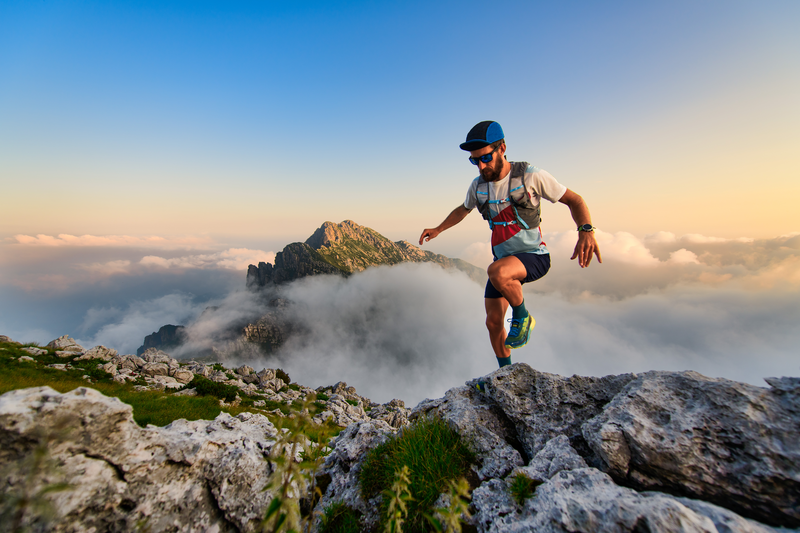
94% of researchers rate our articles as excellent or good
Learn more about the work of our research integrity team to safeguard the quality of each article we publish.
Find out more
ORIGINAL RESEARCH article
Front. Environ. Sci. , 04 April 2024
Sec. Freshwater Science
Volume 12 - 2024 | https://doi.org/10.3389/fenvs.2024.1388262
Under the influence of mining activities, investigating hydrological processes is an important cornerstone of water resources and eco-environment protection. In this study, the spatio-temporal variations of stable isotopic compositions (δD and δ18O) of river water and groundwater in five periods of a hydrological year were analyzed to identify the origin and hydraulic connection of water bodies in the mining district under mining disturbance. The δD and δ18O indicate that the water bodies in the study area are mainly recharged by local precipitation and undergo evaporation. There is a close hydraulic connection between river water, mine water, and groundwater, and the deep confined water is affected by the river water and phreatic water due to the developing water-conducting fractures. The δ18O of phreatic water in each period is depleted in the southwest and enriched in the northeast of the study area. The δ18O of confined water has an obvious spatial variation in the wet season due to the combined effects of opencast working and underground mining, whereas uniform spatial variation is observed in the dry season. Compared with the dry season, mining disturbances have a greater impact on river water and groundwater in the wet season. The difference in spatial variation of δ18O between phreatic and confined water may be attributed to the easier recovery of phreatic water than confined water after mining disturbance. This finding provides important information about the hydrological characteristics of the Pingshuo mining district.
• Spatio-temporal variations in water stable isotopes are obvious in the wet season.
• Close hydraulic connection exists between surface water, mine water, and groundwater.
• Mining disturbance has greater impact on river water and groundwater in the wet season.
As an important part of water resources, river water and groundwater actively participate in the hydrological cycle, which is of great significance to water resources and the ecological environment (Chen et al., 2010; Xiao et al., 2022a; Xiao et al., 2022b; Yang et al., 2021). Large-scale and long-term mining has a destructive influence on the structure of the aquifer system, which will affect groundwater storage and water cycle conditions (Li, 2018). In this case, the natural groundwater dynamic condition and the relationship between recharge, runoff, and drainage of groundwater in the mining district will be changed (Dong et al., 2009; Adhikary and Guo, 2015; Yang et al., 2020). The change in groundwater flow will modify the hydrogeochemical environment; mining activities will lead to serious deterioration of groundwater quality and cause a series of eco-environmental problems (Nordstrom, 2011; Li et al., 2018; 2019; Feng et al., 2019). Therefore, understanding hydrological cycle processes, including water origin, evaporation, and hydraulic connection between water bodies, is significant for the rational exploitation and utilization of water resources and local eco-environment protection, especially in the mining district.
As a component of natural water, stable isotopes of hydrogen (1H and 2H) and oxygen (16O, 17O, and 18O) have a wide range of values due to the large isotopic mass differentiation, which could record the various processes of the hydrological cycle (Qian et al., 2014; Yang et al., 2023). The analysis of hydrogen (δ2H) and oxygen (δ18O) stable isotope ratios in water is an effective tool to solve many complex hydrogeologic issues on a certain spatial and temporal scale, such as the recharge source of water, surface water, groundwater interactions, and the hydraulic connection between different aquifers (El-Sayed et al., 2018; Mokadem et al., 2016; Yang et al., 2021). Previous studies have widely applied the stable isotopes of hydrogen and oxygen to trace the hydrological cycle processes in the mining district, which provide a scientific basis for prevention and cure water design, water bursting prediction, and water resource protection in coal mines (Qu et al., 2018). The stable isotope compositions (δD and δ18O) of water were also used to study deep groundwater circulation and its dynamic characteristics, determine the water source of abandoned mines and mine drainage tunnels, and evaluate the hydrologic connection between mine tunnels and surface water (Gammons et al., 2007; Chen et al., 2008; Walton-Day and Poeter, 2009; Qu et al., 2018). Studying the variation of stable isotopic compositions in water can provide meaningful information with respect to the hydrological cycle in the mining district.
Pingshuo mining district is located in northern Shanxi Province and the eastern part of the Loess Plateau, which is neighboring the supersized coal fields named Shenfu–Dongsheng coalfield, known as the “Black Triangle” of the Loess Plateau. There is the largest strip mine in China, and coal mining has made Pingshuo mining district a fragile eco-environment system with poor stability and strong sensitivity to environmental changes. In addition, water resources in the mining district will be greatly affected, resulting in a greater negative impact on local environmental quality and economic development. Based on the hydrogeological data and groundwater hydrochemical parameters, the groundwater hydrochemical characteristics and their controlling factors in Pingshuo mining district were revealed, which contributes to identifying the formation and evolution of groundwater in this area (Wu et al., 2021; Sun et al., 2022). However, there is still a lack of research about the influence of mining activities on hydrological processes in the Pingshuo mining district, especially surface water and groundwater. Studying the characteristics of surface water and groundwater stable isotopic compositions in the Pingshuo mining district could identify the changes in hydrological processes under the influence of mining activities, which is of great significance for the rational exploitation and utilization of surface water and groundwater resources and eco-environmental protection.
This study is aimed at exploring the influence of mining disturbances on the hydrological cycle processes, taking river water and groundwater, including phreatic water and confined water, as the research objects and comprehensively analyzing their stable isotope compositions to determine the recharge source of river water and groundwater and the hydraulic connection between water bodies. The spatial and temporal variations of stable isotopic values in river water, phreatic water, and confined water were also discussed to indicate the influence of mining activities on hydrological processes associated with river water and groundwater in the Pingshuo mining district.
Pingshuo mining district, located in the east of the Loess Plateau, is part of the northern Ningwu coalfield in northern Shanxi Province (Figure 1). In the study area, the topography is characterized by high elevation in the northwest and low elevation in the southeast, and the loess is widely spread to form a typical landscape of the Loess Plateau with a developed gully (Wu et al., 2021). The study area has a typical continental monsoon climate with a low average annual temperature of 5.4–13.8°C and annual evaporation of approximately 1708.3–2,135.7 mm. According to the meteorological data from 2013 to 2016, the annual average precipitation was approximately 367.68 mm, and the precipitation is mainly concentrated in June, July, and August, accounting for more than 70% of the annual precipitation (Sun et al., 2022). There are multiple large coal mines in the study area, such as Antaibao Strip Mine, Anjialing Strip Mine, and East Strip Mine, as well as several underground coal mines around the strip mines.
In Pingshuo mining district, the strata from old to young are composed of the Ordovician Yeli Formation, Liangjiashan and Majiagou formations, Carboniferous Benxi and Taiyuan formations, Permian Shanxi and Shihezi formations, and Neogene and Quaternary Pleistocene and Holocene (Figure 2). The Taiyuan Formation of Upper Carboniferous is coal-bearing strata, and the water-bearing sandstones are sandwiched between mudstone, sandy mudstone, and coal seam. There are multiple faults in the study area with many anticlines and synclines, and the fractures are often associated with the faults, which provide an important channel for groundwater circulation between different aquifers. The main aquifers from top to bottom are shallow porous aquifers, Shanxi Formation sandstone fissured aquifers, sandstone fissured aquifers between coal measures, and Ordovician limestone fissured aquifers in the Pingshuo mining district. The main recharge source of regional groundwater is atmospheric precipitation, followed by river water and groundwater runoff from adjacent aquifers. The direction of groundwater flow is mainly from the northwest to the southeast of the study area. The gravel-pore water runoff along the valley is from north to south and discharges mainly by evaporation and artificial exploitation for industries and municipalities. The sandstone-fissured water flows from north to south to the piedmont plain and is finally mainly discharged in point form or mining drainage. As the main potential water inrush source in the mining district, Ordovician limestone fissured water (karst groundwater) is controlled by topography and structure with an aquifer thickness of 80–360 m and a water level of 1068–1077 m. Based on pumping and draining tests in the mining area, the specific capacity is 0.0313–2.59 L/(s·m). Karst collapse columns, fracture zones, and fissures are common natural water channels in the study area, and poorly sealed boreholes are common man-made water channels, which play a role in groundwater drainage and form local hydraulic connection (Wu et al., 2021).
Figure 2. Hydrogeological profile of the Pingshuo mining district. The profile is (A,A’) in Figure 1, and the water table belongs to the Ordovician limestone aquifer.
In this study, water samples were collected at 55 sampling sites 5 times in May, August, and October 2020 and July and November 2021, including 16 river water samples, 5 mine water samples, and 34 groundwater samples at a time. The river water samples were collected near the river bank using a hand-held pump attached to a tube, and the samples were collected where the river channel was straight, the water flow was stable, the distance was greater than 30 cm from the river bank, and several centimeters underwater. The groundwater samples include phreatic water and confined water, 19 phreatic water samples were collected from shallow pore water, and 15 confined water samples were taken from Ordovician limestone aquifers. Before collecting groundwater samples, we first let the water pump to pump water continuously for 5 min to eliminate the impact of water accumulation in the water pipes on the test results. The water sample was filtered using a 0.45 um filter into a 100-mL polyethylene bottle, rinsed 2–3 times with the water sample, and then stored at a low temperature of 4°C after collection. The water samples were sent to the laboratory as soon as possible for testing stable isotopes of hydrogen and oxygen (δ18O and δD) using a liquid water isotope analyzer (U-LWIA-915) in the Shanxi Environment Monitoring Center. Before each sample is tested on the machine, the test equipment is calibrated using standard samples (2E: δD is −123.8‰ ± 0.5‰ and δ18O is −16.71‰ ± 0.15‰; 3E: δD is −79.6‰ ± 0.5‰ and δ18O is −11.04‰ ± 0.15‰; and 4E: δD is −49.2‰ ± 0.5‰ and δ18O is −7.81‰ ± 0.15‰). The test for each sample was performed six times, the first two datasets were removed to exclude the influence of the last water sample, and the final isotope data were obtained by averaging the test data of the last four times. Repeated tests were conducted to ensure the accuracy of the data.
The stable isotope concentrations of water were reported in delta δ notation with permil (‰) relative to the standard sample (Vienna Standard Mean Ocean Water, VSMOW); the equation is expressed as follows:
where Rsample and Rstandard represent the heavy and light isotope ratios of water and standard samples, respectively. The analytical precision is less than 0.15‰ for δ18O and 0.5‰ for δD. The statistical results are summarized in Table 1.
Table 1. Statistical values of δD and δ18O in river water, phreatic water, confined water, and mine water in the Pingshuo mining district.
On the basis of stable isotopic data, the relationship between δD and δ18O in river water and groundwater was used to trace the water origin, and δD, δ18O, and d-excess in river water and groundwater in different periods were summarized to infer the temporal variation characteristics. The Kriging interpolation module in ArcGIS is used to draw the contour map of δ18O in the study area to obtain the spatial distribution characteristics.
Stable isotopes of hydrogen and oxygen (δD and δ18O) were extensively used to trace the recharge source of water (Walton-Day and Poeter, 2009; Yang et al., 2018). In this study, the stable isotopic compositions of river water, mine water, and groundwater were analyzed to indicate the water origin and hydraulic connection between different water bodies in the Pingshuo mining district (Figure 3). The stable isotopic compositions of water bodies show a broad range of values, which vary from −105.15‰ to −32.93‰ with an average of −70.94‰ for δD and from −13.79‰ to −4.34‰ with an average of −8.85‰ for δ18O. As can be seen from Figure 3, most water sample points plot close to the global meteoric water line (GMWL: δD = 8δ18O + 10; Craig 1961), indicating that the water in the study area is mainly derived from atmospheric precipitation. Due to the difficulty of precipitation sampling, there is a lack of the local meteoric water line (LMWL) in the study area. The LMWL established by the δD and δ18O of precipitation collected in Ningwu, which is located in the south and approximately 50 km away from the study area, was selected as a reference (δD = 6.88δ18O-1.33; Zhao et al., 2018). The slope and intercept of the LMWL are lower than those of the GMWL, being less than 8 and 10 respectively, which suggest the arid climate and evaporation in the study area (Mook and De Vries, 2000; Yang and Wang, 2020). Most water sample points are close to and fall below the LMWL, reflecting that the water is mainly recharged by local precipitation and undergoes variation after precipitation recharge. The destruction of rock formation structures caused by mining activities could result in surface and underground fissures, which provide a good channel for precipitation infiltration to recharge groundwater. According to the stable isotopes of water, the water samples could be divided into Group 1 and Group 2; the d-excess of water samples in Group 1 is greater than that in Group 2. The stable isotopes in river water and groundwater in Groups 1 and 2 show a tendency toward enrichment, which may be due to evaporation after receiving recharge in the arid climate. It is worth noting that the water samples of Group 2 with small d-excess exhibit obvious “oxygen drift,” which may be due to the mixing of palaeo-groundwater with long-term water–rock interaction (Yang et al., 2020).
Figure 3. δD vs. δ18O of river water, mine water, phreatic water, and confined water in the Pingshuo mining district.
The δD and δ18O of river water span a broad range from −105.15‰ to −32.93‰ and from −12.93‰ to −4.83‰, with average values are −67.56‰ and −8.39‰, respectively. As for phreatic water, the ranges of δD and δ18O are from −81.37‰ to −57.09‰ and from −12.00‰ to −4.34‰, with average values of −70.05‰ and −8.62‰, respectively. Similar to phreatic water, δD and δ18O of confined water vary from −89.31‰ to −65.01‰ and from −13.79‰ to −5.68‰, with average values of −74.54‰ and −9.41‰, respectively. The stable isotopic compositions of river water, mine water, and groundwater are similar (Figure 3), and their distribution locations are also close. It shows that river water, mine water, and groundwater have the same recharge source and a close hydraulic connection. The developed fissure provides an important channel for the hydraulic connection between aquifers. Although the stable isotope compositions are similar in range and represent a relationship among different types of water, river water > phreatic water > confined water, which is related to the formation of different types of water. The stable isotopic compositions of mine water are close to those of confined water, which range from −80.14‰ to −70.70‰ for δD and from −10.80 to −9.33‰ for δ18O, with average values of −75.07‰ and −10.11‰, respectively. In addition, the distribution location of mine water samples is closer to that of confined water samples (Figure 3), indicating that the mine water mainly derives from confined water. The hydraulic connection between river water, phreatic water, and confined water allows them to have similar stable isotopic compositions. The confined water is generally unaffected by evaporation; similar evaporation characteristics show that deep confined water is affected by river water and phreatic water due to the developing water-conducting fractures formed under long-term mining activities.
Although the origin of the different water bodies in the study area is precipitation, the recharge characteristics are different in different seasons, which may lead to variations in the stable isotopes in water. For determining the temporal variation in water stable isotopes, this study analyzed the characteristics of δD, δ18O, and d-excess in water collected in five periods, which cover the wet and dry seasons (Figure 4).
Figure 4. Variation characteristics of δD, δ18O, and d-excess in water in five periods. (A–C) river water and (D–F) groundwater.
It can be found that the temporal variations in δD, δ18O, and d-excess in river water are consistent with those of groundwater, which further indicate the hydraulic connection between different water bodies. δD of river water and groundwater in the five periods are similar and did not change significantly. Different from water δD, δ18O and d-excess in river water and groundwater have obviously changed for the water collected in July 2021. δ18O and d-excess of river water and groundwater were abnormal in July 2021, with an obvious enrichment in δ18O and a decrease in d-excess. The stable isotopic compositions of river water and groundwater in May and October 2020 and November 2021 are similar, which are steady in the dry season. As mentioned earlier, the water bodies in the study are mainly recharged by precipitation, which is concentrated in June, July, and August, accounting for approximately 74% of the annual precipitation. The recharge mainly occurred in the wet season; thus, the river water and groundwater in the dry season are relatively steady, resulting in little variation in stable isotopes in the water. It is worth noting that the δ18O and d-excess of river water and groundwater collected in August 2020 are the same as those taken during the dry season, which indicates that the precipitation recharge is not the main reason for the significant change of stable isotopes in river water and groundwater. In general, there is little change in δD, δ18O, and d-excess of river water and groundwater in a hydrologic year except for the period of July 2021, when the river water and groundwater remained relatively stable. Compared with other periods, mining disturbances had an obvious effect on the river water and groundwater in July 2021.
To determine the variation characteristics of river water and groundwater stable isotopes in different periods, the δD–δ18O relationships were plotted, respectively, in each period (Figure 5). It can be seen clearly from Figure 5A that all the water sample points are located to the right of the LMWL and close to the LMWL, except for the water samples collected in July 2021. The degree of deuterium dynamic fractionation is smaller than that of δ18O, which will lead to the enrichment of δ18O in the resident water and a slope of less than 8 (Yurtsever and Gat, 1981; Wang et al., 2015). The δD and δ18O in river water and groundwater are enriched in five periods, indicating that the river water and groundwater in all periods have been affected by evaporation. In addition, the linear regression lines of confined water (δD and δ18O) in each period were established (Figures 5B–F), and the slopes of the linear regression lines in May, August, and October 2020 and July and November 2021 are 5.12, 4.65, 4.12, 4.19, and 4.50, respectively. The five lines have similar slopes and are significantly less than 8, indicating that confined water has been similarly affected by evaporation. This may be caused by the hydraulic connection between confined water and river water. The intercept value of a meteoric water line indicates the effects of atmospheric recycling of the water (Walton-Day and Poeter, 2009). The intercepts of the linear regression line are −21.95, −25.82, −33.42, −47.28, and −29.73 in May, August, and October 2020 and July and November 2021, respectively. Moreover, the intercept of the linear regression line decreases from May 2020 to July 2021 and then increases from July 2021 to October 2021. In addition, beyond that, the deviation degree of river water and groundwater sample points from the GMWL and LMWL was increasing from May 2020 to July 2021, which decreased from July 2021 to October 2021. The change in the deviation degree and intercepts of the linear regression line indicates the degree of “oxygen drift” in groundwater and river water. Long-term water–rock interactions may lead to the “oxygen drift.” Deep groundwater circulates slowly and has a great potential for 18O exchange with minerals. The mining activities affect the hydraulic connection between aquifers, making the deep groundwater flow into the overlying aquifer along the fracture network caused by mining activities. The results agree with those obtained by hydrochemical analysis (Wu et al., 2021). Mining disturbances will lead to the development of fractures, and the fractures will be gradually closed with the weakening of mining activities (Qu et al., 2022). Strip mines are affected by the cold climate (Bai et al., 2015). The coal mining method in the study area is opencast working and underground mining (Xu, 2015), and the mining progress is slowed down in the cold season, resulting in smaller disturbances to aquifers. The continuous development of fractures increases the recharge of deep groundwater to the overlying aquifer, resulting in gradually obvious “oxygen drift” from May 2020 to July 2021. On the contrary, the recharge of deep groundwater to the overlying aquifer is reduced as the fractures reclogged, resulting in gradually weak “oxygen drift” from July 2021 to October 2021. A previous study showed that the groundwater level and the concentrations of Ca2+, HCO3−, and TDS in groundwater tend to recover due to the reclogging of fractures after mining activities (Qu et al., 2022). No matter which period, the stable isotopic compositions of hydrogen and oxygen in river water and groundwater have the same variation characteristics, indicating that there is a good hydraulic connection between them, which is basically unchanged with the change of time.
Figure 5. Hydrogen and oxygen stable isotope compositions of river water and groundwater in May, August, and October 2020 and July and November 2021.
Hydrogen and oxygen stable isotope ratios in water molecules (δD and δ18O) can be used to trace the water dynamics within a given watershed (Ayenew et al., 2008; Lyon et al., 2009) and provide essential information about the recycling of water and residence times of water bodies using their spatial variability (Gat et al., 1994). In order to further reflect the spatial variation characteristics of groundwater in the mining district, the isotopic spatial variation of phreatic and confined water in five periods was analyzed by taking δ18O as an example (Figure 6). As mentioned above, there is a good hydraulic connection between phreatic and confined water, but there are some differences in the spatial variation between them. For phreatic water, regardless of the dry or wet period, water δ18O in each period has a certain spatial variation characteristic (Figures 6A–E). However, δ18O of confined water has an obvious spatial variation in the wet period and a small and uniform spatial variation in the dry period (Figures 6F–J).
Figure 6. δ18O spatial variations of phreatic water and confined water in May, August, and October 2020 and July and November 2021. (A–E) Phreatic water and (F–J) confined water.
In May and October 2020 and July and November 2021, δ18O in phreatic water exhibited a spatial phenomenon that was depleted in the southwest of the study area and enriched in the northeast of the study area (Figures 6A, C–E). The δ18O spatial variation of phreatic water in August 2020 was slightly different from that in other periods (Figure 6B), which tends to be depleted in the west and enriched in the east. According to the hydrogeological conditions of the study area, the direction of groundwater flow is from northwest to southeast, which is inconsistent with the trend of stable isotope variation in phreatic water. In the absence of external influences, the isotopic compositions of groundwater are generally depleted along the flow direction. Under the mining disturbance, the recharge characteristics may be changed in the study area to alter the spatial distribution of stable isotope compositions in phreatic water. The overlying Ordovician limestone aquifer under the coal seam has the characteristics of large water pressure and water abundance, which makes it prone to inrush into the upper aquifers through water-conducting faults under the influence of mining activities (Yang et al., 2020). In the case of a phreatic aquifer adjacent to a confined aquifer, the water level in the confined aquifer will exceed the elevation of its confining layer, facilitating interflow toward the phreatic aquifer. Multiple faults developed in the southwestern part of the study area allow groundwater with depleted isotopes from the underlying aquifer to enter the phreatic aquifer and deplete stable isotopes of phreatic water. In terms of spatial or temporal variation, mining activities are more intense in summer (the addition of open-pit mines), and river water and groundwater are more affected, which more likely leads to a change in hydraulic connection between them, resulting in obvious changes in river water and groundwater isotopes.
δ18O of confined water in May 2020 was depleted within the scope of the strip mine and relatively enriched around the study area (Figure 6F), and it is possible that old water from the underlying aquifer in the strip mine flowed into the Ordovician confined aquifer due to the mining activity. In August 2020, the δ18O spatial variation of confined water was similar to that of phreatic water, and δ18O was depleted on the west side and enriched on the east side (Figure 6G). The similarity between the spatial variation in stable isotopes of phreatic water and confined water in the same periods can reflect the strength of the hydraulic connection between them. Therefore, there is a very close hydraulic connection between confined water and phreatic water in August 2020. In October 2020 and November 2021, δ18O of confined water was relatively close in the whole region (Figures 6H, J). Unlike other periods, the stable isotopic composition of confined water was depleted in the northwest and enriched in the southeast in July 2021 (Figure 6I), which was gradually enriched along the direction of groundwater flow. According to the above discussion, the spatial variation in stable isotopes of confined water over different periods is obviously different. The possible reason is opencast working and underground mining in open-pit mining areas, which can lead to a decrease in the groundwater level and the enhancement of hydraulic connections between phreatic water and confined water (Zhu et al., 2012). Coal seams are mainly concentrated in the Taiyuan Formation of Carboniferous, underlying karst aquifer of Ordovician, and the water in the Ordovician confined aquifer is more susceptible to mining disturbance than the phreatic water. However, phreatic water exhibits a rapid regeneration rate, whose stable isotopic compositions are easy to recover within a short time. Compared with phreatic water, the regeneration rate of confined water is slower, and the recovery of stable isotopic compositions is slower after mining disturbance.
This study characterized spatial and temporal variations of stable isotopes (δ18O and δ2H) in surface water and groundwater in the Pingshuo mining district. Local precipitation is the main origin of river water and groundwater, which also undergo evaporation after precipitation recharge. River water, mine water, and groundwater have a close hydraulic connection, which is basically unchanged with the change of time. δD and δ18O of river water and groundwater change relatively little over time in a hydrologic year except for the period of July 2021, with the greater impact of mining activity. Intense mining activity facilitated the deep recharge of groundwater into the overlying aquifer through developed fractures, resulting in a significant “oxygen drift” observed in water samples collected in July 2021. δ18O of phreatic water in five different periods show a similar spatial variation characteristic, which is depleted in the southeast and enriched in the northwest. The spatial variation of δ18O in confined water over different periods is obviously different. The stable isotopic compositions of phreatic water with a rapid regeneration rate are easy to recover within a short time compared with slowly renewing confined water. The results may contribute to enhancing our understanding of the influence of mining disturbances on hydrological processes so as to facilitate the rational utilization of water resources.
The original contributions presented in the study are included in the article/Supplementary material; further inquiries can be directed to the corresponding authors
WZ: writing–original draft and writing–review and editing. JZ: conceptualization, investigation, writing–original draft, and writing–review and editing. NY: conceptualization, data curation, investigation, software, writing–original draft, and writing–review and editing. LD: conceptualization, data curation, formal analysis, funding acquisition, investigation, methodology, project administration, resources, software, supervision, validation, visualization, writing–original draft, and writing–review and editing.
The author(s) declare that financial support was received for the research, authorship, and/or publication of this article. The authors would like to thank all the reviewers who participated in the review. This work is partially supported by the National Natural Science Foundation of China (the Yellow River Water Science Research Joint Fund U2243234), the National Natural Science Foundation of China ( 51939006), the National Key Research and Development Plan Project of China (2021YFC3201200 and 2018YFC0406400), the Major Science and Technology Projects of Inner Mongolia Autonomous Region (2020ZD0009 and 2022EEDSKJXM005), the Inner Mongolia Autonomous Region Science and Technology Leading Talent Team (2022LJRC0007) and the Inner Mongolia Agricultural University Basic Research Business Expenses Project (BR221204 and BR221012).
The authors would like to thank all the reviewers who participated in the review.
The authors declare that the research was conducted in the absence of any commercial or financial relationships that could be construed as a potential conflict of interest.
All claims expressed in this article are solely those of the authors and do not necessarily represent those of their affiliated organizations, or those of the publisher, the editors, and the reviewers. Any product that may be evaluated in this article, or claim that may be made by its manufacturer, is not guaranteed or endorsed by the publisher.
Adhikary, D. P., and Guo, H. (2015). Modelling of longwall mining-induced strata permeability change. Rock Mech. Rock Eng. 48, 345–359. doi:10.1007/s00603-014-0551-7
Ayenew, T., Kebede, S., and Alemyahu, T. (2008). Environmental isotopes and hydrochemical study applied to surface water and groundwater interaction in the Awash River basin. Hydrol. Process. 22, 1548–1563. doi:10.1002/hyp.6716
Bai, R. C., Sun, Y. G., Liu, C., Liu, G. W., and Sun, L. (2015). Economic stripping ratio of the open pit coal mining under the condition of frigid climate. MIETAL MINE 44 (5), 34–38. doi:10.3969/j.issn.1001-1250.2015.05.008
Chen, L. W., Gui, H. R., and Yin, X. X. (2008). Composing characteristic of hydrogen and oxygen stable isotopes and tracing of hydrological cycle. J. CHINA COAL Soc. 33, 1107–1111. doi:10.3321/j.issn:0253-9993.2008.10.006
Chen, Z. Y., Qi, J. X., Zhang, Z. J., Nan, Y. J., Ma, J. Z., Wang, J. Z., et al. (2010). Application of isotope hydrogeological methods in typical basins in northern China. Beijing: Science Press.
Dong, D. L., Wang, H. Z., Wu, C. X., Chen, S. K., Lv, Z. Q., and Li, Y. H. (2009). Seepage characters of roof aquifers in faults and sliding structure and risk analysis of water inrush. Chin. J. Rock Mech. Eng. 28, 373–379. doi:10.3321/j.issn:1000-6915.2009.02.022
El-Sayed, S. A., Morsy, S. M., and Zakaria, K. M. (2018). Recharge sources and geochemical evolution of groundwater in the Quaternary aquifer at Atfih area, the northeastern Nile Valley, Egypt. J. Afr. Earth Sci. 142, 82–92. doi:10.1016/j.jafrearsci.2018.03.001
Feng, H. B., Dong, S. G., Zhang, T., et al. (2019). Evolution mechanism of a groundwater system in the opencast coalmine area in the typical prairie. Hydrogeology Eng. Geol. 46, 163–172. doi:10.16030/j.cnki.issn.1000-3665.2019.01.22
Gammons, C. H., Poulson, S. R., Pellicori, D. A., Reed, P. J., Roesler, A. J., and Petrescu, E. M. (2007). The hydrogen and oxygen isotopic composition of precipitation, evaporated mine water, and river water in Montana, USA. J. Hydrol. 328, 319–330. doi:10.1016/j.jhydrol.2005.12.005
Gat, J. R., Bowser, C. J., and Kendall, C. (1994). The contribution of evaporation from the Great Lakes to the continental atmosphere: estimate based on stable isotope data. Geophys. Res. Lett. 21, 557–560. doi:10.1029/94gl00069
Li, P. Y. (2018). Mine water problems and solutions in China. Mine Water Environ. 37, 217–221. doi:10.1007/s10230-018-0543-z
Li, P. Y., Tian, R., and Liu, R. (2019). Solute geochemistry and multivariate analysis of water quality in the guohua phosphorite mine, guizhou Province, China. Expo. Health 11, 81–94. doi:10.1007/s12403-018-0277-y
Li, P. Y., Wu, J. H., Tian, R., He, S., He, X., Xue, C., et al. (2018). Geochemistry, hydraulic connectivity and quality appraisal of multilayered groundwater in the hongdunzi coal mine, northwest China. Mine Water Environ. 37, 222–237. doi:10.1007/s10230-017-0507-8
Lyon, S. W., Desilets, S. L. E., and Troch, P. A. (2009). A tale of two isotopes: differences in hydrograph separation for a runoff event when using delta D versus delta (18)O. Hydrol. Process. 23, 2095–2101. doi:10.1002/hyp.7326
Mokadem, N., Demdoum, A., Hamed, Y., Bouri, S., Hadji, R., Boyce, A., et al. (2016). Hydrogeochemical and stable isotope data of groundwater of a multi-aquifer system: northern Gafsa basin – Central Tunisia. J. Afr. Earth Sci. 114, 174–191. doi:10.1016/j.jafrearsci.2015.11.010
Mook, W. G., and De Vries, J. J. (2000). Environmental isotopes in the hydrological cycle, principles and applications. IHP–V No. 39. Paris: UNESCO.
Nordstrom, D. K. (2011). Hydrogeochemical processes governing the origin, transport and fate of major and trace elements from mine wastes and mineralized rock to surface waters. Appl. Geochem. 26, 1777–1791. doi:10.1016/j.apgeochem.2011.06.002
Qian, H., Wu, J. H., Zhou, Y. H., and Li, P. (2014). Stable oxygen and hydrogen isotopes as indicators of lake water recharge and evaporation in the lakes of the Yinchuan Plain. Hydrol. Process. 28, 3554–3562. doi:10.1002/hyp.9915
Qu, S., Shi, Z. M., Wang, G. C., and Han, J. (2021). Application of multiple approaches to investigate hydraulic connection in multiple aquifers system in coalfield. J. Hydrology 595, 125673. doi:10.1016/j.jhydrol.2020.125673
Qu, S., Wang, G. C., Shi, Z. M., Xu, Q., Guo, Y., Ma, L., et al. (2018). Using stable isotopes (δD, δ18O, δ34S and 87Sr/86Sr) to identify sources of water in abandoned mines in the Fengfeng coal mining district, northern China. Hydrogeology J. 26, 1443–1453. doi:10.1007/s10040-018-1803-5
Qu, S., Wang, G. C., Shi, Z. M., Zhu, Z., Wang, X., and Jin, X. (2022). Impact of mining activities on groundwater level, hydrochemistry, and aquifer parameters in a coalfield's overburden aquifer. Mine Water Environ. 41, 640–653. doi:10.1007/s10230-022-00875-6
Sun, L., Liu, T. X., Duan, L. M., Zhang, W. R., and Zheng, G. F. (2022). Hydrochemical characteristics and fluorine distribution and causes of different water bodies in Pingshuo mining area. Environ. Sci. 43, 5547–5559. doi:10.13227/j.hjkx.202202176
Walton-Day, K., and Poeter, E. (2009). Investigating hydraulic connections and the origin of water in a mine tunnel using stable isotopes and hydrographs. Appl. Geochem. 24, 2266–2282. doi:10.1016/j.apgeochem.2009.09.015
Wang, X. Y., Li, Z. Q., Tayier, R. Z. H., and Wang, S. (2015). Characteristics of atmospheric precipitation isotopes and isotopic evidence for the moisture origin in Yushugou River basin, Eastern Tianshan Mountains, China. Quat. Int. 380-381, 106–115. doi:10.1016/j.quaint.2014.12.023
Wu, X. L., Zhang, Y., Sun, Y. Y., and Wu, J. C. (2021). Hydrochemical characteristics and formation mechanism of groundwater in Pingshuo mining district. J. NANJING Univ. Nat. Sci. 57, 417–425. doi:10.13232/j.cnki.jnju.2021.03.009
Xiao, Y., Liu, K., Hao, Q., Xiao, D., and Zhang, Y. (2022a). Occurrence, controlling factors and health hazards of fluoride-enriched groundwater in the lower flood plain of Yellow River, northern China. Expo. Health 14, 345–358. doi:10.1007/s12403-021-00452-2
Xiao, Y., Liu, K., Hao, Q. C., Xiao, D., Zhu, Y., Yin, S., et al. (2022b). Hydrogeochemical insights into the signatures, genesis and sustainable perspective of nitrate enriched groundwater in the piedmont of Hutuo watershed, China. CATENA 212, 106020. doi:10.1016/j.catena.2022.106020
Xu, Z. Y. (2015). A review on combined open–underground mining technology in Pingshuo mining area. COAL Eng. 47, 11–14. doi:10.11799/ce201507004
Yang, N., and Wang, G. C. (2020). Moisture sources and climate evolution during the last 30 kyr in northeastern Tibetan Plateau: insights from groundwater isotopes (2H, 18O, 3H and 14C) and water vapour trajectories modeling. Quat. Sci. Rev. 242, 106426. doi:10.1016/j.quascirev.2020.106426
Yang, N., Wang, G. C., Liao, F., Dang, X., and Gu, X. (2023). Insights into moisture sources and evolution from groundwater isotopes (2H, 18O, and 14C) in northeastern qaidam basin, northeast Tibetan plateau, China. Sci. Total Environ. 864 (D18), 160981. doi:10.1016/j.scitotenv.2022.160981
Yang, N., Wang, G. C., Shi, Z. M., Zhao, D., Jiang, W., Guo, L., et al. (2018). Application of multiple approaches to investigate the hydrochemistry evolution of groundwater in an arid region: nomhon, northwestern China. Northwest. China. Water 10, 1667. doi:10.3390/w10111667
Yang, N., Zhou, P. P., Wang, G. C., Zhang, B., Shi, Z., Liao, F., et al. (2021). Hydrochemical and isotopic interpretation of interactions between surface water and groundwater in Delingha, Northwest China. J. Hydrology 598, 126243. doi:10.1016/j.jhydrol.2021.126243
Yang, Z. Y., Huang, P. H., and Ding, F. F. (2020). Groundwater hydrogeochemical mechanisms and the connectivity of multilayer aquifers in a coal mining region. Mine Water Environ. 39, 808–822. doi:10.1007/s10230-020-00716-4
Yurtsever, Y., and Gat, J. R. (1981). “Atmospheric waters,”. IAEA Tech Rept. Ser. 210 in Stable isotope hydrology: deuterium and oxygen-18 in the water cycle. Editors J. R. Gat, and R. Gonfiantini (Vienna, Austria: IAEA), 103–142.
Zhao, P. P., Tan, L. C., Zhang, P., Wang, S., Cui, B., Li, D., et al. (2018). Stable isotopic characteristics and influencing factors in precipitation in the monsoon marginal region of northern China. Atmosphere 9, 97. doi:10.3390/atmos9030097
Keywords: δD and δ18O, river water and groundwater, spatio-temporal variation, hydrological processes, mining disturbance
Citation: Zhang W, Zhang J, Yang N and Duan L (2024) Influence of mining activities on hydrological processes in the mining district, Loess Plateau: insights from spatio-temporal variations of δD and δ18O. Front. Environ. Sci. 12:1388262. doi: 10.3389/fenvs.2024.1388262
Received: 20 February 2024; Accepted: 15 March 2024;
Published: 04 April 2024.
Edited by:
Peiyue Li, Chang’an University, ChinaReviewed by:
Yuanzheng Zhai, Beijing Normal University, ChinaCopyright © 2024 Zhang, Zhang, Yang and Duan. This is an open-access article distributed under the terms of the Creative Commons Attribution License (CC BY). The use, distribution or reproduction in other forums is permitted, provided the original author(s) and the copyright owner(s) are credited and that the original publication in this journal is cited, in accordance with accepted academic practice. No use, distribution or reproduction is permitted which does not comply with these terms.
*Correspondence: Nuan Yang, eWFuZ251YW5AeHVzdC5lZHUuY24=; Limin Duan, ZHVhbmxpbWluODIwMTE2QDE2My5jb20=
Disclaimer: All claims expressed in this article are solely those of the authors and do not necessarily represent those of their affiliated organizations, or those of the publisher, the editors and the reviewers. Any product that may be evaluated in this article or claim that may be made by its manufacturer is not guaranteed or endorsed by the publisher.
Research integrity at Frontiers
Learn more about the work of our research integrity team to safeguard the quality of each article we publish.