tillering and heading stages) and the later stages (filling and maturity stages) across all treatments. In both the CK and the T1–T4 treatments, the Cd concentration determined by SSE remained consistent throughout the growth period, ranging from 0.1170 to 0.3973 μg·L−1. During the maturity stage, the SSE-Cd concentration in the T1–T4 treatments was 0.116–0.672 times higher than that in the CK treatment. Throughout the rice’s growth, the SSE-Cd concentration in the T1–T4 treatments varied from 0.068 to 2.994 times lower than in the CK1–CK4 treatments.
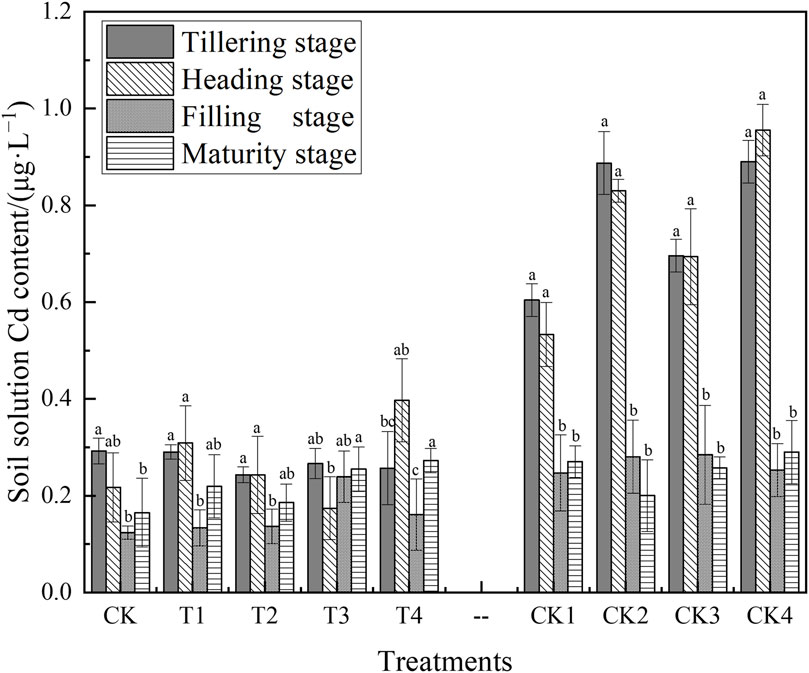
Figure 4. Cadmium concentrations in soil solution across different growth stages of rice. Different lowercase letters indicate significant differences in the same treatment between the different growth stages (p < 0.05).
To clarify the interaction between chloride Cl− and Cd in the soil, Eqs 1–4 were used to calculate the concentrations of each component in the complexes (CdCln2−n). These calculations aid in analyzing the impact of Cl− on the bioavailability of Cd, with the results detailed in Table 2. Generally, Cl− can form complexes such as CdCl+, CdCl20, CdCl3−, and CdCl42− with Cd. However, due to the relatively low concentrations of CdCl3− and CdCl42− (<1 × 10−9 M), these complexes were not considered significant in this study. As shown in Table 2, when the Cd/Cl ratio (m:n) in the soil-water medium ranges from 0.625 to 2.5, an increase in Cl− added also increased the concentrations of CdCl+ and CdCl20 in all treatments, with the concentration of CdCl+ being significantly higher than that of CdCl20. The findings indicate that under these conditions, the predominant complexes formed by Cl− and Cd2+ in the soil solution were mainly CdCl+.
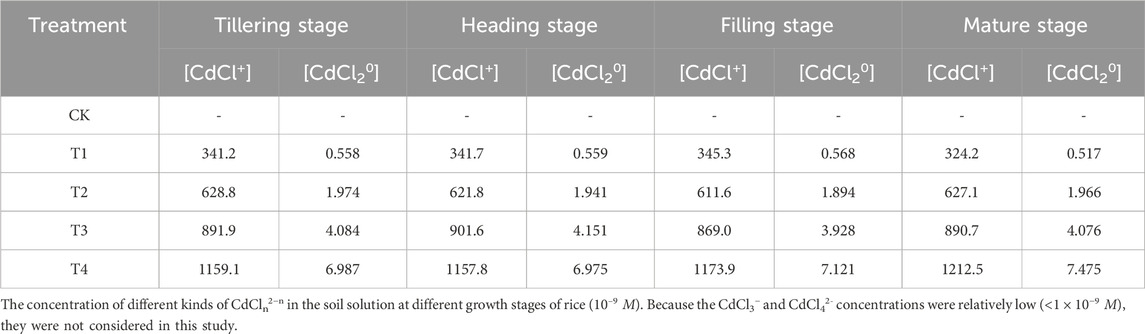
Table 2. The concentration of different kinds of CdCln2−n in the soil solution at different growth stages of rice (10–9 M). Because the CdCl3− and CdCl42- concentrations were relatively small (<1 × 10−9 M), they were not considered in this study.
3.5 Effects of Cl− on the chemical form of soil Cd
Figure 5 illustrates the distribution of different chemical forms of Cd in the soil, both with and without Cl− additions, across various growth stages of rice. Predominantly, the soil Cd was found in the acid-soluble fraction (E1), which accounted for approximately 50%–80% of the total Cd. Relative to the CK, the inclusion of Cl− in treatments T1–T4 significantly enhanced the E1 form of Cd throughout the entire growth period of the rice, showing increases ranging from 4.27% to 16.90%, 1.62%–15.68%, 0.90%–3.99%, to 2.66%–13.47%, respectively. Conversely, the trend observed in the residual fraction (E4) of Cd varied across the different growth periods. During the filling stage, the E4 form of Cd saw an increase of 2.14%–9.83% compared to CK. However, during the other growth periods, there were decreases in E4, specifically 6.07%–19.54%, 2.97%–12.67%, and 6.86%–21.97%, respectively. Over the whole growth period, the addition of Cl− appeared to have minimal impact on the organic fraction (E3) of Cd.
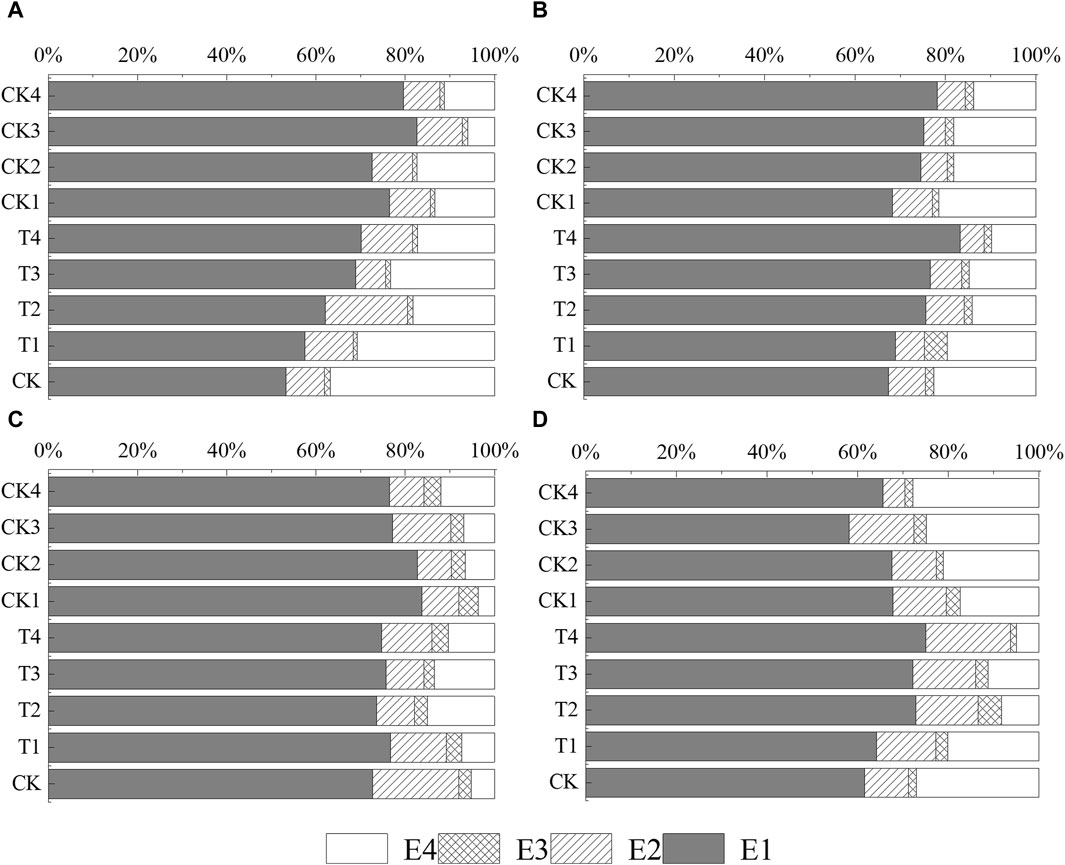
Figure 5. The average percentage soil Cd content obtained by the BCR method in different growth stages of rice. (A–D) are the tillering stage, heading stage, filling stage, and maturity stage, respectively. The terms E1, E2, E3, and E4 refer to the acid-soluble fraction (E1), iron and manganese oxide fraction (E2), organic fraction (E3), and residual fraction (E4).
4 Discussion
The data illustrated in Figure 1 demonstrate that with the increase in Cl− addition compared to the CK, the fresh weight of rice saw an increase ranging from 1.6% to 25.5%. Similarly, in the sand culture experiment without Cd treatment, an evident upward trend in plant height was observed when compared to the SCK group. Conversely, with the addition of Cd, there was a progressive decrease in plant height as Cl− levels increased. This trend can be attributed to chlorine’s role as an essential micronutrient that supports and enhances plant photosynthesis, regulates osmotic pressure within cells, and maintains cellular charge balance (Geilfus, 2018). Consequently, these functions promote rice growth. However, when Cl− and Cd2+ coexist in the soil, Cl-complexes (CdCln2−n) form, reducing the surface charge on plant cells and thus facilitating the transfer and diffusion of Cd within the plant (Hussain et al., 2022). Additionally, during the early stages of rice growth, where protein synthesis is predominant, Cd can bind to proteins containing sulfhydryl groups, disrupting their function and intensifying its toxic effects, particularly during the seedling stage (Cai et al., 2020). As the rice matures, its resistance to Cd toxicity increases, leading to varied responses to Cd toxicity at different growth stages (Li et al., 2020).
It is widely recognized that the uptake of Cd by rice varies across different growth stages (Rodda et al., 2011; Li et al., 2017; Chen et al., 2019b). During the early vegetative growth stages, rice exhibits rapid biomass accumulation, necessitating substantial nutrient uptake. Concurrently, bioavailable Cd from the soil is absorbed by the roots along with these nutrients, predominantly accumulating in the roots (Zare et al., 2018; Yang et al., 2019b; Rehman et al., 2019). Additionally, CdCln2−n complexes can directly enter the roots and/or dissociate at the root surface, subsequently entering cells as Cd2+ (Filipović et al., 2018). This mechanism is supported by the observed higher Cd concentration in rice roots at the tillering stage compared to other stages (Figure 2B). Results from the sand culture experiment indicate that increasing Cl− levels correspond with increased Cd accumulation in the roots, without significant changes in shoot Cd content (Figure 2A). This suggests that Cl− enhances Cd uptake in rice roots.
However, the transport of Cd from roots to shoots may be regulated by physiological mechanisms that limit Cd translocation when shoot concentrations reach a certain threshold, thereby preventing excessive accumulation in aerial parts of the plant (Zhang et al., 2018b; Zhou et al., 2020). Studies have highlighted the role of the natural resistance-associated macrophage protein (NRAMP) family in mediating the transport of divalent metal cations including Fe, Mn, Cd, and Zn (Yuan et al., 2019). Among these, OsNRAMP5, located in rice root cells, is crucial in the uptake and transport of Mn2+, Cd2+, and Fe2+ from roots to shoots, accounting for nearly 90% of Cd uptake (Ishimaru et al., 2012; Huang et al., 2021). OsNRAMP5 functions as an efflux transporter, moving Cd from ectodermal and endodermal cells into the xylem for transfer to the shoots (Hao et al., 2018).
Cd uptake by rice involves its absorption by the OsNRAMP5 transporter in root cells, after which the absorbed Cd is partly sequestered in vesicles, while the remainder is released into the xylem for transfer to the shoots (Skuza et al., 2022). A significant finding by Tang et al. (2017) showed that rice mutants lacking the OsNRAMP5 gene exhibited substantially lower Cd levels in both roots and shoots compared to wild-type plants. This suggests a regulatory mechanism where, upon reaching a specific concentration of Cd in the shoots, the plant suppresses OsNRAMP5 expression, thereby inhibiting further Cd transport from the roots to the shoots.
During the heading and filling stages, the Cd concentrations in rice treated with T1–T4 were notably lower than those in the Ca(NO3)2 treatment group (CK1–CK4). This difference is attributed to the stronger coordination ability of Cl− compared to NO3−, which enables Cl− to form more stable complexes with Cd2+ (general formula CdCln2−n) (Li et al., 2024). The specific composition of these complexes varies depending on the relative concentrations of Cd and Cl−.
The influence of soil type on Cd absorption by rice is well-documented, with significant variations in Cd uptake across different soil conditions (Yu et al., 2016; He et al., 2017). A key study by Ye et al. (2014) across 19 representative paddy soils in China employed aggregated boosted tree analysis to assess how various soil properties contribute to Cd bioaccumulation in rice grains. Their findings highlighted soil pH and organic carbon content as key determinants of Cd uptake. In soils such as purple mud, the predominant clay minerals—montmorillonite and illite—exhibited a 2:1 layer structure enriched with variable cations such as iron, magnesium, and alkali metals, which can undergo isomorphous substitution. This substitution often facilitates the exchange of these cations for others of similar size but lower charge (Moldoveanu and Papangelakis, 2013; Kumari and Mohan, 2021).
From the analyses using the Debye-Hückel limit equation (Eqs 1–4), it was deduced that the predominant complexes formed between chloride Cl− and Cd2+ in the soil solution are mainly CdCl+ (Table 2). This suggests that CdCl+ is more readily adsorbed onto soil particles through ion exchange, thereby reducing the concentration of free Cd2+ in the soil solution (Figure 4). Consequently, Cl− diminishes the mobility of Cd in the soil. Despite this, our study noted a significant increase in Cd accumulation in rice plants treated with Cl− (Figure 3), indicating an enhanced uptake of some CdCl+ by plant roots. This finding confirms reports that the formation of CdCln2−n complexes in soil solution increases Cd uptake, probably by direct uptake of the CdCln2−n complexes by plants (Cheng et al., 2018).
Furthermore, plants exhibit a dynamic and reversible adjustment mechanism to environmental stresses, including ion toxicity. When Cl− is externally supplied, it helps establish an osmotic balance between the cytoplasm and the external environment, alleviating stress by neutralizing cations such as Ca2+ and Cd2+ in the soil solution (Guo et al., 2018). Hence, the presence of free Cd2+ in the soil solution alone does not fully account for the bioavailability of Cd. This necessitates an in-depth analysis based on its chemical form, particularly in the presence of multiple competing anions, to better understand the bioactive forms of Cd under varied agricultural conditions.
Cl− plays a multifaceted role in the soil solution dynamics and the variety of chlorine-based complexes, directly influencing the formation of various chlorine-based complexes. Soil layered alumino-silicate minerals and organic colloids, which are predominantly negatively charged (Doi et al., 2019), exhibit relatively low adsorption strength for CdCl+ and CdCl2 compared to Cd2+. Furthermore, negatively charged complexes such as CdCl3− and CdCl42− may even induce negative adsorption, potentially leading to the desorption of previously adsorbed cadmium from the soil matrix (Cheng et al., 2017).
In the solid phase of the soil, Cl− can destabilize various chemosynthetic states of Cd through competitive interactions with other ligands. Zheng et al. (2023) demonstrated that the intervention of Cl− could result in the redistribution of organically bound Cd to the soil solution as Cd2+. The interaction of Cl− with layered clay minerals provides further insight into these complex dynamics. The strong negative charge on the surface of clay minerals tends to repel Cl−, while the weak positive charge present on the edges of these minerals can adsorb Cl−. This absorption of Cl− at the edges of clay minerals influences the specialized adsorption processes of Cd2+ on the surface of clay oxides (García et al., 2021). The strong coordination ability of Cl− significantly impacts the behavior of Cd in the soil environment, affecting its availability for uptake by plants.
Figure 3 demonstrates that Cd accumulation in rice at maturity was significantly greater compared to other growth stages. Differing concentrations of exogenously added Cl− influenced Cd accumulation in rice, as depicted in Figure 3D. Consistent with our findings, Zhang et al. (2020b) reported that Cd concentration and accumulation in S. glauca shoots were significantly increased by the addition of exogenous Cl-compared to the control. Similarly, Li et al. (2019b) observed that low Cl− concentrations (0.1–2.0 g·kg−1) did not significantly affect DTPA-extracted Cd levels in soil, whereas higher concentrations (5.0 g·kg−1) increased these levels by 24.79%. DTPA-extracted Cd is commonly utilized to assess Cd bioavailability. Our findings suggest that the addition of Cl− to soil enhances Cd absorption and accumulation in rice. At various growth stages, Cl− significantly increased acid-extractable Cd while reducing residual Cd, with the exception of the filling stage, as illustrated in Figure 5. This effect can be attributed to several mechanisms: 1) High concentrations of Cl− facilitate the release of exchangeable, organically bound, and carbonate-bound Cd in the soil; 2) Cl− promotes the secretion of organic acids from rice roots, thereby enhancing the desorption of residual Cd and increasing Cd solubility in the rice rhizosphere (Zeng et al., 2017); and/or 3) Dissolved organic matter (DOM) is one of the most active soil components influencing Cd migration, transformation, bioavailability, and toxicity in soils (Zhang et al., 2022). The organic ligands in DOM can also be complexed with Cd to form soluble organic metal complexes; thus, improving the migration of Cd in the soil (Chen et al., 2021).
5 Conclusion
The purpose of this study was to explore the impact of exogenous Cl− addition on Cd migration across various growth stages of rice within a soil-rice system. Based on the experimental findings, we drew the following conclusions:
(1) Rice sand culture experiments revealed that exogenous Cl− addition within a specific concentration range could enhance the fresh weight and plant height of rice. However, in the presence of Cd, chloride ions facilitated the uptake of Cd by rice roots, resulting in significantly higher Cd levels in the roots compared to the shoots. This increased concentration of Cd in the roots increased its toxic effects on rice, leading to reductions in both fresh weight and plant height.
(2) Pot experiments demonstrated that exogenous chloride ion addition significantly reduced Cd content and accumulation in all parts of rice compared to the Ca(NO3)2 treatment group during both the tassel and filling stages. The reduction was most pronounced at the filling stage, suggesting that this is a critical period for chloride ions to influence Cd accumulation in rice.
(3) Exogenous chloride ions increase the concentration of acid-extractable Cd and CdCl+ in the soil. When the Cd/Cl ratio in the soil-water medium ranged from 0.625 to 2.5, Cl− in the soil formed a complex with free Cd2+ in the solution, resulting in a CdCl+-based complex. This complex enhanced the adsorption of Cd by soil particles and promoted the uptake of CdCl+ by rice roots, thereby increasing the Cd concentration in rice.
In conclusion, in the studied concentration range, Cl− could complex with free Cd2+ to form CdCl+ in the soil solution, which might then be absorbed by rice plants. This complexation process could reduce the mobility of cadmium in the soil, thereby potentially enhancing its uptake and accumulation in rice. For effective field management, it is crucial to consider the level of Cl− input to ensure both the mass production and safe utilization of rice in systems with moderate to light cadmium contamination. Additionally, this study not only guides the evaluation of soil environmental quality but also serves as a reference for the rational application of chemical fertilizers.
Data availability statement
The raw data supporting the conclusion of this article will be made available by the authors, without undue reservation.
Author contributions
HF: Writing–original draft, Data curation, Formal Analysis, Visualization. ST: Data curation, Formal Analysis, Writing–original draft. JL: Funding acquisition, Supervision, Validation, Writing–review and editing. RH: Data curation, Writing–review and editing. ZX: Data curation, Writing–review and editing. HH: Supervision, Writing–review and editing. PP: Supervision, Writing–review and editing.
Funding
The author(s) declare that financial support was received for the research, authorship, and/or publication of this article. This research was funded by the National Natural Science Foundation of China (42107444), the Reuter Foundation of Education Bureau of Hunan Province, China (21B0245), and the National Key Research and Development Program of China (2022YFD1700104).
Conflict of interest
The authors declare that the research was conducted in the absence of any commercial or financial relationships that could be construed as a potential conflict of interest.
The handling editor QL declared a shared affiliation with the authors at the time of review.
Publisher’s note
All claims expressed in this article are solely those of the authors and do not necessarily represent those of their affiliated organizations, or those of the publisher, the editors and the reviewers. Any product that may be evaluated in this article, or claim that may be made by its manufacturer, is not guaranteed or endorsed by the publisher.
References
Acosta, J. A., Jansen, B., Kalbitz, K., Faz, A., and Martínez-Martínez, S. (2011). Salinity increases mobility of heavy metals in soils. Chemosphere 85 (8), 1318–1324. doi:10.1016/j.chemosphere.2011.07.046
Bakircioglu, D., Kurtulus, Y. B., and Ibar, H. (2011). Investigation of trace elements in agricultural soils by BCR sequential extraction method and its transfer to wheat plants. Environ. Monit. Assess. 175 (1), 303–314. doi:10.1007/s10661-010-1513-5
Cai, Y., Zhang, S., Cai, K., Huang, F., Pan, B., and Wang, W. (2020). Cd accumulation, biomass and yield of rice are varied with silicon application at different growth phases under high concentration cadmium-contaminated soil. Chemosphere 242, 125128. doi:10.1016/j.chemosphere.2019.125128
Chen, G., and Xiong, S. (2021). OsHIPP24 is a copper metallochaperone which affects rice growth. Plant Biol. 64, 145–153. doi:10.1007/s12374-020-09287-x
Chen, H. R., Yang, Y., Ye, Y. F., Tao, L., Fu, X., Liu, B., et al. (2019). Differences in cadmium accumulation between indica and japonica rice cultivars in the reproductive stage. Ecotoxicol. Environ. Saf. 186, 109795. doi:10.1016/j.ecoenv.2019.109795
Chen, M. S., Ding, S. M., Li, C., Tang, Y., Fan, X., Xu, H., et al. (2021). High cadmium pollution from sediments in a eutrophic lake caused by dissolved organic matter complexation and reduction of manganese oxide. Water Res. 190, 116711. doi:10.1016/j.watres.2020.116711
Chen, Q., Peng, P. Q., Long, J., Li, X. Y., Ding, X., Hou, H. B., et al. (2019). Cadmium phytoavailability evaluation in rice-soil system using a field capacity-derived soil solution extraction: an entire growth period study in subtropical China. Soil Tillage Res. 194, 104315. doi:10.1016/j.still.2019.104315
Cheng, F., Guo, S., Li, G., Wang, S., Li, F., and Wu, B. (2017). The loss of mobile ions and the aggregation of soil colloid: results of the electrokinetic effect and the cause of process termination. Electrochem. Acta 258, 1016–1024. doi:10.1016/j.electacta.2017.11.153
Cheng, M. M., Wang, A. A., Liu, Z. Q., Gendall, A. R., Rochfort, S., and Tang, C. (2018). Sodium chloride decreases cadmium accumulation and changes the response of metabolites to cadmium stress in the halophyte Carpobrotus rossii. Ann. Bot. 122, 373–385. doi:10.1093/aob/mcy077
Christoph-Martin, G. (2019). Chloride in soil: from nutrient to soil pollutant. Environ. Exp. Bot. 157, 299–309. doi:10.1016/j.envexpbot.2018.10.035
Deng, X., Chen, Y., Yang, Y., Yuan, X., Zeng, H., Zeng, Q., et al. (2020). Cadmium accumulation in rice (Oryza sativa L.) alleviated by basal alkaline fertilizers followed by topdressing of manganese fertilizer. Environ. Pollut. 262, 114289. doi:10.1016/j.envpol.2020.114289
Doi, A., Ejtemaei, M., and Nguyen, A. V. (2019). Effects of ion specificity on the surface electrical properties of kaolinite and montmorillonite. Miner. Eng. 143, 105929. doi:10.1016/j.mineng.2019.105929
Feng, J., Shen, R. F., and Shao, J. F. (2020). Transport of cadmium from soil to grain in cereal crops: a review. Pedosphere 31 (1), 3–10. doi:10.1016/S1002-0160(20)60015-7
Filipović, L., Romić, M., Romić, D., Filipović, V., and Ondrašek, G. (2018). Organic matter and salinity modify cadmium soil (phyto) availability. Ecotoxicol. Environ. Saf. 147, 824–831. doi:10.1016/j.ecoenv.2017.09.041
Fu, Y. H., Li, F. M., Guo, S. H., and Zhao, M. (2021). Cadmium concentration and its typical input and output fluxes in agricultural soil downstream of a heavy metal sewage irrigation area. J. Hazard. Mater. 412, 125203. doi:10.1016/j.jhazmat.2021.125203
García, K. I., Quezada, G. R., Arumí, J. L., Urrutia, R., and Toledo, P. G. (2021). Adsorption of phosphate ions on the basal and edge surfaces of kaolinite in low salt aqueous solutions using molecular dynamics simulations. J. Phys. Chem. C 125 (38), 21179–21190. doi:10.1021/acs.jpcc.1c05995
Gaudino, S., Galas, C., Belli, M., Barbizzi, S., de Zorzi, P., Jaćimović, R., et al. (2007). The role of different soil sample digestion methods on trace elements analysis: a comparison of ICP-MS and INAA measurement results. Accreditation Qual. Assur. 12 (2), 84–93. doi:10.1007/s00769-006-0238-1
Ge, L. Q., Cang, L., Liu, H., and Zhou, D. M. (2016). Effects of warming on uptake and translocation of cadmium (Cd) and copper (Cu) in a contaminated soil-rice system under Free Air Temperature Increase (FATI). Chemosphere 155, 1–8. doi:10.1016/j.chemosphere.2016.04.032
Geilfus, C. M. (2018). Review on the significance of chlorine for crop yield and quality. Plant Sci. 270, 114–122. doi:10.1016/j.plantsci.2018.02.014
Griffin, B. A., and Jurinak, J. J. (1973). Estimation of activity coefficients from the electrical conductivity of natural aquatic systems and soil extracts. Soil Sci. 116 (1), 26–30. doi:10.1097/00010694-197307000-00005
Guo, S. H., Hu, N., Li, Q. S., Yang, P., Wang, L. L., Xu, Z. M., et al. (2018). Response of edible amaranth cultivar to salt stress led to Cd mobilization in rhizosphere soil: a metabolomic analysis. Environ. Pollut. 241, 422–431. doi:10.1016/j.envpol.2018.05.018
Hao, X. G., Zeng, M., Wang, J., Zeng, Z., Dai, J., Xie, Z., et al. (2018). A node-expressed transporter OsCCX2 is involved in grain cadmium accumulation of rice. Front. Plant Sci. 9 (9), 476. doi:10.3389/fpls.2018.00476
He, H., Tam, N. F. Y., Yao, A., Qiu, R., Li, W. C., and Ye, Z. (2017). Growth and Cd uptake by rice (Oryza sativa) in acidic and Cd-contaminated paddy soils amended with steel slag. Chemosphere 189, 247–254. doi:10.1016/j.chemosphere.2017.09.069
Hu, Y., Cheng, H., and Tao, S. (2016). The challenges and solutions for cadmium-contaminated rice in China: a critical review. Environ. Int. 92, 515–532. doi:10.1016/j.envint.2016.04.042
Huang, F., Zhou, H., Gu, J. F., Liu, C., Yang, W., Liao, B., et al. (2020). Differences in absorption of cadmium and lead among fourteen sweet potato cultivars and health risk assessment. Ecotoxicol. Environ. Saf. 203, 111012. doi:10.1016/j.ecoenv.2020.111012
Huang, W. X., Zhang, D. M., Cao, Y. Q., Dang, B. J., Jia, W., Xu, Z. C., et al. (2021). Differential cadmium translocation and accumulation between Nicotiana tabacum L. and Nicotiana rustica L.by transcriptome combined with chemical form analyses. Ecotoxicol. Environ. Saf. 208, 111412. doi:10.1016/j.ecoenv.2020.111412
Hussain, B., Ma, Y., Li, J., Gao, J., Ullah, A., and Tahir, N. (2022). Cadmium in rice is affected by fertilizer-borne chloride and sulfate anions: long-term field versus pot experiments. Processes 10 (7), 1253. doi:10.3390/pr10071253
Hussain, B., Umer, M. J., Li, J., Ma, Y., Abbas, Y., Ashraf, M. N., et al. (2020). Strategies for reducing cadmium accumulation in rice grains. J. Clean. Prod. 286, 125557. doi:10.1016/j.jclepro.2020.125557
Ishimaru, Y., Takahashi, R., Bashir, K., Shimo, H., Senoura, T., Sugimoto, K., et al. (2012). Characterizing the role of riceNRAMP5 in manganese, iron and cadmium transport. Sci. Rep. 2, 286. doi:10.1038/srep00286
Ishtiyaq, S., Kumar, H., D’Souza, R. J., Varun, M., Favas, P. J. C., and Paul, M. S. (2023). Physiological responses and adaptations of the halophyte Atriplex halimus to soil contaminated with Cd, Ni, and NaCl. Soil Syst. 7 (2), 46. doi:10.3390/soilsystems7020046
Kargas, G., Londra, P., and Sgoubopoulou, A. (2020). Comparison of soil EC values from methods based on 1:1 and 1:5 soil to water ratios and ECe from saturated paste extract based method. Water 12 (4), 1010. doi:10.3390/w12041010
Kumari, N., and Mohan, C. (2021). “Basics of clay minerals and their characteristic properties,” in Clay and clay minerals (Brazil: IntechOpen). doi:10.5772/intechopen.97672
León-Romero, M. A., Soto-Ríos, P. C., Fujibayashi, M., and Nishimura, O. (2017). Impact of NaCl solution pretreatment on plant growth and the uptake of multi-heavy metal by the model plant Arabidopsis thaliana. Water, Air, & Soil Pollut. 228 (2), 64. doi:10.1007/s11270-017-3241-8
Li, H., Li, Z., Khaliq, M. A., Xie, T., Chen, Y., and Wang, G. (2019). Chlorine weaken the immobilization of Cd in soil-rice systems by biochar. Chemosphere 235, 1172–1179. doi:10.1016/j.chemosphere.2019.06.203
Li, H., Luo, N., Li, Y. W., Cai, Q. Y., Mo, C. H., Wong, M. H., et al. (2017). Cadmium in rice: transport mechanisms, influencing factors, and minimizing measures. Environ. Pollut. 224, 622–630. doi:10.1016/j.envpol.2017.01.087
Li, J., Hashimoto, Y., Riya, S., Terada, A., Hou, H., Shibagaki, Y., et al. (2019). Removal and immobilization of heavy metals in contaminated soils by chlorination and thermal treatment on an industrial-scale. Chem. Eng. J. 359, 385–392. doi:10.1016/j.cej.2018.11.158
Li, S. X., Huang, L. C., Jia, B. J., Feng, X., Cao, Y., Chen, Y., et al. (2024). Effect and mechanism of inorganic anions on the adsorption of Cd2+ on two-dimensional copper-based metal–organic framework. Inorg. Chem. Commun. 159, 111819. doi:10.1016/j.inoche.2023.111819
Li, X., Peng, P., Long, J., Dong, X., Jiang, K., and Hou, H. (2020). Plant-induced insoluble Cd mobilization and Cd redistribution among different rice cultivars. J. Clean. Prod. 256, 120494. doi:10.1016/j.jclepro.2020.120494
Li, Z., Liu, Z., Anderson, W., Yang, P., Wu, W., Tang, H., et al. (2015). Chinese rice production area adaptations to climate changes, 1949–2010. Environ. Sci. Technol. 49 (4), 2032–2037. doi:10.1021/es505624x
Lu, J., Bai, Z., Velthof, G. L., Wu, Z., Chadwick, D., and Ma, L. (2019). Accumulation and leaching of nitrate in soils in wheat-maize production in China. Agric. Water Manag. 212, 407–415. doi:10.1016/j.agwat.2018.08.039
Lu, Q., Xu, Z., Xu, X., Liu, L., Liang, L., Chen, Z., et al. (2019). Cadmium contamination in a soil-rice system and the associated health risk: an addressing concern caused by barium mining. Ecotoxicol. Environ. Saf. 183, 109590. doi:10.1016/j.ecoenv.2019.109590
Moldoveanu, G. A., and Papangelakis, V. G. (2013). Recovery of rare earth elements adsorbed on clay minerals: II. Leaching with ammonium sulfate. Hydrometallurgy 131, 158–166. doi:10.1016/j.hydromet.2012.10.011
Rehman, M. Z., Rizwan, M., Rauf, A., Ayub, M. A., Ali, S., Qayyum, M. F., et al. (2019). Split application of silicon in cadmium (Cd) spiked alkaline soil plays a vital role in decreasing Cd accumulation in rice (Oryza sativa L.) grains. Chemosphere 226, 454–462. doi:10.1016/j.chemosphere.2019.03.182
Rodda, M. S., Li, G., and Reid, R. J. (2011). The timing of grain Cd accumulation in rice plants: the relative importance of remobilisation within the plant and root Cd uptake post-flowering. Plant Soil 347 (1-2), 105–114. doi:10.1007/s11104-011-0829-4
Skuza, L., Izabela, S. K., Filip, E., and Bożek, I. (2022). Natural molecular mechanisms of plant hyperaccumulation and hypertolerance towards heavy metals. Int. J. Mol. Sci. 23 (16), 9335. doi:10.3390/ijms23169335
Song, W. E., Chen, S. B., Liu, J. F., Chen, L., Song, N., Li, N., et al. (2015). Variation of Cd concentration in various rice cultivars and derivation of cadmium toxicity thresholds for paddy soil by species-sensitivity distribution. J. Integr. Agric. 14 (09), 1845–1854. doi:10.1016/s2095-3119(14)60926-6
Sterckeman, T., Goderniaux, M., Sirguey, C., Cornu, J. Y., and Nguyen, C. (2015). Do roots or shoots control cadmium accumulation in the hyperaccumulator Noccaea caerulescens? Plant Soil 392 (1-2), 87–99. doi:10.1007/s11104-015-2449-x
Sun, Y., Qiu, T., Gao, M., Shi, M., Zhang, H., and Wang, X. (2019). Inorganic and organic fertilizers application enhanced antibiotic resistome in greenhouse soils growing vegetables. Ecotoxicol. Environ. Saf. 179, 24–30. doi:10.1016/j.ecoenv.2019.04.039
Tang, L., Mao, B. G., Li, Y. K., Lv, Q., Zhang, L., Chen, C., et al. (2017). Knockout of OsNramp5 using the CRISPR/Cas9 system produces low Cd-accumulating indica rice without compromising yield. Sci. Rep. 7, 14438. doi:10.1038/s41598-017-14832-9
Wang, P., Chen, H., Kopittke, P. M., and Zhao, F. J. (2019). Cadmium contamination in agricultural soils of China and the impact on food safety. Environ. Pollut. 249, 1038–1048. doi:10.1016/j.envpol.2019.03.063
Wu, W., Wu, J., Liu, X., Chen, X., Wu, Y., and Yu, S. (2017). Inorganic phosphorus fertilizer ameliorates maize growth by reducing metal uptake, improving soil enzyme activity and microbial community structure. Ecotoxicol. Environ. Saf. 143, 322–329. doi:10.1016/j.ecoenv.2017.05.039
Xu, J. H., Wang, M., and Zhang, R. (2020). Advances in the biotoxicity of soil cadmium pollution. J. Ecotoxicol. 15 (05), 82–91. doi:10.7524/AJE.1673-5897.20200424002
Yang, X., Lin, R., Zhang, W., Xu, Y., Wei, X., Zhuo, C., et al. (2019). Comparison of Cd subcellular distribution and Cd detoxification between low/high Cd-accumulative rice cultivars and sea rice. Ecotoxicol. Environ. Saf. 185, 109698. doi:10.1016/j.ecoenv.2019.109698
Yang, Y., Ge, Y., Tu, P., Zeng, H., Zhou, X., Zou, D., et al. (2019). Phytoextraction of Cd from a contaminated soil by tobacco and safe use of its metal-enriched biomass. J. Hazard. Mater. 363, 385–393. doi:10.1016/j.jhazmat.2018.09.093
Ye, X., Li, H., Ma, Y., Wu, L., and Sun, B. (2014). The bioaccumulation of Cd in rice grains in paddy soils as affected and predicted by soil properties. J. soils sediments 14 (8), 1407–1416. doi:10.1007/s11368-014-0901-9
Yu, H. Y., Liu, C., Zhu, J., Li, F., Deng, D. M., Wang, Q., et al. (2016). Cadmium availability in rice paddy fields from a mining area: the effects of soil properties highlighting iron fractions and pH value. Environ. Pollut. 209, 38–45. doi:10.1016/j.envpol.2015.11.021
Yuan, D., Huang, L., and Zeng, L. (2017). Acute toxicity of mercury chloride (Hgcl2) and cadmium chloride (Cdcl2) on the behavior of freshwater fish, Percocypris Pingi. Int. J. Aquac. 3 (3), 066–070. doi:10.17352/2455-8400.000031
Yuan, X., Ma, H., and Ma, Y. F. (2019). Research progress on cadmium accumulation-related gene family in rice. Anhui Agric. Sci. 47 (16), 1–4+17. doi:10.3969/j.issn.0517-6611.2019.16.001
Zare, A. A., Khoshgoftarmanesh, A. H., Malakouti, M. J., Bahrami, H., and Chaney, R. (2018). Root uptake and shoot accumulation of cadmium by lettuce at various Cd: Zn ratios in nutrient solution. Ecotoxicol. Environ. Saf. 148, 441–446. doi:10.1016/j.ecoenv.2017.10.045
Zeng, G., Wan, J., Huang, D., Hu, L., Huang, C., Cheng, M., et al. (2017). Precipitation, adsorption and rhizosphere effect: the mechanisms for phosphate-induced Pb immobilization in soils-a review. J. Hazard. Mater. 339, 354–367. doi:10.1016/j.jhazmat.2017.05.038
Zhai, X., Li, Z., Huang, B., Luo, N., Huang, M., Zhang, Q., et al. (2018). Remediation of multiple heavy metal-contaminated soil through the combination of soil washing and in situ immobilization. Sci. Total Environ. 635, 92–99. doi:10.1016/j.scitotenv.2018.04.119
Zhang, B. L., Ouyang, Y. N., Xu, J. Y., and Liu, K. (2018). Cadmium remobilization from shoot to grain is related to pH of vascular bundle in rice. Ecotoxicol. Environ. Saf. 147, 913–918. doi:10.1016/j.ecoenv.2017.09.064
Zhang, C., Sale, P. W. G., and Tang, C. (2016). Cadmium uptake by Carpobrotus rossii (Haw.) Schwantes under different saline conditions. Environ. Sci. Pollut. Res. 23 (13), 13480–13488. doi:10.1007/s11356-016-6508-5
Zhang, J. Y., Zhou, H., Gu, J. F., Huang, F., Yang, W. J., Wang, S. L., et al. (2020). Effects of nano-Fe3O4-modified biochar on iron plaque formation and Cd accumulation in rice (Oryza sativa L.). Environ. Pollut. 260, 113970. doi:10.1016/j.envpol.2020.113970
Zhang, S. L., Ni, X. L., Arif, M., Yuan, Z., and Li, L. (2020). Salinity influences Cd accumulation and distribution characteristics in two contrasting halophytes, Suaeda glauca and Limonium aureum. Ecotoxicol. Environ. Saf. 191, 110230. doi:10.1016/j.ecoenv.2020.110230
Zhang, X. Q., Li, Y., Ye, J., Chen, Z., Ren, D., and Zhang, S. (2022). The spectral characteristics and cadmium complexation of soil dissolved organic matter in a wide range of forest lands. Environ. Pollut. 299, 118834. doi:10.1016/j.envpol.2022.118834
Zhang, Y., Yin, C., Cao, S., Cheng, L., Wu, G., and Guo, J. (2018). Heavy metal accumulation and health risk assessment in soil-wheat system under different nitrogen levels. Sci. Total Environ. 622, 1499–1508. doi:10.1016/j.scitotenv.2017.09.317
Zheng, X., Zhao, M., Oba, B. T., and Ding, H. (2023). Effects of organo-mineral complexes on Cd migration and transformation: from pot practice to adsorption mechanism. Int. J. Environ. Sci. Technol. 20, 579–586. doi:10.1007/s13762-022-04012-2
Zheng, X. J., Li, Q., Peng, H., Zhang, J. X., Chen, W. J., Zhou, B. C., et al. (2022). Remediation of heavy metal-contaminated soils with soil washing: a review. Sustainability 14 (20), 13058. doi:10.3390/su142013058
Zhou, Y. M., Long, S. S., Li, B. Y., Huang, Y. Y., Li, Y. J., Yu, J. Y., et al. (2020). Enrichment of cadmium in rice (Oryza sativa L.) grown under different exogenous pollution sources. Environ. Sci. Pollut. Res. 27 (35), 44249–44256. doi:10.1007/s11356-020-10282-5
Keywords: agrochemicals, chloride, rice, Cd accumulation, complex
Citation: Fan H, Tang S, Long J, He R, Xiao Z, Hou H and Peng P (2024) Effects of exogenous chloride ions on the migration and transformation of Cd in a soil-rice system. Front. Environ. Sci. 12:1403989. doi: 10.3389/fenvs.2024.1403989
Received: 20 March 2024; Accepted: 30 April 2024;
Published: 16 May 2024.
Edited by:
Qi Liao, School of Metallurgy and Environment, Central South University, ChinaReviewed by:
Hao Li, Kunming University of Science and Technology, ChinaPeng Wang, Chengdu University of Technology, China
Copyright © 2024 Fan, Tang, Long, He, Xiao, Hou and Peng. This is an open-access article distributed under the terms of the Creative Commons Attribution License (CC BY). The use, distribution or reproduction in other forums is permitted, provided the original author(s) and the copyright owner(s) are credited and that the original publication in this journal is cited, in accordance with accepted academic practice. No use, distribution or reproduction is permitted which does not comply with these terms.
*Correspondence: Jian Long, bG9uZ2ppYW5jc3VmdEB5ZWFoLm5ldA==
†These authors have contributed equally to this work and share first authorship