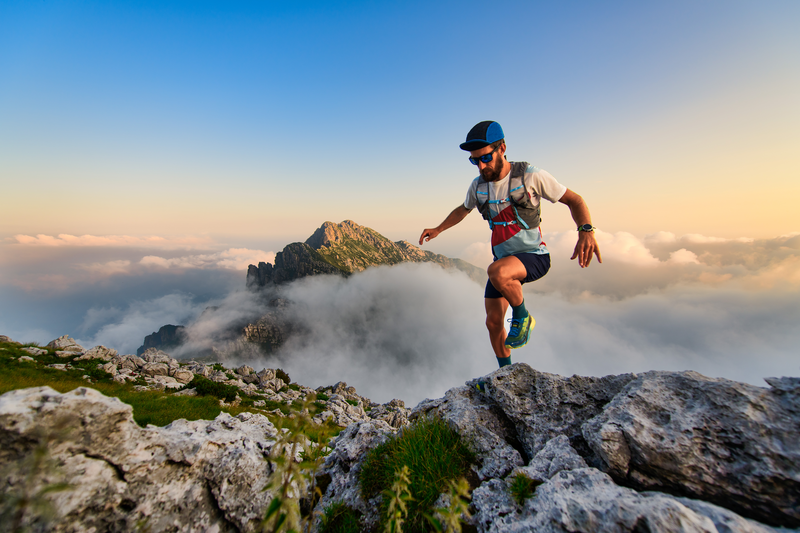
94% of researchers rate our articles as excellent or good
Learn more about the work of our research integrity team to safeguard the quality of each article we publish.
Find out more
ORIGINAL RESEARCH article
Front. Environ. Sci. , 29 August 2024
Sec. Soil Processes
Volume 12 - 2024 | https://doi.org/10.3389/fenvs.2024.1402618
This article is part of the Research Topic By-product Amendments for the Remediation of Metal- and Metalloid-contaminated Soils View all 7 articles
Introduction: The drilling industry is one of the main sectors of the oil industry, and oil drilling is one of the most specialized industrial activities. Large-scale production of sugarcane bagasse in Khuzestan Province creates an environmental opportunity to utilize this agricultural byproduct in different sections as a valuable byproduct. This study aimed to investigate the ability of the raw form of bagasse and its transformed structure in heavy metal fixation in drilling mud and to investigate the efficiency of crude bagasse, processed bagasse, and biochar adsorbents in removing heavy metals from the drilling mud residues of Ahvaz oil field.
Methods: Sampling of drilling mud waste from Mishan Geological Formation (MGF) and Aghajari Geological Formation (AGF) was done on a vibrating sieve. The treatments examined in this research include the contact time in six levels (30, 60, 90, 120, 150, and 180 min), amount of the adsorbent in three levels (0.1, 0.5, and 1 g), adsorbent type in three levels (crude bagasse, processed bagasse, and biochar), and the formation type in two levels (Aghajari and Mishan). After chemical digestion, the samples were placed in contact with crude bagasse, processed bagasse, and biochar according to the designed conditions. Then, the removal percentages of Ba, Ni, V, Cd, Fe, and Pb were calculated. Means were compared using Duncan’s test at the 1% level.
Results and Discussion : The results showed that the biochar adsorbent is the most efficient compared to other adsorbents. The maximum removal percentages of heavy metals Ba, Ni, V, Cd, Fe, and Pb by crude bagasse are 72.53%, 68.89%, 79.49%, 76.88%, 49.42%, and 85%, respectively. In addition, the results showed that the maximum removal percentages of heavy metals Ba, Ni, V, Cd, Fe, and Pb by crude bagasse are 81/72%, 83/89%, 86/67%, 83/44%, 64/41%, and 90.72%, respectively. The maximum efficiency of biochar for adsorbing heavy metals Ba, Ni, V, Cd, Fe, and Pb is 90.70%, 91.84%, 88.89%, 88.75%, 78.59%, and 97.75%, respectively. The maximum amount of heavy metals adsorbed by all adsorbents was 1 gr/L, and the adsorption efficiency increased by increasing the amount of the adsorbent from 0.2 to 1 gr/L. In examining the effect of contact time, the maximum removal percentage of heavy metals barium and cadmium was obtained in 120 min, nickel and lead in 90 min, and vanadium and iron in 60 min. After the above contact times, there was no increase in the maximum percentage of metal removal.
The drilling industry is one of the main sectors of the oil industry, and oil drilling is one of the most specialized industrial activities. In the drilling sector, there are various operational stages such as exploration of hydrocarbon reservoirs (Ma et al., 2022), oil extraction through oil wells (Yang et al., 2023), drilling of development wells, and oil transfer for petrochemical and refinery activities (Pereira et al., 2022). During the drilling and operation of oil and gas wells, significant amounts of dangerous and toxic wastes are produced, which can have harmful effects on the natural ecosystem of the region (Ismail et al., 2017). An important part of the produced waste is drilling mud, which is made up of different materials and chemical compounds and has destructive environmental effects. Drill cuttings waste, particularly from oil and gas exploration, represents a significant environmental challenge due to its hazardous components. This waste primarily consists of the solid materials that are removed from the borehole during drilling operations and contains various contaminants, including heavy metals and petroleum hydrocarbons. Drill cuttings can be categorized into two main types based on the drilling fluids used: water-based drill cuttings (WBDCs), which are generally less hazardous and are often easier to treat and manage (Sharafaddin and Onutu, 2021), and oil-based drill cuttings (OBDCs), which are more problematic due to their high content of petroleum pollutants and can exhibit mutagenic, carcinogenic, and teratogenic properties. OBDCs often contain heavy metals and other hazardous materials, necessitating careful handling and disposal to mitigate the environmental impacts (Xing et al., 2018). The disposal of drill cuttings is regulated to prevent soil, water, and air pollution. If not managed properly, these wastes can lead to significant ecological damage and may pose risks to human health. The increasing volume of drill cuttings generated, particularly with the expansion of shale gas development, has intensified the need for effective waste management strategies (Kazamias and Zorpas, 2021).
Drilling mud is inevitably discharged into the environment around the rig during and after the drilling operation and causes environmental pollution (Khanpour et al., 2014). Drilling mud has some unusual compounds such as heavy metals, which can harm the environment (Kove et al., 2021). In some oil fields, such as Ahvaz oil field, due to special geological conditions, it is necessary to use drilling mud with complex compositions for drilling operations. In this oil field, after the work is completed, the drilling waste is dumped into the ponds around the well, which are called pits. During the drilling of a well with an average depth of 3,000 m, approximately 1,000–1,500 tons of waste containing heavy metals is produced, which is left in a pit with an area of 1–1.5 ha. Heavy metals are a group of elements which when found in concentrations higher than the limits of environmental standards can be pollutants (Sarkar, 2002). After entering the food chain and bioaccumulation in living organisms, heavy metals cause disorders, diseases, and adverse effects in these organisms (Bhat et al., 2019). Heavy metals are particularly important among environmental pollutants due to their non-degradability and physiological effects on living organisms (Sardar et al., 2013). Considering the adverse effects of heavy metals on humans and the environment, heavy metal pollution has gained the attention of many governmental and regulatory organizations that seek to prevent the destruction of the environment (Benhaddya et al., 2016). Therefore, it is necessary to provide a suitable and environmentally friendly method to remove heavy metals. Fixation of heavy metals in drill cuttings is crucial for reducing their mobility and toxicity. Several methods have been developed for this purpose, such as stabilization/solidification, which involves mixing drill cuttings with binding agents (such as cement or fly ash) to immobilize heavy metals (Abdullah et al., 2022). The process reduces the leachability of contaminants, making the waste safer for disposal or potential reuse. Thermal treatment as high-temperature processes, such as thermal distillation, can effectively remove hydrocarbons and reduce heavy metal concentrations (Leonard et al., 2010). The cuttings are heated to volatilize contaminants, which can then be condensed and recovered. This method can achieve high recovery rates for oil and significantly reduce hazardous waste. Chemical treatments use chemical agents to precipitate heavy metals into less soluble forms (Opete et al., 2010). This method often involves the addition of specific reagents that react with the metals to form stable compounds, thus reducing their bioavailability and leachability. One of the appropriate methods, the adsorption method uses organic matters that have relatively high adsorption properties. The adsorption method is applied to transfer some components of the fluid phase to the surface of an adsorbent. Considering that the adsorbent is the most important component of the adsorption process, so far, different types of adsorbents have been used according to their capacity, compatibility, cost, and reproducibility (Mohammadi et al., 2010). Using agricultural residues as an adsorbent of heavy metals can prevent these negative environmental effects (Zhang et al., 2015).
The use of unconventional resources, such as industrial residues and waste materials, has shown promising potential for heavy metal fixation and immobilization in contaminated soils and water bodies. These materials can serve as cost-effective and environmentally friendly alternatives to traditional remediation methods, reducing the overall environmental footprint of the process (Karman et al., 2015; Kokkinos et al., 2022). Several types of industrial residues have been explored for heavy metal fixation, including fly ash, which is derived from coal combustion. Fly ash contains aluminosilicate materials that can effectively immobilize heavy metals through ion exchange and adsorption processes. Slag is a byproduct from metallurgical industries, and slags can be used to precipitate heavy metals and facilitate their removal from wastewater. Red mud is a waste material from alumina refineries and has a high capacity for arsenic removal from contaminated water. Zeolites are natural or synthetic aluminosilicate minerals with large surface area and can adsorb and immobilize heavy metals in soil and water (Kokkinos et al., 2022; Priya et al., 2023). These materials can be used in various remediation techniques, such as in situ immobilization, permeable reactive barriers, and wastewater treatment. Studies have reported removal efficiencies of up to 95% for heavy metals such as arsenic, lead, and cadmium.
The use of industrial residues for heavy metal fixation offers several advantages in terms of reducing the environmental footprint, such as waste recycling and resource conservation, and utilization of waste materials for remediation purposes reduces the need for landfill disposal and conserves natural resources that would otherwise be used for primary raw materials (Schlögl et al., 2023). Recycling industrial residues is generally more cost-effective than using primary raw materials for remediation as it eliminates the costs associated with extraction, processing, and transportation. In some cases, the addition of industrial residues to contaminated soils can enhance their physical and chemical properties, making them suitable for construction applications such as backfill or road subgrade (Zhang et al., 2017). By immobilizing heavy metals in situ or through wastewater treatment, the risk of contaminant migration and exposure to living organisms is significantly reduced, minimizing the overall environmental impacts (Ahmed et al., 2022).
Khuzestan Province in Iran is a significant area for sugarcane cultivation, contributing to both sugar production and the generation of by-products such as bagasse. Approximately 88,300 ha of sugarcane has been cultivated in Iran, with Khuzestan being the primary region for cultivation of this crop (Khosravirad et al., 2019). The province covers approximately 120,000 ha dedicated to sugarcane farming. The total sugarcane production in Iran is approximately 7.4 million tons annually. This production fluctuates but has shown an overall increase over the years, with a notable yield of 6.59 million tons reported in 2014 (Mohammadi et al., 2020). Bagasse is the fibrous residue left after the extraction of juice from sugarcane. In Khuzestan, it is considered a major agricultural waste product with significant potential for energy generation and other applications. The availability of bagasse in large quantities, free or low cost, and their non-toxicity toward the environment are some of the advantages of agricultural residues. Among the various agricultural residues, sugarcane bagasse is found in large quantities. Sugarcane bagasse is a waste from sugarcane cultivation and is currently left unused in the environment in Ahvaz. Therefore, the present study used three different sugarcane bagasse treatments (crude, processed, and biochar) to remove heavy metals from drilling mud.
The study area is the Ahwaz oil field in Khuzestan Province, Iran. The Ahvaz oil field is 63 km long and 6 km wide. In this area, the Mishan Geological Formation (hereafter MGF) and Aghajari Geological Formation (hereafter AGF) were considered for drilling mud sampling. The location of the study area is shown in Figure 1.
To prepare the average sample for each geological formation, the collected samples were thoroughly mixed together, following the methodology proposed by Adewole et al. (2010). This mixing process aimed to obtain a representative sample for each geological formation to be used in subsequent analyses.
This study describes the investigation of the removal percentage of six metals (barium, nickel, vanadium, cadmium, iron, and lead) in drilling mud samples from the Ahvaz oil field. The study aimed to assess the impacts of various factors including contact time, amount of the adsorbent, type of the adsorbent, and geological formation type on the removal efficiency of the target metals.
Six homogeneous samples of drilling mud were collected from the Ahvaz oil field. The samples were weighed to be approximately 1 kg each, except for the samples from the MGF and AGF, which were weighed at 3 kg each. The samples were transported to the laboratory in plastic containers with lids to ensure their integrity during transportation. A total of 324 samples were prepared for the investigation. These samples were prepared to analyze the removal percentage of the six target metals in each drilling mud sample. The factors investigated included contact time, amount of the adsorbent, type of the adsorbent, and geological formation type. For each investigated factor, different levels were considered to assess their influence on metal removal. Contact time was varied at intervals of 30, 60, 90, 120, 150, and 180 min. The amount of the adsorbent was varied at three levels: 0.1, 0.5, and 1 g/L. The type of the adsorbent was also varied, considering three types: crude, processed, and biochar bagasse. Lastly, the geological formation type was examined, with samples obtained from both the Aghajari and Mishan formations.
The DTPA1 method was used for extraction. The samples were passed through a 2-mm sieve and placed in an oven at 110°C for 24 h. Then, 2 g of dry samples was added into a 50-mL flask, and 10 mL of acid (a mixture of 100 mL of nitric acid and 300 mL of hydrochloric acid) was slowly added to the samples in a ratio of 1:3. Then, the samples were placed on the heater for 1 h at 90°C and then for 1 h at 120°C. Before boiling the solution, samples were carefully removed and made up to 1,000 mL using distilled water (Yang et al., 2012). In order to prepare the control sample (before contact with the adsorbent), six samples of the drilling mud extracts (three samples for each formation) were centrifuged and passed through a filter paper. Then, the initial concentration of heavy metals was determined by using the Phoenix-986 atomic absorption device (Ghalib and Ramal, 2021).
Sugarcane bagasse was prepared from the Amir Kabir agriculture and industry company in Khuzestan Province and transported to the laboratory. In order to remove pollutants and increase the absorption capacity, the bagasse sample was washed with nitric acid and exposed to open air for 5 days. In order to prepare processed bagasse, crude bagasse was exposed to 0.01 M NaOH solution for 6 h and then removed from the solution. Then, it was exposed to a temperature of 105°C, and after drying, it was exposed to 0.01 M NaOH three times (Lima et al., 2018). Following that, it was exposed to 0.01 M HCL solution for 6 h three more times, and finally, processed bagasse was obtained. In order to prepare the biochar adsorbent, crude bagasse was placed in an oven at 800°C for 2 h, and crude bagasse was converted into semi-active carbon. Finally, it was immersed in 0.01 M hydrochloric acid to obtain biochar (Lima et al., 2018).
Ten-milliliter samples (digested drilling mud) were placed in contact with 0.1, 0.5, and 1 g of crude, processed, and biochar bagasse. To mix the sample with the adsorbent, a shaker was used with a stirring speed of 100 rpm. In addition, the temperature of the shaker was set at 25°C. After 30, 60, 90, 120, 150, and 180 min, the samples were centrifuged and passed through a filter paper. Then, the secondary concentration of Ba, Ni, V, Cd, Fe, and Pb metals was determined using the Phoenix-986 atomic absorption device.
Equation 1 was used to calculate the percentage removal of heavy metals using adsorbents (Stylianou et al., 2007).
where R = metal removal percentage, C0 = initial metal concentration (mg/L), and Cf = secondary metal concentration (mg/L).
The variance for data was first analyzed using the Kolmogorov–Smirnov test in SAS Ver. 9.4. The normality of the data was tested. Considering that the obtained data are in the form of percentages, the arcsine method was used for the variables whose data were not normal. Then, the data analysis was carried out in four different levels (Table 1) in the form of a completely random design and based on the GLM2 procedure, and to compare the averages, Duncan’s test was used with a probability level of 1%.
The characteristics of the control drilling mud are reported in Table 2. The average pH of the drilling mud of the two formations has a significant difference (p < 0/05), and it is higher in AGF than MGF. The reason can be attributed to the change in the formation lithotype and the composition of the drilling mud. The average EC of drilling mud of the two formations has a significant difference (p < 0/05), and this value is lower for AGF than for MGF. In addition, the average chloride content of drilling mud of the two formations has a significant difference (p < 0.05). This amount for MGF is more than that for AGF. Because AGF and MGF drilling muds were water-based type, the TPH parameter was not checked because this parameter can only be measured in oil-based drilling muds. In this study, the pH, electrical conductivity (EC), and chloride content of two different types of formations, MGF and AGF, were measured. The minimum and maximum values obtained for each parameter are presented in Table 1. The pH levels of the MGF ranged from 8.1 to 8.4, while for the AGF, it ranged from 8.5 to 9.1. The electrical conductivity of the MGF varied between 169.1 and 195 ds/m, whereas for the AGF, it ranged from 61.1 to 78.4 ds/m. The chloride content of the MGF was found to be in the range of 3,000–4,000 mL/kg, while for the AGF, it varied between 1,250 and 1,750 mL/kg. These results indicate that both MGF and AGF have slightly acidic pH values, with the AGF tending to be slightly more acidic than MGF. The electrical conductivity of the AGF was found to be lower than that of the MGF, suggesting a lower concentration of dissolved salts in the AGF. Additionally, the chloride content of AGF was lower than that of MGF, indicating a lower concentration of chloride ions in AGF. The observed variations in pH, electrical conductivity, and chloride content between MGF and AGF could be attributed to differences in their composition and manufacturing processes. Further investigation is required to determine the specific factors contributing to these variations.
The characteristics of different adsorbent treatments are also given in Table 3. The percentage of carbon (C), nitrogen (N), and electrical conductivity (EC) of biochar is higher than that of crude and processed bagasse because it is decomposed by heat (Olabemiwo et al., 2017). The results of the study are presented in Table 1, which provides the data for the adsorbent properties, including electrical conductivity (EC), pH, carbon content (C), and nitrogen content (N). The three types of bagasse analyzed in this study were crude bagasse, processed bagasse, and biochar. The crude bagasse exhibited an electrical conductivity of 2.3 ds/m, a pH of 7.2, a carbon content of 42.1%, and a nitrogen content of 11.9%. In comparison, the processed bagasse had a slightly higher electrical conductivity of 2.6 ds/m, a pH of 9.5, a carbon content of 62.3%, and a nitrogen content of 20.6%. The biochar had the highest electrical conductivity of 2.8 ds/m, a pH of 10.2, a carbon content of 69.7%, and a nitrogen content of 22.3%.
The differences between the three types of bagasse can be observed in the electrical conductivity values. The processed bagasse had a slightly higher electrical conductivity compared to the crude bagasse, and the biochar had the highest electrical conductivity among all three types. This indicates that the processing and biochar production methods had an effect on the electrical conductivity of the bagasse.
The pH values also varied among the different types of bagasse. The crude bagasse had a pH of 7.2, which can be considered slightly acidic. The processed bagasse had a pH of 9.5, indicating a more alkaline nature. The biochar had the highest pH of 10.2, suggesting a highly alkaline character. These pH differences reflect the changes in the chemical composition and structure of the bagasse during processing and biochar production.
The carbon content and nitrogen content in the bagasse also showed variations. The crude bagasse had a carbon content of 42.1% and a nitrogen content of 11.9%. The processed bagasse exhibited a significantly higher carbon content of 62.3% and a nitrogen content of 20.6%. The biochar had the highest carbon and nitrogen contents among the three types, with values of 69.7% and 22.3%, respectively. These differences indicate that the processing and biochar production methods resulted in an increase in the carbon and nitrogen contents of the bagasse. Overall, the results demonstrate that the processing and biochar production methods had an impact on the electrical conductivity, pH, carbon content, and nitrogen content of the bagasse. The processed bagasse and biochar exhibited higher electrical conductivity, pH, carbon content, and nitrogen content than the crude bagasse. These findings suggest that the processing and biochar production methods can potentially enhance the adsorption properties of bagasse, making it a more effective adsorbent material. It is important to note that these results are based on the specific samples analyzed in this study and may not be representative of all bagasse samples. Further research is needed to validate these findings and investigate the potential applications of processed bagasse and biochar as adsorbents in different environmental and industrial contexts.
The results for ANOVA are reported in Table 4. The results presented in this table show that all main effects and interaction effects are significant (except B*D, C*D, and A*C*D for cadmium; A*C*D for iron; and B*D, A*C*D, and B*C*D for lead). The results of the study are presented in Table 4, which shows the source of changes for each element (Ba, Ni, V, Cd, Fe, and Pb) under different factors (geological formation type, adsorbent type, amount of the adsorbent, and time) and their interactions. Geological formation type (A) had a significant effect on the concentration of all elements, except Ni. The concentrations of Ba, V, Cd, Fe, and Pb were significantly influenced by the type of the geological formation. The highest concentration of Ba was observed in geological formation type A (7.018), followed by B (82.63). Similar trends were observed for V, Cd, Fe, and Pb, with higher concentrations in formation type A than in B. However, the concentration of Ni was not significantly affected by geological formation type. Adsorbent type (B) also showed a significant effect on the concentration of all elements, except Ni. The concentrations of Ba, V, Cd, Fe, and Pb were significantly influenced by the type of the adsorbent used. The highest concentration of Ba was observed with adsorbent type B (82.63), followed by A (7.018). Similar trends were observed for V, Cd, Fe, and Pb, with higher concentrations in adsorbent type B than in A. Again, the concentration of Ni was not significantly affected by the adsorbent type. The amount of adsorbent (C) had a significant effect on the concentration of all elements, except Ni and Cd. The concentrations of Ba, V, Fe, and Pb were significantly influenced by the amount of the adsorbent used. Higher concentrations of Ba, V, Fe, and Pb were observed with larger amounts of the adsorbent. However, the concentration of Cd did not show a significant response to the amount of the adsorbent. Time (D) also showed a significant effect on the concentration of all elements, except Ni and Cd. The concentrations of Ba, V, Fe, and Pb were significantly influenced by the duration of exposure. Longer exposure times resulted in higher concentrations of Ba, V, Fe, and Pb. However, the concentration of Cd did not show a significant response to time. The interactions between factors were also investigated. Among these interactions, only A*B*C and B*C*D showed significant effects on the concentrations of certain elements. A*B*C had a significant effect on the concentrations of Ba, V, and Fe, while B*C*D had a significant effect on the concentrations of Ba, V, and Pb. The coefficients of variation (CV %) for each element were also calculated. The CV % values represent the variability of the measurements within each factor. The CV % values ranged from 5.05% to 7.28%, indicating moderate variability in the measurements. Overall, the results of this study demonstrate that the source of changes in the concentrations of Ba, Ni, V, Cd, Fe, and Pb is primarily influenced by the geological formation type, adsorbent type, amount of the adsorbent, and time. The interactions between these factors also play a role in determining the concentrations of certain elements. These findings contribute to our understanding of the factors influencing the distribution and behavior of these elements in geological formations, which has implications for environmental and geological studies.
The results of Duncan’s test for comparing the means of the main effects are given in Figures 2–7. The results of Figure 2 (Ba metal) show that the highest amount of adsorption for the type of formation, the type of the adsorbent, the amount of the adsorbent, and the time are, respectively, related to the MGF and biochar, the amount of adsorbent is 1 gr, and the time is 120–180 min. Likewise, for Ni, the highest amount of adsorption is related to AGF and biochar, the amount of the adsorbent is 1 gr, and time is 90–180 min. Keeping the previous order for V, the highest amount of adsorption is related to AGF and biochar, the amount of the adsorbent is 1 gr, and time is 60–180 min. In addition, respectively, for Va, the highest amount of adsorption is related to MGF and for biochar, the amount of the adsorbent is 1 gr, and time is 120–180 min. In the same way, for Fe, the highest amount of adsorption is related to MGF, and for biochar, the amount of the adsorbent is 1 gr, and time is 60–180 min. Finally, for Pb, the highest amount of adsorption is related to MGF, and for biochar, the amount of the adsorbent is 1 gr, and time is 90–180 min.
It should be noted that due to the limitation of the number of pages in the article, the results of Duncan’s test are presented only for two-level interaction effects in Tables 5–10.
The results reported in Table 5 show that the highest amount of absorption for Ba, Ni, Cd, Fe, and Pb is the interaction effect of MGF–biochar. For V, the maximum amount of absorption is the interaction effect of AGF–biochar. The results indicate that the concentrations of heavy metals varied across the different treatment groups and forms. For Ba, the highest concentration was observed in the biochar form in the AGF treatment group (81.53 mg/kg). In the MGF treatment group, the highest concentration was found in the processed form (76.19 mg/kg). The lowest concentration of Ba was observed in the biochar form in the MGF treatment group (62.02 mg/kg). Similarly, for Ni, the highest concentration was found in the biochar form in the AGF treatment group (84.94 mg/kg). In the MGF treatment group, the highest concentration was observed in the processed form (79.15 mg/kg). The lowest concentration of Ni was found in the processed form in the MGF treatment group (64.87 mg/kg). For V, the highest concentration was observed in the processed form in the AGF treatment group (84.83 mg/kg). In the MGF treatment group, the highest concentration was found in the crude form (77.19 mg/kg). The lowest concentration of V was observed in the biochar form in the MGF treatment group (76.04 mg/kg). In the case of Cd, the highest concentration was found in the biochar form in the AGF treatment group (82.55 mg/kg). In the MGF treatment group, the highest concentration was observed in the crude form (75.36 mg/kg). The lowest concentration of Cd was found in the processed form in the MGF treatment group (69.17 mg/kg). For Fe, the highest concentration was observed in the biochar form in the AGF treatment group (76.5 mg/kg). In the MGF treatment group, the highest concentration was found in the processed form (68.66 mg/kg). The lowest concentration of Fe was observed in the processed form in the MGF treatment group (62.88 mg/kg). Lastly, for Pb, the highest concentration was found in the crude form in the AGF treatment group (91.62 mg/kg). In the MGF treatment group, the highest concentration was observed in the processed form (86.14 mg/kg). The lowest concentration of Pb was found in the biochar form in the MGF treatment group (70.35 mg/kg). Overall, the results indicate that the concentrations of heavy metals varied depending on the treatment group and form. Further statistical analysis is required to determine if these differences are statistically significant.
The results in Table 6 indicate that the highest amount of absorption for Ba, Cd, Fe, and Pb is the interaction effect of the MGF–1 gr adsorbent. For the Ni and V, the highest adsorption value is the interaction of the AGF–1 gr adsorbent.
The results related to the interaction effect of the formation type and time in the adsorption of heavy metals are given in Table 7. The maximum amount of adsorption for Ba, Ni, V, Cd, Fe, and Pb is presented in six parentheses, respectively: (MGF–time 120, 150, and 180), (AGF–time 90, 120, 150, and 180), (AGF–time 60, 90, 120, 150, and 180), (MGF–time 120, 150, and 180), (MGF–time 60, 90, 120, 150, and 180), and (MGF–time 90, 120, 150, and 180).
In Table 8, the interaction effect of the adsorbent type and the amount of the adsorbent can be seen. These results show that the highest amount of adsorption for the metals is related to the interaction effect of biochar–1 gr of the adsorbent.
The results related to the interaction effect of bagasse type and time in the adsorption of heavy metals are given in Table 9. The maximum amount of adsorption for all metals is presented in six parentheses: (biochar–time 120,150, and 180), (biochar–time 90, 120, 150, and 180), (biochar–time 60, 90, 120, 150, and 180), (biochar–time 120, 150, and 180), (biochar–time 60, 90, 120, 150, and 180), and (biochar–time 90, 120, 150, and 180).
The results related to the interaction effect for the amount of adsorbent (gr) and time in the adsorption of heavy metals are given in Table 10. The maximum amount of adsorption for all metals is presented in six parentheses: (1 gr of adsorbent–time 120, 150, and 180), (1 gr of adsorbent–time 90, 120, 150, and 180), (1 gr of adsorbent–time 60, 90, 120, 150, and 180), (1 gr of adsorbent–time 120, 150, and 180), (1 gr of adsorbent–time 60, 90, 120, 150, and 180), and (1 gr of adsorbent–time 90, 120, 150, and 180).
First, the effectiveness of different adsorbents in removing heavy metals was evaluated. It was found that all adsorbents were effective to some extent, but biochar demonstrated the highest efficiency compared to other adsorbents. This observation is consistent with those of previous studies conducted by Isa et al. (2020) and Homagai et al. (2010), which highlighted the high efficiency of crude bagasse in removing heavy metals. Additionally, Liang et al. (2021) and Lee and Shin (2021) also reported on the high efficiency of biochar in heavy metal removal. These findings support the notion that biochar has superior adsorption capacity due to the processing and pyrolysis of bagasse, resulting in increased metal absorption. The adsorption rate of heavy metals was found to increase with the increasing initial concentration of heavy metals in the wastewater. This trend was observed for both types of biochar investigated. Baltrėnaitė-Gedienė and Šilinskaitė (2016) also reported similar results, demonstrating that increasing the concentration of biochar in wastewater enhances the absorption and removal efficiency of heavy metals, including cadmium, zinc, lead, and copper. The present study supports these findings by showing that the amount of heavy metals absorbed on both processed bagasse and biochar followed the reports of previous literature, with iron and lead exhibiting the highest amounts absorbed on the adsorbents, consistent with their higher initial concentrations. The formation type and amount of adsorbent were also found to significantly influence the removal percentage of lead. Specifically, the highest removal percentage was obtained in the treatments of AGF and MGF in the biochar adsorbent, with an adsorbent amount of 1 g. Increasing the amount of the adsorbent from 0.2 g/L to 1 g/L resulted in an increase in the absorption efficiency. This finding aligns with that of the study conducted by Baltrėnaitė-Gedienė and Šilinskaitė (2016), who also reported that increasing the concentration of biochar in wastewater leads to a higher absorption and removal efficiency of heavy metals. The effect of contact time on heavy metal removal was investigated, and it was observed that the maximum removal percentage of different heavy metals varied with time. The maximum removal percentages of barium and cadmium were obtained at 120 min, for nickel and lead at 90 min, and for vanadium and iron at 60 min. After these contact times, no further increase in the maximum percentage of metal removal was observed, indicating that equilibrium had been reached. This suggests that in the initial stages, there are more available sites for absorption, making it easier for the adsorbent to connect with the absorption sites. However, after the equilibrium time, the unoccupied surfaces on the adsorbent become limited, thereby reducing the overall adsorption capacity. In conclusion, the findings of this study demonstrate that the parameters of adsorbent type, amount of adsorbent, formation type, and contact time all play a significant role in the removal percentage of heavy metals. Biochar was found to be the most effective adsorbent compared to crude and processed bagasse, exhibiting higher adsorption capacities for various heavy metals. The results also support those of previous literature on the efficiency of crude bagasse in heavy metal removal and the enhanced capacity of biochar in adsorbing heavy metals. Understanding these parameters and their influence on heavy metal removal is crucial for the development of effective and sustainable wastewater treatment strategies.
The maximum efficiency of adsorption of heavy metals Ba, Ni, V, Ca, Fe, and Pb by crude bagasse is 53.72%, 68.89%, 79.49%, 76.88%, 49.42%, and 85%, respectively. In addition, the results showed that the maximum efficiency of removing heavy metals Ba, Ni, V, Ca, Fe, and Pb using processed bagasse is 81.72%, 83.89%, 86.67%, 83.44%, 64.41%, and 90.72%, respectively. Baltrėnaitė-Gedienė and Šilinskaitė (2016) reported that as the initial concentration of heavy metals in the wastewater increased, the adsorption rate of all heavy metals (cadmium, zinc, lead, and copper) increased by both types of biochar investigated. In this study, the amount of heavy metals absorbed on processed bagasse and biochar was similar to those found in the previous literature, and iron and lead, which had the highest concentration in the initial solution, had the highest amount absorbed on the adsorbents. According to Lajmiri Orak et al. (2021), the application of conocarpus waste (CW) biochar to drill cuttings improved soil properties such as pH, CEC, SOC, and EC. The use of CW biochar, especially at a 10% application rate, reduced the mobility and bioavailability of copper and zinc in the DC samples. The Tessier sequential extraction method showed that the exchangeable fraction of Cu and Zn decreased by 2.34% and 4.13%, respectively, in the 10% biochar treatment compared to the control. The 10% biochar treatment also had a greater effect on reducing the exchangeable and carbonate fractions of Cu and Zn, while increasing the organic matter and residual fractions.
The comparison of the mean interaction effect on the removal percentage of lead showed that the highest removal percentage was obtained in the treatments of AGF and MGF in the biochar adsorbent and the amount of the adsorbent was 1 gr, and by increasing the amount of the adsorbent from 0.2 to 1 g/L, the absorption efficiency increased. Baltrėnaitė-Gedienė and Šilinskaitė (2016) also obtained a similar result and showed that increasing the concentration of biochar in wastewater increases the absorption and removal efficiency of all heavy metals (cadmium, zinc, lead, and copper). Orak et al. (2022) found that the addition of conocarpus biochar, especially at 10% concentration, significantly reduced the bioavailability and mobility of Cd, Cu, and Zn in the drilling waste samples. The biochar treatment reduced the carbonate and exchangeable fractions of the heavy metals, while increasing their distribution in more stable forms, as indicated by the reduced mobility factor and increased partition index. The biosorption kinetics followed a pseudo-second-order model, indicating fast adsorption kinetics. In examining the effect of contact time, the maximum removal percentage of barium and cadmium was obtained in 120 min, for nickel and lead in 90 min, and for vanadium and iron in 60 min. After the above contact times, there was no increase in the maximum percentage of metal removal, so these contact times were considered the equilibrium time. In the early times, there were more sites available for absorption. Therefore, it is easier to connect the absorbing component to the absorption sites. After the equilibrium time, the unoccupied surfaces on the adsorbent surface can hardly be used.
Agricultural residues, such as sugarcane bagasse, have also been explored for heavy metal fixation and immobilization. Among the different adsorbents, biochar derived from bagasse demonstrated the highest efficiency in removing heavy metals. The efficiency of heavy metal removal varied depending on factors such as the formation type, adsorbent type, amount of adsorbent, and contact time. Overall, these findings contribute to the development of effective and sustainable waste management strategies in the drilling industry. The obtained observations from the performed study highlight the potential ability of sugarcane bagasse as an ecofriendly and sustainable agricultural byproduct for remediation of soil-polluted ecosystems. In the industrial scale, linking the agricultural sector and industrial pollution concerns such as drilling activity in regions like Khuzestan Province can be seen as a creative way in forming a circular economy and improving environmental health at the regional scale.
The original contributions presented in the study are included in the article/Supplementary Material; further inquiries can be directed to the corresponding author.
FS: conceptualization and writing–review and editing. SA: investigation, methodology, resources, and writing–original draft. MR: data curation, validation, and writing–review and editing. AE: formal analysis, methodology, visualization, and writing–review and editing.
The author(s) declare that no financial support was received for the research, authorship, and/or publication of this article.
The authors declare that the research was conducted in the absence of any commercial or financial relationships that could be construed as a potential conflict of interest.
All claims expressed in this article are solely those of the authors and do not necessarily represent those of their affiliated organizations, or those of the publisher, the editors, and the reviewers. Any product that may be evaluated in this article, or claim that may be made by its manufacturer, is not guaranteed or endorsed by the publisher.
1Diethylene triamine penta acetic acid.
2Generalized linear model.
Abdullah, R. M., Othman, M. A., and Abdullah, S. M. (2022). Treatment of oil well drill cuttings utilizing different binder options. Sci. J. Univ. Zakho 10, 140–146. doi:10.25271/sjuoz.2022.10.3.945
Adewole, G. M., Adewale, T. M., and Ufuoma, E. (2010). Environmental aspect of oil and water-based drilling muds and cuttings from Dibi and Ewan off-shore wells in the Niger Delta, Nigeria Gbadebo. Afr. J. Environ. Sci. Technol. 4.
Ahmed, S. F., Kumar, P. S., Rozbu, M. R., Chowdhury, A. T., Nuzhat, S., Rafa, N., et al. (2022). Heavy metal toxicity, sources, and remediation techniques for contaminated water and soil. Environ. Technol. and Innov. 25, 102114. doi:10.1016/j.eti.2021.102114
Baltrėnaitė-Gedienė, E., and Šilinskaitė, J. (2016). Biochar as adsorbent for removal of heavy metal ions Cadmium (II), Copper (II), Lead (II), Zinc (II) from aqueous phase. Int. J. Environ. Sci. Technol. 13, 471–482. doi:10.1007/s13762-015-0873-3
Benhaddya, M. L., Boukhelkhal, A., Halis, Y., and Hadjel, M. (2016). Human health risks associated with metals from urban soil and road dust in an oilfield area of Southeastern Algeria. Arch. Environ. Contam. Toxicol. 70, 556–571. doi:10.1007/s00244-015-0244-6
Bhat, S. A., Hassan, T., and Majid, S. (2019). Heavy metal toxicity and their harmful effects on living organisms–a review. Int. J. Med. Sci. Diagnosis Res. 3, 106–122.
Ghalib, H. S., and Ramal, M. M. (2021). Spatial and temporal water quality evaluation of heavy metals of Habbaniyah Lake, Iraq. Int. J. Des. Nat. Ecodynamics 16, 467–475. doi:10.18280/ijdne.160414
Homagai, P. L., Ghimire, K. N., and Inoue, K. (2010). Preparation and characterization of charred xanthated sugarcane bagasse for the separation of heavy metals from aqueous solutions. Sep. Sci. Technol. 46, 330–339. doi:10.1080/01496395.2010.506903
Isa, Y. M., Harripersadth, C., Musonge, P., Sayago, A., and Morales, M. G. (2020). The application of eggshells and sugarcane bagasse as potential biomaterials in the removal of heavy metals from aqueous solutions. South Afr. J. Chem. Eng. 34, 142–150. doi:10.1016/j.sajce.2020.08.002
Ismail, A. R., Alias, A. H., Sulaiman, W. R. W., Jaafar, M. Z., and Ismail, I. (2017). Drilling fluid waste management in drilling for oil and gas wells. Chem. Eng. Trans. 56, 1351–1356. doi:10.3303/CET1756226
Karman, S. B., Diah, S. Z. M., and Gebeshuber, I. C. (2015). Raw materials synthesis from heavy metal industry effluents with bioremediation and phytomining: a biomimetic resource management approach. Adv. Mater. Sci. Eng. 2015, 185071–185121. doi:10.1155/2015/185071
Kazamias, G., and Zorpas, A. A. (2021). Drill cuttings waste management from oil and gas exploitation industries through end-of-waste criteria in the framework of circular economy strategy. J. Clean. Prod. 322, 129098. doi:10.1016/j.jclepro.2021.129098
Khanpour, R., Sheikhi-Kouhsar, M. R., Esmaeilzadeh, F., and Mowla, D. (2014). Removal of contaminants from polluted drilling mud using supercritical carbon dioxide extraction. J. Supercrit. Fluids 88, 1–7. doi:10.1016/j.supflu.2014.01.004
Khosravirad, M., Omid, M., Sarmadian, F., and Hosseinpour, S. (2019). Predicting sugarcane yields in khuzestan using a large time-series of remote sensing imagery region. Int. Arch. Photogram. Remote Sens. Spat. Inform. Sci 42, 645–648. doi:10.5194/isprs-archives-xlii-4-w18-645-2019
Kokkinos, N., Nkagbu, D., Marmanis, D., Dermentzis, K., and Maliaris, G. (2022). Evolution of unconventional hydrocarbons: past, present, future and environmental FootPrint. J. Eng. Sci. and Technol. Rev. 15, 15–24. doi:10.25103/jestr.154.03
Kove, E. A., Buah, W. K., Dankwa, O. K., and Mends, E. A. (2021). Attenuation of heavy metals from waste oil-based drilling mud using locally produced coconut shell-based activated carbon. Ghana Min. J. 21, 55–61. doi:10.4314/gm.v21i2.7
Lajmiri Orak, Z., Sabzalipour, S., Panahpour, E., Attar Roshan, S., and Tavakkoli, H. (2021). Chemical fractionation of copper and zinc after addition of conocarpous waste biochar to a drill cutting. J. Adv. Environ. Health Res. 9, 57–68. doi:10.32598/jaehr.9.1.1202
Lee, H.-S., and Shin, H.-S. (2021). Competitive adsorption of heavy metals onto modified biochars: comparison of biochar properties and modification methods. J. Environ. Manag. 299, 113651. doi:10.1016/j.jenvman.2021.113651
Leonard, S. A., Roy, A. D., and Stegemann, J. A. (2010). Stabilization/solidification of petroleum drill cuttings: thermal and microstructural studies of binder hydration products. Environ. Eng. Sci. 27, 889–903. doi:10.1089/ees.2010.0147
Liang, M., Lu, L., He, H., Li, J., Zhu, Z., and Zhu, Y. (2021). Applications of biochar and modified biochar in heavy metal contaminated soil: a descriptive review. Sustainability 13, 14041. doi:10.3390/su132414041
Lima, C. S., Rabelo, S. C., Ciesielski, P. N., Roberto, I. C., Rocha, G. J., and Driemeier, C. (2018). Multiscale alterations in sugar cane bagasse and straw submitted to alkaline deacetylation. ACS Sustain. Chem. and Eng. 6, 3796–3804. doi:10.1021/acssuschemeng.7b04158
Ma, K., Pang, H., Zhang, L., Huang, S., Huo, X., and Chen, J. (2022). Hydrocarbon dynamic field division and its relevance to oil and gas exploration for Paleogene reservoir in Lufeng Depression. Adv. Geo-Energy Res. 6, 415–425. doi:10.46690/ager.2022.05.06
Mohammadi, F., Roedl, A., Abdoli, M. A., Amidpour, M., and Vahidi, H. (2020). Life cycle assessment (LCA) of the energetic use of bagasse in Iranian sugar industry. Renew. Energy 145, 1870–1882. doi:10.1016/j.renene.2019.06.023
Mohammadi, M., Fotovat, A., and Haghnia, G. (2010). Heavy metals removal from industrial wastewater by sand, soil and organic matter. J. Water Wastewater; Ab va Fazilab (in Persian) 20, 71–81.
Olabemiwo, F. A., Tawabini, B. S., Patel, F., Oyehan, T. A., Khaled, M., and Laoui, T. (2017). Cadmium removal from contaminated water using polyelectrolyte-coated industrial waste fly ash. Bioinorg. Chem. Appl. 2017, 1–13. doi:10.1155/2017/7298351
Opete, S. O., Mangibo, I. A., and Iyagba, E. T. (2010). Stabilization/solidification of synthetic Nigerian drill cuttings. Afr. J. Environ. Sci. Technol. 4, 149–153. doi:10.5897/ajest09.012
Orak, Z. L., Sabzalipour, S., Panahpour, E., Roshan, S. A., and Tavakkoli, H. (2022). Thermodynamics and removal process of heavy metals from drilled mud water. Selecting a better model of adsorption isotherm. Desalin. Water Treat. 267, 138–152. doi:10.5004/dwt.2022.28635
Pereira, L. B., Sad, C. M., Castro, E. V., Filgueiras, P. R., and Lacerda, V. (2022). Environmental impacts related to drilling fluid waste and treatment methods: a critical review. Fuel 310, 122301. doi:10.1016/j.fuel.2021.122301
Priya, A., Muruganandam, M., Ali, S., and Kornaros, M. (2023). Clean-up of heavy metals from contaminated soil by phytoremediation: a multidisciplinary and eco-friendly approach. Toxics 11 (5), 422. doi:10.3390/toxics11050422
Sardar, K., Ali, S., Hameed, S., Afzal, S., Fatima, S., Shakoor, M. B., et al. (2013). Heavy metals contamination and what are the impacts on living organisms. GJESM 2, 172–179. doi:10.15580/gjemps.2013.4.060413652
Schlögl, S., Diendorfer, P., Baldermann, A., and Vollprecht, D. (2023). Use of industrial residues for heavy metals immobilization in contaminated site remediation: a brief review. Int. J. Environ. Sci. Technol. 20, 2313–2326. doi:10.1007/s13762-022-04184-x
Sharafaddin, O., and Onutu, I. (2021). An overview of oil based drill cuttings waste environmental effect and disposal treatments. Rom. J. Pet. Gas Technol. 2, 39–47. doi:10.51865/jpgt.2021.01.04
Stylianou, M. A., Kollia, D., Haralambous, K.-J., Inglezakis, V. J., Moustakas, K. G., and Loizidou, M. D. (2007). Effect of acid treatment on the removal of heavy metals from sewage sludge. Desalination 215, 73–81. doi:10.1016/j.desal.2006.11.015
Xing, S., Wang, J., and Feng, L. (2018). Disposal technology of waste oil drilling cuttings in drilling engineering. IOP Conf. Ser. Earth Environ. Sci. 208, 012093. doi:10.1088/1755-1315/208/1/012093
Yang, J., Sun, J., Wang, R., and Qu, Y. (2023). Treatment of drilling fluid waste during oil and gas drilling: a review. Environ. Sci. Pollut. Res. Int. 30, 19662–19682. doi:10.1007/s11356-022-25114-x
Yang, Z., Zhang, S., Liao, Y., Li, Q., Wu, B., and Wu, R. (2012). Remediation of heavy metal contamination in calcareous soil by washing with reagents: a column washing. Procedia Environ. Sci. 16, 778–785. doi:10.1016/j.proenv.2012.10.106
Zhang, Z.-Y., Abuduwaili, J., and Jiang, F.-Q. (2015). Sources, pollution statue and potential ecological risk of heavy metals in surface sediments of Aibi Lake, Northwest China. Huan Jing Ke Xue 36, 490–496.
Keywords: bagasse, biochar, drilling mud, heavy metals, soil treatment
Citation: Saffarian F, Hosseini SA, Mohammadi Roozbahani M and Etminan A (2024) Evaluation of heavy metal fixation ability from drilling waste of oil and gas wells using treated sugarcane bagasse. Front. Environ. Sci. 12:1402618. doi: 10.3389/fenvs.2024.1402618
Received: 17 March 2024; Accepted: 02 August 2024;
Published: 29 August 2024.
Edited by:
Rubén Forján, University of Oviedo, SpainReviewed by:
Nikolaos C. Kokkinos, Democritus University of Thrace, GreeceCopyright © 2024 Saffarian, Hosseini, Mohammadi Roozbahani and Etminan. This is an open-access article distributed under the terms of the Creative Commons Attribution License (CC BY). The use, distribution or reproduction in other forums is permitted, provided the original author(s) and the copyright owner(s) are credited and that the original publication in this journal is cited, in accordance with accepted academic practice. No use, distribution or reproduction is permitted which does not comply with these terms.
*Correspondence: Seyed Ahmad Hosseini, aG9zc2VpbmlzZXllZGFobWFkMDRAZ21haWwuY29t
Disclaimer: All claims expressed in this article are solely those of the authors and do not necessarily represent those of their affiliated organizations, or those of the publisher, the editors and the reviewers. Any product that may be evaluated in this article or claim that may be made by its manufacturer is not guaranteed or endorsed by the publisher.
Research integrity at Frontiers
Learn more about the work of our research integrity team to safeguard the quality of each article we publish.