- 1Chongqing Gas Field, PetroChina Southwest Oil and Gas Field Company, Chongqing, China
- 2Collaborative Innovation Center of Shale Gas Resources and Environment, Chengdu, China
- 3Exploration Division, PetroChina Southwest Oil and Gas Field Company, Chengdu, China
- 4Sichuan Geotech Science and Technology Ltd., Chengdu, China
Due to global geological events and differences in regional sedimentary environments, marine shale reservoirs of Wufeng-Longmaxi Formation in Eastern and Southern Sichuan Basin exhibit significant heterogeneity in organic matter content and mineral composition. In order to reveal the influence of paleoenvironment evolution on reservoir heterogeneity, key geochemical indicators of elements were used to reconstruct the sedimentary environment of marine shale in Eastern and Southern Sichuan Basin. The influence mechanism of paleoenvironment on organic matter content and mineral components was also explored. The results indicate that the Wufeng-Longmaxi Formation in the Southern and Eastern Sichuan Basin can be divided into two third-order sequences (Sq 1 and Sq 2). Each third-order sequence is divided into a transgressive system tract (TST) and a highstand system tract (HST). The average TOC content in the Eastern Sichuan Basin is the highest during the TST1 period with reaching 4.2%, while reached its maximum at 3.9% during the TST2 period in the Southern Sichuan Basin. Due to the influence of high paleo-productivity, the organic matter accumulation and quartz content in the eastern Sichuan region were higher than those in the southern Sichuan region from the TST1 to the middle TST2 period. However, the organic matter accumulation and quartz content in the late TST2 period were lower than those in the southern Sichuan region due to the dilution of terrestrial debris. During the HST2 period, due to the influence of higher paleo-productivity, clay adsorption and preservation condition, the TOC content in the eastern Sichuan region slightly increased in the early stage. At the same time, the marine shale in the southern Sichuan region has a high content of quartz minerals and a low content of clay minerals due to strong weathering intensity and input of coarse-grained debris (silt-size quartz).
Introduction
The Ordovician-Silurian black shale in the Sichuan Basin is a key contributor to China’s breakthrough in shale gas production (Chen et al., 2017a; Jiang et al., 2022). At present, a gas field with an annual production capacity of 20 billion cubic meters has been built in the Ordovician-Silurian black shale with a burial depth of less than 3500 m in the Changning, Weiyuan, and Zhaotong areas in the Southern Sichuan Basin (Chen et al., 2017b; Cai et al., 2023; Fan et al., 2024). However, the Ordovician-Silurian black shale with a burial depth of less than 3500 m only accounts for 16% of the shale gas geological resources (Fu et al., 2019; Dong D. et al., 2022; Li, 2023a), and deep (burial depth>3500 m) marine shale gas is clearly the main driver of future production growth (Fu et al., 2021a; Fu et al., 2021b; Li, 2023b). Due to the influence of regional tectonic environment, there are significant differences in the sedimentary thickness and paleoenvironment of the Ordovician-Silurian shale in the Eastern and Southern Sichuan Basin (Guo et al., 2019; Jin et al., 2020; Huang et al., 2023). The degree of organic matter enrichment and mineral composition in the shale will also vary. Therefore, restoring the paleo-sedimentary evolution process of shale and clarifying its impact on the distribution of organic-rich intervals and mineral components is of great significance for promoting efficient shale gas exploration in the Eastern and Southern Sichuan Basin (Jiang et al., 2018; Wei et al., 2021).
The Ordovician-Silurian black shale was formed during a turbulent geological history transition period (Katian to Aeronian), influenced by global and regional geological events such as volcanic eruptions, orogeny, glaciation, biotic extinction, and global sea-level changes (Wu et al., 2018; Dong T. et al., 2022; Li et al., 2022). In order to analyze the evolutionary characteristics of the black shale sedimentary environment, logging curve cycles and distribution of biogenic graptolite zones were used to establish a sequence stratigraphic framework (Wang et al., 2019), which is helpful for the comparative study of subsequent paleoenvironmental evolution. The sedimentary paleoenvironment mainly affects the enrichment of organic matter by controlling the accumulation process of organic matter (primary productivity, redox conditions, terrestrial input, sedimentation rate), and has produced three commonly used models: the “preservation model”, the “productivity model”, and the most common “multi-factor control model” (Armstrong et al., 2009; Berry, 2010; Zhao et al., 2019). In addition, the indirect impact of global or regional geological events on shale sedimentation and organic matter enrichment should not be ignored (Song et al., 2023; Xie et al., 2023). The control effect of sedimentary paleoenvironment on shale mineral composition can be summarized in three aspects: firstly, it affects the development of biogenic siliceous quartz or biogenic calcareous carbonate (Cai et al., 2022; Chen et al., 2023); secondly, it directly affects the type and content of mineral components in sedimentary areas through input from source and terrestrial sources (Wu et al., 2022); The third is the enrichment of minerals such as authigenic carbonates and pyrite (Liu et al., 2019; Chen et al., 2023).
Compared with the abundant achievements of previous studies, sedimentary and elemental geochemistry methods have been applied in this study, and multiple paleo-environmental factors such as paleo-redox, paleo-productivity, terrestrial debris input, and paleo-climate have been discussed. Under the isochronous stratigraphic framework, the evolutionary characteristics of paleoenvironment in the Eastern and Southern Sichuan Basin have been identified, and the shale sedimentation process has been restored. Meanwhile, the impact of sedimentation on the distribution and mineral composition of organic-rich shale has been discussed. The research results provide a reference basis for predicting and optimizing the “sweet spot area” of shale gas in the Sichuan Basin.
Geological setting
The Ordovician-Silurian black shale was formed during the extinction of the South China Basin and the formation of the South China Orogenic Belt. After the Middle Ordovician, the Huaxia block collided with the Yangtze block. As the main part of the Upper Yangtze Plate, the Sichuan Basin and its periphery have entered the stage of foreland basin evolution (Zhang et al., 2020; Wang et al., 2022). During the Late Ordovician to Early Silurian, with the strengthening of compression from southeast to northwest, the paleo-uplift in the Central Sichuan Basin and the paleo-uplift in the Central Guizhou-Xuefeng outside the basin alternately uplifted (Jiang et al., 2019; Jiang et al., 2020), resulting in the distribution of the foreland depression belt in the “three uplifts sandwiched with one depression” (Zhang et al., 2022), and the sedimentary facies and provenance regions were controlled by the peripheral uplifts. Between the paleo-uplifts, there are semi-closed stagnant basins, forming two depression centers in Southern and Eastern Sichuan (Figure 1). The northern part of southern Sichuan is located at the southern edge of the Leshan-Longnvsi paleo-uplift, while the southern part is blocked by the Central Guizhou paleo-uplift and the Xuefeng paleo-land, forming a semi-enclosed basin with strong water limitations. Due to its proximity to the Central Guizhou paleo-uplift, terrigenous debris material may mainly come from the foreland uplift zone, including the Central Guizhou paleo-uplift, as well as the Xuefeng paleo-uplift, with limited supply from the Central Sichuan paleo-uplift. The western part of the Eastern Sichuan Basin is closer to the paleo-uplift in Central Sichuan, and the northern edge is closer to the Qinling Ocean, which is connected to external water bodies. The limitations of its water bodies are weak, and its provenance may mainly come from the paleo-uplift in Central Sichuan Basin.
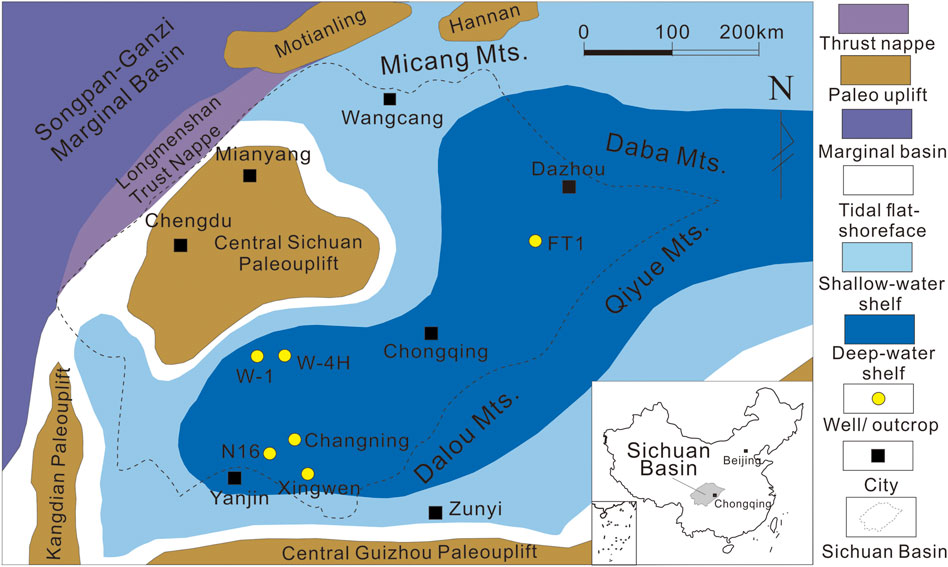
Figure 1. Geological background and location of studied wells during the sedimentary period of the Wufeng Formation-Longmaxi Formation in the Sichuan Basin and its surrounding areas.
The black shale of the Ordovician Wufeng Formation-Silurian Longmaxi Formation is widely developed in southern China, and belongs to the Katian Stage-Aeronian Stage in terms of geological age (Brenchley et al., 2003). Except for the top 1–2 m of the Ordovician Wufeng Formation, which is composed of shell limestone or carbonate-bearing mudstone rich in Hirnantian fauna fossils, all other intervals are shale rich in graptolite fossils.
The shale intervals studied in this article are Katian Stage, Hirnantian Stage, and Rhuddanian Stage, which correspond to the WF1-WF3, WF4-LM1, and LM2-LM5 of the graptolite biozone, respectively (Chen X. et al., 2017; Huang et al., 2023). By combining lithology, logging response, and graptolite biozone, and referring to previous classification schemes (Figure 2), the shale interval can be divided into two third-order sequences (Sq 1 and Sq 2) and four individual tracts (TST1, HST1, TST2, and HST2). The Wufeng Formation and GYQ Member form the first third-order sequence (Sq 1), with black siliceous shale and argillaceous-rich siliceous shale (TST1) from the Wufeng Formation at the bottom and argillaceous limestone (HST1) from the GYQ Member above. The lower part of the Longmaxi Formation is the second third-order sequence (Sq 2), with the bottom boundary in contact with the overlying siliceous shale through transgression. The black siliceous shale in the lower part of the Longmaxi Formation is divided into TST2, and the second maximum flood surface (MFS) has been identified, representing the transition from deep water to shallow water and the beginning of HST2.
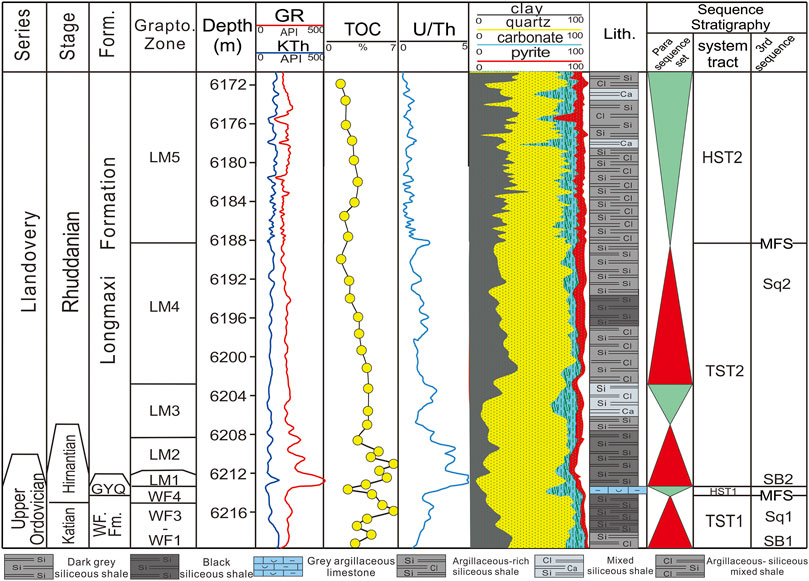
Figure 2. Sequence division of shale interval in Well FT-16. TST, Transgressive system tract; HST, Highstand system tract; SB, Sequence boundary, MFS, Maximum flooding surface).
Samples and methods
Sample collection and measurement
34 shale samples from the Well FT-1 in the Eastern Sichuan Basin. When selecting samples, non matrix parts such as calcite veins were avoided. All samples were measured for TOC value, mineral composition, and bulk element content. Previous studies have obtained detailed elemental geochemical data for marine shale intervals in the southern Sichuan region (Huang et al., 2023), laying the foundation for comparing paleoclimate, terrestrial debris input, paleoproductivity, and bottom water redox conditions with the Eastern Sichuan Basin. In addition, to clarify the provenance and tectonic background of the Southern Sichuan Basin, this study cited 59 sets of data obtained from the Changning and Xingwen Outcrop of the Southern Sichuan Basin (Li, 2017). The XRD experiment was completed at the China Petroleum Exploration and Development Research Institute and measured using a Japanese Nikkei X-ray diffractometer. The determination of element content was completed within Kehui Testing (Tianjin) Technology Co., Ltd. Trace and rare earth elements were determined using ICP-MS (Jena PQ MS) high-resolution plasma spectrometer, and the main elements were determined using XRF-1800 wavelength dispersive X-ray fluorescence spectrometer. The TOC test was conducted using the CS744-MHPC carbon and sulfur analyzer at the unconventional experimental center of CNOOC Energy Development.
Indicator calculation and calibration
The enrichment factor (XEF) can eliminate the influence of terrestrial debris and distinguish the degree of element enrichment in sediments. The calculation formula is: XEF=(X/Al)sample/(X/Al)standard.
X and Al represent the element content and Al content in the sediments, respectively, and “standard” refers to the standardized background values. PAAS (Taylor and McLennan, 1985) and UCC (McLennan, 2001) are frequently used for normalization in previous studies, and we select the PAAS in this study. Al is not easily affected by weathering or post-depositional alteration, so the ratio of element content to Al is used to remove the influence of terrestrial debris.
The calculation method for trace element content in sediments of non detrital origin (biogenic or authigene enrichment) is as follows: Eorg/Ebio = Esample-Alsample×(E/Al)detr
Ebio represents the organic or biological portion of an element that exceeds a specific terrestrial input standard. The portion exceeding the average shale is calculated by subtracting the estimated fragment source from the total element content in the sample. Esample and Alsample represent the abundance of a certain element and Al element in the shale sample, respectively, and (E/Al) detr is the ratio of the average abundance of E and Al under specific standards (McLennan, 2001). In practical research, PAAS is often used to estimate terrestrial inputs, with commonly used (Si/Al) detrs and (Ba/Al) detrs of 3.11 and 0.0075, respectively (Taylor, 1964; Wedepohl, 1971; Dymond et al., 1992). The Chemical Weathering Index (CIA) is commonly used to determine the degree of paleoweathering. In order to exclude the influence of oxides from non terrestrial debris sources, it is necessary to calibrate the CaO content to obtain the CaO content (CaO*) in silicates.
In the formula, if CaO(adjust)>Na2O, then CaO* = Na2O; If CaO(adjust)<Na2O, then CaO* = CaO(adjust). The above units are all in mol
Results
Shale lithofacies and mineral compositions
Using the ternary diagram of mineral composition, shale lithofacies types were classified (Figure 3A). The results indicate that in the Southern Sichuan Basin, the main types are argillaceous-rich siliceous shale, mixed siliceous shale, and siliceous-argillaceous mixed shale. The Eastern Sichuan Basin is mainly composed of siliceous shale, argillaceous-rich siliceous shale, and mixed siliceous shale. The mineral components of different system tracts in the Eastern and Southern Sichuan Basin are shown in Table 1. The carbonate mineral content of marine shale in Southern Sichuan is generally higher than that in Eastern Sichuan, while the feldspar content is generally lower than that in Eastern Sichuan. The content of clay minerals and pyrite in marine shale in the Southern Sichuan Basin is higher in TST1, and lower in other periods than in the Eastern Sichuan Basin. However, the content of quartz is only higher in HST2 period, and lower in other periods than in the Eastern Sichuan Basin.
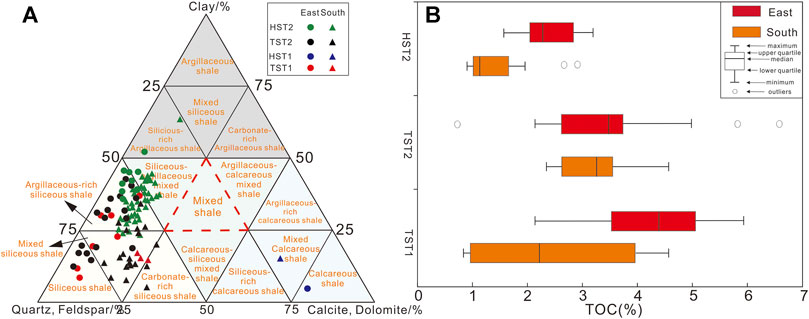
Figure 3. Mineral composition in different system tracts (A) and box-plots of TOC range (B) in Eastern and Southern Sichuan Basin. Total organic carbon (TOC) content range.
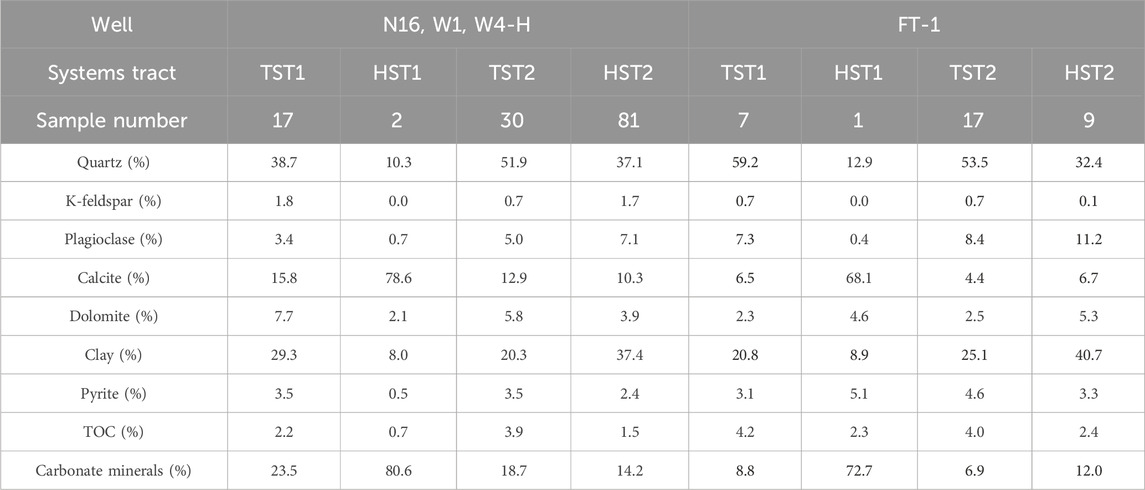
Table 1. Mineral composition characteristics and TOC content of shales in different area and system tracts (Well N16, W1, W4-H in southern Sichuan from Huang et al., 2023).
From the characteristics of changes in different system tracts, from TST1 to HST2, the clay content in the Eastern Sichuan Basin continued to increase while the quartz content continued to decrease. However, the quartz content in the Southern Sichuan Basin shows a trend of first increasing and then decreasing, while the change in clay content is opposite. In addition, the TST1 and TST2 sedimentary samples in the Eastern Sichuan are more dispersed, indicating that the mineral composition during this period is complex and varied. It is possible that the study area is close to the provenance region, and the water is relatively shallow, making it more susceptible to the influence of sea-level changes and terrestrial inputs.
The TOC content of marine shale in the eastern Sichuan region is generally higher than that in the southern Sichuan region (Figure 3B), with the highest average TOC value during the TST1 period, reaching 4.2%. The average TOC value of marine shale in the southern Sichuan region reached its maximum during the TST2 period, at 3.9%. From TST1 to HST2 period, the average TOC in Southern Sichuan showed a trend of first increasing and then decreasing, while the average TOC in Eastern Sichuan continued to decrease. The maximum variation in TOC during the TST1 period in Eastern and Southern Sichuan may be related to rapid changes in the paleoenvironment or frequent geological events during this period.
Paleo-environmental conditions and differences
Paleo-redox condition
The redox level is a key factor in the preservation of organic matter. Redox-sensitive elements Mo, U, V, Cr, Ni, and Co are commonly used for the reconstruction of bottom water redox environments (Tribovillard et al., 2006). These elements are prone to precipitate in sediment under reducing conditions, while they are prone to dissolve in water under oxidizing conditions (Tribovillard et al., 2012). In order to weaken the dilution effect of terrestrial debris, the ratio of element content to Al content was used as an indicator in this study. Figure 4 shows that the reducibility of bottom water in both Eastern and Southern Sichuan increases rapidly during the TST1 period, and reaches its maximum in the early TST2 period before slowly decreasing.
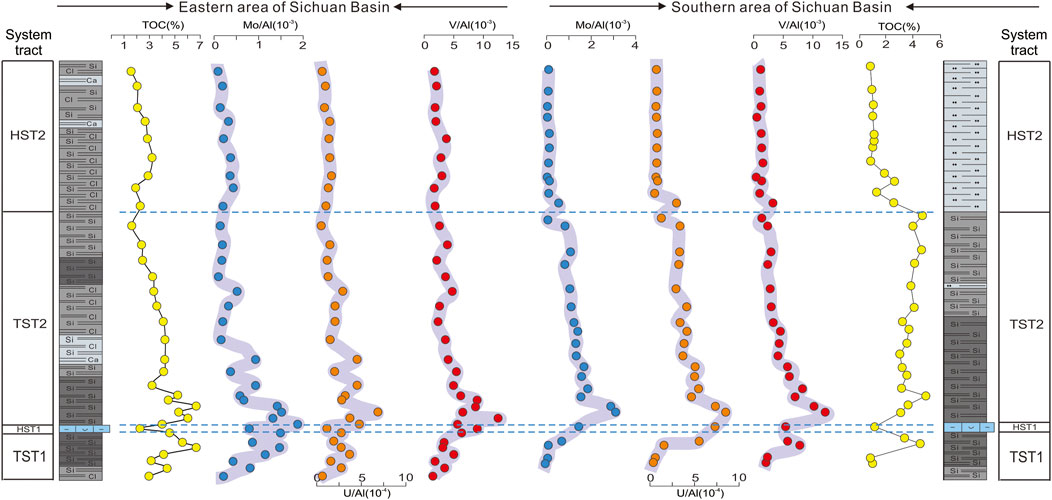
Figure 4. Comparison of common paleo-redox indicators in different system tracts in Eastern and Southern Sichuan Basin.
In order to quantify redox levels in different system tracts and areas, the cross-plots of Th/U-Ni/Co, V/Cr-V/(V + Ni), and MoEF-UEF were applied (Figures 5A–C). In the Southern Sichuan Basin, the data points of TST1 reflect a gradual transition from suboxic to anoxic, sample distribution area and data points exhibit stronger reduction of anoxic bottom water in the early TST2 with some intervals even reaching the euxinic condition, while gradually decrease to weak anoxic or suboxic conditions during the late TST2 and HST2 period. The TST1 period in the Eastern Sichuan Basin is mainly in a anoxic environment, and the bottom water reduction in the TST2 period is also reach its maximum, manifested by some data points being close to the euxinic zone. In addition, a small number of data points in the late TST2 period near the suboxic zone may be related to a decrease in the degree of late reduction. The reducing conditions in HST2 continued slowly decreasing after a slight increase, and it remained mainly in a weak anoxic environment in the early period.
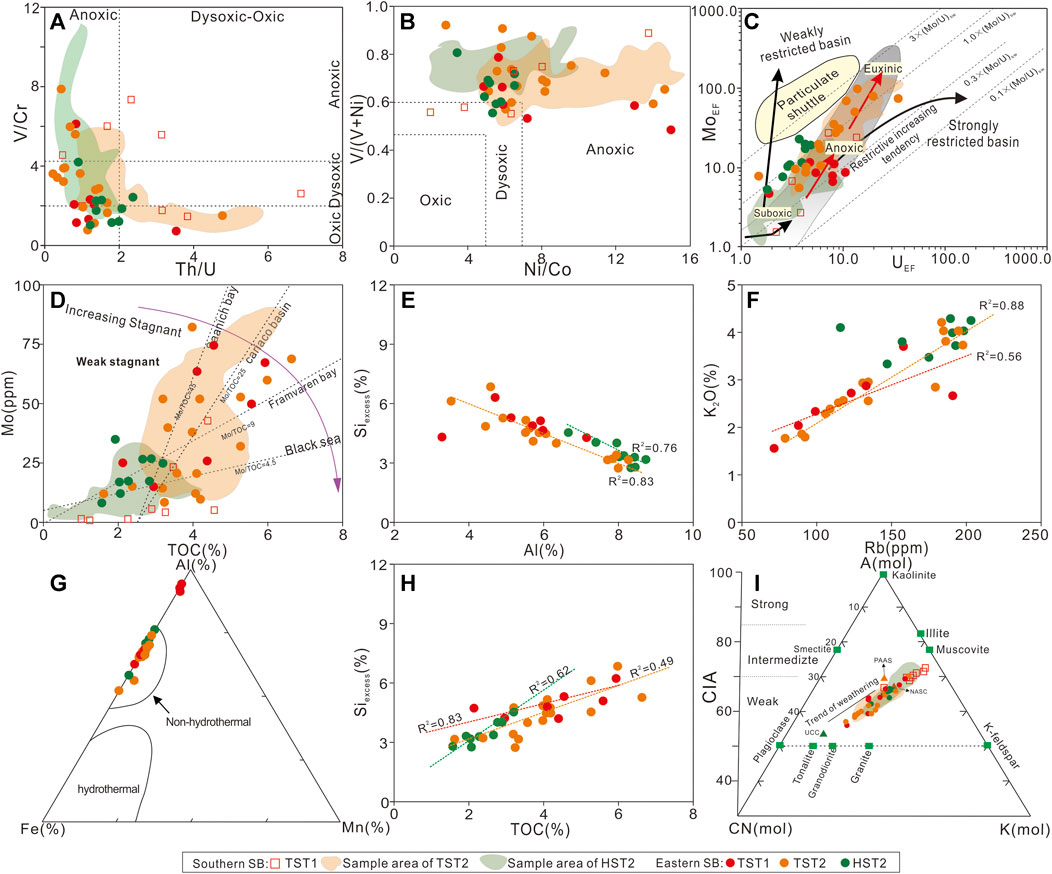
Figure 5. Correlation diagrams of geochemical elements. (A–D) Paleo-redox conditions discrimination diagrams. (E–H) Silicon source discrimination diagrams. (I))A-CN-K diagrams, reflecting weathering intensity and provenance.
Mo/TOC can be used to determine the degree of limitation of water bodies (Algeo and Lyons, 2006; Algeo and Tribovillard, 2009). The data points of TST1 in the Southern Sichuan Basin are relatively scattered (Figure 5D), suggesting an unstable degree of water limitation. Considering the continuous uplift of the surrounding paleo-uplift and the anoxic bottom water environment, the Mo/TOC ratio is mostly less than 4.5, suggesting that the water is strongly restricted in the middle and later periods of TST1. The Mo/TOC enclosed area in the TST2 period is widely distributed, but mainly in a moderately enclosed environment. Its changing characteristics also indicate that as the degree of transgression increases, the degree of restriction gradually strengthens. During the HST2 period, the reducibility of water decreased, but the degree of restriction was significantly reduced in the early suboxic-anoxic environment. The TST1 data points in the Eastern Sichuan are relatively scattered, with Mo/TOC values mostly greater than 4.5, indicating moderate restriction. The limitations of TST2 period have changed significantly, with some data points having Mo/TOC ratios less than 4.5, indicating a significant increase in restriction during certain intervals, but still in a moderately restricted environment. The degree of restriction in HST2 period decreases with the decrease of sea-level.
Paleo-productivity condition
The content of Ba, Cu, Ni, and Zn is closely related to the life activities of marine organisms, Babio and (Cu + Ni + Zn)/Al can serve as reliable indicators for evaluating productivity. The Babio in Figure 6 reflects that the paleo-productivity of the Eastern Sichuan showed an overall increasing trend during the TST1-HST2 period, while showed an increasing trend during the TST1-TST2 period and a decreasing trend in HST2 in Southern Sichuan. The (Cu + Ni + Zn)/Al indicates that the paleo-productivity of these two regions all showed a unimodal distribution, increasing during the TST1 period and reaching its peak in the early TST2 period before slowly decreasing.
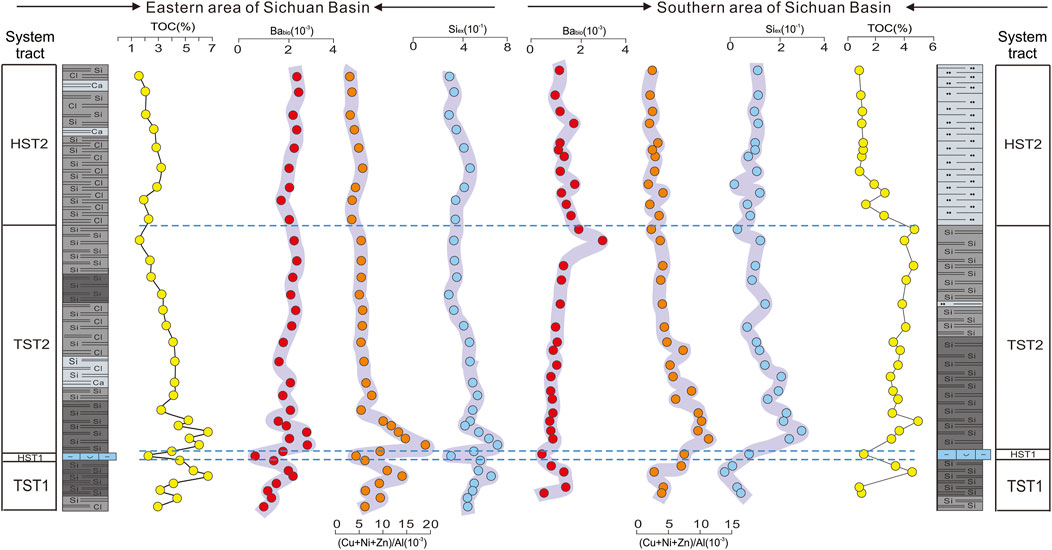
Figure 6. Comparison of common paleo-productivity indicators in different system tracts in Eastern and Southern Sichuan Basin.
The Siexcess in the black shale of the Wufeng-Longmaxi Formation is related to biogenic origin, so this study also used Siexcess to evaluate paleo-productivity (Cai et al., 2022). There is a negative correlation between Siexcess and Al (Figure 5E), ruling out the possibility of terrestrial input and clay mineral transformation to form Siexcess. The positive correlation between K2O-Rb (Figure 5F) indicates that the source of Siexcess is not related to magmatic activity or provenances (Floyd and Leveridge, 1987; Huang et al., 2023). The Al-Fe-Mn data (Figure 5G) points all fall into the non hydrothermal zone, indicating that the formation of Siexcess is independent of hydrothermal activity (Xie et al., 2021). The positive correlation between Siexcess and TOC (Figure 5H) confirms its correlation with biological sources. The Siexcess in the Eastern Sichuan generally maintains a trend of first increasing and then decreasing from TST1 to HST2, with the highest paleo-productivity values distributed in the early period of TST2. The peak of the total amount of Siexcess in Southern Sichuan also appeared in the early period of TST2 and gradually decreased thereafter.
Due to the fact that Babio is not suitable for oxygen-deficient environment, while the mass fraction of Cu, Zn and Ni is closely related to the sedimentation amount of organic matter, and suitable for reducing environment. As a result, there is no consistency in these indicators, and the reasons for this can be fully explained. As for Babio, sulfate reduction reactions are common on the surface and/or bottom of sediments in anoxic and euxinic environments during TST1 and HST2, barium sulfate is a latent source of sulfate, and partial dissolve when sulfate supply is insufficient, resulting in a lower content of barium and estimated productivity. On account of the eastern and southern Sichuan are mainly in a relatively reducing environment, (Cu + Ni + Zn)/Al is basically consistent with the change trend of Siexcess, and that also verified the accuracy of the our results. In conclusion, the paleo-productivity during the depositional process of TST1 and TST2 in Eastern and Southern Sichuan is stronger than that of HST2. In addition, the Siexcess and (Cu + Ni + Zn)/Al in Eastern Sichuan is generally higher than that in Southern Sichuan, reflecting the strong paleo-productivity of the former.
Terrestrial input condition
Al and Zr elements are not easily migrated during transportation and can be used as effective indicators to characterize the intensity of terrestrial inputs. Al is mainly came from fine-grained aluminosilicate clay minerals, while Zr existed in clay minerals and coarse-grained minerals (quartz, zircon, etc.). Therefore, Zr/Al ratio is considered an effective indicator for quantifying the content of coarse-grained debris in terrestrial inputs. Figure 7 indicates that the changes of Zr and Al curves in the Eastern and Southern Sichuan is basically consistent, showing a gradually decreasing trend during the TST1 and HST1 periods, as well as a fluctuating trend during the TST2 period and a gradually increasing trend during the HST2 period. The variation of Al contents suggest that the total terrestrial input amount of fine-grained clay minerals are similar in this two areas. However, the overall Zr content in the Eastern Sichuan Basin is lower than that in the Southern Sichuan Basin, especially during the TST1 period with rapid drop. This indicates that the difference in terrestrial input during the TST1-HST2 sedimentary period between the Eastern and the Southern Sichuan is relatively small, but the input of coarse-grained debris is lower during the TST1-HST2 period in the eastern Sichuan.
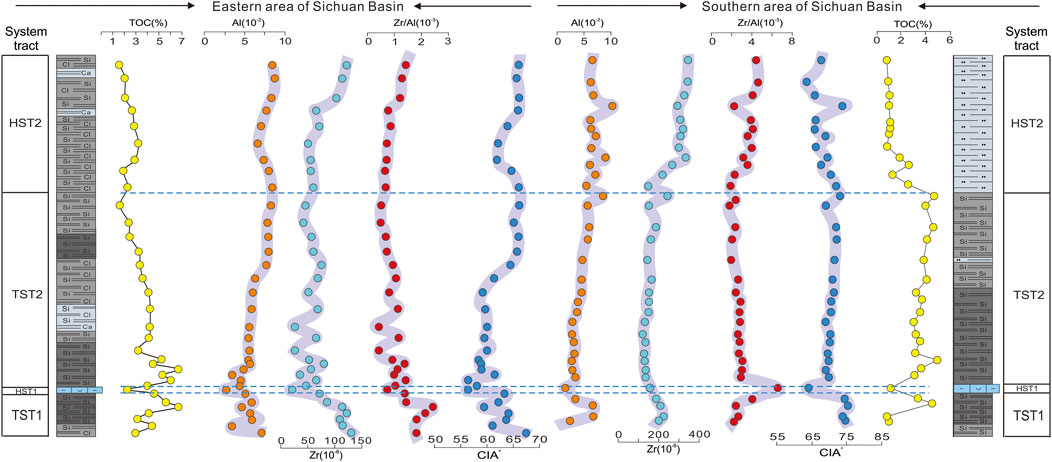
Figure 7. Comparison of common terrigenous clastic input and paleo weathering indicators in different system tracts in Eastern and Southern Sichuan Basin.
Zr/Al in the Eastern Sichuan showed a slow decreasing trend during TST1-TST2, suggesting a significant slowdown in the input rate of coarse-grained debris, while a slow increase during HST2 indicates a faster input of coarse-grained debris. However, the input of coarse-grained debris in the Southern Sichuan Basin remained relatively stable during the TST1-TST2 period, while it also increased during the HST2 period. In addition, the Zr/Al values in the Southern Sichuan were higher than those in the Eastern Sichuan at all periods. This indicates that the input rate of coarse-grained debris in the sediment composition of the Eastern Sichuan Basin is generally lower than that of the Southern Sichuan, especially during the HST2 deposition period. During this period, the development of more mixed shale in the Southern Sichuan can be confirmed. In the late period of TST2, the Al content gradually increased, but the Zr/Al and Zr contents showed a slow decreasing trend in fluctuation. It is speculated that the rapid increase of fine-grained clay minerals diluted coarse-grained minerals, resulting in an opposite trend of Al, Zr, and Zr/Al.
Paleoclimate and weathering intensity
The Chemical Index of Alteration (CIA) is often used to indicate the climate and physical and chemical weathering intensity of provenance region. CIA values of 50–65, 65–80, and 80–100 indicate weak, moderate, and strong weathering degrees, respectively, and correspond to cold-dry, warm-humid, and hot-humid conditions, respectively. In the actual application process, in order to exclude the influence of CaO content that is bound to non-silicates, the corrected CIA (CIA *) was calculated in this study (Figure 5I; Figure 7). The lowest values of CIA * in both the Eastern and Southern Sichuan appear at HST1, indicating a global temperature drop during the Hirantanian glaciation. The CIA * value in the Southern Sichuan ranges from 60 to 75. During the TST1-HST2 period, the CIA * gradually decreases and undergoes an evolutionary process from a hot warm climate to a cold dry climate. The cooling event caused by the Hirnantian glaciation is still accompanied by the early deposition of TST2. The CIA * value in the Eastern Sichuan ranges from 55 to 70 during the TST1 to early TST2 period and a CIA * value below 65, indicating a cold-dry environment. In the late period of TST2, the CIA * was greater than 65, and the climate gradually became mild. However, in the early period of HST2, it experienced a short-term cold-dry climate, and in the late period, it eventually transformed into a warm-humid climate. Compared with the input indicators of terrestrial debris, it is found that the low degree of chemical weathering in the cold climate during the TST tract is an important reason for the low input of terrestrial debris.
Provenance condition and tectonic setting
The differences in provenances not only affect the mineral compositions, but also control the types of terrestrial nutrient inputs. The Al2O3/TiO2 ratio of fine-grained sediments can effectively identify the type of source rocks. Due to the low solubility of Al and Ti oxides at low temperatures, their proportion in sedimentary rocks is very close to that of the source rock. The distribution of Al2O3/TiO2 ratio in<8, 8–21, and>21 indicates basic, neutral, and acidic igneous rocks, respectively. The provenance in the southern Sichuan region is mainly a mixture of neutral and acidic igneous rocks (Figure 8A). The provenance in the Eastern Sichuan is a mixture of neutral and acidic igneous rocks in TST1, while in TST2 and HST2 are neutral igneous rocks. The weathering trend of the A-CN-K diagram (Figure 5I) further confirm that the provenance in Southern Sichuan during TST1-TST2 tends to be neutral igneous rocks, and HST2 tends to be acidic igneous rocks. While in the Eastern Sichuan during the TST1-HST2 tends to have neutral granodiorite sources.
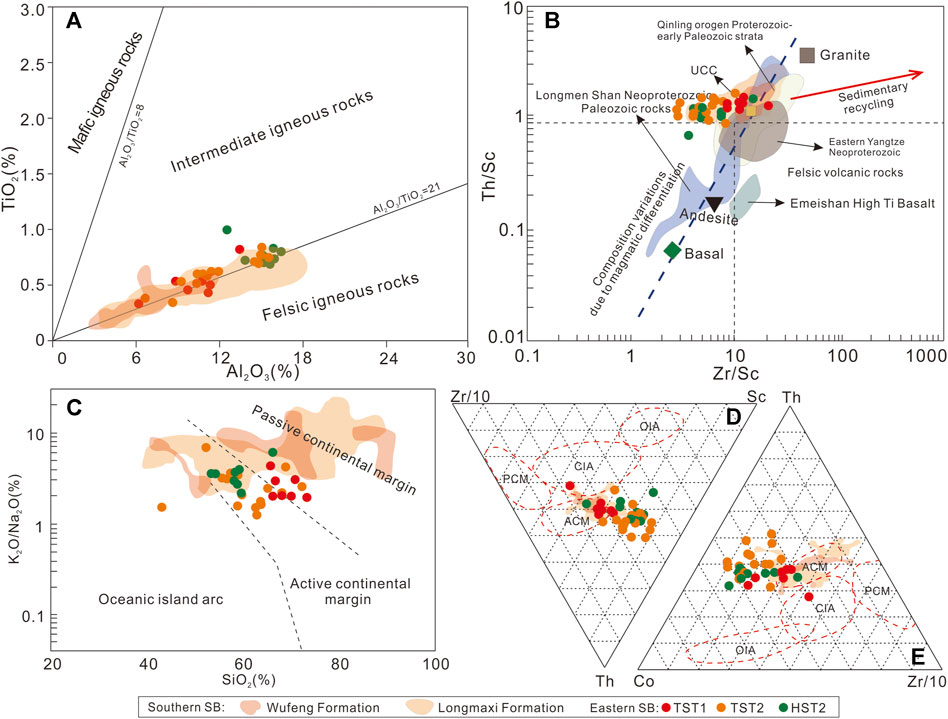
Figure 8. Correlation diagrams of geochemical elements. (A, B) Provenance discrimination diagrams; (C–E) Tectonic setting discrimination diagrams. According to Roser and Korsch (1986); OIA. Oceanic Island Arc; CIA. Continental island arc; ACM. Active continental margin; PM. Passive continental margin.
Due to the higher content of compatible element Sc and lower content of incompatible elements Zr and Th in basic rocks compared to acidic rocks, and the relatively constant abundance of Sc, Zr, and Th during weathering, the Zr/Sc-Th/Sc ratio can be used for source rock type analysis. Figure 8B shows that the distribution plots in the Southern Sichuan is more towards the upper right than that in the Eastern Sichuan, also suggesting that there are more acidic igneous components in provenance. In addition, in the provenance composition of the Eastern Sichuan, TST1 period is more acidic and HST2 period is more neutral.
Roser and Korsch (1986) proposed that the K2O/Na2O-SiO2 cross-plot can be effectively used to distinguish the tectonic environment of fine-grained sedimentary rocks. The results indicate that the Eastern Sichuan is in an active continental margin environment during the early Wufeng period. The data points of the Longmaxi period in the Southern Sichuan are close to the active continental margin, but the data points of the Wufeng period are closer to the passive continental margin environment (Figure 8C). Stable rare earth and trace element combinations (such as La, Th, Sc, Zr, etc.) can also be used to analyze and determine tectonic backgrounds. The Th-Sc-Zr/10 and Th-Co-Zr/10 diagrams established by Bhatia and Crook (1986) were applied for background analysis (Figures 8D, E). The analysis reveals that the majority of data points fall within or proximate to the active continental margin zone, while a minimal proportion is dispersed in the continental island arc zone. Compared to Eastern Sichuan, the Southern Sichuan exhibits a stronger correlation to the active continental margin region. The sedimentary tectonic context in both regions remains steady from Late Ordovician to Early Silurian, characterized predominantly by an active continental margin setting.
Impact of paleo-environmental condition on OM accumulation and mineral composition
The formation of black shale from the Ordovician Wufeng Formation to the Silurian Longmaxi Formation in the Sichuan Basin is the result of geological events and various paleo-environmental conditions. Under this influence, there are significant differences in TOC and mineral composition between different system tracts in the Eastern and Southern Sichuan (Figure 9).
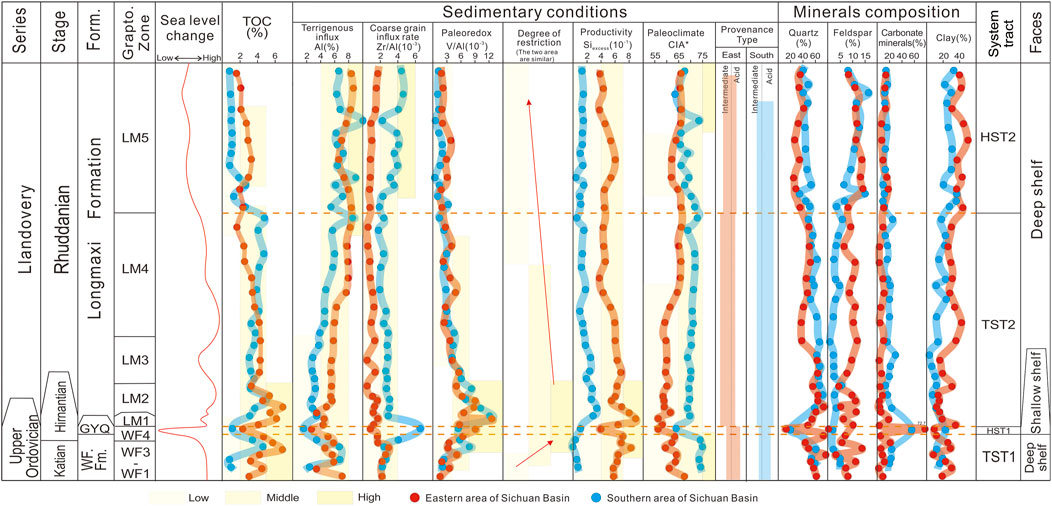
Figure 9. The Paleoenvironment, TOC and minerals composition evolution process in the Southern and Eastern area of Sichuan basin during the Late Ordovician-Early Silurian.
TST 1 period
Under the influence of the Caledonian Movement, the Xuefeng paleo-uplift and the Central Sichuan underwater uplift rapidly uplifted. In the Kaitian stage, relative sea-level rise led to a transformation of the sedimentary environment from an early open platform to a restricted basin, initiating the deposition of black shale in the Wufeng Formation.
In the early TST 1 period, intense tectonic uplift enhanced the intensity of detrital input. The separation effect of paleo-uplift and relative sea-level rise leads to an increasing reduction and restriction of bottom water, but also accompanied by intermittent relative oxic conditions. Active tectonic activity also promotes volcanic eruptions, and the volcanic ash formed by it is transported and deposited in the ocean, providing rich nutrients for surface seawater. Under this setting, plankton such as graptolites have proliferated on a large scale, and the productivity level of the water surface is constantly improving. These plankton can be preserved as organic matter under anoxic conditions after death. In the late TST 1 period, tectonic activity tended to stabilize, but the early large-scale volcanic activity had led to a gradual cooling of the global climate and changes in graptolite biodiversity, triggering the Late Ordovician extinction. In cold climates, the weathering intensity is weak, and the amount of terrestrial input is significantly reduced. A large number of aquatic organisms die, providing organic matter supply and enhancing the reducibility of bottom water through degradation of organic matter. During this period, organic-rich siliceous shale is mainly formed.
During TST 1 period, the trend of TOC variation in the Eastern Sichuan and Southern Sichuan is consistent, but the average value of the former is significantly higher than that of the latter. It is speculated that this phenomenon is related to the high paleo-productivity in the Eastern Sichuan. The high excess Si and a large amount of siliceous organisms confirm the enrichment of organic matter, manifested by a higher quartz content in Eastern Sichuan than in Southern Sichuan (Table 1). The detrital input in the Eastern and Southern Sichuan is basically the same, and both provenances tend to be acidic magmatic rocks. The high content of feldspar in the Eastern Sichuan is related to the relatively cold-arid climate background, where feldspar is weakly affected by weathering during transportation. Due to being closer to the Central Guizhou-Xuefeng paleo-uplift, there is more input of coarse-grained carbonate debris in the Southern Sichuan Basin, which is characterized by high carbonate mineral content, especially in the late TST 1 period. This has also been confirmed by the Zr/Al ratio.
HST 1 period
In the mid to late Hirnantian stage, the formation of glaciers on the Gondwana continent led to a significant decrease in global sea-level (Bertrand et al., 1996), resulting in sharp shallowness of water mass and unfavorable organic matter preservation under the oxic bottom water condition. A thin layer of carbonate-rich argillaceous limestone was deposited in a shallow water environment.
TST 2 period
The Hirnantian glaciation has ended, and global temperatures are rapidly rising. Glacial melting has caused widespread transgression. Volcanic activity and tectonic movements tend to stabilize. In the early TST 2 period, the separation of paleo-uplift and sea-level rise kept the bottom water in a restricted and anoxic condition. Climate warming has led to an increase in weathering intensity and enhanced detrital influx. The glacial melting results in a large amount of cold fresh water flowing into the ocean, which not only brings nutrients but also causes stratification of fresh and saline water, exacerbating anoxic conditions. Planktonic organisms, including radiolarians and graptolites, have once again flourished on a large scale, increasing productivity levels. The continuously sinking biological remains continue to consume dissolved oxygen in the bottom water, maintaining anoxic environments, even euxinic environments. During early TST 2 period, less detrital influx helps to weaken the dilution effect and form organic-rich siliceous shale. During middle TST 2 period, the sea-level continues to rise, increasing the circulation between the restricted basin and the ocean, and reducing the restriction degree of bottom water. However, under moderate to strong paleo-productivity, the consumption of oxygen by the remains of organisms still ensures that the bottom water is in an suboxic-anoxic environment. In the late TST 2 period, the fluctuating sea-level drop results in limited surface water productivity. The increase in dissolved oxygen in bottom water is not conducive to organic matter preservation. The humid climate leads to an increase in the intensity of paleo-weathering, while the increase in terrestrial input disrupts the enrichment of organic matter. Compared to the middle TST 2 period, the organic carbon content is significantly reduced, and the shale lithology is argillaceous-rich siliceous shale or mixed siliceous shale.
During early-middle TST 2 period, the high productivity level in the Eastern Sichuan leads to a higher average TOC content than in the Southern Sichuan. In the late TST 2 period, the paleo-productivity in the Eastern Sichuan is still at a high level, but the rapidly increasing weathering intensity has led to a significantly higher detrital influx than in the Southern Sichuan. The dilution effect of terrestrial debris reduces the accumulation of organic matter in the Eastern Sichuan. The mineral composition indicates that the quartz content in the Eastern Sichuan was higher in the early TST 2 period (Figure 9), which is related to the deposition of a large amount of biogenic quartz, while the late TST 2 period was lower than that in the Southern Sichuan (Table 1). The clay content in the Eastern Sichuan is higher than that in the Southern Sichuan, while the carbonate content is lower than that in the Southern Sichuan. This is related to the large amount of terrestrial debris input and the high proportion of clay minerals. From the perspective of provenance differences, the Eastern Sichuan Basin is mainly composed of neutral igneous rocks, while the Southern Sichuan Basin is mainly composed of acidic igneous rocks. The former has a higher proportion of plagioclase feldspar in sediment supply, while the latter has a higher proportion of potassium feldspar. However, potassium feldspar is prone to kaolinization during transportation, so the mineral composition in eastern Sichuan has a significantly higher content of feldspar, mainly plagioclase feldspar.
HST 2 period
The climate in the late Rhuddanian stage continued to warm, with a slow decrease in sea-level, but there was short-term transgression. The tectonic uplift intensifies the weathering process in the provenance region, and the amount of terrestrial input continues to increase. The productivity level has undergone significant changes (significantly higher in Eastern Sichuan than in Southern Sichuan), but the bottom water conditions are not conducive to the preservation of organic matter. Diluted by terrestrial debris, organic matter is dispersed in sediments. More and more terrestrial quartz and clay minerals have replaced biogenic quartz as the main component of minerals, and the shale lithofacies are gradually transitioning to argillaceous-siliceous mixed shale.
The low productivity, low preservation conditions, and high terrestrial input in the Southern Sichuan Basin are reflected in a rapid decrease in TOC. The TOC in the Eastern Sichuan increased in the early stages and gradually decreased in the later stages, which is speculated to be related to higher paleoproductivity and a high proportion of clay in terrestrial debris. The high productivity in the Eastern Sichuan not only brings organic matter input, but also consumes the oxygen content of the bottom water during deposition, which to some extent increases the reducibility of the bottom water. In addition, high clay content is conducive to the adsorption and rapid settling of organic matter, reducing consumption during the sinking process, and facilitating the aggregation and preservation of organic matter.
The difference in the detrital influx between the Eastern and Southern Sichuan is small, but the quartz mineral content in Southern Sichuan is higher than that in Eastern Sichuan (Table 1), while the clay content is lower than that in Eastern Sichuan. This is related to the slightly stronger weathering intensity and coarse-grained debris input (silt-size quartz) in Southern Sichuan. The provenance sources in the Eastern Sichuan tend to be neutral magmatic rocks, while those in the Southern Sichuan tend to be acidic magmatic rocks. Moreover, the climate in the Eastern Sichuan Basin is cold-dry, with low weathering intensity. Therefore, the mineral composition of plagioclase in the Eastern Sichuan Basin is relatively high, while the content of quartz and potassium feldspar in the Southern Sichuan is higher (Table 1). The content of carbonate rocks in Southern Sichuan is higher than that in Eastern Sichuan, especially in calcite, which is related to the high input of coarse-grained carbonate debris. The average value of pyrite in the Eastern Sichuan is 37.5% higher than that in the Southern Sichuan, which indirectly confirms that the oxygen content in the bottom water of the Eastern Sichuan is lower, which is conducive to the formation of pyrite.
Conclusion
1) The lower part of the Longmaxi Formation-Wufeng Formation in the Southern and Eastern Sichuan Basin can be divided into two third-order sequences (Sq 1 and Sq 2). Sq 1 is composed of the Wufeng Formation and GYQ Member, while Sq 2 is composed of the lower part of the Longmaxi Formation. Each third-order sequence consists of a set of transgressive system tracts (TSTs) and a set of high-level system tracts (HSTs).
2) The TOC content in Eastern Sichuan is higher than that in Southern Sichuan. During the TST1 period, the average TOC content in Eastern Sichuan was the highest, reaching 4.2%. The average TOC content in Southern Sichuan reached its maximum at 3.9% during the TST2 period. From TST1 to HST2, the clay content in eastern Sichuan continued to increase while the quartz content continued to decrease. However, the quartz content in southern Sichuan shows a trend of first increasing and then decreasing, while the change in clay content is opposite.
3) During the TST1 period, the TOC range in Eastern Sichuan is significantly higher than that in Southern Sichuan, which is related to its high paleoproductivity. In the early to middle TST2 period, the high paleoproductivity in Eastern Sichuan resulted in a higher TOC content than in Southern Sichuan. In the late TST2 period, the accumulation of organic matter in Eastern Sichuan was lower than that in Southern Sichuan due to the dilution effect of terrestrial debris. During the HST2 period, the TOC in Southern Sichuan showed a rapid decrease trend. The TOC in Eastern Sichuan slightly increased in the early HST2 period, which is related to higher paleoproductivity and the adsorption and preservation of clay.
4) During the TST1 period, the quartz content in Eastern Sichuan was higher than that in Southern Sichuan, which is related to the abundant accumulation of biogenic silica. During the TST2 period, due to the high input of terrestrial debris and the high proportion of clay minerals, the quartz content in Eastern Sichuan was higher than that in Southern Sichuan in the early period, but the opposite was true in the late period. The mineral content of clay in Eastern Sichuan has always been higher than that in Southern Sichuan. During the HST2 period, compared with Eastern Sichuan, the stronger weathering intensity and coarse-grained debris input (mainly composed of silt-size quartz) in Southern Sichuan resulted in higher quartz mineral content and lower clay mineral content.
Data availability statement
The raw data supporting the conclusion of this article will be made available by the authors, without undue reservation.
Author contributions
XY: Conceptualization, Methodology, Writing–original draft. HY: Formal Analysis, Investigation, Supervision, Writing–review and editing. LY: Conceptualization, Investigation, Project administration, Writing–review and editing. LX: Conceptualization, Investigation, Project administration, Writing–review and editing. JC: Investigation, Methodology, Writing–original draft. DL: Conceptualization, Investigation, Methodology, Writing–original draft. CJ: Methodology, Project administration, Supervision, Writing–review and editing. ZJ: Investigation, Project administration, Software, Validation, Writing–review and editing.
Funding
The author(s) declare that financial support was received for the research, authorship, and/or publication of this article. The research was supported by the National Natural Science Foundation of China (Grant No. 42272171) and Science and Technology Cooperation Program of CNPC−SWPU Innovation Alliance (Grant No. 2020CX020104).
Conflict of interest
Authors XY, HY, LY, LX, JC, and DL were employed by PetroChina Southwest Oil and Gas Field Company. Author CJ was employed by PetroChina Southwest Oil and Gas Field Company. Author ZJ was employed by Sichuan Geotech Science and Technology Ltd. Company.
Publisher’s note
All claims expressed in this article are solely those of the authors and do not necessarily represent those of their affiliated organizations, or those of the publisher, the editors and the reviewers. Any product that may be evaluated in this article, or claim that may be made by its manufacturer, is not guaranteed or endorsed by the publisher.
References
Algeo, T. J., and Lyons, T. W. (2006). Mo-total organic carbon covariation in modern anoxic marine environments: implications for analysis of paleoredox and paleohydrographic conditions. Paleoceanography 21, 16–23. doi:10.1029/2004PA001112
Algeo, T. J., and Tribovillard, N. (2009). Environmental analysis of paleoceanographic systems based on molybdenum-uranium covariation. Chem. Geol. 268, 211–225. doi:10.1016/j.chemgeo.2009.09.001
Armstrong, H. A., Abbott, G. D., Turner, B. R., Makhlouf, I. M., Muhammad, A. B., Pedentchouk, N., et al. (2009). Black shale deposition in an Upper Ordovician–Silurian permanently stratified, peri-glacial basin, southern Jordan. Palaeogeogr. Palaeoclimatol. Palaeoecol. 273, 368–377. doi:10.1016/j.palaeo.2008.05.005
Berry, W. B. N. (2010). Black shales: an Ordovician perspective. Geol. Soc. Am. Special Pap. 466, 141–147. doi:10.1130/2010.2466(09)
Bertrand, P., Shimmield, G., Martinez, P., Grousset, F., Jorissen, F., Paterne, M., et al. (1996). The glacial ocean productivity hypothesis: the importance of regional temporal and spatial studies. Mar. Geol. 130, 1–9. doi:10.1016/0025-3227(95)00166-2
Bhatia, M. R., and Crook, K. A. W. (1986). Trace element characteristics of graywackes and tectonic setting discrimination of sedimentary basins. Contrib. Mineral. Petrol. 92 (2), 181–193. doi:10.1007/BF00375292
Brenchley, P. J., Carden, G. A., Hints, L., Kaljo, D., Marshall, J. D., Martma, T., et al. (2003). High-resolution stable isotope stratigraphy of Upper Ordovician sequences: constraints on the timing of bioevents and environmental changes associated with mass extinction and glaciation. Geol. Soc. Am. Bull. 115, 89–104. doi:10.1130/0016-7606(2003)115<0089:HRSISO>2.0.CO;2
Cai, G., Gu, Y., Jiang, Y., and Wang, Z. (2023). Pore structure and fluid evaluation of deep organic-rich marine shale: a case study from Wufeng–Longmaxi Formation of Southern Sichuan Basin. Appl. Sci. 13, 7827. doi:10.3390/app13137827
Cai, S. Q., Hu, Y. M., Zhao, B. M., Ngia, N., Liu, A., Liao, R. Q., et al. (2022). Source of silica and its implications for organic matter enrichment in the Upper Ordovician-Lower Silurian black shale in western Hubei Province, China: insights from geochemical and petrological analysis. Petroleum Sci. 19 (1), 74–90. doi:10.1016/j.petsci.2021.10.012
Chen, L., Jiang, Z., Liu, K., Tan, J., Gao, F., and Wang, P. (2017a). Pore structure characterization for organic-rich Lower Silurian shale in the Upper Yangtze Platform, South China: a possible mechanism for pore development. J. Nat. Gas Sci. Eng. 46 (2017), 1–15. doi:10.1016/j.jngse.2017.07.009
Chen, L., Jiang, Z., Liu, K., Wang, P., Liu, Y., Bi, H., et al. (2017b). Relationship between pore characteristics and occurrence state of shale gas: a case study of Lower Silurian Longmaxi shale in the Upper Yangtze Platform, South China. Interpretation 5 (3), T437–T449. doi:10.1190/INT-2016-0191.1
Chen, X., Fan, J., Wang, W., Wang, H., Nie, H., Shi, X., et al. (2017c). Stage-progressive distribution pattern of the Lungmachi black graptolitic shales from Guizhou to Chongqing,central China. Sci. China Earth Sci. 60, 1133–1146. doi:10.1007/s11430-016-9031-9
Chen, Y., Zhao, J. H., Hu, Q. H., Liu, K. Y., Wu, W., Luo, C., et al. (2023). Origin of carbonate minerals and impacts on reservoir quality of the Wufeng and Longmaxi shale, Sichuan Basin. Petroleum Sci. 20 (6), 3311–3336. doi:10.1016/j.petsci.2023.08.012
Dong, D., Liang, F., Guan, Q., Jiang, Y., Zhou, S., Yu, R., et al. (2022a). Development model and identification evaluation technology of Wufeng-Longmaxi Formation quality shale gas reservoirs in the Sichuan Basin. Nat. Gas. Ind. 42 (8), 96–111. doi:10.3787/j.issn.1000-0976.2022.08.008
Dong, T., Wang, C., Liang, X., Wang, G., and Jiang, S. (2022b). Paleodepositional conditions and organic matter accumulation mechanisms in the upper ordovician-lower silurian wufeng-longmaxi shales, middle Yangtze region, South China. Mar. Petroleum Geol. 143 (2022), 105823. doi:10.1016/j.marpetgeo.2022.105823
Dymond, J., Suess, E., and Lyle, M. (1992). Barium in deep-sea sediment: a geochemical proxy for paleoproductivity. Paleoceanography 7 (2), 163–181. doi:10.1029/92PA00181
Fan, C. H., Nie, S., Li, H., Radwan, A. E., Pan, Q. C., Shi, X. C., et al. (2024). Quantitative prediction and spatial analysis of structural fractures in deep shale gas reservoirs within complex structural zones: a case study of the Longmaxi Formation in the Luzhou area, southern Sichuan Basin, China. J. Asian Earth Sci. 263 (2024), 106025. doi:10.1016/j.jseaes.2024.106025
Floyd, P. A., and Leveridge, B. E. (1987). Tectonic environment of the Devonian Gramscatho basin, south Cornwall: framework mode and geochemical evidence from turbiditic sandstones. J. Geol. Soc. 144 (4), 531–542. doi:10.1144/gsjgs.144.4.0531
Fu, Y., Jiang, Y., Dong, D., Hu, Q., Lei, Z., Peng, H., et al. (2021b). Microscopic pore-fracture configuration and gas-filled mechanism of shale reservoirsin the western Chongqing area,Sichuan Basin,China. Petroleum Explor. Dev. 48 (5), 1063–1076. doi:10.1016/s1876-3804(21)60091-5
Fu, Y., Jiang, Y., Hu, Q., Luo, T., Li, Y., Lei, Z., et al. (2021a). Fracturing flowback fluids from shale gas wells in western chongqing: geochemical analyses and relevance for exploration and development. J. Nat. Gas Sci. Eng. 88 (2021), 103821. doi:10.1016/j.jngse.2021.103821
Fu, Y., Jiang, Y., Wang, Z., Hu, Q., Xie, J., Ni, G., et al. (2019). Non-connected pores of the Longmaxi shale in southern Sichuan Basin of China. Mar. Petroleum Geol. 110 (2019), 420–433. doi:10.1016/j.marpetgeo.2019.07.014
Guo, X., Qin, Z., Yang, R., Dong, T., He, S., Hao, F., et al. (2019). Comparison of pore systems of clay-rich and silica-rich gas shales in the lower Silurian Longmaxi formation from the Jiaoshiba area in the eastern Sichuan Basin, China. Mar. Petroleum Geol. 101 (2019), 265–280. doi:10.1016/j.marpetgeo.2018.11.038
Huang, Z., Li, Z., Shi, W., Yang, X., Wang, X., and Young, S. (2023). Differential sedimentary mechanisms of upper ordovician-lower silurian shale in southern Sichuan Basin, China. Mar. Petroleum Geol. 148 (2023), 106040. doi:10.1016/j.marpetgeo.2022.106040
Jiang, C., Zhang, H., Zhou, Y., Gan, H., Pu, J., Jiang, Y., et al. (2022). Paleogeomorphic characteristics of Wufeng-Longmaxi formation andits influence on development of high-quality shale in Dazu area,Western Chongqing. J. Central South Univ. Sci. Technol. 53 (9), 3628–3640. doi:10.11817/j.issn.1672-7207.2022.09.026
Jiang, Y., Chen, L., Qi, L., Luo, M., Chen, X., Tao, Y., et al. (2018). Characterization of the lower silurian Longmaxi marine shale in changning area in the South Sichuan Basin, China. Geol. J. 53, 1656–1664. doi:10.1002/gj.2983
Jiang, Y., Fu, Y., Lei, Z., Gu, Y., Qin, L., and Cao, Z. (2019). Experimental NMR analysis of oil and water imbibition during fracturingin Longmaxi shale,SE Sichuan Basin. J. Jpn. Petroleum Inst. 62 (1), 1–10. doi:10.1627/jpi.62.1
Jiang, Z., Song, Y., Tang, X., Li, Z., Wang, X., Wang, G., et al. (2020). Controlling factors of marine shale gas differential enrichment in southern China. Petroleum Explor. Dev. 47 (3), 661–673. doi:10.1016/S1876-3804(20)60083-0
Jin, C., Liao, Z., and Tang, Y. (2020). Sea-level changes control organic matter accumulation in the Longmaxi shales of southeastern Chongqing, China. China. Mar. Petrol. Geol. 119, 104478. doi:10.1016/j.marpetgeo.2020.104478
Li, H. (2023a). Coordinated development of shale gas benefit exploitation and ecological environmental conservation in China: a mini review. Front. Ecol. Evol. 11, 1232395. doi:10.3389/fevo.2023.1232395
Li, H. (2023b). Deciphering the formation period and geological implications of shale tectonic fractures: a mini review and forward-looking perspectives. Front. Energy Res. 11, 1320366. doi:10.3389/fenrg.2023.1320366
Li, Y., Zhou, A., Xie, W., Qiu, X., Dai, Y., Hu, X., et al. (2022). Lithofacies division and main controlling factors of reservoir development in Wufeng Formation-Long1 sub-member shale in the Luzhou region, South Sichuan Basin. Nat. Gas. Ind. 42 (8), 112–123. doi:10.3787/j.issn.1000-0976.2022.08.009
Li, Y. F. (2017). “Geochemical characteristics and organic matter accumulation of Late Ordovician-Early Silurian shale in the Upper Yangtze Platform, and implications for paleoenvironment,” (Gansu, China: Lanzhou University). Master dissertation.
Liu, Z. Y., Chen, D. X., Zhang, J. C., Lü, X. X., Wang, Z. Y., Liao, W. H., et al. (2019). Pyrite morphology as an indicator of paleoredox conditions and shale gas content of the Longmaxi and Wufeng shales in the middle Yangtze area, South China. Minerals 9 (7), 428. doi:10.3390/min9070428
McLennan, S. M. (2001). Relationships between the trace element composition of sedimentary rocks and upper continental crust: trace element composition and upper continental crust. Geochem. Geophys. Geosystems 2, 2000GC000109. doi:10.1029/2000gc000109
Roser, B. P., and Korsch, R. J. (1986). Determination of tectonic setting of sandstone-mudstone suites using SiO2 content and K2O/Na2O ratio. J. Geol. 94, 635–650. doi:10.1086/629071
Song, L. C., Chen, Q., Li, H. J., and Deng, C. Z. (2023). Roller-coaster atmospheric-terrestrial-oceanic-climatic system during Ordovician-Silurian transition: consequences of large igneous provinces. Geosci. Front. 14 (3), 101537. doi:10.1016/j.gsf.2023.101537
Taylor, S. R. (1964). Abundance of chemical elements in the continental crust: a new table. Geochem. Cosmochim. Acta 28 (8), 1273–1285. doi:10.1016/0016-7037(64)90129-2
Taylor, S. R., and McLennan, S. M. (1985). The continental crust: its composition and evolution. Blackwell 312.
Tribovillard, N., Algeo, T. J., Baudin, F., and Riboulleau, A. (2012). Analysis of marine environmental conditions based on molybdenum-uranium covariation-Applications to Mesozoic paleoceanography. Chem. Geol. 324-325, 46–58. doi:10.1016/j.chemgeo.2011.09.009
Tribovillard, N., Algeo, T. J., Lyons, T., and Riboulleau, A. (2006). Trace metals as paleoredox and paleoproductivity proxies: an update. Chem. Geol. 232 (1/2), 12–32. doi:10.1016/j.chemgeo.2006.02.012
Wang, Y., Li, X., Wang, H., Jiang, S., Chen, B., Ma, J., et al. (2019). Developmental characteristics and geological significance of the bentonite in the upper ordovician wufeng-lower silurian Longmaxi formationin eastern Sichuan Basin, SW China. Petroleum Explor. Dev. 46 (4), 687–700. doi:10.1016/s1876-3804(19)60226-0
Wang, Z., Jiang, Y., Fu, Y., Lei, Z., Xu, C., Yuan, J., et al. (2022). 基于核磁共振表征渝西地区五峰组-龙一<sub>1</sub>亚段页岩储层孔隙结构及非均质性. Earth Sci. 47 (2), 490–504. doi:10.3799/dqkx.2021.076
Wedepohl, K. H. (1971). Environmental influences on the chemical composition of shales and clays. Phys. Chem. Earth 8, 307–333. doi:10.1016/0079-1946(71)90020-6
Wei, C., Dong, T., He, Z., He, S., He, Q., Yang, R., et al. (2021). Major, trace-elemental and sedimentological characterization of the upper Ordovician Wufeng-lower Silurian Longmaxi formations, Sichuan Basin, south China: insights into the effect of relative sea-level fluctuations on organic matter accumulation in shales. Mar. Petroleum Geol. 126, 104905. doi:10.1016/j.marpetgeo.2021.104905
Wu, J., Liang, C., Yang, R. C., Hu, Z. Q., Li, W. J., and Xie, J. (2022). The genetic relationship between paleoenvironment, mineral compositions and lithofacies in the Ordovician–Silurian Wufeng–Longmaxi sedimentary succession in the Sichuan Basin, SW China. J. Asian Earth Sci. 236, 105334. doi:10.1016/j.jseaes.2022.105334
Wu, L. Y., Lu, Y. C., Jiang, S., Liu, X., and He, G. (2018). Effects of volcanic activities in ordovician wufeng-silurian Longmaxi period on organic-rich shale in the upper Yangtze area,South China. Petroleum Explor. Dev. 45 (5), 862–872. doi:10.1016/s1876-3804(18)30089-2
Xie, H. R., Liang, C., Wu, J., Cao, Y. C., Han, Y., Liu, Y. D., et al. (2023). Multiple geological events controlling rock types development: a case study of Wufeng-Longmaxi formation in southern Sichuan Basin, China. Geoenergy Sci. Eng. 227, 211826. doi:10.1016/j.geoen.2023.211826
Xie, X. M., Zhu, G. Y., and Wang, Y. (2021). The influence of syngenetic hydrothermal silica fluid on organic matter preservation in lower Cambrian Niutitang Formation, South China. Mar. Petroleum Geol. 129, 105098. doi:10.1016/j.marpetgeo.2021.105098
Zhang, K., Peng, J., Liu, W., Li, B., Xia, Q., Cheng, S., et al. (2020). The role of deep geofluids in the enrichment of sedimentary organic matter: a case study of the late ordovician-early silurian in the upper Yangtze region and early cambrian in the lower Yangtze region, South China. Geofluids 2020, 1–12. doi:10.1155/2020/8868638
Zhang, K., Song, Y., Jia, C., Jiang, Z., Han, F., Wang, P., et al. (2022). Formation mechanism of the sealing capacity of the roof and floor strata of marine organic-rich shale and shale itself, and its influence on the characteristics of shale gas and organic matter pore development. Mar. Petroleum Geol. 140 (2022), 105647. doi:10.1016/j.marpetgeo.2022.105647
Keywords: sedimentary environment, paleoenvironmental condition, ordovician-silurian, organic matter, black shale, Sichuan Basin
Citation: Yang X, Yin H, Yang L, Xu L, Chen J, Liu D, Jiang C and Jiang Z (2024) Evolution of black shale sedimentary environment and its impact on organic matter content and mineral composition: a case study from Wufeng-Longmaxi Formation in Southern and Eastern Sichuan Basin. Front. Environ. Sci. 12:1391445. doi: 10.3389/fenvs.2024.1391445
Received: 25 February 2024; Accepted: 22 April 2024;
Published: 06 May 2024.
Edited by:
Hu Li, Southwest Petroleum University, ChinaReviewed by:
Yifan Gu, Southwest Petroleum University, ChinaLei Gong, Northeast Petroleum University, China
Copyright © 2024 Yang, Yin, Yang, Xu, Chen, Liu, Jiang and Jiang. This is an open-access article distributed under the terms of the Creative Commons Attribution License (CC BY). The use, distribution or reproduction in other forums is permitted, provided the original author(s) and the copyright owner(s) are credited and that the original publication in this journal is cited, in accordance with accepted academic practice. No use, distribution or reproduction is permitted which does not comply with these terms.
*Correspondence: Dongxi Liu, llddxx@163.com